Effects of Endothelin-1 and nitric oxide levels on myocardial ischemia-reperfusion injury
Highlight box
Key findings
• NO plays a dual role in myocardial ischemia-reperfusion, both beneficial and harmful. The combination of NO and ET-1 can reduce the toxic effect of NO.
What is known and what is new?
• ET and NO may play a role in myocardial ischemia-reperfusion.
• The role of the combination of NO and ET-1 in myocardial ischemia-reperfusion was studied in this study.
What is the implication, and what should change now?
• The PKA pathway may be involved in protective effect of IPC on the mitochondria, but the specific mechanism needs to be further studied.
Introduction
The key to the treatment of acute myocardial infarction (AMI) is early restoration of coronary blood flow and myocardial reperfusion (1). However, even after infarct-related coronary recanalization, 10–30% of the myocardium in the ischemic area is still under perfused due to ischemia-reperfusion injury (IRI) (2). Studies have found that IRI is closely related to the active system of nitric oxide (NO) and its main regulator, nitric oxide synthase (NOS) (3-5). NO plays an important role in IRI by regulating blood pressure, dilating blood vessels, inhibiting platelet aggregation, inhibiting leukocyte adhesion and mediating cytotoxic effects (6).
AMI is a phenomenon of myocardial necrosis caused by coronary ischemia and hypoxia (7). At present, percutaneous coronary intervention (PCI) is the standard treatment, and Thrombolysis In Myocardial Infarction (TIMI) grade 3 blood flow can be restored in epicardial coronary arteries in most patients after PCI. However, many patients with normal epicardial blood flow will still have the myocardial “no-reflow” (NR) phenomenon, and reperfusion can also damage cardiomyocytes. Ischemic preconditioning (IPC) refers to the phenomenon of ischemic reperfusion increasing the tolerance of tissues and organs to a longer ischemic period after repeated short reperfusions (8,9). Calcium overload, leukocyte infiltration and free radicals can lead to myocardial reperfusion injury. Endothelin-1 (ET-1) is a vasoconstrictor factor originally identified in vascular endothelial cells, but can also be produced in some vascular smooth muscle and muscle cells, with a strong vasoconstrictor effect (9). The density of ET-1 receptors in myocardial cell membranes increases during myocardial ischemia, and the reactivity of the myocardium and coronary arteries to ET increases, leading to the NR phenomenon during reperfusion, which suggests that ET may be involved in the pathogenesis of myocardial IRI. On the other hand, increased ET aggravates ischemic myocardial damage, reduces coronary blood flow, causes leakage of myoglobin and lactate dehydrogenase from myocardial cells, myocardial lipid peroxidation and calcium accumulation, ET also aggravates reperfusion injury, and promotes extension of myocardial infarction (10). Therefore, the ET-1 level can indicate the extent of myocardial IRI (11).
The vascular endothelium maintains homeostasis through interplay of relaxation factors (RF) and contractile factors (CF), among which ET plays an important role (12). When unbalanced, abnormal vasoconstriction and diastolic function and cardiovascular diseases may occur. ET, as the most powerful vasoconstrictor and endogenous pathogenic factor, mainly includes ET-1, ET-2, ET-3 and vasointestinal constrictor peptide (VIC) (13,14). In recent years, the distribution of ET and VIC in the cardiovascular system, physiological function and the relationship to cardiovascular diseases have been extensively studied and shown that NO maintains the ET-1 validation state, prevents platelet and white cells adhesion and aggregation in the vascular endothelium, inhibits vascular smooth muscle proliferation and thus protects the vascular endothelium from damage (15). NO release and dysfunction play an important role in coronary atherosclerosis, myocardial ischemia and myocardial infarction (16). Under pathological conditions, the protective mechanisms of the vascular endothelium are weakened or disappear, the release of ET and NO is unbalanced, the CF dominates or the response of vascular smooth muscle to these factors is changed, which will lead to and aggravate the occurrence of myocardial ischemia, hypertension and other cardiovascular diseases.
