Application of Neurotoxin-Induced Animal Models in the Study of Parkinson’s Disease-Related Depression: Profile and Proposal
- 1Department of Otolaryngology, Head and Neck Surgery, Yantai Yuhuangding Hospital, Qingdao University, Yantai, China
- 2Department of Neurosurgical Intensive Care Unit, Yantai Yuhuangding Hospital, Qingdao University, Yantai, China
- 3Department of Neurology, Yantai Yuhuangding Hospital, Qingdao University, Yantai, China
- 4Department of Central Laboratory, Yantai Yuhuangding Hospital, Qingdao University, Yantai, China
- 5Department of Nephrology, Yantai Yuhuangding Hospital, Qingdao University, Yantai, China
Depression can be a non-motor symptom, a risk factor, and even a co-morbidity of Parkinson’s disease (PD). In either case, depression seriously affects the quality of life of PD patients. Unfortunately, at present, a large number of clinical and basic studies focused on the pathophysiological mechanism of PD and the prevention and treatment of motor symptoms. Although there has been increasing attention to PD-related depression, it is difficult to achieve early detection and early intervention, because the clinical guidelines mostly refer to depression developed after or accompanied by motor impairments. Why is there such a dilemma? This is because there has been no suitable preclinical animal model for studying the relationship between depression and PD, and the assessment of depressive behavior in PD preclinical models is as well a very challenging task since it is not free from the confounding from the motor impairment. As a common method to simulate PD symptoms, neurotoxin-induced PD models have been widely used. Studies have found that neurotoxin-induced PD model animals could exhibit depression-like behaviors, which sometimes manifested earlier than motor impairments. Therefore, there have been attempts to establish the PD-related depression model by neurotoxin induction. However, due to a lack of unified protocol, the reported results were diverse. For the purpose of further promoting the improvement and optimization of the animal models and the study of PD-related depression, we reviewed the establishment and evaluation strategies of the current animal models of PD-related depression based on both the existing literature and our own research experience, and discussed the possible mechanism and interventions, in order to provide a reference for future research in this area.
Introduction
Parkinson’s disease (PD) is the most common degenerative dyskinesia disease at present. Motor retardation is the core diagnostic symptom of PD (Postuma et al., 2015). Pathologically, the progressive loss of dopaminergic neurons in substantia nigra and the formation of Lewy bodies are its main features (Ross and Bu, 2018). It has been more than 200 years since our recognition of PD (Zeng et al., 2018b), but its real etiology and pathogenesis are still unclear. The mainstream hypothesis is that PD is triggered by multiple factors including environmental, genetic, and aging factors (Fleming, 2017). Under the predicament that overemphasis on the diagnosis and treatment of motor symptoms still fails to stop the progression of the disease, people gradually realize that the non-motor symptoms (such as depression and olfactory disorder) that may manifest before the motor symptoms are potential indicators for early detection and early intervention of PD (Hasegawa, 2012). Depression, as one of the indicators, is receiving more and more attention. Similar to the effects of depression on other neurological diseases, it can cause many adverse consequences in PD patients (Menon et al., 2015) such as increasing the difficulty in the diagnosis and treatment of PD, inducing or aggravating PD symptoms, reducing patient compliance to treatment and rehabilitation, significantly increasing the rates of functional disability, recurrence, and mortality, severely reducing the quality of life of patients, and significantly increasing the economic burden on society. Although physicians and neuroscientists have been trying to solve the problem of PD-related depression, unfortunately, the relationship between depression and PD is still unclear. In the past, it was believed that depression is only a psychological stress reaction to the diagnosis of PD, just like people’s pessimistic reaction to other chronic diseases such as diabetes. However, depression actually more commonly affects PD patients than people with other chronic diseases, it shows that the rate of severe depression is twice that seen in other equivalently disabled patients (Remy et al., 2005). Especially, there is a high risk of depression in the first year after the initial diagnosis of PD (Galts et al., 2019). Therefore, it is definitely not as simple as a psychological stress reaction after the diagnosis of the disease. Of course, it is also not likely that depression occurs solely as a secondary reaction to motor deficits in PD because the natural history of depression in PD does not parallel the progression of physical symptoms (Lemke et al., 2004).
With the progress of the study, people also found that depression may be a non-motor symptom in the whole course of PD, which can occur before (in the prodromal phase), synchronous to, and after the manifestation of motor symptoms (Storch et al., 2008). A recent-year study showed that the incidence of PD is higher in people with depression than in those without depression (Schuurman et al., 2002), which suggested that depression may also be a pathogenic factor of PD (Gustafsson et al., 2015). Based on these, we can see that the relationship between depression and PD is very complex. This also explains why there are big differences in the incidence (between 4% and 75%) and prevalence (between 2.7% and 90%) of PD-related depression based on epidemiological surveys (Reijnders et al., 2008). Whether depression is a premonitory non-motor symptom (Lemke et al., 2004), a risk factor (Ishihara and Brayne, 2006), or a co-morbidity of PD (Yapici Eser et al., 2017) is still unanswered at present. However, in either case, depression will lead to catastrophic consequences, as it increases the risk of developing PD and aggravates the conditions of PD patients. Therefore, early detection, diagnosis, and treatment of PD-related depression are particularly important. Especially if depression acts as a risk factor or non-motor symptom in the prodromal period of PD, we could stretch its potential as a biological marker to assist diagnosis, guide intervention, and predict prognosis before the occurrence of motor impairment, in order to maintain the health of patients.
Unfortunately, the reality is not optimistic. At present, a large number of clinical and basic studies are focused on how to better prevent and treat depression secondary to or combined with PD. Therefore, it is difficult to really achieve the early detection, diagnosis, and treatment. Why is there such a dilemma? The lack of systematic and standardized establishment and application of the animal model of PD-related depression may be an important reason. As we all know, the utilization of PD animal models has been very mature, especially the neurotoxin-induced model (Schober, 2004; Zeng et al., 2018a), and more and more studies have found that many non-motor symptoms similar to clinical patients, including depression-like behavior, can be seen in neurotoxin-induced PD animal models (Faivre et al., 2019). Therefore, it is worth thinking whether the neurotoxin-induced PD model can be used directly or after modification in the study of PD-related depression. This article comprehensively reviewed the general depression-like phenotype of PD animal models induced by different neurotoxins, and summarized the possible mechanisms and effective intervention measures, in order to provide new ideas for the study of PD-related depression.
1-Methyl-4-Phenyl-1,2,3,6-Tetrahydropyridine (MPTP) And PD-Related Depression
The induction of Parkinsonian-like symptoms by MPTP was discovered in the 1980s from the misuse of defective chemosynthetic drugs by drug users (Schintu et al., 2012). Since then, with the in-depth study of the role of environmental toxins in the development of PD, MPTP has received widespread attention as an experimental tool to simulate PD, especially in the establishment of PD animal models. Non-human primates and mice are common MPTP-induced PD model animals, and other models using rats, guinea pigs, and miniature pigs have also been reported. However, it is worth noting that rats are not sensitive to MPTP due to the limited ability of transforming MPTP to its active form 1-methyl-4-phenylpyridium (MPP+). Therefore, rats should be induced directly with MPP+for the modeling of PD. In general, MPTP is mostly administered by intraperitoneal injection (Airavaara et al., 2020), and of course, there are other routes including subcutaneous injection, intramuscular injection, intravascular injection, tube feeding, nasal dripping, and brain stereotactic injection. According to the dose and frequency of intraperitoneal injection of MPTP, the MPTP-induced PD models can be divided into three types: acute, subacute, and chronic (Jackson-Lewis and Przedborski, 2007). In the acute model, MPTP was administered at 15–20 mg/kg every 2 h for four times. In the subacute model, the animals were treated with 25 mg/kg MPTP per day for five consecutive days. In the chronic model, also called the “progressive model”, MPTP was given at 25 mg/kg every 3.5 days for 5 weeks, with the addition of Probenecid before each injection to increase the abundance of MPTP in the brain. Each type has its own pros and cons, and the phenotypes varied with the type and age of animals. In either type, most animal studies simulated the motor impairment symptoms of PD, but non-motor symptoms, especially depression-like behavior, were still inconclusive. With the continuous attempts at modification of the MPTP induction method, some studies have developed potential MPTP-induced animal models that could be used for the study of PD-related depression.
General State of PD-Related Depression Model Induced by MPTP
As summarized in Table 1, the administration route of MPTP in PD-related depression models included intraperitoneal injection (Krupina et al., 2000, 2002; Krupina et al., 2006, 2010; Pankova et al., 2004; Khlebnikova et al., 2009a, b, Khlebnikova et al., 2013; Chung et al., 2015; Zhang et al., 2015, 2016; Li et al., 2018; Sampaio et al., 2018; Kiselev et al., 2019; Okano et al., 2019; Tang et al., 2020; Yan et al., 2020), intranasal injection (Castro et al., 2013; Schamne et al., 2018), and stereotactic injection (Santiago et al., 2010; Barbiero et al., 2014; Moretti et al., 2015; Cunha et al., 2017), of which intraperitoneal injection was the main route. However, there were differences in the dose and time of MPTP administration. Notably, the neurotoxic effects of MPTP seem to be mediated by its active oxidative product MPP+. In addition, it was also reported that MPP+ was directly used to induce PD-related depression (Moretti et al., 2015; Cunha et al., 2017). It is worth noting that in the MPTP-induced model of PD-related depression, some only showed depression behavior without causing motor damage (Castro et al., 2013; Moretti et al., 2015; Cunha et al., 2017), which well simulated PD-related depression that occurs as a premonitory symptom to motor symptoms. Depression-like behaviors in MPTP-induced PD models were mostly evaluated by a set of classical animal behavior tests, such as the learned helplessness paradigm (Winter et al., 2007), including the forced swimming test (FWT), and tail suspension test (TST), which mainly manifested a helpless state of animals. Additionally, the splash test (ST) and the sucrose preference test (SPT), which were performed as an indicator of anhedonia (Matheus et al., 2016) also can be considered using. The brain regions studied were mainly the hippocampus, frontal cortex, and striatum. In particular, some studies reported the incidence of depression in the MPTP-induced PD model. For example, Tang et al. injected MPTP (30 mg/kg/day for 7 days) into 6–8-week old male C57BL/6 mice, and found about 60% of mice showed depression-like behavior (Tang et al., 2020), while Zhang et al. (2015) reported depression behavior in 66.7% of 22–26 g C57BL/6 mice after injection of MPTP (20 mg/kg/day for 7 days). Dissimilarly, some studies performed on MPTP models show no depressive symptoms in the SPT (Vucković et al., 2008; Gorton et al., 2010), FST (Santiago et al., 2010), and TST (Gorton et al., 2010). Another issue is that most MPTP-induced PD-related depression models used male rodents, whereas Schamne et al. induced PD model by intranasal administration of MPTP (1 mg/nostril) to female C57BL/6 mice of different ages (some underwent ovariectomy) to investigate the effects of gender and age (which actually reflect different hormone levels) on PD-related depression. It was found that adult female mice treated with MPTP were more likely to develop PD-related depression, and the manifestations were more diverse (such as easy to despair, anhedonia, and apathy; Schamne et al., 2018). Interestingly, anxiety-like behavior and cognitive memory impairment could also be seen in the MPTP-induced PD-related depression model (Cunha et al., 2017; Li et al., 2018). And PD models with the coexistence of pain and depression were also reported (Krupina et al., 2002, 2010).
