- 1Multidisciplinary Breast Clinic Antwerp University Hospital, University of Antwerp, Edegem, Belgium
- 2Faculty of Medicine, University of Antwerp, Edegem, Belgium
- 3Department of Medical Oncology, Antwerp University Hospital, Edegem, Belgium
- 4Department of Pathology, Antwerp University Hospital, Edegem, Belgium
- 5Biobank, Antwerp University Hospital, Edegem, Belgium
- 6Unit of Gynecologic Oncology, Department of Obstetrics and Gynecology, Antwerp University Hospital, University of Antwerp, Edegem, Belgium
Several retrospective and prospective studies have shown that genomic alterations in Estrogen-receptor one (ESR1) can be characterized not only in tissue samples but also by sequencing circulating tumor DNA (ctDNA) in liquid biopsy. Therefore, liquid biopsy is a potential noninvasive surrogate for tissue biopsy. This meta-analysis was designed to compare the prevalence of ESR 1 mutation detected with liquid biopsy and tissue biopsy. A pooled meta-analysis of studies published between 1 January 2007 and 1 March 2021 was conducted regarding the methodologies used for ESR1 mutation analysis. Liquid biopsy is a valid, inexpensive, and attractive noninvasive alternative to tumor biopsies for the identification of ESR1 mutations. Liquid biopsy for ESR 1 analysis would facilitate regular testing, allowing monitoring of the sensitivity to ET and guiding treatment strategies.
Introduction
Our understanding of cancer biology using minimally invasive techniques to collect circulating tumor DNA (ctDNA) from body fluids is rapidly evolving. The fragmented DNA segments found in blood samples of cancer patients could be used to validate the presence of tumor specific mutations (1–3).
Breast tumors commonly express hormone receptors (HR), including the estrogen receptor (ER) and/or progesterone receptor (PR) (4). Endocrine therapy (ET), which targets the ER pathway, is a major treatment modality for HR-positive cancers. At diagnosis, ER positivity is a favorable prognostic factor for breast cancer (BC). However, this positive prognostic effect degrades over time (5). Resistance to ET is considered an important step in the natural evolution of HR-positive BC and is related to a higher risk of recurrence and increased mortality (6). In the last decade, several clinical trials have assessed the incidence of ESR1 mutations in BC based on liquid biopsies. This knowledge is likely to encompass important information on the development of resistance to ET in real time, and is eventually applied for patient/treatment selection and monitoring of ET efficacy (7, 8).
Currently, the detection and molecular characterization of ctDNA represents one of the most active fields of translational cancer research. The recent development of NGS has expanded the monitoring of ctDNA with a range of diagnostic clinical applications. However, there are several limitations, including difficulties in interpreting novel or rare mutations and cost issues (9). On the other hand, the newly developed digital polymerase chain reaction (dPCR) has the potential to detect rare mutants, in which a variant of a single-nucleotide polymorphism is predominantly present among wild-type sequences (10). Droplet digital polymerase chain reaction (ddPCR), which can perform thousands of PCRs on a nanoliter scale simultaneously, would be an attractive method for massive parallel sequencing to identify the significance of low-frequency rare mutations. ddPCR is the most appropriate method for detecting known hotspot mutations, but is not the most appropriate approach for detecting unknown and ‘not-targeted’ mutations (11). Compared with singleplex reactions, multiplexing ddPCR not only increases the number of targets measured in a single reaction but also reduces the amount of clinical material required to analyze multiple single-nucleotide polymorphisms by measuring >1 target in a single reaction (12).
In BC, as in other solid tumors, the genomic alterations found within a given tumor biopsy may differ depending on the region sampled, as between the primary tumor and metastatic deposits, and even between different metastatic deposits (13). Genomic analyses of BC have provided direct evidence of spatial and temporal intratumoral heterogeneity (14, 15). Currently, clinical and therapeutic decisions are based on individual tissue biopsies that may not be representative of the entire tumor burden or on real-time assessments of the tumor genotype (16). This practical limitation could be overcome by the use of liquid biopsies, which represent a promising technique for decoding tumor heterogeneity.
In this review, we compare the prevalence ESR1 mutations for female patients with ER+ recurrent/metastasized BC pretreated with ET as detected by liquid biopsy versus standard tissue biopsy. This review discusses and summarizes the techniques of DNA sequencing, including ddPCR and NGS, which are used by several laboratories to address the potential clinical needs of ESR1 mutation-specific BC. A thorough understanding of these applications may provide useful information for ESR1 testing, ensure reliable test results for use in clinical practice, and eventually advance personalized therapeutic strategies. Aromatase Inhibitors (AIs) reduce circulating estrogen by inhibiting estrogen synthesis in peripheral tissues by 90% or more, but do not affect estrogen production in the ovaries. ESR1 mutations allow ERα to be activated in the absence of estradiol, eliminating AIs activity and making ESR1 a potential predictive factor.
Materials and methods
A literature search was conducted using two databases: PubMed and Thomson Reuters Web of Science. The following search terms were used: [(‘liquid biopsy’ OR ‘tissue sample’) AND (‘ESR1 mutation’ OR ‘ESR mutation’) AND (‘next generation sequencing’ OR ‘ digital PCR) AND (‘breast cancer’)]. The reviewers performed the procedure of study selection by: (1) assessment of each clinical trial independently in an unblended standardized manner; (2) duplicates were removed afterwards; (3) only full-text English articles were included; (4) after the independent screening, all the results were compared and the articles with conflict were discussed until agreement was established; (5) the article should refer to an interventional trial; reviews, lectures and book sections were excluded; and (6) the final decision for study selection of the remaining articles were treated separately; studies that did not meet the inclusion criteria or did not contain useful information for this systematic review were excluded after consensus.
The included articles were published between 1 January 2007 and 1 March 2021. The extracted data included the type of clinical trial (RCT or non-RCT), characteristics of the study population, number of participants, exclusion of primary disease, nature of biopsy samples (plasma or tissue), and method of mutation analysis (ddPCR or NGS). The selected patients met the following inclusion criteria: 1. Female aged >18 years, 2. ER+ breast cancer cells pre-treated with endocrine therapy, and 3. Disease recurrence and metastases. Patients with primary breast cancer were excluded from this study. Overall incidence of ESR1 mutation was assessed using a meta analysis for proportions. Because of high diversity in type of studies, patients and therapies, a random effects model is used. Heterogeneity is judged by forest plot, Cochran Q and I-squared. Results are presented in a forest plot for proportions. Incidence of ESR1 mutation was compared between plasma versus tissue samples and between ddPCR versus NGS. Subgroup differences are evaluated by the between subgroups heterogeneity statistic in the random effects meta-analysis. P-values were considered statistically significant if it was < 0,05.
Results
A literature search fulfilling the previously explained search criteria and taking place in the proposed time interval resulted in a collection of 153 articles in PubMed and 204 articles in Web of Science. A total of 231 articles were evaluated after excluding duplicates. Articles that did not meet the inclusion criteria or that did not contain useful information for this systematic review, were discarded after consensus. Thereafter, 16 articles, four multicenter double-blinded RCTs, and 12 cohort trials were obtained for this meta-analysis (Figure 1).
