Effects of repetitive transcranial magnetic stimulation on motor function and language ability in cerebral palsy: A systematic review and meta-analysis
- 1School of Education and Psychology, University of Jinan, Jinan, China
- 2Jinan Tongkang Children's Hospital, Jinan, China
- 3Department of Rehabilitation Medicine, Hunan Provincial People's Hospital, The First Affiliated Hospital of Hunan Normal University, Changsha, China
- 4Tongji Hospital, Tongji Medical College, Huazhong University of Science & Technology, Wuhan, China
- 5Department of Rehabilitation Medicine, The Seventh Affiliated Hospital, Sun Yat-Sen University, Shenzhen, China
- 6CAS Key Laboratory of Mental Health, Institute of Psychology, Beijing, China
Objective: This review was conducted to assess the quality of the evidence of effectiveness of repetitive transcranial magnetic stimulation (rTMS) in treating motor and language ability of cerebral palsy (CP).
Method: Medline, Cochrane library, Web of Science, Embase, PubMed, and CNKI databases were searched up to July 2021 by two independent reviewers. Randomized controlled trials (RCTs) that were published in English and Chinese and met the following criteria were included. The population comprised patients who met the diagnostic criteria for CP. Intervention included the following: comparison about rTMS and sham rTMS or comparison about rTMS combine with other physical therapy and other physical therapy. Outcomes included motor function, as follows: gross motor function measure (GMFM), Gesell Development Diagnosis Scale, fine motor function measure (FMFM), Peabody developmental motor scale, and Modified Ashworth scale. For language ability, sign-significant relation (S-S) was included. Methodological quality was assessed using the Physiotherapy Evidence Database (PEDro) scale.
Results: Finally, 29 studies were included in the meta-analysis. Results of evaluation using the Cochrane Collaborative Network Bias Risk Assessment Scale showed that 19 studies specifically explained randomization, among which two studies described allocation concealment, four studies blinded participants and persons and had low risk of bias, and six studies explained that the assessment of outcome measures was blinded. Significant improvements in motor function were observed. The GMFM of total score was determined by using the random-effect model [I2 = 88%; MD = −1.03; 95% CI (−1.35, −0.71); P < 0.0001] and FMFM was determined by using the fixed-effect model [P = 0.40 and I2 = 3%; SMDs = −0.48, 95% CI (−0.65, −0.30); P < 0.01]. For language ability, the language improvement rate was determined using a fixed-effect model [P = 0.88 and I2 = 0%; MD = 0.37, 95% CI (0.23, 0.57); P < 0.01]. According to the PEDro scale, 10 studies had low-quality, four studies had excellent quality, and the other studies had good quality. Using the GRADEpro GDT online tool, we included a total of 31 outcome indicators, as follows: 22 for low quality, seven for moderate quality, and two for very low quality.
Conclusion: The rTMS could improve the motor function and language ability of patients with CP. However, rTMS prescriptions varied, and the studies had low sample sizes. Studies using rigorous and standard research designs about prescriptions and large samples are needed to collect sufficient evidence about the effectiveness of using rTMS to treat patients with CP.
1. Introduction
Cerebral palsy belongs to a group of persistent central motor and postural developmental disorders and activity limitation syndromes that are caused by non-progressive damage to the developing fetal or infant brain (before, during, or after childbirth) (1–4). CP is usually dominated by movement disorders or accompanied by disturbances in sensation, perception, cognition, communication, and behavior (4, 5). The clinical symptoms of CP caused by various etiology before birth up to the neonatal period mostly occurred before 18 months after birth; symptoms of CP caused by brain injury (hypoxia, trauma, poisoning, central nervous system infection, and others) after neonatal period and infancy period are related to the time of brain injury (4). Clinically, these are generally divided into spasticity, dyskinetic, and ataxia according to the mode of movement disorder (1, 6, 7).
Pathological changes in the brain affected by CP are characterized by abnormal brain development, brain damage caused by brain hypoxia, and intracranial hemorrhage (8, 9). The characterization of CP by delayed gross motor responses and difficulty executing movements due to dystonia, muscle weakness, and insufficient muscle coordination (10). Spasms and abnormal motor postures increase energy expenditure and hinder the normal muscle growth during development, leading to secondary muscle and soft tissue contracture and skeletal deformities (11). Children with CP with these movement disorders have functional impairments in activities of daily living (ADL) and ability of self-care (e.g., dressing and feeding) and mobility (12). In Europe, the prevalence of CP was 1.5–3/1,000 births (13). Achieving independence in self-care and mobility is the goal for children with CP.
The most common cause of CP is white matter damage in the brain. CP is a non-progressive disease that leads to worsening of clinical features with the abnormal development of the central nervous system if left unchecked (14). To obtain an effective and long-lasting therapeutic effect, the therapeutic measures need to have a function that affects the neuroplasticity of the brain in the long term (15). Currently, research in the field of pediatric neurology has focused on the efficacy of non-invasive brain stimulation (NIBS) in the treatment of various pediatric neurological disorders (16). NIBS is a means of inducing electrical currents in brain tissue with the effect of promoting immediate and long-term modulation of motor cortex excitability (17). Therefore, it is a non-drug management candidate strategy for the treatment of pediatric movement disorders (18, 19).
As a technique kind of NIBS, the technical features of rTMS are non-invasive and painless (20, 21), which applies electromagnetic principles to brain regions (22), and adjusts the function of various areas of the cerebral cortex by changing the excitability of neurons. rTMS has achieved remarkable therapeutic effects in the treatment of neurological diseases, such as stroke and autism spectrum disorder (23) and has gradually become an important technique for the treatment of these diseases (24).
Nowadays, rTMS is used in the treatment of children with CP increasingly (25). rTMS can improve motor function (26), relieve spasm (27),restore the speech function of patients with CP (28) and can change brain function by modulating developmental plasticity (29). However, studies on rTMS varied in sample size and thus show different results. High-quality evidence-based medical studies that systematically evaluated the efficacy of rTMS in the treatment of CP remain few.
Thus, summarizing studies based on rTMS-related factors is critical to the accurate estimation of the effects of rTMS on CP. The aims of this meta-analysis were as follows: to systematically evaluate the quality and efficacy of rTMS in alleviating motor dysfunction and restoring speech ability in patients with CP according to randomized clinical trials (RCTs); and to search for strong evidence for the effectiveness of using rTMS for CP.
2. Methods
This systematic review was planned and conducted according to the Preferred Reporting Items for Systematic Reviews and Meta-Analyses Guideline and Cochrane Collaboration (30).
2.1. Search strategy
Two reviewers (Ying-Ying Sun and Lei Wang) performed electronic searches in the following publication databases in July 2021 without restrictions on publication year: Medline, Cochrane Library, Web of Science, Embase, PubMed, and China National Knowledge infrastructure (CNKI). Various combinations of keywords or subject words were used as search terms, including the following: “TMS,” “transcranial magnetic stimulation,” “non-invasive brain stimulation,” “cerebral palsy,” and “CP.” Pre-searches were performed. Then, the final search style was selected as follows: PUBMED: “((Cerebral Palsy[Title]) OR (Cerebral Palsy[MeSH Terms])) AND ((((((repetitive transcranial magnetic stimulation[MeSH Terms]) OR (repetitive transcranial magnetic stimulation[Title])) OR (rTMS[Title])) OR (rTMS[MeSH Terms])) OR (repetitive TMS[MeSH Terms])) OR (repetitive TMS[Title])).” The number of manual searches were increased to complement the results and to reduce the number of articles that may have been missed by electronic database searches.
2.2. Eligibility criteria
The Population, Intervention, Comparison, Outcomes, Study Design (PICOS) framework was used to determine the eligibility criteria of the articles to be included in the review. The population included patients who met the diagnostic criteria for CP. Participants clearly stated in the included literature that the compliance or diagnosis was CP and that they were aged under 18 years old. For intervention, the studies using rTMS as intervention and with a well-defined protocol that involved information on the specific training parameters (type, time, intensity, frequency, and duration) were included. For comparison, the experimental groups received rTMS (low- or high-frequency rTMS) or rTMS combined with other physical therapies. The control group received sham TMS or other types of physical therapy. The outcomes (for meta-analysis) were measured by using gross motor function measure (GMFM), Gesell Development Diagnosis Scale (GDDS), fine motor function measure (FMFM), Peabody developmental motor scale (PDMS), and Modified Ashworth Scale (MAS). For language ability, sign-significant relation (S-S) was included. For the study design, only RCTs were included in the review.
2.3. Exclusion criteria
Studies involving animal research, conference paper, protocol study or computer model research, and duplicate papers were excluded. Two authors (Ying-Ying Sun and Yi-jie Huang) independently reviewed the title and abstract sections of the retrieved articles. First, we eliminated duplicate articles by using “Medical Literature King V6” software. Second, we excluded inappropriate articles after reading the title and abstract following the eligibility criteria in the PICOS framework (16). Finally, we downloaded potentially relevant articles for a more detailed full-text review. If the results of the two independent authors differed, then the third author (Pu Wang) participated in the discussion, and final consensus was reached.