Therefore, this study investigated whether L-arginine (LA), the precursor of NO, and ET-1, has synergistic protective effects on myocardial IRI. We present the following article in accordance with the ARRIVE reporting checklist (available at https://atm.amegroups.com/article/view/10.21037/atm-22-4998/rc).
Methods
Experimental groups
The study animals (60 healthy Sprague Dawley rats, 30 male rats and 30 female rats, the age of the rats was 4.52±0.43 months (3–6 months), weight: 30.21±1.47 g (18–40 g); Animal Experimental Center of Tongji University Medical College) were randomly divided into 6 groups of 10 rats. The first 5 groups were the experimental groups: LA10 group, LA100 group, LA10 + ET-1 group, LA100 + ET-1 group, and ET-1 group. The LA10 and LA100 groups were given Krebs-Henseleit (KH) perfusion solution containing 10 and 100 mmol/L LA, respectively, for 30 min before myocardial ischemia, then were reperfused with ET-1 for 90 min. The LA10 + ET-1 and LA100 + ET-1 groups were given KH solution containing ET-1 (1,000 U/L) for 5 min and then continued to be perfused with the respective KH solutions without ET-1 for 25 min. The ET-1 group was given KH perfusion solution containing ET-1 (1,000 U/L) for 5 min at the early stage of myocardial reperfusion without any drug dose before ischemia (17-19).
The 6th group was the control group, and these rats were perfused with KH solution without LA before myocardial ischemia and then were perfused with KH solution without ET-1 for 30 min after cardiac arrest.
A protocol was prepared before the study without registration. Animal experiments were performed under a project license (No. 20210087) granted by institutional ethics board of Jiangxi provincial People’s Hospital, The First Affiliated Hospital of Nanchang Medical College, in compliance with Guide for the Care and Use of Laboratory Animals, 8th edition.
Construction of animal model
The Langendorff perfusion model of isolated rat heart was established and modified. Langendorff retrograde perfusion was performed at 8.3 kPa pressure and all KH solution filled with 95% O2 and 5% CO2 mixture (pH: 7.4, 37 ℃) for 15 min. Cardiac function indicators were recorded (as baseline values), the aortic tube was closed and the aortic root was transferred to 6.0 KPa pressure retrograde perfusion. The surface of the heart was cooled with cold normal saline was refused every 30 min during the period of arrest. Cardiac function indexes were measured and myocardial zymography of the coronary effluent was performed during the period of Langendorff perfusion 30 min after 90 min of cardiac arrest.
Observational indexes
- Heart rate (HR) and left ventricular systolic pressure (LVSP) were recorded by multichannel physiological instrument (CDCLVP111-SP). The maximum rate of change of LVSP [± DP/DTmax (peak first derivative of artery pressure)] was measured on a preischemic basis. The baseline level was the percentage of heart function recovery (%) at 30 min of reperfusion compared with 100%.
- Coronary flow per minute before ischemia and at reperfusion was calculated as the percentage of recovery at reperfusion (%) with a baseline value of 100% before ischemia.
- At 5–10 min of reperfusion, lactic dehydrogenase (LDH) and creatine phosphokinase (CPK) on an automatic biochemical analyzer.
- The NO content in the coronary effluent was determined by nitrate reductase colorimetry.
- Determination of malondialdehyde (MDA) content in myocardial tissue was performed using the kit provided by Nanjing Jiancheng Bioengineering Institute, according to the instructions.
- At the end of the experiment, ultrathin sections of myocardial tissue samples from the same location at the apex were created by the conventional method for electron microscopy.
Sampling and determination of infarct size
At the end of the experiment, 4% Thioflavin-S was infused into the left atrial appendage to stain the reflow area, and 2% Evan’s blue was injected into the left anterior descending artery after ligation to stain the lateral side of the ligated area. Animals were killed with intravenous 15% potassium chloride, the heart was removed and the left ventricle sectioned along the long axis. Tissue blocks of 2×5×10 mm were collected from the normal area, the reflow area and the no-reflow area of the myocardium under fluorescent lamp (the reflow area fluoresced, the no-reflow area did not), and stored in liquid nitrogen for use, and the remaining myocardium was weighed.
