Effects of Dietary Probiotic (Bacillus subtilis) Supplementation on Carcass Traits, Meat Quality, Amino Acid, and Fatty Acid Profile of Broiler Chickens
- 1State Engineering Technology Institute for Karst Desertfication Control, School of Karet Science, Guizhou Normal University, Guiyang, China
- 2State Key Laboratory of Grassland Agro-Ecosystems, School of Life Sciences, Lanzhou University, Lanzhou, China
The aim of the present study was to evaluate the effects of dietary supplementation with or without Bacillus subtilis (B. subtilis) on carcass traits, meat quality, amino acids, and fatty acids of broiler chickens. In total, 160 1-day-old Arbor Acres male broiler chicks were divided into two groups with eight replicates of 10 chicks each. Chickens received basal diets without (CN group) or with 500 mg/kg B. subtilis (BS group) for 42 days. Eight chickens from each group were slaughtered at the end of the trial, and carcass traits, meat quality, chemical composition, amino acid, and fatty acid profile of meat were measured. The results showed that the breast muscle (%) was higher in BS than in CN (p < 0.05), while abdominal fat decreased (p < 0.05). The pH24h of thigh muscle was increased (p < 0.05) when supplemented with BS; however, drip loss, cooking loss of breast muscle, and shear force of thigh muscle decreased (p < 0.05). Lysine (Lys), methionine (Met), glutamic acid (Glu), and total essential amino acid (EAA) in breast muscle and Glu in thigh muscle were greater in BS than in CN (p < 0.05). C16:1, C18:1n9c, and MUFA in breast muscle and thigh muscle were greater in BS than in CN (p < 0.05). In conclusion, dietary supplementation with B. subtilis could improve the carcass traits and meat quality of broilers, which is beneficial for the consumers due to the improved fatty acid profile and amino acid composition.
Introduction
The widespread use of antibiotics has led to the emergence of resistant bacteria and drug residues in animal products, which directly or indirectly endangers human's health and environmental safety (1, 2). Nowadays, with the development of molecular biology, genetic improvements in broiler production have improved body size and growth rate of broilers, resulting in a higher yield of meat in broilers (3). However, the meat quality was decreased drastically with the rapid growth of broilers. Thus, in order to satisfy people's growing demand for high-quality meat, the improvement of meat quality becomes a current hot topic in the field of animal nutrition. Previous studies have demonstrated that probiotics can play a role in improving the microbial balance and intestinal environment (4–7) and are believed to promote the growth performance (8) and meat quality of broilers by multiple ways (9, 10). It is important to note that in all of these studies, Bacillus species, including Bacillus subtilis (B. subtilis), Bacillus cereus (B. cereus), Bacillus clausii (B. clausii), and Bacillus coagulans (B. coagulans), have been identified as effective probiotics to promote animal growth, maintain intestinal barrier function, and promote meat quality of broilers (9, 11–16).
The adverse environment such as low pH value, high temperature, and relatively greater bile salt within gastrointestinal tract is a severe challenge for probiotics survival (17). B. subtilis is a spore gram-positive aerobic bacterium that has a better heat stability and higher acid tolerance, making it a potential feed additive in animal production (18, 19). Previous studies have demonstrated that the properties of probiotic bacteria vary from strain to strain (20). With respect to B. subtilis, multiple strains, including B. subtilis DSM 17299 (19), B. subtilis fmbJ (12), B. subtilis RX7 and B2A (13), B. subtilis C-3102 (21), B. subtilis 29784 (22), B. subtilis BYS2 and BG5 (23), B. subtilis 1781 and 747 (24), and B. subtilis DSM 32315 (25–28), have been gaining interest as they have been indicated to improve the growth performance, feed efficiency, antioxidant capability, immune function, and intestinal microflora balance of broilers. B. subtilis DSM 32315, a unique naturally occurring strain, has shown to improve growth performance and intestinal microflora balance of broilers (25–29). For example, Ma et al. (25) showed that dietary supplementation with B. subtilis DSM 32315 improved growth performance, intestinal structure, and the manipulation of cecal microbial composition of broilers. Sokale et al. (26) indicated that dietary supplementation with B. subtilis DSM 32315 can maintain the intestinal structural integrity and ameliorate the pathology and performance detriments associated with necrotic enteritis challenge. However, previous studies about the application of B. subtilis DSM 32315 in poultry mainly concentrated in growth performance and intestinal health; the effect of dietary supplementation with B. subtilis DSM 32315 on carcass traits and meat quality of broiler chickens has not been investigated in detail. Thus, the present study aims to investigate the effects of B. subtilis DSM 32315 supplementation on carcass traits, meat quality, amino acid (AA), and fatty acid profile of broiler chickens to provide more references for the application of B. subtilis in poultry production.
Materials and Methods
Birds, Experimental Design, Diets, and Management
The experimental procedures involving animals were reviewed and approved by the animal welfare committee of the Guizhou Normal University (Guiyang, China). A total of 160 1-day-old Arbor Acres (AA) male broiler chicks with similar initial body weights were randomly divided into two groups with eight replicates of 10 chicks each. Chickens received basal diets without (CN group) or with 500 mg/kg B. subtilis (BS group, B. subtilis DSM 32315, 2.0 × 109 spores/g, Evonik Nutrition & Care GmbH, Germany) throughout the trial period (25). The basal diets (Table 1) were formulated according to the nutrient requirements of the Chinese Feeding Standard of Chicken (NY/T 33-2004, China's Ministry of Agriculture). The experiment lasted for 42 days, which was divided into two phases (phase 1: days 1–21 and phase 2: days 22–42). All birds were raised in wire cages in an environmentally controlled room with continuous incandescent white light throughout the experiment and had ad libitum access to water and feed. The room temperature was maintained at 33°C for the first 3 days and at 32°C to 30°C for days 4–7, and then reduced by 23°C per week until it reached 22–24°C.