Possible Mechanism of PD-Related Depression Induced by MPTP
Current research hotspot of the mechanism of PD-related depression in the MPTP-induced PD model lies in whether depressive behavior is accompanied by changes in neurotransmitters, neurotrophic factors, neuroinflammatory factors, oxidative stress, nerve regeneration, and synaptic plasticity. However, there have been few reports of altered signaling pathways, one of which is the PKA-CREB pathway (Zhang et al., 2016). The involvement of the mTOR-regulated VTA-mPFC (Ventral tegmental area-medial prefrontal cortex) neural loop on PD-related depression in the MPTP-induced mouse model has been reported (Tang et al., 2020), which for the first time verified the neural loop hypothesis in PD-related depression using PD animal model. Another research team explored the electrophysiological mechanism of MPTP-induced PD-related depression (Pankova et al., 2004). In addition, abnormal peripheral blood granulocyte count (suggesting changes in anti-inflammation and immune status) might play an important role in the development of PD-related depression (Krupina et al., 2000). Some studies also revealed that the changes in prolyl endopeptidase and dipeptidyl peptidase IV activities were also important influencing factors of PD-related depression (Bastías-Candia et al., 2019; Khlebnikova et al., 2009a).
Possible Interventions in PD-Related Depression Induced by MPTP
At present, therapeutic intervention against depression in MPTP-induced PD models mainly includes transmitter supplementation, anti-inflammation, anti-oxidation, promoting nerve regeneration, etc., and most of the drugs used are “traditional” drugs. Among them, the use of lipid-lowering agents has been mentioned by several studies. A nasal drip of Atorvastatin could improve the MPTP-induced depression behavior by increasing the levels of nerve growth factors in the striatum and hippocampus (Castro et al., 2013). Simvastatin treatment alleviated hippocampal nerve inflammation and to some extent reversed the depression symptoms of PD mice (Yan et al., 2020). In addition to statins, Fenofibrate, a fibrate type of lipid-lowering drug, has been found to have antidepressant effects in MPTP-induced PD Wistar rats (Barbiero et al., 2014). At present, among the widely used antidepressants, only Fluoxetine has been used in the treatment of MPTP-induced PD-related depression (Zhang et al., 2015), and its antidepressant effect was not achieved through the classical pathway, but by affecting the expression of the 5-HT2B receptor on astrocytes. The role of Praxol, an antidepressant drug recommended in the clinical guidelines, on MPTP-induced PD-related depression was only mentioned when compared to the antidepressant effect of Selegiline, a monoamine oxidase B (MAOB) inhibitor (Okano et al., 2019). In addition, it was found that 1-[2-(4-Benzyloxyphenoxy) Ethyl] Imidazole could also improve the motor and depression symptoms of MPTP-induced mice by inhibiting MAOB (Chung et al., 2015). Other drugs, such as Crocin (Tang et al., 2020), dopamine D1 receptor agonist (Zhang et al., 2016), Pioglitazone (Barbiero et al., 2014), 7-Fluoro-1, 3-diphenylisoquinoline (Sampaio et al., 2018), Agmatine (Moretti et al., 2015), N-(5-Hydroxynicotinoyl)-L-glutamic acid (Khlebnikova et al., 2009a; Kiselev et al., 2019), benzyloxycarbonyl-methionyl-2(S)-cyanopyrrolidine (Khlebnikova et al., 2009b; Khlebnikova et al., 2013), and serum albumin (Pankova et al., 2004) also showed different degrees of anti-depressant effects in MPTP-induced PD models.
6-Hydroxydopamine (6-OHDA) and PD-Related Depression
6-OHDA is a neurotoxin formed by the reaction of dopamine quinone (a substance produced by the redox reaction of dopamine during oxidation) in the presence of iron. It was found in the 1960s that 6-OHDA could lead to the degeneration of the substantia nigra-striatum system. In the 1970s, 6-OHDA was successfully used to establish the first animal model of PD with substantia nigra-striatum lesions (Airavaara et al., 2020). After that, there were more studies on 6-OHDA-induced PD models in vivo, and all of them have achieved good results. The animals used were mainly rodents (rats, mice, and guinea pigs), cats, and primates, and the most popular method was the injection of 6-OHDA into the medial forebrain bundle (MFB), substantia nigra, and striatum. As more and more studies have confirmed that there is a window between 6-OHDA-induced substantia nigra degeneration and the appearance of dyskinesia, which emphasizes its value in the preclinical study of PD (Branchi et al., 2008).
General State of PD-Related Depression Induced by 6-OHDA
As shown in Table 2, the administration route of 6-OHDA-induced PD-related depression models was only stereotactic cerebral injection. Besides the three common injection sites MFB, substantia nigra, and striatum, injection in the locus ceruleus (Szot et al., 2016; Sampaio et al., 2019), lateral ventricle (Chenu et al., 2007), and ventral tegmental area of the midbrain (Furlanetti et al., 2016) have also been reported. Injections can be bilateral or unilateral, and the MFB is the most common site for unilateral injection, whereas the striatum is the most common site for bilateral injections. One study compared bilateral striatum injection and unilateral substantia nigra injection (Chen et al., 2014). In terms of the choice of animals, rats were most commonly used and mice accounted for only 8 out of 45 studies. The dosage of 6-OHDA is not uniform at present. The depression phenotypes were evaluated by behavioral tests such as SPT, FST, TST, and ST, but it is worth noting that more than half of the studies (28/45) used only one test to evaluate depression-like behavioral changes, which was mostly FST (16/28). PD-related depression is more common in 6-OHDA-induced animals with motor impairment and is sometimes combined with other non-motor manifestations such as anxiety, cognitive memory impairment, olfactory disorder, and rhythm disorder. Meanwhile, it is encouraging that the study of depression as a prodrome of motor impairment is increasing (Branchi et al., 2008; Matheus et al., 2016; Szot et al., 2016; Marques et al., 2019; Sampaio et al., 2019), in which a small dose injection of 6-OHDA in locus ceruleus is worthy of attention. In addition, it is worth further investigation that some 6-OHDA-induced models developed transient hypokinesia that spontaneously remitted, but with a persistent depressant phenotype. The exploration of the influence of gender on PD-related depression has also been reported in the 6-OHDA-induced model (Sullivan et al., 2014). Interestingly, this study not only looked into the gender factor but also compared the correlations between each cerebral hemisphere and PD-related depression. Other studies on 6-OHDA induction combined life stress (Furlanetti et al., 2015; Dallé et al., 2020) suggested that stress+neurotoxin might be a better composite model for the study of PD-related depression.
Possible Mechanism of PD-Related Depression Induced by 6-OHDA
The exploration of the mechanism of PD-related depression using the 6-OHDA induction model is currently focused on the changes in nerve regeneration, neurotransmitter, oxidative stress, nerve inflammation, neuronutrition, etc. The hotspot brain area of study included the striatum, hippocampus, nucleus accumbens, lateral habenular nucleus, and ventral tegmental area, but there were still few studies on signaling pathways, including the BDNF-TrkB (brain-derived neurotrophic factor-tyrosine kinase receptor B; Tuon et al., 2014; Sun et al., 2016) and Wnt/β-catenin signaling pathways (Singh et al., 2017; Mishra et al., 2019). In the studies of 6-OHDA-induced PD-related depression, there was no report on the involvement of the neural loop. But some studies, suggested that the ipsilateral and contralateral connections between the midbrain dopamine system and the medial prefrontal cortex might play an important role (Petri et al., 2015).
Possible Interventions in PD-Related Depression Induced by 6-OHDA
Among the many studies that discussed the prevention and treatment of PD-related depression in the 6-OHDA-induced models, a drug intervention is the most common method. Dixipramine (Kamińska et al., 2017), Piroxicam (Santiago et al., 2014), 5-HT4R agonists (Guo et al., 2021), Reboxetine (Bonito-Oliva et al., 2014), and Fluvoxamine (Dallé et al., 2020) could improve the depression symptoms in the 6-OHDA-induced models by increasing the level of relevant neurotransmitters. Etazolate (Alzoubi et al., 2018), aqueous extract of albizia leaves (Beppe et al., 2015), 1-(7-imino-3-propyl-2, 3-dihydrothiazolo [4, 5-d] pyrimidin-6(7H)-yl)urea (IDPU; Kumari et al., 2018), hesperidin (Antunes et al., 2014) and methanol extract of cottonrose hibiscus leaves (Foyet et al., 2011) could antagonize 6-OHDA-induced PD-related depression through the antioxidant stress response. Schisanhenol A (Yan et al., 2019) has been reported to improve depression symptoms in the 6-OHDA-induced PD model through the anti-inflammatory pathway. Although the antidepressant effect of Praxol has been verified in the 6-OHDA-induced PD model, its mechanism might involve changes in hippocampal regeneration (Chiu et al., 2015). In addition, MK-801 (Diazolizepine; Singh et al., 2017) and D1 receptor agonist (Mishra et al., 2019) were also suggested to improve depression behavior in 6-OHDA-induced PD model by promoting hippocampal nerve regeneration. Other drugs that could improve depression behaviors in 6-OHDA-induced PD models included Agametine (Souza et al., 2018), progesterone (Casas et al., 2011), granulocyte colony stimulating factor (G-CSF; Prakash et al., 2013), β3-adrenergic receptor agonist (Sampaio et al., 2019), Pioglitazone (Bonato et al., 2018), guanosine (Marques et al., 2019), Ketamine (Vecchia et al., 2018), imipramine (Vecchia et al., 2018), neuropeptide-S (Szot et al., 2016), Rapamycin (Masini et al., 2018) and Apamin (a selective blocker of small conductance calcium-activated potassium channels; Chen et al., 2014). It is alarming that Agomelatine has the risk of aggravating rhythm disorder besides its antidepressant effect (Souza et al., 2018). Excitingly, transcranial direct current stimulation (Feng et al., 2020), deep brain stimulation (Furlanetti et al., 2015, 2016), electroacupuncture (Sun et al., 2016; Yu et al., 2020), and exercise therapy (Goes et al., 2014; Tuon et al., 2014; Hsueh et al., 2018) also demonstrated certain effects on the improvement of depression in 6-OHDA-induced PD models, and most of them had multiple targets.
