- 1Institutes for Systems Genetics, Frontiers Science Center for Disease-related Molecular Network, West China Hospital, Sichuan University, Chengdu, China
- 2Center for Systems Biology, Soochow University, Suzhou, China
Emerging evidence has shown a strong association between neuropathic pain and chronic diseases. In recent years, the treatment of neuropathic pain has attracted more attention. Natural products, such as capsaicin and resiniferatoxin, have been well utilized to treat this disease. In this study, we aim to compare the regulatory effects of capsaicin and resiniferatoxin on pain-related genes as well as on genes with no direct association with pain. Public transcriptomic and microarray data on gene expression in the dorsal root ganglia and genes associated with TRPV1 (+) neurons were obtained from the GEO database and then analyzed. Differentially expressed genes were selected for further functional analysis, including pathway enrichment, protein-protein interaction, and regulatory network analysis. Pain-associated genes were extracted with the reference of two pain gene databases and the effects of these two natural drugs on the pain-associated genes were measured. The results of our research indicate that as compared to capsaicin, resiniferatoxin (RTX) regulates more non pain-associated genes and has a negative impact on beneficial genes (off-targets) which are supposed to alleviate nociception and hypersensitivity by themselves. So, based on this study, we may conclude that capsaicin may be less potent when compared to RTX, but it will elicit considerably less adverse effects too. Thereby confirming that capsaicin could be used for the efficient alleviation of neuropathic pain with possibly fewer side effects.
Introduction
Neuropathic pain is one of the most critical neurological diseases and disorders. It affects approximately 3.3 to 17.9% of the population (Cohen and Mao, 2014; He et al., 2020). A total of 14.7% of Chinese patients suffering from chronic pain in Hong Kong had neuropathic characteristics (Cheung et al., 2017). For the neuropathic and ischemic pain, neuromodulative treatment is a modern and effective mode of treatment (Sokal et al., 2011). Neuromodulation can be achieved by electrical or chemical methods, it aims to alter communication between nerves which can lead to an increase in pain threshold by modifying nociception, hypersensitivity, and analgesia (Richards et al., 2012). Traditionally, natural products were used for the treatment of various ailments, and now due to advancements in scientific research fields, natural products are being validated by scientific methods and are being used in the scientific treatment of various diseases and disorders (Scotti et al., 2016; Singla and Dubey, 2019; Singla et al., 2019). Researchers have also validated the role of natural products from terrestrial plants and marine sources, for the treatment of neuropathic pain (Alonso et al., 2003; Quintans et al., 2014).
Capsaicin, a well-known component obtained from various species of the Capsicum genus (chili peppers), is a very potent agent for the treatment of neuropathic pain. Apart for its potential in the treatment of neuropathic pain, capsaicin also acts as an anti-oxidant, anti-obesity, and is cardioprotective (Lu et al., 2020; Qiao et al., 2020). Its neuropathic mechanism is widely thought to be modulated through the TRPV1 channel (Caterina et al., 1997; Bais and Greenberg, 2020). After clinical level validation, the topical formulation of capsaicin is widely used and quite popular, specifically in the form of an 8% topical cutaneous patch (Baron et al., 2017; Anand et al., 2019), 0.075% topical cream (Derry et al., 2009), and 0.075% lotion (Kulkantrakorn et al., 2019).
Resiniferatoxin (RTX), a capsaicin analogue obtained from the cactus-like plant, Euphorbia resinifera (Hergenhahn et al., 1984), is 1,000 times more potent than capsaicin for the alleviation of neuropathic pain (Maggi et al., 1990). The mode of neuropathic pain alleviation in the case of RTX is also via the TRPV1 channel (Kissin and Szallasi, 2011). Sorreto Therapeutics, along with other organizations, are conducting clinical trials to establish the clinical efficacy of RTX in treating severe pain in cancer and knee osteoarthritis (Heiss et al., 2014; Hockman et al., 2018; Unger et al., 2019). The treatment of sensory neurons with capsaicin or RTX causes calcium cytotoxicity that rapidly sutures and selectively deletes TRPV1(+) neurons (Isensee et al., 2014).
Isensee and co-workers had studied the effect of capsaicin and RTX in the dorsal root ganglion of rats and submitted the gene expression data in the NCBI (GSE59727). In their research, though they had used and collected data on both capsaicin and RTX, in their manuscript, they mainly focused on the characterization of genes associated with TRPV1(+) neurons. In their published manuscript, they had not correlated and compared the gene regulation of capsaicin and RTX in detail. Very little information has been made available for readers on capsaicin. Further, to the best of our knowledge, there has been no detailed comparison on the effect of capsaicin and RTX on pain genes and non pain-associated genes (Isensee et al., 2014). As RTX is proposed to be highly potent when compared to the already established capsaicin, and the evidence collection for its use in cancer and knee osteoarthritis is in process, it is extremely important to look at both of the drugs in terms of proteomics and gene regulations. This will help in the assessment of the possible adverse effects on the long-term usage of the drugs.
So, in our present study, we have reassessed the transcriptomic data submitted by the team of Isensee and analyzed it in the perspective as mentioned.
Materials and Methods
Microarray Data
The raw data of GSE59727, including 12 tissues (4 tissues for DMSO, 4 tissues for capsaicin, and 4 tissues for RTX), were obtained from the GEO database (https://www.ncbi.nlm.nih.gov/geo/), using the key words “capsaicin” AND “Rattus norvegicus” [porgn::txid10116] AND “neuron” and “tissues” (attribute name). These data were based on the GPL6101 platforms (Illumina ratRef-12 v1.0 expression beadchip) contributed to by Isensee and co-workers (Isensee et al., 2014). Four replicate experiments with RNA from the dorsal root ganglia (DRG) neurons of one rat per experiment were performed. Segregated neurons were split up into three parts, treated with solvent DMSO (0.1%), capsaicin (10 µM), or RTX (100 nM), and were followed by gradient centrifugation for 30 min (Isensee et al., 2014).
Data Preprocessing and Differentially Expressed Gene Identification
The R package “limma V3.40.6” was used to identify DEGs in rat tissues compared with corresponding tissues treated with DMSO (0.1%) and capsaicin (10μM) or DMSO (0.1%) and RTX (100nM). We used log2-transformation to normalized the data, after that we used the normalized data for further analysis. We used the t-test and Benjamini–Hochberg methods to calculate the p-value and adjusted the p-value and logFC accordingly. To get the differentially expressed genes we set the threshold value as; p-value < 0.01 and | logFC | > 0.5, in both cases.
Enrichment Analysis
ShinyGO is a graphical web application that displays the functional acuteness from a set of genes; which was developed under different R/Bioconductor packages. In this study, we use ShinyGO (http://bioinformatics.sdstate.edu/go/) to perform enrichment analysis (Ge et al., 2020). For the enrichment analysis we set the cutoff p-value = 0.05.
Analysis of the Protein–Protein Interaction (PPI) Network of the DEGs
We used the Search Tool for the Retrieval of Interacting Genes (STRING v11.0) database (http://string-db.org) for constructing the PPI network of DEGs both from capsaicin and RTX-treated conditions. Then, the Cytoscape software (version 3.8.0) with the MCODE and Networkanalyzer app was used to display the PPI networks between the DEGs.
Differentiation Between Pain-Associated Genes and Non-Pain Genes
The DEGs for capsaicin and RTX were then subjected to the Pain Networks database (http://www.painnetworks.org/rat/) for filtering of the pain-related genes with categorization with the Pain Gene Enrichment (PGE) score (Perkins et al., 2013). Further, DEGs for capsaicin and RTX were then subjected to the pain gene database (http://www.jbldesign.com/jmogil/PainGenedb_content.html) for more precise filtering of pain-related genes as the mentioned database also specifies the default function of the pain-related genes (Lacroix-Fralish et al., 2007).