Carcass Traits
At the end of the feeding trial, the diet was removed 12 h before slaughter. Eight middle-weighted birds (one bird/replicate) in each treatment were selected for sample collection. All selected animals were weighed individually, and then slaughtered by severing the jugular vein after electroshock. Then, the carcasses were cut according to a standardized procedure (NY/T 823-2004) to determine the dressing percentage, semi-eviscerated percentage (%), eviscerated percentage (%), breast muscle (%), and abdominal fat (%). After defeathering, head removal, hock cut, and evisceration, the carcass weight, semi-eviscerated weight, and eviscerated weight were recorded. The dressing percentage (%) was calculated by dividing final body weight with carcass weight [(carcass weight/body weight) × 100], the semi-eviscerated percentage (%) was calculated by dividing final body weight with semi-eviscerated weight [(semi-eviscerated weight/body weight) × 100], and the eviscerated percentage (%) was calculated by dividing final body weight with eviscerated weight [(eviscerated weight/body weight) × 100]. The breast muscle, leg muscle, and abdominal fat were removed and weighed. The breast muscle (%) was calculated by dividing eviscerated weight with breast muscle weight [(breast muscle weight/eviscerated weight) × 100], the leg muscle (%) was calculated by dividing eviscerated weight with leg muscle weight [(leg muscle weight/eviscerated weight) × 100], and the abdominal fat (%) was calculated by dividing eviscerated weight plus abdominal fat weight with abdominal fat weight [abdominal fat weight/(eviscerated weight + abdominal fat weight) × 100]. Approximately 5.0 g of muscles from the medial pectoralis major and thigh were sampled for chemical composition measurement. The remaining muscles from each bird were kept at 4°C for the analysis of meat quality.
Meat Quality Assessment
Meat quality was assessed by determining pH, meat color, drip loss, shear force (N), and cooking loss of the breast and thigh muscle. The pH of breast and thigh muscle was measured at 24 h (pH24h) postmortem by insertion of a handheld pH meter (Russell CD700, Russell pH Limited, Germany). Meat color, including lightness (L*), redness (a*), and yellowness (b*), was measured using Chroma Meter (Opto-Star Lab, Matthaus, Germany) according to the manufacturer's instructions. The measurements of drip loss from 0 to 24 h were conducted as described by Zhou et al. (16). Briefly, ~30 g of muscle sample was weighed and put into zip-lock plastic bags and stored in a chilling room at 4°C for 24 h and then reweighed after wiping out the surface moisture, and the drip loss was calculated as: drip loss (%) = [(initial weight – final weight)/final weight] × 100. The measurements of shear force and cooking loss were conducted as described by Cai et al. (3) with appropriate modification. Approximately 20 g of a sample of muscle sample was weighted and heated in a water bath to a final internal temperature of 75°C. After cooling to room temperature, the muscle sample was reweighed after wiping out the surface moisture and the cooking loss was calculated as: cooking loss (%) = [(initial weight – final weight)/final weight] × 100. The sample used for the measurement of cooking loss was then used for the measurement of shear force. The sample was cut into 2 cm (length) × 1 cm (width) × 0.5 cm (height) along the direction of the muscle fibers, and the shear force was measured using a digital texture analyzer (Model 2000D, G-R, US).
Chemical Composition Analysis
Muscle samples were cut into slices, dried in a vacuum-freeze dryer, and then ground into powder. The moisture, crude ash, crude protein (CP), and extracted fat (EE) were measured according to the methods described by AOAC [2000; (30)].
To measure the AA content in breast and thigh muscle, the samples were pretreated according to AOAC [2000; (30)]. Briefly, ~200 mg of fresh muscle sample was accurately weighed into the hydrolysis tube (about 15 ml), 10 ml of hydrochloric acid (6 mol/L) was added, and the mixed sample was filled with nitrogen, sealed, and put in a constant temperature drying box at 110°C for hydrolysis for 24 h; after cooling and mixing well, 100 μl of mixed sample was taken out and dried at 55°C and then 1,000 μl of AA sample diluent was added and mixed well; 1 ml of mixed sample was filtered used a needle filter (diameter 0.2 μm) and then analyzed using an automatic AA analyzer (L-8800; Hitachi, Tokyo, Japan) according to the manufacturer's instructions, and the contents of AA were expressed as mg/g wet tissue.
To measure the fatty-acid profiles in breast and thigh muscle, the samples were pretreated according to AOAC [2000; (30)]. Briefly, ~50–200 mg of freeze-dried powder samples was accurately weighed into a 10-ml glass tube, and 3 ml of chloroform–methanol–water (volume ratio: 8:4:3) mixture was added. The mixture was shaken in a whirlpool for 1 min, followed by ultrasound for 5 min in an ice bath, repeated three to five times, left for 2 h, and centrifuged at 1,500 rpm for 15 min. The chloroform layer was transferred to another 10-ml glass centrifuge tube and dried in a vacuum drying oven to obtain the mixture of fatty acid and glyceryl ester; 2 ml of KOH-CH3OH (0.5 mol/L) was added and placed in a slightly boiling water bath for 10 min; after cooling for 3 min, 2 ml of BF3-CH3OH solution (10%) was added and then placed in a water bath at 80°C to heat reflux for 2 min, cooled to room temperature, added 1 ml n-hexane and 3 ml of saturated NaCl solution, fully mixed, and centrifuged at room temperature at 2,000 rpm for 5 min, and then the upper n-hexane layer was absorbed into the gas phase vial for the fatty-acid profiles analyzed using an automatic fatty acid analyzer (Shimadzu GC-2010 plus, Japan) according to the manufacturer's instructions.
Statistical Analysis
All the data were subjected to the paired t-test using SPSS 21.0 programs (SPSS, Inc., Chicago, IL, USA). Results are expressed as the mean ± standard deviation (SD). Probability values < 0.05 were taken to indicate statistical significance.
Results
Carcass Traits
The effects of B. subtilis supplementation on carcass traits of broiler chickens are presented in Table 2. Compared to the control group, diet supplementation with B. subtilis improved the breast muscle percentage (27.14 vs. 25.04%; p < 0.05), but decreased the abdominal fat percentage (1.52 vs. 1.74%; p < 0.05). No influences were observed in carcass weight, dressing percentage, semi-eviscerated percentage, eviscerated percentage, and leg muscle percentage (P > 0.10) among the treatments.
Meat Quality
The effects of B. subtilis supplementation on meat quality of broiler chickens are presented in Table 3. Supplementation with B. subtilis improved the pH24h value (5.97 vs. 5.73; p < 0.05), but decreased the shear force (15.19 vs. 18.04; p < 0.05) in the thigh muscle compared to the control group. For the breast muscle, dietary supplementation with B. subtilis significantly increased Yellowness (b*) (7.89 vs. 7.61; p < 0.05) compared to the control group, but decreased the drip loss (3.24 vs. 4.08; p < 0.05) and the cooking loss (16.66 vs. 18.27; p < 0.05) compared to the control group. In addition, dietary supplementation with B. subtilis had a tendency to decrease shear force (19.81 vs. 24.67; p = 0.072) in the breast muscle compared to the control group. It seems that the thigh muscle had a lower Lightness (L*), Yellowness (b*), drip loss, cooking loss, and shear force (p < 0.05), but had a higher Redness (a*) (P < 0.05) than breast muscle.