Rotenone and PD-Related Depression
With the in-depth epidemiological investigation of PD, more and more attention has been attracted to the relationship between environmental toxins, especially pesticides used in agriculture, and the development of PD, and Rotenone is one of them. Rotenone is an insecticidal flavonoid found in many legumes and has been used as fish poison in Peru. It was later found to have high lipophilicity and therefore easy to pass through the blood-brain barrier. Like MPTP, Rotenone leads to PD-like symptoms by inhibiting mitochondrial complex I and causing oxidative stress (Heinz et al., 2017). Also, it mediates the degeneration of dopaminergic neurons in the substantia nigra striatum caused by the accumulation of α-synuclein. Notably, rotenone leads to non-specific toxicity and motor impairment unrelated to nigral cell damage as well (Fleming et al., 2004; Klein et al., 2011). At present, Rotenone is often used to induce PD models in zebrafish and rodents, and the latter is mainly achieved by systemic injection or direct stereotactic injection. High-dose Rotenone effectively induces motor dysfunction but produces a significant lethal effect. Based on this, people have tried to modify its application to achieve a proper balance between efficacy and lethality. It is under this background that Rotenone-induced non-motor phenotypes of PD have also attracted much attention (Fontoura et al., 2017), among which the depression phenotype is one of the research hot spots.
General State of PD-Related Depression Induced by Rotenone
As shown in Table 3 (Santiago et al., 2010; Morais et al., 2012; Noseda et al., 2014; Zaminelli et al., 2014; Shin et al., 2017; Wang et al., 2017; Madiha and Haider, 2019), there are less than 10 reports on Rotenone-induced PD-related depression by now, with most reports using rats as the model animal (6/8), and one report each with mouse and zebrafish. The number of reports of each administration route of Rotenone were: brain stereotactic injection (3/8), intraperitoneal injection (2/8), subcutaneous injection (2/8), and addition in water (similar to environmental exposure; 1/8). And the dosage of Rotenone was different in each report, even with the same administration route. As predicted, the brain areas affected were mostly the hippocampus and striatum, and in one article the dorsal raphe nucleus was affected (Shin et al., 2017). In terms of behavioral evaluation, due to the restriction in the living environment of zebrafish, the depression-like behavioral changes could only be evaluated by limited methods, which was the dark box test in this study (Wang et al., 2017). For the behavioral evaluation of rats and mice induced by rotenone, only SPT and FST, but no TST or ST were reported. In addition, the depression-like behavioral changes mostly occurred after the manifestation of dyskinesia, of course, occasionally accompanied by anxiety, olfactory disorders, and other non-motor symptoms. In addition, some studies have used a compound model of rotenone combined with rapid eye movement sleep deprivation (Noseda et al., 2014).
Possible Mechanism of PD-Related Depression Induced by Rotenone
Like the small number of total reports, there were few literatures on the mechanism of PD-related depression induced by Rotenone, which generally involved oxidative stress or neurotransmitter changes, whereas no specific signaling pathways or neural loops were involved.
Possible Interventions in PD-Related Depression Induced by Rotenone
In the limited number of reports, curcumin (Madiha and Haider, 2019), ibuprofen (Zaminelli et al., 2014), the melatonin MT2 receptor-selective antagonist 4-P-PDOT (Noseda et al., 2014), and exercise therapy (Shin et al., 2017) were found to improve the depression symptoms in the Rotenone-induced PD model.
Lipopolysaccharide (LPS) and PD-Related Depression
LPS is an endotoxin released from the destroyed outer cell wall of Gram-negative bacteria. LPS can activate epithelial cells, mononuclear macrophages, and endothelial cells to synthesize and release many inflammatory mediators and cytokines through a variety of signaling pathways, thus triggering a series of reactions in the body (Morris and Li, 2012). In general, a high concentration of LPS causes an extensive and strong inflammatory response, while an appropriate concentration can initiate a moderate immune response to enhance immune function. With the increasing attention on the role of neuroinflammation in the development of PD (Vivekanantham et al., 2015), the successful establishment of animal PD models induced by LPS (Joers et al., 2017), and the in-depth exploration of the function of LPS in depression, the reports of LPS in the study of PD-related depression are also gradually increasing.
General Status of PD-Related Depression Induced by LPS
As shown in Table 4, there were fewer reports of PD-related depression induced by LPS. In the six studies we retrieved, the PD models were mainly induced by intraperitoneal injection of LPS in mice (4/6), with variance in sex and dose. The remaining two articles reported LPS-induced depression in PD rats, one by intraperitoneal injection and the other by brain stereotactic injection. Interestingly, despite the limited number of articles, several studies attempted low-dose LPS induction that caused only depression but no motor damage. In addition, FST was used in all six studies, either alone or in combination with SPT, SP, or TST. The only other non-motor symptom manifested was anxiety-like behavioral changes in these models.
Possible Mechanism of PD-Related Depression Induced by LPS
As LPS induces a strong inflammatory response, the mechanism of PD-related depression induced by LPS is generally considered to be related to neuroinflammation and neuroimmunity. As a matter of fact, neuronutrition and oxidative stress may also play important roles, especially the changes in the BDNF signaling pathway.
Possible Interventions in PD-Related Depression Induced by LPS
Based on the pro-inflammatory role of LPS, in theory, anti-inflammatory therapy should be the first choice of intervention for LPS-induced PD-related depression. However, among the interventional studies, there was only one literature that suggested improved depression symptoms in the LPS-induced PD model “simply” through anti-inflammatory therapy using not conventional drugs but dopamine receptor agonist Praxol (Lieberknecht et al., 2017). In addition, BCG vaccination could improve LPS-induced PD-related depression through immune regulation, which was a far-fetched evidence of the interventional efficacy of anti-inflammation on PD-related depression (Yang et al., 2016). Although Besarotene was reported to play certain roles against LPS-induced PD-related depression through anti-inflammation, this effect might be mainly related to the restoration of dysregulated CREB/BDNF/ERK signaling pathway (Yuan et al., 2020). More surprisingly, the anti-depressant effect of Exendin-4, a glucagon-like peptide 1 (GLP-1) receptor peptide agonist, does not involve anti-inflammation, but to a large extent related to its effect on the synthesis or metabolism of dopamine (Ventorp et al., 2017). As previously mentioned, oxidative stress is one of the mechanisms of LPS-induced PD-related depression, Resveratrol might effectively intervene the depression symptoms in the LPS-induced PD model through blockade of oxidative stress (Chen et al., 2017).
Others: Paraquat and Lactocin and PD-Related Depression
In addition to the above neurotoxins, paraquat, and lactocin were also reported to induce depression in PD animal models. Since there were only two reports of depression induced by paraquat and one report by lactocin, they are discussed together here.
Paraquat and PD-Related Depression
Paraquat (N,N’-dimethyl-4,4’-bipyridine) is an herbal compound with a similar structure to MPP+, and is used as a herbicide in agriculture. It is specifically toxic to many organs of the human body. It crosses the blood-brain barrier into brain dopaminergic neurons unreliable on DAT but may be transported by neutral amino acid transporters in an age-dependent manner. The mechanism of paraquat-induced cell death is also different from that of MPP+ and rotenone, because it cannot effectively block mitochondrial complex I, but involves the mitochondrial endogenous pathway (Fei et al., 2008). As paraquat can induce the accumulation of α-synuclein and the degeneration of dopamine neurons in substantia nigra, it was also used to establish PD animal models (Bastías-Candia et al., 2019). However, the phenotypes of models constructed by different studies were not consistent, and even contradictive in some cases, which more or less restricted its application. With the accumulating evidences that combined the use of paraquat with other compounds (such as manganic manganese) could cause more severe motor damage, its application in the establishment of PD models has regained new attention. Of course, its effects on PD-related depression and other non-motor disorders are also worthy of study.
Between the two studies of paraquat-induced PD models, one of them successfully induced PD-related depression by combined use of paraquat with mancozeb (Hou et al., 2019). Three-month-old male C57BL/6J mice received intraperitoneal injection of paraquat (10 mg/kg) + mancozeb (30 mg/kg) twice a week for 4 weeks. Depression-like behavior was verified by FST. At the same time, this model also displayed non-motor functional changes such as constipation, learning difficulty, and memory impairment. In addition, this study inversely proved through pharmacological approaches that depression symptoms in the PD model might not be related to locus coeruleus and norepinephrine. In another report, a miniature osmotic pump was implanted in the subscapular area to introduce paraquat at a rate of 0.7 mg/day for 28 days, which successfully induced motor disorders as well as depression and anxiety (Campos et al., 2013).
Lactocin and PD-Related Depression
Lactocin, a proteasome inhibitor, is derived from the metabolites of Streptomyces in soil and widely exists in the environment (Kisselev and Goldberg, 2001). When susceptible people ingest Lactocin through daily contact and diet, it can cause functional deficiency of the ubiquitin-proteasome system by inhibiting the 20S/26S proteasome function, leading to the accumulation of abnormal proteins, and inducing a variety of diseases including PD. This is mainly because it can cause synuclein accumulation similar to the effect of paraquat, selectively kill dopaminergic neurons in the substantia nigra-striatum system, and decrease the level of striatal dopamine, resulting in motor dysfunction (Niu et al., 2009).
Some researchers have successfully constructed PD animal models using Lactocin, which showed typical PD symptoms that progressively aggravated (Harrison et al., 2019). There was no toxin accumulation in the animals and did not cause animal death or paralysis. However, some researchers believed that this model has poor repeatability which might be due to the poor absorption of Lactocin by the brain tissue due to the low solubility of Lactocin before administration or due to the formation of precipitation after injection, which could easily lead to the failure of the experiment. But it is still a method worth exploring if this problem can be solved. Recent studies have optimized the dose of Lactocin or used it in combination with other drugs such as LPS and observed a more significant phenotype of PD (Deneyer et al., 2019). However, the research on non-motor symptoms such as depression in Lactocin-induced models is still rarely reported. Only one study detected signs of depression for the first time in a preclinical experimental model of PD rat induced by intranasal administration of Lactocin (Ekimova et al., 2018).
Reflection and Conclusion
The negative influences of depression in PD patients are more and more recognized. However, because of the complex relationship between depression and PD, the definition and classification of “PD-related depression” are not uniform at present. Accumulating clinical evidences show that depression is not only a risk factor for PD, but also a non-motor symptom, or even a co-morbidity of PD. When regarded as a non-motor symptom of PD, depression can appear synchronous with, before or after the motor impairment. Based on previous reports and our own clinical experience, we summarized the above issues in Figure 1, and put forward some thoughts on PD-related depression. Firstly, all the clinical cases of “depression” and “PD” encountered at present can be collectively referred to as “depression related to PD”. When depression is “as a risk factor” of PD, it can be called “PD associated with depression”, when “as a non-motor symptom” can be called “depression in PD”, and when “as a co-morbidity” can be called “PD with depression.” Secondly, it is unfortunate for individual patients no matter which of the above situations, therefore proper identification and treatment are most important. But from the aspect of community prevention and control, the situations shown in “1” and “2a” in Figure 1 are the key nodes to achieve early detection and early intervention to avoid or delay subsequent dopaminergic neuronal damage, and to achieve proper neuroprotection. Thirdly, in the current clinical prevention and treatment guidelines for PD, treatment of depression is mostly based on the treatment of motor impairments. This is inevitable because as required by the current evidence-based medical principles, a patient with depression but without motor symptoms cannot be treated as PD-related depression in the first place even if the patient later developed PD symptoms. To resolve this dilemma, on one hand, it is necessary to launch large-scale prospective cohort studies in the target population, for example, the “Taicang cohort study” led by Professor Chun-feng Liu of our research team, which is currently carried out in Taicang, China. On the other hand, it is necessary to establish a suitable preclinical animal model for basic research. This is because when the neurobiological nature of PD-related depression is not yet fully understood, the use of animal models is still of great significance to improve our understanding of the etiology, pathophysiology, and molecular mechanism of PD. Besides, the research time for constructing animal models is relatively short. It will undoubtedly accelerate the research of PD-related depression if we can establish a suitable animal model.
