- 1Drug Research and Development Center, School of Pharmacy, Third-Grade Pharmacology Laboratory of State, Administration of Traditional Chinese Medicine, Anhui Provincial Engineering Research Center for Polysaccharide Drugs, Wannan Medical College, Wuhu, China
- 2Department of Cardiology, Beijing Anzhen Hospital, Capital Medical University, Beijing, China
Background: Triptolide (TP), a naturally derived compound from Tripterygium wilfordii, has been proven effective in protecting against cardiovascular system, but the molecular mechanisms underlying its protective effects are poorly understood. In the current study, we sought to test the potential protective role of TP in the regulation of vascular calcification in a rat model and explore whether TP attenuates medial vascular calcification by upregulating miRNA-204.
Methods: Vitamin D3 plus nicotine (VDN) was used to induce a vascular calcification (VC) model of rat aorta. Von Kossa and Hematoxylin-Eosin staining were applied to assess the degree of calcification of rat aortas. Calcium content and alkaline phosphatase activity were measured. Quantitative reverse-transcription polymerase chain reaction (qRT-PCR) was applied to quantify miRNA-204 expression. The localization of runt-related transcription factor-2 (RUNX2) and bone morphogenetic protein-2 (BMP2) expressions were detected by immunohistochemistry and western blotting.
Results: Administration of TP greatly reduced vascular calcification in a dose-dependent manner compared with VC controls. The increase in ALP activity and calcium content was ameliorated by TP. Moreover, protein expression levels of BMP2 and RUNX2 were significantly reduced in calcified aortas. MiRNA-204 expression was increased in the TP-treated groups compared with VC controls and the effects of TP were reversed by the intravenous injection of miRNA-204-interfering lentivirus. However, the miRNA-204-overexpressing lentivirus had no additional effects on ALP activity, calcium content, BMP2 and RUNX2 expressions compared with those from TP group.
Conclusion: TP inhibited BMP2 and RUNX2 expression and attenuated vascular calcification via upregulating the level of miRNA-204. TP appears to be a potential new therapeutic option for treating vascular calcification.
Introduction
Vascular calcification (VC) is recognized a common complication of chronic kidney disease (CKD), diabetes mellitus and aging (Elliott and McGrath, 1994; Vattikuti and Towler, 2004; Shanahan et al., 2011; Schlieper et al., 2016). It is also associated with a varirty of other pathological conditions, such as hypertension, atherosclerosis, osteoporosis and rheumatoid arthritis (Demer and Tintut, 2008; Lanzer et al., 2014). The pathological deposition of calcium and phosphate is the most common form of medial vascular calcification in layer of the arteries. It is considered as a major risk factor for cardiovascular events and linked to increased cardiovascular morbidity and mortality (Johnson et al., 2006). MicroRNAs (miRNAs) are involved in the regulation of a range of different intracellular pathways controlling the osteo-/chondrogenic phenotypic switch of vascular smooth muscle cells (VSMCs) including the WNT/β-catenin pathway and TGFβ1/Smad signaling (Song et al., 2017; Zhang et al., 2018). Evidence suggests that miRNA-204 can downregulate RUNX2 expression and inhibit the transformation of VSMCs to an osteogenic phenotype, thereby negatively regulating the occurrence of vascular calcification (Cui et al., 2012).
Triptolide (TP), a bioactive product of epoxidation of two terpene lactone compounds from Tripterygium wilfordii, exhibits effective immunomodulatory and anti-inflammatory activities (Qiu and Kao, 2003; Liu, 2011; Chen et al., 2018). Our preceding studies also demonstrate that TP inhibits neointimal hyperplasia and exerts anti-inflammatory effects (Ge et al., 2011). Animal studies have also shown that TP can reduce arterial medial calcification in CKD mice fed a high-phosphorus diet (Yoshida et al., 2017). Although previous studies have revealed some important roles of TP, the possible pharmacological mechanism behind these effects is still limited (Hou et al., 2019; Noel et al., 2019). In recent years, studies have shown that TP may play a pharmacological role by regulating miRNAs (Li et al., 2018; Xue et al., 2018). It was suggested that the expression of miRNA-142–3 could be induced to regulate heat shock protein 70 by TP (MacKenzie et al., 2013). Another study reveals that TP could induce spontaneous programmed death (apoptosis) of T-cell lymphocytic leukemia cells probably through downregulating miRNA-16–1 (Jiang et al., 2015).
Based on these discoveries, we evaluated the vascular calcification model rats induced by administration of VDN to examine the effects of pharmacological intervention with TP. Besides, the current study investigated protective mechanisms of TP using miRNA-204-overexpressing lentivirus and miRNA-204-interfering lentivirus.
Material and Methods
Drug and Reagents
TP (purity >99%) was from National Institute of Biological Products and Materia Medica. VDN was bought from Sigma Chemical Co. (St. Louis, MO, USA). Anti-BMP2, RUNX2 antibodies, anti-GAPDH antibody and HRP-conjugated anti-rabbit or mouse IgG secondary antibodies were purchased from Santa Cruz Biotechnology (Santa Cruz, CA, USA). Trizol reagent and enhanced chemiluminescent (ECL) detection kits were provided by Beijing Applygen Technologies (Beijing, China). Protein gel electrophoresis and membrane transfer system (Bio-Rad, USA), and calcium detection kit and alkaline phosphatase (ALP) assay kits (Nanjing Jiancheng Bioengineering Research Institute) were also used. All other reagents were of analytical grade. qRT-PCR kits were purchased from BIO-LAB (Tianjin, China). The PCR primers were also provided by BIO-LAB (Jerusalem, Israel). The forward and reverse PCR primers (rat) for miRNA-204 were 5′-TGGTTCCCTTTGTCATCCT-3′ and 5′- GTCGTATCCAGTGCAGGGTCCGAGGTATTCGCACTGGATACGACAGGCAT-3′, respectively.
Administration of miRNA-204-shRNA and miRNA-204-Overexpression by Lentiviral Delivery
The miRNA-204-shRNA (CGTTCCCTTTGTCATCCTATGCCTTCAAGAGAGGCATAGGATGACAAAGGGAATTTTTT) and gene encoding miRNA-204 (Gene ID: 406,987) were inserted into the pLKO.1-EGFP-Puro vectors. Lentiviral vectors plenty 6.3-LTR-RRE-U6-CMV EYFP-WRE-SV40 BSD-LTR were generated. The miRNA-204-interfering lentivirus (1.4×107 TU/ml/day) and miRNA-204-overexpression lentivirus (2.8×107 TU/ml/day) were given by intravenous injection for three days, and the empty lentiviral vectors (2.3×107 TU/ml/day) were used as control.