Meat Chemical Composition
The effects of B. subtilis supplementation on meat chemical composition (moisture, crude protein, ether extract, and crude ash) of broiler chickens are presented in Table 4. Dietary supplementation with B. subtilis had no effect on chemical composition of both breast and thigh muscles of broilers, and only had a tendency (p = 0.093) to increase ether extract content in the thigh muscle. In addition, the content of crude protein in breast muscle was higher, and ether extract was much lower than thigh muscle (P < 0.05).
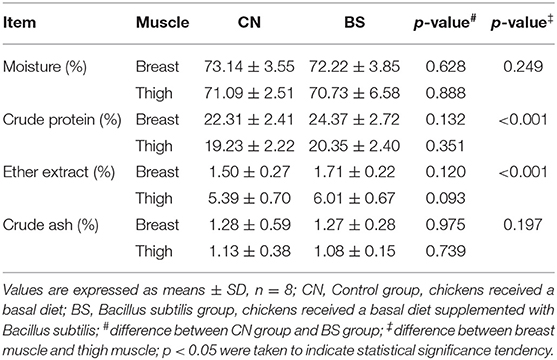
Table 4. Effects of Bacillus subtilis supplementation on conventional chemical composition of broiler chickens.
Meat Amino Acid Composition
The AA contents in breast muscle and thigh muscle are presented in Table 5. In the breast muscle, the content of lysine (Lys, 20.26 vs. 18.53 mg/g; p < 0.05), methionine (Met, 4.28 vs. 3.74 mg/100 g; p < 0.05), glutamic acid (Glu, 18.03 vs. 16.88 mg/100 g; p < 0.05), and the total essential amino acid (EAA, 81.19 vs. 77.20 mg/100 g; p < 0.05) was greater when supplemented with B. subtilis than the control group. In addition, dietary supplementation with B. subtilis had a tendency to increase the total AA (164.32 vs. 155.44 mg/100 g; p = 0.073) content in the breast muscle compared to the control group. In the thigh muscle, dietary B. subtilis supplementation increased the Glu (16.83 vs. 15.78 mg/100 g; p < 0.05) content and had a tendency to increase the Lys content (17.98 vs. 16.70 mg/100 g; p = 0.078) compared to the control group. It seems that most AAs [except phenylalanine (Phe), cysteine (Cys), glycine (Gly), and proline (Pro)] in the breast muscle were much higher than in the thigh muscle (p < 0.05).
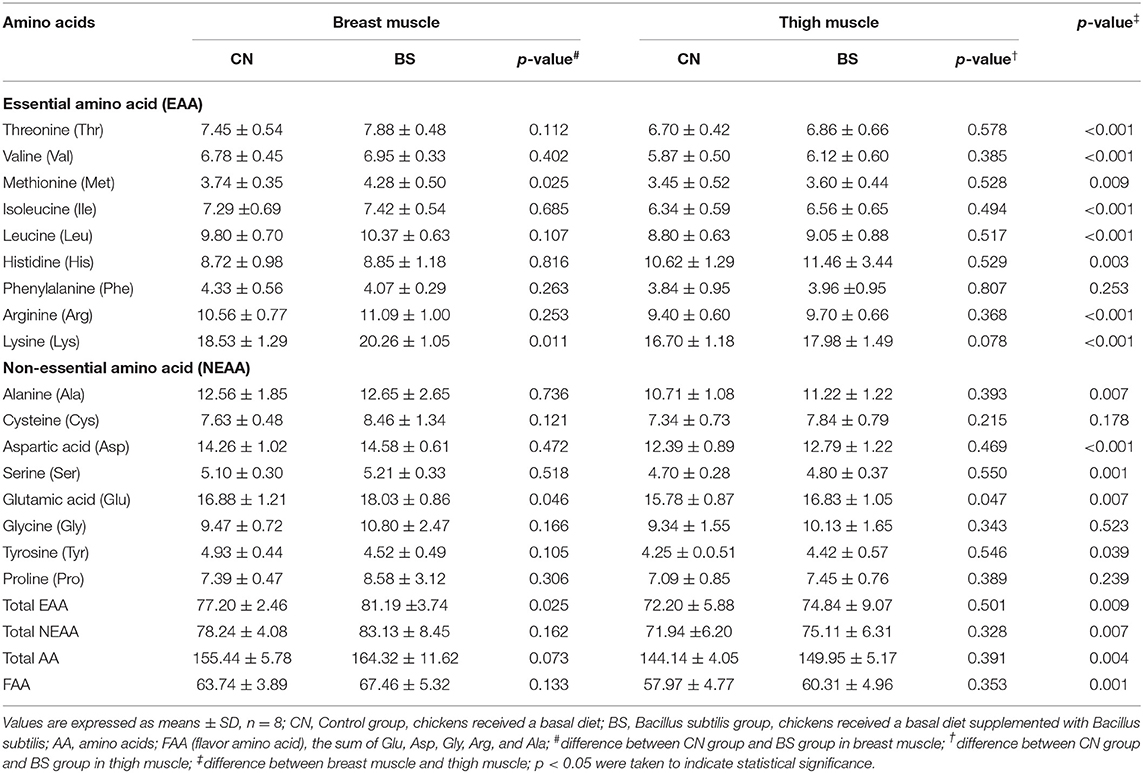
Table 5. Effects of Bacillus subtilis supplementation on amino acid content in breast muscle and thigh muscle of broiler chickens (mg/100 g DM).