Determination of tissue water content
The left ventricle and septum were separated, and their wet weight was determined before placement in a drying oven. After 48 h, the dry weight was weighed (accurate to 0.1 mg). In addition, 0.3 g of myocardial tissue from the normal area, reflow area and non-reflow area were weighed and then placed in a drying oven for 48 h. The dry weight (accurate to 0.01 mg) was measured and the tissue water content was = (wet weight – dry weight)/wet weight × 100%.
Measurement of cardiomyocyte cross-sectional area
Sections stained with hematoxylin-eosin (HE) were observed under high magnification (×10), 50 round or oval cardiomyocytes with nuclei were selected in the field of view, and their transverse diameters and areas were measured, and the mean values were recorded.
Statistical analysis
Data of each group are expressed as mean ± standard deviation. The baseline value of each group before ischemia was the recovery value during reperfusion (LVSP ± DP/DTmax) and LDH/CPK release bar NO content and MDA content were determined by one-way analysis of variance. SPSS26.0 (IBM, Chicago, USA) was used for statistical processing.
Results
Changes in cardiac function and coronary flow
Among the groups there was no significant difference in baseline values before myocardial ischemia or in HR during reperfusion. The recovery value of the left ventricular function index (LVSP ± DP/DTmax) in the LA10 group was higher than in the Control group (P<0.01). The recovery value of LV function index in ET-1 group (LVSP ± DP/DTmax) was also higher than in the Control group (P<0.01), but there was no significant difference between the ET-1 and LA10 groups. There was no significant difference in CF/min between the ET-1 and Control groups. The recovery value of the left ventricular function index and CF value in the LA100 group were significantly lower than in the Control group (P<0.05), whereas they were higher in the LA10 + ET-1 group than in the LA10 and ET-1 groups (Table 1).
Table 1
Group | HR (Times/min) | LVSP (Pa) | CF (mL/min) |
---|---|---|---|
LA10 | 86.8±3.1 | 70.3±3.9 | 88.5±14.1 |
LA100 | 87.3±3.0 | 31.6±4.1 | 41.8±11.7 |
LA10 + ET-1 | 87.6±4.1 | 79.7±3.7 | 99.6±15.3 |
LA100 + ET-1 | 86.6±3.8 | 88.6±3.1 | 110.2±11.1 |
Control | 88.6±3.5 | 51.1±4.4 | 63.2±10.1 |
Data were presented as mean ± standard deviation. HR, heart rate; LVSP, left ventricular systolic pressure; CF, contractile factors; LA, L-arginine; ET-1, endothelin-1.
Release of LDH and CPK
After 5–10 min of reperfusion, the LDH-CPK leakage of both the LA10 and ET-1 groups decreased compared with the Control group, but without statistical difference between the LA10 and ET-1 groups. The LDH-CPK leakage of the LA100 group was significantly increased compared with the Control group. Compared with the LA10 + ET-1 group, the LA100 + ET-1 group showed a significant decrease in LDH-CPK leakage, and was the least among the groups (Table 2).
Table 2
Group | LDH | CPK |
---|---|---|
LA10 | 56±4.1 | 78±6.4 |
LA100 | 150±15.9 | 157±20.8 |
LA10 + ET-1 | 49±3.8 | 69±5.8 |
LA100 + ET-1 | 32±3.5 | 5±4.7 |
Control | 73±8.2 | 106±8.6 |
Data were presented as mean ± standard deviation. LDH, lactic dehydrogenase; CPK, phosphokinase; LA, L-arginine; ET-1, endothelin-1.
Determination of NO in coronary effluent
There was no significant difference in the NO content in coronary effluent between the LA10 group and LA100 group at 5–10 min after reperfusion. The LA10 group was higher than the Control group and the LA100 + ET-1 group was significantly higher than the LA100 group (Table 3).