Results and Discussion
Identification of Differentially Expressed Genes
We separated the datasets into two parts, one (capsaicin treatment group) is, DMSO (0.1%) and capsaicin (10µM); the other (RTX treatment group) is, DMSO (0.1%) and RTX (100nM). A total of 274 DEGs were identified, including 84 upregulated and 190 downregulated genes in the case of the capsaicin treatment group. But in the case of the RTX treatment group, a total of 444 DEGs were identified, including 171 upregulated and 273 downregulated genes (Figure 1). The top 30 upregulated and downregulated DEGs for capsaicin and RTX are displayed in Tables S1 and S2, respectively. Further on the basis of the filters adopted, we found 102 genes which were common in both RTX and capsaicin (Figure 2).
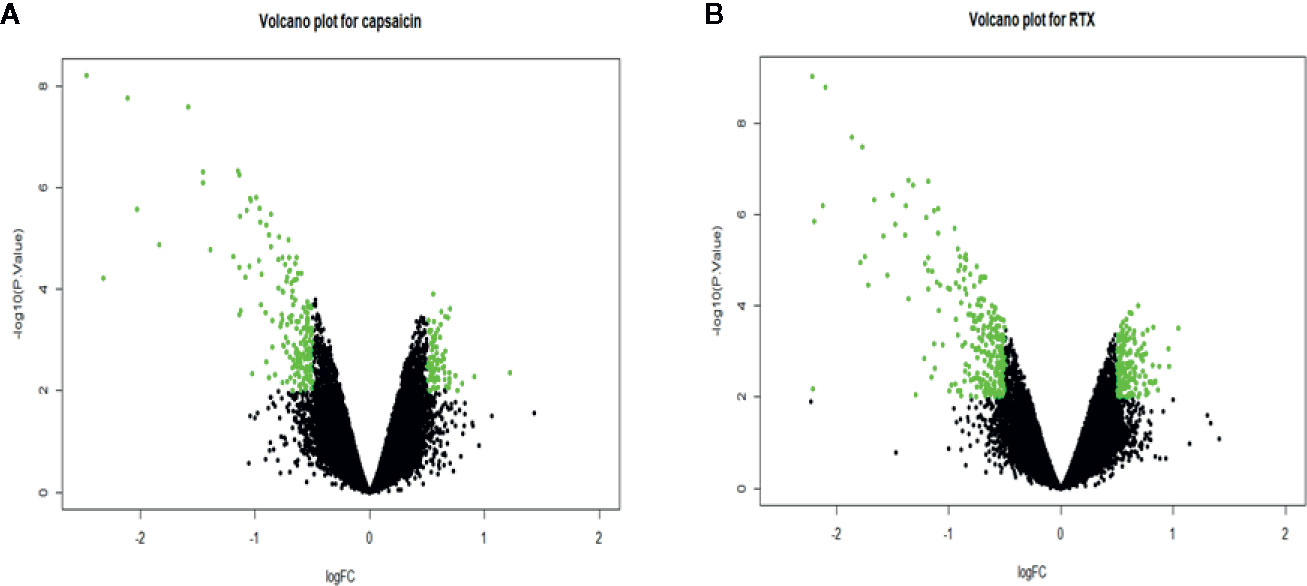
Figure 1 Volcano plot for representing the upregulated and downregulated genes in the capsaicin treatment group (A) and the RTX treatment group (B).
Enrichment Analysis
Gene ontology (GO) enrichment analysis showed that DEGs for both RTX and capsaicin-treated conditions were significantly enriched in the biological process (BP), cellular component (CC), and molecular function (MF). Furthermore, KEGG pathway enrichment analysis indicated that DEGs were significantly enriched in 30 pathways (Figure 3). Both the RTX and capsaicin-treated genes share some GO (biological process) like ion transport, the regulation of ion transport, the regulation of transport, cation transport and the regulation of the multicellular organismal process. In the case of GO (cellular component), they share common cellular components like cell body, neuronal cell body, plasma membrane parts, neuron parts, and synapses. In GO (molecular function) enrichment, they share hormone activity, neuropeptide receptor binding, G protein-coupled receptor binding, molecular function regulators, and neuropeptide hormone activity. The KEGG pathways show that genes after treatment with both RTX and capsaicin are involved in neuronal functions, as well as other functions which are closely related with pain (Figures 3A, B). There is a KEGG pathway which is common to both the RTX and capsaicin-treated genes, like the neuroactive ligand-receptor interaction (Figures 3A, B). These genes appear in several signaling pathways that are specific to neurons, pain, and also to cellular machinery/organelles that are found in most cell types.
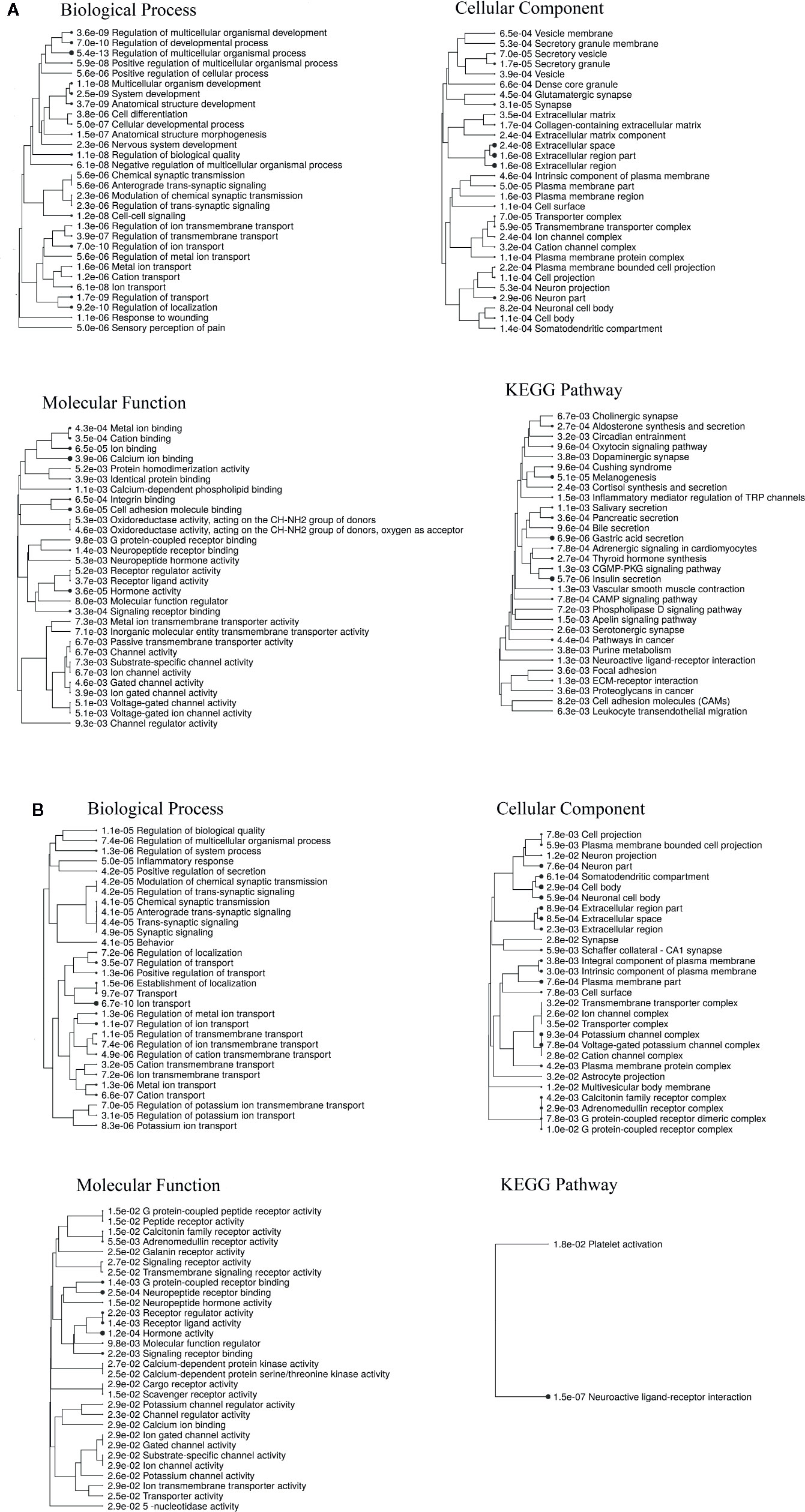
Figure 3 (A) Enrichment analysis of the DEGs for RTX. (B) Enrichment analysis of the DEGs for capsaicin.
