- 1Laboratory of Extremophile Plants, Centre of Biotechnology of Borj Cedria, Hammam-Lif, Tunisia
- 2Faculty of Sciences of Tunis, University of Tunis El Manar, El Manar Tunis, Tunisia
- 3Environmental Biotechnology Laboratory, Department of Biotechnology, University of the Western Cape, Bellville, South Africa
- 4Plant Stress Tolerance Laboratory, University of Mpumalanga, Mbombela, South Africa
- 5Laboratory of Bioactive Substances, Centre of Biotechnology of Borj Cedria, Hammam-Lif, Tunisia
- 6Council for Agricultural Research and Economics, Research Centre for Animal Production and Aquaculture, Lodi, Italy
- 7Department of Bioengineering, Adana Alparslan Türkeş Science and Technology University, Adana, Türkiye
- 8DSI-NRF Centre of Excellence in Food Security, University of the Western Cape, Bellville, South Africa
Salinity and Phoma medicaginis infection represent significant challenges for alfalfa cultivation in South Africa, Europe, Australia, and, particularly, Tunisia. These constraints have a severe impact on both yield and quality. The primary aim of this study was to establish the genetic basis of traits associated with biomass and growth of 129 Medicago sativa genotypes through genome-wide association studies (GWAS) under combined salt and P. medicaginis infection stresses. The results of the analysis of variance (ANOVA) indicated that the variation in these traits could be primarily attributed to genotype effects. Among the test genotypes, the length of the main stem, the number of ramifications, the number of chlorotic leaves, and the aerial fresh weight exhibited the most significant variation. The broad-sense heritability (H²) was relatively high for most of the assessed traits, primarily due to genetic factors. Cluster analysis, applied to morpho-physiological traits under the combined stresses, revealed three major groups of accessions. Subsequently, a GWAS analysis was conducted to validate significant associations between 54,866 SNP-filtered single-nucleotide polymorphisms (SNPs) and seven traits. The study identified 27 SNPs that were significantly associated with the following traits: number of healthy leaves (two SNPs), number of chlorotic leaves (five SNPs), number of infected necrotic leaves (three SNPs), aerial fresh weight (six SNPs), aerial dry weight (nine SNPs), number of ramifications (one SNP), and length of the main stem (one SNP). Some of these markers are related to the ionic transporters, cell membrane rigidity (related to salinity tolerance), and the NBS_LRR gene family (associated with disease resistance). These findings underscore the potential for selecting alfalfa genotypes with tolerance to the combined constraints of salinity and P. medicaginis infection.
1 Introduction
Tunisia boasts a rich and diverse range of forage and pasture biodiversity, with over 960 pasture legume species, including 336 that are unique to the Mediterranean region (Ibidhi et al., 2018; Ferchichi et al., 2021). However, due to increasing purchasing power, the demand for ruminant products is expected to rise significantly in the coming years, making high levels of forage crop production imperative. The country’s livestock sector heavily relies on forage crops, particularly alfalfa (Medicago sativa), which covers an area of approximately 12,410 hectares (Guiza et al., 2022). Alfalfa plays a vital role in supporting livestock nutrition and ensuring food security.
Presently, the Tunisian livestock sector is grappling with a persistent shortage of forage. Like many other regions globally, it faces the challenge of climate change and its associated environmental stresses, among which soil salinization and pathogen infections are particularly important for alfalfa productivity (Djebali, 2008; Arraouadi et al., 2011; Badri et al., 2021). Furthermore, the interaction between salinity and Phoma medicaginis infection can exacerbate the adverse effects of these stresses on alfalfa plants (Castell-Miller et al., 2007; Kaiwen et al., 2020; Maiza et al., 2021). The coexistence of these stresses creates a more hostile environment for the crop to thrive. Under saline conditions, reduced water uptake and compromised nutrient absorption weaken the plant’s defenses against strain Phoma medicaginis 8 (Pm8) infection, making the plant more susceptible to disease progression (Djébali et al., 2013). Therefore, the increasing prevalence of salinity stress and P. medicaginis infection poses a significant threat to alfalfa production in Tunisia.
Despite its significance as a forage legume, there has been limited work on varietal selection for this species in Tunisia, with only two registered local varieties (Gabès and El Hamma) (Jabri et al., 2021; Maiza et al., 2021). Therefore, it is advisable to initiate new varietal selection efforts for alfalfa, utilizing diverse germplasm, to develop new varieties with robust agronomic performance under the combined constraints of salinity and P. medicaginis infection in the context of climate change.
Moreover, the socio-economic implications of the shortage of forage in Tunisia, particularly its impact on the livestock sector and food security, warrant further exploration and discussion.
As a result, diverse studies focusing on enhancing alfalfa have led to the development of cultivars showcasing superior attributes such as increased yield, enhanced stress tolerance, and improved forage quality (Badri et al., 2021). A significant challenge in alfalfa enhancement lies in pinpointing the genetic underpinnings responsible for variations within the species across various agronomic traits (Sakiroglu and Brummer, 2017). Addressing these implications is essential for devising effective strategies to mitigate the challenges faced by farmers and ensure sustainable agricultural practices.
Furthermore, a comparative analysis of alfalfa production and management strategies such as cropping systems-, and soil-related management practices (Thivierge et al., 2023) in other regions facing similar environmental stresses could provide valuable insights and lessons learned that can inform the development of tailored solutions for Tunisia and similar ecogeographic regions (Tlahig et al., 2020). By examining approaches adopted in different contexts, we can identify best practices and adapt them to the Tunisian agricultural landscape, thereby enhancing the resilience and productivity of the alfalfa sector.
Alfalfa is an autotetraploid species with a chromosome count of 32 (2n = 4x = 32) and a genome size ranging from 800 to 1000 Mb. Several challenges affect its genetic improvement because of various factors such as intra-varietal variation, environmental influences, and the complex life cycle of the plant (Liu et al., 2023). Due to these hindrances, conventional breeding in alfalfa has been difficult and only moderately efficient (Annicchiarico et al., 2015a). The progression of genomic technology could play a pivotal role in allowing association mapping studies that could accelerate and strengthen crop improvement (Zhang et al., 2020).
Genomic investigations, including those based on genotyping-by-sequencing (GBS) markers, have enabled the detection of QTLs that influence various important agricultural characteristics in alfalfa. These traits encompass biomass yield, tolerance to factors such as aluminum, drought, and salt, as well as the ability to withstand freezing and maintain biomass yield during drought stress (Filho et al., 2023). Genome-wide association studies (GWAS) offer a potent strategy for deciphering genomic regions involved in targeted traits by scrutinizing the association between genome-wide single-nucleotide polymorphism (SNP) markers and phenotypic variation with exceptional precision compared to other approaches (Porter and O’Reilly, 2017; Larkin et al., 2019; Lin et al., 2023). Indeed, the GWAS approach enables the detection of more than one distinct allele for each locus in the studied accessions, and it would allow for a much more precise identification of candidate genes potentially involved in the genetic determination of the agronomic traits of interest (Liu et al., 2023).
Previously, GWAS has been employed in alfalfa to pinpoint loci markers associated with forage yield (Sakiroglu et al., 2012), drought resistance (Zhang et al., 2015), salt tolerance (Yu et al., 2016), forage quality (Sakiroglu and Brummer, 2017; Biazzi et al., 2017), and resistance to Verticillium wilt (Yu et al., 2017). No information is available for candidate genes underlying possible alfalfa tolerance to combined stresses of P. medicaginis infection and salinity.
The current work aimed to (i) characterize the response of alfalfa accessions to the combination of salinity and P. medicaginis infection, and (ii) identify the genetic determinants of tolerance to these constraints in this species through GWAS, to predict breeding values using SNP markers with allele dosage in breeding populations of alfalfa.
2 Materials and methods
2.1 Plant materials and phenotyping
A set of 126 alfalfa parent plants was randomly chosen from a Mediterranean reference population obtained from crosses between the elite cultivars (Erfoud 1, Mamuntanas, and Sardi 10) over two generations. These cultivars have demonstrated strong adaptation and different adaptive patterns in Mediterranean environments (Annicchiarico et al., 2011). These plants underwent ApekI-based GBS characterization as described in Annicchiarico et al. (2015b), and were polycrossed in isolation to obtain half-sib progeny seed used for phenotyping experiments. The seeds of these half-sib families (named as “accessions” hereafter) and those of the three parent cultivars were germinated in Petri dishes within a culture chamber for six days. After the radicle appearance, they were transplanted in 1-liter pots (diameter of 22.8 cm and height of 18 cm) filled with a mixture of soil and compost (2: 1) in a greenhouse with a temperature of 25/18°C (day/night), a relative humidity of 60–80%, and a photoperiod of 16/8 hours (light/dark). After 2 months of cultivation, plants at the flowering bud stage were subjected to a saline treatment of 150 mM NaCl (for 21 days) until the appearance of symptoms related to saline stress. At this stage, they were infected with the strain Pm8 of Phoma medicaginis (5*106 conidies µL-1) as described by Zaidi et al. (2021). Previous optimization studies by Badri et al. (2021) and Jabri et al. (2021) on alfalfa grown under a range of salt concentrations (NaCl) have revealed that 150 mM NaCl is the most appropriate for analyzing the behavior of accessions of this species under this constraint. The rationale for starting the inoculation 21 days after the salt treatment is based on previous research findings (Haddoudi et al., 2021) that indicate a specific timeframe during which the plants exhibit maximum susceptibility to infection post-salt stress exposure. This interval ensures that the plants are at a stage where their physiological responses to salt stress have been initiated, thereby facilitating a more accurate assessment of the interaction between salt stress and pathogen infection. The trial was conducted with a split-plot design, employing a completely randomized setup with three distinct blocks. Within each block, three replicates were allocated for every genotype (129 genotypes), and the plants were cultivated in pots within a greenhouse environment. This resulted in a total of 1,161 plants, with nine replicates for each accession and treatment.