Meat Fatty Acid Composition
The fatty acid contents in breast muscle and thigh muscle are presented in Table 6. The palmitoleic acid (C16:1, 3.228 vs. 1.938%; p < 0.05), oleic acid (C18:1n9c, 36.671 vs. 33.904%; p < 0.05), Cis-8,11,14-eicosatrienoate (C20:3n6, 1.387 vs. 1.109%; p < 0.05), and total monounsaturated fatty acid (MUFA, 41.190 vs. 37.074%; p < 0.05) were higher when supplemented with B. subtilis, but the palmitic acid (C16:0, 26.762 vs. 29.049%; p < 0.05) and arachidic acid (C20:0, 0.256 vs. 0.437%; p < 0.05) decreased in the breast muscle. In addition, dietary supplementation with B. subtilis had a tendency to increase eicosadienoic acid (C20:2, 0.468 vs. 0.569%) and the total unsaturated fatty acids (UFA, 59.160 vs. 55.514%, p = 0.083), and had a tendency to decrease the total saturated fatty acid (SFA, 40. 842 vs. 44.486%, p = 0.059) in the breast muscle compared to the control group. For the thigh muscle, dietary supplementation with B. subtilis increased the C16:1 (4.972 vs. 3.874%; p < 0.05), C18:1n9c (36.116 vs. 33.261%; p < 0.05), and MUFA (43.062 vs. 38.880%; p < 0.05), and decreased the C16:0 (23.747 vs. 24.924%; p < 0.05) and the total SFA (37.061 vs. 40.247%; p < 0.05) compared to the control group. There is a tendency to increase the myristoleic acid (C14:1, 0.060 vs. 0.054%, p = 0.057), nervonic acid (C24:1n9, 0.052 vs. 0.032%, p = 0.076), and the total UFA (62.939 vs. 59.752%, p = 0.066) in the thigh muscle when supplemented with B. subtilis. The results from the present study showed that the fatty acid content in breast muscle and thigh muscle is greatly different.
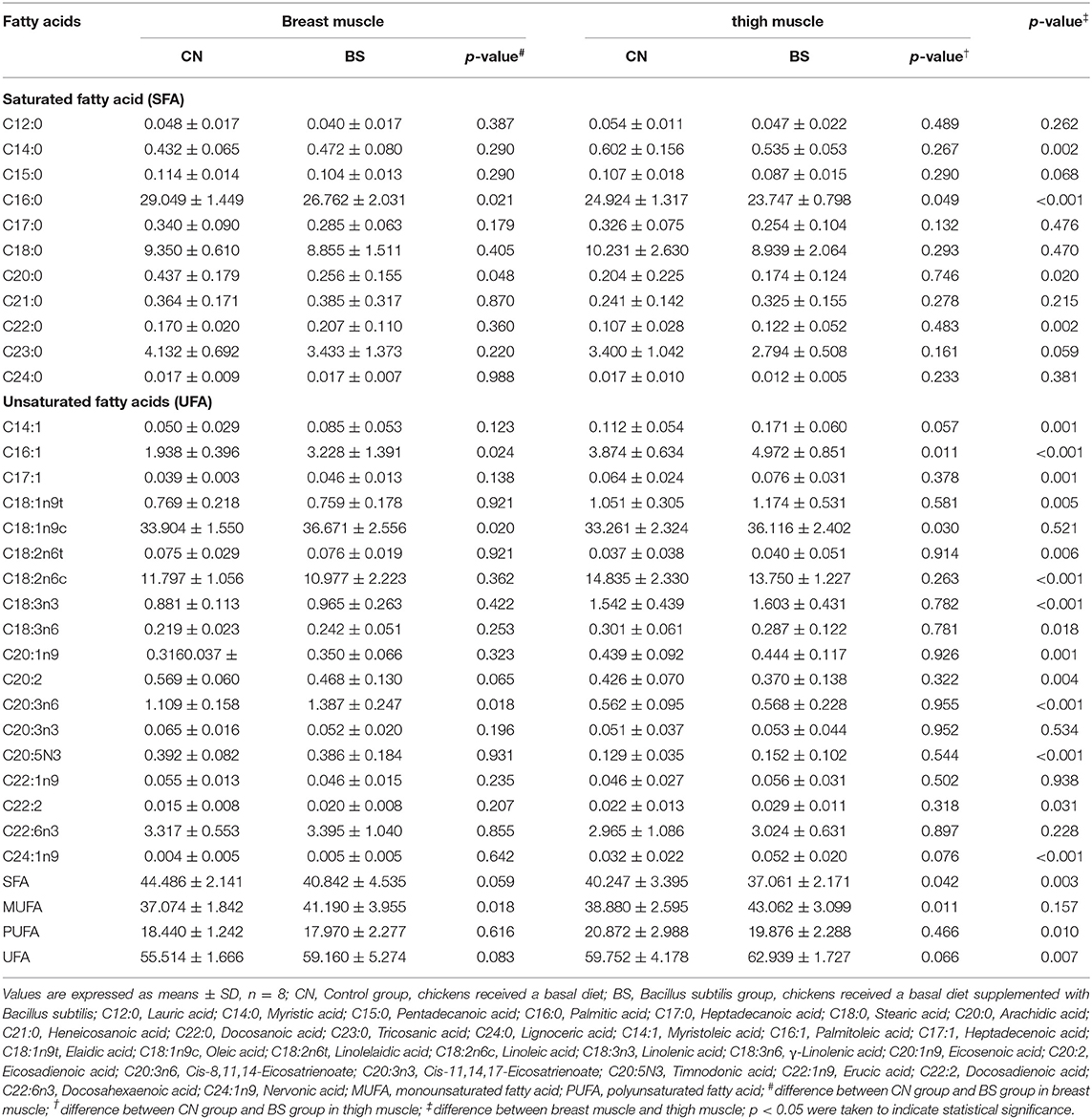
Table 6. Effects of Bacillus subtilis supplementation on fatty acids content in breast muscle and thigh muscle of broiler chickens (%).
Discussion
In the present study, we mainly investigated the effects of dietary supplementation with B. subtilis DSM 32315 on carcass traits, meat quality, AA, and fatty acid profile of broiler chickens. The results of the present study showed that dietary supplementation with B. subtilis DSM 32315 has a remarkable effect on carcass traits and could improve the meat quality and meat flavor of broiler chickens through improving the AA and fatty acid profiles. Carcass traits provide important indices in broiler production. In the present study, dietary supplementation with B. subtilis significantly increased the breast muscle and significantly decreased the abdominal fat of broiler chickens. However, B. subtilis supplementation had no effect on the carcass weight, dressing percentage, semi-eviscerated percentage, eviscerated percentage, and leg muscle, which is consistent with the results from Yadav et al. (31) who reported that dietary supplementation with B. subtilis (DM 03, TAM 4 and IQB 350) significantly increased the breast muscle weight of broilers. In contrast, Cramer et al. (11) reported that dietary supplementation with B. subtilis did not affect the breast muscle weight of broilers exposed to chronic heat stress, and Park and Kim (13) reported that dietary supplementation with B. subtilis RX7 or B2A did not affect the abdominal fat of broilers challenged with Salmonella typhimurium. This is probably because the beneficial effects of B. subtilis in broilers were markedly strain-dependent (7, 32). Abdominal fat is an important index used to measure lipid deposition in broilers (33, 34). Excessive fat deposition is undesirable, because it degrades meat quality, decreases feed efficiency, and increases production and health costs (35, 36). The lower abdominal fat in broiler chickens supplemented with B. subtilis in the present study indicated that B. subtilis can lead to a higher economic efficiency since the public tends to favor low-fat-content chicken.