Table 3
Group | NO (μmol/mL) |
---|---|
LA10 | 69±8.2 |
LA100 | 120±11.3 |
LA10 + ET-1 | 73±6.8 |
LA100 + ET-1 | 162±13.5 |
Control | 36±6.2 |
Data were presented as mean ± standard deviation. NO, nitric oxide; LA, L-arginine; ET-1, endothelin-1.
MDA content in myocardial tissue
Compared with the Control group, the MDA content in the myocardium of the LA100 group was significantly increased (P<0.01), reflecting the obvious aggravation of lipid peroxidation. The MDA content in myocardium was significantly decreased (P<0.01), but there was no statistical difference in MDA content between the LA10 and Control groups (Table 4).
Table 4
Group | MDA (μmol/mg) |
---|---|
LA10 | 2.06±0.15 |
LA100 | 4.5±0.23 |
LA10 + ET-1 | 0.92±0.1 |
LA100 + ET-1 | 0.80±0.08 |
Control | 2.18±0.18 |
Data were presented as mean ± standard deviation. MDA, malondialdehyde; LA, L-arginine; ET-1, endothelin-1.
MRI features of myocardial infarction
MRI was performed with a Siemens Magnetom Avanto 1.5T 32-channel machine (gradient field of 45 mT/m; gradient switching rate of 200 T/m/s). Imaging was triggered by ECG-gated R wave with the phased-front ring. Myocardial perfusion scanning used the turbo fast low angle shot magnetic resonance (FLASH) sequence (Figure 1).
Morphological changes of myocardial tissue under light microscopy
Myocardial cells were arranged neatly, showing no degeneration, interstitial edema or bleeding. In the Control group, the myocardial cells were disordered, and there was obvious local degeneration, interstitial edema and bleeding. The myocardial sarcoplasm showed eosinophilic staining, blurred or nonexistent transverse lines, obvious disorder of muscle fibers and myofilaments, and infiltration of inflammatory cells in the interstitium. Edema and degeneration were reduced compared with the control group. Myocardial cellulose was disordered with local corrugations, some muscle fibers had dissolved and liquefied, and a small number of red blood cells appeared in the myocardial interstitium (Figure 2).
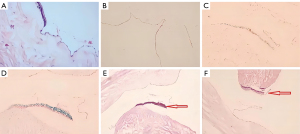
Cell immunofluorescence analysis
The cell immunofluorescence assay showed that most of the myocardial tissues in sham operation group showed negative staining, and a few areas showed brown-blue positive expression. Most of the myocardial tissues showed dark brown-yellow particles, which were mostly expressed in the cytosol or intercellular space (Figure 3).
Discussion
Myocardial IRI refers to that after resuming blood reperfusion, the ischemic myocardium will aggravate its structural damage, cause cell death, expand the scope of myocardial infarction, cause further damage to cardiac function, and affect the prognosis of patients with myocardial infarction. At present, the reason is not completely clear. At present, the main reasons include calcium overload, leukocyte infiltration and free radical damage. NO is the endoderm-derived relaxing factor outer endothelium-derived relaxing factor (EDRF) and it is proved that the N-terminal guanidine deamination of LA is formed in mammalian vascular endothelial cells by NADPH left Ca2+/calmodulin dependent NO synthase NO and L-citrullinated Comply act like messenger transmitters by activating guanylate cyclase to increase intracellular cGMP levels (20). The relationship between the dose of NO and myocardial IRI has attracted more and more attention and currently it is considered that NO release from vascular endothelial cells in the basal state plays an important role in maintaining the cardiovascular system in a constant diastolic active state (21-25).