Fourteen days after the disease inoculation, all the accessions were screened for a set of seven morphological traits, including four traits related to growth and biomass parameters (aerial fresh weight, AFW; aerial dry weight, ADW; length of the main stem, LMS; and number of ramifications, NR) and three traits related to the leaf aspect (number of chlorotic leaves, NCL; number of healthy leaves, NHL; and number of chlorotic necrotic leaves, NCNL).
2.2 Phenotypic data analyses
An ANOVA was performed for each trait to assess the response of accessions to combined salt and strain Pm8 infection stresses, testing the equality of variance and marginal means. Meanwhile, a normality test was conducted for each trait using the Kolmogorov-Smirnov tests. Pearson correlation was calculated between traits using trait means. All analyses were performed using SPSS 20 (http://www.ibm.com/software/analytics/spss/). Broad-sense heritability (H2) was estimated using the formula:
where Vg and Ve were genotypic variance and environmental variance, respectively.
The genotypic coefficient of variation (GCV) was calculated using the formula:
where X is the average of each trait of the whole association panel (Ahsan et al., 2015).
Furthermore, a principal component analysis (PCA) and a hierarchical clustering analysis (HCA) were performed to identify the grouping of the measured traits and the patterns of differentiation among accessions under combined stresses. The PCA and the HCA were performed using XLSTAT version 2022 based on the standardized mean values of all traits.
2.3 Alignment to Medicago truncatula, GWAS and marker-trait association analyses
We estimated suitable LS-means values and applied them to standardize our phenotypic data, ensuring that the prerequisite of normality for conducting genome-wide association analyses was met. Following this standardization process, we conducted the Kolmogorov-Smirnov test for each phenotype to confirm the attainment of a normal distribution in our phenotypic data.
With no alfalfa reference currently available, the Medicago truncatula genome may be a potential reference genome, owing to the presence of a high level of synteny between the M. sativa linkage map and the physical map of M. truncatula due to its small genome size (Li et al., 2015). SNP markers identified by GBS underwent an additional filtration step, wherein those with a minor allele frequency (MAF) below 0.05 were excluded. The resulting set of 54,866 SNPs was employed to conduct an analysis of associations between markers and traits using the TASSEL software v5.0 (http://www.maizegenetics.net/tassel) (Bradbury et al., 2007) for the GWAS. To account for potential population structure effects, a mixed linear model (MLM) incorporating a kinship (K) matrix was employed during the association mapping process. Statistical significance of markers was ascertained using the false discovery rate (FDR) method with a threshold of 0.05, as outlined by Benjamini and Hochberg (1995).
False discovery rate in GWAS may be controlled by Bonferroni’s multiple testing correction method, which proved to be too conservative in practice. We preferred to use a conservative P level corresponding to an association score [–Log10 (p-value)] 4, as suggested in earlier studies (Kang et al., 2015; Sakiroglu and Brummer, 2017). M. truncatula-aligned SNPs that were significantly associated with one or more alfalfa studied traits were mapped on Mt4.0v1 using the Jbrowse tool in the plant comparative genomics portal ensemble Plants (http://plants.ensembl.org/index.html) to identify putative genes with significant SNPs, searching through 10 kb upstream and downstream of each SNP. Knowledge on the function of those genes is useful for selecting significant SNPs to integrate into breeding programs for targeted traits.
3 Results
3.1 Morphophysiological variation, heritability, and correlations among traits
Results from ANOVA showed a significant effect of the genotype factor on all measured traits (Table 1). Notably, this factor exerted a strong influence on both the morphological parameters related to growth and biomass production (LMS, NR, AFW, and ADW) and the leaf traits affected by the combination of Pm8 inoculation and salt stress (NHL, NCL, NCNL). A moderate to high coefficient of variation (CV) was noted among genotypes for LMS (22.01%), ADW (55.43%), NCL (52.87%) and NHL (50.48%). Overall, the broad-sense heritability (H2) was relatively high for most traits under combined stresses. It ranged from 0.35 for NCL to 0.84 for NHL, with all other traits showing an intermediate heritability value of about 0.5-0.6 (Table 2).
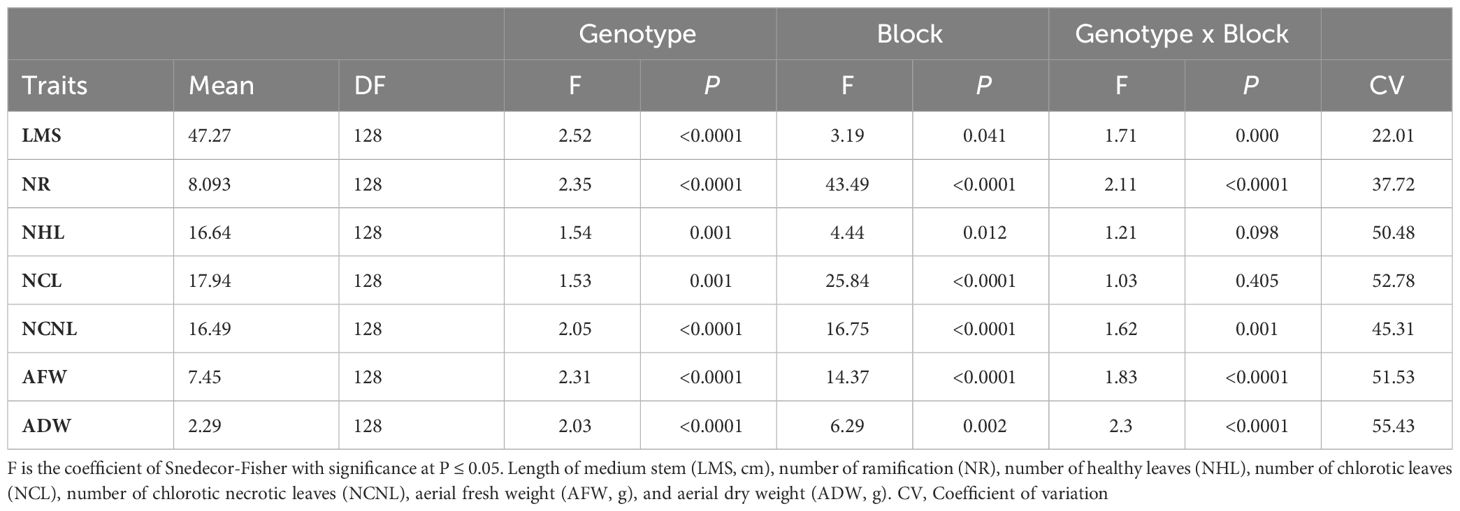
Table 1 Influence of accession, block, and the interaction between accession and block on the variability of morphological traits measured for alfalfa (Medicago sativa) accessions cultivated under the combination of salinity and Phoma medicaginis (strain Pm8) infection.
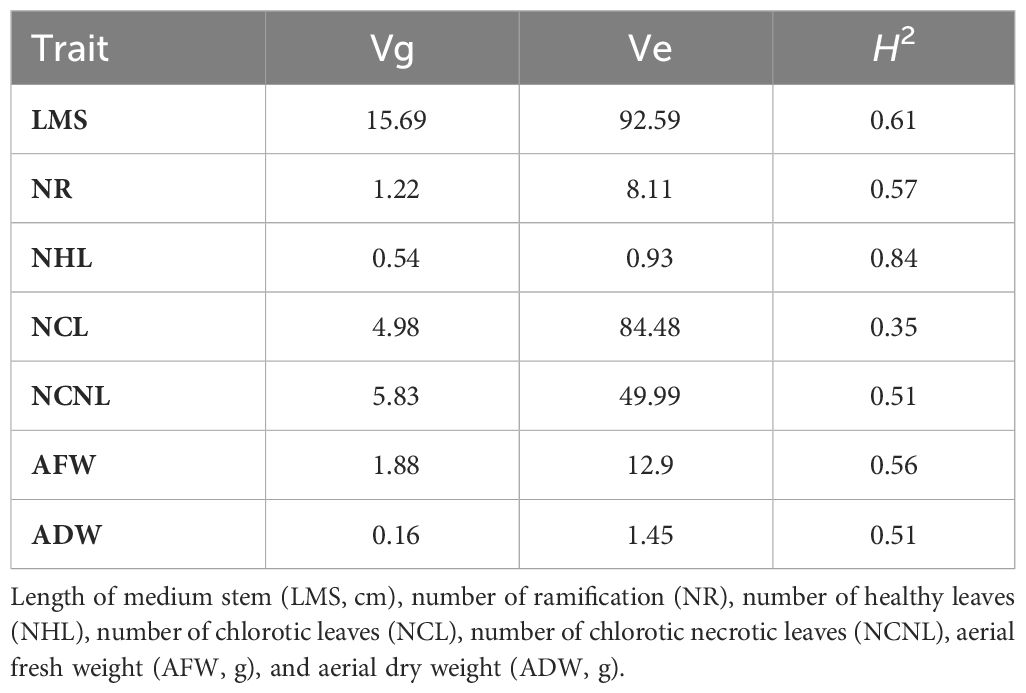
Table 2 Genotypic variation (Vg), environmental variation (Ve), and heritability (H2) of seven morphological traits in alfalfa (Medicago sativa) accessions cultivated under the combination of salinity and P. medicaginis (strain Pm8) infection.
Of the 21 possible pairwise correlations, 20 were significant and positive (Table 3). Noticeably, a significant positive correlation was also observed between NHL and NCNL (r=0.668; P ≤ 0.0001) which seems to indicate that NCNL is not an independent parameter due to the effect of combined stresses but it is simply related to the potential number of healthy leaves that the genotype is able to express.