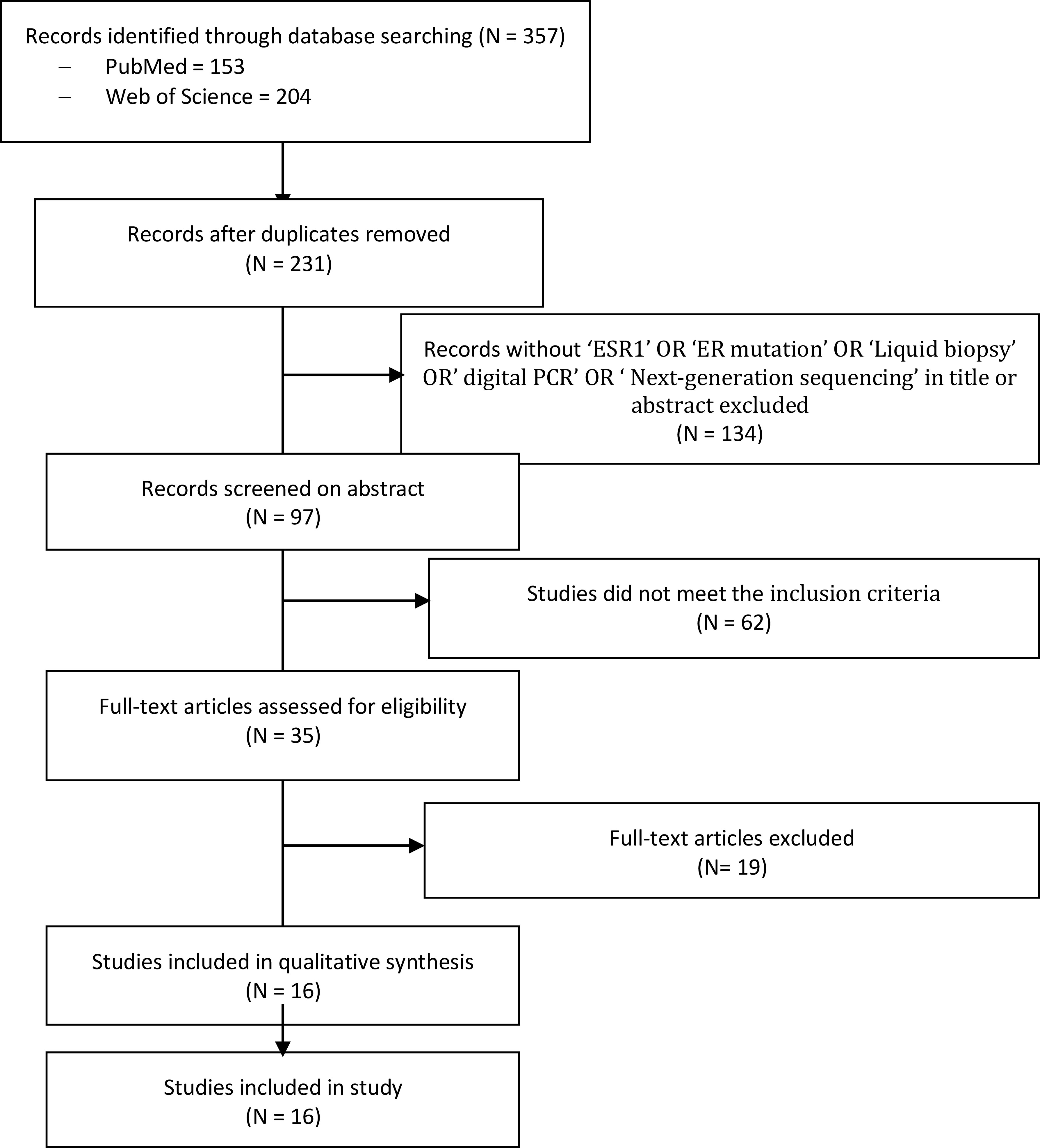
Figure 1 PRISMA flow diagram and the process of data selection. Selection of studies was performed using predefined data fields, taking study quality indicators into consideration. Eligibility criteria included terms with ‘ESR1’, ‘ESR mutation’ and ‘liquid biopsy’ or ‘tissue sample’, and/or ‘next generation sequencing’ and/or ‘ddPCR’ in the abstract or title by using the endnote library search option.
From the reviewed studies, we included 2,744 pooled tissues and plasma samples for this analysis. Plasma samples were used in 57.1% (1,568/2,744) of the study population, tissue samples in 37.7% (1,033/2,744), and tissue-plasma pairs in 5.2% (143/2,744). Tissue samples were obtained from loco regional or distant metastatic sites in four and six studies, respectively. Both archived and recent plasma samples were used for ESR1 analysis in four and two studies, respectively. ESR1 analysis was performed using ddPCR in 61.3% (1,684/2,744) of the study population and NGS in 38.7% (1,060/2,744).
Of the 2,744 samples pooled for this study, the overall incidence of ESR1 mutation is 23% (95 CI 18%–28%) (Figure 2). However, the different studies demonstrated a considerable variability in the prevalence of ESR1 mutations ranged from 11% in Schiavon et al. (17) and Yanagawa et al. (18) to 55% in Robinson et al. (19) The wide range in incidence rate of ESR1 mutation could be attributed to heterogeneity in the study populations.
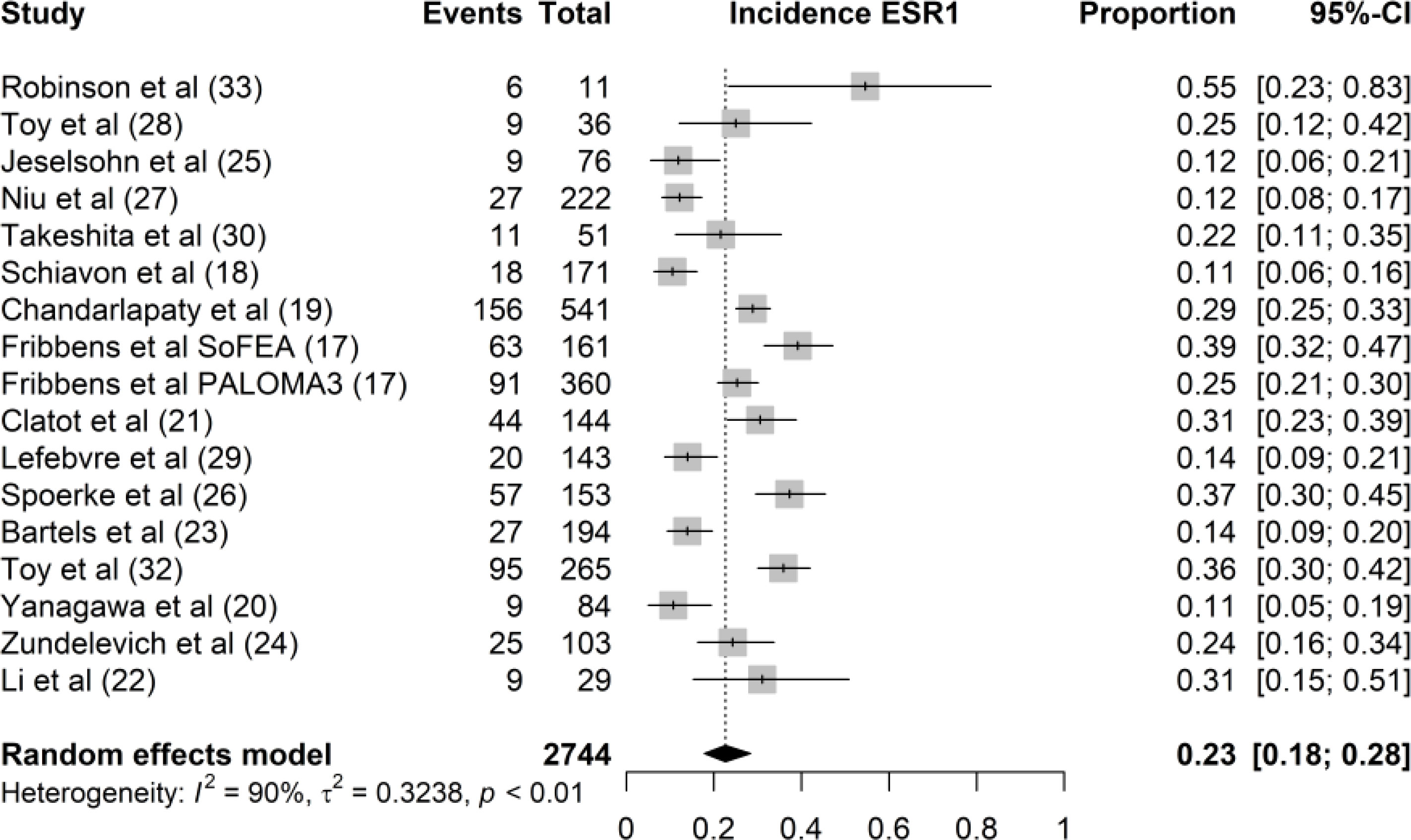
Figure 2 Forest plot of the overall incidence rate of ESR1 mutation. The proportion of ESR1 mutation per study is displayed with a grey box, with the 95%-CI visualized by horizontal lines. The overall frequency of ESR1 mutations was 0.23 (95%-CI: 0.18 0.28), as indicated by the black diamond at the bottom of the forest plot.