2.4. Data extraction
We extracted the following data: general information including first author, year of publication, sample size, gender, age, treatment course, and intervention measures; outcome indicators including GMFM, GDDS, FMFM, PDMS, and MAS; and language ability, S-S. The collection of data and general information were conducted by two authors (Ying-Ying Sun and Yi-jie Huang).
2.5. Quality assessment
The methodological quality of the intervention studies was assessed using the Physiotherapy Evidence Database (PEDro) scale (25). According to the PEDro scale, the quality of papers were classified: studies with scores of lower than six points were considered low-quality studies (scores <6), good-quality studies (scores = 6 or 7), and excellent -quality studies(scores >7) (31).
GRADEpro GDT online tool was used in evaluating the level of evidence quality of outcome indicators. The indicators of outcome quality included five degrading factors, namely, risk of bias, inconsistency, indirectness, imprecision, and other considerations. The quality of evidence can be divided into four levels, namely, “high,” “moderate,” “low,” and “very low.”
Two reviewers (Ying-Ying Sun and Jin-lin Peng) independently evaluated the quality of the included studies, If the results of the two independent authors differ, then a third author (Pu Wang) participated in the discussion and decided the final consensus.
2.6. Risk of bias assessment in individual studies
To minimize errors and potential biases in the evaluation, the quality of the included studies was evaluated, and their scores were compared in a consensus meeting between two independent authors (Wang Lei and Fu-qiang Qiao). In case of disagreements, a third author (Pu Wang) was included in the discussion to achieve a final consensus. The Cochrane risk of bias assessment tool was used to assess the risk of bias of these articles. Each article was assessed for selection, performance, detection, attrition, and reporting biases. Each domain was rated as having high risk of bias, unclear bias, or low risk of bias. The risk map of bias of these studies' quality was prepared with RevMan 5.3 software.
2.7. Statistical analysis
The Review Manager 5.2 software of Cochrane Collaboration was used in the meta-analysis. The outcome variables were continuous. Thus, the mean difference (MD) was calculated, and the 95% CI of the statistical results was reported. A P value of less than 0.05 indicated statistical significance for an overall effect (Z). Chi-square test was used to calculate the heterogeneity of the included articles. When heterogeneity was P > 0.1 and I2 < 50%, a fixed-effect model was used. When heterogeneity was I2 > 50%, the causes of heterogeneity were analyzed by subgroup or sensitivity analysis. When the results still had heterogeneity, the random-effect mode was used for summary analysis.
3. Results
3.1. Search results
At different stages of retrieval and screening, different numbers of studies were excluded. The detailed reasons and procedures are shown in Figure 1. A total of 625 abstracts were retrieved, and all were imported into the Document Management Software of “Medical Literature King V6.” A total of 230 duplicate studies were eliminated, and 325 studies were excluded after reading the titles and abstracts. Seventy studies were left after the screening process, which involved reading the abstracts. The full texts were downloaded for further screening. Twenty-five studies were excluded, because they were conference articles. Eleven studies were excluded, because they included non-randomized controlled trials. One study was excluded, because it did not contain original text. After excluding the abovementioned studies, 33 studies were included in the qualitative analysis. After the article outcome indicators were read, three studies (26, 32, 33) were excluded, because their outcome indicators did not meet the inclusion criteria. The data of one study (34) only reported the P-value, and the original data were not obtained even after contacting the studies' authors; thus, the requirement for data analysis of the meta-analysis was not met. Finally, 29 studies were included in the meta-analysis.
3.2. Assessment of quality
The studies evaluated according to the PEDro scale are listed in Table 1. Ten studies had low quality (27, 35, 41, 43, 44, 48, 50, 51, 55, 56), four studies had excellent quality (26, 32–34), and the other studies had good quality.
3.3. Risk of bias assessment in individual studies
The results of risk of bias are shown in Figures 2, 3. Nineteen studies specifically explained the random methods used, 10 studies (27, 35, 41, 43–45, 50, 51, 55, 56) did not report random sequence generation, two studies (34, 54) described allocation concealment. Four studies (26, 32–34) blinded the participants and persons and had low risk of bias, because the intervention method was rTMS vs. sham rTMS. Six studies (26, 32, 36, 38, 45, 59) explained that the assessment of outcome measures was blinded. Reporting and attrition biases were low risk of bias.
3.4. Study characteristics
As shown in Table 2, the characteristics included in the studies were first author, sample size, age, gender, and diagnosis criteria. As shown in Table 3, the characteristics included in the studies were content of intervention program, site of stimulation, duration of rTMS, number of rTMS sessions, outcomes measured, and assessment time points.
3.5. Outcomes
3.5.1. GMFM
The GMFM consists of 88 items grouped into five domains, namely, (A) lying and rolling, (B) sitting, (C) crawling/kneeling, (D) standing, and (E) walking/running/jumping. The analysis was performed according to the five domains and total score.
3.5.1.1. A
A total of 408 participants were included in six studies (35, 37, 50, 51, 53, 56) [I2 = 67%; MD = 1.86, 95% CI (0.38, 3.35); P = 0.01]. We performed a subgroup analysis, because heterogeneity was observed. According to the duration of TMS, the group was divided into two subgroups, namely, 30 min (51, 53, 56) and 20 min (35, 37, 50), and the result favors rTMS, as shown in Figure 4.
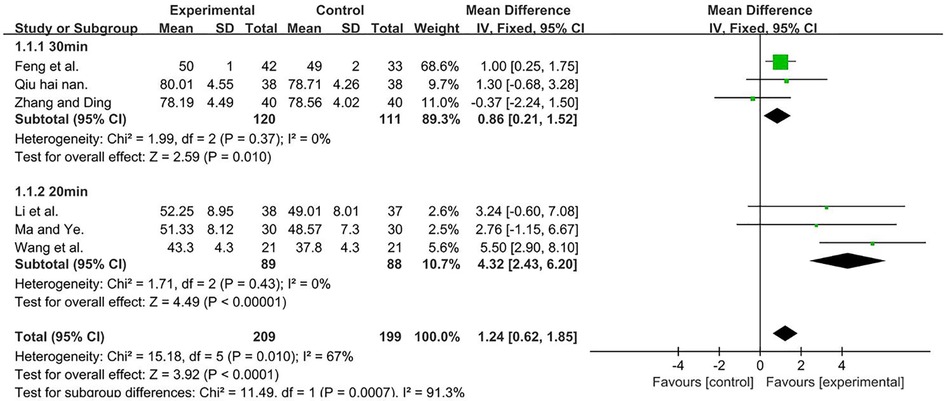
Figure 4. Forest plot showing MD (with 95% CI) for GMFM-A of the included studies comparing the experimental and control groups. Note: For the 30 min subgroup, P = 0.37 and I2 = 0%, fixed-effect model [MD = 0.86, 95% CI (0.21, 1.52); P = 0.01]. For the 20 min subgroup, P = 0.43 and I2 = 0%, fixed-effect model [MD = 4.32; 95% CI (2.43, 6.20); P < 0.001]. The analysis results of both subgroups were statistically significant.
3.5.1.2. B
A total of 408 participants were included in six studies (35, 37, 50, 51, 53, 56) [I2 = 67%; MD = 4.44; 95% CI (3.36, 5.51); P < 0.001]. Heterogeneity was found, and thus, we performed a subgroup analysis. According to the manufacturer of TMS, the group was divided into three subgroups: Beijing Huaxing Kangtai (35, 51), Shenzhen Kangli (53, 56), and others (37, 50), the result favors rTMS, as shown in Figure 5.
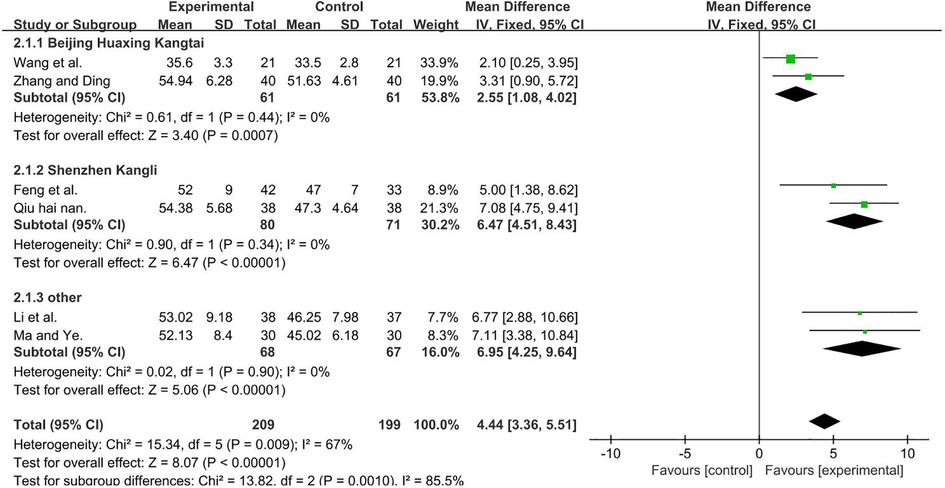
Figure 5. Forest plot showing MD (with 95% CI) for GMFM-B of the included studies comparing the experimental and control groups. Note: For the Beijing Huaxing Kangtai subgroup, P = 0.44 and I2 = 0%., fixed-effect model [MD = 2.55, 95% CI (1.08, 4.02); P < 0.001]. For the Shenzhen Kangli subgroup, P = 0.34 and I2 = 0%, fixed-effect model [MD = 6.47; 95% CI (4.51, 8.43); P < 0.001]. For the other subgroup, P = 0.9, and I2 = 0%, fixed-effect model [MD = 6.95; 95% CI (4.25, 9.64); P < 0.001]. The analysis results of the three subgroups were statistically significant.