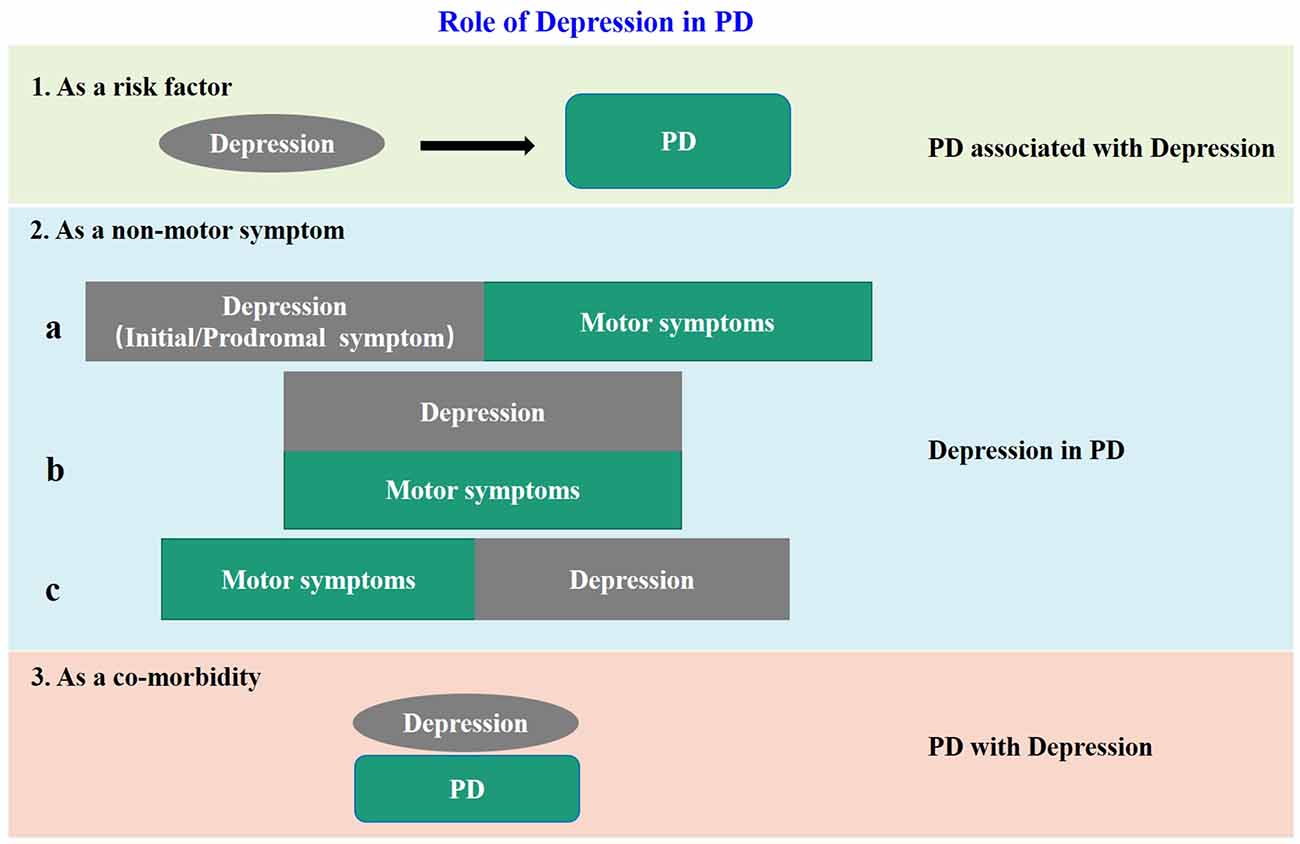
Figure 1. The model diagram of the relationship between depression and PD. In case 1, depression is a risk factor for PD; in case 2, depression is a non-motor symptom of PD, and there are three sub cases, that is, it appears before motor symptoms as a prodromal symptom: (a) occurs simultaneously with motor symptoms; (b) and appears after motor symptoms; and (c) in case 3, it appears as an independent disease and comorbidity of PD.
At present, many animal models of PD have been recognized and widely used, which can be divided into two categories (Blesa et al., 2012): one is by damaging dopaminergic neurons using natural (e.g., Rotenone, LPS, Lactocin) or synthetic neurotoxins (e.g., MPTP, 6-OHDA, paraquat), and the other is by using transgenic animals containing PD-related gene variants. At present, the transgenic PD model is actually limited to transgenic mice with synaptic dopamine depletion, and the behavioral phenotype is usually not obvious. In addition, although there are several gene variants associated with the development of non-motor symptoms in PD patients (e.g., LRRK2, SNCA, Parkin, and VPS35), only a few studies have explored their effects on depression-like behavior in mice (Fontoura et al., 2017). It is worth noting that some studies have constructed a depression-PD co-morbidity animal model using Pitx3 (a risk gene of PD) deficient mice (Kim et al., 2014) or mice treated intraperitoneally with reserpine (Skalisz et al., 2002). Another study found Reserpine could be used to establish the depression-PD association animal model. An ideal PD-related depression animal model has four characteristics: (1) with significant and detectable depress phenotype; (2) with typical and detectable PD-specific motor impairment that aggravates progressively with aging; (3) simulates the clinical pathophysiological evolution of patients with PD-related depression; and (4) reproduces the progressive changes of neurotransmitters in different brain regions of patients with PD-related depression. Regardless of whether the above co-morbidity models meet these characteristics, they still fail the “early detection and early intervention” requirement in situations “1” and “2a” (Figure 1). Although situation “1” has been achieved through initial induction of PD by stress, followed by induction of motor impairment by neurotoxin (Hemmerle et al., 2012), this protocol might actually complicate the situation, because more and more studies found that the PD model induced by neurotoxin alone exhibited depression-like behavior. And as we have previously concluded, after administration of neurotoxin, depression changes can occur before motor damage, which simulates the “2a” situation, and may even partially represent the “1” situation. In summary, under the current condition of lacking spontaneous PD-related depression models, the PD models induced by neurotoxins may be used at this stage or even for a long time in the future. Due to the differences in the phenotype of PD-related depression induced by different neurotoxins, it is a difficult problem for the majority of researchers at present to select the appropriate neurotoxin, method, and dose in the construction of PD-related depression models. Facing this predicament, researchers compared the effects of different neurotoxins on PD-relation depression models. For example, a study used rats matched with age, body weight, and sex and induced by stereotactic injection of MPTP, 6-OHDA, Rotenone, and LPS in bilateral substantia nigra (Santiago et al., 2010). All but LPS induced depression-like behavior, and 6-OHDA had the best phenotype. Another study compared the non-motor symptoms of three PD models (Campos et al., 2013): 6-OHDA (8 or 6 μg, stereotactic injection of bilateral substantia nigra pars compacta), paraquat (micro-osmotic pump implanted in the subscapular area at the rate of 0.7 mg/day for 28 days) and α-synuclein overexpression transgenic model, and found that 6-OHDA and paraquat-induced depression-like behavioral changes in model animals. From the above two comparative studies, it is not difficult to find that the 6-OHDA might have the best effect in inducing PD-related depression. From Tables 1–4 we can also find that there were the largest number of reports of 6-OHDA-induced PD-related depression, and unilateral MFB brain stereotactic injection was the first choice of administration route. However, the specific dosage of 6-OHDA should be determined according to the actual conditions of animals, as there is no unified standard at present, which inevitably leads to a variety of study results. What we can do is to carefully analyze and identify the genuinely useful information.
In addition to the usage of different types of neurotoxins and their dosage, the success rate of model construction also needs to be considered. At present, the incidence of PD–related depression induced by neurotoxins has only been reported in MPTP-induced models. Of course, the conditions of animals such as species, sex, age, bodyweight, etc., also need to be concerned, especially the sex factor itself has an impact on depression and PD in humans (both occur more in women than in men), which have been particularly emphasized in some studies (Sullivan et al., 2014; Schamne et al., 2018). Another factor that requires comprehensive analysis is the behavioral evaluation of model animals. As we have summarized in Tables 1–4; a single behavioral test was used in many neurotoxin-induced PD-related depression models, which would affect the extrapolation of the results to certain extents. For example, FST, TST, and ST all involve “movement”, therefore, it is necessary to rule out the interference of motor impairment. On the other hand, as the SPT test involves the taste function as well as the motor function of the animal, the interference of both factors should be excluded for proper evaluation of depression behaviors. At the same time, different behavioral testing operations are different (specific operations are not described here due to limited space and specific literature can be searched), and their sensitivities are also different. Therefore, we suggest that multiple behavioral tests should be used for cross-validation, and even introduce a rescue experiment for forward and backward validation. Of course, if the model modulates situations “1” and “2a”, the interference of motor factor is neglectable, but it is important to truly rule out motor dysfunction through movement experiments. Further, there is also an important challenge that the results of these depressive behavior tests have not been convincingly coupled to neuropathological changes (Nestler et al., 2002).
Last but not least, the purpose of establishing a PD-related depression animal model is to facilitate the study of the pathogenesis and prevention and treatment strategies of PD-related depression. At present, the research on the mechanism of PD-related depression in neurotoxin-induced animal models is mostly originated from the hypothetical factors of the development of PD-related depression, such as the neurotransmitters, neuronutrition, nerve regeneration, nerve inflammation, and oxidative stress. However, there are very few studies on the involvement of the nerve loop, which is the focus of clinical study, in the PD-related depression model induced by neurotoxin. Moreover, the intrinsic differences of toxins in generic models may also play a role in the mechanisms underlying the depression phenotype. It is worth mentioning that their effects on the neurotransmitter system are different. For example, both MPTP and 6-OHDA can act on the mitochondrial complex in the cell and produce cytotoxicity, resulting in the disorder of neurotransmitters in the dopamine system, but it seems that 6-OHDA can also cause other neurotransmitter changes, such as 5-HT (Zhang et al., 2010). More interestingly, rotenone increased the level of 4-hydroxy-3-methoxyphenylacetic, a norepinephrine metabolite, in the striatum (Thiffault et al., 2000). These tips NA-5HT-DA cross-link in the neurotoxin PD models may be an important breakthrough to explore the mechanism of PD depression. As is known to all, structures define functions, so the structural alterations of depression-related brain areas (Remy et al., 2005), for example, the amygdala and hippocampus, in the neurotoxin PD model should also be concerned. With the continuous improvement of optogenetics and single-cell analysis technology, we believed that there will be a more and more precise exploration in this field in the future. In the meantime, it is encouraging that in the exploration of effective prevention and treatment of PD-related depression using neurotoxin-induced models, deep brain stimulation, electrostimulation, and exercise therapy have emerged besides pharmacological interventions. However, there has been no report using the noninvasive transcranial magnetic stimulation (TMS) that may be effective in the clinical treatment of PD-related depression patients. We look forward to future research that uses TMS in animal models of PD-related depression induced by neurotoxin.