Protein–Protein Interaction (PPI) Network of the DEGs
Based on the STRING online database for Rattus norvegicus, a total of 274 DEGs (84 upregulated and 190 downregulated genes) from capsaicin-treated conditions and 444 DEGs (171 upregulated and 273 downregulated genes) were filtered into the DEGs PPI network complex from RTX-treated conditions. Initially, in the case of capsaicin, the original number of nodes and edges was 183 and 164, respectively, where the expected number of edges was 59 (Figure S1). Hence the network had significantly more interactions than expected. Here the average node degree was 1.79 and the average local clustering coefficient was 0.363. But in order to have better protein-protein interaction analysis, this was further expanded to a level where the number of nodes and edges were 243 and 559, respectively, with the expected number of edges at 221. Average node degree was improved to 4.6 with an average local clustering coefficient of 0.484 (Figure S2). Similarly, in case of RTX, the original number of nodes and edges was 325 and 531, respectively, with an average node degree of 3.27 where the expected number of edges was 192, and the average local clustering coefficient was 0.36 (Figure S3). It was further expanded to a level where number of nodes and edges were 375 and 1,101, respectively, with an expected number of edges of 438. The average node degree was improved to 5.87 with an average local clustering coefficient of 0.425 (Figure S4). In both cases, the PPI enrichment p-value was less than 1.0e-16.
After subjecting the STRING dataset to Cytoscape 3.8.0, the protein-protein interactions were analyzed using the Networkanalyzer and MCODE application and different genes were categorized on the basis of degree of centrality (DoC). The genes having higher DoC have been represented both by bigger size as well as by different color for the ease of readers. In case of capsaicin, the color gradient is as follows: red for 15-27 DoC; green for 9.99-15 DoC; yellow for 3-9.99 DoC, and grey for below 3 DoC. The genes in the capsaicin-treated group with a higher DoC were Sst (upregulated), Crh (downregulated), and Tac1 (downregulated) (Figure 4). There were some more genes which were shown to have a higher DoC like Calca, Cxcl12, Gnb1, and Epha2, but they were not originally expressed by capsaicin. They were in fact the extended network genes by STRING. Other genes with a good DoC after capsaicin treatment were Gal (downregulated), Nmu (upregulated), RT1-Da (upregulated), Ccl4 (upregulated), Npy5R (downregulated), Lpar3 (downregulated), and Fancl (upregulated). Three main clusters of hub genes have also been identified using the MCODE app in the capsaicin-treated group (Figure 5). The fact that cluster B had 11 genes is of importance and some of the significant genes will be briefly discussed later.
In the case of RTX, the color gradient is as follows: red for 29.78-61 DoC; green for 20-29.78 DoC; blue for 15.5-20 DoC; yellow to blue for 4.3-15.5 DoC; and grey for below 4.3 DoC. The genes in the RTX-treated group with a higher DoC were Fn1 (upregulated), Edn1 (upregulated), Spp1 (upregulated), and Cd44 (downregulated) (Figure 6). There were some more genes which were shown to have a higher DoC like Src, Ngf, Gnb1, and F2, but they were not the originally expressed by RTX. They were in fact extended network genes by STRING. Other genes with a good DoC after RTX treatment were Ins2 (downregulated), Col1a1 (upregulated), Vcam1 (upregulated), Ptgs2 (upregulated), Tac1 (downregulated), and Ntrk1 (downregulated). Three main clusters of hub genes have also been identified using the MCODE app in RTX-treated group (Figure 7). All these three clusters A-C are of importance and some of the significant genes from them will be briefly discussed later.
After the filtering of DEGs for capsaicin and RTX through the pain networks database, the DEGs were categorized by their PGE score. In the case of the capsaicin-treated group, out of 190 downregulated genes, there were only 42 genes with a PGE score ranging from 1-100. The genes with a PGE>20 were Gnaq, Tnr, Tac1, Gfra2, Acpp, Ptgir, Nt5e, Amigo2, Kcnip1, Adcyap1, Rgs4, Lpar3, Grik1, Kcnip2, Trpv1, Hrh2, Kcnj5, Gal, Plcl1, Kcnk2, and Iapp (arranged in increasing order). Out of 84 upregulated genes in the capsaicin-treated group, only 15 genes had a PGE score ranging from 1-100. The genes with a PGE>20 were Btk, Adrb1, Fcer1g, and Nmu (arranged in increasing order).
In the case of the RTX-treated group, out of 273 downregulated genes, there were only 75 genes with a PGE score ranging from 1-100. The genes with a PGE>20 were Camk2a, Gnaq, Plcb3, Comt, Tnr, Inpp5d, Ucp2, Tac1, Gfra2, Acpp, Adcy5, Ntrk1, Fgf13, Arhgap22, Nfe2l3, Ticam2, Nt5e, Amigo2, Adcyap1, Kcnip1, Cd22, Rgs4, Lpar3, Grik1, Kcnip2, Scn10a, Trpv1, Plcl1, Ece2, Scn11a, Hrh2, Kcnj5, Gal, Kcnk2, and Iapp (arranged in increasing order). Out of 171 upregulated genes in the RTX-treated group, only 43 genes had a PGE score ranging from 1-100. The genes with a PGE>20 were Sparc, Edn1, S100b, Itga5, Ptgs2, Sparcl1, Tyrp1, Fzd1, and Myl9 (arranged in increasing order). It is quite evident from the above data, that even at a low concentration, RTX is capable of regulating more pain-associated genes than capsaicin. But closely looking at the data, it also revealed that the effect of RTX is more non-specific when compared to capsaicin, because in the case of RTX, there are indeed more DEGs with no PGE score.
Further, filtering the DEGs through the Pain Genes database has its own significance as there are inherently few genes in our system which have been designed to alleviate pain, so the downregulation of such genes are actually not appropriate. As per filtering through this database, it has come to our notice that out of 274 DEGs in the capsaicin-treated group, only two upregulated and 21 downregulated DEGs had a direct influence on nociception, hypersensitivity, and analgesia (Table 1). On the other hand, in the case of the RTX-treated group, six upregulated and 31 downregulated DEGs had a direct influence on nociception, hypersensitivity, and analgesia (Table 2).
After overlapping the data obtained from both the databases, there were some interesting observations. For instance, there are a few pain-related genes like Ctss, Galr2, Kcnt1, and Ptgdr, which have a zero PGE score, but from the pain gene database, it is very clear that these genes have a pain-related function. Further, after cross-validation with the original source manuscript of Isensee and co-workers (Isensee et al., 2014) whose transcriptomic data have been reprocessed by our team, it is also observable that there are a few pain-associated genes like S100b, Spp1, Sparc, Tyrp1, Edn1, Gfra2, Hrh2, Ucp2, Ntrk1, Ece2, Plcl1, Runx1, Comt, and Scn10a which have not been discussed by them in their published manuscript, or been provided as information in form of the supplementary data.
There are few indirect genes associated with pain. For instance, the Ina gene, which is downregulated by RTX (LogFC: -0.905) and capsaicin (LogFC: -0.795), is not directly a pain gene, but is associated with multiple genes which are associated with an increase in nociception like Cdk5, CdK5r1, Csk, Camk2a, Prkce, Pmp22, and Tyrp1 and decreases analgesia like Grk5, Prkce, and Prkcc. So, the downregulation of the Ina gene somehow affects these genes as well (Lacroix-Fralish et al., 2007).
