Abstract
By combining our improved visual orbit with the last spectroscopic solution of the single-lined spectroscopic binary, MCA 74 (ADS 16672Aa, Ab; HD 219834A), we derived its orbital solution to have a period of 6.321 years. Using the Gaia parallax, a systemic mass of 1.87 ± 0.09 and a mass ratio of q = 0.725 were obtained, which yielded individual masses of 1.07 ± 0.06
and 0.80 ± 0.04
for the main and the secondary components, respectively. The mass and the absolute magnitude of +3.52 confirm that the primary is a late G5–G8 subgiant, while the same parameters of the secondary (Mv = +6.52) suggest that it is an early K2–K3 dwarf.
Export citation and abstract BibTeX RIS
1. Introduction
The orbit determination of visual binaries with a known double-lined spectroscopic orbit is very important for stellar astronomy since a combined visual-spectroscopic solution allows the direct derivation of all of the geometric orbital elements, the individual masses, and other fundamental astrophysical parameters for the components as well as the orbital parallax without any additional assumptions. In some cases, the derived component masses are already at the 2%–3% precision level that is sufficient to constrain modern stellar models (Torres et al. 2010). Moreover, the recently published Gaia parallaxes generate similar data for single-lined spectroscopic binaries as we describe in the present work for the case of the MCA 74 binary.
The 94 Aqr system (STF 2998; ADS 16672AB; WDS 23191-1328AB) is a wide binary discovered by F. G. W. Struve in 1830 (Struve 1837) who detected a secondary component, B (V = 7.60; Bidelman 1985), at a distance of 14'' from the bright (V = 5.2) primary star, A. As for now, almost 250 astrometric measurements of the AB pair have been conducted (the last one in 2017), which all indicate a very slow orbital motion (the position angle is changed by 7° only).
Changes in the radial velocity (RV) of the A component were first detected by Campbell (1922) who suggested it to be a single-lined spectroscopic binary. On the basis of an extended set of the RV measurements, Sarma (1962) presented the first spectroscopic orbit with a period of 6.362 years. More recently, using high-precision (≈15 m s−1) RV measurements obtained with the Echelle spectrograph attached to the Okayama Astrophysical Observatory 1.88 m telescope, Katoh et al. (2013) derived an improved spectroscopic orbit with a period of 6.292 years.
The A component was first resolved astrometrically by McAlister & Hartkopf (1982) by means of the speckle interferometry technique and the binary name, MCA 74, was assigned to the Aa,Ab pair. These authors also calculated a visual orbit whose elements were in reasonable agreement with the previous spectroscopic solution (Sarma 1962). It is worth noting that McAlister & Hartkopf (1982) reported a period of 6.216 years, a systemic mass of , a magnitude difference of 1.5 mag, and a mass ratio of 0.4, assuming a trigonometric parallax of 33 mas (Hoffleit 1964). These values yielded masses of
and
and absolute magnitudes of +3.1 and +4.6 for the Aa and the Ab components, respectively. At the same time, they noticed some inconsistency between the parallax-implied GV5 and the published K2 V spectral types of the B component.
A combined visual-spectroscopic orbit with a period of 6.281 years was reported by Docobo et al. (1991) using the available set of data and applying an original methodology (Docobo et al. 1988). The visual orbit was refined by Söderhjelm (1999) and then improved by Docobo & Tamazian (2012) and Docobo et al. (2017).
With regard to the astrophysical data for the A component, we found several spectral type determinations such as G5IV (Abt 1985), G8.5 IV (Gray et al. 2006), and G8 IV (Cincunegui et al. 2007) all indicating that it belongs to the late G subgiants with a temperature close to 5400 K (Allende Prieto & Lambert 1999; Muñoz Bermejo et al. 2013; Luck 2017). Additional astrophysical parameters such as the age, the luminosity, etc. can be found in Luck (2017).