In conclusion, in order to better reveal the relevant changes in the development of PD-related depression and its possible mechanism, and to develop targeted prevention and treatment strategies, it is the key point in current studies to establish a preclinical animal model that is generally in line with the three validity criteria [predictive validity (the ability of the model to predict certain clinical events), face validity (phenomenological similarities between models and clinical conditions), and construct validity (similarities between potential mechanisms of animal behavior and psychological or neurobiological mechanisms under clinical conditions)] (Skalisz et al., 2002) and infinitely close to the four characteristics of ideal PD-related depression models. Neurotoxin-induced PD-related depression models are worthy of further improvement and optimization.
Author Contributions
S-ZW: conception and design. CR and Y-QJ: administrative support. X-YS, J-HW, and Y-QJ: provision of study materials. X-YY, L-NG, and Y-KM: collection and assembly of data. CR and S-ZW: data analysis and interpretation. All authors: manuscript writing and final approval of manuscript. All authors contributed to the article and approved the submitted version.
Funding
This work was supported by the key project of Shandong Provincial Natural Science Foundation (ZR2020KH024), Yantai Science and technology innovation development plan (2022YD004), and the outstanding young talents project in health of Qilu.
Conflict of Interest
The authors declare that the research was conducted in the absence of any commercial or financial relationships that could be construed as a potential conflict of interest.
Publisher’s Note
All claims expressed in this article are solely those of the authors and do not necessarily represent those of their affiliated organizations, or those of the publisher, the editors and the reviewers. Any product that may be evaluated in this article, or claim that may be made by its manufacturer, is not guaranteed or endorsed by the publisher.
References
Airavaara, M., Parkkinen, I., Konovalova, J., Albert, K., Chmielarz, P., and Domanskyi, A. (2020). Back and to the future: from neurotoxin-induced to human Parkinson’s disease models. Curr. Protoc. Neurosci. 91:e88. doi: 10.1002/cpns.88
Alzoubi, K. H., Mokhemer, E., and Abuirmeileh, A. N. (2018). Beneficial effect of etazolate on depression-like behavior and, learning and memory impairment in a model of Parkinson’s disease. Behav. Brain Res. 350, 109–115. doi: 10.1016/j.bbr.2018.05.004
Antunes, M. S., Goes, A. T., Boeira, S. P., Prigol, M., and Jesse, C. R. (2014). Protective effect of hesperidin in a model of Parkinson’s disease induced by 6-hydroxydopamine in aged mice. Nutrition 30, 1415–1422. doi: 10.1016/j.nut.2014.03.024
Barbiero, J. K., Santiago, R. M., Persike, D. S., da Silva Fernandes, M. J., Tonin, F. S., da Cunha, C., et al. (2014). Neuroprotective effects of peroxisome proliferator-activated receptor alpha and gamma agonists in model of parkinsonism induced by intranigral 1-methyl-4-phenyl-1,2,3,6-tetrahyropyridine. Behav. Brain Res. 274, 390–399. doi: 10.1016/j.bbr.2014.08.014
Bastías-Candia, S., Zolezzi, J. M., and Inestrosa, N. C. (2019). Revisiting the paraquat-induced sporadic Parkinson’s disease-like model. Mol. Neurobiol. 56, 1044–1055. doi: 10.1007/s12035-018-1148-z
Beppe, G. J., Dongmo, A. B., Foyet, H. S., Dimo, T., Mihasan, M., and Hritcu, L. (2015). The aqueous extract of Albizia adianthifolia leaves attenuates 6-hydroxydopamine-induced anxiety, depression and oxidative stress in rat amygdala. BMC Complement. Altern. Med. 15:374. doi: 10.1186/s12906-015-0912-0
Blesa, J., Phani, S., Jackson-Lewis, V., and Przedborski, S. (2012). Classic and new animal models of Parkinson’s disease. J. Biomed. Biotechnol. 2012:845618. doi: 10.1155/2012/845618
Bonato, J. M., Bassani, T. B., Milani, H., Vital, M. A. B. F., and de Oliveira, R. M. W. (2018). Pioglitazone reduces mortality, prevents depressive-like behavior and impacts hippocampal neurogenesis in the 6-OHDA model of Parkinson’s disease in rats. Exp. Neurol. 300, 188–200. doi: 10.1016/j.expneurol.2017.11.009
Bonito-Oliva, A., Masini, D., and Fisone, G. (2014). A mouse model of non-motor symptoms in Parkinson’s disease: focus on pharmacological interventions targeting affective dysfunctions. Front. Behav. Neurosci. 8:290. doi: 10.3389/fnbeh.2014.00290
Branchi, I., D’Andrea, I., Armida, M., Cassano, T., Pèzzola, A., Potenza, R. L., et al. (2008). Nonmotor symptoms in Parkinson’s disease: investigating early-phase onset of behavioral dysfunction in the 6-hydroxydopamine-lesioned rat model. J. Neurosci. Res. 86, 2050–2061. doi: 10.1002/jnr.21642
Campos, F. L., Carvalho, M. M., Cristovão, A. C., Je, G., Baltazar, G., Salgado, A. J., et al. (2013). Rodent models of Parkinson’s disease: beyond the motor symptomatology. Front. Behav. Neurosci. 7:175. doi: 10.3389/fnbeh.2013.00175
Casas, S., García, S., Cabrera, R., Nanfaro, F., Escudero, C., and Yunes, R. (2011). Progesterone prevents depression-like behavior in a model of Parkinson’s disease induced by 6-hydroxydopamine in male rats. Pharmacol. Biochem. Behav. 99, 614–618. doi: 10.1016/j.pbb.2011.06.012
Castro, A. A., Wiemes, B. P., Matheus, F. C., Lapa, F. R., Viola, G. G., Santos, A. R., et al. (2013). Atorvastatin improves cognitive, emotional and motor impairments induced by intranasal 1-methyl-4-phenyl-1,2,3,6-tetrahydropyridine (MPTP) administration in rats, an experimental model of Parkinson’s disease. Brain Res. 1513, 103–116. doi: 10.1016/j.brainres.2013.03.029
Chen, L., Deltheil, T., Turle-Lorenzo, N., Liberge, M., Rosier, C., Watabe, I., et al. (2014). SK channel blockade reverses cognitive and motor deficits induced by nigrostriatal dopamine lesions in rats. Int. J. Neuropsychopharmacol. 17, 1295–1306. doi: 10.1017/S1461145714000236
Chen, W. J., Du, J. K., Hu, X., Yu, Q., Li, D. X., Wang, C. N., et al. (2017). Protective effects of resveratrol on mitochondrial function in the hippocampus improves inflammation-induced depressive-like behavior. Physiol. Behav. 182, 54–61. doi: 10.1016/j.physbeh.2017.09.024
Chenu, F., Dailly, E., and Bourin, M. (2007). Effect of antidepressant drugs on 6-OHDA-treated mice in the FST. Eur. Neuropsychopharmacol. 17, 187–193. doi: 10.1016/j.euroneuro.2006.04.006
Chiu, W. H., Depboylu, C., Hermanns, G., Maurer, L., Windolph, A., Oertel, W. H., et al. (2015). Long-term treatment with L-DOPA or pramipexole affects adult neurogenesis and corresponding non-motor behavior in a mouse model of Parkinson’s disease. Neuropharmacology 95, 367–376. doi: 10.1016/j.neuropharm.2015.03.020
Chung, J. Y., Lee, J. W., Ryu, C. H., Min, H. K., Yoon, Y. J., Lim, M. J., et al. (2015). 1–[2-(4-Benzyloxyphenoxy)Ethyl]Imidazole inhibits monoamine oxidase B and protects against neuronal loss and behavioral impairment in rodent models of Parkinson’s disease. J. Neurosci. Res. 93, 1267–1278. doi: 10.1002/jnr.23577
Cunha, M. P., Pazini, F. L., Lieberknecht, V., Budni, J., Oliveira, Á., Rosa, J. M., et al. (2017). MPP+-lesioned mice: an experimental model of motor, emotional, memory/learning and striatal neurochemical dysfunctions. Mol. Neurobiol. 54, 6356–6377. doi: 10.1007/s12035-016-0147-1
Dallé, E., Daniels, W. M. U., and Mabandla, M. V. (2020). Long-term treatment with fluvoxamine decreases nonmotor symptoms and dopamine depletion in a postnatal stress rat model of Parkinson’s disease. Oxid. Med. Cell Longev. 2020:1941480. doi: 10.1155/2020/1941480
Deneyer, L., Albertini, G., Bentea, E., and Massie, A. (2019). Systemic LPS-induced neuroinflammation increases the susceptibility for proteasome inhibition-induced degeneration of the nigrostriatal pathway. Parkinsonism Relat. Disord. 68, 26–32. doi: 10.1016/j.parkreldis.2019.09.025
Ekimova, I. V., Gazizova, A. R., Karpenko, M. N., and Plaksina, D. V. (2018). Priznaki angedonii i destruktivnye izmeneniia v ventral’noĭ oblasti pokryshki srednego mozga v modeli doklinicheskol stadii bolezni Parkinsona v éksperimente [Signs of anhedonia and destructive changes in the ventral tegmental area of the midbrain in the model of the preclinical Parkinson’s disease stage in experiment]. Zh. Nevrol Psikhiatr. Im S S Korsakova 118, 61–67. doi: 10.17116/jnevro201811809161
Faivre, F., Joshi, A., Bezard, E., and Barrot, M. (2019). The hidden side of Parkinson’s disease: studying pain, anxiety and depression in animal models. Neurosci. Biobehav. Rev. 96, 335–352. doi: 10.1016/j.neubiorev.2018.10.004
Fei, Q., McCormack, A. L., Di Monte, D. A., and Ethell, D. W. (2008). Paraquat neurotoxicity is mediated by a Bak-dependent mechanism. J. Biol. Chem. 283, 3357–3364. doi: 10.1074/jbc.M708451200
Feng, X. J., Huang, Y. T., Huang, Y. Z., Kuo, C. W., Peng, C. W., Rotenberg, A., et al. (2020). Early transcranial direct current stimulation treatment exerts neuroprotective effects on 6-OHDA-induced Parkinsonism in rats. Brain Stimul. 13, 655–663. doi: 10.1016/j.brs.2020.02.002
Fleming, S. M. (2017). Mechanisms of gene-environment interactions in Parkinson’s disease. Curr. Environ. Health Rep. 4, 192–199. doi: 10.1007/s40572-017-0143-2