3.5.1.3. C
A total of 408 participants were included in six studies (35, 37, 50, 51, 53, 56) [I2 = 75%; MD = 4.88, 95% CI (3.89, 5.87); P < 0.001]. Heterogeneity was found. Further sensitivity analysis revealed that one study (51) (Zhang Yu Qiong and Ding Jian Ying) used six frequencies, which are recycled (5, 10, 20, 30, 40, and 50 Hz) and differed from other studies that only had one frequency. This was analyzed as a possible cause of heterogeneity, and the analysis was performed after its removal, and the result favors rTMS, as shown in Figure 6.
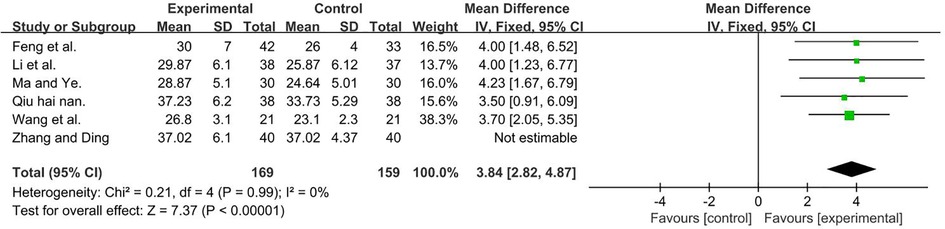
Figure 6. Forest plot showing MD (with 95% CI) for GMFM-C of the included studies comparing the experimental and control groups. Note: (P = 1 and I2 = 0%), fixed-effect model [MD = 3.84, 95% CI (2.82, 4.87); P < 0.001]. The analysis results of three subgroups were statistically significant.
3.5.1.4. D
A total of 448 participants were included in seven studies (35, 37, 41, 50, 51, 53, 56); I2 = 75% [MD = 2.97, 95% CI (2.28, 3.65); P < 0.001]. Heterogeneity existed. We performed a subgroup analysis. According to the frequency of TMS, the group was divided into two subgroups: 1 and 5 HZ (35, 37, 41, 50, 56) and other (51, 53), and the result favors rTMS, as shown in Figure 7.
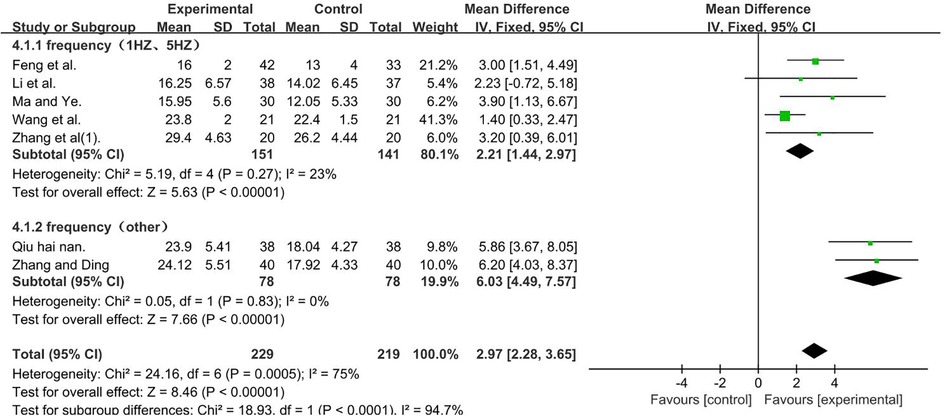
Figure 7. Forest plot showing MD (with 95% CI) for GMFM-D of the included studies comparing the experimental and control groups. Note: For the subgroup with frequencies of 1 and 5 HZ, P = 0.27 and I2 = 23%. The fixed-effect model [MD = 2.21, 95% CI (1.44,2.97); P < 0.001]. For the subgroup with the frequency of other, P = 0.83 and I2 = 0%. fixed-effect model [MD = 6.03, 95% CI (4.49, 7.57); P < 0.001]. The analysis results of the two subgroups were statistically significant.
3.5.1.5. E
A total of 448 participants were included in seven studies (35, 37, 41, 50, 51, 53, 56); I2 = 92% [MD = 1.80, 95% CI (1.29, 2.31); P < 0.001]. Heterogeneity existed. We performed a subgroup analysis. According to the frequency of TMS, the group was divided into two subgroups, namely, 1 and 5 HZ (35, 37, 41, 50, 56) and other (51, 53), and the result favors rTMS, as shown in Figure 8.
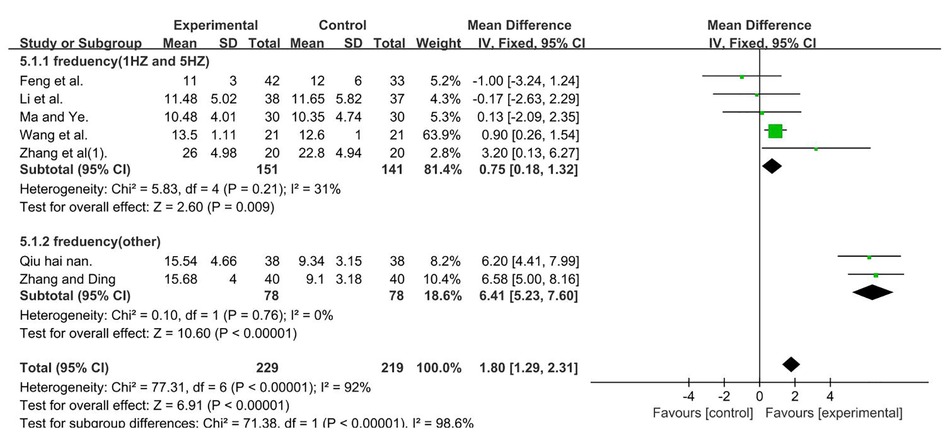
Figure 8. Forest plot showing MD (with 95% CI) for GMFM-E of the included studies comparing the experimental and control groups. Note: For the subgroup with frequencies of 1 and 5 Hz, P = 0.21 and I2 = 31%. fixed-effect model [MD = 0.75, 95% CI (0.18, 1.32); P < 0.05]. For the subgroup with frequency (other), P = 0.76 and I2 = 0%, fixed-effect model [MD = 6.41; 95% CI (5.23, 7.60); P < 0.001]. The analysis results of the two subgroups were statistically significant.
3.5.1.6. ALL
A total of 1,653 participants were included in 11 studies (27, 37, 38, 40, 43–45, 47–49, 51, 53–56) [I2 = 88%; MD = 1.09, 95% CI (0.99, 1.20); P < 0.001]. Heterogeneity was found. Through subgroup and sensitivity analyses, no significant change in heterogeneity was found. We selected the random-effect model, and the result favors rTMS, as shown in Figure 9.
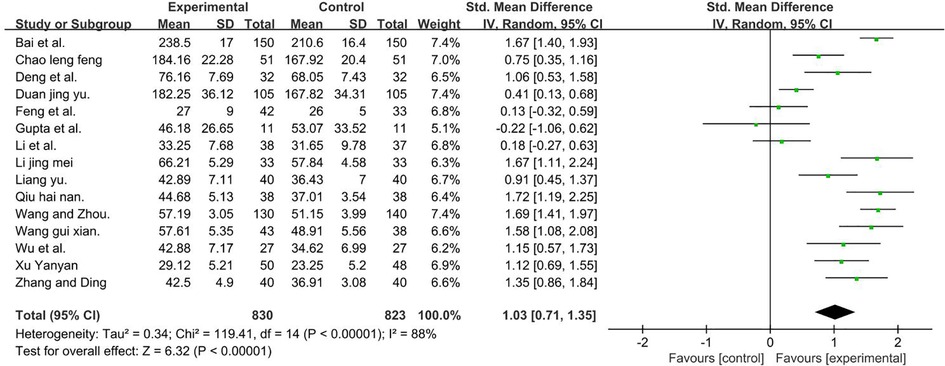
Figure 9. Forest plot showing MD (with 95% CI) for GMFM-ALL of the included studies comparing the experimental and control groups. Note: [I2 = 88%; MD = 1.03; 95% CI (0.71, 1.35); P < 0.001]. The analysis results were statistically significant.
3.5.2. GDDS
The GDDS had five domains, namely, adaptability, gross motor, fine motor, language, and personal–social responses. Analysis was conducted according to the five domains and the total score.