In the articles under review, nine studies used tissue biopsy while five studies used plasma biopsy. In a trial by Yanagawa et al. (18), whole-exon sequencing of the ESR1 gene was performed separately in tissue and plasma samples. In 15 of the 16 studies included, the incidence rates of ESR1 mutations in plasma and tissue samples were 26% (95% CI, 18%–35%) and 21% (95% CI, 15%–28%), respectively (Figure 3). We found no significant difference in ESR1 mutation incidence between plasma and tissue samples (P = 0.34). The samples from Lefebvre et al. (20) were excluded from the comparative analysis between liquid and tissue biopsies because ESR1 sequencing was performed in tissue-blood pairs. In this study, the mutational profiles of 143 tissue-blood pairs from patients with hormone receptor-positive (HR+) metastatic BC were analyzed. Twelve genes (TP53, PIK3CA, GATA3, ESR1, MAP3K1, CDH1, AKT1, MAP2K4, RB1, PTEN, CBFB, and CDKN2A) were significantly mutated in MBC. This study concluded that ESR1 mutation was the most frequent mutation in the HR+ MBC subgroup (n = 143). In total, 22 mutations were identified in 20 of 143 patients with HR+/HER2− BC (14%). Li et al. demonstrated that ESR1 mutations could be detected by serial monitoring of ctDNA. In this study, mutation profiles, including ESR1, were highly concordant between plasma and paired tissue samples from 45 patients with MBC (20).
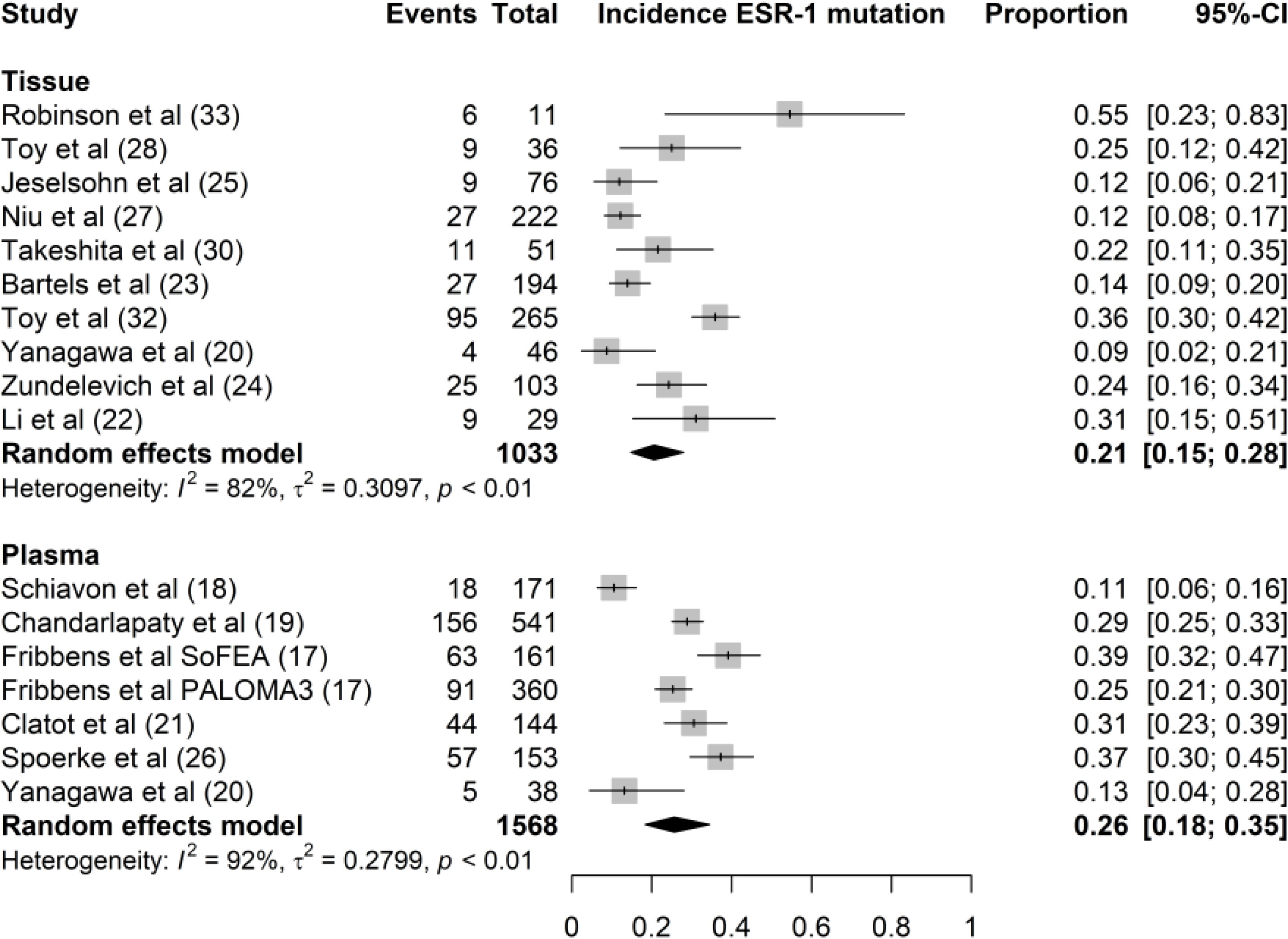
Figure 3 Forest plot of the comparison of ESR1 mutation in tissue versus plasma samples. Grey boxes indicate the proportion of ESR1 mutations in each study, with a horizontal line representing the 95% CI. Overall proportion and 95% CI in tissue and plasma subgroup is displayed with a black diamond. We found no significant difference in ESR1 mutation incidence between plasma and tissue samples (P=0.34).
Both ddPCR and NGS were used to determine ESR1 mutations in the tissue and plasma samples. ddPCR was used in seven studies and NGS was used in nine studies. ddPCR is the standard method for ESR1 testing in liquid biopsies, except in the study by Yanagawa et al. NGS was used to analyze both tissue and plasma samples. However, both NGS and ddPCR have been used for ESR1 testing of tissue biopsies. The incidence rates of ESR1 mutations using ddPCR and NGS were 26% (95% CI, 20%–33%) and 19% (95% CI, 13%–27%), respectively (Figure 4). We found no significant difference in ESR1 mutation incidence between ddPCR and NGS techniques (P = 0.15).