Fleming, S. M., Zhu, C., Fernagut, P. O., Mehta, A., DiCarlo, C. D., Seaman, R. L., et al. (2004). Behavioral and immunohistochemical effects of chronic intravenous and subcutaneous infusions of varying doses of rotenone. Exp. Neurol. 187, 418–429. doi: 10.1016/j.expneurol.2004.01.023
Fontoura, J. L., Baptista, C., Pedroso, F. B., Pochapski, J. A., Miyoshi, E., and Ferro, M. M. (2017). Depression in Parkinson’s disease: the contribution from animal studies. Parkinsons Dis. 2017:9124160. doi: 10.1155/2017/9124160
Foyet, H. S., Hritcu, L., Ciobica, A., Stefan, M., Kamtchouing, P., and Cojocaru, D. (2011). Methanolic extract of Hibiscus asper leaves improves spatial memory deficits in the 6-hydroxydopamine-lesion rodent model of Parkinson’s disease. J. Ethnopharmacol. 133, 773–779. doi: 10.1016/j.jep.2010.11.011
Furlanetti, L. L., Coenen, V. A., Aranda, I. A., and Döbrössy, M. D. (2015). Chronic deep brain stimulation of the medial forebrain bundle reverses depressive-like behavior in a hemiparkinsonian rodent model. Exp. Brain Res. 233, 3073–3085. doi: 10.1007/s00221-015-4375-9
Furlanetti, L. L., Coenen, V. A., and Döbrössy, M. D. (2016). Ventral tegmental area dopaminergic lesion-induced depressive phenotype in the rat is reversed by deep brain stimulation of the medial forebrain bundle. Behav. Brain Res. 299, 132–140. doi: 10.1016/j.bbr.2015.11.036
Galts, C. P. C., Bettio, L. E. B., Jewett, D. C., Yang, C. C., Brocardo, P. S., Rodrigues, A. L. S., et al. (2019). Depression in neurodegenerative diseases: common mechanisms and current treatment options. Neurosci. Biobehav. Rev. 102, 56–84. doi: 10.1016/j.neubiorev.2019.04.002
Goes, A. T., Souza, L. C., Filho, C. B., Del Fabbro, L., De Gomes, M. G., Boeira, S. P., et al. (2014). Neuroprotective effects of swimming training in a mouse model of Parkinson’s disease induced by 6-hydroxydopamine. Neuroscience 256, 61–71. doi: 10.1016/j.neuroscience.2013.09.042
Gorton, L. M., Vuckovic, M. G., Vertelkina, N., Petzinger, G. M., Jakowec, M. W., and Wood, R. I. (2010). Exercise effects on motor and affective behavior and catecholamine neurochemistry in the MPTP-lesioned mouse. Behav. Brain Res. 213, 253–262. doi: 10.1016/j.bbr.2010.05.009
Guo, Y., Zhang, L., Zhang, J., Lv, S. X., Du, C. X., Wang, T., et al. (2021). Activation and blockade of serotonin-4 receptors in the lateral habenula produce antidepressant effects in the hemiparkinsonian rat. Neuropsychobiology 80, 52–63. doi: 10.1159/000508680
Gustafsson, H., Nordström, A., and Nordström, P. (2015). Depression and subsequent risk of Parkinson disease: a nationwide cohort study. Neurology 84, 2422–2429. doi: 10.1212/WNL.0000000000001684
Harrison, I. F., Powell, N. M., and Dexter, D. T. (2019). The histone deacetylase inhibitor nicotinamide exacerbates neurodegeneration in the lactacystin rat model of Parkinson’s disease. J. Neurochem. 148, 136–156. doi: 10.1111/jnc.14599
Hasegawa, K. (2012). [Does early detection of non-motor symptoms facilitate early treatment of Parkinson’s disease?]. Brain Nerve 64, 453–461. doi: 10.11477/mf.1416101175
Heinz, S., Freyberger, A., Lawrenz, B., Schladt, L., Schmuck, G., and Ellinger-Ziegelbauer, H. (2017). Mechanistic investigations of the mitochondrial complex I inhibitor rotenone in the context of pharmacological and safety evaluation. Sci. Rep. 7:45465. doi: 10.1038/srep45465
Hemmerle, A. M., Herman, J. P., and Seroogy, K. B. (2012). Stress, depression and Parkinson’s disease. Exp. Neurol. 233, 79–86. doi: 10.1016/j.expneurol.2011.09.035
Hou, L., Sun, F., Sun, W., Zhang, L., and Wang, Q. (2019). Lesion of the locus coeruleus damages learning and memory performance in paraquat and maneb-induced mouse Parkinson’s disease model. Neuroscience 419, 129–140. doi: 10.1016/j.neuroscience.2019.09.006
Hritcu, L., and Gorgan, L. D. (2014). Intranigral lipopolysaccharide induced anxiety and depression by altered BDNF mRNA expression in rat hippocampus. Prog. Neuropsychopharmacol. Biol. Psychiatry 51, 126–132. doi: 10.1016/j.pnpbp.2014.01.016
Hsueh, S. C., Chen, K. Y., Lai, J. H., Wu, C. C., Yu, Y. W., Luo, Y., et al. (2018). Voluntary physical exercise improves subsequent motor and cognitive impairments in a rat model of Parkinson’s disease. Int. J. Mol. Sci. 19:508. doi: 10.3390/ijms19020508
Ilkiw, J. L., Kmita, L. C., Targa, A. D. S., Noseda, A. C. D., Rodrigues, L. S., Dorieux, F. W. C., et al. (2019). Dopaminergic lesion in the olfactory bulb restores olfaction and induces depressive-like behaviors in a 6-OHDA model of Parkinson’s disease. Mol. Neurobiol. 56, 1082–1095. doi: 10.1007/s12035-018-1134-5
Ishihara, L., and Brayne, C. (2006). A systematic review of depression and mental illness preceding Parkinson’s disease. Acta Neurol. Scand. 113, 211–220. doi: 10.1111/j.1600-0404.2006.00579.x
Jackson-Lewis, V., and Przedborski, S. (2007). Protocol for the MPTP mouse model of Parkinson’s disease. Nat. Protoc. 2, 141–151. doi: 10.1038/nprot.2006.342
Joers, V., Tansey, M. G., Mulas, G., and Carta, A. R. (2017). Microglial phenotypes in Parkinson’s disease and animal models of the disease. Prog. Neurobiol. 155, 57–75. doi: 10.1016/j.pneurobio.2016.04.006
Kamińska, K., Lenda, T., Konieczny, J., Czarnecka, A., and Lorenc-Koci, E. (2017). Depressive-like neurochemical and behavioral markers of Parkinson’s disease after 6-OHDA administered unilaterally to the rat medial forebrain bundle. Pharmacol. Rep. 69, 985–994. doi: 10.1016/j.pharep.2017.05.016
Khlebnikova, N. N., Krupina, N. A., Bogdanova, N. G., Orlova, I. N., and Zolotov, N. N. (2013). Effect of prolyl endopeptidase inhibitor benzyloxycarbonyl-methionyl-2(S)-cyanopyrrolidine on the course of experimental depressive syndrome in rats. Bull. Exp. Biol. Med. 155, 190–193. doi: 10.1007/s10517-013-2109-1
Khlebnikova, N. N., Krupina, N. A., Bogdanova, N. G., Zolotov, N. N., and Kryzhanovskii, G. N. (2009a). Effects of prolylendopeptidase inhibitor benzyloxycarbonyl-methionyl-2(S)-cyanopyrrolidine on experimental depressive syndrome development in rats. Bull. Exp. Biol. Med. 147, 26–30. doi: 10.1007/s10517-009-0458-6
Khlebnikova, N. N., Krupina, N. A., Orlova, I. N., Bogdanova, N. G., Zolotov, N. N., and Kryzhanovskii, G. N. (2009b). Effect of a prolyl endopeptidase inhibitor benzyloxycarbonyl-alanyl-proline on the development of experimental depressive syndrome in rats. Bull. Exp. Biol. Med. 147, 291–295. doi: 10.1007/s10517-009-0494-2
Kim, K. S., Kang, Y. M., Kang, Y., Park, T. S., Park, H. Y., Kim, Y. J., et al. (2014). Pitx3 deficient mice as a genetic animal model of co-morbid depressive disorder and parkinsonism. Brain Res. 1552, 72–81. doi: 10.1016/j.brainres.2014.01.023
Kiselev, A. V., Vedenkin, A. S., Stovbun, I. S., Sergienko, V. I., and Kalinina, T. S. (2019). Calcium salt of N-(5-Hydroxynicotinoyl)-L-glutamic acid weakens depressive-like behavior and parkinsonian syndrome in experiment on rodents. Bull. Exp. Biol. Med. 168, 48–51. doi: 10.1007/s10517-019-04643-5
Kisselev, A. F., and Goldberg, A. L. (2001). Proteasome inhibitors: from research tools to drug candidates. Chem. Biol. 8, 739–758. doi: 10.1016/s1074-5521(01)00056-4
Klein, A., Gidyk, D. C., Shriner, A. M., Colwell, K. L., Tatton, N. A., Tatton, W. G., et al. (2011). Dose-dependent loss of motor function after unilateral medial forebrain bundle rotenone lesion in rats: a cautionary note. Behav. Brain Res. 222, 33–42. doi: 10.1016/j.bbr.2011.03.018
Krupina, N. A., Davydova, T. V., Fomina, V. G., Kryzhanovskii, G. N., and Orlova, I. N. (2000). Count and phagocytic activity of leukocytes in rats with experimental depressive syndrome caused by systemic administration of 1-methyl-4-phenyl-1,2,3,6-tetrahydropyridine. Bull. Exp. Biol. Med. 130, 1041–1044. doi: 10.1007/BF02688173
Krupina, N. A., Khlebnikova, N. N., Orlova, I. N., Grafova, V. N., Smirnova, V. S., Rodina, V. I., et al. (2010). Experimental model of combined pain and depression status in rats. Bull. Exp. Biol. Med. 149, 479–484. doi: 10.1007/s10517-010-0975-3
Krupina, N. A., Orlova, I. N., Khlebnikova, N. N., Grafova, V. N., Smirnova, V. S., Kukushkin, M. L., et al. (2002). Experimental depressive-pain syndrome in rats with initial various anxiety-phobic levels: a behavioral study. Bull. Exp. Biol. Med. 133, 548–552. doi: 10.1023/a:1020217421991
Krupina, N. A., Zolotov, N. N., Bogdanova, N. G., Orlova, I. N., Khlebnikova, N. N., and Kryzhanovskii, G. N. (2006). Activities of prolyl endopeptidase and dipeptidyl peptidase IV in brain structures of rats with dopamine deficiency-dependent MPTP-induced depressive syndrome. Bull. Exp. Biol. Med. 142, 554–556. doi: 10.1007/s10517-006-0416-5
Kumari, N., Agrawal, S., Kumari, R., Sharma, D., and Luthra, P. M. (2018). Neuroprotective effect of IDPU (1-(7-imino-3-propyl-2,3-dihydrothiazolo [4,5-d]pyrimidin-6(7H)-yl)urea) in 6-OHDA induced rodent model of hemiparkinson’s disease. Neurosci. Lett. 675, 74–82. doi: 10.1016/j.neulet.2018.03.040