GDDS—gross motor, a total of 235 participants were included in five studies (35, 46, 57, 60). Data were compared using different scales; thus, we calculated pooled statistics with SMDs [I2 = 88%; SMDs = 1.11; 95% CI (0.29, 1.94); P < 0.001]. Heterogeneity was found. Further sensitivity analysis revealed that one study (35) (Wang Li Fan et al.) used the DA of GDDS and differed from other studies, which used the DQ of GDDS, and was considered a possible cause of heterogeneity. The analysis was performed after its removal, as well as selected means, and standard deviations (MD) (P = 0.94 and I2 = 0%). GDDS—fine motor, a total of 178 participants were included in three studies (35, 46, 57) (P = 0.56 and I2 = 0%). GDDS–adaptability, a total of 334 participants were included in four studies (35, 46, 57, 59). Given that data were compared using different scales, we calculated pooled statistics according to SMDs [I2 = 93%; SMDs = 1.12; 95% CI (0.88, 1.36); P < 0.001]. Heterogeneity was found. Further sensitivity analysis revealed that one study (59) (Wang Ying Hong and Ma Bing Xiang) had an extremely large sample size, which was considerably higher than the those of the other studies. This factor was analyzed as a possible cause of heterogeneity, and analysis was performed after its removal. SMDs were selected (P = 0.70 and I2 = 0%). GDDS–language, a total of 178 participants were included in three studies (35, 46, 57). Given that data were compared using different scales, we calculated pooled statistics by using SMDs [I2 = 85%, SMDs = 0.50; 95% CI (0.19, 0.80); P < 0.001]. Heterogeneity was found. Further sensitivity analysis revealed that one study (35) (Wang Li Fan et al.) used the cerebral hemisphere motor cortex site of stimulation in contrast to the other studies, which used the bilateral cerebral motor cortex. This factor was analyzed as a possible cause of heterogeneity, and analysis was performed after its removal. SMDs were selected (P = 0.59 and I2 = 0%). GDDS–personal–social responses, a total of 178 participants were included in three studies (35, 46, 57). Given that data were compared using different scales, we calculated pooled statistics by using SMDs (P = 0.51 and I2 = 0%). The analysis results were statistically significant and those results favors rTMS, as shown in Figure 10.
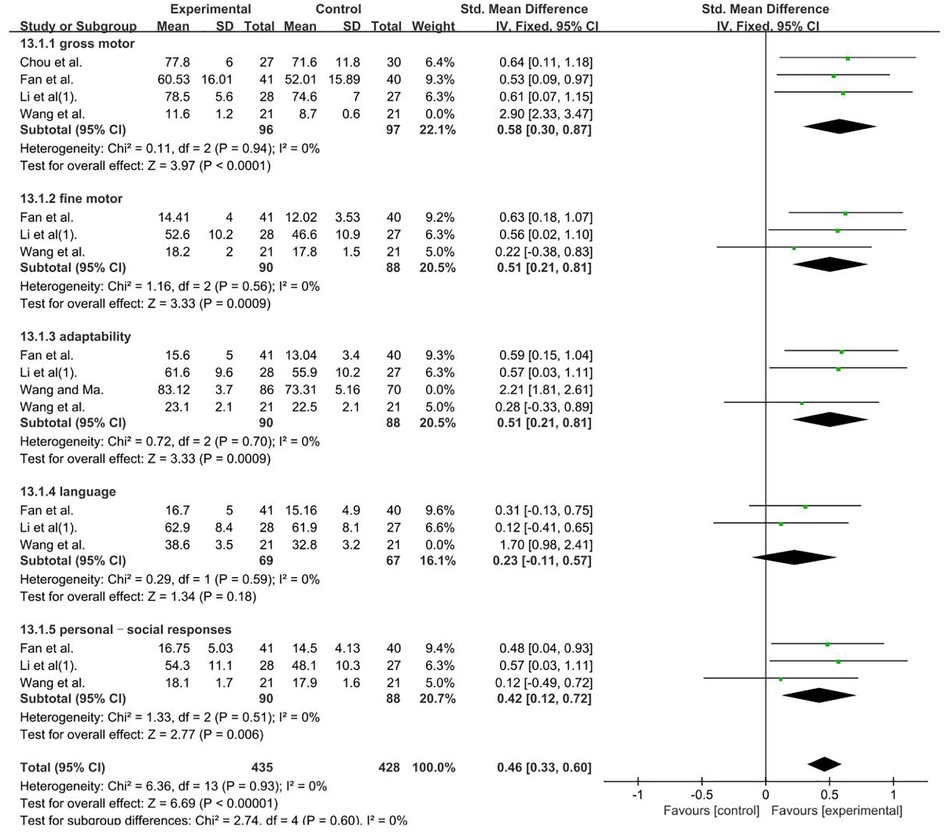
Figure 10. Forest plot showing SMD (with 95% CI) for GDDS of the included studies comparing the experimental and control groups. Note: Gross motor: fixed-effect model [MD = 0.58 95% CI (0.30, 0.87); P < 0.001]; GDDS—fine motor: fixed-effect model [MD = 0.51; 95% CI (0.21, 0.81); P < 0.05]. GDDS–adaptability: fixed-effect model [SMDs = 0.51; 95% CI (0.21, 0.81); P < 0.001]. GDDS–language: fixed-effect model [SMDs = 0.23, 95% CI (0.11, 0.57); P = 0.18]. GDDS–personal–social responses: fixed-effect model [SMDs = 0.42; 95% CI (0.12, 0.72); P < 0.05]. The analysis results were statistically significant.
3.5.3. FMFM
A total of 532 participants were included in six studies (37, 39, 42, 54–56). Given that data were compared using different scales, we calculated pooled statistics by using SMDs (P = 0.40 and I2 = 3%). We used a fixed-effect model and the result favors rTMS, as shown in Figure 11.
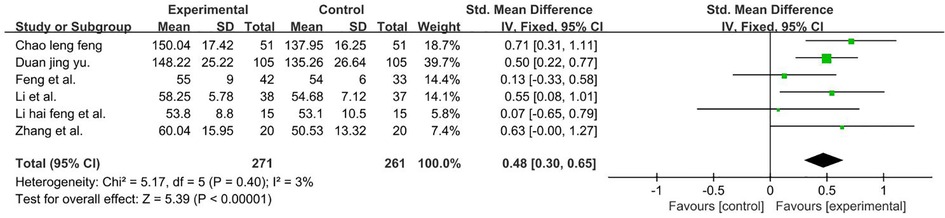
Figure 11. Forest plot showing MD (with 95% CI) for FMFM of the included studies comparing the experimental and control groups. Note: fixed-effect model [SMDs = 0.48; 95% CI (0.30, 0.65); P < 0.001]. The analysis results were statistically significant.
3.5.4. PDMS
The PDMS included four domains of the study, namely, grasping, visual–motor integration, Gross Motor Quotient (GMQ), and Fine Motor Quotient (FMQ) score (63). The analysis was performed according to the four domains.
PDMS–visual–motor integration, a total of 119 participants were included in two studies (46, 47) (P = 0.80 and I2 = 0%). We used a fixed-effect model. PDMS–grasping, a total of 119 participants were included in two studies (46, 47) (P = 0.49 and I2 = 0%). We used a fixed-effect model. PDMS–FMQ, a total of 224 participants were included in three studies (36, 44, 48) [I2 = 76%; MD = 10.00; 95% CI (7.82, 12.17); P < 0.01]. Subgroup and sensitivity analyses found no significant change in heterogeneity. We selected the random-effect model. PDMS–GMQ, a total of 300 participants were included in two studies (38, 42) (P = 0.24 and I2 = 26%). We used a fixed-effect model. Those result favors rTMS, as shown in Figure 12.
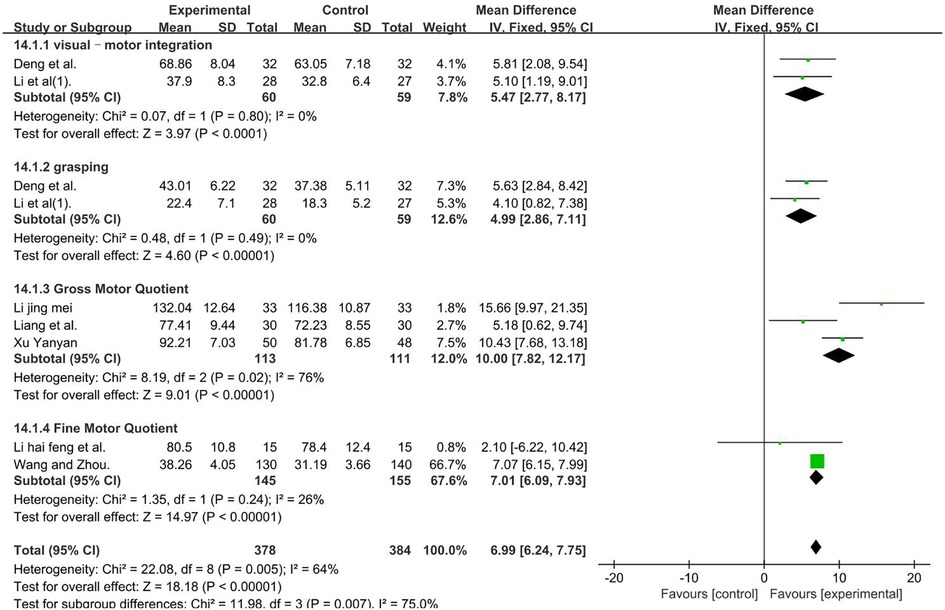
Figure 12. Forest plot showing MD (with 95% CI) for PDMS of the included studies comparing the experimental and control groups. Note: PDMS–visual–motor integration: fixed-effect model [MD = 5.47; 95% CI (2.77, 8.17); P < 0.01]. PDMS–grasping: fixed-effect model [MD = 4.99; 95% CI (2.86, 7.11); P < 0.01]. PDMS–FMQ: random-effect model [I2 = 76%; MD = 10.20; 95% CI (5.24, 15.15); P < 0.01]. PDMS–GMQ: fixed-effect model [MD = 7.01; 95% CI (6.09, 7.93); P < 0.01]. Those analysis results were statistically significant.
