- 1Key Laboratory of Land Surface Process and Climate Change in Cold and Arid Regions, Northwest Institute of Eco-Environment and Resources, Chinese Academy of Sciences, Lanzhou, China
- 2School of Geography, Liaoning Normal University, Dalian, China
Introduction: Light use efficiency (LUE) is a crucial determinant of plant productivity, while leaf functional traits directly affect ecosystem functions. However, it remains unclear how climate warming affects LUE and leaf functional traits of dominant species in alpine meadows.
Methods: We conducted a 4-year in-situ field warming experiment to investigate the eco-physiological characteristics for a dominant species (Elymus nutans) and a common species (Potentilla anserina) on the Tibetan Plateau. The leaf traits, photosynthesis and fluorescence characteristics were measured, along with the soil physical-chemical properties associated with the two species.
Results and discussions: Experimental warming increased the leaf LUE, maximum photochemical efficiency, non-photochemical quenching, relative water content and specific leaf area for both species. However, there was a decrease in leaf and soil element content. Different species exhibit varying adaptability to warming. Increasing temperature significantly increased the photosynthetic rate, stomatal conductance, transpiration rate, total water content, and specific leaf volume of E. nutans; however, all these traits exhibited an opposite trend in P. anserina. Warming has a direct negative impact on leaf LUE and an indirectly enhances LUE through its effects on leaf traits. The impact of warming on plant photosynthetic capacity is primarily mediated by soil nutrients and leaf traits. These results indicate that the two different species employ distinct adaptive strategies in response to climate change, which are related to their species-specific variations. Such changes can confer an adaptive advantage for plant to cope with environmental change and potentially lead to alterations to ecosystem structure and functioning.
1 Introduction
The global surface temperature has risen by approximately 1.09°C since the 1850s (Masson-Delmotte et al., 2021). Climate warming is recognized as a significant driver of global change and poses a substantial threat to ecological integrity and function (Hughes et al., 2018). In the alpine ecosystem, the temperature increase has been twice as high as the global average, with a rate of 0.3-0.4°C per decade, and this trend becomes more pronounced with increasing altitude (Fu et al., 2021). Temperature is widely recognized as a major limiting factor in alpine ecosystems, and alpine vegetation exhibits a high sensitivity to temperature (Duan et al., 2019). While warming partially meets the heat requirements of plants, it also alters the micro-climate environment of plant communities, directly or indirectly impacting the photosynthetic physiological processes and consequently influencing plant growth and development (Lee et al., 2020).
Plant physiological processes are extremely sensitive to temperature, and even slight temperature fluctuations leading to significant modifications in these processes (Atkin et al., 2005). Leaf photosynthetic properties can reflect plant responses to environmental changes and play a crucial role in plant growth, thereby impacting the structure and function of ecosystems (Zhang et al., 2022). Recent research indicates that gross primary productivity is affected by photosynthesis, rather than canopy structure (Li et al., 2024). Light use efficiency (LUE) is a crucial indicator of a plant’s ability to convert absorbed light energy into chemical energy through photosynthesis (Medlyn, 1998). The strong solar radiation and long-term sunshine in the alpine region are conducive to the photosynthesis and LUE of alpine plants. However, low temperatures, significant temperature variations, short growth periods, and other factors impose limitations on plant growth and photosynthetic capacity. Warming can increase air and soil temperature, leading to a reduction in soil moisture, which in turn affects plant photosynthetic capacity and LUE (Forkel et al., 2016; Allison et al., 2018). Warming also caused a shift in plant functional traits, leading to an increase in acquisitive characteristics such as larger leaves, higher photosynthetic resource-use efficiency, thinner roots, and greater specific root length and nutrient concentrations (Wei et al., 2023). Yao et al. (2023) observed inconsistent responses in the net photosynthetic rate (Pn) of sedge, grass, and shrubs under future climate scenarios. Notably, grass exhibited the least sensitivity to future temperature and CO2. Some studies have reported generally positive effects of climate change on plant photosynthesis, but there have also been reports of insignificant or even negative effects, which vary depending on the species-specific characteristics (Jassey and Signarbieux, 2019; Meng et al., 2023). However, it remains unclear how plant physiology will change as temperatures rise and how these changes will affect their LUE. To comprehend natural processes and ensure the long-term sustainability of alpine meadow development, it is essential to understand how physiological traits and photosynthetic capacity of alpine plants respond to climate warming.
In order to improve their survival fitness and competitive ability, plants modify their morphological and physiological characteristics in response to environmental changes and interactions with other organisms (Wang et al., 2021). A growing number of studies have demonstrated that leaf traits, such as leaf lifespan, leaf area, and leaf nutrient content, are highly sensitive to climate warming (Buzzard et al., 2019; Bjorkman et al., 2020). In both the alpine meadow and swamp ecosystems, Guittar et al. (2016) proposed that warming led to an increase in specific leaf area (SLA) for two dominant species, E. nutans and C. scabrirostris. The response of plants to climate change is also reflected in the trade-offs between resource availability and utilization. With increasing temperatures, a significant increase (Bai et al., 2013; Šímová et al., 2017) or decrease (Shen et al., 2022; Storkey and Macdonald, 2022) in leaf nitrogen content was observed. Leaf nitrogen content is consistently positively correlated with plant photosynthetic capacity due to the presence of photosynthetic enzymes and chlorophyll, which constitute a major portion of leaf nitrogen (Fu et al., 2015). The correlations between leaf traits and photosynthetic carbon assimilation are commonly employed for estimating primary production at various scales, ranging from individual leaves to global levels (Feng and Dietze, 2013).
Plant nutrient allocation reflects how plants respond to environmental changes, and the availability of soil nutrients determines the spatial and temporal patterns of leaf traits as well as plant resource utilization (Huang et al., 2021). Previous studies have indicated a close relationship between structural allocation trait (such as leaf area) and the functions of photosynthetic carbon capture, belowground nutrient acquisition, and resource transport (Kleyer et al., 2019). Changes in soil nutrients under climate warming can affect plant photosynthesis and physiological characteristics, which may further influence species composition by altering plant facilitation and competitive exclusion (Xu et al., 2022). According to Yang et al. (2022), experimental warming did not alter the content of soil organic carbon (SOC) during the growing season in an alpine meadow. Wei et al. (2023) demonstrated that plants exhibit consistent adaptive strategies in both above- and belowground traits, favoring more acquisitive traits in warmer environments. These changes could confer an adaptive advantage to plants in response to environmental change. Currently, the trade-offs between plant leaf traits and soil nutrients under climate change remain unclear, as well as the key factors that can have a greater impact on plant LUE.
Alpine meadows, as one of the typical grassland ecosystem types on the Qinghai-Tibetan Plateau (QTP), are highly sensitive to climate change (Immerzeel et al., 2020). A previous study demonstrated that the alpine meadow is becoming increasingly susceptible to the direct impacts of climate extremes, which affect ecosystem function and phenology by altering key traits of plant species (Knapp et al., 2020). The impact of global warming led to alterations in plant growth and soil nutrients in the alpine meadows. Due to low temperatures, significant diurnal temperature fluctuations, and short growth periods experienced by alpine plants, their LUE and productivity are generally very low. Therefore, gaining a better understanding of how alpine plants physiologically respond to environmental changes will greatly enhance predictions for vegetation productivity under climate change. In this study, we conducted warming experiment to investigate the effects of short-term (one year) and medium-term (four-year) warming on two alpine species in the eastern QTP. Our hypothesis was that warming could induce changes in leaf traits, chlorophyll fluorescence, and soil nutrients, thereby altering the photosynthetic potential (photosynthesis and LUE) of these plants. The specific objectives of our study were to: 1) investigate the differential impacts of short-term and medium-term warming on the physiological performances of two alpine plants; 2) elucidate the key processes that contribute to changes in leaf photosynthetic capacity under warming conditions. This research is essential for enhancing our comprehension of the species-specific differences and the potential adaptation mechanisms employed by alpine plants in response to global warming.
2 Materials and methods
2.1 Study site
The research was carried out at the Zoige Alpine Wetland Ecosystem Research Station (3440 m, 33°51′52″N, 102°08′46″E) in the eastern Tibetan Plateau. The area has a typical plateau continental semi-humid climate with no frost-free period throughout the year. The annual average air temperature is 1.1°C, and there is around 600 mm of precipitation per year, mostly during the growing season from June to September.
Based on the categorization by the US Department of Agriculture, the soil type in the research area was identified as silt clay loam, consisting of 31.2% sand, 56.0% silt, and 12.8% clay in the top 30 cm of soil. Elymus nutans and Kobresia setschwanensis are the dominant species in the study area, while other associated species include Potentilla anserina, Roegneria nutans, Poa pratensis, Plantago depressa, Leymus secalinus, and Ajania tenuifolia, etc. We choose E. nutans and P. anserina as the focal species for investigating the impacts of warming on alpine plants. The two species representing two major plant functional groups (grasses and forbs) and naturally co-exist within our study site. Elymus nutans is the dominant perennial grass species in the alpine meadow. Potentilla anserina is a common companion species and is widely distributed in alpine meadows. Due to its wide ecological amplitude and vegetative reproduction ability, P. anserina is considered a prime species for ecological restoration in alpine regions (Wu et al., 2022). Our field investigation at the study site found that E. nutans and P. anserina together account for nearly 60% of the total vegetation coverage. E. nutans and P. anserina display distinct stratified structures. E. nutans predominantly occupies the upper part of the community and grow in full-sun conditions, while the leaves of P. anserina were restricted to the lower parts of the community close to soil surface. Consequently, they display distinct resource utilization patterns, particularly in terms of light and soil nutrients (Zhou et al., 2021). The upper leaf layer within the canopy typically absorbs light beyond its saturation point and dissipates excess energy through heat dissipation mechanisms. In contrast, lower layer leaves often face limitations due to insufficient available light.
2.2 Experimental design
In April 2015, we launched our in-situ warming experiment using open-top chambers (OTCs) to assess the impacts of warming on the alpine meadow ecosystem. The OTC had a 6.4 m2 surface area, a height of 2 m, a bottom side length of 1.15 m, and a regular octagon form with an outside diameter of 3 m. Open areas (OAs) were developed as control regions with characteristics comparable to those of the OTCs. The detailed layout of the experimental design was mentioned in our previous articles (Zhou et al., 2021).