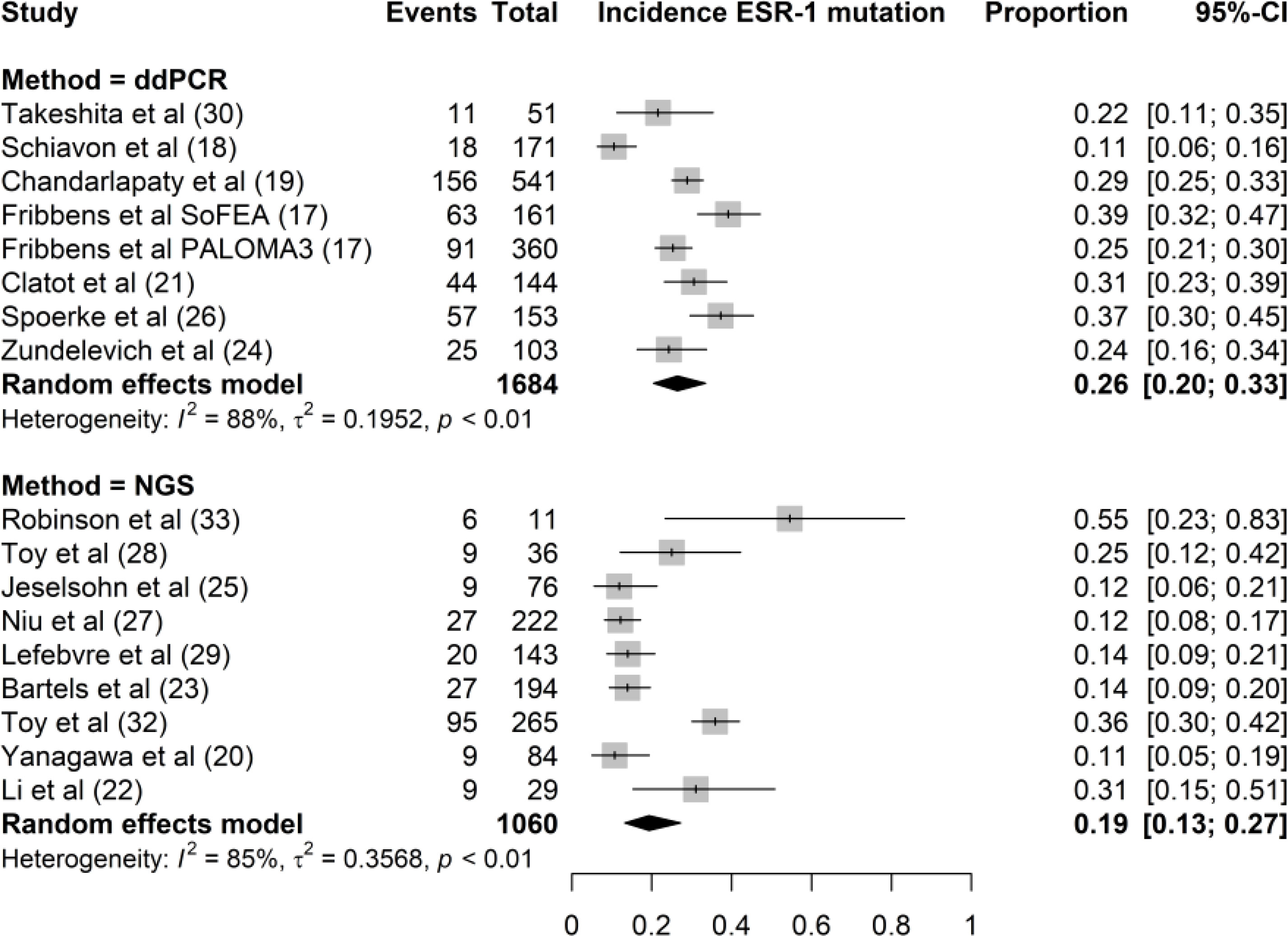
Figure 4 Forest plot of the comparison of the proportion of ESR1 mutation using NGS versus ddPCR techniques. Grey boxes indicate the proportion of ESR1 mutations in each study, with a horizontal line representing the 95% CI. Overall proportion and 95% CI in NGS and ddPCR subgroup is displayed with a black diamond. We found no significant difference in ESR1 mutation incidence between the two techniques (P=0.15).
All studies on both plasma and tissue samples have described their methodology regarding collection, processing, and analysis in a more or less complete manner, despite some missing pre-analytical aspects (17–19, 21–32). Of the six studies researching plasma samples, only one used NGS (18), while the other studies used ddPCR (17, 21–24). Two of the 10 studies used ddPCR (29, 32), while eight other studies used NGS (18, 19, 25–28, 30, 31). Remarkably, all studies performing ddPCR, whether on tissue samples or plasma samples, used the same platform (Bio-Rad QX200 ddPCR system) and more or less the same pre-analytical and DNA-quantification steps; however, the hotspot mutation panel might differ according to the respective study (17, 21–24, 29, 32). In contrast, many different NGS platforms are used, with many different library preparation kits and quantification tools. Some of the NGS platforms used are the Illumina HiSeq 2000 series and the Ion Torrent platform (18, 19, 25–27, 30, 31). Additionally and important to note, genomic profiling was performed by Foundation Medicine on the Foundation One platform in one study on tissue samples (28). As this study did not aim to investigate the different aspects of the ESR1 analysis methodology, we will not go into detail in the different preanalytical, DNA-quantification, and mutation analysis steps. Nonetheless, these data can be found in the schematic overview of the available preanalytical and analytical parameters provided in Tables 1, 2. We previously published in an earlier review a detailed ESR1 specific mutational profile analysis, including D538G, Y537S, and Y537N as the most prevalent mutations (33).
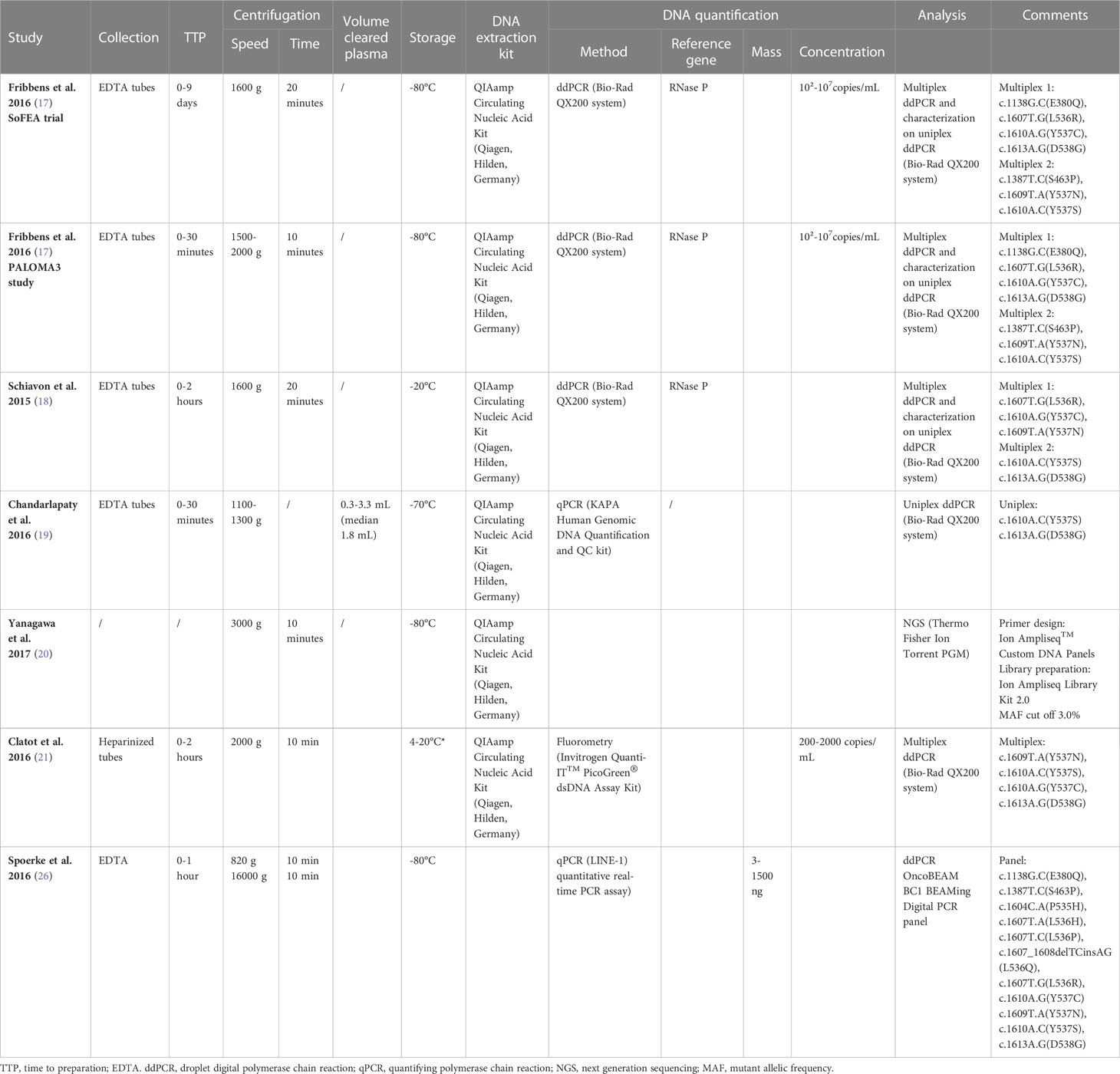
Table 1 Overview of the collection, processing and ESR1 mutation analysis in all studies concerning the analysis of plasma samples.