The pH value, meat color (lightness, redness, and yellowness), drip loss, cooking loss, and shear force are commonly used indices for evaluating meat quality (37). Previous studies have reported that dietary supplementation with B. subtilis could improve the carcass traits and meat quality of broilers by improving the pH, tenderness, and color (11–13). pH is related to shelf life, color, and water-holding capacity of the meat, which are determined mostly by postmortem conversion of muscle glycogen to lactic acid, and often used as an important indicator to evaluate meat quality (38, 39). The higher pH24h in the muscle of broilers fed with B. subtilis indicated a better shelf life, color, and water-holding capacity of meat. Similarly, Cramer et al. (11) also showed that dietary supplementation with B. subtilis increased the pH24h of breast muscle. In contrast, Park and Kim (13) and Bai et al. (12) reported that dietary supplementation with B. subtilis had no effect on the pH value of meat. B. subtilis supplementation did not affect the meat color (a*, b*, and L*) in the present study, which is consistent with Jeong and Kim (21) and Cramer et al. (11), who also reported that B. subtilis had no effect on the meat color. However, in contrast, Bai et al. (12) reported that B. subtilis could decrease L* and b*, and increase a*. Drip loss and cooking loss could reflect the water-holding capacity and are related to nutrition, flavor, and juiciness, because some nutrients are easily lost during exudation by water loss (40, 41). In the present study, dietary supplementation with B. subtilis decreased the drop loss and cooking loss of the breast muscle after storage for 1 day, which suggested the increase of water-holding capacity of meat. The results of our study support the previous research, which concluded that dietary supplementation with B. subtilis RX7 or B2A in broiler diets increased the drip loss of breast meat (13). However, the detailed reasons for B. subtilis decreased the drip loss and cooking loss was unclear. Tenderness is considered as the most important trait for consumers, which can be represented by shear force (42). It was clear from our study that the administration of B. subtilis had a beneficial effect on meat tenderness with a decreased shear force. Inconsistent results between studies have been reported for the impact of B. subtilis supplementation on meat tenderness. Similarly to the present study, Bai et al. (43) reported that the administration of B. subtilis in the diets could decrease the shear force and thus improve muscle tenderness, whereas Cramer et al. (11) and Abdulla et al. (44) had found that dietary supplementation with B. subtilis had no difference in shear force. Therefore, the present study indicated that dietary supplementation with B. subtilis could improve the meat quality of broilers by improving the pH and tenderness and by decreasing the drop loss and cooking loss.
In the current study, B. subtilis treatment did not affect the routine nutrients (moisture, crude protein, ether extract, and crude ash) in both breast and thigh muscles compared to the control group, but it showed that dietary supplementation with B. subtilis had a tendency to improve the ether extract content in the thigh muscle. Similarly, Zhou et al. (16) indicated that dietary probiotic Bacillus coagulans supplementation did not affect moisture, crude protein, ether extract, and crude ash content in the muscles of Guangxi Yellow chicken. In contrast, Abdulla et al. (44) showed that dietary supplementation with B. subtilis significantly decreased the fat content in breast and thigh muscles of broilers. Intramuscular fat content is an important sensory characteristic of meats and is related to tenderness, succulence, and flavor of meats (45). The higher fat content of thigh muscle from B. subtilis treatment in the present study suggested better tenderness, succulence, and flavor of meats.
The AA content in meat is important to evaluate the quality and flavor of meat. EAA determines the muscle protein quality, and flavor amino acid (FAA), such as alanine (Ala), glycine (Gly), Glu, aspartic acid (Asp), and serine (Ser), especially Glu, can greatly contribute to the taste of meat (38, 46). In the breast muscle, Met, Lys, Glu, total EAA, and total AA were increased by B. subtilis treatment in the present study. In the thigh muscle, only Glu content was increased by B. subtilis treatment. The reasons for this phenomenon might be due to the higher content of total AA in the breast muscle that made it more sensitive to FAAs. The results from Guo et al. (37) and Chen et al. (47) also support this opinion, who reported that breast muscle contained higher levels of total AAs than those of thigh muscle in broiler chickens. Lys and Met are two kinds of important EAA in meat of broilers. The higher Lys and Met in the meat of broilers fed with B. subtilis meant that B. subtilis had a beneficial effect on improving the protein quality and nutrition value of muscle. Glu is one of the most important FAA, which is the primary flavor molecule and functions in meat freshness and buffering salty and sour tastes (48). The Glu content in breast muscle and thigh muscle both increased due to B. subtilis treatment, indicating that B. subtilis has a role in promoting the meat flavor. In a word, B. subtilis could improve the meat protein quality as well as meat flavor of broilers.
It is well-known that fatty acids, including MUFA, polyunsaturated fatty acids (PUFA), and SFA, are an important indicator to evaluate the quality and nutritional value of meat and they also are the basis for the characteristic flavor of meat (49). The major fatty acids in chicken meat are C16:0, stearic acid (C18:0), C18:1n9c, and linoleic acid (C18:2n6c) (50). It is generally accepted that higher dietary intakes of SFA, especially C16:0 and myristic acid (C14:0), are positively correlated with increased risk of coronary heart disease, due to their ability to raise the total cholesterol and low-density lipoprotein (51, 52). In the present study, B. subtilis treatment significantly decreased C14:0, C16:0, and C20:0 in the breast muscle, and significantly decreased C16:0 and total SFA in the thigh muscle, which indicated that the muscles from broilers treated with B. subtilis might be more beneficial to human health. The UFA not only is the precursor substance to meat flavor, but also plays a wide range of roles in promoting growth, scavenging free radicals, and regulating lipid metabolism (49). In the present study, B. subtilis treatment significantly increased C16:1, C18:1n9c, C20:3n6, and total MUFA in the breast muscle, and significantly increased C16:1, C18:1n9c, and total MUFA in the thigh muscle. C18:1n9c is the most abundant UFA, which is suggested to be positively associated with the softness of fat and the sensory quality of meat; thus, rich oleic acid content can make meat tasty (51, 52). The higher C18:1n9c content in muscles from broilers treated with B. subtilis meant a better meat quality. C18:2n6c was reported to cause a negative flavor in meat (53). B. subtilis treatment had no effect on C18:2n6c content in both breast and thigh muscles, which meant that there was no adverse effect on meat flavor when broilers are supplemented with B. subtilis. In summary, B. subtilis could improve the composition of muscle fatty acids by decreasing the SFA content and increasing the UFA content, thus improving the meat quality as well as meat flavor.