2.3 Environmental factors measurement
The ambient temperature (Ta, °C) and relative humidity (RH, %) were measured every 30 minutes by HOBO (U23-002, Pocasset, MA, USA), which was placed in the middle of the OTCs and OAs at a height of 1.5 m above the soil surface. Using an ECH2O-TE sensor and EM50 data collecting system (Decagon Devices, Inc., USA), we automatically monitored soil temperature and soil moisture (volumetric soil moisture, V/V%) at a depth of 5 cm with 30-minute intervals during the experiment. Following four years of warming, the air temperature consistently increased year over year. In 2015–2018, the daily mean air temperature in the OTCs was 0.65°C, 0.74°C, 0.75°C, and 0.75°C higher than that in the OAs respectively. The average soil temperatures over the four-year period were recorded as 14.0°C in the OTCs and 13.2°C in the OAs. Warming led to a decline in soil moisture, with the OTCs experiencing reductions of 12.5%, 13.4%, 16.7%, and 10.1% during the growing seasons of 2015-2018. The lowest amount of precipitation during the growing season was recorded in 2015 (435 mm), while the highest amount was recorded in 2018 (669 mm) (see details in Supplementary Figure S1).
2.4 Gas exchange and chlorophyll fluorescence measurement
The gas exchange properties were measured using a portable photosynthetic system (LI-6400, LI-COR, Lincoln, USA). The experiments were conducted during the vigorous growth period (mid-July and mid-August) in each of the years spanning from 2015 to 2018. For comparative analysis, we selected 2015 (representing short-term warming) and 2018 (representing medium-term warming). The measurements were typically taken three times per month on clear days. Fully expanded and exposed leaves were selected, and measurements were taken from 09:00 to 13:00 with local time (which is 72 minutes later than Beijing time). For each replication, three individuals in a similar healthy state were selected. The following parameters were measured: net photosynthetic rate (Pn), transpiration rate (Tr), stomatal conductance (gs), stomatal limitation (Ls), and intercellular CO2 concentration (Ci). After that, the leaves were collected and their areas were scanned and precisely calculated using Image J software (version 1.47v, USA).
Leaf light use efficiency (LUE, mmol·CO2·mol-1·photons) was calculated based on the Equation 1:
Where PARi represents the incident photosynthetic active radiation (μmol·m–2·s–1).
A portable modulated chlorophyll fluorometer was used to assess the chlorophyll fluorescence characteristics immediately following photosynthesis measurements in the same plant (PAM-2100, Walz, Germany). The leaves were pre-adapted in the dark for 30 minutes before being measured hourly from 09:00-13:00 h. The maximal photochemical efficiency of PS II (Fv/Fm), photochemical quenching (qP), non-photochemical quenching (qN), and effective photochemical efficiency (Yield) of the chlorophyll fluorescence parameters were measured.
2.5 Leaf traits measurement
Fresh leaf samples were collected from the sunny side of each species, immediately weighed (fresh weight, FW), and then submerged in distilled water in the dark until saturated. After determining the saturated fresh weight (SW), the leaves were oven-dried at 70°C for 48 hours to estimate the dry weight (DW). The Equation 2 was used to obtain the relative water content (RWC, %) of the leaf:
The leaf area of the fresh leaves was measured using Image J, and specific leaf area (SLA, cm2·g-1) was calculated as the ratio of one side’s area of each leaf in each set to its dry mass (Perez-Harguindeguy et al., 2013). The drainage method was used to compute the specific leaf volume (SLV, cm3·g-1), which is the ratio of leaf volume to dry mass. Leaf dry matter content (LDMC, mg·g-1) was computed as the ratio of leaf dry weight to leaf saturated fresh weight. In mid-July and mid-August, three repetitions of measurements were conducted for each leaf trait index of the two species.
2.6 Leaf stoichiometry
After sampling, the leaves were mixed to form composite samples from ten to fifteen different individuals. The materials were crushed, sieved through an 80-mesh screen, and then dried at 70°C for 24 hours before being packed in plastic bags for measurement. A Vario Macro Cube Elemental analyzer (Elementar, Hanau, Germany) was used to assess total contents of carbon and nitrogen. Total phosphorus contents were measured using a molybdenum antimony resistance colorimetric method. For each species, the measurements were repeated three times.
2.7 Soil respiration and soil nutrients
The LI-8100 automatic soil CO2 flux system (LI-COR, Lincoln, USA) and its 20 cm survey chamber (8100-103) were used to assess soil respiration (Rs). The soil collars (8100-103) were positioned with their tops 2-3 cm above the soil surface and installed one day before the measurements. The litter in the collar was cleared before each measurement, and the above-ground of the plant was subtracted.
After 24 hours of equilibration, the soil respiration rate (Rs, μmol·m-2·s-1) returned to its initial level before collar insertion. Measurements were taken once per hour for two minutes between 9:00 to 13:00 with three repetitions. The Rs was computed based on the Equation 3:
where SC is the area of soil that the survey chamber covers (0.03 m2). VC (m3) is computed as the sum of the volume of the 20 cm survey chamber (4.82×10-3 m3) and the product of the chamber offset (the distance from the settled collar to the ground inside it) and the soil area (SC), which represents the total volume of soil respiration system. is the rate of change in chamber CO2 during soil respiration measurements (μmol CO2 mol-1 s-1), where P is the atmospheric pressure (Pa), T is chamber air temperature (°C), and R is the gas constant (8.314 Pa m3 mol-1 K-1).
The potassium dichromate oxidation titration was used to measure the content of soil organic carbon (SOC). The semi-trace Kjeldahl technique was used to measure the soil total nitrogen (TN), and the vanadium molybdate blue colorimetric method was employed to determine the soil total phosphorus (TP) (Xu et al., 2022). Alkaline hydrolysis was used to estimate soil available nitrogen (AN), and the molybdenum blue technique was employed to evaluate soil available phosphorus (AP) after extracting soil samples with sodium bicarbonate. After extraction with ammonium acetate, soil available potassium (AK) was measured using a flame photometric method. Using an autoanalyzer (SmartChem140, AMS Alliance, Italy), the content of soil NH4+-N was determined in extracts of 2 M KCl (1:4, soil: extractant).
2.8 Statistical analysis
The data was statistically analyzed using SPSS 20.0 (SPSS Inc., Chicago, USA), and the results were presented as means ± standard error (SE). Two-way ANOVA analysis was used to compare the photosynthetic physiological characteristics of two different species under varying warming treatments and years, followed by post hoc Duncan multiple comparison for further analysis. Principal component analysis (PCA) was employed to compare the variance in leaf traits among species and treatments over the course of four years. The plspm package in structural equation modeling (SEM) was utilized to investigate the influence of leaf functional traits and environmental factors on leaf photosynthetic capacity. The analyses were conducted using R program v3.4.4, and figures were generated with Origin Pro 2021 (OriginLab Corporation, United States).
3 Results
3.1 Leaf physiological and biochemical characteristics
3.1.1 Leaf photosynthesis and light use efficiency
Different warming years had significant impacts on the photosynthesis rate (Pn) and transpiration rate (Tr) of both species. Specifically, short-term warming in 2015 resulted in decreased Pn and Tr for E. nutans, while increasing Pn and Tr for P. anserina. Conversely, medium-term warming in 2018 led to increased Pn and Tr for E. nutans but decreased for P. anserina (Figures 1A, 2B). Significant differences in Pn and Tr were observed between the two species. When comparing the Pn of both species in 2015 and 2018 under warming conditions, it was found that the Pn of both species decreased with the prolonged duration of warming.
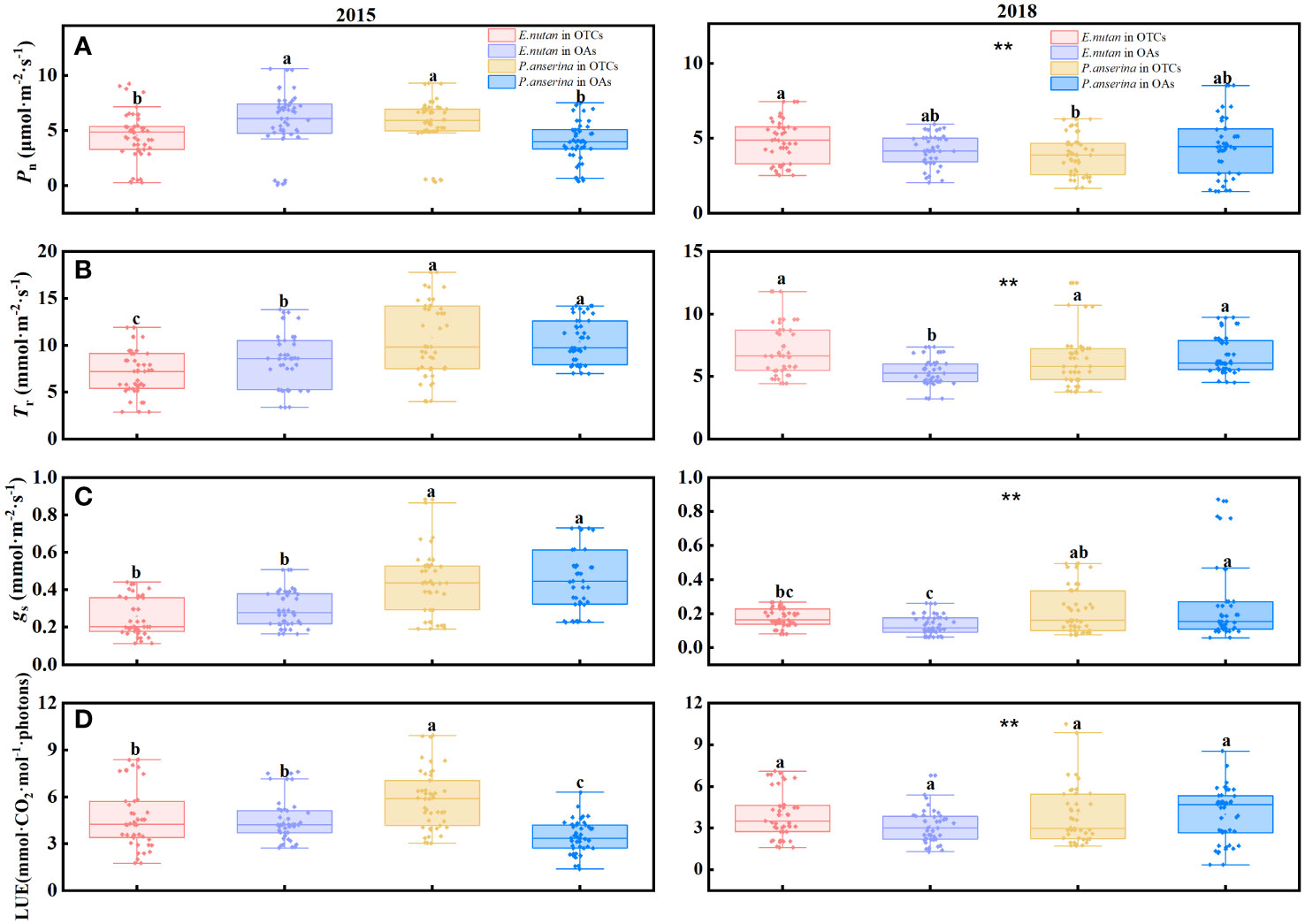
Figure 1 Effects of warming on photosynthesis and light use efficiency of E nutans and P. anserina in different years. The values are presented as means ± SE, with different letters indicating significant differences among species in the same year under warming at p< 0.05. ** indicates significant differences between different years. (A) net photosynthetic rate, (B) transpiration rate, (C) stomatal conductance, (D) light use efficiency. (The comparison of differences between species, treatments and years is shown in Supplementary Table S1).