Animal Model and Experimental Design
Male SD (Sprague Dawley, 150–180 g) rats were from Animal Center of Beijing Anzhen Hospital, Capital Medical University and Beijing Institute of Heart Lung And Blood Vessel Diseases. All experiments were approved by the Committee for Experimental Animal Management of Beijing Anzhen Hospital, Capital Medical University. The ambient temperature and relative humidity for raising animals were 22 ± 3°C and 50% ± 10%, respectively.
As previously reported (Huang et al., 2003), a rat model of VC induced by VDN was established. After 1 week of adaptive feeding, 80 rats were used for treatment with VC + TP or a vehicle control. Rats were administrated vitamin D3 (300,000 IU/kg in arachis oil, intramuscularly) as well as nicotine (25 mg/kg in 5 ml peanut oil, intragastrically) at 09:00 on the first day, and nicotine was re-administered at 7:00 pm on the same day. Then 30 randomly chosen VC model rats which were grouped as TP-L, M and H groups were given an intragastrically administration of TP (10, 20 and 40 mg/kg) for 4 weeks, respectively. After that, 20 VC model rats and 20 VC + TP (TP-H) rats were treated with miRNA-204-interfering lentivirus and miRNA-204- overexpression-lentivirus for three days by intravenous injection, respectively. The remaining VC rats were regarded as the calcification controls. The other ten rats were given corresponding solvents as a vehicle control.
After four weeks, rats were anesthetized by 1% sodium perborate and blood samples were withdrawn from the abdominal aorta by heparinized syringes. The supernatants after centrifugation were transferred into tubes preparing to measure ALP activity and calcium content. Then, all of the rats were sacrificed by bloodletting while the aortas were quickly removed.
Von Kossa and Hematoxylin-Eosin Staining
Approximately 1 cm of tissue was taken from the descending thoracic aorta under the arcus aortae, from which adipose tissue was discarded. The sample was then fixed with 10% triformol and embedded in paraffin, followed by sectioning of the tissue and staining. Areas with calcium deposition appeared black, while other tissues exhibited counterstaining. The slides were washed with 1% acidic alcohol after hematoxylin nuclear staining, followed by staining the slides using eosin. Afterward, the slides were washed and dehydrated. Finally, the aortic slides were treated with xylene and evaluated using the Image Pro Plus 6.0 system (MediaCybernetics, Inc, SilverSpring.MD).
Determination of Calcium Content
The calcium content in aortic tissue was measured using a calcium assay kit in accordance with the manufacturer’s instructions. Briefly, rat homogenates from the aortas were collected and centrifuged for 4 min at 3,000×g. The resulting supernatants were spun at 10, 000×g for 30 min. Protein content was determined with BSA as a standard according to Bradford (Bradford, 1976). Supernatants were diluted to a protein concentration of 0.5 mg/ml for determination. Then, nitric acid was used to nitrify the dried tissue. The calcium was dissolved in solution containing 27 nM KCl and 27 μM LaCl3 after removing the nitric acid by heating. Eventually, the calcium content of the aorta was measured and the results were normalized to the total protein concentration.
Detecting Alkaline Phosphatase Activity
ALP activity in aortic tissue was determined in accordance with the instructions of ALP assay kit. Briefly, the aortic tissue homogenate was mixed with a reaction mixture consisting of stock substation solution and alkaline buffer at a ratio of 1:1. Then, the mixture was incubated for 15 min at 37°C following the addition of 1.5 ml of developer solution. The absorbance of each well was measured using a microplate reader at 520 nm. The values of ALP in specimens were calculated in conformity to the established standard curve.
Quantitative Real-Time PCR Analysis
Trizol reagent was utilized to extract the RNA from the aortas, and the quantification was conducted depending on an ultramicro spectrophotometer (Biophotometer, Nanodrop 2000, USA). For each sample, 2 µg of total RNA was reverse transcribed to cDNA with reverse transcriptase and oligo (dT) 15 primer. The PCR reaction was performed in a total volume of 20 μL. For miRNA-204 expression analysis, reverse transcription and qRT-PCR were carried out using BIO-LAB miRNA qPCR Primer Set for mouse miRNA-204 and U6 snRNA in accordance with the manufacturer’s instructions. Primer sequences for mouse miRNA2 and U6 snRNA are put forward. The 2−ΔΔCT method was used to determine the expression of miRNA-204 relative to U6.
Immunohistochemical Staining of Bone Morphogenetic Protein-2 and Runt-Related Transcription Factor-2
The rat aortic samples were fixed by 4% formaldehyde in phosphate-buffered saline, and embedded in paraffin. Specimens were cut into 6 mm sections and then subjected to immunohistochemical staining. The processed sections from rats were incubated overnight in the primary antibody at 4°C. After washes, the secondary antibodies were added to the sections for 1 h incubation. Negative controls were established by omitting the primary antibody and including only the secondary antibody. In all cases, staining for negative control indicated insignificant. Immunostaining data were quantified by individuals blinded to the treatment groups.
Western Blotting
Protein extracts from the aortas were resuspended in sample buffer containing 2% SDS, 2% mercaptoethanol, 50 mmol/L Tris-HCl (pH 6.8), 10% glycerol and 0.05% bromophenol blue. Goat anti-rat BMP2 primary antibody and goat anti-rat RUNX2 primary antibody were diluted at ratio of 1:1,000 in TBS-T and applied to the membrane, and incubated at 4°C overnight with agitation. The secondary antibody at 1:2000 dilution in TBS-T was added to the membrane, and incubated at room temperature for 1 h with agitation. After the addition of an enhanced chemiluminescence substrate, the membrane was immediately visualized on a phosphor imager.
Statistical Analyses
All statistical data were analyzed using Statistical Product and Service Solutions (SPSS) software (Version 22.0, SPSS Inc, US). The results were shown as means ± standard deviation (SD). Statistical analysis was performed by a one-way analysis of variance (ANOVA) followed by Tukey’s test for multiple comparisons. A non-parametric test was applied for comparing the band density values between groups. The values of p < 0.05 was considered statistically significant.