2. Improvement of the Visual Orbit
In Table 1, a summary of the spectroscopic, visual, and combined visual-spectroscopic orbits as well as some complementary parameters of MCA 74 is given. As we have already mentioned, the first visual orbit obtained for this pair was the one published by McAlister & Hartkopf (1982). In this article, the authors have made use of the spectroscopic orbit of Sarma (1962) in order to provide the masses of the two components. At that time, the last available parallax was that of 0033 taken from Hoffleit (1964).
Table 1. Summary of Spectroscopic, Visual, and Combined Visual-spectroscopic Orbits of MCA 74
Element | Spectroscopic (Sp.), Visual (Vis.), and combined visual-spectroscopic (Vis-Sp.) orbits | |||||||
---|---|---|---|---|---|---|---|---|
Sp. | Vis. | Vis-Sp. | Vis. | Vis. | Sp. | Vis. | Vis. | |
P (year) | 6.362 ± 0.029 | 6.216 ± 0.080 | 6.281 | 6.30 | 6.314 | 6.292 ± 0.007 | 6.321 | 6.321 ± 0.010 |
T | 1939.12 ± 0.06 | 1980.840 ± 0.024 | 1980.844 | 1993.5 | 1980.73 | 2006.034 ± 0.008 | 2012.286 | 2012.301 ± 0.020 |
e | 0.082 ± 0.06 | 0.185 ± 0.012 | 0.163 | 0.18 | 0.191 | 0.162 ± 0.020 | 0.193 | 0.173 ± 0.020 |
a ('') | ⋯ | 0.180 ± 0.003 | 0.183 | 0.189 | 0.187 | ⋯ | 0.188 | 0.189 ± 0.002 |
i (°) | ⋯ | 47.6 ± 1.7 | 46.1 | 45 | 45.8 | ⋯ | 45.5 | 44.5 ± 1.0 |
Ω (°) | ⋯ | 170.3 ± 1.9 | 165.6 | 157 | 162.6 | ⋯ | 340.7 | 341.9 ± 1.5 |
ω(°) | 225.7 ± 44.5 | 209.8 ± 1.9 | 213.6 | 42 | 208.3 | 212.13 ± 0.43 | 29.5 | 28.3 ± 1.5 |
K (km s−1) | +5.49 ± 0.37 | ⋯ | +4.8 | ⋯ | ⋯ | 6.025 ± 0.008 | ⋯ | ⋯ |
γ (km s−1) | +10.8 | ⋯ | +11.0 | ⋯ | ⋯ | +17.6 ± 0.07 | ⋯ | ⋯ |
a sin i (km) | 174.8 × 106 | ⋯ | ⋯ | ⋯ | ⋯ | (187.9 ± 0.518) × 106 | ⋯ | ⋯ |
![]() ![]() |
0.040 | ⋯ | ⋯ | ⋯ | ⋯ | 0.05004 ± 0.0003 | ⋯ | ⋯ |
q | ⋯ | 0.4 | ⋯ | 0.55 ± 0.11 | ⋯ | 0.51 ± 0.03 | ⋯ | ⋯ |
π used (mas) | ⋯ | 33a | 33a | 40.28 ± 1.51b | 47.35 ± 2.47c | 48.22 ± 5.25d | 47.35 ± 2.47c | 44.90 ± 0.55e |
![]() ![]() |
⋯ | 4.2 | ⋯ | 2.60 | ⋯ | ⋯ | 1.57 | 1.87 ± 0.9 |
![]() ![]() |
⋯ | ⋯ | 3.0 | ⋯ | 1.68 ± 0.25 | ⋯ | 0.87 | 1.07 ± 0.06 |
![]() ![]() |
⋯ | ⋯ | 1.2 | ⋯ | 0.92 ± 0.17 | ⋯ | 0.69f | 0.80 ± 0.04 |
Δm | ⋯ | 1.5 | ⋯ | 3.27 | ⋯ | ⋯ | 3.1 | 3.1 |
rms(θ) (°) | ⋯ | 14.10 | 6.64 | 3.81 | 3.80 | ⋯ | 3.90 | 3.26 |
rms(ρ) ('') | ⋯ | 0.016 | 0.012 | 0.007 | 0.008 | ⋯ | 0.007 | 0.007 |
Referenceg | 1 | 2 | 3 | 4 | 5 | 6 | 7 | 8 |
Notes.