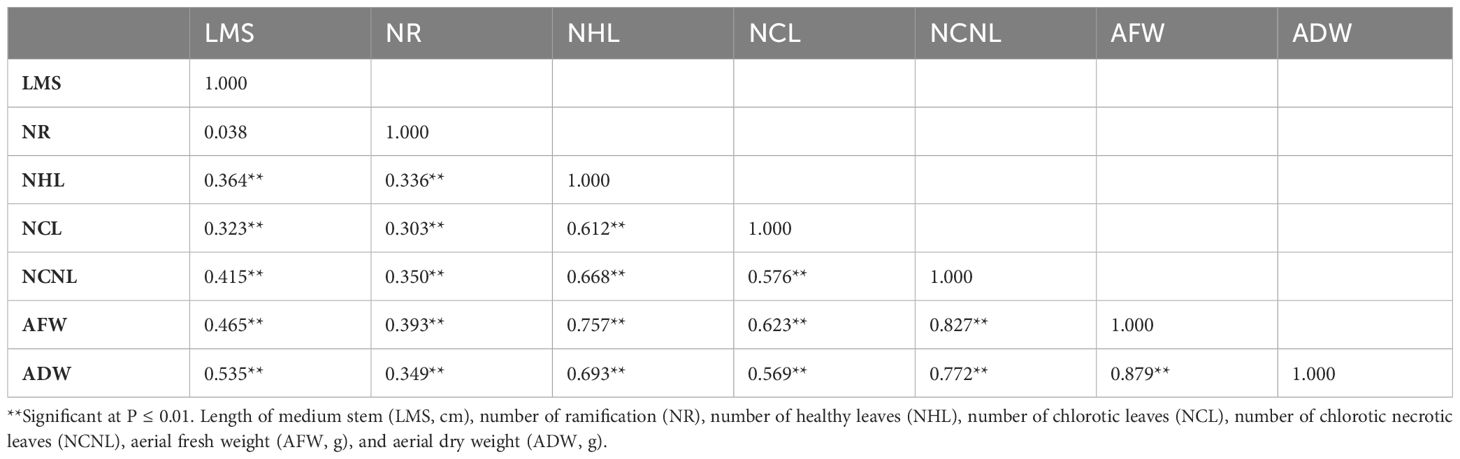
Table 3 Correlation matrix of seven morphological traits for alfalfa (Medicago sativa) accessions cultivated under the combination of salinity and Phoma medicaginis (strain Pm8) infection.
3.2 Principal component analysis and cluster analysis
PCA explained most of the variation among genotypes by the first two axes (PC1 and PC2), which represented 61.15% and 16.81%, of the total variance, respectively (Figure 1). All the recorded characters were positively correlated with PC1 (r > 0.54) except NCNL, which displayed a high negative association with this axis (Figure 1A). Surprisingly, NCNL had a contrasting bearing on PC1 compared with the two other leaf-related traits (NHL and NCL) despite the positive phenotypic correlations among the three leaf traits (Table 2). PC1 was strictly associated with the two traits related to biomass production (AFW and ADW), whereas the second axis was somewhat associated to the traits related to plant morphology (NR) (Figure 1A). The genotypes were found mostly in an extensive overlap around the origin in both sides of PC1 and PC2 with a few scattered genotypes. The two outlier genotypes R20 and R224, in particular, showed peculiar traits that contributed to their position on the PC plan. R20 had the highest number of ramification and R224 the maximum AFW, ADW, LMS, and the minimum NCL followed by R166, R165 and R174 (Figure 1B). The rest of the genotypes appeared not clearly separated from each other on the basis of the measured traits and were more or less equally partitioned into the four quadrants of the PCA (Figures 1B, C).
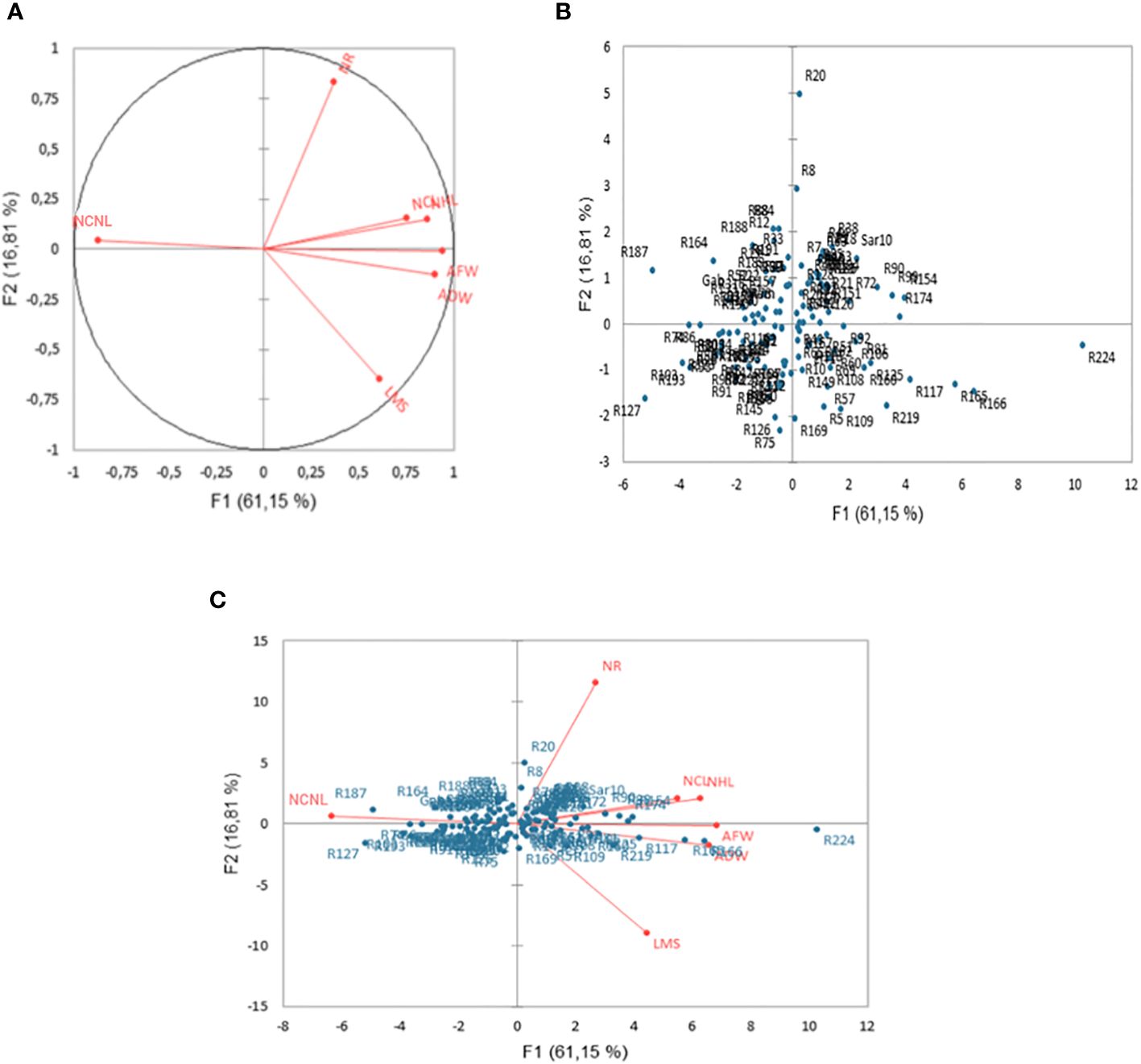
Figure 1 Two-dimensional PCA plot showing the relationships among measured traits (A) for the accessions (B) and Biplot (genotype, trait) (C) representing alfalfa (Medicago sativa) under a combination of salinity and Phoma medicaginis (Strain Pm8) infection. Length of medium stem (LMS, cm), number of ramifications (NR), number of healthy leaves (NHL), number of chlorotic leaves (NCL), number of chlorotic necrotic leaves (NCNL), aerial fresh weight (AFW, g), and aerial dry weight (ADW, g).
3.3 GWAS and marker-trait association analyses
The Manhattan plots (Figures 2, 3) illustrate the p-values (transformed to negative logarithmic values) of markers in relation to their genetic positions for each trait. We identified 110 significant SNPs across the seven recorded traits. These SNPs were distributed across all chromosomes, with the maximum of 24 SNPs that were located on chromosome 3 and the minimum of 10 markers on chromosome 2.