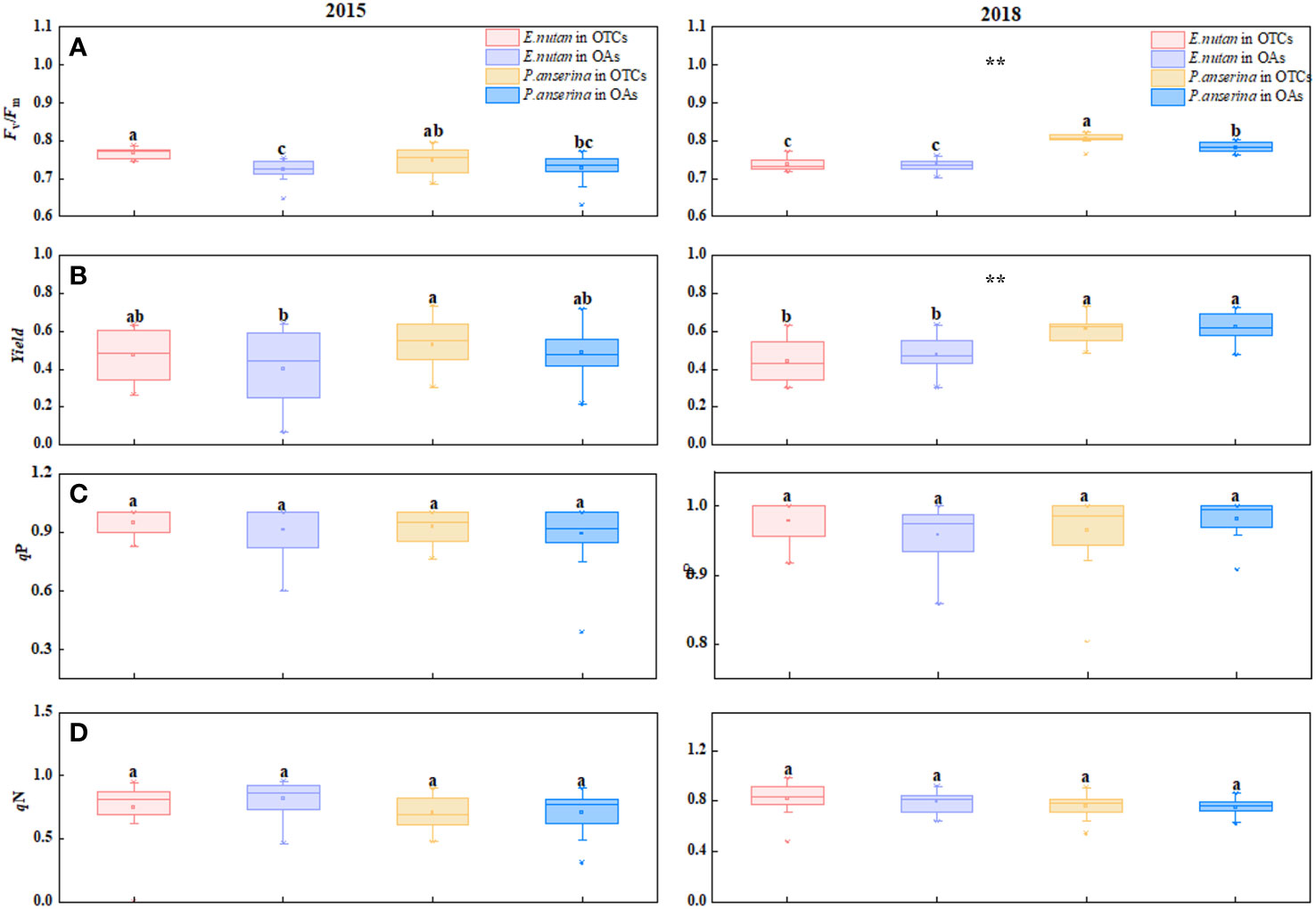
Figure 2 Effect of warming on chlorophyll fluorescence characteristics of E nutans and P. anserina in different years. The values are presented as means ± SE, with different letters indicating significant differences among species in the same year under warming at p< 0.05. ** indicates significant differences between different years. (A) maximum photochemical efficiency of PSII (Fv/Fm), (B) effective photochemical efficiency (yield), (C) photochemical quenching (qP), (D) non-photochemical quenching (qN). (The comparison of differences between species, treatments and years is shown in Supplementary Table S1).
In short and medium-term warming, the stomatal conductance (gs) of P. anserina was significantly higher than that of E. nutans (Figure 1C). There were significant differences in gs between E. nutans and P. anserina across different years of warming. In 2015, both E. nutans and P. anserina experienced a reduction in gs due to warming, while in 2018, the gs of E. nutans increased while that of P. anserina decreased.
The LUE of E. nutans and P. anserina exhibited an increase with warming in the short and medium-terms (Figure 1D). In 2015, the LUE of E. nutans was measured at 4.64 and 4.49 mmol·CO2·mol-1·photons in the OTCs and OAs, respectively, while the LUE of P. anserina was recorded as 5.80 and 3.43 mmol·CO2·mol-1·photons in the OTCs and OAs. In 2018, warming increased the LUE of both E. nutans and P. anserina. However, there was no statistically significant difference in LUE between the two species (p > 0.05).
3.1.2 Chlorophyll fluorescence characteristics
In both 2015 and 2018, warming increased the Fv/Fm values of both species (Figure 2A). In 2015, the Fv/Fm values of E. nutans were 0.776 and 0.723 in OTCs and OAs, respectively, showing a statistically significant difference (p< 0.05). The Fv/Fm values of P. anserina also increased with warming, but the difference was not statistically significant (p > 0.05). In 2018, the Fv/Fm value of P. anserina was higher than that of E. nutans. The yield values of the two species exhibited opposite trends under short-term and medium-term warming, with P. anserina having a higher yield than E. nutans, and the yield values of the two plants exhibited significant differences (Figure 2B). Under short-term warming, the yield of E. nutans and P. anserina increased, while in medium-term warming, the yield of both species decreased.
The qP values of the two species exhibited significant differences across different warming years (p< 0.05). In 2015, warming increased the qP values of both species, with E. nutans exhibiting a higher value compared to P. anserina (Figure 2C). In 2018, warming increased the qP values of E. nutans and decreased those of P. anserina, but the difference was not statistically significant (p > 0.05). Short-term warming (2015) reduced the qN values of both E. nutans and P. anserina, while medium-term warming increased them (Figure 2D).
3.1.3 Leaf physiological traits
Regarding the evaluated leaf physiological parameters, E. nutans and P. anserina responded differentially to in-situ warming (Table 1). E. nutans and P. anserina had significant differences in the contents of TWC, SLA, SLV, and LDMC under different warming treatments (p< 0.05). During the vigorous growth periods (July and August) in 2015, there were significant changes in the leaf physiological parameters of P. anserina, while those of E. nutans remained relatively stable. Warming increased the contents of TWC, SLA, and SLV in E. nutans, and reduced the contents of TWC, RWC, SLA, and SLV in P. anserina. Different trends were observed in E. nutans and P. anserina under medium-term warming (2018). The TWC and SLV contents of E. nutans increased under in-situ warming, while decreased in P. anserina. Meanwhile, the RWC and SLA contents of E. nutans and P. anserina both increased. The content of LDMC decreased in E. nutans and increased in P. anserina in the OTCs.
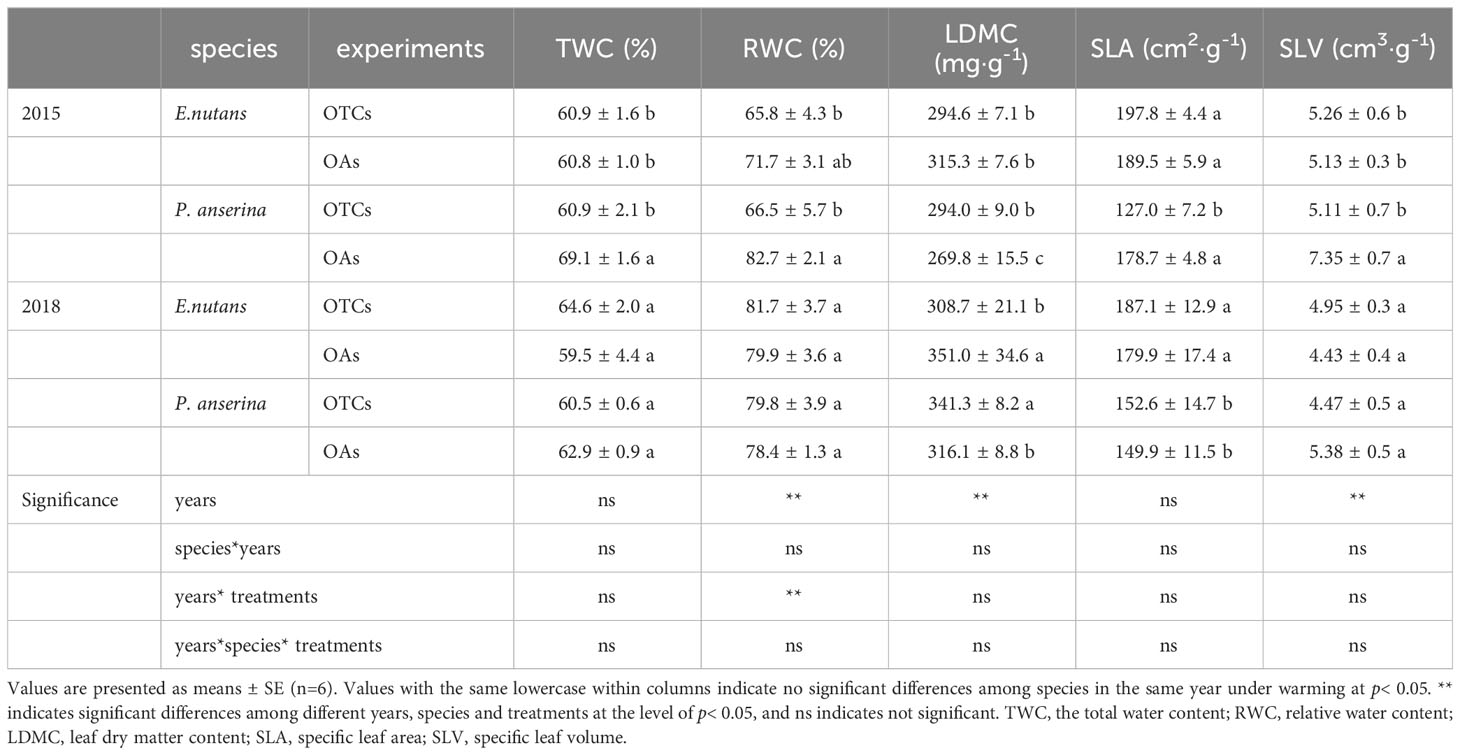
Table 1 Changes in leaf traits of E. nutans and P. anserina under simulated warming in different years.
