A review on the chemical constituents and pharmacological efficacies of Lindera aggregata (Sims) Kosterm
- 1College of Pharmaceutical Sciences, Zhejiang University of Technology, Hangzhou, China
- 2School of Public Health, North China University of Science and Technology, Tangshan, China
- 3Ningbo Municipal Hospital of Traditional Chinese Medicine, Affiliated Hospital of Zhejiang Chinese Medical University, Ningbo, China
Lindera aggregata (Sims) Kosterm. (L. aggregata), which belongs to the genus Lindera in the family Lauraceae, is widely distributed in Asia and the temperate, tropical regions of North America. Its roots and leaves have been used for thousands of years as traditional Chinese medicine and/or functional food. To further explore its underlying nutritional value, this review provided a comprehensive insight into chemical constituents and pharmacological effects on L. aggregata. The phytochemical investigation of different parts of L. aggregata led to the identification of up to 349 components belonging to sesquiterpenoids, alkaloids, flavonoids, essential oils, and other compounds. Among them, sesquiterpenoids, flavonoids, and alkaloids are assessed as representative active ingredients of L. aggregata. A wide variety of pharmacological effects of L. aggregata, such as anti-hyperlipidemic, anti-tumor, anti-inflammatory, analgesic, and anti-oxidant, have been proved in vitro and in vivo. In summary, this review aims to provide a scientific basis and reference for further research and utilization of L. aggregata and lay the foundation for developing functional foods with potential active ingredients for the prevention and management of related diseases.
1. Introduction
The genus Lindera is a member of the Lauraceae family, containing approximately 100 species and widely distributed in tropical, subtropical, and temperate zones of Asia and Midwestern America (1). Lindera plants are widely used in traditional medicine, of which Lindera aggregata (Sims) Kosterm. [Lindera strychnifolia (Siebold et Zucc.) Fern.-VIll] is a representative one (2). It is known chiefly as “Wu-Yao,” a folk plant in China, which mainly grows in the eastern, central, southern, and southwestern parts of China, such as Zhejiang, Jiangxi, and Hunan provinces (3). The roots and leaves of L. aggregata are suggested as medicinal and edible parts. More specifically, as an edible plant, the tender leaves of L. aggregata are consumed as a functional tea or dietary supplement for its health-promoting benefits, such as anti-hepatic injury and lipid-lowing activity (4). Also, as an herbal medicine, it has been reported that the roots of L. aggregata (LA-R) could treat the diseases of gastrointestinal tract, metabolism, inflammation, urinary system, etc. LA-R is included in 24 formulae in Chinese Pharmacopoeia (2020ed) (1, 5). In addition, the leaves of L. aggregata (LA-L) are beneficial for treating mastitis, acute cellulitis, carbuncles, and rheumatoid arthritis (6, 7). The health benefits of L. aggregata are mainly attributed to its diverse bioactive constituents, such as sesquiterpenoids, alkaloids, flavonoids, and essential oils. And these bioactive components contribute to its multiple health functions, including hepatoprotective, anti-inflammatory, anti-virus, anti-bacterial, anti-tumor, anti-oxidant efficacies, etc. (8).
Although the chemical composition and biological activity of L. aggregata have been analyzed extensively, existing reviews fail to offer a comprehensive and systematic overview, owing to the complexity of the natural medicinal plants and the advance of novel analytical techniques. A detailed overview of the phytochemistry and medicinal properties was carried out for the whole genus of Lindera plants in 2016 (1). Two reviews on the new progress in phytochemistry and biological activities of L. aggregate has been reviewed in the last 5 years (3, 8), but they were still found incomplete in both the summary of chemical compounds and the in-depth discussion on pharmacological mechanism. With the increasing focus on L. aggregata, a systematic review is urgently needed on the recent progress in the discovery of components, together with pharmacological investigations in different parts of L. aggregate, aiming to inspire the interest of phytochemists and promote the development and utilization of this valuable plant for various remedies.
2. Chemical compounds
Many studies have been conducted to explore the phytoconstituents from L. aggregata. Up to now, 349 compounds have been isolated and identified from L. aggregata. Based on the chemical structures, these compounds can be divided into six groups: 127 sesquiterpenoids (including dimeric and trimeric sesquiterpenoids), 37 alkaloids (including amides), 32 flavonoids, 35 other components, and 118 essential oils (except those sesquiterpenoids already mentioned before). The information about different types of compounds is summarized below in Figure 1.
2.1. Sesquiterpenoids
Sesquiterpenoids possess notable biological activities and are the primary active ingredients in L. aggregata. They are constructed by three isoprene units (15 carbon atoms) with chains, rings, and other diverse skeletons of structures. To date, 127 sesquiterpenoids have been reported, mostly isolated from roots and a few obtained from whole plants and leaves. As shown in Table 1 and Figure 2, modern phytochemistry studies reveal that the sesquiterpenoids isolated from L. aggregata are mainly featured as monomer-, dimer-, and hybrid forms. The sesquiterpenoid monomers include eudesmane- (1–22), lindenane- (23–52), germacrane- (53–69), elemane- (70–73), guaiane- (74–76), cadinane- (77–78), copane- (79), eremophilane- (80), and other types (81–84) (9–27). So far, the sesquiterpenes of L. aggregata account for more than half of this kind of compounds in the whole genus Lindera, especially the eudesmane-, lindenane-, and germacrane-types, which were almost all found in L. aggregata (1). Thereinto, linderagalactone A (84) is a halogenated sesquiterpene lactone possessing a unique rearranged carbon skeleton (13). Besides that, eudesmanes exhibit liver protection (3, 8) (13), anti-cancer (18, 19) (15), anti-fibrotic (1, 14) (9), and anti-inflammatory properties (11, 12) (14); lindenanes exhibit anti-inflammatory properties (36, 37) and anti-oxidant activity (23) (20, 27); germacranes (53, 54) (13) exhibit liver protection activity. Furthermore, in elemanes, the representative compound isolinderalactone (ILL) (70) has significant anti-tumor activity (28–31). And compound 83 shows anti-inflammatory properties (16).
Sesquiterpene dimers are a characteristic class of constituents with C30 cores in L. aggregata, which are plausibly biosynthesized via the coupling of two identical or different sesquiterpenoid molecules (32). Forty-three dimers have been reported, among which lindenane-type sesquiterpenoid dimers are the most representative structures, such as linderaggrenolides A–N (88–101) and linderanoids H–O (109–116), where linderaggrenolides A–N have an oxygen bridge (32, 33). The remaining dimeric sesquiterpene components, such as linderanoids A–G (102–108), include 3 sesquiterpenoid dimers comprising a lindenane and a noreudesmane unit (102–104); 2 dimers consisting of a lindenane and an eudesmane unit (105–106), and 2 dimers containing a lindenane and an elemanolide unit (107–108) (33). Furthermore, 6 oligomeric sesquiterpenoids, aggreganoids A–F (118–123), were isolated from LA-R. Aggreganoid A and B (118–119) are 2 previously undiscovered methine- or methylene-bridged sesquiterpenoid trimers with a unique C46 skeleton. At the same time, aggreganoids C–F (120–123) are the first examples of carbon-bridged disesquiterpenoids with a C33 or C31 skeleton discovered in the plant kingdom (34). Linderalides A–C (124–126) are characterized by the unique disesquiterpenoid-geranylbenzofuranone hybrids directly linked by two C–C bonds. Linderalide D (127) possesses an unprecedented carbon skeleton with an unusual linearly 6/6/5/6/6 pentacyclic ring system fused by a sesquiterpenoid unit and a geranylbenzofuranone moiety (35). In addition, compound 86 shows moderate anti-coagulant activity, and compounds 95, 96, and 106 are excellent inhibitors against transforming growth factor-β (TGF-β) (19, 32, 33).
2.2. Alkaloids
Alkaloids are a massive group of naturally occurring organic compounds that contain one or more nitrogen atoms (amino or amide in some cases) in their structures. They are one of the active ingredients in L. aggregata, although the total content is not high (about 0.3%) (24). LA-R extract is the primary natural source of alkaloids. Thirty-seven alkaloids (1–37) have been successfully identified from L. aggregata, including 15 aporphine alkaloids (1–15), 8 benzyl tetrahydroisoquinoline alkaloids (16–23), 2 morphinan alkaloids (24, 25), 5 bis-benzyl tetrahydroisoquinoline alkaloids (26–30), a β-carboline alkaloid (31) and 6 amides (32–37) (13, 16, 24, 36–43). Their structures and molecular formulae are shown in Table 2 and Figure 3. Of these, isoquinoline alkaloids (1–30) account for a large proportion of the total alkaloids, and they have strong biological activities, such as anti-inflammatory (1–3, 12) (16, 37) and anti-cancer (5, 30) (37). Among them, argemexirine (20) is the first time to be isolated from Lauraceae (41). Apart from this, the only β-carboline alkaloid (31) found in LA-R displays significant potential against the superoxide anion generation (43).
2.3. Flavonoids
Flavonoids are one of the significant dietary polyphenols, mainly found in LA-L. Approximately 32 flavonoids and their glycosides have been isolated and identified (seen in Table 3 and Figure 3). The structural types include 27 flavonols (1–27), 3 flavanones (28–30), a flavone (31), and a dihydrochalcone (32) (4, 7, 18, 44–48). Among these, quercetin-3-O-β-D-glucoside (4), quercetin-3-O-β-D-arabinofuranoside (5), quercetin-3-O-α-L-rhamnopyranoside (QI) (6), quercetin-5-O-β-D-glucoside (9), kaempferol-7-O-α-L-rhamnopyranoside (24) were isolated and prepared by high-speed countercurrent chromatography (47). Furthermore, the total flavonoids extracted from LA-L have good antioxidant activity (49), and especially, QI (6) is an excellent antioxidant (50).
2.4. Others
The current investigation on the phytochemicals obtained from L. aggregata is scarce, where 12 tannin-type components have been reported, including (+)-catechin (1), (−)-epigallocatechin (2), (−)-epicatechin (3), and diploid epicatechin-(4β-8, 2-O-7)-epicatechin (4), epicatechin-(4β-8, 2-O-7)-catechin (5), epicatechin-(4β-8)-catechin (6), procyanidin B1 (7) procyanidin B2 (8), and triploid aesculitannin B (9), cinnamtanin B1 (10), lindetannin trimer (12), and tetraploid Cinnamtannin B2 (11). In addition, procyanidin B2 (8) and aesculitannin B (9) are separated from the roots (48, 51–53). Additionally, epicatechin (3) and aesculitannin B (9) are non-competitive inhibitors against prolyl endopeptidase from Flavobacterium meningosepticum, and these three compounds (7, 10–11) have inhibitory activities against HIV-1 integrase (52, 53).
In addition to the above-mentioned extensive components in L. aggregata, there are also several other rare components, including 2 benzenoids (13–14), a benzene-type glycoside (15), 3 lindera cyclopentenedione derivatives (16–18), 3 bi-linderone derivatives (19, 20a, 20b), a butenolide (21), 3 lignans (22–24), 10 phenolics (25–34) and a linderaspirone (35) (16, 39, 54, 55). They also demonstrate good pharmacological activities, such as compound 21 has anti-tumor activity, and compounds 19 and 35 show good anti-diabetic properties due to their significant insulin resistance alleviation (55–57). The isolated phytochemicals are tabulated in Table 4 and Figure 4.