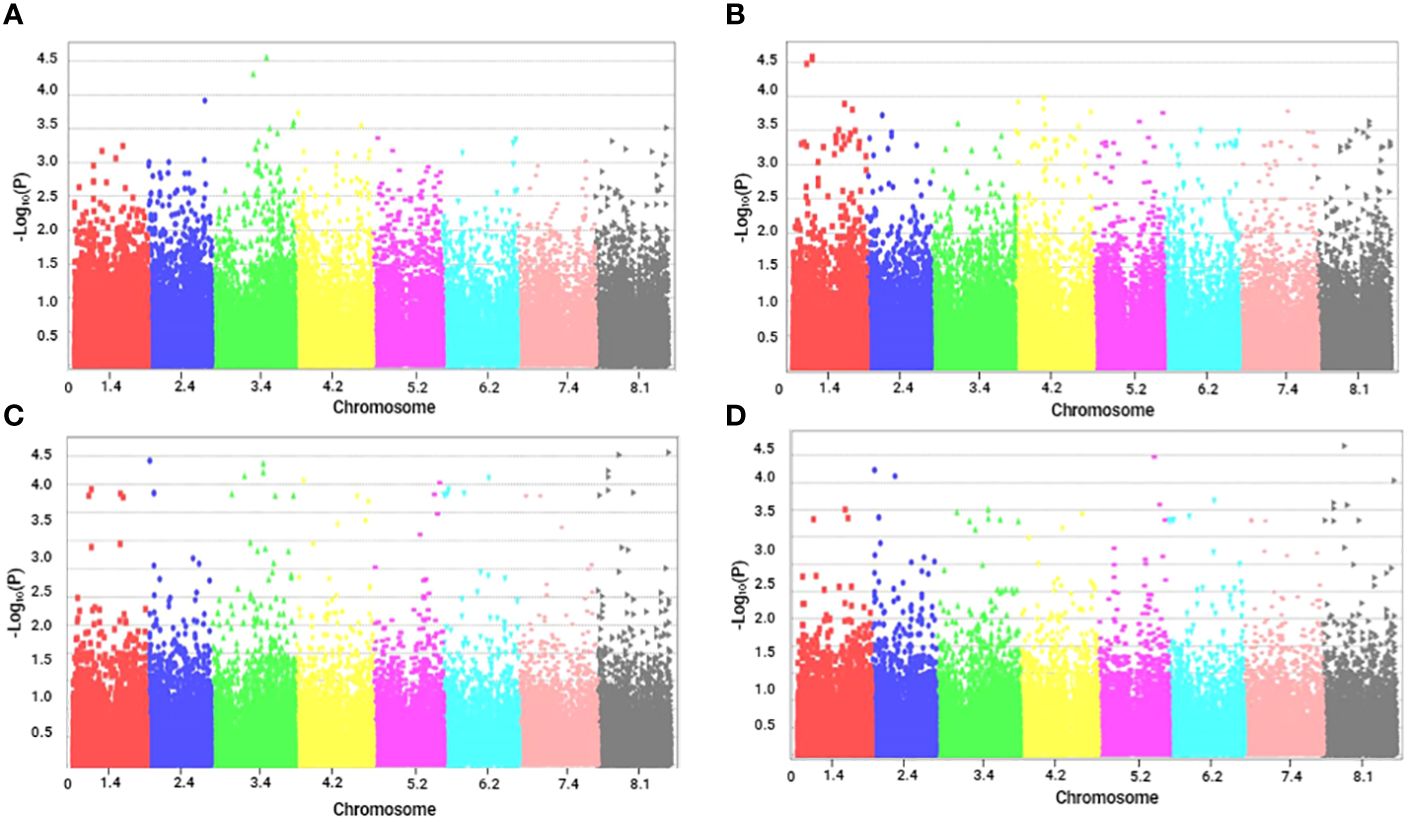
Figure 2 Manhattan plots of marker–trait associations for tolerance traits of alfalfa (M. sativa) to a combination of salinity and Phoma medicaginis (strain Pm8) infection. Significant markers that passed a cutoff log (p-value) of 4. The X-axis represents the physical location of SNPs on chromosomes (color-coded) and the Y-axis represents the −log10 p-value of SNP phenotype associations. (A) length of the main stem (LMS, cm); (B) number of ramifications (NR), (C) aerial fresh weight (AFW, g) and (D) aerial dry weight (ADW, g).
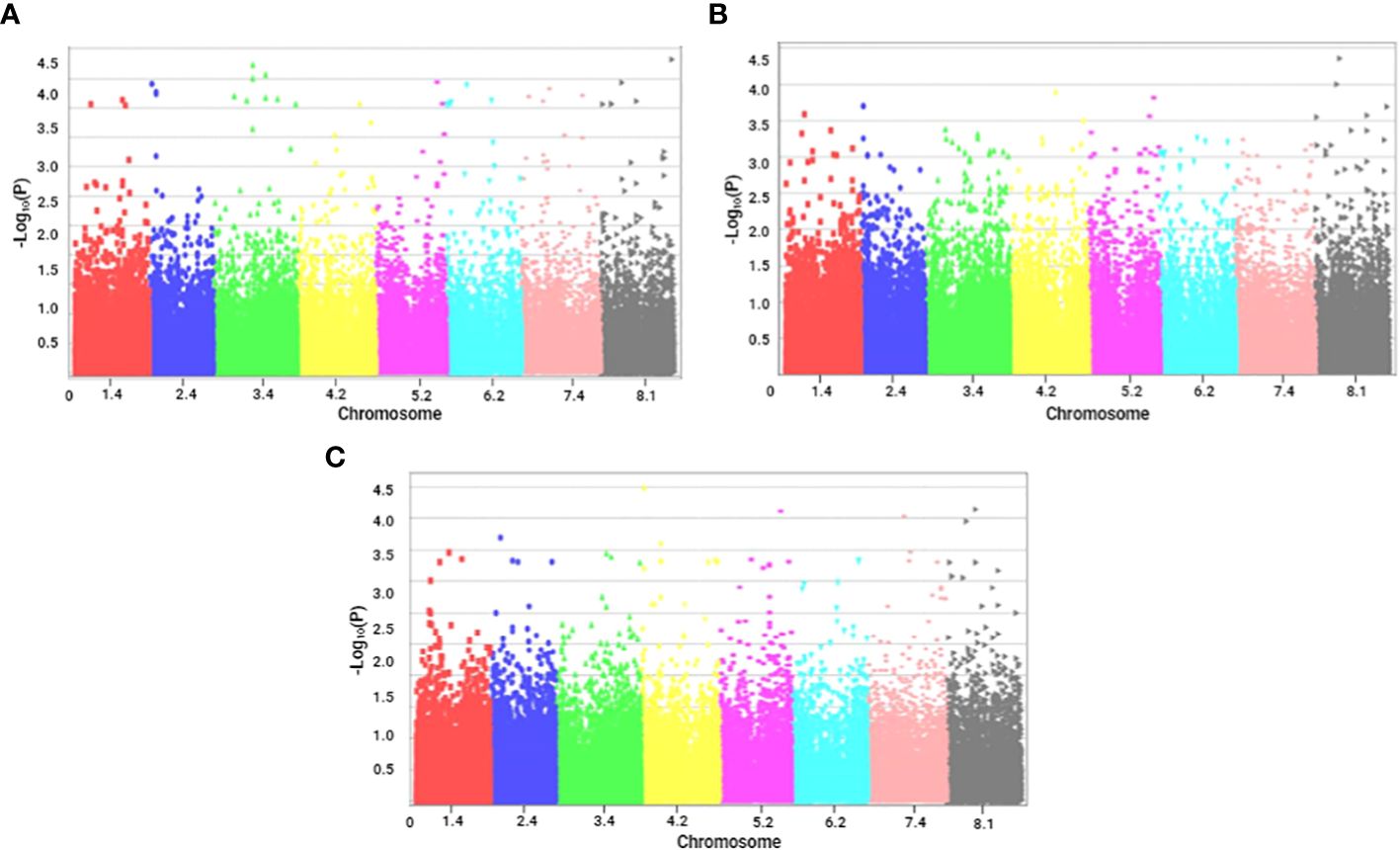
Figure 3 Manhattan plots of marker–trait associations for tolerance traits of alfalfa (M. sativa) to a combination of salinity and Phoma medicaginis (Strain Pm8) infection. Significant markers that passed a cutoff log (p-value) of 4. The X-axis represents the physical location of SNPs on chromosomes (color-coded) and the Y-axis represents the −log10 p-value of SNP phenotype associations. (A) number of chlorotic leaves (NCL); (B) number of chlorotic necrotic leaves (NCNL); (C) number of healthy leaves (NHL).
Among the growth and biomass traits, the characters LMS (Figure 2A) and NR (Figure 2B) had two significant markers each, distributed on chromosome 1 and 3, respectively. The highest number of significant markers (35) was observed for AFW (Figure 2C), followed by 28 for ADW (Figure 2D).
Regarding leaf infection traits, the highest number of significant markers (34) was observed for NCL (Figure 3A), 8 significant markers were noted for NCNL (Figure 3B), and two significant markers distributed on chromosome 8 were found for NHL (Figure 3C),
3.4 Assigning significant markers to known genes
The results revealed that 27 SNPS within 20 putative M. truncatula genes were associated with the recorded traits, as indicated in Table 4.
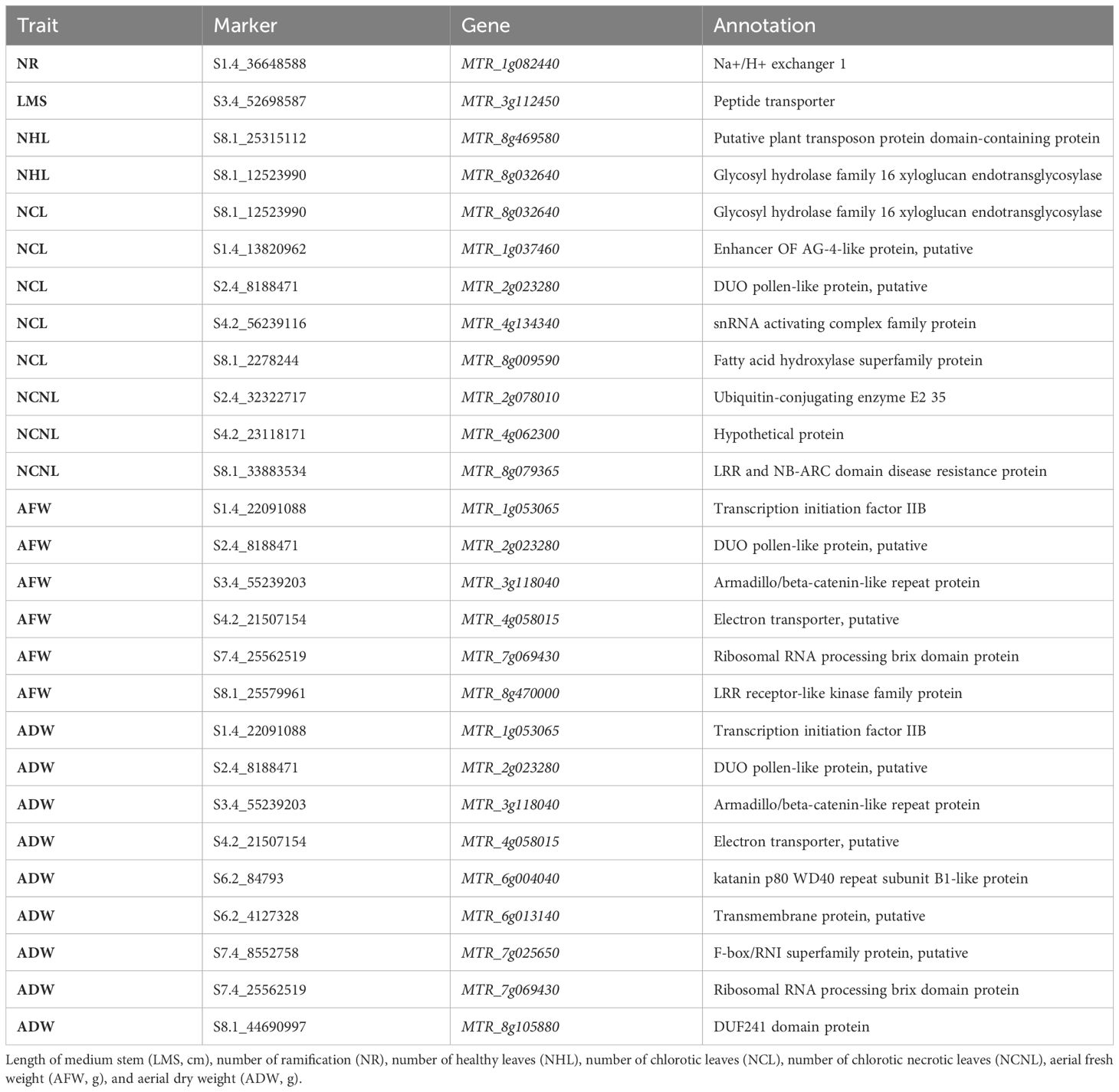
Table 4 Significant markers associated with seven morphological traits in alfalfa (M. sativa) accessions cultivated under the combination of salinity and P. medicaginis (Strain Pm8), identified using the M. truncatula genome as a reference.