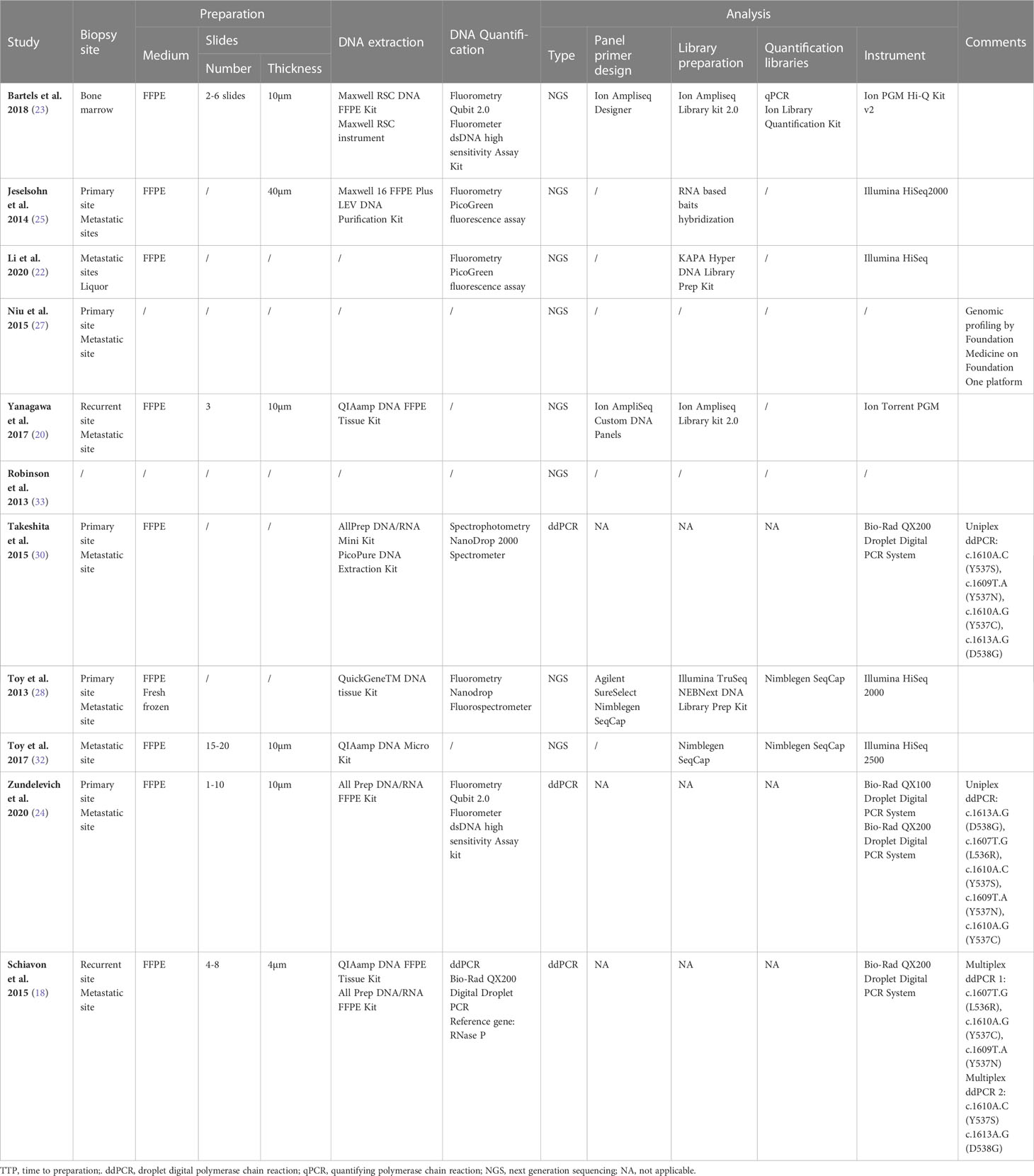
Table 2 Overview of the collection, processing and ESR1 mutation analysis in all studies concerning the analysis of Tissue samples.
Discussion
A growing body of clinical trials on ER+ BC strongly supports the use of ESR1 as a valid predictor of response to ET. Understanding the mechanisms of acquired resistance to ET can impact therapeutic strategies to overcome the effects of mutant genes responsible for ET failure. Analysis of ESR1 mutations conferring resistance to ET has already been demonstrated in patients with ER+ advanced stage BC (31, 33). Furthermore, in vitro studies have shown that ESR1 mutations are likely to be acquired because of ET deprivation (34). However, ESR1 mutations are rare in endocrine therapy-naive ER+/HER2− BC, and the frequency is even lower if an AI has not been administered in the adjuvant setting: 3%–6% (17, 35). In contrast, studies that enrolled patients after first-line AI therapy found that approximately 30% of patients have ESR1mutated (17, 21, 36–41). In the current meta-analysis, the incidence rate of ESR1 was 23%, which was consistent with the results of previous trials.
To date, screening for ESR1 in ER+ BC is not considered the standard of care; tumor tissue sampling remains the standard method for addressing tumor biology, despite issues in terms of acquisition and utility; tissue biopsies are invasive and do not have potential complications, and sample preservation may hamper the use of tumor tissue for cancer sequencing (42). Intra/inter-tumor heterogeneity, mostly observed in advanced cancers, is also a major limitation of tumor biopsy (13, 43). This heterogeneity is partially attributed to dynamic genetic changes that occur after therapeutic selective pressure (44). Therefore, tissue biopsy may not be the most appropriate method for mutational analysis of metastatic BC, especially when looking for rare point mutations in a background of wild-type sequences, as in the case of ESR1.
Liquid biopsy is a rapid, cost-effective, and noninvasive technique, capable of capturing molecular heterogeneity during disease evolution and potentially overcoming the aforementioned issues (44, 45). Cell-free DNA (cfDNA) is a potential surrogate for the entire tumor genome (46). The cfDNA fragments provide a representative reflection of genomic alterations of the original tumor because cfDNA fragments are derived from all tumor sites in a patient’s body circulation (45, 47). Acquired resistance to endocrine therapy prior to disease progression could also be monitored by longitudinally analysis of ESR1 mutations (33). ctDNA analyses are highly sensitive because DNA is abundant in most advanced malignancies, allowing the successful tracking of ESR1 mutations (44). Therefore, liquid biopsy is widely available and easier to perform than standard tumor biopsies (48). Recent improvements in PCR techniques for analyzing cfDNA provide a potential alternative to tumor biopsies, provide information on tumor genetic alterations, and have been used as diagnostic, prognostic, or even predictive tools (49). Our results showed no statistical difference in ESR1 incidence for plasma-tissue comparison (21% vs. 26%; P = 0.34), in accordance with the results of previous reports (21, 23, 29, 30, 32).
At present, ddPCR represents a low-cost and effective technique that has been recently commercialized to detect and quantify small amounts of genetic material (50, 51). ddPCR is a potential alternative to next-generation sequencing (NGS); however, it is only suitable for testing known mutations. Recently, PCR-based digital investigations have been coupled with techniques that use NGS to enumerate rare mutant variants in complex DNA mixtures (52). Both techniques support the screening and clinical validity of genomic alterations in ctDNA as a ‘liquid biopsy’ in breast cancer, including ESR1 mutants (53, 54). ddPCR is particularly useful for the detection of rare mutant DNA sequences in large quantities of background wild-type sequences. Our results showed no statistical difference in ESR1 incidence between the ddPCR-NGS comparisons (26% vs. 19%; P = 0.15).