2.5. Essential oils
Essential oils are found in different parts of L. aggregata, including leaves, seeds, peels, tubers, and taproots. The composition of essential oils from L. aggregata is very complex, mainly composed of monoterpenes and sesquiterpenes, which usually exist in the form of oxygen-containing derivatives (alcohols, ketones, and lactones) and hydrocarbons, and GC-MS analysis is generally used to identify various essential oils from L. aggregata (58). Due to the distinctive bioactivities of sesquiterpenes, it has been listed separately in Section “2.1. Sesquiterpenoids” for a more explicit demonstration. They have important physiological and biological activities, such as anti-bacterial and anti-cancer activities (59, 60). Meanwhile, researchers have shown that the main components and contents of the essential oils from LA-R and LA-L are obviously different. The major compounds of the leaf oil are sesquithuriferol (35.90%), 14-oxy-α-muurolene (16.45%), etc. In comparison, the root oil is rich in zerumbone (26.66%), geranyl acetate (12.45%), (E)-β-ocimene (10.27%) (60). Due to different geographic origins, growing years, and/or harvest times, another research report showed that the main components of leaf oil were curzerene (12.60%), 1,4-diethyl-benzene (11.01%), and 2-methyl-6-(2-propenyl)-phenol (10.25%), while roots oil was rich in linderene (39.44%) and lindenenol (20.93%) (61). The phytochemical research on different parts of L. aggregata further laid the foundation for developing and utilizing its whole plant resources.
3. Pharmacology
As a traditional medicine and edible plant, L. aggregata has been reported with multiple pharmacological activities and health functions based on in vitro and in vivo studies, including anti-hyperlipidemic, anti-oxidant, anti-tumor, anti-inflammatory, hepatoprotective, deworming, etc. Reports on the pharmacological activity of L. aggregata mainly focus on its crude extracts, while reports on active compounds mainly focus on flavonoids, alkaloids, sesquiterpenes, and essential oils. In the following parts, the primary pharmacological activities, health functions, and related molecular mechanisms of the crude extracts of L. aggregata and its bioactive compounds are summarized and discussed in detail, as illustrated in Figure 5 and Table 5.
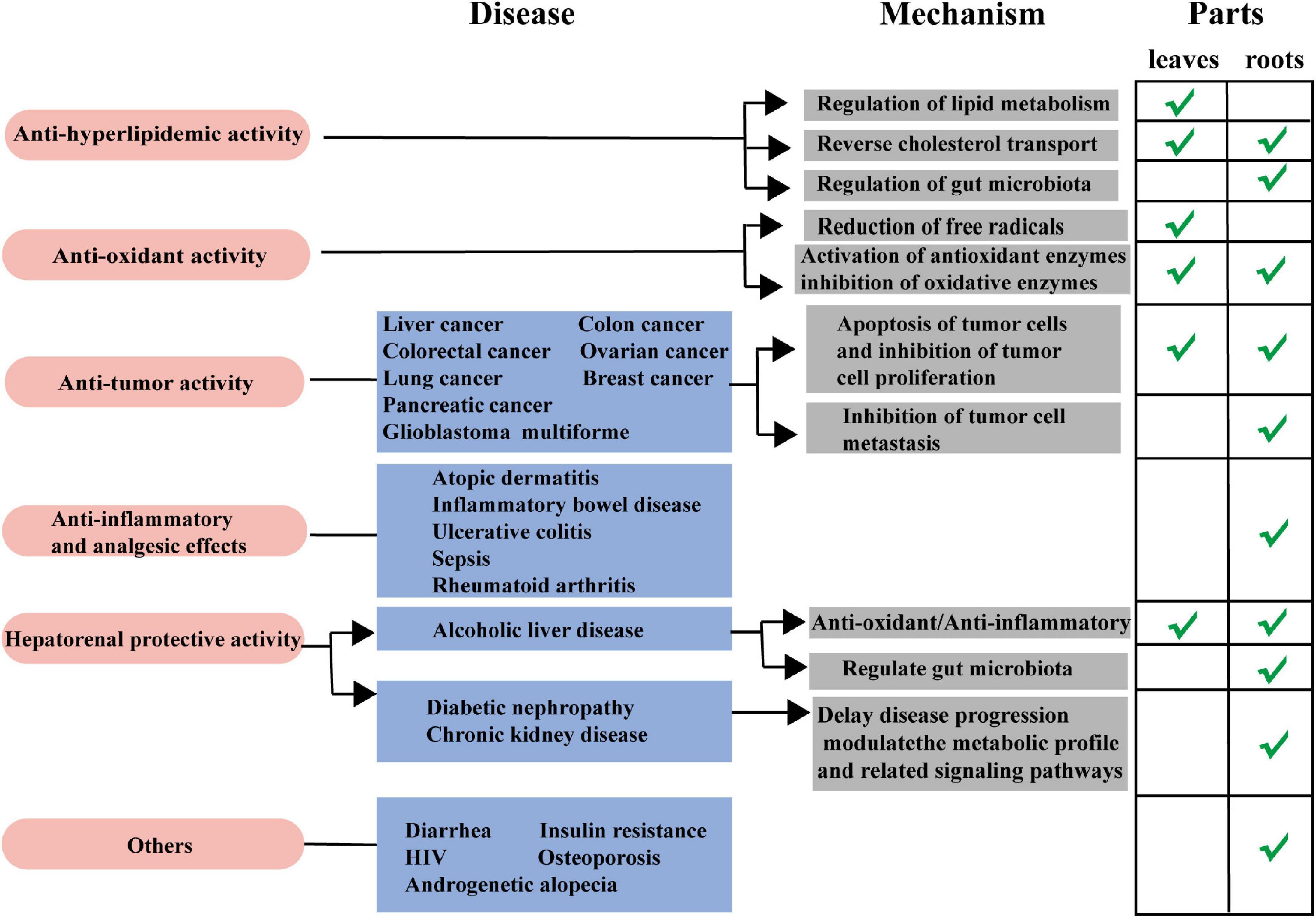
Figure 5. Schematic diagram of pharmacological activities from the roots and leaves of L. aggregata.
3.1. Anti-hyperlipidemic activity
Hyperlipidemia (HLP) is a common metabolic disorder, one of the principal positive risk factors for the development and progression of atherosclerosis and cardiovascular disease, caused by abnormal lipid metabolism or transport, and high levels of total cholesterol (TC), triglycerides (TG), low-density lipoproteins cholesterol (LDL-C), and high-density lipoprotein cholesterol (HDL-C). LA-R and LA-L have been proven to have good hypolipidemic effects (62). The hypolipidemic effects of L. aggregata are mediated by various mechanisms, including regulation of lipid metabolism, reverse cholesterol transport, and regulation of gut microbiota (Figure 6).
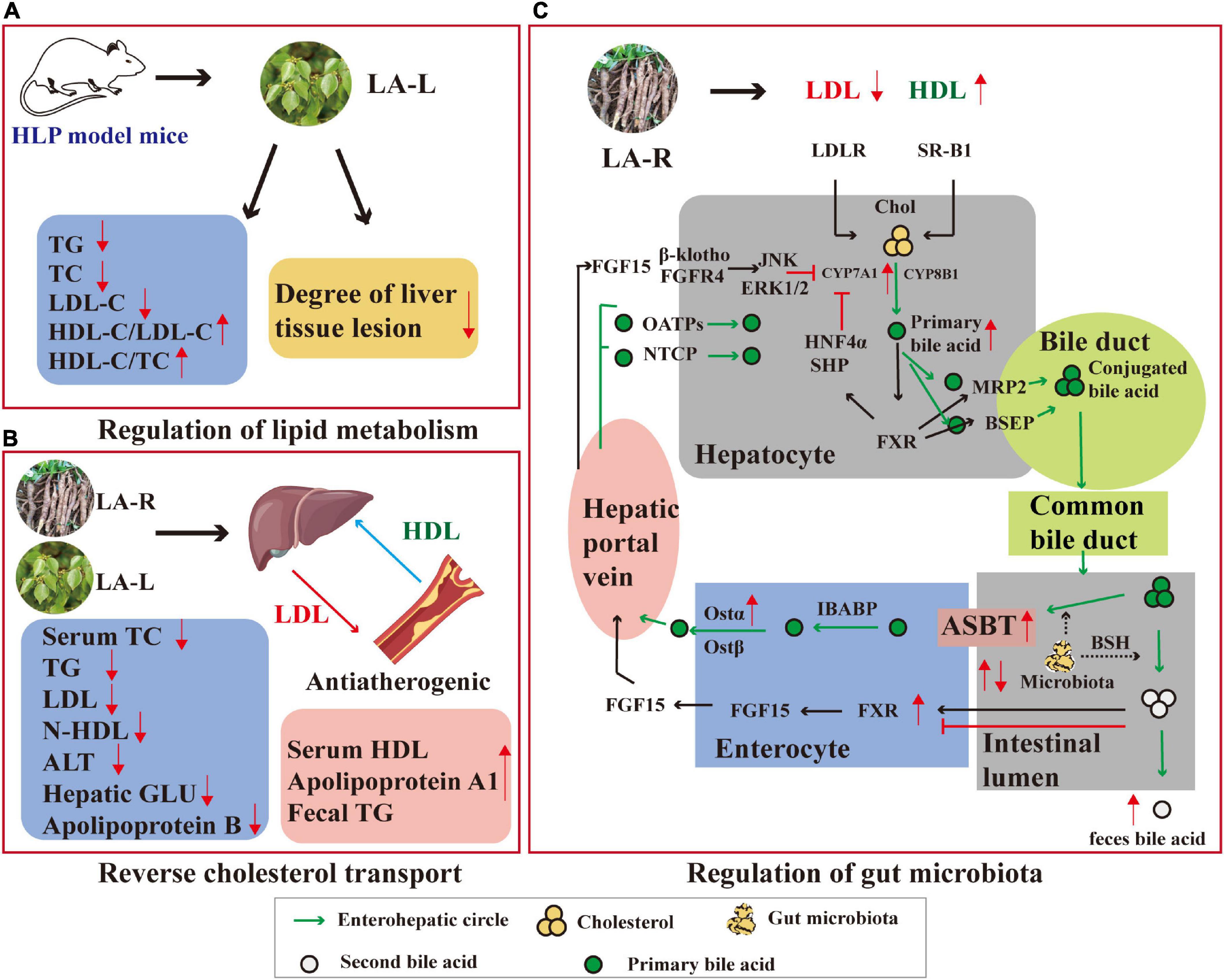
Figure 6. Anti-hyperlipidemic mechanism of action of L. aggregata. (A) Regulation of lipid metabolism. (B) Reverse cholesterol transport. (C) Regulation of gut microbiota. CYP8B1, Cytochrome P450, family 8, subfamily B, polypeptide 1; ERK, extracellular regulated protein kinases; FGF, recombinant fibroblast growth factor; β-klotho, a single-transmembrane receptor; OATPs and NTCP, drug transporters; MRP2 and BSEP, bile acid transporters; FXR, farnesoid X receptor; ASBT, apical sodium-dependent bile acid transporter; OST, organic solute transporter; BSH, bile salt hydrolase; HNF4α, hepatocyte nuclear factor 4α; SHP, small heterodimer partner.