The Hal gene, which is downregulated by capsaicin (LogFC: -0.665), is not a direct pain gene, but is associated with Kcnd2 which is linked with increased hypersensitivity, thus the downregulation of Hal gene somehow influences the effect of Kcnd2 (Lacroix-Fralish et al., 2007).
The Gnal gene, which is downregulated by capsaicin (LogFC: -0.500), is linked with genes like Ccr5, Slc12a5, Slc12a2, and Trpv1 which can increase nociception (Lacroix-Fralish et al., 2007).
The Plat gene, which is upregulated by RTX (LogFC: 0.536), is not directly a pain gene either, but it is associated with the Ptafr gene which is responsible for increased nociception and hypersensitivity. So upregulating the Plat gene will indirectly affects the Ptafr gene as well (Lacroix-Fralish et al., 2007).
Similarly, Lox is not a pain gene but it is upregulated by RTX (LogFC: 0.733), and is associated with many genes which are responsible for a decrease in nociception like Fstl1, Nedd4l, Crip2, Ret, Kcnq2, and Edn1 as well as a decrease in hypersensitivity like Nedd4l, Crip2, and Edn1 (Lacroix-Fralish et al., 2007).
While analyzing the data tabulated in Tables 1 and 2, we have segregated downregulated pain genes into desired/targets and undesired/off-targets. Here, desired targets means that their downregulation will decrease either nociception or hypersensitivity while undesired/off-targets means that those genes are already beneficial genes in alleviating nociception or hypersensitivity. Thus, the downregulation of off-targets here is somehow decreasing the innate capability of the host system to alleviate pain.
Capsaicin Induced Upregulated Genes With No Direct Association With Pain Nociception
We will discuss here the biological functions of the genes which fall under the top 10 upregulated DEGs after capsaicin treatment (Table S1 lists the top 30 upregulated and downregulated genes), without having any direct association with pain nociception. Olr1349 and Olr190 are genes that encode the olfactory receptor which is primarily responsible for the perception of smell (Gibbs et al., 2004; Antunes and Simoes De Souza, 2016). Tmprss5 which is a transmembrane serine protease 5 (also known as spinesin) is reported to be involved in the function of astrocytes in the spinal cord (Yamaguchi et al., 2008). Notch3 is regarded as the key gene for the maintenance and function of vascular smooth muscle cells, including those which are involved in the blood supply to the brain (Lin et al., 2019). But some of recent studies also suggest that Notch3 plays a vital role in oncogenesis, the maintenance of tumors, and chemotherapy resistance (Aburjania et al., 2018). Ccl4 is a monokine possessing chemokinetic and inflammatory properties and is suggested to be involved in neutrophil recruitment in the intrapulmonary region. It is also recorded as one of the major suppressive factors for HIV (Farquhar et al., 2005; Lee et al., 2007). The Car13 gene encodes carbonic anhydrases 13 (CA XIII) which is a cytosolic isoform of carbonic anhydrases. CA XIII, though it has a moderate catalytic activity, may contribute in maintaining the acid-base homeostasis in the kidneys, GI tract, and in the reproductive system (Hilvo et al., 2008). The Napsa gene encodes napsin A aspartic peptidase which is preferentially expressed in the kidneys, lungs, and spleen (Schauer-Vukasinovic et al., 2000). Its expression is commonly reported to be associated with pulmonary adenocarcinomas and renal cell carcinomas (Nam et al., 2016). The Colec12 gene is a collectin sub-family member 12, which encodes the scavenger receptor with the C-type lectin/carbohydrate recognition domain. These receptors are considered as an important member contributing to innate immunity (Nakamura et al., 2001). The Eif2a gene encodes eukaryotic translation initiation factor 2-alpha, which is generally observed as an environmental stress-induced gene, and phosphorylation of which results in the reduction of global protein synthesis (Hong et al., 2016). Though these genes may not have a direct association with pain nociception, most of these genes are found to influence nervous system functionality including inflammation.
Capsaicin Induced Downregulated Genes With No Direct Association With Pain Nociception
We will discuss here the biological functions of the genes which fall under the top 10 downregulated DEGs after capsaicin treatment (Table S1 lists the top 30 upregulated and downregulated genes), without having any direct association with pain nociception. The Cartpt gene encodes to the cocaine and amphetamine-regulated transcript (CART) peptide, which is evidently involved in the negative regulation of estradiol production (Lv et al., 2009), and is also involved in various stages of opioid addiction (Bakhtazad et al., 2016). The Mrgprx3 and Mrgprd genes encode for a G-protein coupled receptor (GPCR) for which adenine acts as the endogenous ligand and which plays a role in nociception, although its clear and specific role has not yet been defined (Bender et al., 2002). The Kcnip1 gene encodes for the potassium voltage gated ion channel, Kcnip1 which is primarily expressed in the brain and testes. Potassium channel blockers are used for the treatment of neuromuscular disorders, thus the downregulation of this gene seems to play an important role in the improvement of the nervous system (Del Pino et al., 2015). The Slc51a gene encodes for the organic solute transporter, and is involved in the transportation of bile acid as well as steroids in the intestine, renal, and biliary epithelia (Ballatori et al., 2005; Christian et al., 2012). The Amdhd1 gene encodes for the aminohydrolase domain which is involved in the formation of intestinal stem cells in adults (Okada et al., 2015). The Trpc3 gene encodes for member 3 of the subfamily C of the transient receptor potential cation channel which is a calcium-activated cation channel, and it is involved in the development of febrile seizures. Thus downregulation of the Trpc3 gene or inhibition of this calcium-activated cation channel significantly attenuates the susceptibility of seizures, neuronal cell death, and neuroinflammation (Sun et al., 2018).
RTX Induced Upregulated Genes With No Direct Association With Pain Nociception
We will discuss here the biological functions of the genes which fall under the top 10 upregulated DEGs after RTX treatment (Table S2 lists the top 30 upregulated and downregulated genes), without any direct association with pain nociception. As discussed above in the capsaicin section, the Notch3 gene is involved in the maintenance of vascular smooth muscle cells (Lin et al., 2019). Itga5, though it has a PGE score when filtered through the pain network database, no direct association was found when screened through the pain gene database. Itga5 encodes for the integrin subunit alpha 5, and is involved in the regulation of spine morphogenesis as well as the formation of synapses in hippocampal neurons (Webb et al., 2007). The Traf3ip3 gene encodes TRAF3 interacting protein 3 which modulates the pathway associated with c-Jun N-terminal kinase signal transduction, and thereby mediates cell growth (Dadgostar et al., 2003). Further, its upregulation has also been observed in various types of cancers (Nasarre et al., 2018). The Hpse gene is encodes for heparanase which is a endo-beta-glucouronidase and catalyzes the cleavage of heparan sulfate. Heparanase is evidently involved in neuroinflammatory responses (Changyaleket et al., 2017). The Mylip gene encodes the myosin regulatory light chain interacting protein which is involved in the Reelin-induced decrease of very low density lipoprotein receptors in neuronal systems (Do et al., 2013). The Pxn gene encodes to paxillin which is involved in the focal adhesion of cells with an extracellular matrix (Torres et al., 2008). Further, paxillin was reported to be involved in the regulation of cytoskeleton proteins like α-actin, α-tubulin, and destrin (Chen et al., 2014). The Aoc3 gene encodes for amine oxidase, copper containing 3, which is also recorded as vascular adhesion protein-1 and found to be involved in leucocyte recruitment (Noda et al., 2008). The Plpp3 gene encodes for the phospholipid phosphatase 3 enzyme and it is involved in the transport carrier’s formation in Golgi bodies (Gutierrez-Martinez et al., 2013). The Bace2 gene encodes for the beta-secretase 2 enzyme which is also recorded as aspartic protease and is involved in the cleavage of the precursor protein of amyloid-β into amyloid-β. Amyloid-β is well known to trigger neurodegenerative diseases like Alzheimer’s disease (Bettegazzi et al., 2011). The S100a16 gene encodes for S100 calcium-binding protein A16 which is involved in adipogenesis and thus weight gain (Zhang et al., 2014).