Conclusions
In conclusion, dietary supplementation with B. subtilis could improve the carcass traits and meat quality of broilers by improving the pH and tenderness, decreasing the drip loss and cooking loss, and adjusting the composition of AAs and fatty acids.
Data Availability Statement
The original contributions presented in the study are included in the article/Supplementary Material, further inquiries can be directed to the corresponding author/s.
Ethics Statement
The experimental procedures involving animals were reviewed and approved by the Animal Welfare Committee of the Guizhou Normal University (Guiyang, China).
Author Contributions
XT and HL: conceptualization, investigation, resources, and methodology. XT and XL: formal analysis and data curation. XT: writing—original draft preparation, writing—review and editing, project administration, funding acquisition, and supervision. All authors have read and agreed to the published version of the manuscript.
Funding
This research was supported by the China Overseas Expertise Introduction Program for Discipline Innovation (No. D17016),the Key Project of Science and Technology Program of Guizhou Province (No. 5411 2017 Qiankehe Pingtai Rencai), the World Top Discipline Program of Guizhou Province (No. 125 2019 Qianjiao Keyan Fa), the Natural Science Research Project of Education Department of Guizhou Province[Qianjiaohe KY Zi (2021) 294], and the Doctoral Launched Scientific Research Program of Guizhou Normal University [GZNUD (2018) 26].
Conflict of Interest
The authors declare that the research was conducted in the absence of any commercial or financial relationships that could be construed as a potential conflict of interest.
Publisher's Note
All claims expressed in this article are solely those of the authors and do not necessarily represent those of their affiliated organizations, or those of the publisher, the editors and the reviewers. Any product that may be evaluated in this article, or claim that may be made by its manufacturer, is not guaranteed or endorsed by the publisher.
Acknowledgments
We thank Prof. Kangning Xiong for kindly revising the paper and financial support.
Supplementary Material
The Supplementary Material for this article can be found online at: https://www.frontiersin.org/articles/10.3389/fvets.2021.767802/full#supplementary-material
References
1. Chiesa LM, Nobile M, Panseri S, Arioli F. Antibiotic use in heavy pigs: comparison between urine and muscle samples from food chain animals analysed by HPLC-MS/MS. Food Chem. (2017) 235:111–8. doi: 10.1016/j.foodchem.2017.04.184
2. Li X, Wang L, Zhen Y, Li S, Xu Y. Chicken egg yolk antibodies (IgY) as non-antibiotic production enhancers for use in swine production: a review. J Anim Sci Biotechn. (2015) 6:40. doi: 10.1186/s40104-015-0038-8
3. Cai K, Shao W, Chen X, Campbell YL, Nair MN, Suman SP, et al. Meat quality traits and proteome profile of woody broiler breast (pectoralis major) meat. Poult Sci. (2018) 97:337–46. doi: 10.3382/ps/pex284
4. Zhang L, Zhang L, Zhan X, Zeng X, Zhou L, Cao G, et al. Effects of dietary supplementation of probiotic, clostridium butyricum, on growth performance, immune response, intestinal barrier function, and digestive enzyme activity in broiler chickens challenged with escherichia coli k88. J Anim Sci Biotechnol. (2016) 7:3. doi: 10.1186/s40104-016-0061-4
5. Hagihara M, Kuroki Y, Ariyoshi T, Higashi S, Fukuda K, Yamashita R, et al. Clostridium butyricum modulates the microbiome to protect intestinal barrier function in mice with antibiotic-induced dysbiosis. iScience. (2020) 23:100772. doi: 10.1016/j.isci.2019.100772
6. Alagawany M, Abd El-Hack ME, Farag MR, Sachan S, Karthik K, Dhama K. The use of probiotics as eco-friendly alternatives for antibiotics in poultry nutrition. Environ Sci Pollut Res Int. (2018) 25:10611–8. doi: 10.1007/s11356-018-1687-x
7. Gadde UD, Oh S, Lee Y, Davis E, Zimmerman N, Rehberger T, et al. Dietary bacillus subtilis-based direct-fed microbials alleviate LPS-induced intestinal immunological stress and improve intestinal barrier gene expression in commercial broiler chickens. Res Vet Sci. (2017) 114:236–43. doi: 10.1016/j.rvsc.2017.05.004
8. Dela Cruz PJD, Dagaas CT, Mangubat KMM, Angeles AA, Abanto OD. Dietary effects of commercial probiotics on growth performance, digestibility, and intestinal morphometry of broiler chickens. Trop Anim Health Prod. (2019) 51:1105–15. doi: 10.1007/s11250-018-01791-0
9. Upadhaya SD, Rudeaux F, Kim IH. Effects of inclusion of bacillus subtilis (Gallipro) to energy- and protein-reduced diet on growth performance, nutrient digestibility, and meat quality and gas emission in broilers. Poult Sci. (2019) 98:2169–78. doi: 10.3382/ps/pey573
10. Yu L, Peng Z, Dong L, Wang H, Shi S. Enterococcus faecium NCIMB 10415 supplementation improves the meat quality and antioxidant capacity of muscle of broilers. J Anim Physiol Anim Nutr. (2019) 103:1099–106. doi: 10.1111/jpn.13097