3.1.1. Regulation of lipid metabolism
The crude extract of LA-L has demonstrated a specific lipid-lowering effect to improve HLP by promoting lipid metabolism. The aqueous extract of LA-L was used in HLP model mice, and blood lipids and histomorphology regulation were observed. The results showed that the aqueous extract of LA-L reduced TG, TC, and LDL-C levels while increasing HDL-C/LDL-C and HDL-C/TC compared with the control group. In the meantime, it relieved liver injury, cell swelling, degeneration, and other lesions in HLP model mice (63). In addition, further by observing the degree of lipid accumulation and detecting the expression of liver kinase B1 (LKB1)-adenosine 5′-monophosphate-activated protein kinase (AMPK) pathway-related proteins in HLP model mice liver, it was found that the possible action mechanism of promoting lipid metabolisms is the activation of AMPKα protein phosphorylation (64).
3.1.2. Reverse cholesterol transport
Reverse cholesterol transport describes HDL’s metabolism and a crucial antiatherogenic function (65). The aqueous extract of LA-L was probed using hypercholesterolemic (HCL) mice for anti-hyperlipidemic effects and potential mechanisms. The outcomes presented that the aqueous extract of LA-L (0.3, 0.6, and 1.2 g/kg) significantly lowered serum TC, TG, LDL, non-HDL, alanine aminotransferase (ALT), hepatic lipid/glucose (GLU), apolipoprotein B, hepatic GLU, and increased serum HDL, apolipoprotein A1, and fecal TG levels in HCL mice. The potential cholesterol-lowering mechanism may involve inhibiting cholesterol synthesis by down-regulation of 3-hydroxy-3-methylglutaryl CoA reductase (HMGCR) and promoting cholesterol transport by up-regulation of cholesterol 7-α-hydroxylase (CYP7A1) and ATP-binding cassette transporter A1 (ABCA1) (66).
Besides, the ethanol extract of LA-R also encourages the conversion of cholesterol to the liver and bile acids, which may be associated with reverse cholesterol transport regulation to restore the abnormalities of bile acid metabolism caused by HLP (67).
3.1.3. Regulation of gut microbiota
According to recent research, HLP is closely related to gut microbiota and high-fat diet (68). Research has shown that the ethanol extract of LA-R improved gut microbiota disturbance caused by a high-fat diet via increasing intestinal microbiota diversity and changing the abundance of the firmicutes, bacteroidetes, and actinobacteria. In addition, the ethanol extract of LA-R can increase bile acid reabsorption and promote fecal excretion through farnesoid X receptor, apical sodium-dependent bile acid transporter, organic solute transporter α, and CYP7A1 thus restoring abnormal bile acid metabolism caused by HLP (69).
3.2. Anti-oxidant activity
Oxidative stress is reflected in various diseases, including cancer, cardiovascular diseases, neurodegenerative diseases, diabetes, ischemia/reperfusion injuries, rheumatoid arthritis, and even the process of aging. So it is increasingly important to explore natural anti-oxidants that can mop up reactive oxygen species that trigger the development of chronic diseases (70).
3.2.1. Reduction of free radicals
Lindera aggregata is a good source of natural antioxidants. As an edible plant, LA-L is consumed as a functional tea in Japan (Xufu Tea). The study showed that the generation of free radicals was significantly reduced during the oxidative denaturation of LDL after young and healthy men drank the tea for a week, which confirms the significant anti-oxidant activity of Xufu Tea in a healthy human environment (71).
3.2.2. Activation of antioxidant enzymes and inhibition of oxidative enzymes
Flavonoids are the main bioactive ingredients responsible for the anti-oxidant effect of L. aggregata. It is noteworthy that flavonoids are rich in LA-L. In mice model with CCl4-induced acute liver injury, LA-L-flavonoids significantly decreased the ALT and aspartate aminotransferase (AST) activities and malondialdehyde (MDA) content while also significantly increasing the activities of superoxide dismutase (SOD) and total anti-oxidation capacity in serum at the concentrations of 50–200 mg/kg. Furthermore, the mRNA expression of thioredoxin, heme oxygenase (HO)-1, and peroxiredoxin-1 in liver tissues was also increased by LA-L-flavonoids (49).
QI (compound 6 in Table 3) is the most representative flavonoid typically extracted from the leaves and has excellent development and utilization value. The anti-oxidant activity of QI and its underlying molecular mechanism in human umbilical vein endothelial cells (HUVEcs) were investigated using hydrogen peroxide-induced HUVEcs and the aging rat model (Figure 7). QI could induce major cellular anti-oxidant enzymes to trigger autophagy by activating nuclear factor-E2-related factor 2 (Nrf2) activation and Nrf2-dependent, thus effectively attenuating H2O2-induced oxidative stress in HUVEcs (50, 72).
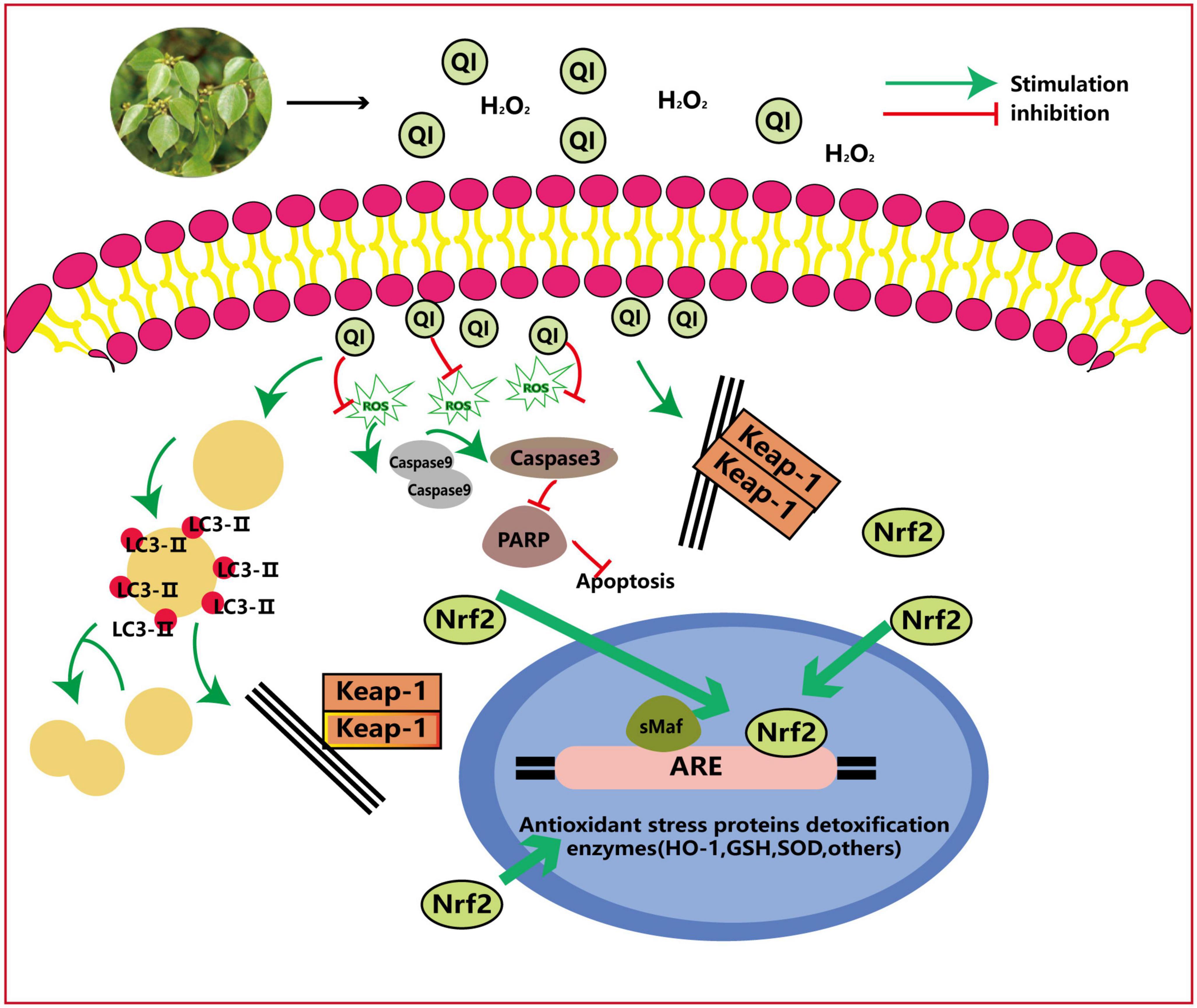
Figure 7. Anti-oxidant mechanism of action of QI: QI induces major cellular anti-oxidant enzymes to trigger autophagy via Nrf2 activation and Nrf2-dependent and effectively attenuates H2O2-induced oxidative stress in HUVEcs. ROS, reactive oxygen species; Lc, light chain; Keap1, Kelch-like EcH-associated protein 1; PARP, poly (AdP-ribose) polymerase; ARE, antioxidant response element; GSH, glutathione.
In addition to anti-oxidant activity in LA-L, another study reported that lindenenyl acetate (compound 23 in Table 1) isolated from the LA-R effectively prevented glutamate-induced oxidative damage. HO enzymes are essential components of the cellular anti-oxidant system, and Nrf2 can induce the expression of HO-1 and glutathione. In the mouse hippocampal HT22 cell line, when the concentration set from 10 to 40 μM, lindenenyl acetate dose-dependently increased HO-1 expression; when the concentration reached 20 μM, lindenenyl acetate dose-dependently increased HO activity. In addition, lindenenyl acetate resulted in nuclear aggregation of Nrf2 and increased the promoter activity of anti-oxidant response elements in the HT22 cell line (27).
3.3. Anti-tumor activity
The anti-tumor activity effects of extracts of L. aggregata have been widely investigated through a series of in vivo and in vitro experiments. Studies have uncovered that the fractions and ingredients isolated from LA-R and LA-L exerted wide-spectrum anti-tumor activity against liver cancer, colon cancer, lung cancer, colorectal cancer (CRC), ovarian cancer (OC), breast cancer, pancreatic cancer, and glioblastoma multiforme. Its anti-tumor action is generally attributed to suppressing tumor cell growth, affecting tumor cell apoptosis, autophagy, migration, and invasion processes (30).
3.3.1. Apoptosis of tumor cells and inhibition of tumor cell proliferation
Different crude extracts of L. aggregata have been shown to have good anti-tumor activity. It is reported that aqueous extract of LA-R specifically inhibited the growth of lung cancer cell lines A549 (IC50: 250 μg/mL) and SBC-3 (IC50: 100 μg/mL). In addition, the extract with 5 mg/kg significantly suppressed the proliferation of the growth of lung cancer cells transplanted in C57BL/6 and BALB/c nude mice after 2 months of treatment (73). Essential oils from the LA-L showed cytotoxic activity against three cancer cell lines, A549, HeLa, and the human hepatocellular carcinomas (HepG2) cell line in vitro (IC50: 22–24 μg/mL) (60).
The sesquiterpenoids of LA-R have been proven to possess good anti-tumor activity in vitro and in vivo. Among them, ILL (70 in Table 1) is the most representative, and it acts on different cancers through different pathways (Figure 8): it can induce apoptosis of human OC cells by increasing the production of mitochondrial superoxide, decreasing the expression of mitochondrial SOD2, and interfering with the signal transducer and activator of transcription 3 (STAT3)-mediated signaling pathway (29). In addition, ILL induced apoptosis of triple-negative breast cancer cells via suppressing STAT3 signaling pathway by regulation of suppressor of cytokine signaling 3 and micro-RNA 30c (28). And the expression levels of X-linked inhibitors of apoptosis and survivin in glioma cells were suppressed after treatment with ILL (30). It also demonstrated anti-tumor activity on CRC cells by inhibiting human CRC cell proliferation, inducing endoplasmic reticulum stress, modulating the G2/M phase of cell cycle progression, and inducing reactive oxygen species-mediated apoptosis through c-Jun N-terminal kinase (JNK)/p38 mitogen-activated protein kinases (MAPK) (31).