RTX Induced Downregulated Genes With No Direct Association With Pain Nociception
We will discuss here the biological functions of the genes which fall under the top 10 downregulated DEGs after RTX treatment (Table S2 lists the top 30 upregulated and downregulated genes), without any direct association with pain nociception. As already described in the relevant capsaicin section, the Cartpt gene is involved in various stages of opioid addiction (Bakhtazad et al., 2016). Though, the Crh gene does not have any association with pain, it possesses a good DoC (Figure 6) which indicates that it is closely associated with many other genes. Further, Figure 4 represents the Crh gene in the capsaicin-treated protein-protein interaction, but in capsaicin, the downregulation of the Crh gene did not fall into the top 30 downregulated genes (Table S1). The Crh gene encodes the corticotrophin releasing hormone which is well known for its mediatory effect in the neuroendocrine stress response (Kozakai et al., 2019). The Mrgprx3 and Mrgprd genes which encode GPCR have recorded significance in nociception, though a specific role is as yet unclear (Bender et al., 2002). The Gfra3 gene encodes for the alpha 3 isoform of GDNF family receptor and is critically involved in neuronal cell protection (Hoke et al., 2000). The functionality of the Slc51a gene which encodes for the organic solute transporter has already been discussed in the capsaicin section (Ballatori et al., 2005; Christian et al., 2012).
There is no question about the potency of RTX, but selectivity should also be considered and a balance of both is what required at the clinical level. With reference to Figure 8, it can be easily observed that the downregulation of desired pain genes affecting nociception like Iapp, Trpv1, Adcyap1, Grik1, Tac1, Hrh2, and Gnaq was more evident in the RTX-treated group when compared to the capsaicin-treated group. Interestingly, only capsaicin was able to downregulate Ptgir, while only RTX was able to downregulate some other desired pain genes like Camk2a, Scn11a, Adcy5, Cacna2d1, Ntrk1, Runx1, and Scn10a.
With reference to Figure 9, it is evident that the downregulation of desired pain genes affecting hypersensitivity like Lpar3, Trpv1, Adcyap1, Gal, and Gnaq was observed more in the RTX-treated group when compared to the capsaicin-treated group. Similarly, only capsaicin was able to downregulate Ptgir while only RTX was able to downregulate Camk2a, Adcy5, Cacna2d1, Runx1, and Scn10a.
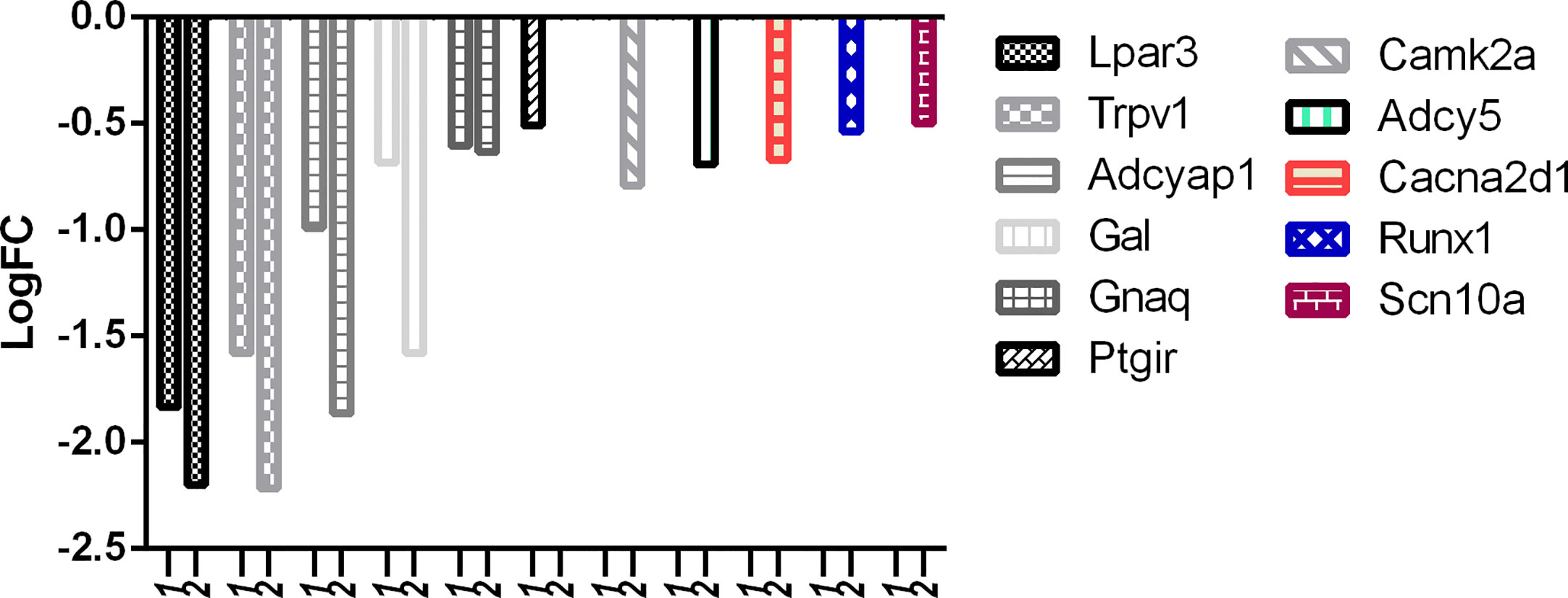
Figure 9 Downregulation of pain genes responsible for increased hypersensitivity. 1: capsaicin; 2: RTX.
But in comparison with capsaicin, RTX was not only able to downregulate more of the target pain genes, but also more of the off-target pain genes. With reference to Figure 10, it is clear that the downregulation of off-target pain genes associated with nociception like Kcnk2, Kcnj5, Gal, Nt5e, Gfra2, and Ptgdr was higher in RTX when compared to capsaicin, except Plcl1 which was downregulated more by capsaicin. Further, only RTX could downregulate the Comt gene. With reference to Figure 11, it can be seen that the downregulation of off-target pain genes associated with hypersensitivity like Kcnk2, Acpp, Nt5e, and Kcnt1 was more evident RTX when compared to capsaicin, except Plcl1 which was downregulated more by capsaicin.