Kuter, K., Kolasiewicz, W., Gołembiowska, K., Dziubina, A., Schulze, G., Berghauzen, K., et al. (2011). Partial lesion of the dopaminergic innervation of the ventral striatum induces "depressive-like" behavior of rats. Pharmacol. Rep. 63, 1383–1392. doi: 10.1016/s1734-1140(11)70702-2
Lemke, M. R., Fuchs, G., Gemende, I., Herting, B., Oehlwein, C., Reichmann, H., et al. (2004). Depression and Parkinson’s disease. J. Neurol. 251, VI24–VI27. doi: 10.1007/s00415-004-1606-6
Li, Y., Jiao, Q., Du, X., Bi, M., Han, S., Jiao, L., et al. (2018). Investigation of behavioral dysfunctions induced by monoamine depletions in a mouse model of Parkinson’s disease. Front. Cell Neurosci. 12:241. doi: 10.3389/fncel.2018.00241
Lieberknecht, V., Cunha, M. P., Junqueira, S. C., Coelho, I. D., de Souza, L. F., Dos Santos, A. R., et al. (2017). Antidepressant-like effect of pramipexole in an inflammatory model of depression. Behav. Brain Res. 320, 365–373. doi: 10.1016/j.bbr.2016.11.007
Madiha, S., and Haider, S. (2019). Curcumin restores rotenone induced depressive-like symptoms in animal model of neurotoxicity: assessment by social interaction test and sucrose preference test. Metab. Brain Dis. 34, 297–308. doi: 10.1007/s11011-018-0352-x
Marques, N. F., Binder, L. B., Roversi, K., Sampaio, T. B., Constantino, L. C., Prediger, R. D., et al. (2019). Guanosine prevents depressive-like behaviors in rats following bilateral dorsolateral striatum lesion induced by 6-hydroxydopamine. Behav. Brain Res. 372:112014. doi: 10.1016/j.bbr.2019.112014
Masini, D., Bonito-Oliva, A., Bertho, M., and Fisone, G. (2018). Inhibition of mTORC1 signaling reverts cognitive and affective deficits in a mouse model of Parkinson’s disease. Front. Neurol. 9:208. doi: 10.3389/fneur.2018.00208
Matheus, F. C., Rial, D., Real, J. I., Lemos, C., Takahashi, R. N., Bertoglio, L. J., et al. (2016). Temporal dissociation of striatum and prefrontal cortex uncouples anhedonia and defense behaviors relevant to depression in 6-OHDA-lesioned rats. Mol. Neurobiol. 53, 3891–3899. doi: 10.1007/s12035-015-9330-z
Menon, B., Nayar, R., Kumar, S., Cherkil, S., Venkatachalam, A., Surendran, K., et al. (2015). Parkinson’s disease, depression and quality-of-life. Indian J. Psychol. Med. 37, 144–148. doi: 10.4103/0253-7176.155611
Mishra, A., Singh, S., Tiwari, V., Parul, , and Shukla, S. (2019). Dopamine D1 receptor activation improves adult hippocampal neurogenesis and exerts anxiolytic and antidepressant-like effect via activation of Wnt/β-catenin pathways in rat model of Parkinson’s disease. Neurochem. Int. 122, 170–186. doi: 10.1016/j.neuint.2018.11.020
Morais, L. H., Lima, M. M., Martynhak, B. J., Santiago, R., Takahashi, T. T., Ariza, D., et al. (2012). Characterization of motor, depressive-like and neurochemical alterations induced by a short-term rotenone administration. Pharmacol. Rep. 64, 1081–1090. doi: 10.1016/s1734-1140(12)70905-2
Moretti, M., Neis, V. B., Matheus, F. C., Cunha, M. P., Rosa, P. B., Ribeiro, C. M., et al. (2015). Effects of agmatine on depressive-like behavior induced by intracerebroventricular administration of 1-Methyl-4-phenylpyridinium (MPP+). Neurotox. Res. 28, 222–231. doi: 10.1007/s12640-015-9540-1
Morris, M., and Li, L. (2012). Molecular mechanisms and pathological consequences of endotoxin tolerance and priming. Arch. Immunol. Ther. Exp. (Warsz) 60, 13–18. doi: 10.1007/s00005-011-0155-9
Nestler, E. J., Barrot, M., DiLeone, R. J., Eisch, A. J., Gold, S. J., and Monteggia, L. M. (2002). Neurobiology of depression. Neuron 34, 13–25. doi: 10.1016/s0896-6273(02)00653-0
Niu, C., Mei, J., Pan, Q., and Fu, X. (2009). Nigral degeneration with inclusion body formation and behavioral changes in rats after proteasomal inhibition. Stereotact. Funct. Neurosurg. 87, 69–81. doi: 10.1159/000202972
Noseda, A. C., Rodrigues, L. S., Targa, A. D., Aurich, M. F., Vital, M. A., Da Cunha, C., et al. (2014). Putative role of monoamines in the antidepressant-like mechanism induced by striatal MT2 blockade. Behav. Brain Res. 275, 136–145. doi: 10.1016/j.bbr.2014.09.007
Okano, M., Takahata, K., Sugimoto, J., and Muraoka, S. (2019). Selegiline recovers synaptic plasticity in the medial prefrontal cortex and improves corresponding depression-like behavior in a mouse model of Parkinson’s disease. Front. Behav. Neurosci. 13:176. doi: 10.3389/fnbeh.2019.00176
Pankova, N. B., Vetrilé, L. A., Basharova, L. A., Krupina, N. A., Khlebnikova, N. N., Rodina, V. I., et al. (2004). Immunization of rats with conjugates of dopamine and serotonin with bovine serum albumin prevents the development of experimental MPTP-induced depressive syndrome (electrophysiological parameters). Neurosci. Behav. Physiol. 34, 131–138. doi: 10.1023/b:neab.0000009207.38914.2b
Petri, D., de Souza Silva, M. A., Chao, O. Y., Schnitzler, A., and Huston, J. P. (2015). Serotonergic interaction between medial prefrontal cortex and mesotelencephalic DA system underlies cognitive and affective deficits in hemiparkinsonian rats. Neuroscience 307, 51–63. doi: 10.1016/j.neuroscience.2015.08.022
Postuma, R. B., Berg, D., Stern, M., Poewe, W., Olanow, C. W., Oertel, W., et al. (2015). MDS clinical diagnostic criteria for Parkinson’s disease. Mov. Disord. 30, 1591–1601. doi: 10.1002/mds.26424
Prakash, A., Chopra, K., and Medhi, B. (2013). Granulocyte-colony stimulating factor improves Parkinson’s disease associated with co-morbid depression: an experimental exploratory study. Indian J. Pharmacol. 45, 612–615. doi: 10.4103/0253-7613.121374
Reijnders, J. S., Ehrt, U., Weber, W. E., Aarsland, D., and Leentjens, A. F. (2008). A systematic review of prevalence studies of depression in Parkinson’s disease. Mov. Disord. 23, 183–189. doi: 10.1002/mds.21803
Remy, P., Doder, M., Lees, A., Turjanski, N., and Brooks, D. (2005). Depression in Parkinson’s disease: loss of dopamine and noradrenaline innervation in the limbic system. Brain 128, 1314–1322. doi: 10.1093/brain/awh445
Ross, O. A., and Bu, G. (2018). A novel link between trafficking and Lewy body disorders. Lancet Neurol. 17, 571–573. doi: 10.1016/S1474-4422(18)30214-X
Sampaio, T. B., Marcondes Sari, M. H., Pesarico, A. P., Mantovani, A. C., Zeni, G., and Nogueira, C. W. (2018). 7-Fluoro-1,3-diphenylisoquinoline reverses motor and non-motor symptoms induced by MPTP in mice: role of striatal neuroinflammation. Eur. J. Pharmacol. 819, 129–135. doi: 10.1016/j.ejphar.2017.12.001
Sampaio, T. B., Soares de Souza, B., Roversi, K., Schuh, T., Poli, A., Takahashi, R. N., et al. (2019). Temporal development of behavioral impairments in rats following locus coeruleus lesion induced by 6-hydroxydopamine: involvement of β3-adrenergic receptors. Neuropharmacology 151, 98–111. doi: 10.1016/j.neuropharm.2019.04.006
Santiago, R. M., Barbieiro, J., Lima, M. M., Dombrowski, P. A., Andreatini, R., and Vital, M. A. (2010). Depressive-like behaviors alterations induced by intranigral MPTP, 6-OHDA, LPS and rotenone models of Parkinson’s disease are predominantly associated with serotonin and dopamine. Prog. Neuropsychopharmacol. Biol. Psychiatry 34, 1104–1114. doi: 10.1016/j.pnpbp.2010.06.004
Santiago, R. M., Barbiero, J., Gradowski, R. W., Bochen, S., Lima, M. M., Da Cunha, C., et al. (2014). Induction of depressive-like behavior by intranigral 6-OHDA is directly correlated with deficits in striatal dopamine and hippocampal serotonin. Behav. Brain Res. 259, 70–77. doi: 10.1016/j.bbr.2013.10.035
Santiago, R. M., Tonin, F. S., Barbiero, J., Zaminelli, T., Boschen, S. L., Andreatini, R., et al. (2015). The nonsteroidal antiinflammatory drug piroxicam reverses the onset of depressive-like behavior in 6-OHDA animal model of Parkinson’s disease. Neuroscience 300, 246–253. doi: 10.1016/j.neuroscience.2015.05.030
Schamne, M. G., Mack, J. M., Moretti, M., Matheus, F. C., Walz, R., Lanfumey, L., et al. (2018). The gender-biased effects of intranasal MPTP administration on anhedonic- and depressive-like behaviors in C57BL/6 mice: the role of neurotrophic factors. Neurotox. Res. 34, 808–819. doi: 10.1007/s12640-018-9912-4
Schintu, N., Zhang, X., and Svenningsson, P. (2012). Studies of depression-related states in animal models of Parkinsonism. J. Parkinsons Dis. 2, 87–106. doi: 10.3233/JPD-2012-12076
Schober, A. (2004). Classic toxin-induced animal models of Parkinson’s disease: 6-OHDA and MPTP. Cell Tissue Res. 318, 215–224. doi: 10.1007/s00441-004-0938-y
Schuurman, A. G., van den Akker, M., Ensinck, K. T., Metsemakers, J. F., Knottnerus, J. A., Leentjens, A. F., et al. (2002). Increased risk of Parkinson’s disease after depression: a retrospective cohort study. Neurology 58, 1501–1504. doi: 10.1212/wnl.58.10.1501
Shin, M. S., Kim, T. W., Lee, J. M., Sung, Y. H., and Lim, B. V. (2017). Treadmill exercise alleviates depressive symptoms in rotenone-induced Parkinson disease rats. J. Exerc. Rehabil. 13, 124–129. doi: 10.12965/jer.1734966.483
Silva, T. P., Poli, A., Hara, D. B., and Takahashi, R. N. (2016). Time course study of microglial and behavioral alterations induced by 6-hydroxydopamine in rats. Neurosci. Lett. 622, 83–87. doi: 10.1016/j.neulet.2016.04.049