Results
Triptolide Protected Rat Aorta From Vitamin D3 plus Nicotine-Induced Vascular Calcification
The effect of VDN on the aortas of rats was analyzed by HE and von Kossa staining. The results of HE staining showed thickened vessel walls, disordered elastic fibers and widespread calcification. Increased calcium deposition was revealed by von Kossa staining (Figures 1A,B). Results for ALP activity and calcium content revealed that vitamin D3 and nicotine elevated the levels of ALP activity in rat aortic VSMCs and led to greatly increased calcium content and calcium area (Figure 1C). The rat aortic vascular calcification model could thus be successfully induced by VDN. Examination of HE and von Kossa staining showed that treatment with TP (20 and 40 mg/kg) significantly decreased VDN-induced vascular calcification. Furthermore, treatment with TP significantly inhibited ALP activity (p < 0.01), a marker of osteoblast differentiation, as well as calcium content in aortic tissues was decreased in TP-M and TP-H groups comparing with model group (p < 0.01) (Figure 1).
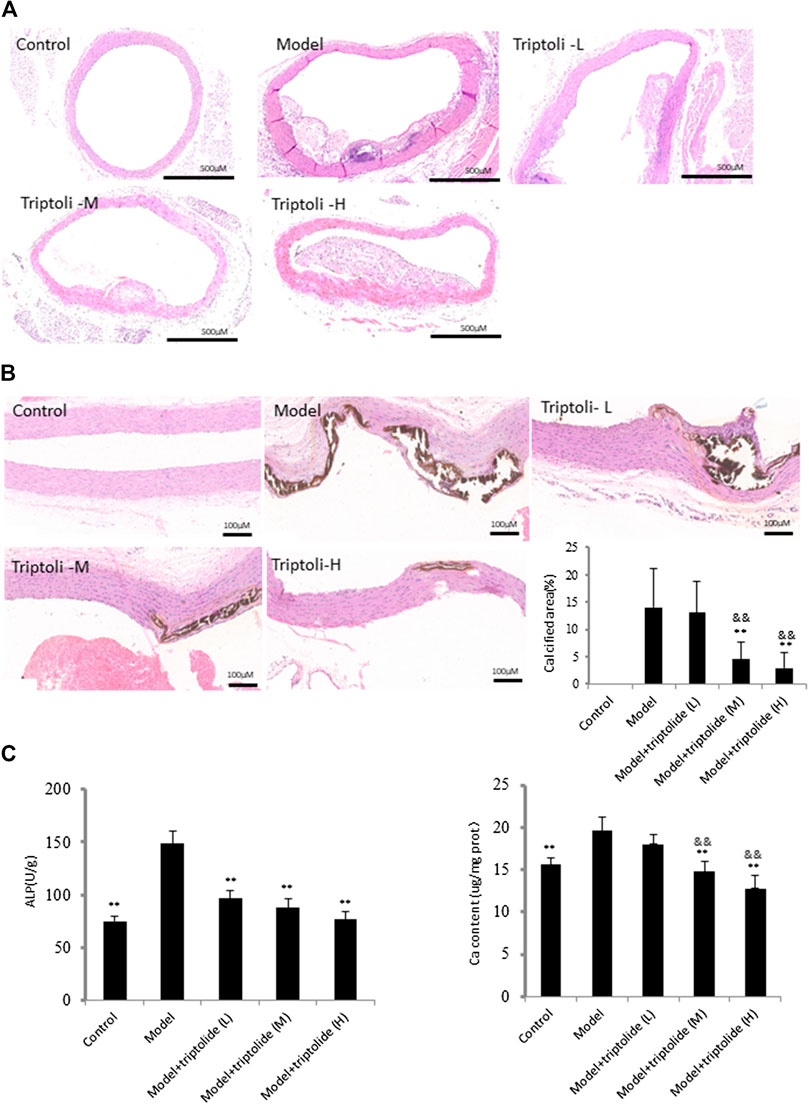
FIGURE 1. TP attenuates vascular calcification induced by VDN (A) HE staining shows thickened vessel walls, disordered elastic fibers and widespread calcification in aortas of rats (B) Left: von Kossa staining shows increased calcium deposition in aortas of rats; right: bar graphs show quantitative evaluation of the von Kossa calcium area (n = 10) (C) Bar graphs show quantitative evaluation of ALP and calcium content (n = 10). **p < 0.01 compared with model group, &&p < 0.01 compared with model + TP-L group.
Triptolide Inhibited the Bone Morphogenetic Protein-2 and Runt-Related Transcription Factor-2 Expressions
BMP2 is a powerful stimulator of VSMC osteoinduction. Its calcification-promoting effects in VSMCs involve the upregulation of SMAD signaling. They also increase the secretion of RUNX2, a core transcription factor involved in the differentiation of osteoblasts and chondrocytes, which accelerates the process of vascular smooth muscle cell differentiation into osteoblasts. To further explore the effects of TP on SMAD signaling pathway, BMP2 and RUNX2 expressions were detected. The results of western blot analysis and immunohistochemistry showed increased BMP2 and RUNX2 expression in model group to the control group (p < 0.05), while TP (20 and 40 mg/kg) inhibted the BMP2 and RUNX2 expression increased by VDN (p < 0.05) (Figure 2).