Although the analysis of cfDNA is a truly growing field, liquid biopsy is not yet routinely used in clinical practice to decode the tumor genome, despite the fact that acquiring plasma samples is more accessible and minimally invasive compared to tissue samples. Furthermore, when comparing the cost-effectiveness of ddPCR and NGS, there was no clear winner. It is generally accepted that ddPCR is a low-cost, time-saving, and effective method for genomic analyses (55, 56). Moreover, ddPCR is designed highly sensitive detection of hotspot mutations, making it more suitable for the detection of low concentrations of cfDNA in plasma samples. NGS relies on different reagents but is capable of testing multiple samples for multiple genes simultaneously. This process is, of course, more time-consuming (7–10 days) and less cost-effective (55, 56). However, assuming a fair number of samples to be tested in routine practice, ddPCR may be a cost-effective and time-sparing method, on the condition that hotspot mutations of interest are known, as is the case for ESR1. In this case, ddPCR may require as little as half the cost of NGS. In our opinion, the analysis of liquid biopsy using ddPCR is the most favorable combination for ESR1 testing in terms of sample feasibility, time, and cost. Table 3 shows the potential advantages of liquid biopsy compared to tissue biopsy.
To our knowledge, this is the largest meta-analysis to carry out a comparative analysis between liquid and tissue biopsies, and between ddPCR and NGS. The results of this review show no significant difference in prevalence of ESR1 mutation detected with liquid biopsy or tissue biopsy. Different studies show a large variability in the prevalence of ESR1 mutations (11% to 55%). The wide range in the incidence rates of ESR1 mutations could be attributed to heterogeneity in the study populations and inter-laboratory findings. A recent review on the progress in detecting ESR1 mutations based on liquid biopsy and different sequencing technologies in ER+ MBC also highlights its potential clinical impacts and prospects in accordance with these conclusions (57).
According to the hypothesis of this review, there was a risk of selection bias because the selected patients had progressive and recurrent BC. Furthermore, meta-analyses on their own may suffer from several sources of bias in individuals and across studies. First, not all trials lead to publication, which induces publication bias for positive findings, and the language of the original publication might have resulted in a selection bias. For some research questions, only a small number of studies were included in the meta-analysis. The quality of the studies varied. Due to the broad scope of our research questions, not only randomized controlled trials, but also case–control and uncontrolled cohort trials were eligible for inclusion in the review. Confounding and baseline differences may be more pronounced in non-randomized or uncontrolled studies than in randomized controlled trials. Furthermore, paired tissue plasma samples were available for only 5.2% of samples. Taken together, these limitations discourage the difficulty of obtaining evidence that plasma is non-inferior to tissue, since both have been measured in different patients and in different studies; solid proof for such a conclusion could only be derived from a large-scale prospective study comparing tissue and plasma samples from the same patients.
This meta-analysis demonstrates that ESR1 mutations are found at high frequency in liquid biopsies of ER+ recurrent/metastasized BC and could be tracked relatively simply and inexpensively using both ddPCR and NGS technologies. Both technologies are equally effective for the identification of ESR1 mutations in tissue and plasma samples; however, ddPCR is inexpensive. Regular ESR1 mutation analysis is needed during endocrine treatment before disease recurrence or progression. The incorporation of cfDNA-based ESR1 analysis is the current challenge for clinicians to ensure that ESR1 testing can be integrated into routine clinical care; however, widespread diagnostic application requires for rigorous studies to demonstrate not only clinical validity but also clinical utility. Recent data from a small cohort of patients suggest that liquid biopsy can reveal the presence of minimal residual disease several years before the appearance of clinically detectable metastatic disease, demonstrating that comprehensive liquid biopsy analysis provides important information for the therapeutic management of breast cancer patients (58). However, the clinical utility of ESR1 analysis as an early predictor needs to be proven in a randomized prospective clinical setting to guide therapeutic decisions on liquid biopsy analysis and on established endpoints (59). Ongoing trials in this setting, such as the SERENA 6, have already addressed the efficacy and safety of switching the ET partner of first-line CDK4/6i therapy at the earliest time point when ESR1m is detected in ctDNA, and before clinical disease progression (60).
In conclusion, the present pooled meta-analysis only provides additional evidence that liquid biopsies can replace tumor tissue biopsies in molecular screening programs for ESR1 mutations in a potentially easier and cost-effective approach. However, the key question of whether changing therapy based on ESR1 mutations before radiologic progression will improve long-term disease control and OS compared to therapy changes based on radiologic progression is yet to be answered.
Author contributions
Study design, analysis, and interpretation of data: ON. Acquisition of data, technical laboratory support: GB. Statistical analysis: ON, KP, and WT. Study supervision: MH, KP, and WT. ON wrote the manuscript. The final manuscript was edited, reviewed and approved by MH, KP, GB, and WT.
Funding
The Ladies Circle Dendermonde, located in Sint-Gillislaan 47,9200 Dendermonde, Belgium, funded this study.
Acknowledgments
This work is dedicated to the spirit of Mme. Frija De Bock, a member of Ladies Circle Dendermonde, who died at the age of 41 years because of breast cancer.
Conflict of interest
The authors declare that the research was conducted in the absence of any commercial or financial relationships that could be construed as a potential conflict of interest.
Publisher’s note
All claims expressed in this article are solely those of the authors and do not necessarily represent those of their affiliated organizations, or those of the publisher, the editors and the reviewers. Any product that may be evaluated in this article, or claim that may be made by its manufacturer, is not guaranteed or endorsed by the publisher.
References
1. Guttery DS, Page K, Hills A, Woodley L, Marchese SD, Rghebi B, Shaw JA. Noninvasive detection of activating estrogen receptor 1 (ESR1) mutations in estrogen receptor-positive metastatic breast cancer. Clin Chem (2015) 61(7):974–82. doi: 10.1373/clinchem.2015.238717
2. Norton SE, Norton SE, Lechner JM, Williams T, Fernando MR. A stabilizing reagent prevents cell-free DNA contamination by cellular DNA in plasma during blood sample storage and shipping as determined by digital PCR. Clin Biochem (2013) 46(15):1561–5. doi: 10.1016/j.clinbiochem.2013.06.002
3. Najim O, Huizing M, Papadimitriou K, Trinh XB, Pauwels P, Goethals S, Tjalma W. The prevalence of estrogen receptor-1 mutation in advanced breast cancer: The estrogen receptor one study (EROS1). Cancer Treat Res Commun (2019) 19:100123. doi: 10.1016/j.ctarc.2019.100123
4. Lim E, Metzger-Filho O, Winer EP. The natural history of hormone receptor-positive breast cancer. Oncol (Williston Park) (2012) 26(8):688–94, 696.