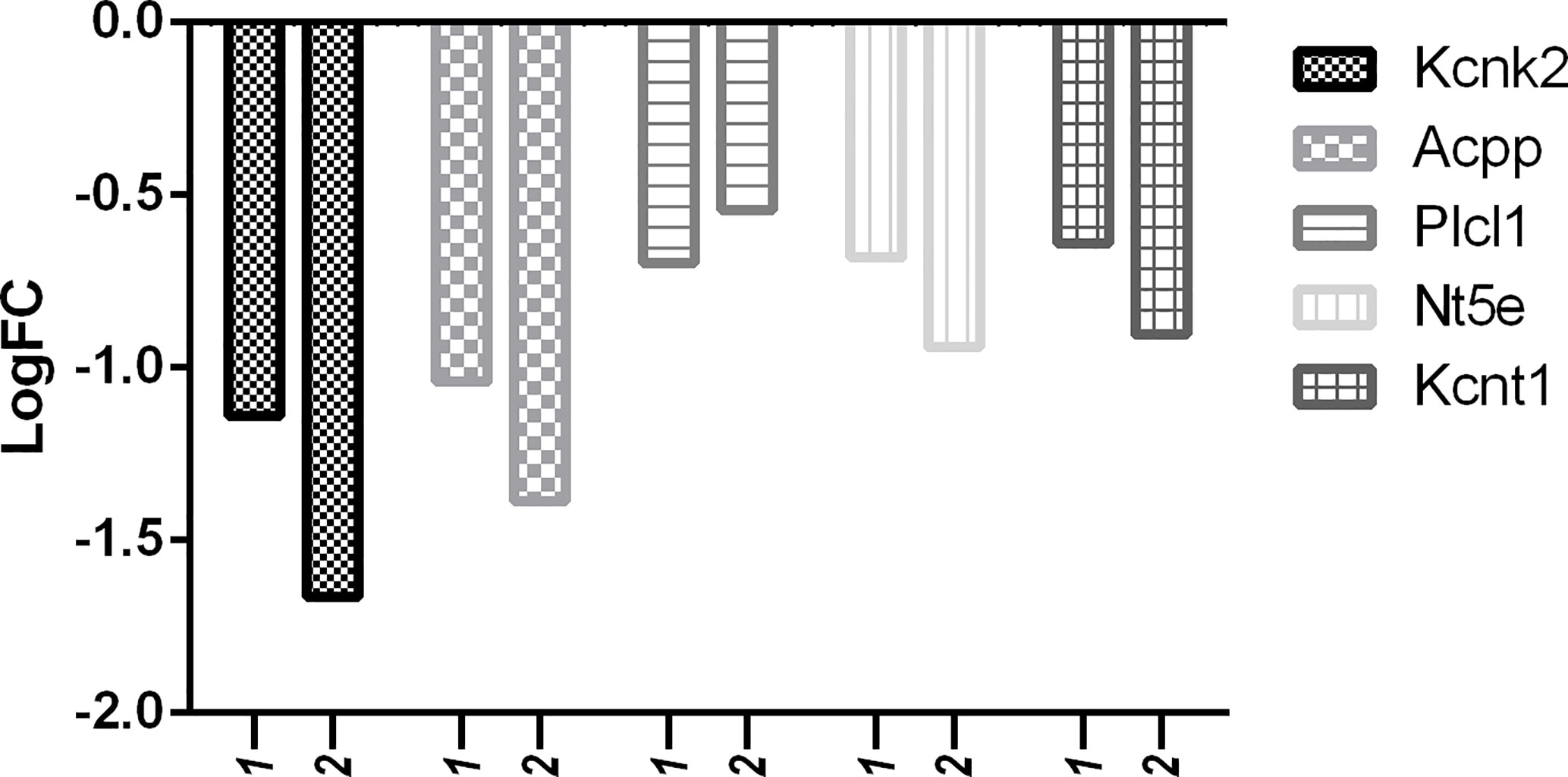
Figure 11 Downregulation of pain genes responsible for decreased hypersensitivity. 1: capsaicin; 2: RTX.
So, after reassessing the transcriptomic data, we have found that when compared to capsaicin, RTX not only upregulates more non-pain related genes, but also downregulates more undesired/off-targets pain genes. This proposes that RTX is a more potent drug compared to capsaicin for its clinical implications.
Data Availability Statement
The datasets presented in this study can be found in online repositories. The names of the repository/repositories and accession number(s) can be found below: https://www.ncbi.nlm.nih.gov/genbank/, GSE59727.
Author Contributions
RS and BS designed the experiment. RS, AS, and MS participated in data analysis, All authors contributed to the article and approved the submitted version.
Funding
This work was supported by the National Key Research and Development Program of China (2016YFC1306605) and the National Natural Science Foundation of China (Grant Nos. 31670851).
Conflict of Interest
The authors declare that the research was conducted in the absence of any commercial or financial relationships that could be construed as a potential conflict of interest.
Supplementary Material
The Supplementary Material for this article can be found online at: https://www.frontiersin.org/articles/10.3389/fphar.2020.551786/full#supplementary-material
References
Aburjania, Z., Jang, S., Whitt, J., Jaskula-Stzul, R., Chen, H., Rose, J. B. (2018). The Role of Notch3 in Cancer. Oncologist 23, 900–911. doi: 10.1634/theoncologist.2017-0677
Alonso, D., Khalil, Z., Satkunanthan, N., Livett, B. G. (2003). Drugs from the sea: conotoxins as drug leads for neuropathic pain and other neurological conditions. Mini Rev. Med. Chem. 3, 785–787. doi: 10.2174/1389557033487746
Anand, P., Elsafa, E., Privitera, R., Naidoo, K., Yiangou, Y., Donatien, P., et al. (2019). Rational treatment of chemotherapy-induced peripheral neuropathy with capsaicin 8% patch: from pain relief towards disease modification. J. Pain Res. 12, 2039–2052. doi: 10.2147/JPR.S213912
Antunes, G., Simoes De Souza, F. M. (2016). Olfactory receptor signaling. Methods Cell Biol. 132, 127–145. doi: 10.1016/bs.mcb.2015.11.003
Bais, S., Greenberg, R. M. (2020). Schistosome TRP channels: An appraisal. Int. J. Parasitol. Drugs Drug Resist. 13, 1–7. doi: 10.1016/j.ijpddr.2020.02.002
Bakhtazad, A., Vousooghi, N., Garmabi, B., Zarrindast, M. R. (2016). Evaluation of CART peptide level in rat plasma and CSF: Possible role as a biomarker in opioid addiction. Peptides 84, 1–6. doi: 10.1016/j.peptides.2016.06.010
Ballatori, N., Christian, W. V., Lee, J. Y., Dawson, P. A., Soroka, C. J., Boyer, J. L., et al. (2005). OSTalpha-OSTbeta: a major basolateral bile acid and steroid transporter in human intestinal, renal, and biliary epithelia. Hepatology 42, 1270–1279. doi: 10.1002/hep.20961
Baron, R., Treede, R. D., Birklein, F., Cegla, T., Freynhagen, R., Heskamp, M. L., et al. (2017). Treatment of painful radiculopathies with capsaicin 8% cutaneous patch. Curr. Med. Res. Opin. 33, 1401–1411. doi: 10.1080/03007995.2017.1322569
Bender, E., Buist, A., Jurzak, M., Langlois, X., Baggerman, G., Verhasselt, P., et al. (2002). Characterization of an orphan G protein-coupled receptor localized in the dorsal root ganglia reveals adenine as a signaling molecule. Proc. Natl. Acad. Sci. U. S. A. 99, 8573–8578. doi: 10.1073/pnas.122016499
Bettegazzi, B., Mihailovich, M., Di Cesare, A., Consonni, A., Macco, R., Pelizzoni, I., et al. (2011). beta-Secretase activity in rat astrocytes: translational block of BACE1 and modulation of BACE2 expression. Eur. J. Neurosci. 33, 236–243. doi: 10.1111/j.1460-9568.2010.07482.x
Caterina, M. J., Schumacher, M. A., Tominaga, M., Rosen, T. A., Levine, J. D., Julius, D. (1997). The capsaicin receptor: a heat-activated ion channel in the pain pathway. Nature 389, 816–824. doi: 10.1038/39807
Changyaleket, B., Chong, Z. Z., Dull, R. O., Nanegrungsunk, D., Xu, H. (2017). Heparanase promotes neuroinflammatory response during subarachnoid hemorrhage in rats. J. Neuroinflam. 14, 137. doi: 10.1186/s12974-017-0912-8
Chen, Y., Yi, B., Wang, Z., Gu, J., Li, Y., Cui, J., et al. (2014). Paxillin suppresses the proliferation of HPS rat serum treated PASMCs by up-regulating the expression of cytoskeletal proteins. Mol. Biosyst. 10, 759–766. doi: 10.1039/c3mb70391f
Cheung, C. W., Choi, S. W., Wong, S. S. C., Lee, Y., Irwin, M. G. (2017). Changes in Prevalence, Outcomes, and Help-seeking Behavior of Chronic Pain in an Aging Population Over the Last Decade. Pain Pract. 17, 643–654. doi: 10.1111/papr.12496
Christian, W. V., Li, N., Hinkle, P. M., Ballatori, N. (2012). beta-Subunit of the Ostalpha-Ostbeta organic solute transporter is required not only for heterodimerization and trafficking but also for function. J. Biol. Chem. 287, 21233–21243. doi: 10.1074/jbc.M112.352245
Cohen, S. P., Mao, J. (2014). Neuropathic pain: mechanisms and their clinical implications. BMJ 348, f7656. doi: 10.1136/bmj.f7656
Dadgostar, H., Doyle, S. E., Shahangian, A., Garcia, D. E., Cheng, G. (2003). T3JAM, a novel protein that specifically interacts with TRAF3 and promotes the activation of JNK(1). FEBS Lett. 553, 403–407. doi: 10.1016/S0014-5793(03)01072-X
Del Pino, J., Frejo, M. T., Baselga, M. J. A., Capo, M. A., Moyano, P., Garcia, J. M., et al. (2015). Neuroprotective or neurotoxic effects of 4-aminopyridine mediated by KChIP1 regulation through adjustment of Kv 4.3 potassium channels expression and GABA-mediated transmission in primary hippocampal cells. Toxicology 333, 107–117. doi: 10.1016/j.tox.2015.04.013
Derry, S., Lloyd, R., Moore, R. A., Mcquay, H. J. (2009). Topical capsaicin for chronic neuropathic pain in adults. Cochrane Database Syst. Rev. 4, CD007393. doi: 10.1002/14651858.CD007393.pub2