3.1.4 Leaf element content
In both 2015 and 2018, P. anserina exhibited a higher total carbon (C) content compared to E. nutans, and there are significant differences between both two species (p< 0.05). In 2015, warming led to a decrease in the total carbon content of both species; however, in 2018, there was an increase in the total carbon content of P. anserina and a decrease in that of E. nutans (Figure 3A). Short-term warming decreased the total nitrogen (N) content of E. nutans while increased the total N content of P. anserina; however, the total N content of E. nutans was higher than that of P. anserina (Figure 3B). The total N content of the two species decreased under medium-term warming. The total phosphorus (P) content of the two species decreased significantly due to warming in the short and medium-terms (Figure 3C), while the leaf N:P ratio showed an opposite trend. The N:P ratios exhibited significant variations between the two species exposed to different warming treatments (Figure 3D). Under different treatments of short and medium-term warming, the N:P ratio of the two species experienced a remarkable increase. In 2015, the N:P ratios of E. nutans were 17.04 in OTCs and 14.42 in OAs, respectively, while those of P. anserina were 15.54 in OTCs and 10.46 in OAs. In 2018, the N:P ratios of E. nutans were 18.30 in OTCs and 13.75 in OAs, respectively, while those of P. anserina were 15.23 in OTCs and 12.57 in OAs.
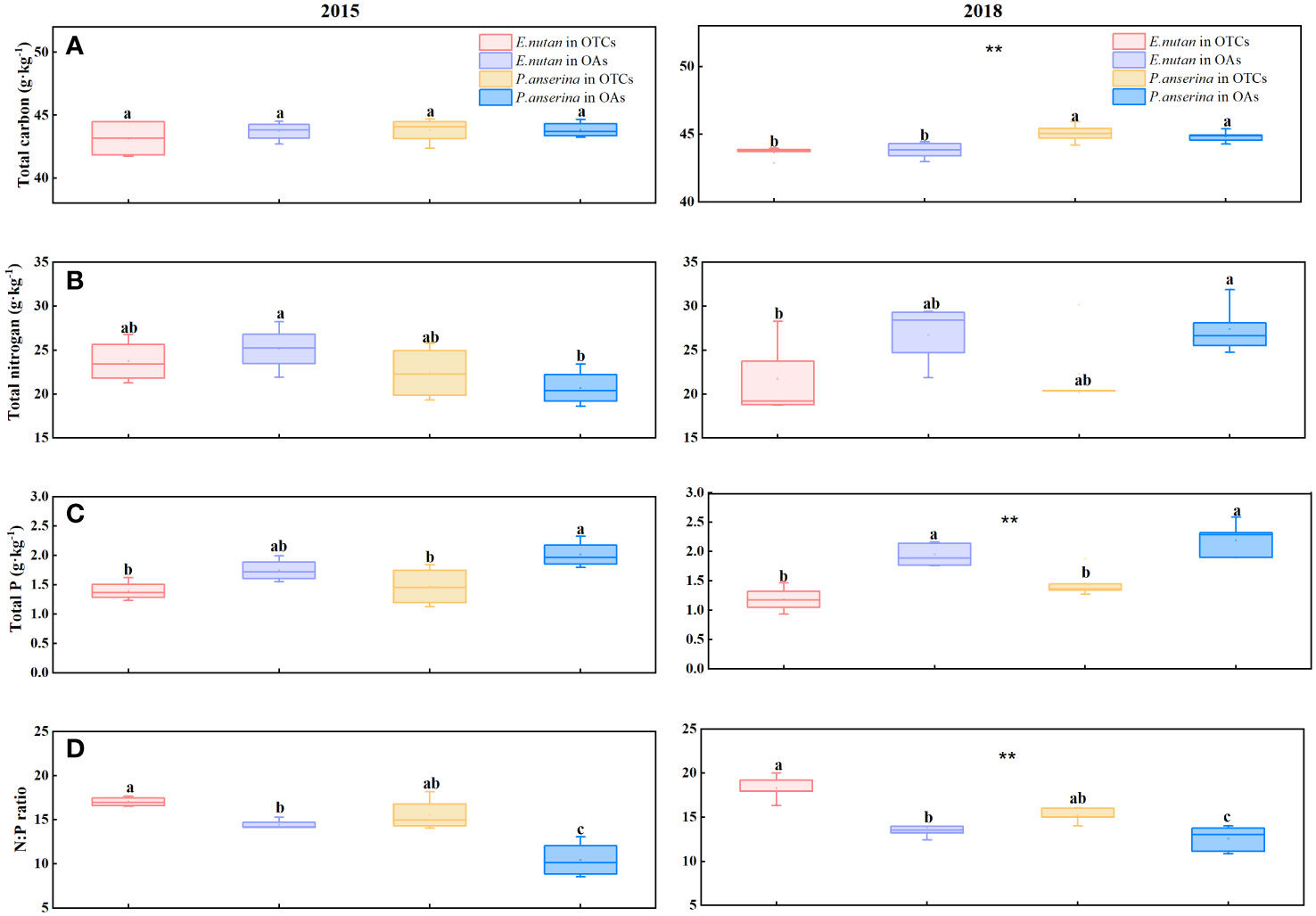
Figure 3 Effects of warming on the element content in E. nutans and P. anserina in different years. The values are presented as means ± SE, with different letters indicating significant differences among species in the same year under warming at p< 0.05. ** indicates significant differences between different years. (A) total carbon content, (B) total nitrogen content, (C) total phosphorus contents, (D) the N:P ratios. (The comparison of differences between species, treatments and years is shown in Supplementary Table S1).
3.2 Soil physicochemical properties
3.2.1 Soil respiration
It has been observed that the gradual increase in temperature has led to a slight rise in soil respiration (Rs) (Figure 4). In 2015, the Rs of OTCs and OAs were recorded as 1.06 and 0.97 μmol·m-2·s-1 respectively, while in 2018, the Rs of OTCs and OAs increased to 1.46 and 1.39 μmol·m-2·s-1 respectively. The impact of warming on Rs varied significantly across different years (p< 0.05), but there was minimal disparity between the various treatments (OTCs vs. OAs, p > 0.05).
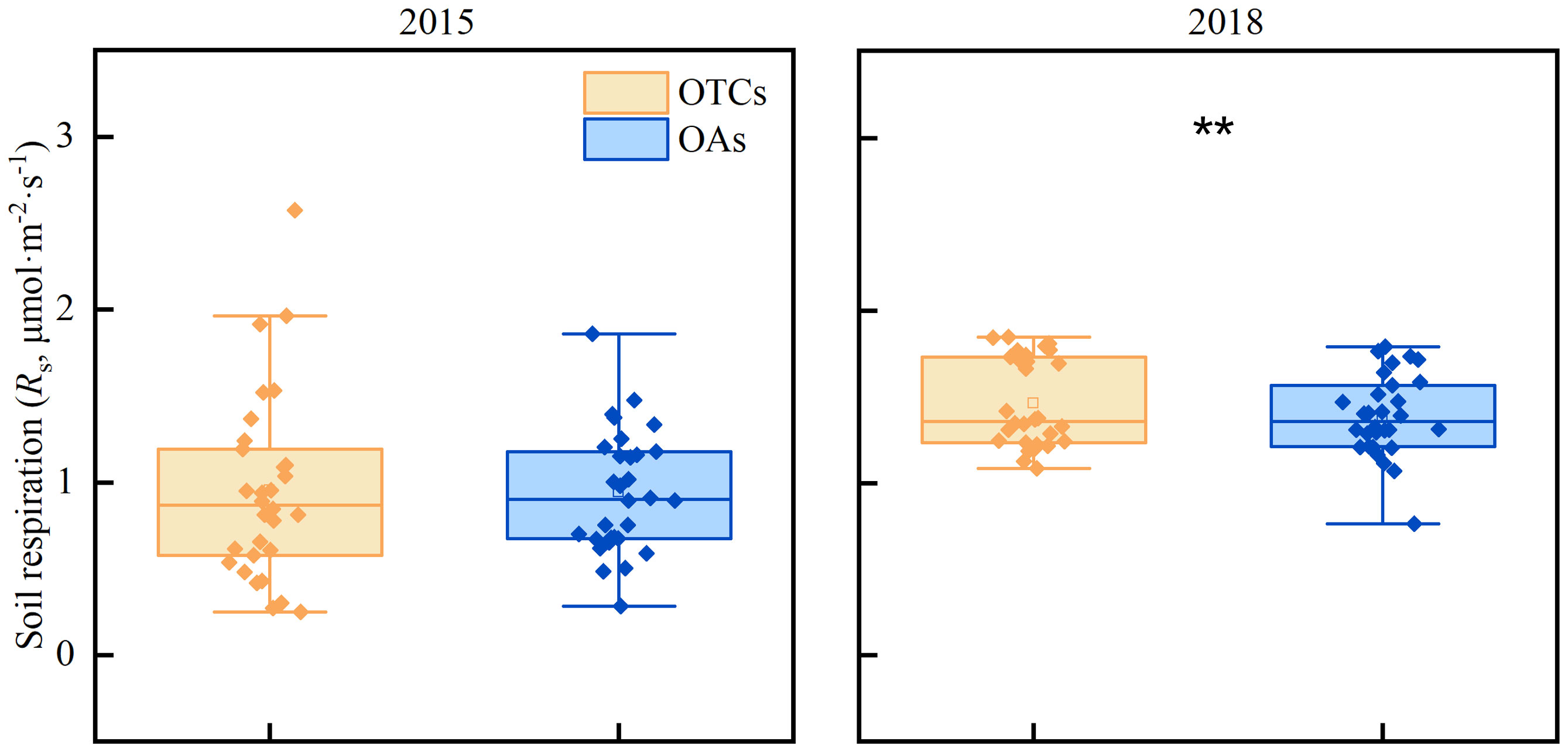
Figure 4 The effect of in-situ warming on soil respiration (Rs) in 2015 and 2018. ** indicates significant differences among different years at the level of p< 0.05.
3.2.2 Soil element content
Short-term and medium-term warming led to a decrease in the content of soil organic carbon (SOC), soil total nitrogen, soil total phosphorus, soil available phosphorus, soil available potassium, and soil NH4+-N (Table 2). In the short-term warming, there was a significant decline in soil available potassium and NH4+-N contents, while in the medium-term warming, there was a significant decrease in soil total phosphorus, and available phosphorus contents (p< 0.05). The total C content increased significantly in both 2015 and 2018 (p< 0.05).