Regarding the growth parameters, for the length of the main stem, one SNP on chromosome 3 (at locus 52698587) is linked to the homologous gene (MTR_3g112450), which encodes a peptide transporter. One gene (MTR_1g082440) is linked to one SNP located at locus 36648588 on chromosome 1, associated with number of ramifications, and encodes a Na+/H+ exchanger.
For the biomass parameters, five common genes were identified in both AFW and ADW traits, with the highest significant marker on chromosome 7 at locus 25562519 linked to the gene MTR_7g069430, which encodes the ribosomal RNA processing brix domain protein. Four other genes were identified on chromosomes 1, 2, 3, and 4, at positions 22091088, 8188481, 55239203, and 1507154, respectively. These genes include transcription initiation factor IIB (MTR_1g053065), DUO pollen-like protein putative (MTR_2g023280), armadillo/beta-catenin-like repeat protein (MTR_3g118040), and electron transporter putative (MTR_4g058015). The gene MTR_8g470000 on chromosome 8 at position 25579961 was associated with AFW only. It encodes for the LRR receptor-like kinase family protein.
However, the ADW trait differed from the AFW trait for the presence of five more significant markers that are linked to four genes. Two of these genes are located on chromosome 6 (MTR_6g004040 and MTR_6g013140), encoding a katanin p80 WD40 repeat subunit B1-like protein and a transmembrane protein, respectively. Additionally, we identified one SNP on chromosome 7 linked to the homologous gene MTR_7g025650 (F-box/RNI superfamily protein, putative), and another SNP on chromosome 8 linked to the homologous gene MTR_7g025650 (DUF241 domain protein).
With respect to infection parameters, the SNPs associated with the number of healthy leaves located at positions 25315112 and 12523990 on the same chromosome 2 were in the coding region of the putative plant transposon protein domain-containing protein (MTR_8g469580) and the glycosyl hydrolase family 16 xyloglucan endotransglycosylase linked to the homologous gene MTR_8g032640.
Five SNPs were significantly associated with the number of chlorotic leaves, distributed on chromosomes 1, 2, 4, and 8. One was the marker S1.4_13820962, which is close to the enhancer OF AG-4-like protein, putatively homologous to gene MTR_1g037460. The DUO pollen-like protein, putatively homologous to gene MTR_2g023280, was linked to the marker S2.4_8188471, and the snRNA activating complex family protein, homologous to gene MTR_4g134340, was linked to the marker S4.2_56239116. On chromosome 8, two markers (S8.1_12523990 and S8.1_2278244) were linked to glycosyl hydrolase family 16 xyloglucan endotransglycosylase and fatty acid hydroxylase superfamily protein, linked to homologous genes MTR_8g032640 and MTR_8g009590, respectively.
For NCNL, we identified three genes. One of them is homologous to the gene encoding ubiquitin-conjugating enzyme E2 35, located on chromosome 2. Another is homologous to the gene encoding a hypothetical protein, located on chromosome 4 (Medtr4g009410). The third gene encodes an LRR and NB-ARC domain disease resistance protein, located on chromosome 8.
4 Discussion
Analyzing the morpho-physiological variation in alfalfa plants under stress conditions has been regarded as a reliable method for assessing its stress tolerance. This process represents a pivotal phase in the development of forthcoming breeding programs (Annicchiarico, 2006; Annicchiarico et al., 2022). Salinity and Phoma medicaginis infection are major issues for alfalfa that affect its growth and productivity. Previous studies have demonstrated that the alfalfa response to salinity varies greatly among accessions (Badri et al., 2021; Jabri et al., 2021).
This study represented an unprecedented example of exploring the morphological variation of alfalfa in response to the simultaneous presence of biotic and abiotic stressors. Our findings revealed a remarkable range of morpho-physiological variation within the examined collection of alfalfa genotypes when subjected to simultaneous stressors (salt and strain Pm8 infection) and, overall, the current results demonstrated significant genetic variation for most of the recorded traits. These findings are in accordance with the conclusions drawn by Annicchiarico et al. (2022) on the same population grown under drought stress. Badri et al. (2023) tested the effect of P. medicaginis on the variation in agronomic traits of different Medicago species, reporting that most of the variation in the measured parameters was explained by the infection treatment. Zaidi et al. (2021) demonstrated that the number of healthy leaves (recorded among the infection parameters of alfalfa genotypes) varied significantly among the genotypes infected by P. medicaginis, which is consistent with the current observations.
In this study, the highest heritability was found for NHL, suggesting that this trait is largely governed by genetic effects. Badri et al. (2023) found that most evaluated traits in infected Medicago species had high heritability values, suggesting their potential as useful criteria for identifying P. medicaginis-tolerant lines. Djaman et al. (2020) found a moderate heritability for the length of main stem in alfalfa, owing to the environmental effects affecting this character besides the genetic ones. The positive correlations observed among phenotypic traits in alfalfa accessions under combined stress of salinity and P. medicaginis infection suggest that these conditions influence multiple traits similarly, leading to correlated responses. The increase in chlorotic and chlorotic necrotic leaves may indicate an enhanced immune response to fungal infection, implying a complex interaction between abiotic and biotic stressors. This interaction may increase plant susceptibility to fungal infection or worsen the effects of saline stress on plant physiology. Additionally, the correlations between phenotypic traits may indicate adaptive strategies employed by plants to mitigate environmental stress damage, such as increasing chlorotic leaves.
In this study, GWAS allowed to identify 110 SNP markers associated with seven morphological traits of alfalfa grown under combined salinity and P. medicaginis infection. To our knowledge, this was the first attempt to locate genes regulating morpho-physiological traits in alfalfa under simultaneous abiotic (salinity) and biotic (strain Pm8 infection) stresses. Liu and Yu (2017) identified 42 SNPs significantly associated with salt tolerance using 198 alfalfa accessions with four physiological traits (dry weight, plant height, leaf chlorophyll content and stomatal conductance). Only 10 SNPs have been identified in a panel of 179 lines of alfalfa for Verticillium wilt tolerance (Yu et al., 2017). Several research studies used diploidized models and the reference genome of M. truncatula to identify putative genes in alfalfa under stress (Biazzi et al., 2017; Medina et al., 2020; Lin et al., 2021).
In this study, 24 putative genes have been linked to homologue molecular and biological responses to combined stresses. The GWAS for the length of the main stem showed a close association of this character with the gene MTR_3g112450, which encodes a peptide transporter necessary for the organic nitrogen (N) supplies and mediated N use efficiency under salt stress (Fang et al., 2017). Similarly, Wang et al. (2022) demonstrated the high expression and regulation of the peptide transporter family induced in alfalfa grown in salinity stress. Overall, the peptide transporter plays a pivotal role in our study to alleviate salt stress in alfalfa by facilitating the uptake of organic nitrogen compounds, which contribute to osmotic adjustment, antioxidant defense, nutrient uptake, and gene regulation (Chen et al., 2021). This coordinated response not only boosts the elongation of the main stems but also enhances the plant’s ability to withstand and recover from salt-induced damage (Wang et al., 2022).
Putative candidate genes that were linked to SNPs located in the first chromosome encode for the Na+/H+ exchanger, and were associated with the number of ramifications, which plays an important role for alfalfa salt tolerance (Guiza et al., 2022). The Na+/H+ exchanger, located in both the plasma and vacuolar membranes, plays a pivotal role in plants by actively expelling excess sodium ions (Na+) from the cytosol or sequestering them into the tonoplast. This mechanism is essential for mitigating the harmful effects of sodium toxicity (Yang et al., 2005). A study conducted by Li et al. (2011) showed the effective expression of SsNHX (Na+/H+ exchanger) in transgenic alfalfa, which could grow in high concentrations of NaCl (up to 400 mM). In addition, Lei et al. (2015) reported that alfalfa genotypes tolerant to salinity maintain moderately more stable expression levels of genes related to Na+/K+ transport and Na+/H+ exchanger. However, tonoplast and plasma membrane antiporters actively remove sodium ions from the cytosol. This process is powered by the proton-motive force, which is produced by the H+-ATPase in the plasma membrane, as well as the H+-ATPase and H+-pyrophosphatase in the vacuolar membrane. Notably, research has demonstrated that Na+/H+ exchanger was identified within the late endosome/vacuolar compartment, and it has been suggested that its functions may encompass sodium transport, control of water movement, regulation of vesicle volume, and possibly play a role in osmo-tolerance (Silva and Gerós, 2009). Our finding suggested that Na+/H+ exchanger gene holds significant importance in enhancing the proliferation of ramification in alfalfa under salt stress conditions. Its pivotal role lies in facilitating the exchange of nitrogen and hydrogen ions, crucial for mitigating the detrimental effects of salinity on plant growth (Li et al., 2018). This gene ensures the maintenance of optimal nutrient levels and pH balance within plant cells (Guiza et al., 2022), promoting the development of a greater number of ramifications in alfalfa even amidst challenging salt stress environments.