NO is a strong vasodilator of the coronary arteries and improves microcirculation (26). It is well known that neutrophils aggregate and attach to the coronary endothelium, causing a respiratory burst and producing large amounts of oxygen free radicals, which play an important role in myocardial IRI (27). NO inhibits platelet chemotactic aggregation and adhesion to the vascular endothelium (28). In recent years, studies have shown that the damage to coronary endothelial cells during myocardial IRI is caused by the presence of (I) LA depletion; (II) LA transport system barriers; and (III) superoxide anion O2 inhibiting the activity of NOS or directly inactivating NO, all of which reduce the release of NO synthase, and the reduction in NO is involved in myocardial IRI (29). The sequence of action is: coronary artery contraction, neutrophil granule cell aggregation, concentration, oxygen free radical explosion, coronary artery abnormal response to platelet aggregation, and spasm and narrowing of the occluded coronary artery (30).
Other studies (31-33) have reported that NO is harmful; namely, it increases myocardial IRI through a mechanism related to superoxide anions. In aqueous solution of NO and superoxide anion, the free radical reaction quickly generates peroxynitrite anions, which are a very strong oxidizing toxic substance leading to cell membrane lipid peroxidation and other components of oxidative damage (34).
As soon as the concept of myocardial IPC was proposed, it became a hot topic of research. IPC can reduce myocardial IRI, and myocardial no-reflow. One of the most important mechanisms of IRI is mitochondrial damage. When mitochondria become dysfunctional, their oxidative phosphorylation, which powers the cardiomyocytes, is lost. In addition, mitochondria also play a very important role in the regulation of apoptosis and necrosis. It was found that the degree of mitochondrial swelling was significantly improved in the IPC group, but not after PKA inhibition (35). Similar studies have shown that IPC counteracts the excess reactive oxygen species clusters produced by mitochondria during reperfusion, and NOS slightly increases, which also suggested that the PKA pathway may be involved in protective effect of IPC on the mitochondria, but the specific mechanism needs to be further studied.
Limitations
Apoptosis also plays an important role in myocardial IRI. However, we did not study the effect of ET-1 and NO levels on apoptosis. Moreover, the detection indicators and the research of signaling pathway were relatively few in our study.
Conclusions
IPC can reduce myocardial no-reflow and reperfusion injury, which may be related to a reduction in myocardial edema. In the protective effect of IPC on myocardium, ET-1 may play an important role in reducing myocardial edema and protecting mitochondrial function.
Acknowledgments
Funding: None.
Footnote
Reporting Checklist: The authors have completed the ARRIVE reporting checklist. Available at https://atm.amegroups.com/article/view/10.21037/atm-22-4998/rc
Data Sharing Statement: Available at https://atm.amegroups.com/article/view/10.21037/atm-22-4998/dss
Conflicts of Interest: All authors have completed the ICMJE uniform disclosure form (available at https://atm.amegroups.com/article/view/10.21037/atm-22-4998/coif). The authors have no conflicts of interest to declare.
Ethical Statement: The authors are accountable for all aspects of the work in ensuring that questions related to the accuracy or integrity of any part of the work are appropriately investigated and resolved. Animal experiments were performed under a project license (No. 20210087) granted by institutional ethics board of Jiangxi provincial People’s Hospital, The First Affiliated Hospital of Nanchang Medical College, in compliance with Guide for the Care and Use of Laboratory Animals, 8th edition.
Open Access Statement: This is an Open Access article distributed in accordance with the Creative Commons Attribution-NonCommercial-NoDerivs 4.0 International License (CC BY-NC-ND 4.0), which permits the non-commercial replication and distribution of the article with the strict proviso that no changes or edits are made and the original work is properly cited (including links to both the formal publication through the relevant DOI and the license). See: https://creativecommons.org/licenses/by-nc-nd/4.0/.
