- 1Department of Neurosurgery, Beijing Neurosurgical Institute and Beijing Tiantan Hospital, Capital Medical University, Beijing, China
- 2Department of Neurosurgery, Beijing Chaoyang Hospital, Capital Medical University, Beijing, China
- 3School of Biomedical Engineering, Capital Medical University, Beijing, China
Objective: The aim of this study was to assess the causal relationships between blood metabolites and intracranial aneurysm, aneurysmal subarachnoid hemorrhage, and unruptured intracranial aneurysm.
Methods: Our exposure sample consisted of 7,824 individuals from a genome-wide association study of human blood metabolites. Our outcome sample consisted of 79,429 individuals (7,495 cases and 71,934 controls) from the International Stroke Genetics Consortium, which conducted a genome-wide association study of intracranial aneurysm, aneurysmal subarachnoid hemorrhage, and unruptured intracranial aneurysm. We identified blood metabolites with a potential causal effect on intracranial aneurysms and conducted sensitivity analyses to validate our findings.
Results: After rigorous screening and Mendelian randomization tests, we found four, two, and three serum metabolites causally associated with intracranial aneurysm, aneurysmal subarachnoid hemorrhage, and unruptured intracranial aneurysm, respectively (all P < 0.05). Sensitivity analyses confirmed the robustness of these associations.
Conclusions: Our Mendelian randomization analysis demonstrated causal relationships between human blood metabolites and intracranial aneurysm, aneurysmal subarachnoid hemorrhage, and unruptured intracranial aneurysm. Further research is required to explore the potential of targeting these metabolites in the management of intracranial aneurysm.
Introduction
The prevalence of intracranial aneurysms (IAs) in the general population worldwide is approximately 3%−5% (1). IAs are characterized by localized deterioration of the arterial wall structure, loss of the internal elastic plate, and disruption of the arterial media (2). The most severe complication of IAs is rupture, accounting for ~85% of spontaneous subarachnoid hemorrhages (SAHs) (3). Aneurysmal SAH (aSAH) from an IA typically leads to a poor prognosis, with high rates of disability and mortality (4, 5). Despite the recognized contribution of genetic and environmental factors and their complex interactions in IAs, a better understanding of the pathogenesis of IAs is required.
Previous investigations have proposed that metabolites function as intermediary components, facilitating exploration of the connection between genetic variation and metabolites (6). In an observational study, Duan et al. found a significantly disrupted metabolic profile in the IA population compared with that in the normal population. Serum adenosine was significantly decreased in patients with unruptured IA (UIA) compared with that in the normal population, whereas serum levels of various glycine-conjugated secondary bile acids were decreased in patients with aSAH (7). Cotinine is a major metabolite of nicotine, and Missori et al. found that in a population of smokers with IA, aneurysms were more likely to rupture in people with high rather than low cotinine levels (8). Therefore, establishing a causal relationship between these two factors is essential for developing effective metabolite-targeted interventions to prevent and manage IAs in clinical practice.
Mendelian randomization (MR) is a statistical technique that enables the inference of causality between exposure and outcome using genetic variants associated with exposure as instrumental variables (IVs) (9–11). Whether an instrumental variable is valid depends on the fulfillment of three basic assumptions: they are required to demonstrate a clear association with the particular risk factor of interest (the relevance assumption); they must have no causal determinants in common with the outcome under investigation (the independence assumption); and they must not have any direct effect on the outcome variable, except through their effect on intermediate risk factors (the exclusion restriction assumption) (10). Compared with observational studies, MR studies are less susceptible to confounding because genetic variants are determined and randomly assigned. Thus, this design, similar to previous MR studies, helps to identify causal associations of a large number of serum metabolites with IAs (12, 13). Large-scale genome-wide association studies (GWASs) have provided promising results for MR analyses of various diseases, including those related to human blood metabolites (14). However, no MR study has been conducted to examine the potential causal relationship between blood metabolites and IAs.
In the present study, we aimed to investigate the possible causal relationships between blood metabolites and IAs through a comprehensive two-sample MR analysis.
Methods
Study design and data sources
The flow of this study is shown in Figure 1. This study used summary-level data from publicly available GWASs. The GWAS examining human blood metabolites was conducted by Shin and colleagues, and the GWAS investigating IAs was performed by the Intracranial Aneurysm working group of the International Stroke Genetics Consortium (14, 15). The GWAS studies had ethical approval and participant consent, and de-identified aggregate data were made available for analysis. This MR study was conducted based on strengthening the reporting of observational studies in epidemiology (STROBE-MR) guidelines (Supplementary Table 1) (16).