GWAS identified the presence of five common markers associated with both AFW and ADW. Among these markers, MTR_1g053065 was linked to a homologous of the transcription initiation factor IIB, which was found to be involved in pollen and endosperm development in Arabidopsis (Dubos et al., 2010). Similarly, Xu et al. (2019) noted that the comparative transcriptome analysis of five alfalfa genotypes grown under cold stress revealed a gene encoding TFIIB (transcription factor IIB). This marker was differentially expressed in Brassica napus under drought stress using GWAS mapping (Khanzada et al., 2020). In addition, the putative electron transporter was significantly associated with ADW and AFW. MTR_4g058015 is also involved in the regulation of Na+/K+ in alfalfa under salt stress (Li et al., 2010), and was also reported in salt tolerance of rice (Patishtan et al., 2018). Thus, the essential role of TFIIB in our study resulted in the regulation of ionic transport and osmotic adjustment leading to the enhancement of nutrient acquisition by alfalfa grown under salinity.
Furthermore, the association analysis for the ADW revealed that this trait was highly associated with the putative genes encoding for F-box/RNI superfamily protein on chromosome 7. Lechner et al. (2006) noted that F-box protein is a crucial element within the ubiquitin ligase complex, facilitating the ubiquitination process of specific target proteins. This molecular mechanism plays a pivotal role in regulating cellular processes associated with plant defense to fungi infection. This may explain the high significance of the marker encoding this gene in the current study, as it mediates the degradation of specific proteins involved in susceptibility pathways. Through targeted protein degradation, the F-box protein regulates key factors that contribute to the virulence of P. medicaginis or the susceptibility of alfalfa. Similarly, a candidate gene encoding F-box protein was identified in alfalfa, using GWAS, as a negative regulator of resistance to root rot caused by Aphanomyces spp (Bonhomme et al., 2014).
The plant NBS_LRR gene family contains a large class of disease resistance genes (Yu et al., 2020). In this study, a LRR receptor-like kinase family protein was positively associated with AFW and a LRR and NB-ARC domain disease resistance protein was linked to a marker located in chromosome 8 for NCNL. These genes encode proteins that are involved in plant disease resistance (Li et al., 2019). GWAS revealed multiple QTLs related to Verticillium wilt resistance on alfalfa chromosome 8 (Yu et al., 2017). These results imply that alfalfa resistance can be improved by stacking major R genes/QTLs for multiple pathogens associated with AFW trait (Fuchs, 2017).
The annotations of the homologue gene MTR_4g062300 (S4.1_23118171) associated with NCNL pertain to hypothetical proteins with unknown functions. Earlier research has revealed that genes responsible for these hypothetical proteins were notably upregulated either at the transcript or translation level following prolonged exposure to high salt levels. This suggests that certain hypothetical proteins play a role in enhancing salt tolerance in forage plants, as demonstrated by Lin et al. (2021).
Five genes associated with the NCL trait were found to be located on M. truncatula chromosomes 1, 2, 4 and 8. Among them, one encodes the protein involved in DUO pollen-like protein. DUO acquired sperm lineage-specific expression in the common ancestor of land plants leading to sperm with distinct morphologies (Warman et al., 2020). The other one encodes for fatty acid hydroxylase superfamily protein, which has a metabolism control function (Marchler-Bauer et al., 2017). Preserving membrane integrity and fluidity is a crucial aspect of stress adaptation. This involves the collaboration between membrane components and the lipid composition to ensure the resilience of the membrane structure when facing challenging conditions. In this study, under combined stress, alfalfa cell membrane fluidity significantly decreased by the appearance of chlorosis spots on leaves, which is a symptom of salinity stress. As a result, the normal physiological function of membrane-bound proteins is altered (Liu et al., 2015). Previous studies reported the presence of the same putative gene using the GWAS approach in maize grown at low temperatures (Zhang et al., 2020), as well as in wheat under salt and drought stresses (Urbanavičiūtė et al., 2021).
A glycosyl hydrolase family 16 xyloglucan endotrans-glycosylase was found to be linked to the marker S8.1_12523990 associated to NHL. Recent studies have shown that xyloglucan endotrans-glycosylase, a prominent cell wall modifying enzyme, causes cell expansion, with loosening/reinforcing cell walls, particularly in response to environmental stress (Panahabadi et al., 2022). They demonstrated that glycosyl hydrolase family 16 xyloglucan endotrans-glycosylase was included in brassinolide hormone biosynthesis and pathways. Besides, Konkolewska et al. (2023) mentioned that the glycosyl hydrolase family 16 xyloglucans are intricate hemi-cellulosic polysaccharides that undergo biosynthesis within the Golgi apparatus before being transported to the cell wall of alfalfa. These molecules play essential roles in processes such as cell growth and expansion, energy metabolism, and signaling. Our finding suggested that the glycosyl hydrolase family 16 xyloglucan endotrans-glycosylase plays a crucial role in maintaining the number of healthy leaves in alfalfa during fungal infection by enhancing cell wall integrity, activating defense responses, regulating leaf morphology, and promoting nutrient transport.
5 Conclusions
Understanding stress tolerance mechanisms and genes in alfalfa helps identifying suitable genotypes for regions with high level of salinity and P. medicaginis infection, ensuring forage quality and quantity. Most traits assessed in the current study showed high heritability due to genetic factors, suggesting that the five alfalfa genotypes identified as tolerant to the combined stresses could be a valuable resource for the breeding. Using GWAS, we identified a total of 24 significant markers associated with seven traits. Notably, markers such as S8.1_12523990, S4.1_23118171, and S8.1_2278244 were linked to traits related to cell wall rigidity and cellular metabolism. We also found a marker associated with ionic transport. Some markers were linked to the NBS_LRR gene family, which plays a role in biomass and infection parameters, possibly contributing to alfalfa disease resistance.
The validation of the identified markers and genes through functional studies and biochemical assays, coupled with the exploration of gene overexpression techniques, represents a crucial step in marker-assisted selection (MAS). This process offers potential avenues for future research, such as investigating gene editing techniques to manipulate key genes associated with combined stress tolerance. Similarly, the most promising combined-tolerant alfalfa genotypes, containing numerous candidate genes for target traits, could be selected as parents in future crosses aimed at producing genetically superior alfalfa varieties with possibly multiple candidate genes for salinity tolerance, thereby allowing for sustained productivity even in saline-affected soils. Besides, resistance to P. medicaginis infection could mitigate crop losses and reduce the need for chemical interventions. However, ensuring the stability and adaptability of stress-tolerant traits across different environmental conditions and geographic regions will be essential for the widespread adoption of new alfalfa varieties
Data availability statement
The original contributions presented in the study are included in the article/supplementary materials. Further inquiries can be directed to the corresponding author.
Author contributions
WM: Data curation, Formal analysis, Software, Validation, Writing – original draft, Writing – review & editing. CJ: Conceptualization, Data curation, Methodology, Validation, Writing – review & editing. NJ: Data curation, Methodology, Validation, Writing – review & editing. NM: Methodology, Validation, Writing – review & editing. AG: Methodology, Validation, Writing – review & editing. NZ: Methodology, Validation, Writing – review & editing. GB: Methodology, Validation, Writing – review & editing. EK: Methodology, Validation, Writing – review & editing. ND: Resources, Validation, Writing – review & editing. LP: Resources, Validation, Writing – review & editing. MH: Conceptualization, Validation, Writing – review & editing. PA: Resources, Validation, Writing – review & editing. MS: Formal analysis, Software, Validation, Writing – review & editing. NL: Conceptualization, Funding acquisition, Validation, Writing – review & editing. MB: Conceptualization, Data curation, Funding acquisition, Investigation, Methodology, Project administration, Supervision, Validation, Writing – original draft, Writing – review & editing.
Funding
The authors declare financial support was received for the research, authorship, and/or publication of this article. This research was funded by the Tunisian-South African project (2019-2024) and the Tunisian Ministry of Higher Education and Scientific Research (LR15 CBBC02). The plant material was generated by the FP7-ArimNet project REFORMA funded by the Governments of Italy, Algeria, Morocco and Tunisia.
Acknowledgments
We would like to express our gratitude to Dr. Nelson Nazzicari (CREA) for his generation of SNP data out of genotyping-by-sequencing rough data, and Dr. Sabrine Hdira (LPE, CBBC) for her valuable discussions on GWAS analysis.
Conflict of interest
The authors declare that the research was conducted in the absence of any commercial or financial relationships that could be construed as a potential conflict of interest.
Publisher’s note
All claims expressed in this article are solely those of the authors and do not necessarily represent those of their affiliated organizations, or those of the publisher, the editors and the reviewers. Any product that may be evaluated in this article, or claim that may be made by its manufacturer, is not guaranteed or endorsed by the publisher.