3.5.5. MAS
A total of 483 participants were included in four studies (39, 44, 49, 52) [I2 = 80%; MD = 0.40; 95% CI (0.31, 0.50); P < 0.001]. Heterogeneity existed. We performed a subgroup analysis. According to the site of muscle spasm test, the group was divided into two subgroups, namely, the upper and lower limbs. For the upper limb subgroup (39, 52), P = 0.71 and I2 = 0%. We selected the fixed-effect model. For the lower limbs subgroup (44, 49), I2 = 93%. The high heterogeneity found in the analysis may be due to the high sample size of the study (49), we used a random-effect model. The analysis results of two subgroups were statistically significant and those results favors rTMS, as shown in Figure 13.
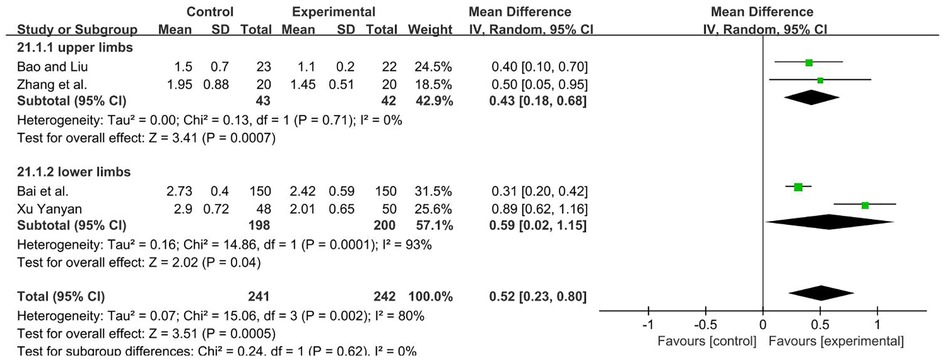
Figure 13. Forest plot showing MD (with 95% CI) for MAS of the included studies comparing the experimental and control groups. Note: For the upper limb subgroup, fixed-effect model [MD = 0.43; 95% CI (0.18, 0.68); P < 0.001]. For the lower limbs subgroup, random-effect model [MD = 0.59; 95% CI (0.02, 1.15); P < 0.05]. The analysis results of two subgroups were statistically significant.
3.5.6. S-S
This research analyzed the language situation according to three aspects, namely, language improvement rate, expression quotient, and comprehension quotient.
3.5.6.1. Language improvement rate
A total of 508 participants were included in five studies (40, 43, 54, 55, 62) (P = 0.88 and I2 = 0%). We used a fixed-effect model and the result favors rTMS, as shown in Figure 14.
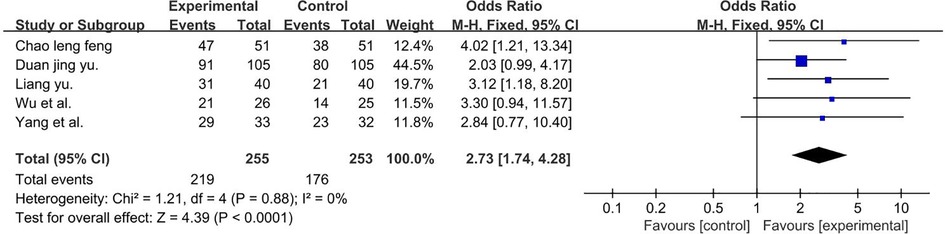
Figure 14. Forest plot showing MD (with 95% CI) for language improvement rate of the included studies comparing the experimental and control groups. Note: fixed-effect model [MD = 2.73; 95% CI (1.74, 4.28); P < 0.01]. The analysis results were statistically significant.
3.5.6.2. Comprehension quotient and expression quotient
A total of 288 participants were included in four studies (40, 58, 61, 62). Subgroup and sensitivity analyses found no significant change in heterogeneity. We selected the random-effect model, and the result favors rTMS, as shown in Figure 15.
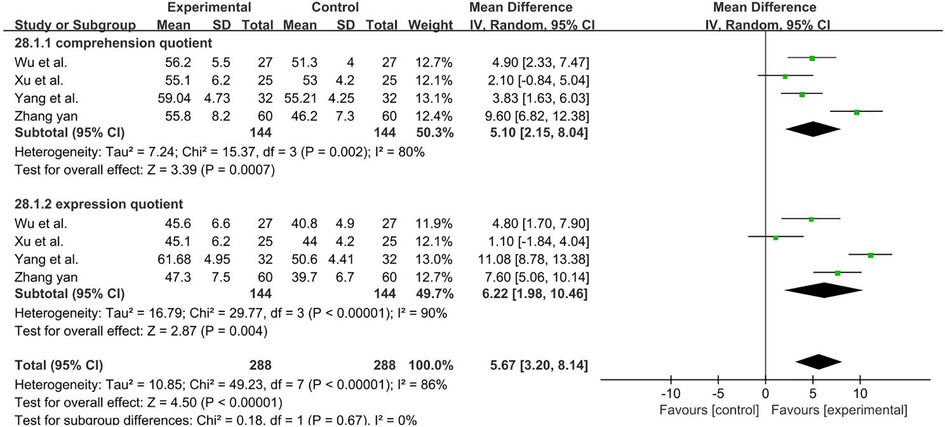
Figure 15. Forest plot showing MD (with 95% CI) for comprehension quotient and expression quotient of the included studies comparing the experimental and control groups. Note: Random-effect model, the result of Comprehension quotient [I2 = 80%; MD = 5.10; 95% CI (2.15, 8.04); P < 0.01], the result of Expression quotient [I2 = 90%; MD = 6.22; 95% CI (1.98, 10.46); P < 0.01]. The analysis results were statistically significant.
3.5.7. Funnel chart
Among all the outcome indicators, only FMFM-ALL included more than 10 studies (15 included studies). Thus, the funnel chart analysis was performed on this outcome indicator. Seven studies were outside the 95% interval, and the two sides of the funnel chart were asymmetrical. These results all showed heterogeneity, as shown in Supplementary Figure S1.
3.5.8. Grade
The GRADEpro GDT online tool was used to evaluate the quality of evidence for the included study outcome indicators. A total of 31 outcome indicators were included, namely, 22 low quality indicators, seven moderate quality indicators, and two very low quality indicators, as shown in Table 4.
4. Discussion
This meta-analysis of the study included 29 studies. According to the PEDro scale, only four studies had excellent quality (26, 32–34), whereas 10 studies had low quality (27, 35, 41, 43, 44, 48, 50, 51, 55, 56). According to the results of evaluation using the Cochrane Collaborative Network Bias Risk Assessment Scale, 10 studies (27, 35, 41, 43–45, 50, 51, 55, 56) did not report random sequence generation, two studies (34, 54) described allocation concealment, four studies (26, 32–34) described the procedures for blinding participants and persons, and six studies (26, 32, 36, 38, 45, 59) explained that the assessment of outcome measures was blinded. The abovementioned issues affected the quality of the results and the risk of bias. The results of GRADE's quality of evidence showed that the main outcome indicators had low quality, and two outcome indicators had very low quality. Seven outcome indicators had moderate quality, and no high quality outcome indicator was found. Overall, the quality of the outcome indicators was low, and the reasons were as follows. 1. Allocation concealment and blinding in the experimental design in the included literature was not strictly controllable. 2. The heterogeneity was biased, which may be related to the prescription of intervention factors, such as differences in stimulation frequency and time. 3. The size of the included literature and the sample size were small.