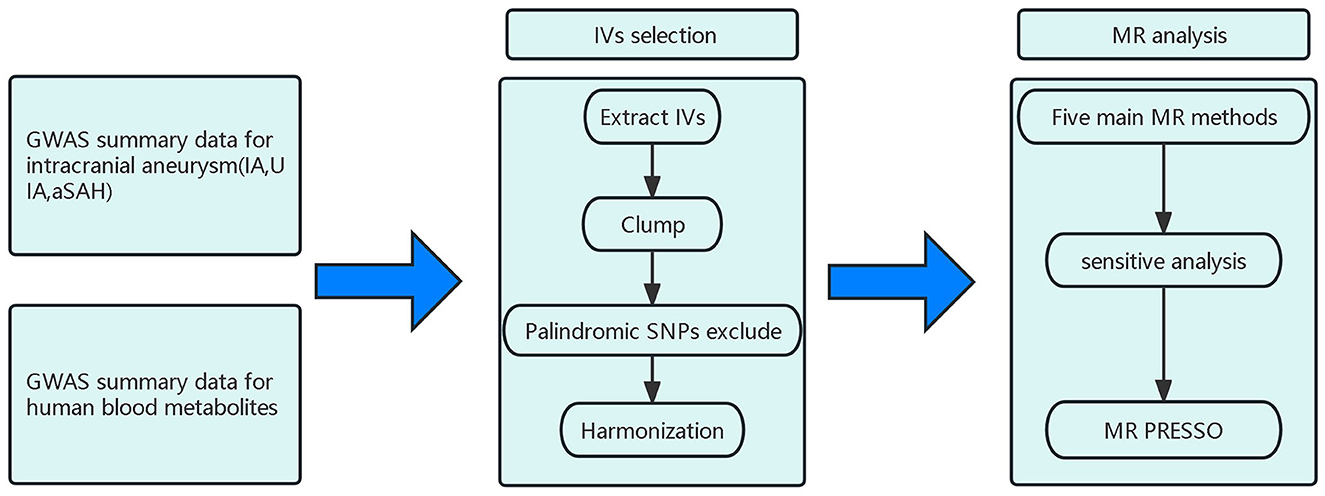
Figure 1. Design and workflow of this study; IA, Intracranial aneurysm; UIA, Unruptured intracranial aneurysm; aSAH, aneurysmal Subarachnoid hemorrhage; IV, Instrumental variable; SNP, Single-nucleotide polymorphism; GWAS, Genome-wide association study; MR, Mendelian randomization.
Blood metabolite samples
The blood metabolite summary statistics were sourced from an extensive investigation conducted by Shin and colleagues, which stands as one of the most comprehensive studies in the field (14). A sample size of 7,824 adults from Europe was collected for the GWAS analysis, with over 2.1 million single-nucleotide polymorphisms (SNPs) analyzed from this population. Following rigorous quality control measures, a total of 486 metabolites were deemed suitable for the GWAS analysis. These metabolites were further classified into 309 known metabolites and 177 unknown metabolites. The 309 known metabolites were categorized into eight distinct biochemical groups, namely amino acids, peptides, lipids, cofactors and vitamins, carbohydrates, energy-related molecules, nucleotides, and exotic organisms.
IA sample
Summary data for IA patients were collected from a GWAS of 23 cohorts with 79,429 individuals, including 7,495 cases and 71,934 controls. Among the total cases, 69% had a ruptured IA, 28% had a UIA, and 3.8% had an unknown rupture status (15). The present study analyzed three summary datasets and applied MR techniques. The three datasets were as follows: a GWAS of IA cases (ruptured, unruptured, and unclear) (n = 7,495), a GWAS of UIA cases (n = 2,070), and a GWAS of aSAH cases (n = 5,140); each dataset was compared with that of the control group (n = 71,934). All participants in the study were of European descent.
Selection of IVs
Genome-wide statistically significant SNPs associated with blood metabolites were used as IVs, and the genetic instruments used a value of P < 5 × 10−6. Several quality control steps were performed to ensure eligible IV selection, including eliminating SNPs with inconsistent alleles between the exposure and outcome samples (i.e., A/G vs. A/C), retaining only independent SNPs from each blood metabolite, setting the linkage disequilibrium threshold for clumping to r2 < 0.01, and using a clumping window size of 500 kb while also excluding alleles with palindromic A/T or G/C combinations. Finally, to evaluate the robustness of the selected SNPs, we evaluated the SNP power using F statistics (F = beta2/se2) and the variance explained by IVs [R2 = 2 × MAF × (1-MAF) × (beta/sd)2] (17, 18). A value of F ≥ 10 indicates a lack of weak instrument bias, and IVs with F statistics <10 were excluded as weak (19). The objective of this analysis was to ensure the validity of the IVs employed in the study.