11. Cramer TA, Kim HW, Chao Y, Wang W, Cheng HW, Kim YHB. Effects of probiotic (Bacillus subtilis) supplementation on meat quality characteristics of breast muscle from broilers exposed to chronic heat stress. Poult Sci. (2018) 97:3358–68. doi: 10.3382/ps/pey176
12. Bai K, Huang Q, Zhang J, He J, Zhang L, Wang T. Supplemental effects of probiotic bacillus subtilis fmbJ on growth performance, antioxidant capacity, and meat quality of broiler chickens. Poult Sci. (2017) 96:74–82. doi: 10.3382/ps/pew246
13. Park JH, Kim IH. The effects of the supplementation of bacillus subtilis RX7 and B2A strains on the performance, blood profiles, intestinal salmonella concentration, noxious gas emission, organ weight and breast meat quality of broiler challenged with salmonella typhimurium. J Anim Physiol Anim Nutr. (2015) 99:326–34. doi: 10.1111/jpn.12248
14. Barbosa TM, Serra CR, La Ragione RM, Woodward MJ, Henriques AO. Screening for bacillus isolates in the broiler gastrointestinal tract. Appl Environ Microbiol. (2005) 71:968–78. doi: 10.1128/AEM.71.2.968-978.2005
15. Li J, Li W, Li J, Wang Z, Xiao D, Wang Y, et al. Screening of differentially expressed immune-related genes from spleen of broilers fed with probiotic bacillus cereus PAS38 based on suppression subtractive hybridization. PLoS ONE. (2019) 14:e0226829. doi: 10.1371/journal.pone.0226829
16. Zhou X, Wang Y, Gu Q, Li W. Effect of dietary probiotic, bacillus coagulans, on growth performance, chemical composition, and meat quality of Guangxi Yellow chicken. Poult Sci. (2010) 89:588–93. doi: 10.3382/ps.2009-00319
17. Sarao LK, Arora M. Probiotics, prebiotics, and microencapsulation: a review. Crit Rev Food Sci Nutr. (2017) 57:344–71. doi: 10.1080/10408398.2014.887055
18. Hong HA, Hong DL, Cutting SM. The use of bacterial spore formers as probiotics. FEMS Microbiol Rev. (2005) 29:813–35. doi: 10.1016/j.femsre.2004.12.001
19. Reis MP, Fassani EJ, Garcia Junior AAP, Rodrigues PB, Bertechini AG, et al. Effect of bacillus subtilis (DSM 17299) on performance, digestibility, intestine morphology, and pH in broiler chickens. J Appl Poult Res. (2017) 26:573–83. doi: 10.3382/japr/pfx032
20. Dowarah R, Verma AK, Agarwal N, Singh P, Singh BR. Selection and characterization of probiotic lactic acid bacteria and its impact on growth, nutrient digestibility, health and antioxidant status in weaned piglets. PLoS ONE. (2018) 13:e0192978. doi: 10.1371/journal.pone.0192978
21. Jeong JS, Kim IH. Effect of bacillus subtilis C-3102 spores as a probiotic feed supplement on growth performance, noxious gas emission, and intestinal microflora in broilers. Poult Sci. (2014) 93:3097–103. doi: 10.3382/ps.2014-04086
22. Jacquier V, Nelson A, Jlali M, Rhayat L, Brinch KS, Devillard E. Bacillus subtilis 29784 induces a shift in broiler gut microbiome toward butyrate-producing bacteria and improves intestinal histomorphology and animal performance. Poult Sci. (2019) 98:2548–54. doi: 10.3382/ps/pey602
23. Guo M, Li M, Zhang C, Zhang X, Wu Y. Dietary administration of the bacillus subtilisenhances immune responses and disease resistance in chickens. Front Microbiol. (2020) 11:1768. doi: 10.3389/fmicb.2020.01768
24. Park I, Zimmerman NP, Smith AH, Rehberger TG, Lillehoj EP, Lillehoj HS. Dietary supplementation with bacillus subtilis direct-fed microbials alters chicken intestinal metabolite levels. Front Vet Sci. (2020) 7:123. doi: 10.3389/fvets.2020.00123
25. Ma Y, Wang W, Zhang H, Wang J, Zhang W, Gao J, et al. Supplemental bacillus subtilis DSM 32315 manipulates intestinal structure and microbial composition in broiler chickens. Sci Rep. (2018) 8:15358. doi: 10.1038/s41598-018-33762-8
26. Sokale AO, Menconi A, Mathis GF, Lumpkins B, Sims MD, Whelan RA, et al. Effect of bacillus subtilis DSM 32315 on the intestinal structural integrity and growth performance of broiler chickens under necrotic enteritis challenge. Poult Sci. (2019) 98:5392–400. doi: 10.3382/ps/pez368
27. Whelan RA, Doranalli K, Rinttilä T, Vienola K, Jurgens G, Apajalahti J. The impact of bacillus subtilis DSM 32315 on the pathology, performance, and intestinal microbiome of broiler chickens in a necrotic enteritis challenge. Poult Sci. (2019) 98:3450–63. doi: 10.3382/ps/pey500
28. Bortoluzzi C, Serpa Vieira B, de Paula Dorigam JC, Menconi A, Sokale A, Doranalli K, et al. Bacillus subtilis DSM 32315 supplementation attenuates the effects of clostridium perfringenschallenge on the growth performance and intestinal microbiota of broiler chickens. Microorganisms. (2019) 7:71. doi: 10.3390/microorganisms7030071
29. Li CL, Wang J, Zhang HJ, Wu SG, Hui QR, Yang CB, et al Intestinal morphologic and microbiota responses to dietary bacillus spp. in a broiler chicken model. Front Physiol. (2019) 9:1968. doi: 10.3389/fphys.2018.01968