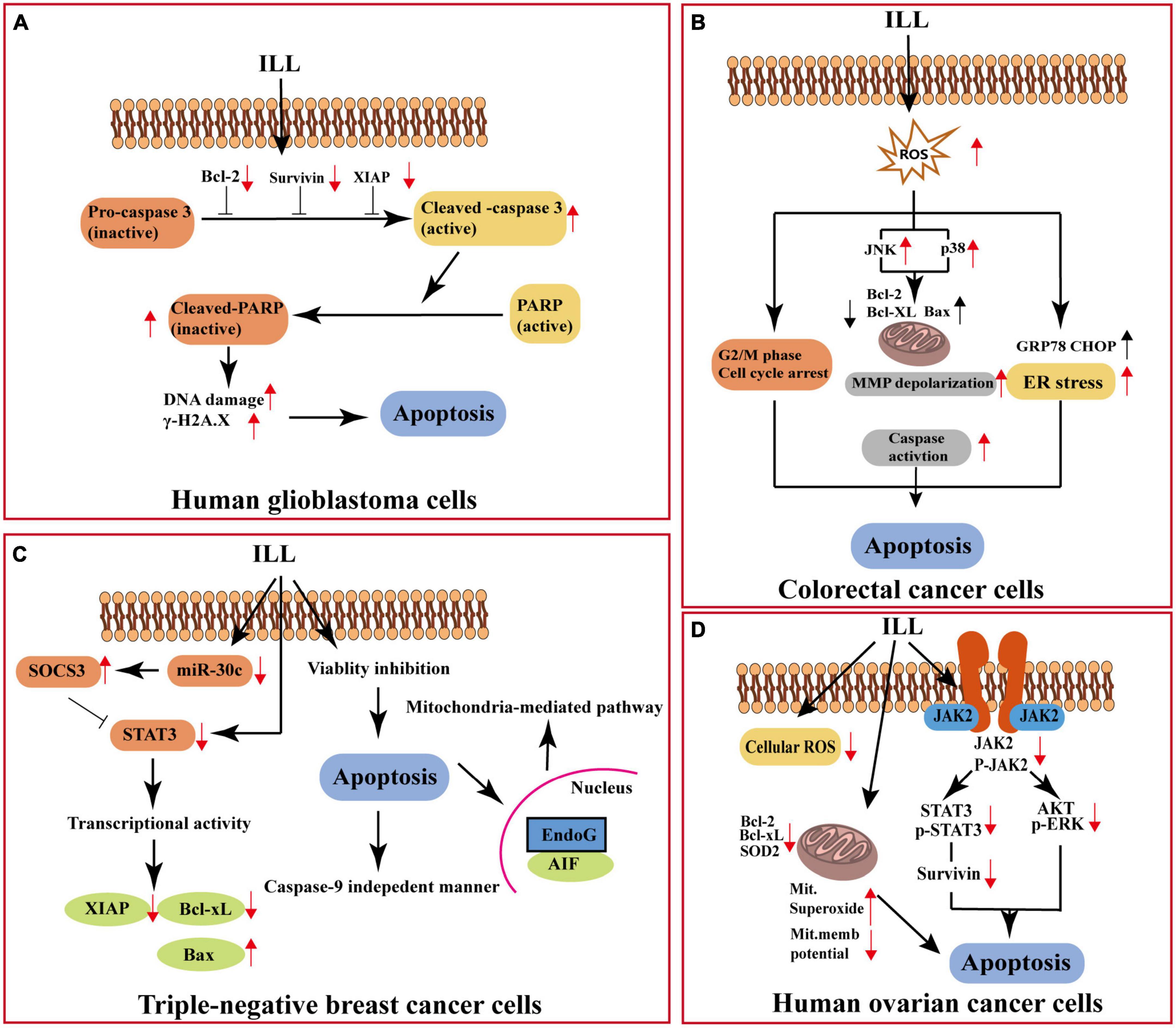
Figure 8. Anti-cancer mechanism of action of ILL. (A) Apoptosis of human glioblastoma cells. (B) Apoptosis of colorectal cancer cells. (C) Apoptosis of triple-negative breast cancer cells. (D) Apoptosis of human ovarian cancer cells. XIAP, X-linked inhibitor of apoptosis protein; PARP, poly (AdP-ribose) polymerase; ROS, reactive oxygen species; MMP, mitochondrial membrane potential; GRP78, 78-KDa glucose-regulated protein; CHOP, C/EBP homologous protein; SOCS3, cytokine signaling 3; miR-30c, microRNA hsa-miR30c-5p; AIF, apoptosis-inducing factor; EndoG, endonuclease G; JAK2, Janus kinase 2; ERK, extracellular regulated protein kinases.
Other sesquiterpenoids from LA-R also have anti-tumor activity, such as 3-oxo-5aH,8bH-eudesma1,4(15),7(11)-trien-8,12-olide (compound 18 in Table 1), 3-oxo-4,5aH,8bH-eudesma-1,7(11)-dien-8,12-olide (compound 19 in Table 1) which showed potent cytotoxicity against human small cell lung cancer SBC-3 (IC50 values of 7.2, 32.2 μM), compared with positive control cisplatin (IC50 value of 8.6 μM) (15). Moreover, it was verified by in vitro and in vivo experiments that linderalactone (compound 53 in Table 1) inhibited the development of pancreatic cancer via negatively regulating the phosphatidylinositol 3 kinase (PI3K)/protein kinase B (AKT) signaling pathway (74). In another study by Yan et al. (75), the essential oils component germacrone showed obvious cytotoxicity to the proliferation of the seven human cancer cell lines tested, especially the inhibitory effect on the proliferation of human esophageal carcinoma Eca-109 and human gastric cancer SGC-7901 cell lines (IC50 = 24.8 μg/mL). Secoaggregatalactone A (compound 21 in Table 4), a seco butanolide from LA-L, exhibited noticeable cytotoxicity (EC50 of 6.61 μg/mL; 22.1 μM) against the HepG2 cell line (55). Furthermore, costaricine (compound 30 in Table 2) and laurolitsine (compound 5 in Table 2) from LA-R showed cytotoxic activities on the human colon carcinoma cell line (HCT-116), with IC50 values of 51.4 and 27.1 μM, respectively (37).
3.3.2. Inhibition of tumor cell metastasis
ILL is a potential therapeutic adjuvant of A549 lung cancer cells and can inhibit the invasion and migration of A549 cancer cells. The author suggests that the possible mechanisms involve the inhibition of matrix metalloproteinase-2 and β-catenin protein expression resulting from the up-regulation of NME/NM23 nucleoside diphosphate kinase 1 expression (76).
3.4. Anti-inflammatory and analgesic effects
Lindera aggregata also exhibits anti-inflammatory properties, showing protective effects against inflammation-related diseases, such as rheumatoid arthritis, atopic dermatitis, inflammatory bowel disease, sepsis, and ulcerative colitis.
For the crude extract, the ethanol extract of LA-R diminished the production and secretion of interleukin (IL)-6, regulated IL-6/STAT3 signal transduction, and modulated the balance of T helper (Th) 17 and Treg cells to attenuate ulcerative colitis (77). The total alkaloids of LA-R have good analgesic and anti-inflammatory effects by observing the number of hind feet licking on a hot plate and times of twisting body in mice induced by acetic acid (78, 79). Using a model of collagen II (CII)-induced arthritis (CIA) rats, the study revealed that the total alkaloids of LA-R at 50, 100, and 200 mg/kg alleviated disease severity in a dose-dependent manner. Assessed by its effect on CII-induced ear swelling in mice, it also decreased serum levels of IgG anti-CII and inhibited delayed-type hypersensitivity at 100 and 200 mg/kg (80). The anti-inflammatory mechanism of the total alkaloids of LA-R may be through downregulating the functions of T lymphocytes and macrophages and nuclear factor kappa-B (NF-κB) and MAPKs signaling pathways (81).
Multiple studies have confirmed that the isoquinoline alkaloid norisoboldine (NOR) (compound 12 in Table 2) from LA-R is the most representative active compound and possesses outstanding anti-arthritis activity (82). In CIA rats, oral NOR (10, 20, and 40 mg/kg) significantly decreased the swelling of paws, arthritis index scores and elevated the lowered body weights of rats. It prevented the infiltration of inflammatory cells and the destruction of bone and cartilage in joints (83). NOR prevented the release of IL-6 from fibroblast-like synoviocytes (FLS), which may be related to the inhibition of protein kinase C (PKC)/MAPKs/NF-κB-p65/cAMP-response element binding protein (CREB) pathway (84). Another study by Wei et al. (85) showed that NOR was demonstrated to block osteoclast differentiation and function in the early stages of the TRAF6-TAK1 (a MAPK kinase) complex and to inhibit the resorptive function of osteoclasts by downregulating the expression of the bone matrix-degrading enzymes (cathepsin K and matrix metallopeptidase 9). The anti-arthritic mechanism of NOR may involve the inhibition of inflammatory synovial hyperplasia by promoting the release of cytochrome C and regulating the expression of B-cell lymphoma-2 (Bcl-2) and Bcl2-Associated X (Bax) proteins. Besides, some studies have shown that the inhibition of synovial angiogenesis and endothelial cell migration are also the contributed to anti-arthritic effect (86–88). NOR could also suppress osteoclast differentiation in rheumatoid arthritis and consequent joint bone impairment in an aryl hydrocarbon receptor-dependent manner (89). It can work by restoring systemic Th17/Treg balance via the induction of intestinal Treg cell generation and the migration of these cells to inflamed joints and synovium (90, 91).
Besides anti-arthritis activity, NOR has other activities. For example, NOR could reduce 2,4-dinitrofluorobenzene-induced dermatitis in mice by inhibiting nuclear factor of activated T-cells (NFAT) (92). It regulates the polarization of macrophages through the M2 pyruvate kinase (PKM2)/hypoxia-inducible factor (HIF-1α)/peroxisome proliferator activated receptor-γ co-activator 1-α (PGC-1α) pathway, thus alleviating sepsis-induced acute lung injury (93). It can also inhibit the activation of inflammatory bodies of protein 3 associated with nod-like receptor hot protein domain to weaken the colitis induced by 2,4,6-trinitrobenzene sulfonic acid in mice. Moreover, it ameliorated ulcerative colitis and alleviated the development of colitis in mice induced by dextran sodium sulfate by promoting the differentiation of Treg cells (94–96).
Other types of alkaloids and sesquiterpenoids also have good anti-inflammatory activity. For example, linderaggrine A (compound 31 in Table 2) (β-carboline alkaloid) showed significant anti-inflammatory activity in superoxide anion generation with an IC50 value of 9.17 ± 0.40 μM as compared to positive control sorafenib (IC50 = 3.23 ± 0.42 μM) (43). The sesquiterpenoid linderaggredin C (compound 83 in Table 1) and the alkaloids (+)-N-methyllaurotetanine (compound 2 in Table 2) and (+)-isoboldine (compound 3 in Table 2) were isolated from the whole plant of L. aggregata, displaying the significant inhibition of the generation of superoxide anion in human neutrophils with IC50 values of 7.45 ± 0.74, 8.36 ± 0.11, and 5.81 ± 0.59 μM, respectively (16). Inhibition of overstimulated inflammatory cytokines and NO is a potential therapeutic target for inflammatory disease. Linderolide O (compound 36 in Table 1), linderolide P (compound 37 in Table 1), NOR, and boldine (compound 1 in Table 2) inhibited lipopolysaccharide (LPS)-stimulated nitric oxide production in murine RAW 264.7 macrophage cells, with IC50 values of 6.3 and 9.6 37.8, and 38.7 μM, respectively (20, 37).