References
Ahsan, M. Z., Majidano, M. S., Bhutto, H., Soomro, A. W., Panhwar, F. H., Channa, A. R., et al. (2015). Genetic variability, coefficient of variance, heritability and genetic advance of some Gossypium hirsutum L. accessions. JAS 7, 147. doi: 10.5539/jas.v7n2p147
Annicchiarico, P. (2006). Diversity, genetic structure, distinctness and agronomic value of Italian lucerne (Medicago sativa L.) landraces. Euphytica 148, 269–282. doi: 10.1007/s10681-005-9024-0
Annicchiarico, P., Barrett, B., Brummer, E. C., Julier, B., Marshall, A. H. (2015a). Achievements and challenges in improving temperate perennial forage legumes. Crit. Rev. Plant Sci. 34, 327–380. doi: 10.1080/07352689.2014.898462
Annicchiarico, P., Nazzicari, N., Bouizgaren, A., Hayek, T., Laouar, M., Cornacchione, M., et al. (2022). Alfalfa genomic selection for different stress-prone growing regions. Plant Genome 15, e20264. doi: 10.1002/tpg2.20264
Annicchiarico, P., Nazzicari, N., Li, X., Wei, Y., Pecetti, L., Brummer, E. C. (2015b). Accuracy of genomic selection for alfalfa biomass yield in different reference populations. BMC Genom. 16, 1020. doi: 10.1186/s12864-015-2212-y
Annicchiarico, P., Pecetti, L., Abdelguerfi, A., Bouizgaren, A., Carroni, A. M., Hayek, T., et al. (2011). Adaptation of landrace and variety germplasm and selection strategies for lucerne in the Mediterranean basin. Field. Crops. Res. 120, 283–291. doi: 10.1016/j.fcr.2010.11.003
Arraouadi, S., Badri, M., Zitoun, A., Huguet, T., Aouani, M. E. (2011). Analysis of NaCl stress response in Tunisian and reference lines of Medicago truncatula. Russ. J. Plant Physio. 58, 316–323. doi: 10.1134/S1021443711020026
Badri, M., Ayadi, A., Mahjoub, A., Benltoufa, A., Chaouachi, M., Ranouch, R., et al. (2023). Variability in responses to phoma medicaginis infection in a Tunisian collection of three annual Medicago species. Plant Pathol. J. 39, 171. doi: 10.5423/PPJ.OA.09.2022.0134
Badri, M., Rafik, K., Jabri, C., Ludidi, N. (2021). Analysis of salinity tolerance in two varieties of Medicago sativa at the vegetative stage. J. Oasis Agric. Sustain. Dev. 3, 25–29. doi: 10.56027/JOASD.spiss042021
Benjamini, Y., Hochberg, Y. (1995). Controlling the false discovery rate: A practical and powerful approach to multiple testing. J. R. Stat. Soc Ser. B Methodol 57, 289–300. doi: 10.1111/j.2517-6161.1995.tb02031.x
Biazzi, E., Nazzicari, N., Pecetti, L., Brummer, E. C., Palmonari, A., Tava, A., et al. (2017). Genome-wide association mapping and genomic selection for alfalfa (Medicago sativa) forage quality traits. PloS One 12, e0169234. doi: 10.1371/journal.pone.0169234
Bonhomme, M., André, O., Badis, Y., Ronfort, J., Burgarella, C., Chantret, N., et al. (2014). High-density genome-wide association mapping implicates an F-box encoding gene in Medicago truncatula resistance to Aphanomyces euteiches. New Phytol. 201, 1328–1342. doi: 10.1111/nph.12611
Bradbury, P. J., Zhang, Z., Kroon, D. E., Casstevens, T. M., Ramdoss, Y., Buckler, E. S. (2007). and TASSEL: software for association mapping of complex traits in diverse samples. Bioinformatics 23, 2633–2635. doi: 10.1093/bioinformatics/btm308
Castell-Miller, C. V., Zeyen, R. J., Samac, D. A. (2007). Infection and development of Phoma medicaginis on moderately resistant and susceptible alfalfa genotypes. Can. J. Plant Pathol. 29, 290–298. doi: 10.1080/07060660709507472
Chen, Z., Niu, J., Guo, Z., Sui, X., Xu, N., Kareem, H. A., et al. (2021). Graphene enhances photosynthesis and the antioxidative defense system and alleviates salinity and alkalinity stresses in alfalfa (Medicago sativa L.) by regulating gene expression. Environ. Sci. Nano. 8, 2731–2748. doi: 10.1039/D1EN00257K
Djaman, K., Owen, C., Koudahe, K., O’Neill, M. (2020). Evaluation of different fall dormancy-rating alfalfa cultivars for forage yield in a semiarid environment. Agronomy 10, 146. doi: 10.3390/agronomy10010146
Djebali, N. (2008). Etude des mécanismes de résistance de la plante modèle Medicago truncatula vis-à-vis de deux agents pathogènes majeurs des légumineuses cultivées : Phoma medicaginis et Aphanomyces euteiches. Université Toulouse III - Paul Sabatier, Castanet-Tolosan, France. 191.
Djébali, N., Aribi, S., Taamalli, W., Arraouadi, S., Aouani, M. E., Badri, M. (2013). Natural variation of Medicago truncatula resistance to Aphanomyces euteiches. Eur. J. Plant Pathol. 135, 831–843. doi: 10.1007/s10658-012-0127-x
Dubos, C., Stracke, R., Grotewold, E., Weisshaar, B., Martin, C., Lepiniec, L. (2010). MYB transcription factors in Arabidopsis. Trends Plant Sci. 15, 573–581. doi: 10.1016/j.tplants.2010.06.005
Fang, Z., Bai, G., Huang, W., Wang, Z., Wang, X., Zhang, M. (2017). The rice peptide transporter OsNPF7.3 is induced by organic nitrogen, and contributes to nitrogen allocation and grain yield. Front. Plant Sci. 8. doi: 10.3389/fpls.2017.01338
Ferchichi, Y., Sakhraoui, A., Ltaeif, H. B., Ben Mhara, Y., Elimem, M., Ben Naceur, M., et al. (2021). Eco-geographical, morphological and molecular characterization of a collection of the perennial endemic species Medicago tunetana (Murb.) A.W. Hill (Fabaceae) from Tunisia. Plants 10, 1923. doi: 10.3390/plants10091923
Filho, C. C. F., Andrade, M. H. M. L., Nunes, J. A. R., Jarquin, D. H., Rios, E. F. (2023). Genomic prediction for complex traits across multiples harvests in alfalfa (Medicago sativa L.) is enhanced by enviromics. Plant Genome 16, e20306. doi: 10.1002/tpg2.20306
Fuchs, M. (2017). Pyramiding resistance-conferring gene sequences in crops. Curr. Opin. Virol. 26, 36–42. doi: 10.1016/j.coviro.2017.07.004
Guiza, M., Benabdelrahim, M. A., Brini, F., Haddad, M., Saibi, W. (2022). Assessment of alfalfa (Medicago sativa L.) cultivars for salt tolerance based on yield, growth, physiological, and biochemical traits. J. Plant Growth Regul. 41, 3117–3126. doi: 10.1007/s00344-021-10499-9
Haddoudi, I., Mhadhbi, H., Gargouri, M., Barhoumi, F., Romdhane, S. B., Mrabet, M. (2021). Occurrence of fungal diseases in faba bean (Vicia faba L.) under salt and drought stress. Eur. J. Plant Pathol. 159, 385–398. doi: 10.1007/s10658-020-02169-5
Ibidhi, R., Frija, A., Jaouad, M., Ben Salem, H. (2018). Typology analysis of sheep production, feeding systems and farmers strategies for livestock watering in Tunisia. Small Rumin. Res. 160, 44–53. doi: 10.1016/j.smallrumres.2018.01.010
Jabri, C., Zaidi, N., Maiza, N., Rafik, K., Ludidi, N., Badri, M. (2021). Effects of salt stress on the germination of two contrasting Medicago sativa varieties. J. Oasis Agric. Sustain. Dev. 3, 13–18. doi: 10.56027/JOASD.spiss022021
Kaiwen, G., Zisong, X., Yuze, H., Qi, S., Yue, W., Yanhui, C., et al. (2020). Effects of salt concentration, pH, and their interaction on plant growth, nutrient uptake, and photochemistry of alfalfa (Medicago sativa) leaves. Plant Signal. Behav. 15, 1832373. doi: 10.1080/15592324.2020.1832373
Kang, Y., Sakiroglu, M., Krom, N., Stanton-Geddes, J., Wang, M., Lee, Y.-C., et al. (2015). Genome-wide association of drought-related and biomass traits with HapMap SNPs in Medicago truncatula. Plant Cell Environ. 38, 1997–2011. doi: 10.1111/pce.12520
Khanzada, H., Wassan, G. M., He, H., Mason, A. S., Keerio, A. A., Khanzada, S., et al. (2020). Differentially evolved drought stress indices determine the genetic variation of Brassica napus at seedling traits by genome-wide association mapping. J. Adv. Res. 24, 447–461. doi: 10.1016/j.jare.2020.05.019
Konkolewska, A., Phang, S., Conaghan, P., Milbourne, D., Lawlor, A., Byrne, S. (2023). Genomic prediction of seasonal forage yield in perennial ryegrass. Grassland Res. 2 (3), 841882. doi: 10.1002/glr2.12058
Larkin, D. L., Lozada, D. N., Mason, R. E. (2019). Genomic selection—considerations for successful implementation in wheat breeding programs. Agronomy 9, 479. doi: 10.3390/agronomy9090479
Lechner, E., Achard, P., Vansiri, A., Potuschak, T., Genschik, P. (2006). F-box proteins everywhere. Curr. Opin. Plant Biol. 9, 631–638. doi: 10.1016/j.pbi.2006.09.003
Lei, L., Singh, A., Bashline, L., Li, S., Yingling, Y. G., Gu, Y. (2015). Cellulose synthase interactive1 is required for fast recycling of cellulose synthase complexes to the plasma membrane in Arabidopsis. Plant Cell 27, 2926–2940. doi: 10.1105/tpc.15.00442
Li, X., Alarcón-Zúñiga, B., Kang, J., Nadeem Tahir, M. H., Jiang, Q., Wei, Y., et al. (2015). Mapping fall dormancy and winter injury in tetraploid alfalfa. Crop Sci. 55, 1995–2011. doi: 10.2135/cropsci2014.12.0834
Li, R., Shi, F., Fukuda, K., Yang, Y. (2010). Effects of salt and alkali stresses on germination, growth, photosynthesis and ion accumulation in alfalfa (Medicago sativa L.). Soil Sci. Plant Nutr. 56, 725–733. doi: 10.1111/j.1747-0765.2010.00506.x