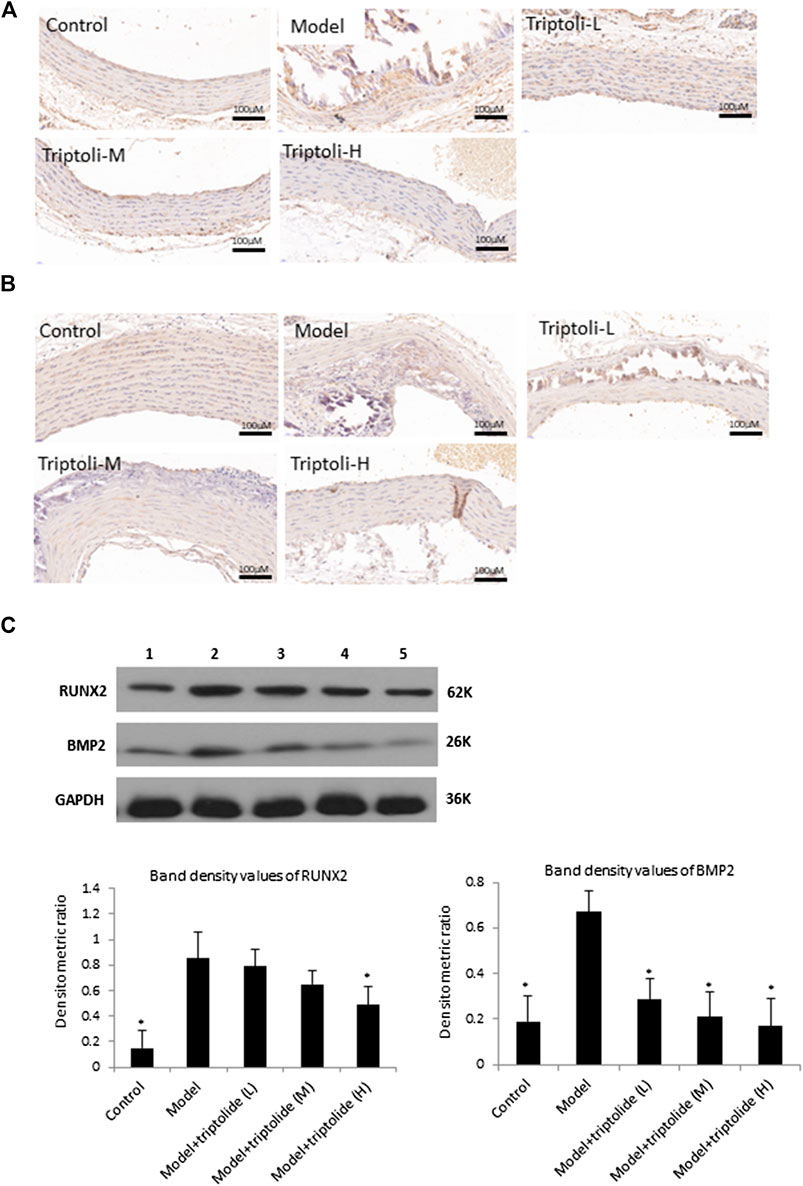
FIGURE 2. TP suppresses BMP2 and RUNX2 expression induced by vascular calicification to the aortas (A) Immunohistochemical staining of BMP2 (B) Immunohistochemical staining of RUNX2 (C) Western blot analysis of BMP2 and RUNX2 (lane 1: Control, lane 2: Model, lane 3: Model + TP-L, lane 4: Model + TP-M, lane 5: Model + TP-H. Upper: representative images of BMP2 and RUNX2 expression, an antibody for DAPDH was used to show equal protein loading; under: bar graphs show quantitative evaluation of BMP2 and RUNX2 expression (n = 3). Data are reported as mean ± S.D, *p < 0.05 compared with model group.
Effect of miRNA-204 on Rat Aorta
To investigate the effect of miRNA-204 on rat aorta, we constructed miRNA-204-overexpressing lentivirus and miRNA-204-interfering lentivirus. RT-PCR results demonstrated miRNA-204 levels were obviously increased in the aortas of rat transfected with miRNA-204-overexpressing lentivirus and decreased in the aortas of rat transfected with miRNA-204-interfering lentivirus (Figure 3A). In the miRNA-204-overexpressing group, von Kossa staining showed reduced calcium deposition of vasorum in the tunica media, and the calcium deposition in the interference group was expressively increased compared with that in the VC model group (Figure 3B). The consequence demonstrated that the expression of miRNA-204 was dramatically decreased during vascular calcification. In addition, by increasing the expression level of miRNA-204, vascular calcification was significantly inhibited.
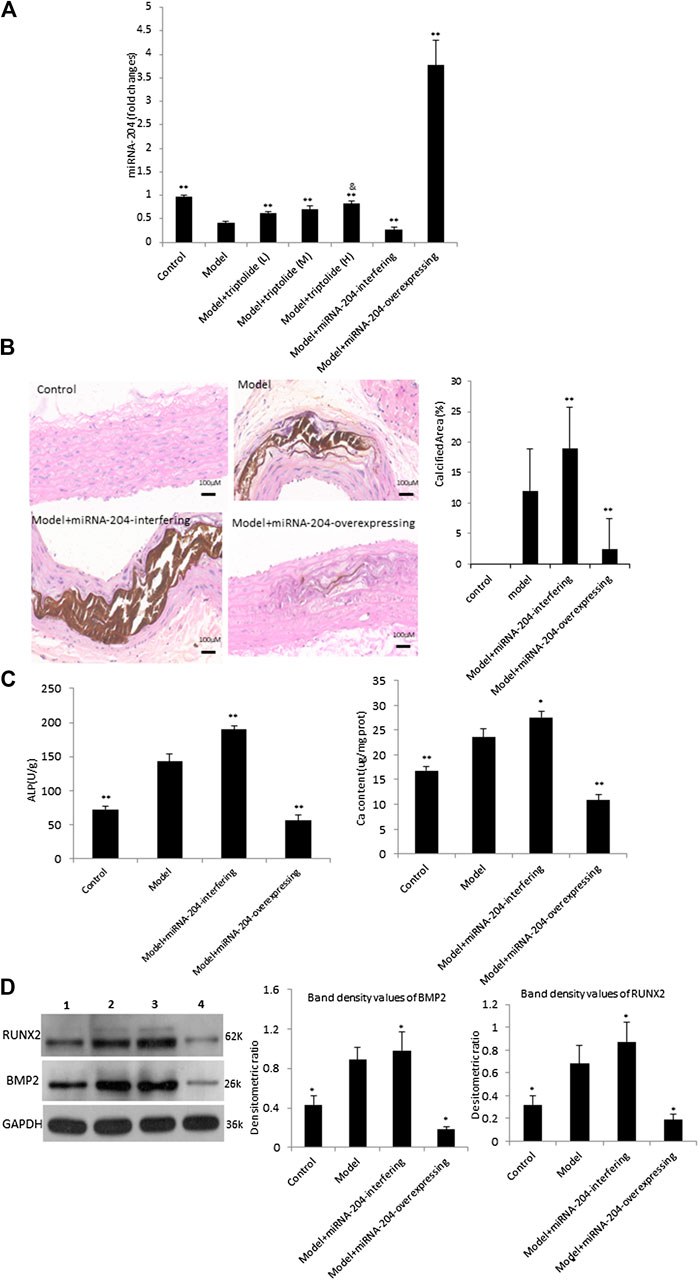
FIGURE 3. miRNA-204 disturbs vascular calcification induced by VDN (A) Real time PCR showed the expression of the miRNA-204 in the different groups (n = 10). **p < 0.01 compared with model, &p < 0.05 compared with model + TP-L (B) Left: von Kossa staining shows calcium deposition in aortas of rats, right: bar graphs show quantitative evaluation of the vonkossa calcium area (n = 10). **p < 0.01 compared with model group (C) Bar graphs show quantitative evaluation of the ALP activity and calcium content. *p < 0.05, **p < 0.01 compared with model (D) Western blot analysis of BMP2 and RUNX2. Left: representative images of BMP2 and RUNX2 expression, an antibody for DAPDH was used to show equal protein loading (lane 1: Control, lane 2: Model, lane 3: Model + miRNA-204-interfering, lane 4: Model + miRNA-204-overexpression); Right: bar graphs show quantitative evaluation of BMP2 and RUNX2 expression (n = 3). Data are reported as the mean ± S.D, *p < 0.05 compared with model group.