3.3 Main factors affect leaf photosynthetic capacity under warming
The data representing the interaction pathways between soil nutrients, atmospheric CO2, leaf traits, and leaf photosynthetic capability in response to warming was adequately fit by structural equation models (SEM). It can be seen that soil nutrients have significant effects on leaf traits both in OTCs and OAs. The SEM analysis revealed that warming impacts leaf photosynthetic capability indirectly through soil nutrients, atmospheric CO2, and leaf traits in both OAs and OTCs. Firstly, the temperature had a significant effect on atmospheric CO2 and leaf traits in OTCs but not in OAs. Secondly, the photosynthetic capability of OTCs was more affected by temperature variations than that of OAs. Thirdly, the effects of temperature variation on leaf traits were greater in OTCs than in OAs. Furthermore, the correlation between leaf traits and photosynthetic capacity increased in OTCs, while the correlation between soil nutrients and photosynthetic capacity decreased (Figure 5). Finally, atmospheric CO2 had a significant effect on leaf photosynthetic capacity in OAs but not in OTCs, indicating that the effect of atmospheric CO2 on leaf photosynthetic capacity decreased with increasing temperature.
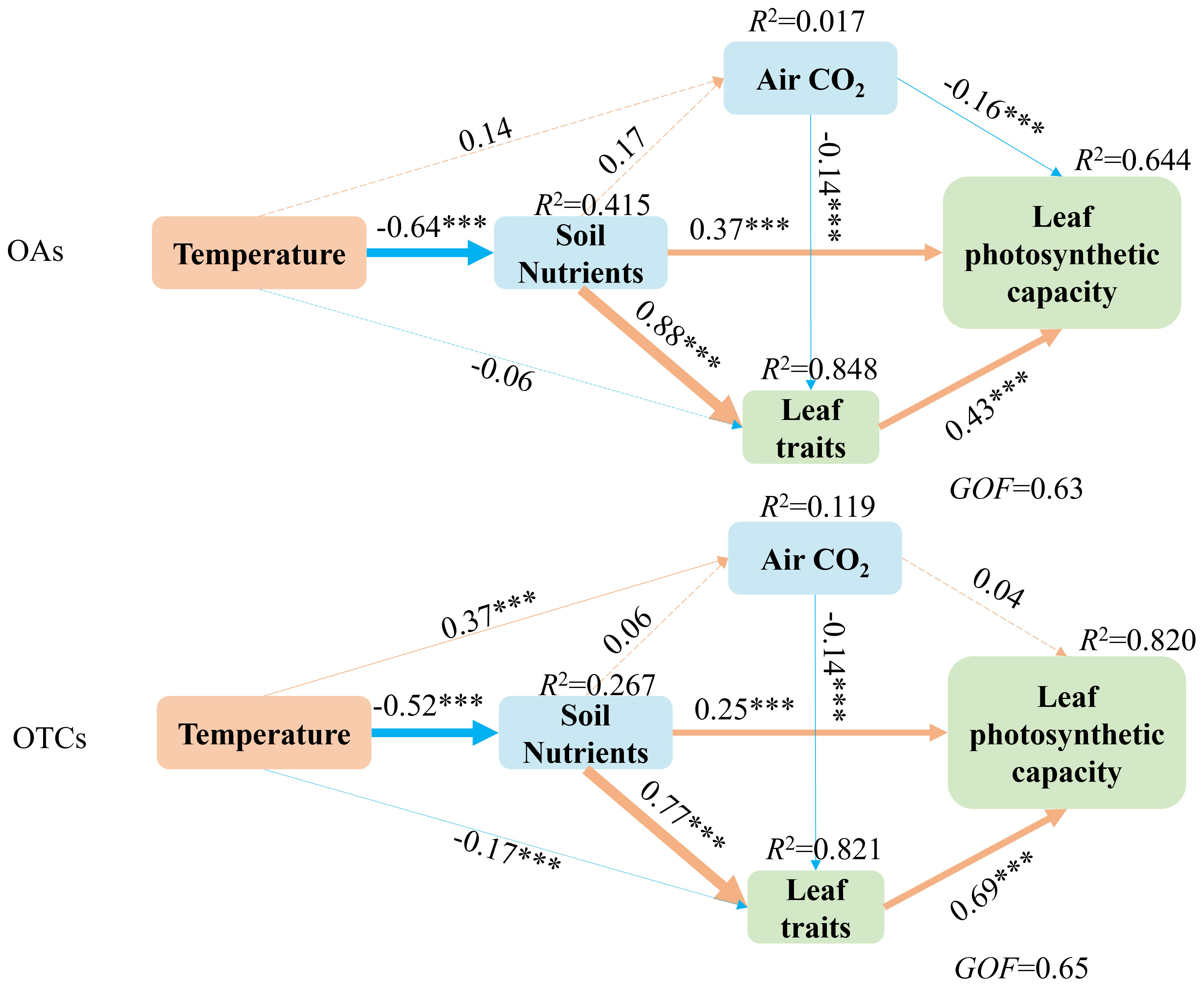
Figure 5 Structural equation models reveal direct and indirect influences of soil nutrients, atmospheric CO2, leaf traits, and leaf photosynthetic capacity on warming. Single-arrowed pathways indicate the directional effect between variables. The values associated with pathways are the standardized path coefficients. The R2-values are provided for soil nutrients, atmospheric CO2, leaf traits, and leaf photosynthetic capacity to indicate the variance explained by the model (R2). The width of the arrows indicates the strength of the relationships. Orange arrows indicate significant positive relationships, while blue arrows indicate significant negative relationships. The numbers on the line represent standardized path coefficients, and stars indicate significant correlations. ***p< 0.001.
A PCA-Biplot was used to compare the variance in leaf traits among different species and treatments over a period of four years. The first and second PC axes explained 27.3% and 20.1%, respectively. For the two species, E. nutans showed a strong correlation with Ls, SLA, RWC, TN, TC, NP, qN, Yield, Fv/Fm and LDMC. P. anserina exhibited a strong correlation with Pn, Gs, Tr, LUE, SLV, TWC and TP (see the Supplementary Figure S2A). The warming treatment (OTCs) had a significant impact on the photosynthetic capability (photosynthesis and fluorescence) of species (see the Supplementary Figure S2B).
4 Discussion
4.1 Responses of leaf photosynthetic and LUE to climate warming
Alpine plants are largely restricted by low temperatures, and warming might directly reduce the impact of low temperatures on plant growth, even change the community structure and species composition of alpine meadows (Li et al., 2018; Zhou et al., 2021). Photosynthesis can reflect the physiological adaptability of plants under specific environmental conditions. Several studies suggest that alpine plants exhibit higher photosynthetic capacities and leaf nitrogen concentrations compared to the global average (Wright et al., 2004; He et al., 2006). The majority research has shown that warming has positive effects on plant photosynthesis. Fu et al. (2015) discovered that warming significantly increased in the Pn of the alpine plants on the Tibetan Plateau, which was related to the increase of stomatal conductance (gs), chlorophyll (Chl) content, yield, and non-photochemical quenching of Chl fluorescence. Carroll et al. (2017) found that the photosynthesis of three dominant species (Pinus contorta, P. ponderosa, and Populus tremuloides) had different responses to warming. Climatic warming also affects leaf photosynthetic physiological parameters, such as Chl fluorescence, gs, and intercellular CO2 concentration (Ci), which are all temperature-dependent (Ruiz-Vera et al., 2013). In our research, higher gs was linked to better photosynthetic carbon absorption capability. The gs affects CO2 diffusion from the atmosphere into the intercellular space of leaves, and high gs promotes plant photosynthesis and C assimilation (Sugiura et al., 2020). Moreover, we observed a significant difference in the gs of both species between the two durations of warming. Specifically, compared to the short-term warming, the two species exhibited a decrease in gs during the medium-term warming, which can be attributed to a simultaneous decrease in Pn.
Photosynthesis provides a comprehensive depiction of a plant’s physiological condition, which can be used to quantify growth differences between plants and the degree of environmental impact (Lin et al., 2017). The response of photosynthesis may differ between short-term and medium-term warming. Under short-term warming, the Pn of E. nutans decreased while that of P. anserina increased. However, the Pn of the two species had an opposite trend during medium-term warming. The Pn of E. nutans increased, while that of P. anserina decreased. Phenotypic plasticity is widely recognized as a primary mechanism by which plants adapt to variations in environmental factors, serving as an observed adaptation to short-term fluctuations in the environment (Gratani, 2014). The contrasting effects of short-term warming on Pn and Tr for E. mutans versus P. anserina, possibly attributed to phenotypic plasticity rather than adaptation. In the alpine meadow, E. nutans was the dominant species, while P. anserina was a common subordinate species. The height of a plant plays a crucial role in determining its ability to compete for light. Both of these two species have a distinct layered structure, and their photosynthetic capacity and LUE are significantly different. The competitive coexistence of different functional groups in alpine meadows is primarily attributed to their disparities in canopy photosynthetically active radiation, soil nutrient acquisition (Kleyer et al., 2019; Rao et al., 2023). Climate warming has resulted in a decline in soil nutrient and moisture, intensifying the competition between E. nutans and P. anserina. Consequently, this competition has led to changes in their leaf traits, ultimately impacting their photosynthetic capacity. In addition, climate change can directly influence plant photosynthesis through alterations in temperature and precipitation patterns. Ma et al. (2017) suggested that the two dominant species, E. nutans and Stipa aliena, were relatively insensitive to environmental changes, probably because of their greater ability to acquire nutrients and light. Warming increased the photoinhibition of E. nutans but decreased the photoinhibition of P. anserina, which is consistent with previous researches (Shi et al., 2010; Zhou et al., 2021).
The Fv/Fm value is a sensitive indicator of photoinhibition. In our study, the Fv/Fm value of the two species in the OTCs was higher than that of the OAs, indicating that the alpine plants were restricted by low temperatures, and warming improved their ability to resist stress (Figure 2). Under medium-term warming, the Fv/Fm value of P. anserina was higher than that of E. nutans, indicating more effective carboxylation and quicker light-harvesting by the PSII antenna complexes. Changes in Fv/Fm value reflect up-or down-regulation of PSII, which is associated with changes in qP or qN in PSII (Wu et al., 2018). qN is an indicator of a plant’s ability to reduce heat dissipation in its photosynthetic membranes, thereby minimizing chloroplast damage (Ware et al., 2015). E. nutans in the OTCs activated photoprotection and reduced photoinhibition mechanisms, resulting in a greater qN to dissipate excess heat and sustain C assimilation capacity.