In the study characteristics, most studies focused on the comparison between conventional rehabilitation and conventional rehabilitation combined with rTMS. Only four studies (26, 32–34) described the comparison between sham and real TMS, and the above two research methods showed the effectiveness of rTMS in improving motor function and language ability in patients with CP. The included studies examined the site of stimulation, the duration of rTMS, and the number of rTMS sessions; stimulation frequencies of TMS were 0.2 (53), 1 (33), 5 (27), 10 (50), and 30 Hz (60). Studies on the efficacy of rTMS at different frequencies are few, and no clear evidence that stimulation of frequency contributes to CP treatment is available. High-frequency rTMS (stimulation rate >1 Hz), produces an excitatory after effect (64, 65). Conversely, low-frequency rTMS (stimulation rate ≤ 1 Hz) depresses excitability (26, 66, 67). This is applicable to stroke patients, and whether it is applicable to patients with CP remains to be discovered. Valle et al. (32) reported the stimulation frequency of rTMS (sham vs. 1 Hz vs. 5 Hz) and showed significant reduction in spasticity after 5 Hz. Gupta et al. (1) reported repetitive transcranial magnetic stimulation pulses (1,500 vs. 2,000 vs. 2500) and showed that the overall improvement rates in motor functions were 2.33% in 1,500 pulses, 3.58% in 2000 pulses, and 5.17% in 2,500 pulses. The 2,500 pulse groups showed significant improvement in motor function. Therefore, the efficacy of rTMS in the treatment of CP is affected by factors, such as stimulation frequency, intensity, duration, and pulse sequence.
Motor dysfunction is among the common symptoms of CP. Therefore, many studies on motor dysfunction in patients with CP have been conducted. In our review, GMFM, FMFM, PDMS, and GDDS were used in evaluating results. GMFM is a criterion-referenced observational measure for assessing gross motor function in children with CP. It is a reliable method for assessing the gross motor functional ability and quality of movement in children with CP (68). Our review of the five domains of GMFM, namely, (A) lying and rolling, (B) sitting, (C) crawling/kneeling, (D) standing, and (E) walking/running/jumping, showed that rTMS can improve the aspects of gross motor function. In addition, the result of GDDS-gross motor showed that rTMS can improve these aspects in children with CP. For the evaluation of the fine motor of patients with CP, we used FMFM and PDMS. TMS can improve the aspects of fine motor function. The result of GDDS-fine motor showed that rTMS can improve the aspects of fine motor function in children with CP. Marzbani et al. (14) demonstrated the 1 Hz rTMS could improve motor function in children with CP. Dadashi et al. (67) showed that after 3 weeks of rTMS training, the balance control of children with CP can improve, indicating that rTMS may improve balance control by promoting the function of corticospinal tract and ascending pathways. However, studies with larger sample sizes are needed to confirm this finding.
Spasticity is the main cause of motor function disability in children with CP (69). It is an important factor affecting the quality of life of patients with CP, because long-term spasticity can lead to musculoskeletal complications, such as contracture, pain, and subluxation. In addition, the elimination of spasms can improve the motor function of these patients (70). Our review included four studies (39, 44, 49, 52) [I2 = 80%; MD = 0.40; 95% CI (0.31, 0.50); P < 0.0001], and the analysis results for these studies were statistically significant. Guptal et al. (71) showed that conventional treatment had no obvious effect on the improvement of muscle spasm and that rTMS combined with conventional treatment significantly reduced muscle tightness. In 2019, Guptal et al. (27) compared rTMS and conventional physical therapy. The MAS score of the rTMS treatment group showed that the spasm of the muscles in the lower extremity was significantly reduced, and the motor function greatly improved. Valle et al. (32) showed that high-frequency stimulation was more effective in improving spasticity, although their evidence was insufficient.
The language development disorder in children with CP may be due to many reasons, such as speech motor control, cognition, language, and sensory/perception (72). European epidemiological data showed that 60% of children with CP have communication disorders (73). In addition, language development disorders can have many adverse effects on children with CP and are not conducive to social communication (74) and quality of life (74). Our review analyzed the language situation from three aspects, namely, language improvement rate, expression quotient, and comprehension quotient. The rTMS can improve the abovementioned various aspects of language ability. Expression and comprehension quotients significantly improved compared with those in the control group. In addition, GDDS results showed that language and personal–social responses were more obviously improved by rTMS. However, studies on the treatment of language disorders with rTMS are few, and the sample size is relatively low. Further expansion and improvement of research are needed.
4.1. Study limitations
Our findings are based on articles written in English and Chinese. Articles in other languages were not included, and their exclusion may have implications for our research. In the inclusion of outcome indicators, the data were all derived from the scale. Only S-S was included in the indicators of language ability, which had a certain impact on this study.
5. Conclusions
This review suggested that rTMS could improve the motor function and language ability of patients with CP. However, the review indicated large differences among studies in terms of rTMS prescription, particularly in stimulation frequency, intensity, duration, and pulse train. Therefore, the standardization of prescriptions needs to be explored and improved. Studies using large sample size and rigorous research designs are needed to obtain sufficient evidence on the effectiveness of using rTMS to treat patients with CP.
Data availability statement
The original contributions presented in the study are included in the article/Supplementary Material, further inquiries can be directed to the corresponding author/s.
Author contributions
Y-YS and LW: Data collection, collation and article writing. J-lP: Summary and arrangement of data. F-qQ: Summary and arrangement of data. Y-jH: Summary and arrangement of data. PW: Guidance, supervision and control of the entire research process. All authors contributed to the article and approved the submitted version.
Funding
This study was supported by Social Science Planning Research Fund, Shandong Province (21CSZJ12).
Conflict of interest
The authors declare that the research was conducted in the absence of any commercial or financial relationships that could be construed as a potential conflict of interest.
Publisher's note
All claims expressed in this article are solely those of the authors and do not necessarily represent those of their affiliated organizations, or those of the publisher, the editors and the reviewers. Any product that may be evaluated in this article, or claim that may be made by its manufacturer, is not guaranteed or endorsed by the publisher.
Supplementary material
The Supplementary Material for this article can be found online at: https://www.frontiersin.org/articles/10.3389/fped.2023.835472/full#supplementary-material.
References
1. Gupta X. Neuromodulatory effect of repetitive transcranial magnetic stimulation pulses on functional motor performances of spastic cerebral palsy children. J Med Eng Technol. (2018) 42(5):352–8. doi: 10.1080/03091902.2018.1510555
2. Tang J, Qin J, Zou L, Li X, Ma B. Chinese Rehabilitation guidelines for cerebral palsy (2015): part one. Chin J Rehab Med. (2015) 30(07):747–54. doi: 10.3969/j.issn.1001-1242.2015.07.028
3. Soleimani F, Vameghi R, Biglarian A. Antenatal and intrapartum risk factors for cerebral palsy in term and near-term newborns. Arch Iran Med. (2013) 16(4):213–6. PMID: 23496363
4. Colver A, Fairhurst C, Pharoah PO. Cerebral palsy. Lancet. (2014) 383(9924):1240–9. doi: 10.1016/S0140-6736(13)61835-8
5. Reddihough D. Cerebral palsy in childhood. Aust Fam Physician. (2011) 40(4):192–6. http://www.americanchildneurologyuae.com/ar/files/neurological-diseases/cp/cp2.pdf21597527
6. Chen X, Li S. Definition, classification and diagnostic conditions of cerebral palsy in children. Chin J Phys Med Rehab. (2007) 29(05):309. doi: 10.3760/j:issn:0254-1424.2007.05.026