Furthermore, examination of serial aortic tissues showed that the expressions of BMP2, RUNX2, ALP activity and calcium content were markedly increased by miRNA-204- interfering lentivirus and were decreased by miRNA-204-overexpressing lentivirus (Figures 3C,D). Additional studies indicated that the miRNA-204 level in the TP group was upregulated compared with that in the model group. These results revealed that TP may regulate miRNA-204 levels in a dose-dependent manner. Then, the high dosage of TP (40 mg/kg) was chosen in the next experiments.
Triptolide Reduced Vascular Calcification and Inhibited Bone Morphogenetic Protein-2 and Runt-Related Transcription Factor-2 Expression by Upregulating Expression of miRNA-204
To further explore the phenomenon that TP plays roles by way of miRNA-204, miRNA-204-overexpressing lentivirus and miRNA-204-interfering lentivirus, respectively, were utilized to TP-group. The control and VDN model group were given corresponding lentiviral control vectors. Von Kossa staining, analyses of ALP activity and calcium content were performed to confirm that treatments of miRNA-204-interfering lentivirus can sufficiently block TP effects. The calcium deposition area was increased in rats co-treated with miRNA-204-interfering lentivirus and TP (Figures 4A,B).
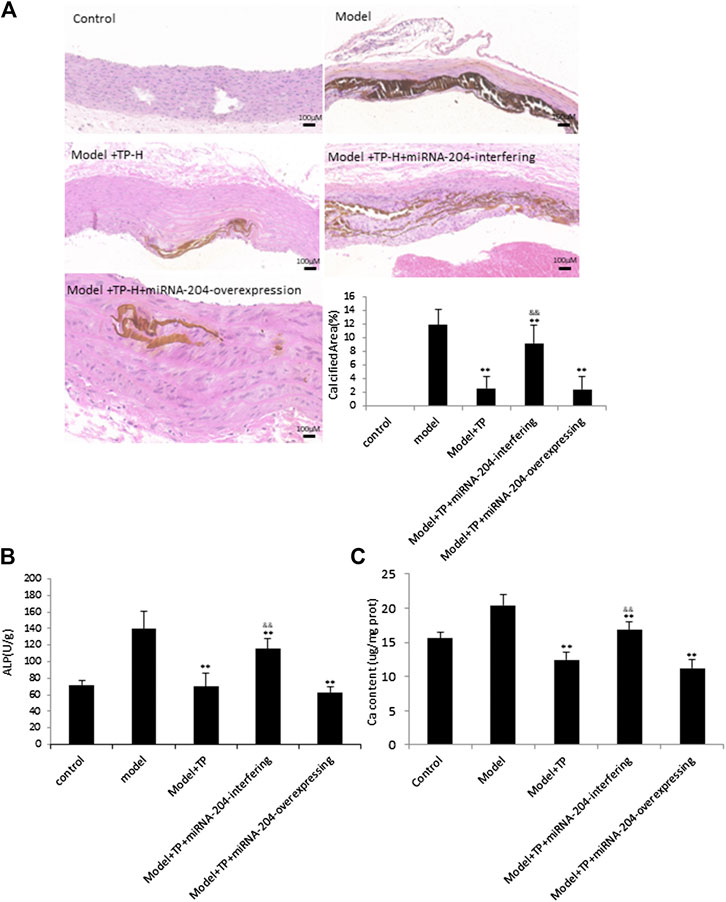
FIGURE 4. TP protects against vascular calcification induced by VDN in a miRNA-204 increasing manner (A) Left: von Kossa staining shows calcium deposition in aortas of rats; right: bar graphs show quantitative evaluation of the vonkossa calcium area (n = 10). **p < 0.01 compared with model group, &&p < 0.01 compared with model + TP group (B &C) Bar graphs show quantitative evaluation of the ALP activity and calcium content, respectively (n = 10). *p < 0.05, **p < 0.01 compared with model group, &&p < 0.01 compared with model + TP group.
Furthermore, results of western blot analysis and immunohistochemistry showed that the inhibitory effects of TP (40 mg/kg) on the expressions of BMP2 and RUNX2 were significantly blocked by miRNA-204-interfering lentivirus (Figure 5). However, the miRNA-204-overexpressing lentivirus had no additional effects on ALP activity, calcium content and BMP2 and RUNX2 expressions compared with those from TP-H group. The results indicated that miRNA-204 plays a negative role in vascular calcification through downregulating BMP2 and RUNX2 protein levels, and TP may reduce vascular calcification and inhibited BMP2 and RUNX2 expression by upregulating expression of miRNA-204.