References
- Fan Q, Tao R, Zhang H, et al. Dectin-1 Contributes to Myocardial Ischemia/Reperfusion Injury by Regulating Macrophage Polarization and Neutrophil Infiltration. Circulation 2019;139:663-78. [Crossref] [PubMed]
- Li W, Li W, Leng Y, et al. Ferroptosis Is Involved in Diabetes Myocardial Ischemia/Reperfusion Injury Through Endoplasmic Reticulum Stress. DNA Cell Biol 2020;39:210-25. [Crossref] [PubMed]
- Zhang XJ, Liu X, Hu M, et al. Pharmacological inhibition of arachidonate 12-lipoxygenase ameliorates myocardial ischemia-reperfusion injury in multiple species. Cell Metab 2021;33:2059-2075.e10. [Crossref] [PubMed]
- Mokhtari-Zaer A, Marefati N, Atkin SL, et al. The protective role of curcumin in myocardial ischemia-reperfusion injury. J Cell Physiol 2018;234:214-22. [Crossref] [PubMed]
- Zhao WK, Zhou Y, Xu TT, et al. Ferroptosis: Opportunities and Challenges in Myocardial Ischemia-Reperfusion Injury. Oxid Med Cell Longev 2021;2021:9929687. [Crossref] [PubMed]
- Wang K, Li Y, Qiang T, et al. Role of epigenetic regulation in myocardial ischemia/reperfusion injury. Pharmacol Res 2021;170:105743. [Crossref] [PubMed]
- Li T, Tan Y, Ouyang S, et al. Resveratrol protects against myocardial ischemia-reperfusion injury via attenuating ferroptosis. Gene 2022;808:145968. [Crossref] [PubMed]
- Shan X, Lv ZY, Yin MJ, et al. The Protective Effect of Cyanidin-3-Glucoside on Myocardial Ischemia-Reperfusion Injury through Ferroptosis. Oxid Med Cell Longev 2021;2021:8880141. [Crossref] [PubMed]
- Deguchi H, Ikeda M, Ide T, et al. Roxadustat Markedly Reduces Myocardial Ischemia Reperfusion Injury in Mice. Circ J 2020;84:1028-33. [Crossref] [PubMed]
- Tian L, Cao W, Yue R, et al. Pretreatment with Tilianin improves mitochondrial energy metabolism and oxidative stress in rats with myocardial ischemia/reperfusion injury via AMPK/SIRT1/PGC-1 alpha signaling pathway. J Pharmacol Sci 2019;139:352-60. [Crossref] [PubMed]
- Nah J, Zhai P, Huang CY, et al. Upregulation of Rubicon promotes autosis during myocardial ischemia/reperfusion injury. J Clin Invest 2020;130:2978-91. [Crossref] [PubMed]
- Ye B, Chen X, Dai S, et al. Emodin alleviates myocardial ischemia/reperfusion injury by inhibiting gasdermin D-mediated pyroptosis in cardiomyocytes. Drug Des Devel Ther 2019;13:975-90. [Crossref] [PubMed]
- Nie C, Ding X, A R, et al. Hydrogen gas inhalation alleviates myocardial ischemia-reperfusion injury by the inhibition of oxidative stress and NLRP3-mediated pyroptosis in rats. Life Sci 2021;272:119248. [Crossref] [PubMed]
- Xu S, Wu B, Zhong B, et al. Naringenin alleviates myocardial ischemia/reperfusion injury by regulating the nuclear factor-erythroid factor 2-related factor 2 (Nrf2) /System xc-/ glutathione peroxidase 4 (GPX4) axis to inhibit ferroptosis. Bioengineered 2021;12:10924-34. [Crossref] [PubMed]
- Huang ZQ, Xu W, Wu JL, et al. MicroRNA-374a protects against myocardial ischemia-reperfusion injury in mice by targeting the MAPK6 pathway. Life Sci 2019;232:116619. [Crossref] [PubMed]
- Zhang P, Yu Y, Wang P, et al. Role of Hydrogen Sulfide in Myocardial Ischemia-Reperfusion Injury. J Cardiovasc Pharmacol 2021;77:130-41. [Crossref] [PubMed]
- Cao L, Huang C, Wang N, et al. ET-1/NO: a controversial target for myocardial ischemia-reperfusion injury. Cardiology 2014;127:140. [Crossref] [PubMed]