Statistical analysis
This study used the following five methods of MR analysis to examine the association between blood metabolites and IAs: inverse-variance weighted (IVW), weighted median, MR-Egger, weighted mode, and simple mode (20). To address the issue of multiple testing, we employed the Bonferroni correction and set the significance threshold to P < 0.05/n (where n is the number of metabolites finally included in the MR analysis) (21). Suggestive associations were considered when the P value for the uncorrected IVW method was <0.05. When the P value after Bonferroni correction was <0.0001 (0.05 divided by 486), the serum metabolite was considered to be significantly associated with IAs. We performed sensitivity analyses only for metabolites with P < 0.05 for both the IVW method and weighted median method results in the MR analysis. MR-Egger regression analysis was conducted to evaluate potential directional pleiotropy. We used the MR Pleiotropy RESidual Sum and Outlier (MR-PRESSO) global test to detect overall horizontal pleiotropy and eliminated any pleiotropic effects by removing outliers (22). We employed Cochran's Q test to assess heterogeneity among SNPs associated with each blood metabolite. We also conducted a leave-one-out analysis to determine whether a single SNP was responsible for significant results. All statistical analyses were performed using R (version 4.2.2), and the MR and sensitivity analyses were conducted using the two-sample MR and MR-PRESSO R packages.
Results
Selection of IVs
After a series of quality controls, we identified 4,065 independent SNPs associated with 486 blood metabolites. Specific information on all SNPs used as genetic IVs can be found in Supplementary Table 2. The F-values calculated for all IVs were >10, indicating that there were no weak IVs selected from the 486 metabolites.
Effects of genetically determined metabolites on IA
To ensure the robustness of the results, only results with a P < 0.05 for both the IVW method and the weighted median method were included in the final study results (Supplementary Table 3). As shown in Figure 2, we detected five IA-associated, four aSAH-associated, and three UIA-associated metabolites. Among the blood metabolites associated with IA, three metabolites, mannose [odds ratio (OR) = 0.19, 95% confidence interval (CI): 0.07–0.48, PIVW=0.001], theobromine (OR = 0.23, 95% CI: 0.10–0.53, PIVW = 0.001), and 1-arachidonoylglycerophosphocholine (OR = 0.40, 95% CI: 0.17–0.94, PIVW = 0.036), were suggestive associated with a reduced risk of IA, and two metabolites, androsterone sulfate (OR = 1.22, 95% CI: 1.00–1.47, PIVW = 0.046) and X-12644 (OR = 2.41, 95% CI: 1.02–5.72, PIVW = 0.045), were suggestive associated with an increased risk of IA. Specifically, each standard deviation (SD) increase in levels of mannose, theobromine, and 1-arachidonoylglycerophosphocholine was suggestive of an associated reduction in the risk of IA by 81%, 77%, and 60%, respectively. Each SD increase in androsterone sulfate and X-12644 levels was suggestive of an associated increase in the risk of IA by 22% and 141%, respectively.