5. Bentzon N, Düring M, Rasmussen BB, Mouridsen H, Kroman N. Prognostic effect of estrogen receptor status across age in primary breast cancer. Int J Cancer (2008) 122(5):1089–94. doi: 10.1002/ijc.22892
6. Clarke R, Tyson JJ, Dixon JM. Endocrine resistance in breast cancer - An overview and update. Mol Cell Endocrinol (2015) 418:220–34. doi: 10.1016/j.mce.2015.09.035
7. Tabarestani S, Motallebi M, Akbari ME. Are estrogen receptor genomic aberrations predictive of hormone therapy response in breast cancer? Iran J Cancer Prev (2016) 9(4):e6565. doi: 10.17795/ijcp-6565
8. Alix-Panabieres C, Pantel K. Circulating tumor cells: liquid biopsy of cancer. Clin Chem (2013) 59(1):110–8. doi: 10.1373/clinchem.2012.194258
9. Yohe S, Thyagarajan B. Review of clinical next-generation sequencing. Arch Pathol Lab Med (2017) 141(11):1544–57. doi: 10.5858/arpa.2016-0501-RA
10. Vogelstein B, Kinzler KW. Digital PCR. Proc Natl Acad Sci U.S.A. (1999) 96(16):9236–41. doi: 10.1073/pnas.96.16.9236
11. Angus L, Beije N, Jager A, Martens JW, Sleijfer S. ESR1 mutations: Moving towards guiding treatment decision-making in metastatic breast cancer patients. Cancer Treat Rev (2017) 52:33–40. doi: 10.1016/j.ctrv.2016.11.001
12. Huggett JF, Whale A. Digital PCR as a novel technology and its potential implications for molecular diagnostics. Clin Chem (2013) 59(12):1691–3. doi: 10.1373/clinchem.2013.214742
13. Gerlinger M, Rowan AJ, Horswell S, Larkin J, Endesfelder D, Gronroos E, Swanton C. Intratumor heterogeneity and branched evolution revealed by multiregion sequencing. N Engl J Med (2012) 366(10):883–92. doi: 10.1056/NEJMoa1113205
14. Nik-Zainal S, Van Loo P, Wedge DC, Alexandrov LB, Greenman CD, Lau KW, et al. The life history of 21 breast cancers. Cell (2012) 149(5):994–1007. doi: 10.1016/j.cell.2012.04.023
15. Yates LR, Gerstung M, Knappskog S, Desmedt C, Gundem G, Van Loo P, Campbell PJ. Subclonal diversification of primary breast cancer revealed by multiregion sequencing. Nat Med (2015) 21(7):751–9. doi: 10.1038/nm.3886
16. De Mattos-Arruda L, Caldas C. Cell-free circulating tumour DNA as a liquid biopsy in breast cancer. Mol Oncol (2016) 10(3):464–74. doi: 10.1016/j.molonc.2015.12.001
17. Schiavon G, Hrebien S, Garcia-Murillas I, Cutts RJ, Pearson A, Tarazona N, Turner NC. Analysis of ESR1 mutation in circulating tumor DNA demonstrates evolution during therapy for metastatic breast cancer. Sci Transl Med (2015) 7(313):313ra182. doi: 10.1126/scitranslmed.aac7551
18. Yanagawa T, Kagara N, Miyake T, Tanei T, Naoi Y, Shimoda M, et al. Detection of ESR1 mutations in plasma and tumors from metastatic breast cancer patients using next-generation sequencing. Breast Cancer Res Treat (2017) 163(2):231–40. doi: 10.1007/s10549-017-4190-z
19. Robinson DR, Wu YM, Vats P, Su F, Lonigro RJ, Cao X, et al. Activating ESR1 mutations in hormone-resistant metastatic breast cancer. Nat Genet (2013) 45(12):1446–51. doi: 10.1038/ng.2823
20. Lefebvre C, Bachelot T, Filleron T, Pedrero M, Campone M, Soria JC, et al. Mutational profile of metastatic breast cancers: A retrospective analysis. PloS Med (2016) 13(12):e1002201. doi: 10.1371/journal.pmed.1002201
21. Chandarlapaty S, Chen D, He W, Sung P, Samoila A, You D, et al. Prevalence of ESR1 mutations in cell-free DNA and outcomes in metastatic breast cancer: A secondary analysis of the BOLERO-2 clinical trial. JAMA Oncol (2016) 2(10):1310–5. doi: 10.1001/jamaoncol.2016.1279
22. Clatot F, Perdrix A, Augusto L, Beaussire L, Delacour J, Calbrix C, et al. Kinetics, prognostic and predictive values of ESR1 circulating mutations in metastatic breast cancer patients progressing on aromatase inhibitor. Oncotarget (2016) 7(46):74448–59. doi: 10.18632/oncotarget.12950
23. Fribbens C, O'Leary B, Kilburn L, Hrebien S, Garcia-Murillas I, Beaney M, et al. Plasma ESR1 mutations and the treatment of estrogen receptor-positive advanced breast cancer. J Clin Oncol (2016) 34(25):2961–8. doi: 10.1200/JCO.2016.67.3061
24. Spoerke JM, Gendreau S, Walter K, Qiu J, Wilson TR, Savage H, et al. Heterogeneity and clinical significance of ESR1 mutations in ER-positive metastatic breast cancer patients receiving fulvestrant. Nat Commun (2016) 7:11579. doi: 10.1038/ncomms11579
25. Bartels S, Christgen M, Luft A, Persing S, Jödecke K, Lehmann U, et al. Estrogen receptor (ESR1) mutation in bone metastases from breast cancer. Mod Pathol (2018) 31(1):56–61. doi: 10.1038/modpathol.2017.95
26. Jeselsohn R, Yelensky R, Buchwalter G, Frampton G, Meric-Bernstam F, Gonzalez-Angulo AM, et al. Emergence of constitutively active estrogen receptor-alpha mutations in pretreated advanced estrogen receptor-positive breast cancer. Clin Cancer Res (2014) 20(7):1757–67. doi: 10.1158/1078-0432.CCR-13-2332
27. Li X, Lu J, Zhang L, Luo Y, Zhao Z, Li M. Clinical implications of monitoring ESR1 mutations by circulating tumor DNA in estrogen receptor positive metastatic breast cancer: A pilot study. Transl Oncol (2020) 13(2):321–8. doi: 10.1016/j.tranon.2019.11.007
28. Niu J, Andres G, Kramer K, Kundranda MN, Alvarez RH, Klimant E, et al. Incidence and clinical significance of ESR1 mutations in heavily pretreated metastatic breast cancer patients. Onco Targets Ther (2015) 8:3323–8. doi: 10.2147/OTT.S92443
29. Takeshita T, Yamamoto Y, Yamamoto-Ibusuki M, Inao T, Sueta A, Fujiwara S, et al. Droplet digital polymerase chain reaction assay for screening of ESR1 mutations in 325 breast cancer specimens. Transl Res (2015) 166(6):540–553 e2. doi: 10.1016/j.trsl.2015.09.003
30. Toy W, Shen Y, Won H, Green B, Sakr RA, Will M, et al. ESR1 ligand-binding domain mutations in hormone-resistant breast cancer. Nat Genet (2013) 45(12):1439–45. doi: 10.1038/ng.2822
31. Toy W, Weir H, Razavi P, Lawson M, Goeppert AU, Mazzola AM, et al. Activating ESR1 mutations differentially affect the efficacy of ER antagonists. Cancer Discovery (2017) 7(3):277–87. doi: 10.1158/2159-8290.CD-15-1523
32. Zundelevich A, Dadiani M, Kahana-Edwin S, Itay A, Sella T, Gadot M, et al. ESR1 mutations are frequent in newly diagnosed metastatic and loco-regional recurrence of endocrine-treated breast cancer and carry worse prognosis. Breast Cancer Res (2020) 22(1):16. doi: 10.1186/s13058-020-1246-5
33. Najim O, Seghers S, Sergoynne L, Van Gaver H, Papadimitriou K, Wouters K, Tjalma W. The association between type of endocrine therapy and development of estrogen receptor-1 mutation(s) in patients with hormone-sensitive advanced breast cancer: A systematic review and meta-analysis of randomized and non-randomized trials. Biochim Biophys Acta Rev Cancer (2019) 1872(2):188315.