- Venardos KM, Rajapakse NW, Williams D, et al. Cardio-protective effects of combined l-arginine and insulin: Mechanism and therapeutic actions in myocardial ischemia-reperfusion injury. Eur J Pharmacol 2015;769:64-70. [Crossref] [PubMed]
- Venardos KM, Zatta AJ, Marshall T, et al. Reduced L-arginine transport contributes to the pathogenesis of myocardial ischemia-reperfusion injury. J Cell Biochem 2009;108:156-68. [Crossref] [PubMed]
- Di Palo KE, Barone NJ. Hypertension and Heart Failure: Prevention, Targets, and Treatment. Heart Fail Clin 2020;16:99-106. [Crossref] [PubMed]
- Truby LK, Rogers JG. Advanced Heart Failure: Epidemiology, Diagnosis, and Therapeutic Approaches. JACC Heart Fail 2020;8:523-36. [Crossref] [PubMed]
- Borlaug BA. Evaluation and management of heart failure with preserved ejection fraction. Nat Rev Cardiol 2020;17:559-73. [Crossref] [PubMed]
- Crespo-Leiro MG, Metra M, Lund LH, et al. Advanced heart failure: a position statement of the Heart Failure Association of the European Society of Cardiology. Eur J Heart Fail 2018;20:1505-35. [Crossref] [PubMed]
- van der Meer P, Gaggin HK, Dec GW. ACC/AHA Versus ESC Guidelines on Heart Failure: JACC Guideline Comparison. J Am Coll Cardiol 2019;73:2756-68. [Crossref] [PubMed]
- González A, Schelbert EB, Díez J, et al. Myocardial Interstitial Fibrosis in Heart Failure: Biological and Translational Perspectives. J Am Coll Cardiol 2018;71:1696-706. [Crossref] [PubMed]
- Brennan EJ. Chronic heart failure nursing: integrated multidisciplinary care. Br J Nurs 2018;27:681-8. [Crossref] [PubMed]
- Boorsma EM, Ter Maaten JM, Damman K, et al. Congestion in heart failure: a contemporary look at physiology, diagnosis and treatment. Nat Rev Cardiol 2020;17:641-55. [Crossref] [PubMed]
- Skrzypek A, Mostowik M, Szeliga M, et al. Chronic heart failure in the elderly: still a current medical problem. Folia Med Cracov 2018;58:47-56.
- Špinar J, Špinarová L, Vítovec J. Pathophysiology, causes and epidemiology of chronic heart failure. Vnitr Lek 2018;64:834-8.
- Wilcox JE, Fang JC, Margulies KB, et al. Heart Failure With Recovered Left Ventricular Ejection Fraction: JACC Scientific Expert Panel. J Am Coll Cardiol 2020;76:719-34. [Crossref] [PubMed]
- Pagel PS, Tawil JN, Boettcher BT, et al. Heart Failure With Preserved Ejection Fraction: A Comprehensive Review and Update of Diagnosis, Pathophysiology, Treatment, and Perioperative Implications. J Cardiothorac Vasc Anesth 2021;35:1839-59. [Crossref] [PubMed]
- Raj L, Maidman SD, Adhyaru BB. Inpatient management of acute decompensated heart failure. Postgrad Med J 2020;96:33-42. [Crossref] [PubMed]
- Sîrbu O, Floria M, Dascalita P, et al. Anemia in heart failure - from guidelines to controversies and challenges. Anatol J Cardiol 2018;20:52-9. [Crossref] [PubMed]
- Goldstein D, Frishman WH. Diastolic Heart Failure: A Review of Current and Future Treatment Options. Cardiol Rev 2021;29:82-8. [Crossref] [PubMed]
- Heidenreich PA, Bozkurt B, Aguilar D, et al. 2022 AHA/ACC/HFSA Guideline for the Management of Heart Failure: Executive Summary: A Report of the American College of Cardiology/American Heart Association Joint Committee on Clinical Practice Guidelines. Circulation 2022;145:e876-94. [Crossref] [PubMed]
(English Language Editor: J. Gray)