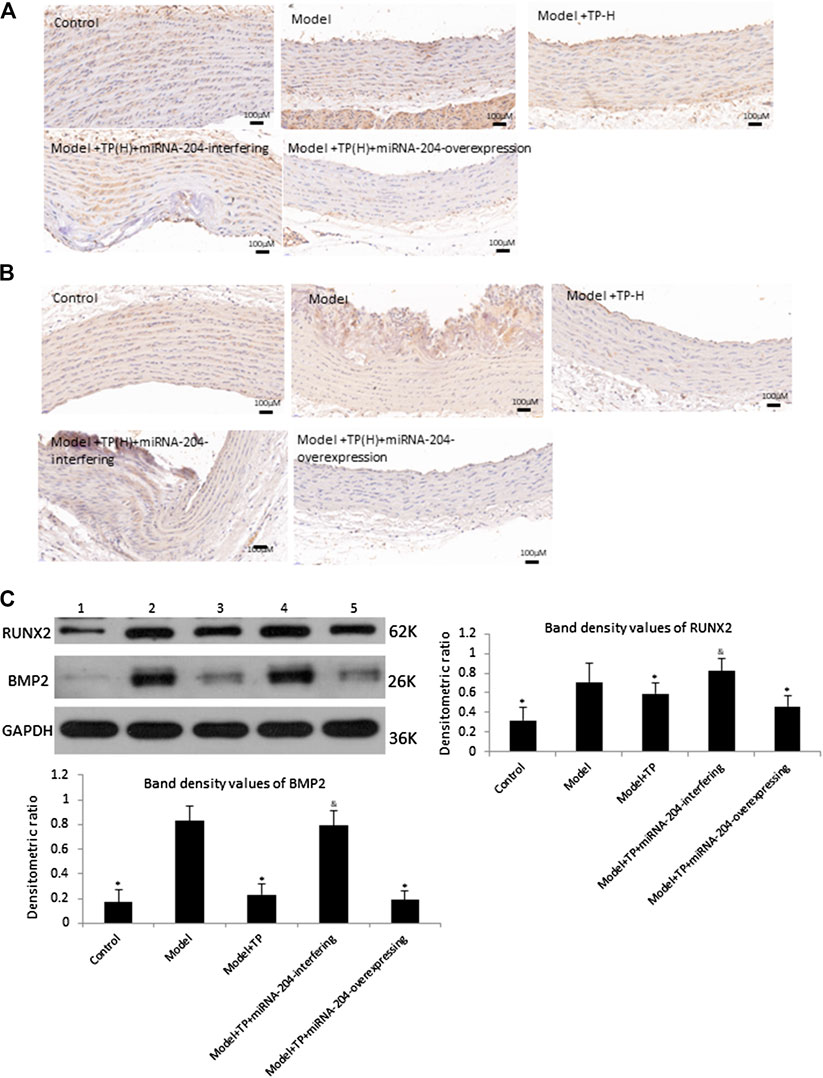
FIGURE 5. TP reduces BMP2 and RNUX2 expression in a miRNA-204 increasing manner (A) Immunohistochemical staining of BMP2 (B) Immunohistochemical staining of RUNX2 (C) Western blot analysis of BMP2 and RUNX2 (lane 1: Control, lane 2: Model, lane 3: Model + TP, lane 4: Model + TP + miRNA-204-interfering, lane 5: Model + TP + miRNA-204-overexpression). Upper and left: representative images of BMP2 and RUNX2 expression, an antibody for DAPDH was used to show equal protein loading; under and right: bar graphs show quantitative evaluation of BMP2 and RUNX2 expression (n = 3). Data are reported as the mean ± S.D. *p < 0.05 compared with model group; &p < 0.05 compared with model + TP group.
Discussion
In this study, we observed that administration of VDN can be used to establish a classic rat aortic calcification model with the presence of dispersed and calcified nodules among the elastic fibers. These results are consistent with those of previous reports (Zhang et al., 2016). We identified that TP treatment attenuated this calcification in a dose-dependent manner and prevented the acquisition of an osteogenic phenotype, as indicated by the decreased expression of RUNX2 and BMP2 in rat aortas. Even though this research provides extensive evidence for downregulated RUNX2 and BMP2 expressions being the main mechanisms leading to TP induced aortas protection and recovery, its influence on other mechanisms remain poorly understood. A recent study suggested that the key determinants for vascular calcification in CK were dysregulation of mineral homeostasis and elevated phosphate levels. (Giachelli, 2003). Hyperphosphatemia is associated with an increased risk of not only cardiovascular events and death, but also vascular calcification (Abramowitz et al., 2010; Lanzer et al., 2014). In response to high extracellular phosphate levels, VSMCs can change their phenotype into osteo/chondroblast-like cells actively promoting vascular mineralization (Voelkl et al., 2019). Researchers have also shown that the transdifferentiation of VSMCs into osteoblasts is an important mechanism of vascular calcification (Liberman et al., 2013; Shroff et al., 2013). These transdifferentiated VSMCs lose their contractile phenotype supporting a more mesenchymal one and earn properties similar to those of osteoblasts and chondroblasts (Demer and Tintut, 2008). RUNX2, belonging to osteogenic transcription factors, exert a crucial role in vascular calcification (Schlieper et al., 2016). Vascular osteo-/chondrogenic transdifferentiation and calcification are prevented due to the deficiency of RUNX2 in VSMCs (Speer et al., 2010). The expression of osteogenic- and chondrogenic-specific proteins in VSMCs such as bone morphogenetic protein-2 (BMP2) or ALP could be further induced by osteo-/chondrogenic transcription factors (Lanzer et al., 2014). Similar to the case in bone, ALP degrades inorganic pyrophosphate to allow unrestrained tissue mineralization (Johnson et al., 2006). Furthermore, increased expression of BMP2 is a well-known promoter of VSMC calcification. Therefore, the emerging evidence supports the existence of a direct relationship between the abnormal expression of BMP2/RUNX2 and vascular calcification.
In addition, miRNA-204 also acts as a negative regulator of VSMC calcification that potentially functions through repressing BMP2 and RNUX2 expression. MiRNAs, a cluster of small noncoding RNA molecules, play a vital role in a diversity of physiological and pathological processes in humans (Huntzinger and Izaurralde, 2011). A study identified that the downregulation of miRNA-204 may conduce to β-glycerophosphate-induced VSMCs calcification through modulating RNUX2 expression (Sluijter, 2013). MiRNA-204 is proposed as a significant new regulator of VSMC calcification (Cui et al., 2012). Thus, miRNA-204 represents a potential therapeutic target in medial artery calcification Since miRNA-204 has been found to restrain vascular calcification in vitro and vivo, TP may present a certain regulatory effect on vascular calcification via regulating miRNA-204. Our experiments revealed that overexpression of miRNA-204 decreased RNUX2 and BMP2 protein levels and relieved the medial artery calcification caused by VDN. The findings illustrate that miRNA-204 inhibits medial artery calcification by downregulating BMP2 and RNUX2 protein expression in vivo. Therefore, we further explored the relationship between TP and miRNA-204 in calcified aortas, and finally revealed that TP may attenuate vascular calcification via upregulating miRNA-204 level. RT-PCR analysis indicated that the expression of miRNA-204 is reduced in the aortas of VC model rats and TP increased the miRNA-204 expression. Furthermore, inhibitory effects of TP on the expressions of BMP2 and RNUX2 were significantly blocked by miRNA-204-interfering lentivirus, indicating that TP treatment downregulated the expression of BMP2 and RUNX2 by increased the miRNA-204 expression. Therefore, our research indicated that the pathological mechanism of vascular calcification might be associated with the abnormal expression of miRNA-204, which would lead to downregulated expression level of osteogenic-specific proteins, thereby affecting VSMC function.