7. Hayes C. Cerebral palsy: classification, diagnosis and challenges of care. Br J Nurs. (2010) 19(6):368–73. doi: 10.12968/bjon.2010.19.6.47249
8. Mann JR, McDermott S, Griffith MI, Hardin J, Gregg A. Uncovering the complex relationship between pre-eclampsia, preterm birth and cerebral palsy. Paediatr Perinat Epidemiol. (2011) 25(2):100–10. doi: 10.1111/j.1365-3016.2010.01157.x
9. Wu YW, Kuzniewicz MW, Wickremasinghe AC, Walsh EM, Wi S, McCulloch CE, et al. Risk for cerebral palsy in infants with total serum bilirubin levels at or above the exchange transfusion threshold: a population-based study. JAMA Pediatr. (2015) 169(3):239–46. doi: 10.1001/jamapediatrics.2014.3036
10. Green LB, Hurvitz EA. Cerebral palsy. Phys Med Rehabil Clin N Am. (2007) 18(4):859–82, vii. doi: 10.1016/j.pmr.2007.07.005
11. Bar-On L, Molenaers G, Aertbeliën E, Van Campenhout A, Feys H, Nuttin B, et al. Spasticity and its contribution to hypertonia in cerebral palsy. Biomed Res Int. (2015) 2015:317047. doi: 10.1155/2015/317047
12. Diego A, Leung AW. Transcranial direct current stimulation for improving gross motor function in children with cerebral palsy: a systematic review. Br J Occup Ther. (2020) 83(7):030802261989788. doi: 10.1177/0308022619897885
13. Surveillance of Cerebral Palsy in Europe. Surveillance of cerebral palsy in Europe: a collaboration of cerebral palsy surveys and registers. Surveillance of Cerebral Palsy in Europe (SCPE). Dev Med Child Neurol. (2000) 42(12):816–24. doi: 10.1017/s0012162200001511
14. Marzbani H, Shahrokhi A, Irani A, Mehdinezhad M, Kohanpour M, Mirbagheri MM. The effects of low frequency repetitive transcranial magnetic stimulation on white matter structural connectivity in children with cerebral palsy. Annu Int Conf IEEE Eng Med Biol Soc. (2018) 2018:2491–4. doi: 10.1109/EMBC.2018.8512866
15. Azizi S, Marzbani H, Raminfard S, Birgani PM, Rasooli AH, Mirbagheri MM. The impact of an anti-gravity treadmill (AlterG) training on walking capacity and corticospinal tract structure in children with cerebral palsy. Annu Int Conf IEEE Eng Med Biol Soc. (2017) 2017:1150–3. doi: 10.1109/EMBC.2017.8037033
16. Hameed MQ, Dhamne SC, Gersner R, Kaye HL, Oberman LM, Pascual-Leone A, et al. Transcranial magnetic and direct current stimulation in children. Curr Neurol Neurosci Rep. (2017) 17(2):11. doi: 10.1007/s11910-017-0719-0
17. Pascual-Leone A, Walsh V, Rothwell J. Transcranial magnetic stimulation in cognitive neuroscience–virtual lesion, chronometry, and functional connectivity. Curr Opin Neurobiol. (2000) 10(2):232–7. doi: 10.1016/s0959-4388(00)00081-7
18. Kirton A. Advancing non-invasive neuromodulation clinical trials in children: lessons from perinatal stroke. Eur J Paediatr Neurol. (2017) 21(1):75–103. doi: 10.1016/j.ejpn.2016.07.002
19. Chung MG, Lo WD. Noninvasive brain stimulation: the potential for use in the rehabilitation of pediatric acquired brain injury. Arch Phys Med Rehabil. (2015) 96(4 Suppl):S129–37. doi: 10.1016/j.apmr.2014.10.013
20. Chung MG, Lo WD. Noninvasive brain stimulation: the potential for use in the rehabilitation of pediatric acquired brain injury. Arch Phys Med Rehabil. (2015) 96(4):S129–37. doi: 10.1016/j.apmr.2014.10.013
21. Tekgul H. A transcranial magnetic stimulation study for the investigation of corticospinal motor pathways in children with cerebral palsy. J Clin Neurosci. (2020) 78:153–8. doi: 10.1016/j.jocn.2020.04.087
22. Pascual-Leone A, Amedi A, Fregni F, Merabet LB. The plastic human brain cortex. Annu Rev Neurosci. (2005) 28:377–401. doi: 10.1146/annurev.neuro.27.070203.144216
23. Casanova MF, Shaban M, Ghazal M, El-Baz AS, Casanova EL, Opris L, et al. Effects of transcranial magnetic stimulation therapy on evoked and induced gamma oscillations in children with autism Spectrum disorder. Brain Sci. (2020) 10(423):1–19. doi: 10.3390/brainsci10070423
24. Wei Q, Chen X, Wang K. Research progress of transcranial magnetic stimulation in the treatment of neurological diseases. J Int Neurol Neurosurg. (2011) 38(05):492–6. 10.2011-05-024
25. Elbanna ST, Elshennawy S, Ayad MN. Noninvasive brain stimulation for rehabilitation of pediatric motor disorders following brain injury: systematic review of randomized controlled trials. Arch Phys Med Rehabil. (2019) 100(10):1945–63. doi: 10.1016/j.apmr.2019.04.009
26. Gillick BT, Krach LE, Feyma T, Rich TL, Moberg K, Thomas W, et al. Primed low-frequency repetitive transcranial magnetic stimulation and constraint-induced movement therapy in pediatric hemiparesis: a randomized controlled trial. Dev Med Child Neurol. (2014) 56(1):44–52. doi: 10.1111/dmcn.12243
27. Gupta M, Rajak BL, Bhatia D, Mukherjee A. Effect of repetitive transcranial magnetic stimulation on motor function and spasticity in spastic cerebral palsy. Int J Biomed Eng Technol. (2019) 31(4):365–74. doi: 10.1504/IJBET.2019.103251
28. Naeser MA, Martin PI, Nicholas M, Baker EH, Seekins H, Helm-Estabrooks N, et al. Improved naming after TMS treatments in a chronic, global aphasia patient–case report. Neurocase. (2005) 11(3):182–93. doi: 10.1080/13554790590944663
29. Kirton A. Modeling developmental plasticity after perinatal stroke: defining central therapeutic targets in cerebral palsy. Pediatr Neurol. (2013) 48(2):81–94. doi: 10.1016/j.pediatrneurol.2012.08.001
30. Cumpston M, Li T, Page MJ, Chandler J, Welch VA, Higgins JP, et al. Updated guidance for trusted systematic reviews: a new edition of the cochrane handbook for systematic reviews of interventions. Cochrane Database Syst Rev. (2019) 10:ED000142. doi: 10.1002/14651858.ED000142
31. Maher CG, Sherrington C, Herbert RD, Moseley AM, Elkins M. Reliability of the PEDro scale for rating quality of randomized controlled trials. Phys Ther. (2003) 83(8):713–21. doi: 10.1093/ptj/83.8.713
32. Valle AC, Dionisio K, Pitskel NB, Pascual-Leone A, Orsati F, Ferreira M, et al. Low and high frequency repetitive transcranial magnetic stimulation for the treatment of spasticity. Dev Med Child Neurol. (2007) 49(7):534–8. doi: 10.1111/j.1469-8749.2007.00534.x
33. Kirton A, Chen R, Friefeld S, Gunraj C, Pontigon AM, Deveber G. Contralesional repetitive transcranial magnetic stimulation for chronic hemiparesis in subcortical paediatric stroke: a randomised trial. Lancet Neurol. (2008) 7(6):507–13. doi: 10.1016/S1474-4422(08)70096-6
34. Kirton A, Andersen J, Herrero M, Nettel-Aguirre A, Carsolio L, Damji O, et al. Brain stimulation and constraint for perinatal stroke hemiparesis the plastic champs trial. Neurology. (2016) 86(18):1659–67. doi: 10.1212/WNL.0000000000002646
35. Wang L, Liu J, Zhang Y, Zeng F, Li N, Qi J. Observation on the effect of repeated transcranial magnetic stimulation on children with cerebral palsy. Chin J Rehab. (2020) 35(02):91–4. doi: 10.3870/zgkf.2020.02.010
36. Liang C, Gu Q, Li M, Su M. Effects of repetitive transcranial magnetic stimulation combined with mandatory induction therapy on upper limb function in children with hemiplegic cerebral palsy. Chin J Phys Med Rehabil. (2020) 42(06):515–8. doi: 10.3760/cma.j.issn.0254-1424.2020.06.007
37. Li X, Qiu A, Jin X, Yang Z, Li L, Li Z. The effect of repetitive transcranial magnetic stimulation combined with rehabilitation training on the promotion of motor function development and intelligence improvement in children with cerebral palsy. Chin J Pract Nervous Dis. (2015) 18(23):17–9. doi: 10.3969/j.issn.1673-5110.2015.23.010
38. Wang Y, Zhou Z. Effects of repetitive transcranial magnetic stimulation on motor function in children with cerebral palsy complicated with epilepsy. Chin J Rehab Theory Pract. (2016) 22(01):98–102. doi: 10.3969/j.issn.1006-9771.2016.01.022
39. Zhang L, Li X, Zhang Y, Tang J. Effects of repetitive transcranial magnetic stimulation on upper limb motor function in children with spastic hemiplegic cerebral palsy. Heilongjiang Med Pharm. (2018) 41(05):187–9. doi: 10.3969/j.issn.1008-0104.2018.05.090
40. Wu G, Bao K, Li Z, Li J, Yang Z. Effects of repetitive transcranial magnetic stimulation on language development and motor function in children with spastic cerebral palsy. Prog Mod Biomed. (2017) 17(29):5716–5719 + 5772. CNKI:SUN:SWCX.0.2017-29-028
41. Zhang L, Xi S, Zhang L, Wang L, Ma Q, Wang J. Effects of repetitive transcranial magnetic stimulation on spasticity and motor function in children with spastic cerebral palsy. Chin J Rehabil. (2015) 30(03):171–3. doi: 10.3870/zgkf.2015.03.003
42. Li H, Yin H, Zou Y, Wang H, Chen T, Wang J. Effects of repetitive transcranial magnetic stimulation on motor function of children with spastic hemiplegic cerebral palsy. Chin J Phys Med Rehab. (2016) 38(06):433–5. doi: 10.3760/cma.j.issn.0254-1424.2016.06.009
43. Liang Y. The effect of repeated transcranial magnetic stimulation on the recovery of balance function in children with spastic hemiplegic cerebral palsy. Heilongjiang Med Pharm. (2020) 43(04):160–2. CNKI:SUN:KXJY.0.2020-04-072