Understanding the LUE of alpine plants is crucial for enhancing their productivity and mitigating the degradation of alpine meadows. Zhang et al. (2015) believed that the changes in plant height and coverage under warming directly affect their competition for light energy and LUE. Our study revealed that warming enhanced the LUE of both two species, with significant variations observed among different species and over different years of warming (Figure 1). In contrast to our findings, Zhou et al. (2016) suggest that simulated warming may reduce the LUE of alpine meadows due to the negative impact of warming-induced dry micro-environment on LUE, which masks the favorable effect of temperature rises. Overall, our results imply that both of the two species were impacted by climate change and that the short and medium-term impacts on various species varied. Warming improved the LUE of the two species, which was beneficial to the growth and productivity of alpine plants.
4.2 Response of leaf traits to climate warming
Leaf traits are important indicators of plant adaptation to environmental change because they are linked to the efficiency of plant resource acquisition and use (Li et al., 2018; Xu et al., 2018; Li et al., 2021). In addition, leaf traits account for the majority of the variation in ecosystem productivity (Sigdel et al., 2023). Numerous studies have demonstrated that leaf traits, such as leaf lifespan, leaf area, and leaf nutrient content, are highly sensitive to climate warming (Myers-Smith et al., 2019; Bjorkman et al., 2020). Warming directly affects leaf traits and indirectly affects plant photosynthesis and LUE through these traits. Our research indicate that the impact of warming on leaf photosynthetic capacity varies among the two species, which is related to their functional traits and soil nutrient availability (Figure 5). Under medium-term warming, both species’ RWC and SLA increased. Additionally, the TWC and SLV of E. nutans increased and the LDMC of P. anserina also increased. Our findings support the hypothesis that phenotypic plasticity in certain plant traits can serve as a predictor for community performance under climate change.
SLA and leaf nitrogen content play a crucial role in carbon fixation (Díaz et al., 2016). According to Kattge et al. (2020), SLA exhibits sensitive to climate change and is closely associated with species-specific resource utilization. It plays a crucial role in influencing photosynthesis, light interception, and plant growth, while also serving as a predictor of competitiveness and environmental tolerance (Worthy et al., 2020). Plants with a larger leaf area are more efficient in capturing light and carbon. Our research showed that the SLA of the dominant species E. nutans is enhanced by warming, which implies higher resource acquisition in warmer climates. This finding is consistent with many other studies (Guittar et al., 2016; Liu et al., 2018). SLV is an important leaf functional trait, is influenced by factors such as leaf thickness, overall dimension, and dry matter content. It serves as an indicator of a plant’s ability to adapt to extreme environments like cold and arid conditions (Su et al., 2018). Leaf volume is determined by the combination of photosynthetic area and thickness, representing the entirety of photosynthetic organs. This trait facilitates better comparison among different plant species. Su et al. (2018) proposed the concept of SLV and suggested that alpine plants with higher SLV would exhibit greater resistance to harsh environmental conditions. In our study, we observed a strong positive correlation between leaf SLV and leaf Pn during the medium-term warming (Figure 5). The higher the leaf Pn of plants, the greater the SLV; however, short-term warming exhibited an opposite trend. These results indicate that during the process of long-term adaptation to the environment, the photosynthetic capacity of plants and leaf traits are mutually adapted and coordinated.
Leaf nitrogen content is strongly correlated with photosynthetic capacity, as nitrogen is essential for the synthesis of Rubisco, which is the key enzyme in photosynthesis (Reich et al., 1994). We observed a positive correlation between leaf C and N content and LUE. Peng et al. (2020) suggested that warming slightly increased the coverage of legumes and the C: N ratio of all plants in the alpine meadow. Our investigation revealed that the C: N and N: P ratios of the two species’ leaves increased under warming, which is consistent with Peng’s findings. The alpine meadow subjected to experimental warming displayed higher leaf C: N and N: P ratios, indicating that plants were more efficient in utilizing nitrogen for growth. Plant communities exhibit both positive and negative interactions between different plant species (Callaway and Walker, 1997). Cui et al. (2023) confirmed that grasses exhibited a higher competitive ability compared to other functional groups, primarily attributed to their increased investment in roots and enhanced capacity for resource uptake.
4.3 Effects of environmental factors on light use efficiency of plants
The productivity of grasslands is influenced by the interactions between soil and climatic conditions (Shen et al., 2022). Our structural equation modeling revealed that warming indirectly impacts leaf photosynthetic capacity through factors such as soil nutrients, atmospheric CO2, and leaf traits (Figure 5). Our previous studies have demonstrated that the optimal temperature range for alpine plants is between 20°C and 25°C (Zhou et al., 2017). During the vigorous growth period in 2015 and 2018, between 09:00 and 13:00 hours, we used an LI-6400 portable photosynthesis system to measure average air temperatures of approximately 28.5°C and 31.5°C. These temperatures exceeded the optimal range for alpine plants, resulting in a negative impact on leaf photosynthetic capacity.
Soil plays a crucial role in providing the majority of nutrients necessary for plant growth, and these nutrients are closely associated with how plant leaves utilize resources (Gao et al., 2019). The soil nutrients (N) have a significant impact on leaf N and plant photosynthesis (Yu et al., 2019), and a low soil phosphorus (P) may result in reduced leaf P content, thereby limiting overall plant function (Sun et al., 2019). Climate change modifies the physical and chemical properties of soil, thereby impacting the functioning of the alpine meadow ecosystem. Our findings indicate that short and medium-term experimental warming have similar impacts on soil nutrients. Warming reduced soil nutrient contents at depths of 0-30 cm (Table 2), indicating that warming stimulated soil nutrient cycling and organic matter decomposition, resulting in a decrease in soil C and N contents (Xu et al., 2022). However, some studies have shown inconsistent results regarding the responses of soil nutrients to climate warming. While some suggest that warming has no impact on soil C and N contents (Ning et al., 2019; Yang et al., 2022), others indicate an increase (White-Monsant et al., 2017). These contradictory results may be attributed to variations in temperature and duration of warming, as well as the diversity of grassland ecosystems. In our study, soil nutrient content did not change significantly under warming. This is because, in the case of relatively short-term warming experiments (< 5 years), it was challenging to significantly alter the vast soil carbon pool due to the substantial spatial heterogeneity in soil organic carbon among plots and the limited number of repeated warming experiments.
Several studies indicate a positive correlation between warming-induced changes in plant total biomass, above-ground biomass, and below-ground biomass with soil nutrient content. This subsequently impacts the LUE of plants (Song et al., 2019; Zhou et al., 2022). Shen et al. (2022) found that the change in soil pH and nutrient imbalance caused by N and P enrichment were the main factors impacting the photosynthetic characteristics of plant in the alpine steppe. In our study, the effects of soil nutrients on LUE were primarily mediated through their impact on leaf traits. The content of soil nitrogen significantly influences leaf N content and plant photosynthesis, while inadequate levels of soil P can lead to reduced leaf P content and limit leaf function (Gong and Gao, 2019; Sun et al., 2019).
Soil respiration (Rs) is expected to have positive feedback on global warming (Wang et al., 2021). Some studies suggest that short-term warming promotes soil respiration, but there is no consistent pattern under long-term warming and variations exist among ecosystems (Chen et al., 2016; Ganjurjav et al., 2018). According to García-Palacios et al. (2021), Rs is highly sensitive to temperature, and warming will stimulate Rs activation and accelerate its rate. Yu et al. (2019) found no significant effects of experimental warming on Rs. Our findings were similar to prior research that alpine meadow Rs increased in response to both short- and medium-term warming.
5 Conclusions
In this study, we conducted a 4-year in-situ field experiment to investigate the effects of warming on photosynthesis and LUE of alpine plants on the Tibetan Plateau. Our findings suggest that the two typical alpine species were affected by climate warming, and the effects of short and medium-term warming on different species exhibited significant species-specific variations. Warming improved the LUE of both species, which was beneficial to the growth and productivity of alpine plants. During the medium-term adaptation to the environment, the photosynthetic capacity of plants and leaf traits are mutually adapted and coordinated. Leaf traits, such as SLV, showed a significant positive correlation with leaf Pn. Additionally, warming primarily enhances leaf functional traits by altering soil nutrients and thus affects leaf photosynthetic capacity. Our findings will be useful in understanding the underlying mechanisms of alpine plant responses to global warming.
Data availability statement
The original contributions presented in the study are included in the article/Supplementary Material, further inquiries can be directed to the corresponding author/s.
Author contributions
ZZ: Writing – original draft, Writing – review & editing. PS: Supervision, Writing – review & editing. JY: Writing – review & editing, Visualization, Data curation. RS: Writing – review & editing, Investigation. XD: Writing – review & editing, Investigation.
Funding
The author(s) declare that financial support was received for the research, authorship, and/or publication of this article. This research was financially supported by the Strategic Priority Research Program of Chinese Academy of Sciences (XDA20050102), the Natural Science Foundation of Gansu Province (23JRRA608), and the National Natural Science Foundation of China (41701106, 41871043).
Conflict of interest
The authors declare that the research was conducted in the absence of any commercial or financial relationships that could be construed as a potential conflict of interest.
Publisher’s note
All claims expressed in this article are solely those of the authors and do not necessarily represent those of their affiliated organizations, or those of the publisher, the editors and the reviewers. Any product that may be evaluated in this article, or claim that may be made by its manufacturer, is not guaranteed or endorsed by the publisher.
Supplementary material
The Supplementary Material for this article can be found online at: https://www.frontiersin.org/articles/10.3389/fpls.2024.1353762/full#supplementary-material
Supplementary Figure 1 | Air temperature at a height of 1.5 m (°C), soil temperature at 5 cm depth (°C), soil moisture at 5 cm depth (%), and daily precipitation (mm) during the growing season (from May to September) in open-top chambers (OTCs) and open areas (OAs) from 2015 to 2018.
Supplementary Figure 2 | Principal Component Analysis (PCA) for the variance in leaf traits among different species and treatments. The horizontal axis represents the first principal component (PC1); the vertical axis represents the second principal component (PC2). E: Elymus nutans, P: Potentilla anserina, Pn: net photosynthetic rate, Tr: transpiration rate, gs: stomatal conductance, LUE: light use efficiency, Fv/Fm: maximum photochemical efficiency of PSII, yield: effective photochemical efficiency, qP: photochemical quenching, qN: non-photochemical quenching (qN), TC: total carbon content, TN: total nitrogen content, TP: total phosphorus contents, NP: the N:P ratios. TWC: the total water content, RWC: relative water content, LDMC: leaf dry matter content, SLA: specific leaf area, SLV: specific leaf volume.