Li, X., Wang, G., Fu, J., Li, L., Jia, G., Ren, L., et al. (2018). QTL mapping in three connected populations reveals a set of consensus genomic regions for low temperature germination ability in Zea mays L. Front. Plant Sci. 9, 65. doi: 10.3389/fpls.2018.00065
Li, T.-G., Wang, B.-L., Yin, C.-M., Zhang, D.-D., Wang, D., Song, J., et al. (2019). The Gossypium hirsutum TIR-NBS-LRR gene GhDSC1 mediates resistance against Verticillium wilt. Mol. Plant Patho. 20, 857–876. doi: 10.1111/mpp.12797
Li, W., Wang, D., Jin, T., Chang, Q., Yin, D., Xu, S., et al. (2011). The vacuolar Na+/H+ antiporter gene SsNHX1 from the halophyte salsola soda confers salt tolerance in transgenic alfalfa (Medicago sativa L.). Plant Mol. Biol. Rep. 29, 278–290. doi: 10.1007/s11105-010-0224-y
Lin, S., Medina, C. A., Norberg, O. S., Combs, D., Wang, G., Shewmaker, G., et al. (2021). Genome-Wide Association Studies identifying multiple loci associated with alfalfa forage quality. Front. Plant Sci. 12. doi: 10.3389/fpls.2021.648192
Lin, S., Medina, C. A., Wang, G., Combs, D., Shewmaker, G., Fransen, S., et al. (2023). Identification of genetic loci associated with five agronomic traits in alfalfa using multi-environment trials. Theor. Appl. Genet. 136, 121. doi: 10.1007/s00122-023-04364-4
Liu, C., Hao, Z., Zhang, D., Xie, C., Li, M., Zhang, X., et al. (2015). Genetic properties of 240 maize inbred lines and identity-by-descent segments revealed by high-density SNP markers. Mol. Breed. 35, 146. doi: 10.1007/s11032-015-0344-z
Liu, Z., Lan, J., Li, W., Ma, H. (2023). Reseeding improved soil and plant characteristics of degraded alfalfa (Medicago sativa) grassland in loess hilly plateau region. China. Ecol. Eng. 190, 106933. doi: 10.1016/j.ecoleng.2023.106933
Liu, X. P., Yu, L. X. (2017). Genome-wide association mapping of loci associated with plant growth and forage production under salt stress in alfalfa (Medicago sativa L.). Front. Plant Sci. 8. doi: 10.3389/fpls.2017.00853
Maiza, N., Jabri, C., Zaidi, N., Rafik, K., Khiari, B., Djébali, N., et al. (2021). High diversity of responses among Medicago truncatula lines to Phoma medicaginis infection. J. Oasis Agric. Sustain. Dev. 3, 52–57. doi: 10.56027/JOASD.spiss082021
Marchler-Bauer, A., Bo, Y., Han, L., He, J., Lanczycki, C. J., Lu, S., et al. (2017). CDD/SPARCLE: functional classification of proteins via subfamily domain architectures. Nucleic Acids Res. 45, 200–203. doi: 10.1093/nar/gkw1129
Medina, C. A., Hawkins, C., Liu, X.-P., Peel, M., Yu, L.-X. (2020). Genome-Wide Association and prediction of traits related to salt tolerance in autotetraploid alfalfa (Medicago sativa L.). Int. J. Mol. Sci. 21, 3361. doi: 10.3390/ijms21093361
Panahabadi, R., Ahmadikhah, A., Farrokhi, N. (2022). Genetic dissection of monosaccharides contents in rice whole grain using genome-wide association study. Plant Genome 6, e20292. doi: 10.1002/tpg2.20292
Patishtan, J., Hartley, T. N., Fonseca de Carvalho, R., Maathuis, F. J. M. (2018). Genome-wide association studies to identify rice salt-tolerance markers. Plant Cell Environ. 41, 970–982. doi: 10.1111/pce.12975
Porter, H. F., O’Reilly, P. F. (2017). Multivariate simulation framework reveals performance of multi-trait GWAS methods. Sci. Rep. 7, 38837. doi: 10.1038/srep38837
Sakiroglu, M., Brummer, E. C. (2017). Identification of loci controlling forage yield and nutritive value in diploid alfalfa using GBS-GWAS. Theor. Appl. Genet. 130, 261–268. doi: 10.1007/s00122-016-2782-3
Sakiroglu, M., Sherman-Broyles, S., Story, A., Moore, K. J., Doyle, J. J., Charles Brummer, E. (2012). Patterns of linkage disequilibrium and association mapping in diploid alfalfa (M. sativa L.). Theor. Appl. Genet. 125, 577–590. doi: 10.1007/s00122-012-1854-2
Silva, P., Gerós, H. (2009). Regulation by salt of vacuolar H+-ATPase and H+-pyrophosphatase activities and Na+/H+ exchange. Plant Signal Behav. 4, 718–726. doi: 10.4161/psb.4.8.9236
Thivierge, M.-N., Bélanger, G., Jégo, G., Delmotte, S., Rotz, C. A., Charbonneau, É. (2023). Perennial forages in cold-humid areas: Adaptation and resilience-building strategies toward climate change. Agron. J. 115, 1519–1542. doi: 10.1002/agj2.21354
Tlahig, S., Karmous, I., Mustapha, G., Takwa, J., Loumerem, M. (2020). Effect of cutting time on the performance of alfalfa (Medicago sativa L.) genotypes cropped in arid environment. Pol. J. Environ. Stud. 30, 1817–1829. doi: 10.15244/pjoes/124757
Urbanavičiūtė, I., Bonfiglioli, L., Pagnotta, M. A. (2021). One hundred candidate genes and their roles in drought and salt tolerance in wheat. Int. J. Mol. Sci. 22, 6378. doi: 10.3390/ijms22126378
Wang, Y., Yang, P., Zhou, Y., Hu, T., Zhang, P., Wu, Y. (2022). A proteomic approach to understand the impact of nodulation on salinity stress response in alfalfa (Medicago sativa L.). Plant Biol. 24, 323–332. doi: 10.1111/plb.13369
Warman, C., Panda, K., Vejlupkova, Z., Hokin, S., Unger-Wallace, E., Cole, R. A., et al. (2020). High expression in maize pollen correlates with genetic contributions to pollen fitness as well as with coordinated transcription from neighboring transposable elements. PloS Genet. 16, e1008462. doi: 10.1371/journal.pgen.1008462
Xu, L., Tang, X., Wang, B., Xin, X., Sun, Q., Li, Y., et al. (2019). Comparative transcriptome analysis of five Medicago varieties reveals the genetic signals underlying freezing tolerance. Crop Pasture Sci. 70, 273. doi: 10.1071/CP18165
Yang, Q., Wu, M., Wang, P., Kang, J., Zhou, X. (2005). Cloning and expression analysis of a vacuolar Na+/H+ antiporter gene from Alfalfa. DNA Seq. 16, 352–357. doi: 10.1080/10425170500272742
Yu, L.-X., Liu, X., Boge, W., Liu, X.-P. (2016). Genome-Wide Association study identifies loci for salt tolerance during germination in autotetraploid alfalfa (Medicago sativa L.) using genotyping-by-sequencing. Front. Plant Sci. 7, 956. doi: 10.3389/fpls.2016.00956
Yu, L.-X., Zhang, F., Culma, C. M., Lin, S., Niu, Y., Zhang, T., et al. (2020). Construction of high-density linkage maps and identification of quantitative trait loci associated with verticillium wilt resistance in autotetraploid alfalfa (Medicago sativa L.). Plant Dis. 104, 1439–1444. doi: 10.1094/PDIS-08-19-1718-RE
Yu, L. X., Zheng, P., Zhang, T., Rodringuez, J., Main, D. (2017). Genotyping-by-sequencing-based genome-wide association studies on Verticillium wilt resistance in autotetraploid alfalfa (Medicago sativa L.). Mol. Plant Pathol. 18, 187–194. doi: 10.1111/mpp.12389
Zaidi, N., Jabri, C., Maiza, N., Rafik, K., Khiari, B., Djébali, N., et al. (2021). Variation of Medicago sativa varieties tolerance to Phoma medicaginis infection.: M. sativa. J. Oasis Agric. Sustain. Dev. 3, 58–63. doi: 10.56027/JOASD.spiss092021
Zhang, T., Yu, L. X., Zheng, P., Li, Y., Rivera, M., Main, D., Stephanie, L. G., et al. (2015). Identification of loci associated with drought resistance traits in heterozygous autotetraploid alfalfa (Medicago sativa L.) using genome-wide association studies with genotyping by sequencing. PLoS ONE 10 (9), e0138931. doi: 10.1371/journal.pone.0138931
Keywords: alfalfa, biomass traits, genetic resources, GWAS, Phoma medicaginis, salinity, stress tolerance
Citation: Mnafgui W, Jabri C, Jihnaoui N, Maiza N, Guerchi A, Zaidi N, Basson G, Keyster EM, Djébali N, Pecetti L, Hanana M, Annicchiarico P, Sakiroglu M, Ludidi N and Badri M (2024) Discovering new genes for alfalfa (Medicago sativa) growth and biomass resilience in combined salinity and Phoma medicaginis infection through GWAS. Front. Plant Sci. 15:1348168. doi: 10.3389/fpls.2024.1348168
Received: 01 December 2023; Accepted: 15 April 2024;
Published: 02 May 2024.
Edited by:
Sushil Satish Chhapekar, University of Missouri, United StatesReviewed by:
Liping Ban, China Agricultural University, ChinaQaiser Shakeel, Islamia University of Bahawalpur, Pakistan
Copyright © 2024 Mnafgui, Jabri, Jihnaoui, Maiza, Guerchi, Zaidi, Basson, Keyster, Djébali, Pecetti, Hanana, Annicchiarico, Sakiroglu, Ludidi and Badri. This is an open-access article distributed under the terms of the Creative Commons Attribution License (CC BY). The use, distribution or reproduction in other forums is permitted, provided the original author(s) and the copyright owner(s) are credited and that the original publication in this journal is cited, in accordance with accepted academic practice. No use, distribution or reproduction is permitted which does not comply with these terms.
*Correspondence: Mounawer Badri, mounawer.badri@cbbc.rnrt.tn
†These authors have contributed equally to this work