3.5. Hepatorenal protective activity
Liver and kidney injuries are common pathological processes. Liver injury can result in fatty liver, cirrhosis, fibrosis, and even cancer, while kidney injury can lead to arterial hypertension, proteinuria, hematuria, edema, etc. (97–99). According to records, L. aggregata is an excellent natural plant for protecting the liver and kidney. Numerous studies have investigated the hepatorenal protective effects of L. aggregata on alcoholic liver disease (ALD), diabetic nephropathy, and chronic kidney disease.
3.5.1. Liver protection through anti-oxidants and anti-inflammatory activities
The LA-R extract may improve ALD through anti-oxidation and anti-inflammatory. Its experimental result includes improved histopathological status and reduced serum ALT, AST, TG, TC, and MDA levels; decreased MDA and inflammatory mediators, including NF-κB, tumor necrosis factor-α (TNF-α), and IL-1β in liver tissues; decreased ethanol treatment-induced overexpression of cytochrome P450 2E1 mRNA (100), as displayed in Figure 9.
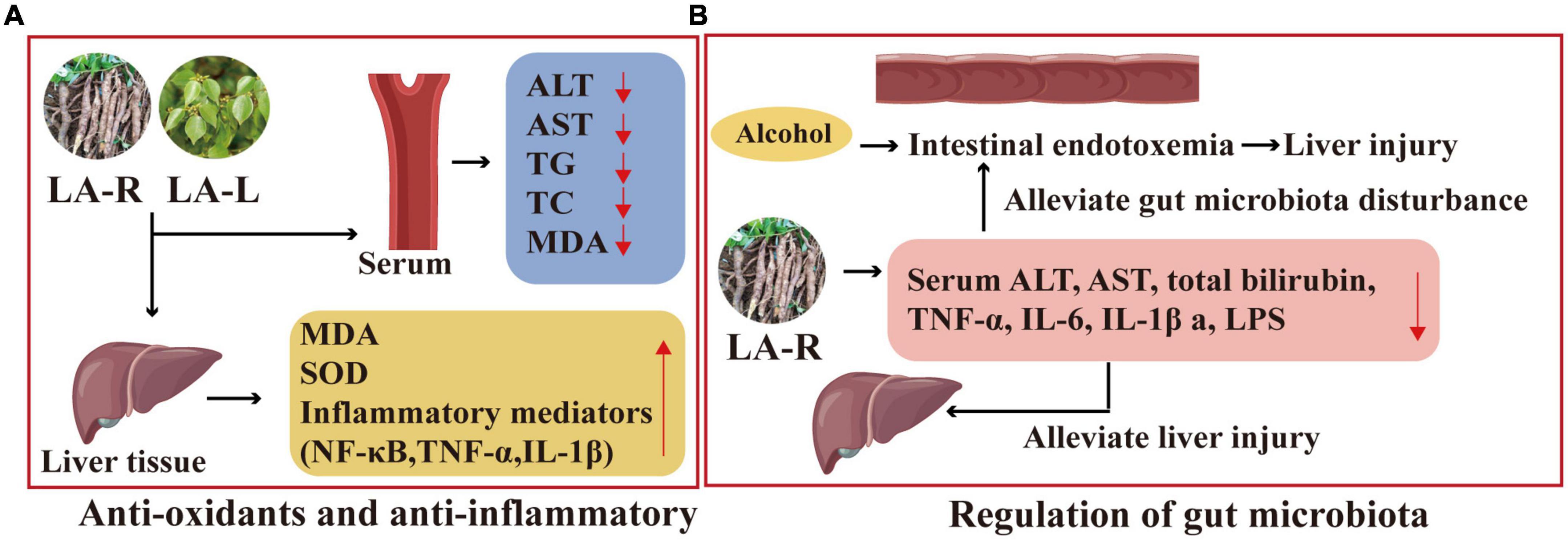
Figure 9. Liver protection mechanism of action of L. aggregata. (A) Anti-oxidants and anti-inflammatory. (B) Regulation of gut microbiota.
LA-L-flavonoids (50–200 mg/kg) remarkably decreased ALT and AST activities and MDA concentration, and increased SOD activity and total anti-oxidation capacity in the serum of the mice model of CCl4-induced acute liver injury (49).
Linderagalactone E (compound 8 in Table 1), linderane (compound 54 in Table 1), hydroxylindestenolide (compound 3 in Table 1), and linderalactone from LA-R (EC50 values of 67.5, 167.0, 42.4, and 98.0 μM) have shown hepatoprotective activity against H2O2-induced oxidative damages on HepG2 cells. The mechanisms may be related to anti-oxidative stress as well as inhibiting the cytochrome P450 2E1 mRNA expression in rat liver (13).
3.5.2. Liver protection through regulating gut microbiota
The prebiotic effect is essential for LA-R to attenuate the disturbance of gut microbiota in liver disease. Since intestinal permeability and intestinal endotoxemia caused by excessive alcohol consumption are the key pathogenic factors in the occurrence of ALD, improving intestinal function to relieve intestinal endotoxemia will be an effective method for the treatment of ALD (101).
Studies have shown that the ethanol extract of LA-R has a protective effect on the intestinal barrier and can alleviate gut microbiota disturbance, thereby reducing intestinal endotoxemia associated with alcoholic liver injury (102). Furthermore, compared with the ALD mouse model group, the 4 g/kg LA-R group inhibited alcohol-induced intestinal permeability by reducing serum ALT, AST, total bilirubin, TNF-α, IL-6, IL-1β, and LPS, which could alleviate liver injury (103), as indicated in Figure 9.
3.5.3. Kidney protection through delaying disease progression, modulating the metabolic profile and related signaling pathways
The aqueous extract of LA-R (730 mg/kg/day) was orally administered to C57BL/KsJ-db/db mice to observe the progression of diabetic nephropathy. The results showed that it gradually worsened in the control group but remained unchanged in the treatment group, indicating that it can slow the progression of the disease and improve renal function (104). Another study found that LA-R attenuated adenine-induced chronic kidney disease mechanisms: modulation of metabolic profiles and TGF-β/Smad signaling (98).
3.6. Others
In addition to the bioactivities mentioned above, L. aggregata has also been observed other biological activities, such as insulin sensitivity, anti-osteoporosis (OP) effect, anti-viral activity, insecticidal activity, and anti-microbial activity. At a concentration of 1 μg/mL, bi-linderone (compound 19 in Table 4), (±)-linderaspirone A (compound 35 in Table 4) showed significant activity against glucosamine-induced insulin resistance in HepG2 cells (56, 57). Using a network pharmacology approach to explore the active components and underlying mechanisms of LA-R in OP treatment, the anti-OP activity of LA-R was validated in a prednisone-induced zebrafish model (105). Compared with the model group, the ethanol extract of LA-R significantly reduced the mRNA expression of both cathepsin K and acid phosphatase type 5a in zebrafish, indicating that it could significantly reduce osteoclast bone resorption by regulating the receptor activator of NF-κB/receptor activator of ligand/osteoclastogenesis inhibitory factor system and down-regulating cathepsin K and acid phosphatase type 5a (105). Oligomeric proanthocyanidins inhibited HIV-1 integrase with IC50 values ranging from 5.2 to 31.3 μM (52). The essential oils of L. aggregate was found to exhibit insecticidal activity against two-grain storage insects (Sitophilus zeamais and Tribolium castaneum) with LC50 values of 61.65 and 18.47 μg/adult (106). Apart from that, LA-R improves diarrhea and can treat androgenetic alopecia by controlling the scalp microbiome (107, 108).
4. Conclusion
Lindera aggregata has been widely used in traditional practices due to its potential efficacies for treating and preventing several diseases. This review provides a comprehensive investigation of the phytochemical constituents and pharmacological properties of L. aggregata. The primary chemical components isolated from the plant are sesquiterpenoids, alkaloids, and flavonoids. More importantly, they have shown interesting biological properties in various scientific investigations. Although a great deal of progress has been made in the study of L. aggregata, there are still some gaps and challenges in the findings of the existing research.
First of all, many studies have focused on validating the traditional pharmacological activities of crude extracts or a few unique chemical constituents. In contrast, the comprehensive phytochemical analysis of the assessed extract still needs to be improved, and the functional components still need to be discovered. As is known to all, the natural medicinal plant usually contains extremely complex phytochemical components. Different medicinal parts, such as LA-R and LA-L, contain various ingredients with distinctive skeletons. Different phytochemical profiles of herbs may result in various potencies in biological assessments. The synergistic effect of different components may also affect their pharmacological activities. Therefore, further development of phytochemical analysis is essential to determine the correlation between the components of different parts of L. aggregata and their pharmacological activities and to discover their promising precursors for health food or medicine.
As mentioned above, different parts of L. aggregata have different chemical compositions, which will affect its efficacy. The research cited in this review has focused on the tuberous roots and leaves of L. aggregata, and there is little research on the taproots. However, in the actual process, the mixing of taproot tubers and tuberous roots will lead to the quality decline of medicinal materials. The distinctions between these two types of roots of L. aggregata have been proved by comparing transcriptome, metabolome, and analgesic effects in 2020 (109). Simultaneously, in the latest research in the first half of 2022 (110), the portable short-wavelength infrared microscope hyperspectral imager combined with a machine learning algorithm was used to distinguish different types of roots (taproot tubers and tuberous roots) and different geographical origins of L. aggregata with high accuracy. Accordingly, future studies can focus on developing a more comprehensive, accurate, and convenient method to distinguish the taproots, tuberous roots, and the different origins to control the quality of L. aggregata.
Third, most of the above pharmacological studies have assessed pharmacological activity using simple in vitro cell lines or in vivo animal models, with only in-depth mechanism-of-action studies for a particular ingredient but no further investigation of potential mechanisms of action for most of the ingredients. Because the natural medicinal plant has the characteristics of multi-component and multi-target, further pharmacological research is needed to clarify it fully.
Fourth, there are few studies on the systemic toxicity of L. aggregata. As a medicinal and edible plant with development value, systematic safety assessment is crucial for evaluating the acute, chronic, reproductive, and genotoxicity of crude extracts and/or bioactive constituents in different experimental organisms.
Fifth, according to the unique components found in other plants of genus Lindera, such as phenylpropanoids, it is predicted that new components in L. aggregata may be further discovered.
Research in vitro and in vivo has been conducted, and the mechanism of action still needs to be in-depth. Still, the existing research lays the foundation for further exploration of new therapeutic uses of L. aggregata. As a new resource of functional food ingredients, the potential of LA-L in developing health products has attracted more and more attention, and its chemical composition and pharmacological research will continue to deepen.
Author contributions
YL: conceptualization, writing, and editing of the manuscript. YZ and XZ: collection and compilation of information. BL, CC, and XP: conception, writing, review, and editing. All authors contributed to the article and approved the submitted version.
Funding
This study was supported by the National Natural Science Foundation of China (No. 82073979), the Major Science and Technology Projects of Breeding New Varieties of Agriculture in Zhejiang Province (No. 2021C02074), and Key R&D Projects of Zhejiang Province (No. 2021C02029).
Conflict of interest
The authors declare that the research was conducted in the absence of any commercial or financial relationships that could be construed as a potential conflict of interest.