aHoffleit (1964). breported by the author. cvan Leeuwen (2007). dPerryman et al. (1997). eGaia Collaboration et al. (2018). fMinimum mass. gReferences: 1. Sarma (1962); 2. McAlister & Hartkopf (1982); 3. Docobo et al. (1991); 4. Söderhjelm (1999); 5. Docobo & Tamazian (2012); 6. Katoh et al. (2013); 7. Docobo et al. (2017); 8. This work.Download table as: ASCIITypeset image
A slight discordance between the elements of the spectroscopic orbit with those of the visual one and especially the residuals of the latter derived from the subsequent speckle observations was the reason for which Docobo et al. (1991) revised the visual orbit and presented a combined (visual-spectroscopic) solution, changing the period from 6.218 to 6.2808 years. However, that solution still yielded an excessively large global mass for the spectral types of the components, which certainly was a result of the adopted parallax underestimation. Later on, by combining the Hipparcos astrometry and the intermediate Transit Data with the ground-based observations, Söderhjelm (1999) suggested a period of 6.30 years.
Using eight new speckle observations, the visual orbit was revised again by Docobo & Tamazian (2012) who obtained a slightly increased period of 6.314 years. Later improvements of the orbit reported in Docobo et al. (2017) and in the present work (both with a similar period of 6.321 years) are mainly motivated by the publication of a new spectroscopic orbit (Katoh et al. 2013), which allowed us to obtain the masses with the highest possible precision. In the present work, we take into account three new speckle observations in 2014, of which two were reported by Tokovinin et al. (2015) and the last one was conducted by us with the 6 m telescope of SAO (Russia). In addition, we have made use of the recently published Gaia parallax (Gaia Collaboration et al. 2016, 2018) for the mass calculation. This is not a joint solution of RV and astrometric data. From Katoh et al. (2013), we have made use of the K value only. Indeed, this solution should be considered as visual rather than visual-spectroscopic. However, the linear value of the semimajor axis was obtained from the spectroscopic orbit using the orbital elements P, e, and i of the visual orbit.
As seen from the data given in Table 1, our present solution provides better quality controls (root mean square of residuals, rms) both in θ and ρ. The apparent orbit of the MCA 74 system with the corresponding measurements is represented in Figure 1. Finally, the ephemerides derived for the coming years are given in Table 2.
Figure 1. Apparent orbit of MCA74. The blue dots represent the observations, and the dashed line represents the line of the nodes.
Download figure:
Standard image High-resolution imageTable 2. Ephemerides for the Coming Years
Epoch | 2018.00 | 2018.25 | 2018.50 | 2018.75 | 2019.00 | 2019.25 | 2019.50 | 2019.75 |
θ (°) | 326.7 | 340.6 | 355.2 | 11.8 | 31.7 | 55.3 | 79.9 | 101.3 |
ρ ('') | 0.160 | 0.159 | 0.153 | 0.141 | 0.128 | 0.120 | 0.123 | 0.137 |
Epoch | 2020.00 | 2020.25 | 2020.50 | 2020.75 | 2021.00 | 2021.25 | 2021.50 | 2021.75 |
θ (°) | 118.2 | 131.2 | 141.8 | 150.7 | 158.8 | 166.4 | 174.0 | 181.8 |
ρ ('') | 0.156 | 0.175 | 0.193 | 0.206 | 0.214 | 0.218 | 0.216 | 0.210 |
Epoch | 2022.00 | 2022.25 | 2022.50 | 2022.75 | 2023.00 | 2023.25 | 2023.50 | 2023.75 |
θ (°) | 190.3 | 199.8 | 210.8 | 223.7 | 238.9 | 256.1 | 274.2 | 291.8 |
ρ ('') | 0.200 | 0.187 | 0.173 | 0.159 | 0.148 | 0.141 | 0.141 | 0.145 |
Download table as: ASCIITypeset image
3. Calculation of Masses
In order to determine the individual masses, we will use the elements of our visual orbit. In any case, the orbital elements of the visual and spectroscopic solutions are very close. Nevertheless, the value of K1 from the spectroscopic orbit is necessary to obtain the semimajor axis of the orbit of the main component with respect to the center of mass, a1.