Conclusion
To conclude, TP has been demonstrated to attenuate vascular calcification by regulating the expression of miRNA-204 and modulating BMP2 and RNUX2 dependent on VSMC osteoinduction. Lastly, these findings imply that miRNA-204 could be developed as a promising therapeutic target modulating vascular calcification and as a target for probing the possibility of TP as a therapeutic agent.
Data Availability Statement
The original contributions presented in the study are included in the article/supplementary files, further inquiries can be directed to the corresponding author/s.
Ethics Statement
The animal study was reviewed and approved by Committee for Experimental Animal Management of Beijing Anzhen Hospital.
Author Contributions
YP and YD were devoted to conception and design of the research study. YZ and HG performed the research study. QX, RW, YW, and DC contributed to collecting analysis data. JY, JL, and QM worked on writing and revising the article and inspected the final manuscript.
Funding
This study was supported by grants from the National Natural Scientific Foundation of China (No. 81573744, 81973841). Talent funding projects of Wannan Medical College (No. WYRCQD2018009).
Conflict of Interest
The authors declare that the research was conducted in the absence of any commercial or financial relationships that could be construed as a potential conflict of interest.
Acknowledgments
The authors are grateful to Hua Zhu from Ohio State University Wexner Medical Center for their valuable advice about writing in this manuscript.
Supplementary Material
The Supplementary Material for this article can be found online at: https://www.frontiersin.org/articles/10.3389/fphar.2020.581230/full#supplementary-material.
References
Abramowitz, M., Muntner, P., Coco, M., Southern, W., Lotwin, I., Hostetter, T. H., et al. (2010). Serum alkaline phosphatase and phosphate and risk of mortality and hospitalization. Clin. J. Am. Soc. Nephrol. 5 (6), 1064–1071. doi:10.2215/cjn.08621209
Bradford, M. M. (1976). A rapid and sensitive method for the quantitation of microgram quantities of protein utilizing the principle of protein-dye binding. Anal. Biochem. 72 (1–2), 248–254. doi:10.1016/0003-2697(76)90527-3
Chen, S.-R., Dai, Y., Zhao, J., Lin, L., Wang, Y., and Wang, Y. (2018). A mechanistic overview of triptolide and celastrol, natural products from Tripterygium wilfordii Hook F. Front. Pharmacol. 9, 104. doi:10.3389/fphar.2018.00104
Cui, R.-R., Li, S.-J., Liu, L.-J., Yi, L., Liang, Q.-H., Zhu, X., et al. (2012). MicroRNA-204 regulates vascular smooth muscle cell calcification in vitro and in vivo. Cardiovasc. Res. 96 (2), 320–329. doi:10.1093/cvr/cvs258
Demer, L. L., and Tintut, Y. (2008). Vascular calcification. Circulation 117 (22), 2938–2948. doi:10.1161/circulationaha.107.743161
Elliott, R. J., and McGrath, L. T. (1994). Calcification of the human thoracic aorta during aging. Calcif. Tissue Int. 54 (4), 268–273. doi:10.1007/bf00295949
Ge, H., Zhou, Y., Ma, H., Liu, L., Liu, X., Wang, Z., et al. (2011). Effectiveness of triptolide-coated stent on decreasing inflammation and attenuation of intimal hyperplasia in a pig after coronary angioplasty. Angiology 62 (3), 265–269. doi:10.1177/0003319710385337
Giachelli, C. M. (2003). Vascular calcification: in vitro evidence for the role of inorganic phosphate. J. Am. Soc. Nephrol. 14, 300S–304S. doi:10.1097/01.asn.0000081663.52165.66
Hou, W., Liu, B., and Xu, H. (2019). Triptolide: medicinal chemistry, chemical biology and clinical progress. Eur. J. Med. Chem. 176, 378–392. doi:10.1016/j.ejmech.2019.05.032
Huang, Z., Li, J., Jiang, Z., Qi, Y., Tang, C., and Du, J. (2003). Effects of adrenomedullin, C-type natriuretic peptide, and parathyroid hormone-related peptide on calcification in cultured rat vascular smooth muscle cells. J. Cardiovasc. Pharmacol. 42 (1), 89–97. doi:10.1097/00005344-200307000-00014
Huntzinger, E., and Izaurralde, E. (2011). Gene silencing by microRNAs: contributions of translational repression and mRNA decay. Nat. Rev. Genet. 12 (2), 99–110. doi:10.1038/nrg2936
Jiang, C.-B., Wei, M.-G., Tu, Y., Zhu, H., Li, C.-Q., Jing, W.-M., et al. (2015). Triptolide attenuates podocyte injury by regulating expression of miRNA-344b-3p and miRNA-30b-3p in rats with adriamycin-induced nephropathy. Evid. base Compl. Alternative Med. 2015, 1. doi:10.1155/2015/107814
Johnson, R. C., Leopold, J. A., and Loscalzo, J. (2006). Vascular calcification: pathobiological mechanisms and clinical implications. Circ. Res. 99 (10), 1044–1059. doi:10.1161/01.Res.0000249379.55535.21
Lanzer, P., Boehm, M., Sorribas, V., Thiriet, M., Janzen, J., Zeller, T., et al. (2014). Medial vascular calcification revisited: review and perspectives. Eur. Heart J. 35 (23), 1515–1525. doi:10.1093/eurheartj/ehu163
Li, S.-G., Shi, Q.-W., Yuan, L.-y., Qin, L.-p., Wang, Y., Miao, Y.-Q., et al. (2018). C-Myc-dependent repression of two oncogenic miRNA clusters contributes to triptolide-induced cell death in hepatocellular carcinoma cells. J. Exp. Clin. Canc. Res. 37 (1), 1–13. doi:10.1186/s13046-018-0698-2