Publisher’s note
All claims expressed in this article are solely those of the authors and do not necessarily represent those of their affiliated organizations, or those of the publisher, the editors and the reviewers. Any product that may be evaluated in this article, or claim that may be made by its manufacturer, is not guaranteed or endorsed by the publisher.
Abbreviations
L. aggregata, Lindera aggregata (Sims) Kosterm.; LA-R, the roots of L. aggregata; LA-L, the leaves of L. aggregata; TGF-β, transforming growth factor-β; HLP, hyperlipidemia; TC, total cholesterol; TG, triglycerides; LDL-C, low-density lipoproteins cholesterol; HDL-C, high-density lipoprotein cholesterol; LKB1, liver kinase B1; AMPK, adenosine 5‘-monophosphate-activated protein kinase; HCL, hypercholesterolemic; ALT, alanine aminotransferase; Glu, glucose; HMGCR, 3-hydroxy-3-methylglutaryl CoA reductase; CYP7A1, cholesterol 7-α-hydroxylase; ABCA1, ATP-binding cassette transporter A1; AST, aspartate aminotransferase; SOD, superoxide dismutase; MDA, malondialdehyde; QI, Quercetin-3-O-α-L-rhamnopyranoside; HUVEcs, human umbilical vein endothelial cells; Nrf2, nuclear factor-E2-related factor 2; HO, heme oxygenase; CRC, colorectal cancer; OC, ovarian cancer; HepG2, human hepatocellular carcinomas; ILL, isolinderalactone; STAT3, signal transducer and activator of transcription 3; JNK, c-Jun N-terminal kinase; PI3K, phosphatidylinositol 3 kinase; AKT, protein kinase B; CII, collagen II; CIA, CII-induced arthritis; Th, T helper; MAPK, mitogen-activated protein kinases; NOR, norisoboldine; FLS, fibroblast-like synoviocytes; PKC, protein kinase C; CREB, cAMP-response element binding protein; Bcl-2, B-cell lymphoma-2; Bax, Bcl2-Associated X; NFAT, nuclear factor of activated T-cells; PKM2, M2 pyruvate kinase; PGC-1α, peroxisome proliferator activated receptor-γ co-activator 1-α; ALD, alcoholic liver disease; NF-κ B, nuclear factor kappa-B; TNF-α, tumor necrosis factor-α; LPS, lipopolysaccharide; OP, osteoporosis; IL, interleukin; HIF, hypoxia-inducible factor.
References
1. Cao Y, Xuan B, Li C, Chai X, Tu P. The genus Lindera: a source of structurally diverse molecules having pharmacological significance. Phytochem Rev. (2016) 15:869–906. doi: 10.1007/s11101-015-9432-2
2. Luo L, Tian J, Yang S. Advances in chemical constituents of medicinal plants of Piper. Asia Pac Tradit Med. (2007) 3:57–60. doi: 10.3969/j.issn.1673-2197.2007.10.018
3. Xing M, Tian C, Xia D. Review on chemical constituents and pharmacological effects of Lindera aggregata. Nat Prod Res Dev. (2017) 29:2147–51. doi: 10.16333/j.1001-6880.2017.12.025
4. Sun C, Yu S, Li S, Yuan L, Wang F, Peng X. Chemical constituents from the leaves of Lindera aggregata. Chin Tradit Pat Med. (2022) 44:464–9. doi: 10.3969/j.issn.1001-1528.2022.02.024
5. Hai P, Gao Y, Li T, Wang F. Chemical constituents from roots of Lindera aggregata. Chin Tradit Herb Drugs. (2016) 47:872–5. doi: 10.7501/j.issn.0253-2670.2016.06.002
6. Cheng H, Cai X, Xiang J, Li Z. Analysis of medicinal value of roots and leaves of Lindera aggregata (Sims) Kosterm. Zhejiang J Integr Tradit Chin West Med. (2014) 24:563–5. doi: 10.3969/j.issn.1005-4561.2014.06.046
7. Zhao J, Lu X, Xu X, Luo L, Fu H, Li J. Chemical constituents of leaves of Lindera aggregata (Sims) Kosterm. Chin Pharm J. (2012) 47:1702–5.
8. Universiti P, Salleh W. Lindera aggregata (Sims) kosterm.: review on phytochemistry and biological activities. Boletin Latinoam Caribe Plantas Med Aromat. (2020) 19:527–41. doi: 10.37360/blacpma.20.19.6.37
9. Liu Q, Ahn J, Kim S, Lee C, Hwang B, Lee M. Sesquiterpene lactones from the roots of Lindera strychnifolia. Phytochemistry. (2013) 87:112–8. doi: 10.1016/j.phytochem.2012.11.004
10. Qiang Y, Yang Z, Yang J, Gao K. Sesquiterpenoids from the root tubers of Lindera aggregata. Planta Med. (2011) 77:1610–6. doi: 10.1055/s-0030-1270922
11. Cheng X, Ma S, Wei F, Wang G, Xiao X, Lin RC. A new sesquiterpene isolated from Lindera aggregata (Sims) Kosterm. Chem Pharm Bull. (2007) 55:1390–2. doi: 10.1248/cpb.55.1390
12. Yang H, Kwon E, Li W. Linderolide U, a new sesquiterpene from Lindera aggregata root. Nat Prod Res. (2022) 36:1914–8. doi: 10.1080/14786419.2020.1821021
13. Gan L, Zheng Y, Mo J, Liu X, Li X, Zhou C. Sesquiterpene lactones from the root tubers of Lindera aggregata. J Nat Prod. (2009) 72:1497–501. doi: 10.1021/np900354q
14. Sumioka H, Harinantenaina L, Matsunami K, Otsuka H, Kawahata M, Yamaguchi K. Linderolides A–F, eudesmane-type sesquiterpene lactones and linderoline, a germacrane-type sesquiterpene from the roots of Lindera strychnifolia and their inhibitory activity on NO production in RAW 264.7 cells in vitro. Phytochemistry. (2011) 72:2165–71. doi: 10.1016/j.phytochem.2011.08.004
15. Ohno T, Nagatsu A, Nakagawa M, Inoue M, Li Y, Minatoguchi S. New sesquiterpene lactones from water extract of the root of Lindera strychnifolia with cytotoxicity against the human small cell lung cancer cell. SBC-3. Tetrahedron Lett. (2005) 46:8657–60. doi: 10.1016/j.tetlet.2005.10.051
16. Kuo P, Wu Y, Hung H, Lam S, Ma G, Kuo L. Anti-inflammatory principles from Lindera aggregata. Bioorg Med Chem Lett. (2020) 30:127224. doi: 10.1016/j.bmcl.2020.127224
17. Gui C, Nakamura N, Chao M, Guo X. Seven new sesquiterpene lactones from Lindera aggregata. J China Pharm Univ. (2000) 78:827–34. doi: 10.1246/bcsj.78.827
18. Zhang C, Sun Q, Chou G, Wang Z. Studies on the flavonoids from leaves of Lindera aggregata (Sims) kosterm.(2). J Shenyang Pharm Univ. (2003) 20, 342–4. doi: 10.3969/j.issn.1006-2858.2003.05.009
19. Wen S, Wang Y, Xu J, Liu Q, Zhang L, Zheng J. Two new sesquiterpenoid lactone derivatives from Lindera aggregata. Nat Prod Res. (2021) 4:1–9. doi: 10.1080/14786419.2021.1939332
20. Liu Q, Jo Y, Kim S, Jin Q, Hwang B, Lee M. Sesquiterpenes from the roots of Lindera strychnifolia with inhibitory effects on nitric oxide production in RAW 264.7 cells. Bioorg Med Chem Lett. (2016) 26:4950–4. doi: 10.1016/j.bmcl.2016.09.012
21. Kouno I, Hirai A, Fukushige A, Jiang Z, Tanaka T. New eudesmane sesquiterpenes from the root of Lindera strychnifolia. J Nat Prod. (2001) 64:286–8. doi: 10.1021/np000154s
22. Li J, Ding Y, Li W. A new sesquiterpene from the roots of Lindera strychnifolia. Chin Chem Lett. (2002) 13, 965–7. doi: 10.1021/cm010622z
23. Zhang C, Nakamura N, Tewtrakul S, Hattori M, Sun Q, Wang Z. Sesquiterpenes and alkaloids from Lindera chunii and their inhibitory activities against HIV-1 Integrase. Chem Pharm Bull. (2002) 50:1195–200. doi: 10.1248/cpb.50.1195
24. Chen H. Study on the consitituents and antitumer activity of Lindera aggregata (Sims) kosterm. Ph.D. thesis. Chengdu: Southwest Jiaotong University (2015).
25. Mimura A, Sumioka H, Matsunami K, Otsuka H. Conjugates of an abscisic acid derivative and phenolic glucosides, and a new sesquiterpene glucoside from Lindera strychnifolia. J Nat Med. (2010) 64:153–60. doi: 10.1007/s11418-010-0391-z
26. Kim J, Jeon J, Kim J, Jung E, Lee Y, Gao E, et al. Bioassay-guided isolation of two eudesmane sesquiterpenes from Lindera strychnifolia using centrifugal partition chromatography. Molecules. (2021) 26:5269. doi: 10.3390/molecules26175269
27. Li B, Jeong G, Kang D, Lee H, Kim Y. Cytoprotective effects of lindenenyl acetate isolated from Lindera strychnifolia on mouse hippocampal HT22 cells. Eur J Pharmacol. (2009) 614:58–65. doi: 10.1016/j.ejphar.2009.04.056
28. Yen M, Shih Y, Hsu Y, Lin E, Lin Y, Tsai E. Isolinderalactone enhances the inhibition of SOCS3 on STAT3 activity by decreasing miR-30c in breast cancer. Oncol Rep. (2016) 35:1356–64. doi: 10.3892/or.2015.4503
29. Rajina S, Kim W, Shim J, Chun K, Joo S, Shin H. Isolinderalactone induces cell death via mitochondrial superoxide- and STAT3-mediated pathways in human ovarian cancer cells. Int J Mol Sci. (2020) 21:7530. doi: 10.3390/ijms21207530
30. Hwang J, Park J, Kim M, Kim W, Ha K, Choi B. Isolinderalactone regulates the BCL-2/caspase-3/PARP pathway and suppresses tumor growth in a human glioblastoma multiforme xenograft mouse model. Cancer Lett. (2019) 443:25–33. doi: 10.1016/j.canlet.2018.11.027
31. Kwak A, Park J, Lee S, Lee J, Seo J, Goo Y. Isolinderalactone sensitizes oxaliplatin-resistance colorectal cancer cells through JNK/p38 MAPK signaling pathways. Phytomedicine. (2022) 105:154383. doi: 10.1016/j.phymed.2022.154383
32. Liu X, Fu J, Yang J, Huang A, Li R, Bai L. Linderaggrenolides A–N, oxygen-conjugated sesquiterpenoid dimers from the roots of Lindera aggregata. ACS Omega. (2021) 6:5898–909. doi: 10.1021/acsomega.0c06349
33. Liu X, Fu J, Shen R, Wu X, Yang J, Bai L. Linderanoids A–O, dimeric sesquiterpenoids from the roots of Lindera aggregata (Sims) kosterm. Phytochemistry. (2021) 191:112924. doi: 10.1016/j.phytochem.2021.112924
34. Liu X, Yang J, Fu J, Yao X, Wang J, Liu L. Aggreganoids A–F, carbon-bridged sesquiterpenoid dimers and trimers from Lindera aggregata. Org Lett. (2019) 21:5753–6. doi: 10.1021/acs.orglett.9b02166
35. Liu X, Yang J, Yao X, Yang X, Fu J, Bai L. Linderalides A–D, disesquiterpenoid–geranylbenzofuranone conjugates from Lindera aggregata. J Org Chem. (2019) 84:8242–7. doi: 10.1021/acs.joc.9b00522
36. Chou G, Norio N, Ma C, Wang Z, Masao H. Isoquinoline alkaloids from Lindera aggregata. Chin J Nat Med. (2005) 3:272–5.