As is usual, the total mass of the system is calculated from Kepler's Third Law, that is to say

where a'' and P are the semimajor axis and the period of the visual orbit, π'' is the Gaia parallax, and a is the semimajor axis expressed in astronomical units.
Taking into account the values of these parameters,
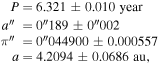
the result is

On the other hand, we can obtain the semimajor axis of the orbit of the main component with respect to the center of mass by means of

In this expression, we have taken K1 = 6.025 ± 0.008 km s−1 = 1.2709 ± 0.0017 au yr−1 from the spectroscopic orbit, and e, , and i from the visual orbit.
Now, we are also able to calculate the semimajor axis of the orbit of the secondary, a2,

and, remembering that

we have determined the sum (2) and the ratio of masses (5). From them, the individual masses are deduced:
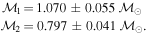
Finally, we can check our results against the mass function of the spectroscopic orbit, in effect, from
- 1.the spectroscopic orbit,
and
- 2.the visual orbit,
4. Discussion
With a magnitude difference of Δm = 1.5, the a sin i value taken from Sarma (1962) and assuming a parallax of 33 mas, McAlister & Hartkopf's visual orbit (1982) led to individual masses of 3.0 (MVAa = +3.1) and 1.2
(MVAb = +4.6) for the Aa and the Ab components, respectively. As noticed by these authors, the luminosities were generally consistent with a G5 subgiant and a G0–G2 dwarf. On the other hand, in practically all subsequent speckle observations of MCA 74, the magnitude difference oscillates in a narrow range between 2.8 and 3.2 mag, and (averaging the Ia/Ib ratios) a more feasible value of Δm = 3.1 should thus be applied to this pair.
Our visual orbit reported in the present work, the application of the Gaia parallax (44.90 mas), and a high-precision value of the RV semi-amplitude given in Katoh et al. (2013) yielded much lower masses of (MVAa = +3.52) and
(MVAb = +6.52) suggesting that the Aa and Ab components are a late G8 subgiant and a K2–K3 dwarf, respectively (Schmidt-Kaler 1982; Gray 2005).
Finally, adopting the Gaia parallax of 44.90 mas, we readily obtain for the distant B component a luminosity of MVB = +5.92 corresponding to a K0 dwarf (Schmidt-Kaler 1982). This result is close to G. Kuiper's K2 V classification (Bidelman 1985) thus fixing the inconsistency of its spectral type (G5V) obtained by McAlister & Hartkopf (1982) due to the underestimated parallax value they used.
5. Conclusions
New speckle measurements performed with the 4.0 m SOAR telescope (Tokovinin et al. 2015) and with the 6.0 m SAO telescope (unpublished) have yielded a precise visual orbit for the 94 Aqr A binary. Also, using the last spectroscopic orbit (Katoh et al. 2013) of this system and the recent published value of the Gaia parallax allowed us to determine the individual masses with the uncertainty of less than 5%.
Apart from this, the use of the Gaia parallax permitted us to remove the inconsistency in the spectral type of the distant B component, which is now close to the previously determined spectral type, K2V (MV = +5.9).
This work used data from the European Space Agency (ESA) Gaia mission (https://www.cosmos.esa.int/gaia), processed by the Gaia Data Processing and Analysis Consortium (DPAC). Funding for the DPAC has been provided by national institutions, in particular, the institutions participating in the Gaia Multilateral Agreement. This research made use of the Washington Double Star Catalogs maintained at the U.S. Naval Observatory. This paper was supported by the Spanish Ministerio de Economía, Industria y Competitividad under the Project AYA-2016-80938-P (AEI/FEDER, UE) and by the Xunta de Galicia under the Rede IEMath-Galicia, ED341DR 2016/022 grant.