Do, H. T., Bruelle, C., Tselykh, T., Jalonen, P., Korhonen, L., Lindholm, D. (2013). Reciprocal regulation of very low density lipoprotein receptors (VLDLRs) in neurons by brain-derived neurotrophic factor (BDNF) and Reelin: involvement of the E3 ligase Mylip/Idol. J. Biol. Chem. 288, 29613–29620. doi: 10.1074/jbc.M113.500967
Farquhar, C., Mbori-Ngacha, D. A., Redman, M. W., Bosire, R. K., Lohman, B. L., Piantadosi, A. L., et al. (2005). CC and CXC chemokines in breastmilk are associated with mother-to-child HIV-1 transmission. Curr. HIV Res. 3, 361–369. doi: 10.2174/157016205774370393
Ge, S. X., Jung, D., Yao, R. (2020). ShinyGO: a graphical enrichment tool for animals and plants. Bioinformatics 36, 2628–2629. doi: 10.1093/bioinformatics/btz931
Gibbs, R. A., Weinstock, G. M., Metzker, M. L., Muzny, D. M., Sodergren, E. J., Scherer, S., et al. (2004). Genome sequence of the Brown Norway rat yields insights into mammalian evolution. Nature 428, 493–521. doi: 10.1038/nature02426
Gutierrez-Martinez, E., Fernandez-Ulibarri, I., Lazaro-Dieguez, F., Johannes, L., Pyne, S., Sarri, E., et al. (2013). Lipid phosphate phosphatase 3 participates in transport carrier formation and protein trafficking in the early secretory pathway. J. Cell Sci. 126, 2641–2655. doi: 10.1242/jcs.117705
He, L., Han, G., Wu, S., Du, S., Zhang, Y., Liu, W., et al. (2020). Toll-like receptor 7 contributes to neuropathic pain by activating NF-kappaB in primary sensory neurons. Brain Behav. Immun. 87, 840–851. doi: 10.1016/j.bbi.2020.03.019
Heiss, J., Iadarola, M., Cantor, F., Oughourli, A., Smith, R., Mannes, A. (2014). (364) A Phase I study of the intrathecal administration of resiniferatoxin for treating severe refractory pain associated with advanced cancer. J. Pain 15, S67. doi: 10.1016/j.jpain.2014.01.275
Hergenhahn, M., Kusumoto, S., Hecker, E. (1984). On the active principles of the spurge family (Euphorbiaceae). V. Extremely skin-irritant and moderately tumor-promoting diterpene esters from Euphorbia resinifera Berg. J. Cancer Res. Clin. Oncol. 108, 98–109. doi: 10.1007/BF00390980
Hilvo, M., Innocenti, A., Monti, S. M., De Simone, G., Supuran, C. T., Parkkila, S. (2008). Recent advances in research on the most novel carbonic anhydrases, CA XIII and XV. Curr. Pharm. Des. 14, 672–678. doi: 10.2174/138161208783877811
Hockman, T. M., Cisternas, A. F., Jones, B., Butt, M. T., Osborn, K. G., Steinauer, J. J., et al. (2018). Target engagement and histopathology of neuraxial resiniferatoxin in dog. Vet. Anaesth. Analg. 45, 212–226. doi: 10.1016/j.vaa.2017.10.005
Hoke, A., Cheng, C., Zochodne, D. W. (2000). Expression of glial cell line-derived neurotrophic factor family of growth factors in peripheral nerve injury in rats. Neuroreport 11, 1651–1654. doi: 10.1097/00001756-200006050-00011
Hong, M. N., Nam, K. Y., Kim, K. K., Kim, S. Y., Kim, I. (2016). The small molecule ‘1-(4-biphenylylcarbonyl)-4-(5-bromo-2-methoxybenzyl) piperazine oxalate’ and its derivatives regulate global protein synthesis by inactivating eukaryotic translation initiation factor 2-alpha. Cell Stress Chaperones 21, 485–497. doi: 10.1007/s12192-016-0677-5
Isensee, J., Wenzel, C., Buschow, R., Weissmann, R., Kuss, A. W., Hucho, T. (2014). Subgroup-elimination transcriptomics identifies signaling proteins that define subclasses of TRPV1-positive neurons and a novel paracrine circuit. PloS One 9, e115731. doi: 10.1371/journal.pone.0115731
Kissin, I., Szallasi, A. (2011). Therapeutic targeting of TRPV1 by resiniferatoxin, from preclinical studies to clinical trials. Curr. Top. Med. Chem. 11, 2159–2170. doi: 10.2174/156802611796904924
Kozakai, Y., Hori, K., Aye-Mon, A., Okuda, H., Harada, S. I., Hayashi, K., et al. (2019). The role of peripheral corticotropin-releasing factor signaling in a rat model of stress-induced gastric hyperalgesia. Biochem. Biophys. Res. Commun. 519, 797–802. doi: 10.1016/j.bbrc.2019.09.040
Kulkantrakorn, K., Chomjit, A., Sithinamsuwan, P., Tharavanij, T., Suwankanoknark, J., Napunnaphat, P. (2019). 0.075% capsaicin lotion for the treatment of painful diabetic neuropathy: A randomized, double-blind, crossover, placebo-controlled trial. J. Clin. Neurosci. 62, 174–179. doi: 10.1016/j.jocn.2018.11.036
Lacroix-Fralish, M. L., Ledoux, J. B., Mogil, J. S. (2007). The Pain Genes Database: An interactive web browser of pain-related transgenic knockout studies. Pain 1313, e1–e4. doi: 10.1016/j.pain.2007.04.041
Lee, J. S., Rosengart, M. R., Kondragunta, V., Zhang, Y., Mcmurray, J., Branch, R. A., et al. (2007). Inverse association of plasma IL-13 and inflammatory chemokines with lung function impairment in stable COPD: a cross-sectional cohort study. Respir. Res. 8, 64. doi: 10.1186/1465-9921-8-64
Lin, X., Li, S., Wang, Y. J., Wang, Y., Zhong, J. Y., He, J. Y., et al. (2019). Exosomal Notch3 from high glucose-stimulated endothelial cells regulates vascular smooth muscle cells calcification/aging. Life Sci. 232, 116582. doi: 10.1016/j.lfs.2019.116582
Lu, M., Chen, C., Lan, Y., Xiao, J., Li, R., Huang, J., et al. (2020). Capsaicin-the major bioactive ingredient of chili peppers: bio-efficacy and delivery systems. Food Funct. 11, 2848–2860. doi: 10.1039/D0FO00351D
Lv, L., Jimenez-Krassel, F., Sen, A., Bettegowda, A., Mondal, M., Folger, J. K., et al. (2009). Evidence supporting a role for cocaine- and amphetamine-regulated transcript (CARTPT) in control of granulosa cell estradiol production associated with dominant follicle selection in cattle. Biol. Reprod. 81, 580–586. doi: 10.1095/biolreprod.109.077586
Maggi, C. A., Patacchini, R., Tramontana, M., Amann, R., Giuliani, S., Santicioli, P. (1990). Similarities and differences in the action of resiniferatoxin and capsaicin on central and peripheral endings of primary sensory neurons. Neuroscience 37, 531–539. doi: 10.1016/0306-4522(90)90421-Y