37. Yang J, Chen Y, Guo M, Chou G. Chemical constituents from the roots of Lindera aggregata and their biological activities. J Nat Med. (2020) 74:441–7. doi: 10.1007/s11418-019-01385-6
38. Gan L, Yao W, Mo J, Zhou C. Alkaloids from Lindera aggregata. Nat Prod Commun. (2009) 4:43–6. doi: 10.1002/med.20140
39. Ma G, Lin C, Hung H, Wang S, Shieh P, Wu T. New benzenoids from the roots of Lindera aggregata. Nat Prod Commun. (2015) 10:2131–3. doi: 10.1177/1934578X1501001229
40. Zhu Y, Liu M, Hu D, Lin H, Xue W, Yang S. Chemical constituents of Lindera aggregata. Chin J Exp Tradit Med Formulae. (2012) 18:123–6. doi: 10.13422/j.cnki.syfjx.2012.16.085
41. Duan X, Zhang X, He P, Qin M, Lin P, Zhao J, et al. New benzylisoquinoline alkaloid from the root tuber of Lindera aggregata. J Chin Med Mater. (2021) 44:76–8. doi: 10.13863/j.issn1001-4454.2021.01.014
42. Gan L, Zhao X, Yao W, Wu L, Li L, Zhou CX. A novel bisbenzylisoquinoline alkaloid from Lindera Aggregata. J Chem Res. (2008) 2008:285–6. doi: 10.3184/030823408X320674
43. Kuo P, Li Y, Hwang T, Ma G, Yang M, Lee E, et al. Synthesis and structural characterization of an anti-inflammatory principle purified from Lindera aggregata. Tetrahedron Lett. (2014) 55:108–10. doi: 10.1016/j.tetlet.2013.10.126
44. Zhang C, Sun Q, Zhao Y, Wang Z. Studies on flavonoids from leaves of Lindera aggregata (Sims) kosterm. Chin J Med Chem. (2001) 11, 28–30. doi: 10.3969/j.issn.1005-0108.2001.05.006
45. Luo L, Zhang L, Tian J, Yang S. Chemical constituents from leaves of Lindera aggregate. Chin Tradit Herb Drugs. (2009) 40:856–8. doi: 10.3321/j.issn:0253-2670.2009.06.006
46. Xiao M, Cao N, Fan J, Shen Y, Xu Q. Studies on flavonoids from the leaves of Lindera aggregate. J Chin Med Mater. (2011) 34:62–4. doi: 10.13863/j.issn1001-4454.2011.01.024
47. Liu Y, Chou G. Isolation and preparation of flavones from the leaves of Lindera aggregata using high speed counter-current chromatography. Chin J Chromatogr. (2007) 25:735–9. doi: 10.3321/j.issn:1000-8713.2007.05.024
48. Chou G, Li Q, Wang Z, Xu L, Xu G, Norio N. Compositions and antirheumatic effect of LEF fraction from the root of Lindera aggregata (Sims) Kosterm. J Plant Resour Environ. (1999) 8:1–6.
49. Gu L, Luo Q, Xiao M, Wu X, He G, Sun Y. Anti- oxidative and hepatoprotective activities of the total flavonoids from the leaf of Lindera aggregata (Sims) kosterm. Against mice liver injury induced by carbon tetrachioride. Tradit Chin Drug Res Clin Pharmacol. (2008) 19:447–50. doi: 10.19378/j.issn.1003-9783.2008.06.010
50. Han H, Xu B, Amin A, Li H, Yu X, Gong M. Quercetin-3-O−α−L-rhamnopyranoside derived from the leaves of Lindera aggregata (Sims) kosterm. Evokes the autophagy-induced nuclear factor erythroid 2-related factor 2 antioxidant pathway in human umbilical vein endothelial cells. Int J Mol Med. (2018) 43:461–74. doi: 10.3892/ijmm.2018.3976
51. Zhang C, Chou G, Sun Q, Wang Z, Masao H. Tannins from the stems of Lindera aggregata I. Chin J Nat Med. (2003) 1:204–6.
52. Zhang C, Sun Q, Wang Z, Masao H, Supinya T. Inhibitory activities of tannins extracted from stem of Lindera aggregata against HIV-1 integrase. Chin Pharm J. (2003) 38, 17–20. doi: 10.3321/j.issn:1001-2494.2003.12.006
53. Kobayashi W, Miyase T, Sano M, Umehara K, Warashina T, Noguchi H. Prolyl endopeptidase inhibitors from the roots of Lindera strychnifolia F. VILL. Biol Pharm Bull. (2002) 25:1049–52. doi: 10.1248/bpb.25.1049
54. Chen L, Liu B, Deng J, Zhang J, Li W, Ahmed A. Lindera cyclopentenedione intermediates from the roots of Lindera aggregata. RSC Adv. (2018) 8:17898–904. doi: 10.1039/C8RA03094D
55. Lin C, Chu F, Chang S, Chueh P, Su Y, Wu K. Secoaggregatalactone-A from Lindera aggregata induces apoptosis in human hepatoma Hep G2 Cells. Planta Med. (2007) 73:1548–53. doi: 10.1055/s-2007-993739
56. Wang F, Gao Y, Zhang L, Bai B, Hu Y, Dong ZJ. A pair of windmill-shaped enantiomers from Lindera aggregata with activity toward improvement of insulin sensitivity. Org Lett. (2010) 12:3196–9. doi: 10.1021/ol1011289
57. Wang F, Gao Y, Zhang L, Liu J. Bi-linderone, a Highly modified methyl-linderone dimer from Lindera aggregata with activity toward improvement of insulin sensitivity in vitro. Org Lett. (2010) 12:2354–7. doi: 10.1021/ol1007247
58. Fu J, Li J, Chen S, Yao X, Wang Q. Studies on the chemical constituents of essential oils from Lindera aggregata (Sims) Kosterm. leaves. Chin Tradit Herb Drugs. (2009) 40:112–4.
59. Yan R, Peng X. Chemical composition and pharmacological activity of Lindera strychnifolia essential oil. Lishizhen Med Mater Medica Res. (2014) 25:2747–9. doi: 10.3969/j.issn.1008-0805.2014.11.075
60. Yan R, Yang Y, Zeng Y, Zou G. Cytotoxicity and antibacterial activity of Lindera strychnifolia essential oils and extracts. J Ethnopharmacol. (2009) 121:451–5. doi: 10.1016/j.jep.2008.06.010
61. Luo Y, Cai H, Sha X, Chen H, Shen X, Zhou G. GC-MS analysis of volatile components in different parts of Lindera aggregata. J Chin Med Mater. (2019) 42:1319–22. doi: 10.13863/j.issn1001-4454.2019.06.022
62. Stewart J, McCallin T, Martinez J, Chacko S, Yusuf S. Hyperlipidemia. Pediatr Rev. (2020) 41:393–402. doi: 10.1542/pir.2019-0053
63. Chen J, Luo H, Liu Y, Hu Z, Liu Z, Qu X. The effect of leaves of Lindera aggregata on the regulation of blood lipids and histomorphology in hyperlipidemia rats. J Toxicol. (2020) 34:305–10. doi: 10.16421/j.cnki.1002-3127.2020.04.007
64. Sun Y, Huang J, Kang Y, Mao P, Wang Z, Luan H. Effect of Lindera aggregata extract on lipid-lowering and hepatic LKB1-AMPK pathway in hyperlipidemic rat model. Chin J Mod Appl Pharm. (2020) 37:821–5. doi: 10.13748/j.cnki.issn1007-7693.2020.07.009
65. von Eckardstein A, Nofer J, Assmann G. High density lipoproteins and arteriosclerosis. Arterioscler Thromb Vasc Biol. (2001) 21:13–27. doi: 10.1161/01.ATV.21.1.13
66. Wang J, Wang F, Yuan L, Wu Y, Peng X, Kai G. Aqueous extracts of Lindera aggregate (Sims) Kosterm. leaves regulate serum/hepatic lipid and liver function in normal and hypercholesterolemic mice. J Pharmacol Sci. (2020) 143:45–51. doi: 10.1016/j.jphs.2020.01.009
67. Liu H, Huang J, Huang M, Jiang T, Lv G, Li B. Study on anti-hyperlipidemia effect of Linderae Radix via regulating reverse cholesterol transport. China J Chin Mater Med. (2021) 46:1795–802. doi: 10.19540/j.cnki.cjcmm.20200104.401
68. Gong X, Li X, Xia Y, Xu J, Li Q, Zhang C, et al. Effects of phytochemicals from plant-based functional foods on hyperlipidemia and their underpinning mechanisms. Trends Food Sci Technol. (2020) 103:304–20. doi: 10.1016/j.tifs.2020.07.026
69. Jiang T, Xu C, Liu H, Liu M, Wang M, Jiang J. Linderae Radix ethanol extract alleviates diet-induced hyperlipidemia by regulating bile acid metabolism through gut microbiota. Front Pharmacol. (2021) 12:627920. doi: 10.3389/fphar.2021.627920
70. Sies H, Berndt C, Jones D. Oxidative stress. Annu Rev Biochem. (2017) 86:715–48. doi: 10.1146/annurev-biochem-061516-045037
71. Liang X, Zhou Q. Study on antioxidant effect of Lindera aggregata leaf tea. J Hyg Res. (2006) 35:636–8. doi: 10.3969/j.issn.1000-8020.2006.05.033
72. Han H. Study on pharmacological activities and mechanism of quercitrin from the leaves of Lindera aggregate (Sims) Kosterm. About the cardiovascular disease. Ph.D. thesis. Hangzhou: Zhejiang University (2017).