References
Allison, S. D., Romero-Olivares, A. L., Lu, Y., Taylor, J. W., Treseder, K. K. (2018). Temperature sensitivities of extracellular enzyme Vmax and Km across thermal environments. Global Change Biol. 24, 2884–2897. doi: 10.1111/gcb.14045
Atkin, O., Bruhn, D., Hurry, V., Tjoelker, M. (2005). The hot and the cold: unravelling the variable response of plant respiration to temperature. Evans review no. 2. Funct. Plant Biol. 32, 87–105. doi: 10.1071/FP03176
Bai, E., Li, S. L., Xu, W. H., Li, W., Dai, W. W., Jiang, P. (2013). A meta-analysis of experimental warming effects on terrestrial nitrogen pools and dynamics. New Phytol. 199, 441–451. doi: 10.1111/nph.12252
Bjorkman, A. D., García Criado, M., Myers-Smith, I. H., Ravolainen, V., Jónsdöttir, I. S., Westergaard, K. B., et al. (2020). Status and trends in Arctic vegetation: evidence from experimental warming and long-term monitoring. Ambio 49, 678–692. doi: 10.1007/s13280-019-01161-6
Buzzard, V., Michaletz, S. T., Deng, Y., He, Z., Ning, D., Shen, L., et al. (2019). Continental scale structuring of forest and soil diversity via functional traits. Nat. Ecol. Evol. 3, 1298–1308. doi: 10.1038/s41559-019-0954-7
Callaway, R. M., Walker, L. R. (1997). Competition and facilitation: A synthetic approach to interactions in plant communities. Ecology 78, 1958–1965. doi: 10.1890/0012-9658(1997)078[1958:CAFASA]2.0.CO;2
Carroll, C. J., Knapp, A. K., Martin, P. H. (2017). Dominant tree species of the Colorado Rockies have divergent physiological and morphological responses to warming. For. Ecol. Manage. 402, 234–240. doi: 10.1016/j.foreco.2017.07.048
Chen, J., Luo, Y., Xia, J., Shi, Z., Jiang, L., Niu, S., et al. (2016). Differential responses of ecosystem respiration components to experimental warming in a meadow grassland on the Tibetan Plateau. Agric. For. Meteorol. 220, 21–29. doi: 10.1016/j.agrformet.2016.01.010
Cui, G., Pugnaire, F. I., Yang, L., Zhao, W., Ale, R., Shen, W., et al. (2023). Shrub mediated effects on soil nitrogen determines shrub-herbaceous interactions in drylands of the Tibetan Plateau. Front. Plant Sci. 14. doi: 10.3389/fpls.2023.1137365
Díaz, S., Kattge, J., Cornelissen, J. H. C., Wright, I. J., Lavorel, S., Dray, S., et al. (2016). The global spectrum of plant form and function. Nature 529, 167–171. doi: 10.1038/nature16489
Duan, M., Li, A., Wu, Y., Zhao, Z., Peng, C., DeLuca, T. H., et al. (2019). Differences of soil CO2 flux in two contrasting subalpine ecosystems on the eastern edge of the Qinghai-Tibetan Plateau: A four-year study. Atmos. Environ. 198, 166–174. doi: 10.1016/j.atmosenv.2018.10.067
Feng, X. L., Dietze, M. (2013). Scale dependence in the effects of leaf ecophysiological traits on photosynthesis: Bayesian parameterization of photosynthesis models. New Phytol. 200, 1132–1144. doi: 10.1111/nph.12454
Forkel, M., Carvalhais, N., Rödenbeck, C., Keeling, R., Heimann, M., Thonicke, K., et al. (2016). Enhanced seasonal CO2 exchange caused by amplified plant productivity in northern ecosystems. Science 351, 696–699. doi: 10.1126/science.aac4971
Fu, Y. H., Gao, X. J., Zhu, Y. M., Guo, D. (2021). Climate change projection over the Tibetan Plateau based on a set of RCM simulations. Adv. Clim. Change Res. 12, 313–321. doi: 10.1016/j.accre.2021.01.004
Fu, G., Shen, Z. X., Sun, W., Zhong, Z. M., Zhang, X. Z., Zhou, Y. T. (2015). A meta-analysis of the effects of experimental warming on plant physiology and growth on the Tibetan Plateau. J. Plant Growth. Regul. 34, 57–65. doi: 10.1007/s00344-014-9442-0
Ganjurjav, H., Hu, G., Wan, Y., Li, Y., Danjiu, L., Gao, Q. (2018). Different responses of ecosystem carbon exchange to warming in three types of alpine grassland on the central Qinghai–Tibetan Plateau. Ecol. Evol. 8, 1507–1520. doi: 10.1002/ece3.3741
Gao, J., Song, Z., Liu, Y. (2019). Response mechanisms of leaf nutrients of endangered plant (Acer catalpifolium) to environmental factors varied at different growth stages. Glob. Ecol. Conserv. 17, e00521. doi: 10.1016/j.gecco.2019.e00521
García-Palacios, P., Crowther, T. W., Dacal, M., Hartley, I. P., Reinsch, S., Rinnan, R., et al. (2021). Evidence for large microbial-mediated losses of soil carbon under anthropogenic warming. Nat. Rev. Earth Env. 2, 507–517. doi: 10.1038/s43017-021-00178-4
Gong, H., Gao, J. (2019). Soil and climatic drivers of plant SLA (specific leaf area). Glob. Ecol. Conserv. 20, e00696. doi: 10.1016/j.gecco.2019.e00696
Gratani, L. (2014). Plant phenotypic plasticity in response to environmental factors. Adv. Bot. 2014, 208747. doi: 10.1155/2014/208747
Guittar, J., Goldberg, D., Klanderud, K., Telford, R. J., Vandvik, V. (2016). Can trait patterns along gradients predict plant community responses to climate change? Ecology 97, 2791–2801. doi: 10.1002/ecy.1500
He, J. S., Wang, Z. H., Wang, X. P., Schmid, B., Zuo, W. Y., Zhou, M., et al. (2006). A test of the generality of leaf trait relationships on the Tibetan Plateau. New Phytol. 170, 835–848. doi: 10.1111/j.1469-8137.2006.01704.x
Huang, W., Wang, W., Cao, M., Fu, G., Xia, J., Wang, Z., et al. (2021). Local climate and biodiversity affect the stability of China’s grasslands in response to drought. Sci. Total Environ. 768, 145482. doi: 10.1016/j.scitotenv.2021.145482
Hughes, T. P., Kerry, J. T., Baird, A. H., Connolly, S. R., Dietzel, A., Eakin, C. M., et al. (2018). Global warming transforms coral reef assemblages. Nature 556, 492–496. doi: 10.1038/s41586-018-0041-2
Immerzeel, W. W., Lutz, A. F., Andrade, M., Bahl, A., Biemans, H., Bolch, T., et al. (2020). Importance and vulnerability of the world’s water towers. Nature 577, 364–369. doi: 10.1038/s41586-019-1822-y
Jassey, V. E., Signarbieux, C. (2019). Effects of climate warming on Sphagnum photosynthesis in peatlands depend on peat moisture and species-specific anatomical traits. Glob. Change Biol. 25, 3859–3870. doi: 10.1111/gcb.14788
Kattge, J., Bönisch, G., Díaz, S., Lavorel, S., Prentice, I. C., Leadley, P., et al. (2020). TRY plant trait database–enhanced coverage and open access. Glob. Change Biol. 26, 119–188. doi: 10.1111/gcb.14904
Kleyer, M., Trinogga, J., Cebrián-Piqueras, M. A., Trenkamp, A., Fløjgaard, C., Ejrnæs, R., et al. (2019). Trait correlation network analysis identifies biomass allocation traits and stem specific length as hub traits in herbaceous perennial plants. J. Ecol. 107, 829–842. doi: 10.1111/1365-2745.13066
Knapp, A. K., Chen, A., Griffin-Nolan, R. J., Baur, L. E., Carroll, C. J. W., Gray, J. E., et al. (2020). Resolving the Dust Bowl paradox of grassland responses to extreme drought. Proc. Natl. Acad. Sci. U.S.A. 117, 22249–22255. doi: 10.1073/pnas.1922030117
Lee, J. H., Kim, J. Y., Kim, J. I., Park, Y. J., Park, C. M. (2020). Plant thermomorphogenic adaptation to global warming. J. Plant Biol. 63, 1–9. doi: 10.1007/s12374-020-09232-y
Li, C., Lai, C., Peng, F., Xue, X., You, Q., Liu, F., et al. (2021). Dominant plant functional group determine the response of the temporal stability of plant community biomass to 9-year warming on the Qinghai–Tibetan plateau. Front. Plant Sci. 1871. doi: 10.3389/fpls.2021.704138
Li, D., Li, X. X., Li, Z. S., Fu, Y., Zhang, J. T., Zhao, Y. J., et al. (2024). Drought limits vegetation carbon sequestration by affecting photosynthetic capacity of semi-arid ecosystems on the Loess Plateau. Sci. Total. Environ. 912, 168778. doi: 10.1016/j.scitotenv.2023.168778
Li, W., Wang, J. L., Zhang, X. J., Shi, S. L., Cao, W. X. (2018). Effect of degradation and rebuilding of artificial grasslands on soil respiration and carbon and nitrogen pools on an alpine meadow of the Qinghai-Tibetan Plateau. Ecol. Eng. 111, 134–142. doi: 10.1016/j.ecoleng.2017.10.013
Lin, J., Wang, Y., Sun, S., Mu, C., Yan, X. (2017). Effects of arbuscular mycorrhizal fungi on the growth, photosynthesis and photosynthetic pigments of Leymus chinensis seedlings under salt-alkali stress and nitrogen deposition. Sci. Total Environ. 576, 234e241. doi: 10.1016/j.scitotenv.2016.10.091
Liu, G., Wang, L., Jiang, L., Pan, X., Huang, Z., Dong, M., et al. (2018). Specific leaf area predicts dryland litter decomposition via two mechanisms. J. Ecol. 106, 218–229. doi: 10.1111/1365-2745.12868
Ma, Z., Liu, H., Mi, Z., Zhang, Z., Wang, Y., Xu, W., et al. (2017). Climate warming reduces the temporal stability of plant community biomass production. Nat. Commun. 8, 15378. doi: 10.1038/ncomms15378
Masson-Delmotte, V., Zhai, P., Pirani, A., Connors, S., Péan, C., Berger, S., et al. (2021). Contribution of working group I to the sixth assessment report of the intergovernmental panel on climate change. Climate Change: Phys. Sci. Basis. doi: 10.1017/9781009157896
Medlyn, B. E. (1998). Physiological basis of the light use efficiency model. Tree Physiol. 18, 167–176. doi: 10.1093/treephys/18.3.167
Meng, F., Liu, D., Wang, Y., Wang, S., Wang, T. (2023). Negative relationship between photosynthesis and late-stage canopy development and senescence over Tibetan Plateau. Glob. Change Biol 29, 3147–3158. doi: 10.1111/gcb.16668
Myers-Smith, I. H., Thomas, H. J. D., Bjorkman, A. D. (2019). Plant traits inform predictions of tundra responses to global change. New Phytol. 221, 1742–1748. doi: 10.1111/nph.15592