Nakamura, K., Funakoshi, H., Tokunaga, F., Nakamura, T. (2001). Molecular cloning of a mouse scavenger receptor with C-type lectin (SRCL)(1), a novel member of the scavenger receptor family. Biochim. Biophys. Acta 1522, 53–58. doi: 10.1016/S0167-4781(01)00284-6
Nam, S. J., Kim, S., Kim, J. E., Lim, M. S., Elenitoba-Johnson, K. S., Kim, C. W., et al. (2016). Aberrant expression of napsin A in a subset of malignant lymphomas. Histol. Histopathol. 31, 213–221. doi: 10.14670/HH-11-669
Nasarre, P., Bonilla, I. V., Metcalf, J. S., Hilliard, E. G., Klauber-Demore, N. (2018). TRAF3-interacting protein 3, a new oncotarget, promotes tumor growth in melanoma. Melanoma Res. 28, 185–194. doi: 10.1097/CMR.0000000000000440
Noda, K., She, H., Nakazawa, T., Hisatomi, T., Nakao, S., Almulki, L., et al. (2008). Vascular adhesion protein-1 blockade suppresses choroidal neovascularization. FASEB J. 22, 2928–2935. doi: 10.1096/fj.07-105346
Okada, M., Miller, T. C., Fu, L., Shi, Y. B. (2015). Direct Activation of Amidohydrolase Domain-Containing 1 Gene by Thyroid Hormone Implicates a Role in the Formation of Adult Intestinal Stem Cells During Xenopus Metamorphosis. Endocrinology 156, 3381–3393. doi: 10.1210/en.2015-1190
Perkins, J. R., Lees, J., Antunes-Martins, A., Diboun, I., Mcmahon, S. B., Bennett, D. L., et al. (2013). PainNetworks: a web-based resource for the visualisation of pain-related genes in the context of their network associations. Pain 1542586, e2581–e2512. doi: 10.1016/j.pain.2013.09.003
Qiao, Y., Hu, T., Yang, B., Li, H., Chen, T., Yin, D., et al. (2020). Capsaicin Alleviates the Deteriorative Mitochondrial Function by Upregulating 14-3-3eta in Anoxic or Anoxic/Reoxygenated Cardiomyocytes. Oxid. Med. Cell Longev. 2020, 1750289. doi: 10.1155/2020/1750289
Quintans, J. S., Antoniolli, A. R., Almeida, J. R., Santana-Filho, V. J., Quintans-Junior, L. J. (2014). Natural products evaluated in neuropathic pain models - a systematic review. Basic Clin. Pharmacol. Toxicol. 114, 442–450. doi: 10.1111/bcpt.12178
Richards, B. L., Whittle, S. L., Buchbinder, R. (2012). Neuromodulators for pain management in rheumatoid arthritis. Cochrane Database Syst. Rev. 1, CD008921. doi: 10.1002/14651858.CD008921.pub2
Schauer-Vukasinovic, V., Wright, M. B., Breu, V., Giller, T. (2000). Cloning, expression and functional characterization of rat napsin. Biochim. Biophys. Acta 1492, 207–210. doi: 10.1016/S0167-4781(00)00069-5
Scotti, L., Singla, R. K., Ishiki, H. M., Mendonca, F. J., Da Silva, M. S., Barbosa Filho, J. M., et al. (2016). Recent Advancement in Natural Hyaluronidase Inhibitors. Curr. Top. Med. Chem. 16, 2525–2531. doi: 10.2174/1568026616666160414123857
Singla, R. K., Dubey, A. K. (2019). Molecules and Metabolites from Natural Products as Inhibitors of Biofilm in Candida spp. pathogens. Curr. Top. Med. Chem. 19, 2567–2578. doi: 10.2174/1568026619666191025154834
Singla, R. K., Kumar, R., Khan, S., Mohit, Kumari, K., Garg, A. (2019). Natural Products: Potential Source of DPP-IV Inhibitors. Curr. Protein Pept. Sci. 20, 1218–1225. doi: 10.2174/1389203720666190502154129
Sokal, P., Harat, M., Paczkowski, D., Rudas, M., Birski, M., Litwinowicz, A. (2011). Results of neuromodulation for the management of chronic pain. Neurol. Neurochir. Pol. 45, 445–451. doi: 10.1016/S0028-3843(14)60312-1
Sun, D., Ma, H., Ma, J., Wang, J., Deng, X., Hu, C., et al. (2018). Canonical Transient Receptor Potential Channel 3 Contributes to Febrile Seizure Inducing Neuronal Cell Death and Neuroinflammation. Cell Mol. Neurobiol. 38, 1215–1226. doi: 10.1007/s10571-018-0586-5
Torres, A. J., Vasudevan, L., Holowka, D., Baird, B. A. (2008). Focal adhesion proteins connect IgE receptors to the cytoskeleton as revealed by micropatterned ligand arrays. Proc. Natl. Acad. Sci. U. S. A. 105, 17238–17244. doi: 10.1073/pnas.0802138105
Unger, M. D., Pleticha, J., Steinauer, J., Kanwar, R., Diehn, F., Lavallee, K. T., et al. (2019). Unilateral Epidural Targeting of Resiniferatoxin Induces Bilateral Neurolysis of Spinal Nociceptive Afferents. Pain Med. 20, 897–906. doi: 10.1093/pm/pny276
Webb, D. J., Zhang, H., Majumdar, D., Horwitz, A. F. (2007). alpha5 integrin signaling regulates the formation of spines and synapses in hippocampal neurons. J. Biol. Chem. 282, 6929–6935. doi: 10.1074/jbc.M610981200
Yamaguchi, T., Watanabe, Y., Tanaka, M., Nakagawa, M., Yamaguchi, N. (2008). cAMP-dependent regulation of spinesin/TMPRSS5 gene expression in astrocytes. J. Neurosci. Res. 86, 610–617. doi: 10.1002/jnr.21517
Keywords: neuromodulation, resiniferatoxin, capsaicin, DEGs (differentially expressed genes), TRPV1, neuropathic pain (NP)
Citation: Singla RK, Sultana A, Alam MS and Shen B (2020) Regulation of Pain Genes—Capsaicin vs Resiniferatoxin: Reassessment of Transcriptomic Data. Front. Pharmacol. 11:551786. doi: 10.3389/fphar.2020.551786
Received: 14 April 2020; Accepted: 11 September 2020;
Published: 29 October 2020.
Edited by:
Heike Wulff, University of California, Davis, United StatesReviewed by:
Francesca Luisa Conforti, University of Calabria, ItalyGiacinto Bagetta, University of Calabria, Italy
Copyright © 2020 Singla, Sultana, Alam and Shen. This is an open-access article distributed under the terms of the Creative Commons Attribution License (CC BY). The use, distribution or reproduction in other forums is permitted, provided the original author(s) and the copyright owner(s) are credited and that the original publication in this journal is cited, in accordance with accepted academic practice. No use, distribution or reproduction is permitted which does not comply with these terms.
*Correspondence: Rajeev K. Singla, rajeevsingla26@gmail.com; Bairong Shen, bairong.shen@scu.edu.cn
†These authors have contributed equally to this work