Liberman, M., Pesaro, A. E. P., Carmo, L. S., and Serrano Jr, C. V. (2013). Vascular calcification: pathophysiology and clinical implications. Nstn (So Paulo) 11 (3), 376–382. doi:10.1590/s1679-45082013000300021
Liu, Q. (2011). Triptolide and its expanding multiple pharmacological functions. Int. Immunopharm. 11 (3), 377–383. doi:10.1016/j.intimp.2011.01.012
MacKenzie, T. N., Mujumdar, N., Banerjee, S., Sangwan, V., Sarver, A., Vickers, S., et al. (2013). Triptolide induces the expression of miR-142-3p: a negative regulator of heat shock protein 70 and pancreatic cancer cell proliferation. Mol. Canc. Therapeut. 12 (7), 1266–1275. doi:10.1158/1535-7163.Mct-12-1231
Noel, P., Von Hoff, D. D., Saluja, A. K., Velagapudi, M., Borazanci, E., and Han, H. (2019). Triptolide and its derivatives as cancer therapies. Trends Pharmacol. Sci. 40 (5), 327–341. doi:10.1016/j.tips.2019.03.002
Qiu, D., and Kao, P. N. (2003). Immunosuppressive and anti-inflammatory mechanisms of triptolide, the principal active diterpenoid from the Chinese medicinal herb Tripterygium wilfordii Hook. F. Drugs In R & D 4 (1), 1–18. doi:10.2165/00126839-200304010-00001
Schlieper, G., Schurgers, L., Brandenburg, V., Reutelingsperger, C., and Floege, J. (2016). Vascular calcification in chronic kidney disease: an update. Nephrol. Dial. Transplant. 31 (1), 31–39. doi:10.1093/ndt/gfv111
Shanahan, C. M., Crouthamel, M. H., Kapustin, A., and Giachelli, C. M. (2011). Arterial calcification in chronic kidney disease: key roles for calcium and phosphate. Circ. Res. 109 (6), 697–711. doi:10.1161/circresaha.110.234914
Shroff, R., Long, D. A., and Shanahan, C. (2013). Mechanistic insights into vascular calcification in CKD. J. Am. Soc. Nephrol. 24 (2), 179–189. doi:10.1681/asn.2011121191
Sluijter, J. P. G. (2013). MicroRNAs in cardiovascular regenerative medicine: directing tissue repair and cellular differentiation. Int. Scholarly Res. Notices, 2013, 1. doi:10.1155/2013/593517
Song, R., Fullerton, D. A., Ao, L., Zhao, K.-s., and Meng, X. (2017). An epigenetic regulatory loop controls pro-osteogenic activation by TGF-β1 or bone morphogenetic protein 2 in human aortic valve interstitial cells. J. Biol. Chem. 292 (21), 8657–8666. doi:10.1074/jbc.M117.783308
Speer, M. Y., Li, X., Hiremath, P. G., and Giachelli, C. M. (2010). Runx2/Cbfa1, but not loss of myocardin, is required for smooth muscle cell lineage reprogramming toward osteochondrogenesis. J. Cell. Biochem. 110 (4), 935–947. doi:10.1002/jcb.22607
Vattikuti, R., and Towler, D. A. (2004). Osteogenic regulation of vascular calcification: an early perspective. Am. J. Physiol-Endoc. M. 286 (5), E686–E696. doi:10.1152/ajpendo.00552.2003
Voelkl, J., Lang, F., Eckardt, K.-U., Amann, K., Kuro-o, M., Pasch, A., et al. (2019). Signaling pathways involved in vascular smooth muscle cell calcification during hyperphosphatemia. Cell. Mol. Life Sci. 76 (11), 2077–2091. doi:10.1007/s00018-019-03054-z
Xue, M., Cheng, Y., Han, F., Chang, Y., Yang, Y., Li, X., et al. (2018). Triptolide attenuates renal tubular epithelial-mesenchymal transition via the MiR-188-5p-mediated PI3K/AKT pathway in diabetic kidney disease. Int. J. Biol. Sci. 14 (11), 1545. doi:10.7150/ijbs.24032
Yoshida, T., Yamashita, M., Horimai, C., and Hayashi, M. (2017). Smooth muscle-selective nuclear factor‐κb inhibition reduces phosphate‐induced arterial medial calcification in mice with chronic kidney disease. J. Am. Heart Assoc. 6 (11), e007248. doi:10.1161/jaha.117.007248
Zhang, H., Chen, J., Shen, Z., Gu, Y., Xu, L., Hu, J., et al. (2018). Indoxyl sulfate accelerates vascular smooth muscle cell calcification via microRNA-29b dependent regulation of Wnt/β-catenin signaling. Toxicol. Lett. 284, 29–36. doi:10.1016/j.toxlet.2017.11.033
Keywords: vascular calcification, smooth muscle cell, miRNA-204, triptolide, Morphogenetic protein-2
Citation: Pei Y, Zheng Y, Ding Y, Xu Q, Cao D, Wu Y, Wang R, Yang J, Liang J, Ma Q, Ge H (2021) Triptolide Attenuates Vascular Calcification by Upregulating Expression of miRNA-204. Front. Pharmacol. 11:581230. doi: 10.3389/fphar.2020.581230
Received: 08 July 2020; Accepted: 28 October 2020;
Published: 11 January 2021.
Edited by:
Benzhi Cai, Second Affiliated Hospital of Harbin Medical University, ChinaReviewed by:
Xu Teng, Hebei Medical University, ChinaShanzhi Wang, University of Arkansas at Little Rock, United States
Copyright © 2021 Pei, Zheng, Ding, Xu, Cao, Wu, Wang, Yang, Liang, Ma and Ge. This is an open-access article distributed under the terms of the Creative Commons Attribution License (CC BY). The use, distribution or reproduction in other forums is permitted, provided the original author(s) and the copyright owner(s) are credited and that the original publication in this journal is cited, in accordance with accepted academic practice. No use, distribution or reproduction is permitted which does not comply with these terms.
*Correspondence: Hai-long Ge, gehailong@126.com
†These authors have contributed equally to this work