Ning, Z., Li, Y., Yang, H., Zhang, Z., Zhang, J. (2019). Stoichiometry and effects of carbon, nitrogen, and phosphorus in soil of desertified grasslands on community productivity and species diversity. Acta Ecol. Sin. 39(10), 3537–3546. doi: 10.5846/stxb201711242094
Peng, A., Klanderud, K., Wang, G., Zhang, L., Xiao, Y., Yang, Y. (2020). Plant community responses to warming modified by soil moisture in the Tibetan Plateau. Arct. Antarct. Alp. Res. 52, 60–69. doi: 10.1080/15230430.2020.1712875
Perez-Harguindeguy, N., Diaz, S., Garnier, E., Lavorel, S., Poorter, H., Jaureguiberry, P., et al. (2013). New handbook for standardised measurement of plant functional traits worldwide. Aust. Bot. 61, 167–234. doi: 10.1071/BT12225
Rao, Q. Y., Chen, J. F., Chou, Q. C., Ren, W. J., Cao, T., Zhang, M., et al. (2023). Linking trait network parameters with plant growth across light gradients and seasons. Funct. Ecol. 37, 1732–1746. doi: 10.1111/1365-2435.14327
Reich, P. B., Walters, M. B., Ellsworth, D. S., Uhl, C. (1994). Photosynthesis– nitrogen relations in Amazonian tree species. 1. Patterns among species and communities. Oecologia 97, 72–62. doi: 10.1007/BF00317909
Ruiz-Vera, U. M., Siebers, M., Gray, S. B., Drag, D. W., Rosenthal, D. M., Kimball, B. A., et al. (2013). Global warming can negate the expected CO2 stimulation in photosynthesis and productivity for soybean grown in the Midwestern United States. Plant Physiol. 162, 410–423. doi: 10.1104/pp.112.211938
Shen, R. N., Zhang, Y. J., Zhu, J. T., Chen, N., Chen, Y., Zhao, G., et al. (2022). The interactive effects of nitrogen addition and increased precipitation on gross ecosystem productivity in an alpine meadow. J. Plant Ecol. 15, 168–179. doi: 10.1093/jpe/rtab081
Shi, F., Wu, Y., Wu, N., Luo, P. (2010). Different growth and physiological responses to experimental warming of two dominant plant species Elymus nutans and Potentilla anserina in an alpine meadow of the eastern Tibetan Plateau. Photosynthetica 48, 437–445. doi: 10.1007/s11099-010-0058-8
Sigdel, S. R., Liang, E. Y., Rokaya, M. B., Rai, S., Dyola, N., Sun, J., et al. (2023). Functional traits of a plant species fingerprint ecosystem productivity along broad elevational gradients in the Himalayas. Funct. Ecol. 37, 383–394. doi: 10.1111/1365-2435.14226
Šímová, I., Rueda, M., Hawkins, B. A. (2017). Stress from cold and drought as drivers of functional trait spectra in North American angiosperm tree assemblages. Ecol. Evol. 7, 7548–7559. doi: 10.1002/ece3.3297
Song, J., Wan, S., Piao, S., Knapp, A. K., Classen, A. T., Vicca, S., et al. (2019). A meta-analysis of 1,119 manipulative experiments on terrestrial carbon-cycling responses to global change. Nat. Ecol. Evol. 3, 1309–1320. doi: 10.1038/s41559-019-0958-3
Storkey, J., Macdonald, A. J. (2022). The role of long-term experiments in validating trait-based approaches to achieving multifunctionality in grasslands. Front. Agric. Sci. Eng. 9, 187–196. doi: 10.15302/J-FASE-2021438
Su, P., Shi, R., Zhou, Z., Xie, T. (2018). Characteristics and relationships of foliar element content and specific leaf volume of alpine plant functional groups. Int. J. Agric. Biol. 20, 1663–1671. doi: 10.17957/IJAB/15.0704
Sugiura, D., Terashima, I., Evans, J. R. (2020). A decrease in mesophyll conductance by cell-wall thickening contributes to photosynthetic downregulation. Plant Physiol. 183, 1600–1611. doi: 10.1104/pp.20.00328
Sun, J., Liu, B., You, Y., Li, W., Liu, M., Shang, H., et al. (2019). Solar radiation regulates the leaf nitrogen and phosphorus stoichiometry across alpine meadows of the Tibetan Plateau. Agric. For. Meteorol. 271, 92–101. doi: 10.1016/j.agrformet.2019.02.041
Wang, Y., Song, C., Liu, H., Wang, S., Zeng, H., Luo, C., et al. (2021). Precipitation determines the magnitude and direction of interannual responses of soil respiration to experimental warming. Plant Soil 458, 75–91. doi: 10.1007/s11104-020-04438-y
Ware, M. A., Belgio, E., Ruban, A. V. (2015). Photoprotective capacity of non-photochemical quenching in plants acclimated to different light intensities. Photosynth. Res. 126, 261–274. doi: 10.1007/s11120-015-0102-4
Wei, B., Zhang, D. Y., Wang, G. Q., Liu, Y., Li, Q. L., Zheng, Z. H., et al. (2023). Experimental warming altered plant functional traits and their coordination in a permafrost ecosystem. New Phytol. 240, 1802–1816. doi: 10.1111/nph.19115
White-Monsant, A., Clark, G., Chuen, M. N. K., Tang, C. (2017). Experimental warming and antecedent fire alter leaf element composition and increase soil C: N ratio in sub-alpine open heathland. Sci. Total Environ. 595, 41–50. doi: 10.1016/j.scitotenv.2017.03.237
Worthy, S. J., Laughlin, D. C., Zambrano, J., Umaña, M. N., Zhang, C., Lin, L., et al. (2020). Alternative designs and tropical tree seedling growth performance landscapes. Ecology 101, e03007. doi: 10.1002/ecy.3007
Wright, I. J., Reich, P. B., Westoby, M., Ackerly, D. D., Baruch, Z., Bongers, F., et al. (2004). The worldwide leaf economics spectrum. Nature 428, 821–827. doi: 10.1038/nature02403
Wu, Y. J., Ren, C., Tian, Y., Zha, T. S., Liu, P., Bai, Y. J., et al. (2018). Photosynthetic gas-exchange and PSII photochemical acclimation to drought in a native and non-native xerophytic species (Artemisia ordosica and Salix psammophila). Ecol. Indic. 94, 130–138. doi: 10.1016/j.ecolind.2018.06.040
Wu, L., Ren, Y., Wan, J. Z., Wang, M., Wang, Z., Fu, F., et al. (2022). Effects of precipitation change and nitrogen and phosphorus additions on traits and abundance of potentilla anserina in an alpine meadow. Atmosphere 13, 1820. doi: 10.3390/atmos13111820
Xu, B., Wang, J., Wu, N., Wu, Y., Shi, F. (2018). Seasonal and interannual dynamics of soil microbial biomass and available nitrogen in an alpine meadow in the eastern part of Qinghai–Tibet Plateau, China. Biogeosciences 15, 567–579. doi: 10.5194/bg-15-567-2018
Xu, M., Zhao, Z., Zhou, H., Ma, L., Liu, X. (2022). Plant allometric growth enhanced by the change in soil stoichiometric characteristics with depth in an alpine meadow under climate warming. Front. Plant Sci. 13. doi: 10.3389/fpls.2022.860980
Yang, Y., Shi, G., Liu, Y., Ma, L., Zhang, Z., Jiang, S., et al. (2022). Experimental warming has not affected the changes in soil organic carbon during the growing season in an alpine meadow ecosystem on the Qinghai–Tibet plateau. Front. Plant Sci. 13. doi: 10.3389/fpls.2022.847680
Yao, H. Y., Li, X. Y., Zhang, C. C., Wang, P., Shi, F. Z., Deng, Y. J. (2023). Tradeoff in the supply and demand for CO2 dominates the divergence of net photosynthesis rates of functional plants in alpine ecosystems. Ecohydrology 16, e2487. doi: 10.1002/eco.2487
Yu, C. Q., Wang, J. W., Shen, Z. X., Fu, G. (2019). Effects of experimental warming and increased precipitation on soil respiration in an alpine meadow in the Northern Tibetan Plateau. Sci. Total. Environ. 647, 1490–1497. doi: 10.1016/j.scitotenv.2018.08.111
Zhang, X. Z., Shen, Z. X., Fu, G. (2015). A meta-analysis of the effects of experimental warming on soil carbon and nitrogen dynamics on the Tibetan Plateau. Appl. Soil. Ecol. 87, 32–38. doi: 10.1016/j.apsoil.2014.11.012
Zhang, Z., Sun, J., Liu, M., Shang, H., Wang, J., Wang, J., et al. (2022). Context-dependency in relationships between herbaceous plant leaf traits and abiotic factors. Front. Plant Sci. 13. doi: 10.3389/fpls.2022.757077
Zhou, Z. J., Su, P. X., Shi, R., Xie, T. T. (2017). Light use efficiency of plants and the environmental impact factors in different alpine ecosystems. Chin. J. Ecol. 36, 1570. doi: 10.13292/j.1000-4890.201706.025
Zhou, Z. J., Su, P. X., Wu, X. K., Shi, R., Ding, X. J. (2021). Leaf and community photosynthetic carbon assimilation of alpine plants under in-situ warming. Front. Plant Sci. 12. doi: 10.3389/fpls.2021.690077
Zhou, G., Terrer, C., Huang, A., Hungate, B. A., van Gestel, N., Zhou, X., et al. (2022). Nitrogen and water availability control plant carbon storage with warming. Sci. Total. Environ. 851, 158243. doi: 10.1016/j.scitotenv.2022.158243
Keywords: photosynthesis, leaf traits, soil nutrients, climate change, alpine plants
Citation: Zhou Z, Su P, Yang J, Shi R and Ding X (2024) Warming affects leaf light use efficiency and functional traits in alpine plants: evidence from a 4-year in-situ field experiment. Front. Plant Sci. 15:1353762. doi: 10.3389/fpls.2024.1353762
Received: 11 December 2023; Accepted: 27 February 2024;
Published: 19 March 2024.
Edited by:
Samuel Kuria Kiboi, University of Nairobi, KenyaReviewed by:
Shalik Ram Sigdel, Institute of Tibetan Plateau Research, Chinese Academy of Sciences (CAS), ChinaJalal Kassout, National Institute for Agricultural Research, Morocco
Copyright © 2024 Zhou, Su, Yang, Shi and Ding. This is an open-access article distributed under the terms of the Creative Commons Attribution License (CC BY). The use, distribution or reproduction in other forums is permitted, provided the original author(s) and the copyright owner(s) are credited and that the original publication in this journal is cited, in accordance with accepted academic practice. No use, distribution or reproduction is permitted which does not comply with these terms.
*Correspondence: Peixi Su, supx@lzb.ac.cn