34. Dustin D, Gu G, Fuqua SAW. ESR1 mutations in breast cancer. Cancer (2019) 125(21):3714–28. doi: 10.1002/cncr.32345
35. Bidard FC, Callens C, Dalenc F, Pistilli B, De La Motte Rouge T, Clatot F, Bachelot T. Prognostic impact of ESR1 mutations in ER+ HER2-MBC patients prior treated with first line AI and palbociclib: An exploratory analysis of the PADA-1 trial. (2020), 1010–0. doi: 10.1200/JCO.2020.38.15_suppl.1010
36. Bidard F-C, Kaklamani VG, Neven P, Streich G, Montero AJ, Forget F, et al. Elacestrant (oral selective estrogen receptor degrader) versus standard endocrine therapy for estrogen receptor–positive, human epidermal growth factor receptor 2–negative advanced breast cancer: results from the randomized phase III EMERALD trial. J Clin Oncol (2022) 40(28):3246. doi: 10.1200/JCO.22.00338
37. O’Leary B, Hrebien S, Morden JP, Beaney M, Fribbens C, Huang X, et al. Early circulating tumor DNA dynamics and clonal selection with palbociclib and fulvestrant for breast cancer. Nat Commun (2018) 9:896. doi: 10.1038/s41467-018-03215-x
38. Turner NC, Swift C, Kilburn L, Fribbens C, Beaney M, Garcia-Murillas I, et al. ESR1 mutations and overall survival on fulvestrant versus exemestane in advanced hormone receptor–positive breast cancer: a combined analysis of the phase III SoFEA and efect trials. Clin Cancer Res (2020) 26(19):5172–7. doi: 10.1158/1078-0432.CCR-20-0224
39. Goetz MP, Hamilton EP, Campone M, Hurvitz SA, Cortes J, Johnston SR, et al. Acquired genomic alterations in circulating tumor DNA from patients receiving abemaciclib alone or in combination with endocrine therapy. (2020), 3519–9. doi: 10.1200/JCO.2020.38.15_suppl.3519
40. Hanna D, Hutchins J, Yu J, Marino E, Kumar N, Kucharlapati R, et al. Abstract PS18-15: Real-world clinical-genomic data identifies the ESR1 clonal and subclonal circulating tumor DNA (ctDNA) landscape and provides insight into clinical outcomes. Cancer Res (2021) 81(4_Supplement):PS18–15. doi: 10.1158/1538-7445.SABCS20-PS18-15
41. Martin M, Zielinski C, Ruiz-Borrego M, Carrasco E, Turner N, Ciruelos EM, Gil-Gil M. Palbociclib in combination with endocrine therapy versus capecitabine in hormonal receptor-positive, human epidermal growth factor 2-negative, aromatase inhibitor-resistant metastatic breast cancer: A phase III randomised controlled trial—PEARL. Ann Oncol (2021) 32(4):488–99. doi: 10.1016/j.annonc.2020.12.013
42. Aparicio S, Caldas C. The implications of clonal genome evolution for cancer medicine. N Engl J Med (2013) 368(9):842–51. doi: 10.1056/NEJMra1204892
43. Holdhoff M, Schmidt K, Donehower R, Diaz LA. Analysis of circulating tumor DNA to confirm somatic KRAS mutations. J Natl Cancer Inst (2009) 101(18):1284–5. doi: 10.1093/jnci/djp240
44. Diaz LA Jr., Bardelli A. Liquid biopsies: genotyping circulating tumor DNA. J Clin Oncol (2014) 32(6):579–86. doi: 10.1200/JCO.2012.45.2011
45. Murtaza M, Dawson SJ, Tsui DW, Gale D, Forshew T, Piskorz AM, Rosenfeld N. Non-invasive analysis of acquired resistance to cancer therapy by sequencing of plasma DNA. Nature (2013) 497(7447):108–12. doi: 10.1038/nature12065
46. Heitzer E, Ulz P, Geigl JB. Circulating tumor DNA as a liquid biopsy for cancer. Clin Chem (2015) 61(1):112–23. doi: 10.1373/clinchem.2014.222679
47. Diaz LA Jr., Williams RT, Wu J, Kinde I, Hecht JR, Berlin J, Vogelstein B. The molecular evolution of acquired resistance to targeted EGFR blockade in colorectal cancers. Nature (2012) 486(7404):537–40. doi: 10.1038/nature11219
48. Misale S, Yaeger R, Hobor S, Scala E, Janakiraman M, Liska D, et al. Emergence of KRAS mutations and acquired resistance to anti-EGFR therapy in colorectal cancer. Nature (2012) 486(7404):532–6. doi: 10.1038/nature11156
49. Iwahashi N, Sakai K, Noguchi T, Yahata T, Matsukawa H, Toujima S, Ino K. Liquid biopsy-based comprehensive gene mutation profiling for gynecological cancer using CAncer Personalized Profiling by deep Sequencing. Sci Rep (2019) 9(1):10426. doi: 10.1038/s41598-019-47030-w
50. Hudecova I. Digital PCR analysis of circulating nucleic acids. Clin Biochem (2015) 48(15):948–56. doi: 10.1016/j.clinbiochem.2015.03.015
51. Pinheiro LB, Coleman VA, Hindson CM, Herrmann J, Hindson BJ, Bhat S, et al. Evaluation of a droplet digital polymerase chain reaction format for DNA copy number quantification. Anal Chem (2012) 84(2):1003–11. doi: 10.1021/ac202578x
52. Forshew T, Murtaza M, Parkinson C, Gale D, Tsui DW, Kaper F, et al. Noninvasive identification and monitoring of cancer mutations by targeted deep sequencing of plasma DNA. Sci Transl Med (2012) 4(136):136ra68. doi: 10.1126/scitranslmed.3003726
53. Board RE, Wardley AM, Dixon JM, Armstrong AC, Howell S, Renshaw L, et al. Detection of PIK3CA mutations in circulating free DNA in patients with breast cancer. Breast Cancer Res Treat (2010) 120(2):461–7. doi: 10.1007/s10549-010-0747-9
54. Beaver JA, Jelovac D, Balukrishna S, Cochran RL, Croessmann S, Zabransky DJ, et al. Detection of cancer DNA in plasma of patients with early-stage breast cancer. Clin Cancer Res (2014) 20(10):2643–50. doi: 10.1158/1078-0432.CCR-13-2933
55. Ding PN, Becker T, Bray V, Chua W, Ma Y, Xu B, et al. Plasma next generation sequencing and droplet digital PCR-based detection of epidermal growth factor receptor (EGFR) mutations in patients with advanced lung cancer treated with subsequent-line osimertinib. Thorac Cancer (2019) 10(10):1879–84. doi: 10.1111/1759-7714.13154
56. Garcia J, Forestier J, Dusserre E, Wozny AS, Geiguer F, Merle P, et al. Cross-platform comparison for the detection of RAS mutations in cfDNA (ddPCR Biorad detection assay, BEAMing assay, and NGS strategy). Oncotarget (2018) 9(30):21122–31. doi: 10.18632/oncotarget.24950
57. Liao H, Huang W, Pei W, Li H. Detection of ESR1 mutations based on liquid biopsy in estrogen receptor-positive metastatic breast cancer: clinical impacts and prospects. Front Oncol (2020) 10:5876715. doi: 10.3389/fonc.2020.587671
58. Stergiopoulou D, Markou A, Strati A, Zavridou M, Tzanikou E, Mastoraki S, et al. Comprehensive liquid biopsy analysis as a tool for the early detection of minimal residual disease in breast cancer. Sci Rep (2023) 13(1):1258. doi: 10.1038/s41598-022-25400-1
59. Brett JO, Spring LM, Bardia A, Wander SA. ESR1 mutation as an emerging clinical biomarker in metastatic hormone receptor-positive breast cancer. Breast Cancer Res (2021) 23:1–155. doi: 10.1186/s13058-021-01462-3
Keywords: ESR1, liquid biopsy, next-generation sequencing, digital PCR, metastasized breast cancer
Citation: Najim O, Papadimitriou K, Broeckx G, Huizing M and Tjalma W (2023) Validation of liquid biopsy for ESR1-mutation analysis in hormone-sensitive breast cancer: a pooled meta-analysis. Front. Oncol. 13:1221773. doi: 10.3389/fonc.2023.1221773
Received: 12 May 2023; Accepted: 28 July 2023;
Published: 22 August 2023.
Edited by:
Zhi-Gang Zhuang, Shanghai First Maternity and Infant Hospital, ChinaCopyright © 2023 Najim, Papadimitriou, Broeckx, Huizing and Tjalma. This is an open-access article distributed under the terms of the Creative Commons Attribution License (CC BY). The use, distribution or reproduction in other forums is permitted, provided the original author(s) and the copyright owner(s) are credited and that the original publication in this journal is cited, in accordance with accepted academic practice. No use, distribution or reproduction is permitted which does not comply with these terms.
*Correspondence: Omar Najim, omar.najim@azr.be