30. AOAC. Official Methods of Analysis. 17th ed. Gaithersburg, MD: Assoc. Off. Analysis Chemistry (2000).
31. Yadav M, Dubey M, Yadav M, Shankar KS. Effect of supplementation of probiotic (bacillus subtilis) on growth performance and carcass traits of broiler chickens. Int J Curr Microbiol App Sci. (2018) 7:4840–9. doi: 10.20546/ijcmas.2018.708.510
32. Rhayat L, Jacquier V, Brinch KS, Nielsen P, Nelson A, Geraert PA, et al. Bacillus subtilis strain specificity affects performance improvement in broilers. Poult Sci. (2017) 96:2274–80. doi: 10.3382/ps/pex018
33. Wan X, Yang Z, Ji H, Li N, Yang Z, Xu L, et al. Effects of lycopene on abdominal fat deposition, serum lipids levels and hepatic lipid metabolism-related enzymes in broiler chickens. Anim Biosci. (2021) 34:385–92. doi: 10.5713/ajas.20.0432
34. Chao X, Guo L, Wang Q, Huang W, Liu M, Luan K, et al. miR-429-3p/LPIN1 axis promotes chicken abdominal fat deposition via PPARγ pathway. Front Cell Dev Biol. (2020) 8:595637. doi: 10.3389/fcell.2020.595637
35. Na W, Wu YY, Gong PF, Wu CY, Cheng BH, Wang YX, et al. Embryonic transcriptome and proteome analyses on hepatic lipid metabolism in chickens divergently selected for abdominal fat content. BMC Genomics. (2018) 19:384. doi: 10.1186/s12864-018-4776-9
36. Zhang M, Liu L, Chen D, Zhang X, Zhou C, Gan Q, et al. Functional microRNA screening for dietary vitamin E regulation of abdominal fat deposition in broilers. Br Poult Sci. (2020) 61:344–349. doi: 10.1080/00071668.2020.1736265
37. Guo S, Zhang Y, Cheng Q, Xv J, Hou Y, Wu X, et al. Partial substitution of fermented soybean meal for soybean meal influences the carcass traits and meat quality of broiler chickens. Animals. (2020) 10:225. doi: 10.3390/ani10020225
38. Xu X, Li L, Li B, Guo W, Ding X, Xu F. Effect of fermented biogas residue on growth performance, serum biochemical parameters, and meat quality in pigs. Asian Australas J Anim Sci. (2017) 30:1464–70. doi: 10.5713/ajas.16.0777
39. Drazbo A, Kozłowski K, Ognik K, Zaworska A, Jankowski J. The effect of raw and fermented rapeseed cake on growth performance, carcass traits, and breast meat quality in turkey. Poult Sci. (2019) 98:6161–9. doi: 10.3382/ps/pez322
40. Chen H, Dong X, Yao Z, Xu B, Zhen S, Li C, et al. Effects of prechilling parameters on water-holding capacity of chilled pork and optimization af prechilling parameters using response surface methodology. J Anim Sci. (2012) 90:2836–41. doi: 10.2527/jas.2011-4239
41. Shen MM, Zhang LL, Chen YN, Zhang YY, Han HL, Niu Y, et al. Effects of bamboo leaf extract on growth performance, meat quality, and meat oxidative stability in broiler chickens. Poult Sci. (2019) 98:6787–96. doi: 10.3382/ps/pez404
42. Zhuang H, Savage EM, Smith DP, Berrang ME. Effect of dry-air chilling on warner-bratzler shear force and water- holding capacity of broiler breast meat deboned four hours postmortem. Int J Poult Sci. (2008) 7:743–8. doi: 10.3923/ijps.2008.743.748
43. Bai W K, Zhang FJ, He TJ, Su PW, Ying XZ, Zhang LL, et al. Dietary probiotic bacillus subtilis strain fmbj increases antioxidant capacity and oxidative stability of chicken breast meat during storage. PLoS ONE. (2016) 11:e0167339. doi: 10.1371/journal.pone.0167339
44. Abdulla NR, Zamri ANM, Sabow AB, Kareem KY, Nurhazirah S, Ling FH, et al. Physico-chemical properties of breast muscle in broiler chickens fed probiotics, antibiotics or antibiotic–probiotic mix. J Appl Anim Res. (2017) 45:64–70. doi: 10.1080/09712119.2015.1124330
45. Chartrin P, Méteau K, Juin H, Bernadet MD, Guy G, Larzul C, et al. Effects of intramuscular fat levels on sensory characteristics of duck breast meat. Poult Sci. (2006) 85:914–22. doi: 10.1093/ps/85.5.914
46. Lorenzo JM, Franco D. Fat effect on physico-chemical, microbial and textural changes through the manufactured of dry-cured foal sausage Lipolysis, proteolysis and sensory properties. Meat Sci. (2012) 92:704–14. doi: 10.1016/j.meatsci.2012.06.026
47. Chen Y, Qiao Y, Xiao Y, Chen H, Zhao L, Huang M, et al. Differences in physicochemical and nutritional properties of breast and thigh meat from crossbred chickens, commercial broilers, and spent hens. Asian Australas J Anim Sci. (2016) 29:855–64. doi: 10.5713/ajas.15.0840
48. Heyer A, Lebret B. Compensatory growth response in pigs: effects on growth performance, composition of weight gain at carcass and muscle levels, and meat quality. J Anim Sci. (2002) 85:769–78. doi: 10.2527/jas.2006-164
49. Wood JD, Richardson RI, Nute GR, Fisher AV, Campo MM, Kasapidou E, et al. Effects of fatty acids on meat quality: a review. Meat Sci. (2003) 66:21–32. doi: 10.1016/S0309-1740(03)00022-6
50. Amorim A, Rodrigues S, Pereira E, Teixeira A. Physicochemical composition and sensory quality evaluation of capon and rooster meat. Poult Sci. (2016), 95:1211–1219. doi: 10.3382/ps/pev448
51. Liu T, Wu J, Lei Z, Zhang M, Gong X, Cheng S, et al. Fatty acid profile of muscles from crossbred angus-simmental, wagyu-simmental, and chinese simmental cattles. Food Sci Anim Resour. (2020) 40:563–77. doi: 10.5851/kosfa.2020.e33
52. Zong G, Li Y, Wanders AJ, Alssema M, Zock PL, Willett WC, et al. Intake of individual saturated fatty acids and risk of coronary heart disease in US men and women: two prospective longitudinal cohort studies. BMJ. (2016) 355:i5796. doi: 10.1136/bmj.i5796
Keywords: Bacillus subtilis, broiler, carcass trait, meat quality, amino acid, fatty acid
Citation: Tang X, Liu X and Liu H (2021) Effects of Dietary Probiotic (Bacillus subtilis) Supplementation on Carcass Traits, Meat Quality, Amino Acid, and Fatty Acid Profile of Broiler Chickens. Front. Vet. Sci. 8:767802. doi: 10.3389/fvets.2021.767802
Received: 31 August 2021; Accepted: 22 October 2021;
Published: 22 November 2021.
Edited by:
Rita Payan Carreira, University of Evora, PortugalReviewed by:
Ayman Abdel-Aziz Swelum, Zagazig University, EgyptSamiru Sudharaka Wickramasuriya, Agricultural Research Service (USDA), United States
Copyright © 2021 Tang, Liu and Liu. This is an open-access article distributed under the terms of the Creative Commons Attribution License (CC BY). The use, distribution or reproduction in other forums is permitted, provided the original author(s) and the copyright owner(s) are credited and that the original publication in this journal is cited, in accordance with accepted academic practice. No use, distribution or reproduction is permitted which does not comply with these terms.
*Correspondence: Xiaopeng Tang, tangxiaopeng110@126.com orcid.org/0000-0002-4789-5687; Hu Liu, liuh2018@lzu.edu.cn orcid.org/0000-0002-7506-774X