44. Xu Y. Effects of virtual reality training combined with repetitive transcranial magnetic stimulation on motor function of children with cerebral palsy. J Qiqihar Med Univ. (2019) 40(11):1361–2. CNKI:SUN:QQHB.0.2019-11-017
45. Wang G. Effects of rehabilitation training combined with repetitive transcranial magnetic stimulation on motor function of children with cerebral palsy complicated by epilepsy. Mod Diagn Treat. (2017) 28(18):3354–5. CNKI:SUN:XDZD.0.2017-18-012
46. Li X, Song W, Qiu A, Jin X, Wang Y, Li Z, et al. Effects of transcranial magnetic stimulation combined with rehabilitation training on the development of daily fine motor and cognitive function in children with cerebral palsy. Chin Gen Pract. (2016) 19(18):2184–7. doi: 10.3969/J.Issn.1007-9572.2016.18.014
47. Deng Y, Cao M, Zhang M, Wu X. Transcranial magnetic stimulation combined with muscle strength training to improve the cognitive and motor function of children with cerebral palsy. Chin J Woman Child Health Res. (2019) 30(01):15–8. CNKI:SUN:SANE.0.2019-01-005
48. Li J. Effects of transcranial magnetic stimulation combined with muscle strength training on cognitive and motor function in children with cerebral palsy. Chin J Convalesc Med. (2020) 29(02):149–51. doi: 10.13517/j.cnki.ccm.2020.02.015
49. Bai Y, Wang X, Chen H. Application of transcranial magnetic stimulation combined with core strength training in children with spastic cerebral palsy. Chin Gen Pract. (2020) 23(02):177–82. doi: 10.12114/j.issn.1007-9572.2019.00.307
50. Ma X, Ye H. The effect of transcranial magnetic stimulation combined with comprehensive rehabilitation training in the treatment of 60 children with cerebral palsy. Ningxia Med J. (2017) 39(12):1145–6. doi: 10.13621/j.1001-5949.2017.12.1145
51. Zhang Y, Ding J. Effects of transcranial magnetic stimulation on gross motor function in children with cerebral palsy. Chin J Rehab Theory Pract. (2012) 18(06):515–7. doi: 10.3969/j.issn.1006-9771.2012.06.005
52. Bao N, Liu C. The effect of low-frequency repetitive transcranial magnetic stimulation combined with occupational therapy on upper limb function in children with hemiplegic cerebral palsy. Chin Foreign Med Res. (2016) 14(35):5–7. doi: 10.14033/j.cnki.cfmr.2016.35.003
53. Qiu H. Ultra-low frequency transcranial magnetic stimulation combined with comprehensive rehabilitation to improve gross motor function in children with cerebral palsy. Neural Injury Funct Reconstr. (2015) 10(04):364–5. doi: 10.3870/sjsscj.2015.04.033
54. Duan L. The application value of ultra-low frequency transcranial magnetic stimulation combined with rehabilitation training in the treatment of children with cerebral palsy. Henan Med Res. (2020) 29(10):1769–71. CNKI:SUN:HNYX.0.2020-10-015
55. Cao L. The effect of ultra-low frequency transcranial magnetic stimulation combined with rehabilitation training on motor function and language function in children with cerebral palsy. Contemp Med. (2021) 27(04):54–6. doi: 10.3969/j.issn.1009-4393.2021.04.020
56. Feng J, Jia F, Jiang H, Li N, Li H, Du L. Effects of ultra-low frequency transcranial magnetic stimulation on motor function in children with spastic cerebral palsy. Chin J Contemp Pediatr. (2013) 15(03):187–91. doi: 10.7499/j.issn.1008-8830.2013.03.006
57. Fan Y, Liang J, Li J, Huang Z, Cen Y. The effect of comprehensive rehabilitation training combined with transcranial magnetic stimulation on improving the developmental quotient of children with cerebral palsy. China Med Pharm. (2016) 6(07):219–21+225. CNKI:SUN:GYKX.0.2016-07-066
58. Xu J, Fan Y, Gao J, Huang S. The effect of repeated transcranial magnetic stimulation on the language development of children with cerebral palsy. Chin Pediatr Integr Tradit Western Med. (2015) 7(04):367–9. 0.3969/j.issn.1674-3865.2015.04.028
59. Wang Y, Ma B. The effect of repetitive transcranial magnetic stimulation on the mental development of children with cerebral palsy complicated with epilepsy. Chin J Rehab Theory Pract. (2014) 20(12):1153–5. doi: 10.3969/j.issn.1006-9771.2014.12.014
60. Qiu A, Li X, Dong J, Yang Z, Li L, Li Z, et al. Application of neuromodulation therapy with transcranial magnetic stimulation in the rehabilitation of children with hypotonia cerebral palsy. Chin Gen Pract. (2015) 18(36):4510–3. doi: 10.3969/j.issn.1007-9572.2015.36.023
61. Zhang Y. Observation on the effect of transcranial magnetic stimulation combined with early rehabilitation training in the treatment of cerebral palsy with language development disorder. Med J Chin People’s Health. (2018) 30(16):83–4. doi: 10.3969/j.issn.1672-0369.2018.16.038
62. Yang J, Liu Q, Li X. The clinical effect of transcranial magnetic stimulation combined with language intervention in the treatment of language retardation in children with cerebral palsy. China Mod Med. (2020) 27(02):136–8. CNKI:SUN:ZGUD.0.2020-02-042
63. Tavasoli A, Azimi P, Montazari A. Reliability and validity of the Peabody Developmental Motor Scales-second edition for assessing motor development of low birth weight preterm infants. Pediatr Neurol. (2014) 51:522–6. doi: 10.1016/j.pediatrneurol.2014.06.010
64. Chang WH, Kim YH, Bang OY, Kim ST, Park YH, Lee PK. Long-term effects of rTMS on motor recovery in patients after subacute stroke. J Rehabil Med. (2010) 42:758–64. doi: 10.2340/16501977-0637
65. Khedr EM, Etraby AE, Hemeda M, Nasef AM, Razek AA. Long-term effect of repetitive transcranial magnetic stimulation on motor function recovery after acute ischemic stroke. Acta Neurol Scand. (2010) 121:30–7. doi: 10.1111/j.1600-0404.2009.01195.x
66. Mansur CG, Fregni F, Boggio PS, Riberto M, Gallucci-Neto J, Santos CM, et al. A sham stimulation-controlled trial of rTMS of the unaffected hemisphere in stroke patients. Neurology. (2005) 64:1802–4. doi: 10.1212/01.WNL.0000161839.38079.92
67. Dadashi F, Shahroki A, Nourian R, Irani A, Molavi M, Rafieenazari Z, et al. The effects of repetitive transcranial magnetic stimulation (rTMS) on balance control in children with cerebral palsy. (2019) 2019:5241–4. doi: 10.1109/EMBC.2019.8857361
68. Ko J, Kim M. Inter-rater reliability of the K-GMFM-88 and the GMPM for children with cerebral palsy. Ann Rehabil Med. (2012) 36:233–9. doi: 10.5535/arm.2012.36.2.233
69. Vry J. Altered cortical inhibitory function in children with spastic diplegia: a TMS study. Exp Brain Res. (2008) 186(4):611–8. doi: 10.1007/s00221-007-1267-7
70. Flett PJ. Rehabilitation of spasticity and related problems in childhood cerebral palsy. J Paediatr Child Health. (2003) 39:6–14. doi: 10.1046/j.1440-1754.2003.00082.x
71. Gupta M, Lal Rajak B, Bhatia D, Mukherjee A. Effect of r-TMS over standard therapy in decreasing muscle tone of spastic cerebral palsy patients. (2016) 40:210–6. doi: 10.3109/03091902.2016.1161854
72. Hustad KC, Gorton K, Lee J. Classification of speech and language profiles in 4-year-old children with cerebral palsy: a prospective preliminary study. J Speech Lang Hear Res. (2010) 53:1496–513. doi: 10.1044/1092-4388(2010/09-0176)
73. Bax M, Tydeman C, Flodmark O. Clinical and MRI correlates of cerebral palsy: the European Cerebral Palsy Study. JAMA. (2006) 296:1602–8. doi: 10.1001/jama.296.13.1602
Keywords: cerebral palsy, motor dysfunction, rehabilitation, repetitive transcranial magnetic stimulation, non-invasive brain stimulation
Citation: Sun Y, Wang L, Peng J, Huang Y, Qiao F and Wang P (2023) Effects of repetitive transcranial magnetic stimulation on motor function and language ability in cerebral palsy: A systematic review and meta-analysis. Front. Pediatr. 11:835472. doi: 10.3389/fped.2023.835472
Received: 14 December 2021; Accepted: 26 January 2023;
Published: 16 February 2023.
Edited by:
Rafael Galindo, Washington University in St. Louis, United StatesReviewed by:
Iona Novak, The University of Sydney, AustraliaJunlong Luo, Shanghai Normal University, China
© 2023 Sun, Wang, Peng, Huang, Qiao and Wang. This is an open-access article distributed under the terms of the Creative Commons Attribution License (CC BY). The use, distribution or reproduction in other forums is permitted, provided the original author(s) and the copyright owner(s) are credited and that the original publication in this journal is cited, in accordance with accepted academic practice. No use, distribution or reproduction is permitted which does not comply with these terms.
*Correspondence: Lei Wang wangleipx168@163.com Pu Wang wangpu1831@163.com
†These authors contributed equally to this work and share first authorship
Specialty Section: This article was submitted to Pediatric Neurology, a section of the journal Frontiers in Pediatrics