Sinen, O., Bülbül, M., Derin, N., Ozkan, A., Akcay, G., Aslan, M. A., et al. (2021). The effect of chronic neuropeptide-S treatment on non-motor parameters in experimental model of Parkinson’s disease. Int. J. Neurosci. 131, 765–774. doi: 10.1080/00207454.2020.1754213
Singh, S., Mishra, A., Srivastava, N., and Shukla, S. (2017). MK-801 (Dizocilpine) regulates multiple steps of adult hippocampal neurogenesis and alters psychological symptoms via Wnt/β-catenin signaling in Parkinsonian rats. ACS Chem. Neurosci. 8, 592–605. doi: 10.1021/acschemneuro.6b00354
Skalisz, L. L., Beijamini, V., Joca, S. L., Vital, M. A., Da Cunha, C., and Andreatini, R. (2002). Evaluation of the face validity of reserpine administration as an animal model of depression–Parkinson’s disease association. Prog. Neuropsychopharmacol. Biol. Psychiatry 26, 879–883. doi: 10.1016/s0278-5846(01)00333-5
Souza, L. C., Martynhak, B. J., Bassani, T. B., Turnes, J. M., Machado, M. M., Moura, E., et al. (2018). Agomelatine’s effect on circadian locomotor rhythm alteration and depressive-like behavior in 6-OHDA lesioned rats. Physiol. Behav. 188, 298–310. doi: 10.1016/j.physbeh.2018.02.033
Storch, A., Ebersbach, G., Fuchs, G., Jost, W. H., Odin, P., Reifschneider, G., et al. (2008). Depression beim idiopathischen Parkinson-Syndrom. Teil 1: Epidemiologie, Pathophysiologie, Klinik und Diagnostik [Depression in Parkinson’s disease. Part 1: epidemiology, signs and symptoms, pathophysiology and diagnosis]. Fortschr. Neurol. Psychiatr. 76, 715–724. doi: 10.1055/s-2008-1038293
Sullivan, R. M., Dufresne, M. M., Siontas, D., Chehab, S., Townsend, J., and Laplante, F. (2014). Mesocortical dopamine depletion and anxiety-related behavior in the rat: sex and hemisphere differences. Prog. Neuropsychopharmacol. Biol. Psychiatry 54, 59–66. doi: 10.1016/j.pnpbp.2014.05.002
Sun, M., Wang, K., Yu, Y., Su, W. T., Jiang, X. X., Yang, J., et al. (2016). Electroacupuncture alleviates depressive-like symptoms and modulates BDNF signaling in 6-hydroxydopamine rats. Evid. Based Complement. Alternat. Med. 2016:7842362. doi: 10.1155/2016/7842362
Szot, P., Franklin, A., Miguelez, C., Wang, Y., Vidaurrazaga, I., Ugedo, L., et al. (2016). Depressive-like behavior observed with a minimal loss of locus coeruleus (LC) neurons following administration of 6-hydroxydopamine is associated with electrophysiological changes and reversed with precursors of norepinephrine. Neuropharmacology 101, 76–86. doi: 10.1016/j.neuropharm.2015.09.003
Tadaiesky, M. T., Dombrowski, P. A., Figueiredo, C. P., Cargnin-Ferreira, E., Da Cunha, C., and Takahashi, R. N. (2008). Emotional, cognitive and neurochemical alterations in a premotor stage model of Parkinson’s disease. Neuroscience 156, 830–840. doi: 10.1016/j.neuroscience.2008.08.035
Tang, J., Lu, L., Wang, Q., Liu, H., Xue, W., Zhou, T., et al. (2020). Crocin reverses depression-like behavior in Parkinson disease mice via VTA-mPFC pathway. Mol. Neurobiol. 57, 3158–3170. doi: 10.1007/s12035-020-01941-2
Thiffault, C., Langston, J. W., and Di Monte, D. A. (2000). Increased striatal dopamine turnover following acute administration of rotenone to mice. Brain Res. 885, 283–288. doi: 10.1016/s0006-8993(00)02960-7
Tuon, T., Valvassori, S. S., Dal Pont, G. C., Paganini, C. S., Pozzi, B. G., Luciano, T. F., et al. (2014). Physical training prevents depressive symptoms and a decrease in brain-derived neurotrophic factor in Parkinson’s disease. Brain Res. Bull. 108, 106–112. doi: 10.1016/j.brainresbull.2014.09.006
Vecchia, D. D., Kanazawa, L. K. S., Wendler, E., de Almeida Soares Hocayen, P., Bruginski, E., Campos, F. R., et al. (2018). Effects of ketamine on vocal impairment, gait changes and anhedonia induced by bilateral 6-OHDA infusion into the substantia nigra pars compacta in rats: therapeutic implications for Parkinson’s disease. Behav. Brain Res. 342, 1–10. doi: 10.1016/j.bbr.2017.12.041
Ventorp, F., Bay-Richter, C., Nagendra, A. S., Janelidze, S., Matsson, V. S., Lipton, J., et al. (2017). Exendin-4 treatment improves LPS-induced depressive-like behavior without affecting pro-inflammatory cytokines. J. Parkinsons Dis. 7, 263–273. doi: 10.3233/JPD-171068
Vivekanantham, S., Shah, S., Dewji, R., Dewji, A., Khatri, C., and Ologunde, R. (2015). Neuroinflammation in Parkinson’s disease: role in neurodegeneration and tissue repair. Int. J. Neurosci. 125, 717–725. doi: 10.3109/00207454.2014.982795
Vucković, M. G., Wood, R. I., Holschneider, D. P., Abernathy, A., Togasaki, D. M., Smith, A., et al. (2008). Memory, mood, dopamine and serotonin in the 1-methyl-4-phenyl-1,2,3,6-tetrahydropyridine-lesioned mouse model of basal ganglia injury. Neurobiol. Dis. 32, 319–327. doi: 10.1016/j.nbd.2008.07.015
Wang, Y., Liu, W., Yang, J., Wang, F., Sima, Y., Zhong, Z. M., et al. (2017). Parkinson’s disease-like motor and non-motor symptoms in rotenone-treated zebrafish. Neurotoxicology 58, 103–109. doi: 10.1016/j.neuro.2016.11.006
Winter, C., von Rumohr, A., Mundt, A., Petrus, D., Klein, J., Lee, T., et al. (2007). Lesions of dopaminergic neurons in the substantia nigra pars compacta and in the ventral tegmental area enhance depressive-like behavior in rats. Behav. Brain Res. 184, 133–141. doi: 10.1016/j.bbr.2007.07.002
Yan, J., Liu, A., Fan, H., Qiao, L., Wu, J., Shen, M., et al. (2020). Simvastatin improves behavioral disorders and hippocampal inflammatory reaction by NMDA-mediated anti-inflammatory function in MPTP-treated mice. Cell. Mol. Neurobiol. 40, 1155–1164. doi: 10.1007/s10571-020-00804-7
Yan, T., Sun, Y., Gong, G., Li, Y., Fan, K., Wu, B., et al. (2019). The neuroprotective effect of schisandrol A on 6-OHDA-induced PD mice may be related to PI3K/AKT and IKK/IκBα/NF-κB pathway. Exp. Gerontol. 128:110743. doi: 10.1016/j.exger.2019.110743
Yang, J., Qi, F., and Yao, Z. (2016). Neonatal Bacillus Calmette-Guérin vaccination alleviates lipopolysaccharide-induced neurobehavioral impairments and neuroinflammation in adult mice. Mol. Med. Rep. 14, 1574–1586. doi: 10.3892/mmr.2016.5425
Yapici Eser, H., Bora, H. A., and Kuruoğlu, A. (2017). Depression and Parkinson disease: prevalence, temporal relationship and determinants. Turk. J. Med. Sci. 47, 499–503. doi: 10.3906/sag-1603-101
Yu, J., Min, D., Bai, Y., Qu, L., Zou, T., and Wang, S. (2020). Electroacupuncture alleviates Parkinson disease and regulates the expression of brain-gut peptides. Exp. Anim. 69, 448–460. doi: 10.1538/expanim.19-0153
Yuan, C., Dai, C., Li, Z., Zheng, L., Zhao, M., and Dong, S. (2020). Bexarotene improve depression-like behaviour in mice by protecting against neuro-inflammation and synaptic damage. Neurochem. Res. 45, 1500–1509. doi: 10.1007/s11064-020-03012-3
Zaminelli, T., Gradowski, R. W., Bassani, T. B., Barbiero, J. K., Santiago, R. M., Maria-Ferreira, D., et al. (2014). Antidepressant and antioxidative effect of Ibuprofen in the rotenone model of Parkinson’s disease. Neurotox. Res. 26, 351–362. doi: 10.1007/s12640-014-9467-y
Zeng, X. S., Geng, W. S., Jia, J. J., Chen, L., and Zhang, P. P. (2018a). Cellular and molecular basis of neurodegeneration in Parkinson disease. Front. Aging Neurosci. 10:109. doi: 10.3389/fnagi.2018.00109
Zeng, X. S., Geng, W. S., and Jia, J. J. (2018b). Neurotoxin-induced animal models of Parkinson disease: pathogenic mechanism and assessment. ASN Neuro 10:1759091418777438. doi: 10.1177/1759091418777438
Zhang, T., Hong, J., Di, T., and Chen, L. (2016). MPTP impairs dopamine d1 receptor-mediated survival of newborn neurons in ventral hippocampus to cause depressive-like behaviors in adult mice. Front. Mol. Neurosci. 9:101. doi: 10.3389/fnmol.2016.00101
Zhang, X., Song, D., Gu, L., Ren, Y., Verkhratsky, A., and Peng, L. (2015). Decrease of gene expression of astrocytic 5-HT2B receptors parallels development of depressive phenotype in a mouse model of Parkinson’s disease. Front. Cell Neurosci. 9:388. doi: 10.3389/fncel.2015.00388
Keywords: Parkinson’s disease, non-motor symptoms, depression, animal model, neurotoxin
Citation: Mou Y-K, Guan L-N, Yao X-Y, Wang J-H, Song X-Y, Ji Y-Q, Ren C and Wei S-Z (2022) Application of Neurotoxin-Induced Animal Models in the Study of Parkinson’s Disease-Related Depression: Profile and Proposal. Front. Aging Neurosci. 14:890512. doi: 10.3389/fnagi.2022.890512
Received: 06 March 2022; Accepted: 27 April 2022;
Published: 13 May 2022.
Edited by:
Danling Wang, University of South China, ChinaReviewed by:
Roberta Marongiu, Cornell University, United StatesXian-Si Zeng, Jiaxing University, China
Copyright © 2022 Mou, Guan, Yao, Wang, Song, Ji, Ren and Wei. This is an open-access article distributed under the terms of the Creative Commons Attribution License (CC BY). The use, distribution or reproduction in other forums is permitted, provided the original author(s) and the copyright owner(s) are credited and that the original publication in this journal is cited, in accordance with accepted academic practice. No use, distribution or reproduction is permitted which does not comply with these terms.
*Correspondence: Shi-Zhuang Wei, weishizhuangyhdyy@163.com; Chao Ren, renchaotg@126.com; Yong-Qiang Ji, boltonjq@126.com
† These authors have contributed equally to this work