73. Li Y, Ohno Y, Minatoguchi S, Fukuda K, Ikoma T, Ohno T. Extracts from the roots of Lindera strychifolia Induces apoptosis in lung cancer cells and prolongs survival of tumor-bearing mice. Am J Chin Med. (2003) 31:857–69. doi: 10.1142/S0192415X03001545
74. Xu D, Tian M, Chen W, Bian Y, Xia X, Liu Q. Linderalactone suppresses pancreatic cancer development in vitro and in vivo via negatively regulating PI3K/AKT signaling pathway. J Oncol. (2022) 2022:1–12. doi: 10.1155/2022/8675096
75. Yan R, Hua J. In vitro antitumor activity of Lindera strychnifolia root essential oil and its active constituent. J Wuhan Univ Sci Ed. (2014) 60:345–8. doi: 10.14188/j.1671-8836.2014.04.025
76. Chuang C, Wang L, Wong Y, Lin E. Anti-metastatic effects of isolinderalactone via the inhibition of MMP-2 and up regulation of NM23-H1 expression in human lung cancer A549 cells. Oncol Lett. (2018) 15:4690–6. doi: 10.3892/ol.2018.7862
77. Lai H, Yang Z, Lou Z, Li F, Xie F, Pan W. Root Extract of Lindera aggregata (Sims) kosterm. Modulates the Th17/Treg balance to attenuate DSS-induced colitis in mice by IL-6/STAT3 signaling pathway. Front Pharmacol. (2021) 12:615506. doi: 10.3389/fphar.2021.615506
78. Chou D, Lam K, Chen J, Yao P, Dong T, Xiong A. Alkaloids of Linderae Radix suppressed the lipopolysaccharide-induced expression of cytokines in cultured macrophage RAW 264.7 cells. TANG Humanit Med. (2014) 4:28.1–27. doi: 10.5667/TANG.2014.0019
79. Zhang J, Luo R, Yang Y, Liu B. Pharmacology researches on Anti-inflammatory and analgesia test of total alkaloids from Lindera Radix. Hubei Agric Sci. (2016) 55:5101–3. doi: 10.14088/j.cnki.issn0439-8114.2016.19.053
80. Wang C, Dai Y, Yang J, Chou G, Wang C, Wang Z. Treatment with total alkaloids from Radix Linderae reduces inflammation and joint destruction in type II collagen-induced model for rheumatoid arthritis. J Ethnopharmacol. (2007) 111:322–8. doi: 10.1016/j.jep.2006.11.031
81. Luo Y, Liu M, Yao X, Xia Y, Dai Y, Chou G. Total alkaloids from Radix Linderae prevent the production of inflammatory mediators in lipopolysaccharide-stimulated RAW 264.7 cells by suppressing NF-κB and MAPKs activation. Cytokine. (2009) 46:104–10. doi: 10.1016/j.cyto.2008.12.017
82. Bhattacharya S, Mandal S, Akhtar S, Dastider D, Sarkar S, Bose S, et al. Phytochemicals in the treatment of arthritis: current knowledge. Int J Curr Pharm Res. (2020) 12:1–6. doi: 10.22159/ijcpr.2020v12i4.39050
83. Luo Y, Liu M, Xia Y, Dai Y, Chou G, Wang Z. Therapeutic effect of norisoboldine, an alkaloid isolated from Radix Linderae, on collagen-induced arthritis in mice. Phytomedicine. (2010) 17:726–31. doi: 10.1016/j.phymed.2010.01.013
84. Wei Z, Wang F, Song J, Lu Q, Zhao P, Xia Y. Norisoboldine inhibits the production of interleukin-6 in fibroblast-like synoviocytes from adjuvant arthritis rats through PKC/MAPK/NF-κB-p65/CREB pathways. J Cell Biochem. (2012) 113:2785–95. doi: 10.1002/jcb.24156
85. Wei Z, Tong B, Xia Y, Lu Q, Chou G, Wang Z. Norisoboldine suppresses osteoclast differentiation through preventing the accumulation of TRAF6-TAK1 complexes and activation of MAPKs/NF-κB/c-Fos/NFATc1 pathways. PLoS One. (2013) 8:e59171. doi: 10.1371/journal.pone.0059171
86. Luo Y, Wei Z, Chou G, Wang Z, Xia Y, Dai Y. Norisoboldine induces apoptosis of fibroblast-like synoviocytes from adjuvant-induced arthritis rats. Int Immunopharmacol. (2014) 20:110–6. doi: 10.1016/j.intimp.2014.02.023
87. Lu Q, Tong B, Luo Y, Sha L, Chou G, Wang Z. Norisoboldine suppresses VEGF-induced endothelial cell migration via the cAMP-PKA-NF-κB/Notch1 pathway. PLoS One. (2013) 8:e81220. doi: 10.1371/journal.pone.0081220
88. Lu Q, Lu S, Gao X, Luo Y, Tong B, Wei Z. Norisoboldine, an alkaloid compound isolated from Radix Linderae, inhibits synovial angiogenesis in adjuvant-induced arthritis rats by moderating Notch1 pathway-related endothelial tip cell phenotype. Exp Biol Med. (2012) 237:919–32. doi: 10.1258/ebm.2012.011416
89. Wei Z, Lv Q, Xia Y, Yue M, Shi C, Xia Y. Norisoboldine, an anti-arthritis alkaloid isolated from Radix Linderae, attenuates osteoclast differentiation and inflammatory bone erosion in an aryl hydrocarbon receptor-dependent manner. Int J Biol Sci. (2015) 11:1113–26. doi: 10.7150/ijbs.12152
90. Tong B, Dou Y, Wang T, Yu J, Wu X, Lu Q. Norisoboldine ameliorates collagen-induced arthritis through regulating the balance between Th17 and regulatory T cells in gut-associated lymphoid tissues. Toxicol Appl Pharmacol. (2015) 282:90–9. doi: 10.1016/j.taap.2014.11.008
91. Tong B, Yuan X, Dou Y, Wu X, Chou G, Wang Z. Norisoboldine, an isoquinoline alkaloid, acts as an aryl hydrocarbon receptor ligand to induce intestinal Treg cells and thereby attenuate arthritis. Int J Biochem Cell Biol. (2016) 75:63–73. doi: 10.1016/j.biocel.2016.03.014
92. Gao S, Li W, Lin G, Liu G, Deng W, Zhai C. Norisoboldine, an alkaloid from Radix linderae, inhibits NFAT activation and attenuates 2,4-dinitrofluorobenzene-induced dermatitis in mice. Immunopharmacol Immunotoxicol. (2016) 38:327–33. doi: 10.1080/08923973.2016.1202961
93. Chen Q, Shao X, He Y, Lu E, Zhu L, Tang W. Norisoboldine attenuates sepsis-induced acute lung injury by modulating macrophage polarization via PKM2/HIF-1α/PGC-1α pathway. Biol Pharm Bull. (2021) 44:1536–47. doi: 10.1248/bpb.b21-00457
94. Lv Q, Wang K, Qiao S, Dai Y, Wei Z. Norisoboldine, a natural aryl hydrocarbon receptor agonist, alleviates TNBS-induced colitis in mice, by inhibiting the activation of NLRP3 inflammasome. Chin J Nat Med. (2018) 16:161–74. doi: 10.1016/S1875-5364(18)30044-X
95. Lv Q, Wang K, Qiao S, Yang L, Xin Y, Wei Z. Norisoboldine, a natural AhR agonist, promotes Treg differentiation and attenuates colitis via targeting glycolysis and subsequent NAD+/SIRT1/SUV39H1/H3K9me3 signaling pathway. Cell Death Dis. (2018) 9:258. doi: 10.1038/s41419-018-0297-3
96. Lv Q, Qiao S, Xia Y, Shi C, Xia Y, Chou G. Norisoboldine ameliorates DSS-induced ulcerative colitis in mice through induction of regulatory T cells in colons. Int Immunopharmacol. (2015) 29:787–97. doi: 10.1016/j.intimp.2015.08.040
97. Selby N, Taal M. An updated overview of diabetic nephropathy: diagnosis, prognosis, treatment goals and latest guidelines. Diabetes Obes Metab. (2020) 22:3–15. doi: 10.1111/dom.14007
98. Cai H, Wang J, Luo Y, Wang F, He G, Zhou G. Lindera aggregata intervents adenine-induced chronic kidney disease by mediating metabolism and TGF-β/Smad signaling pathway. Biomed Pharmacother. (2021) 134:111098. doi: 10.1016/j.biopha.2020.111098
99. Zhang H, Wang H, Zhong G, Zhu J. Molecular mechanism and research progress on pharmacology of traditional Chinese medicine in liver injury. Pharm Biol. (2018) 56:594–611. doi: 10.1080/13880209.2018.1517185
100. Wang J, Chen X, Hu P, Tan M, Tang X, Huang M, et al. Effects of Linderae radix extracts on a rat model of alcoholic liver injury. Exp Ther Med. (2016) 11:2185–92. doi: 10.3892/etm.2016.3244
101. Fairfield B, Schnabl B. Gut dysbiosis as a driver in alcohol-induced liver injury. JHEP Rep. (2021) 3:100220. doi: 10.1016/j.jhepr.2020.100220
102. Lou Z, Wang J, Chen Y, Xu C, Chen X, Shao T. Linderae radix ethanol extract attenuates alcoholic liver injury via attenuating inflammation and regulating gut microbiota in rats. Braz J Med Biol Res. (2019) 52:e7628. doi: 10.1590/1414-431x20197628
103. Wang J, Tan M, Ji M, Hu P, Chen X, Lou Z. Relationship between alcoholic liver injury and endotoxin leakage from gut and intervention effects of Linderae radix extracts. Chin Arch Tradit Chin Med. (2017) 35:2515–7. doi: 10.13193/j.issn.1673-7717.2017.10.013
104. Ohno T, Takemura G, Murata I, Kagawa T, Akao S, Minatoguchi S. Water extract of the root of Lindera strychnifolia slows down the progression of diabetic nephropathy in db/db mice. Life Sci. (2005) 77:1391–403. doi: 10.1016/j.lfs.2005.04.018
105. Wang F, Rong P, Wang J, Yu X, Wang N, Wang S. Anti-osteoporosis effects and regulatory mechanism of Lindera aggregata based on network pharmacology and experimental validation. Food Funct. (2022) 13:6419–32. doi: 10.1039/D2FO00952H
106. Liu Z, Chu S, Jiang C, Hou J, Liu Q, Jiang G. Composition and insecticidal activity of the essential oil of Lindera aggregata Root Tubers against Sitophilus zeamais and Tribolium castaneum. J Essent Oil Bear Plants. (2016) 19:727–33. doi: 10.1080/0972060X.2014.960275
107. Shu Y. Effects of Lindera aggregata water extract on gastrointestinal function and brain-gut peptide level in IBS-D rats. Ph.D. thesis. Changsha: Hunan University of Chinese Medicine (2017).
108. Filaire E, Dreux A, Boutot C, Ranouille E, Berthon J. Characteristics of healthy and androgenetic alopecia scalp microbiome: effect of Lindera strychnifolia roots extract as a natural solution for its modulation. Int J Cosmet Sci. (2020) 42:615–21. doi: 10.1111/ics.12657
109. Peng X, Luo Y, Wang J, Ji T, Yuan L, Kai G. Integrated analysis of the transcriptome, metabolome and analgesic effect provide insight into potential applications of different parts of Lindera aggregata. Food Res Int. (2020) 138:109799. doi: 10.1016/j.foodres.2020.109799
Keywords: Lindera aggregata (Sims) Kosterm., chemical constituents, pharmacological activity, nutrient, Chinese medicines
Citation: Lv Y, Zou Y, Zhang X, Liu B, Peng X and Chu C (2023) A review on the chemical constituents and pharmacological efficacies of Lindera aggregata (Sims) Kosterm. Front. Nutr. 9:1071276. doi: 10.3389/fnut.2022.1071276
Received: 16 October 2022; Accepted: 28 December 2022;
Published: 16 January 2023.
Edited by:
Yuyun Lu, National University of Singapore, SingaporeReviewed by:
Xiaoxiao Huang, Shenyang Pharmaceutical University, ChinaCaili Fu, NUS (Suzhou) Research Institute (NUSRI), China
Xingyun Chai, Beijing University of Chinese Medicine, China
Copyright © 2023 Lv, Zou, Zhang, Liu, Peng and Chu. This is an open-access article distributed under the terms of the Creative Commons Attribution License (CC BY). The use, distribution or reproduction in other forums is permitted, provided the original author(s) and the copyright owner(s) are credited and that the original publication in this journal is cited, in accordance with accepted academic practice. No use, distribution or reproduction is permitted which does not comply with these terms.
*Correspondence: Xin Peng, pengx@nit.zju.edu.cn; Chu Chu,
chuchu@zjut.edu.cn