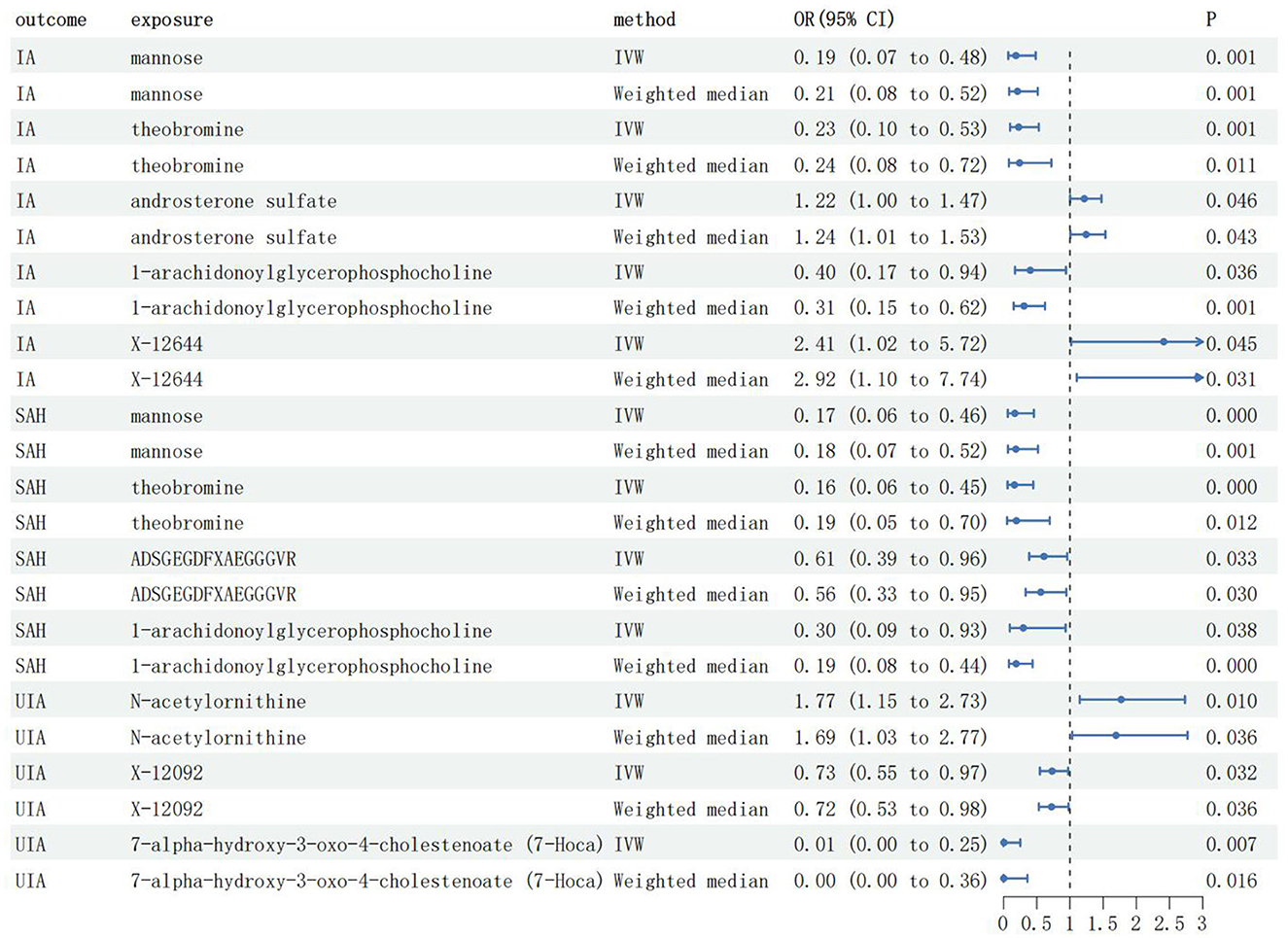
Figure 2. The main results of MR analysis; IA, Intracranial aneurysm; UIA, Unruptured intracranial aneurysm; aSAH, aneurysmal Subarachnoid hemorrhage; IVW, Inverse-variance weighted; OR, Odds ratio; CI, Confidence interval.
Effects of genetically determined metabolites on aSAH
Among the blood metabolites associated with aSAH, four specific metabolites exhibited notable findings. Mannose (OR = 0.17, 95% CI: 0.06–0.46, PIVW < 0.001), theobromine (OR = 0.16, 95% CI: 0.06–0.45, PIVW < 0.001), ADSGEGDFXAEGGGVR (OR = 0.61, 95% CI: 0.39–0.96, PIVW = 0.033), and 1-arachidonoylglycerophosphocholine (OR = 0.30, 95% CI: 0.09–0.93, PIVW = 0.038) were suggestive associated with a reduced risk of aSAH. Specifically, SD increases in mannose, theobromine, ADSGEGDFXAEGGGVR, and 1-arachidonoylglycerophosphocholine levels were suggestive of an associated reduction in the aSAH risk by 83%, 84%, 39%, and 70%, respectively.
Effects of genetically determined metabolites on UIA
Among the blood metabolites associated with UIA, two specific metabolites, X-12092 (OR = 0.73, 95% CI: 0.55–0.97, PIVW = 0.032) and 7-alpha-hydroxy-3-oxo-4-cholestenoate (OR = 0.02, 95% CI: 0.01–0.25, PIVW = 0.007), were suggestive associated with a reduced risk of IA. Conversely, N-acetylornithine (OR = 1.77, 95% CI: 1.15–2.73, PIVW = 0.010) was suggestive associated with an increased risk of UIA. Specifically, SD increases in X-12092 and 7-alpha-hydroxy-3-oxo-4-cholestenoate levels were associated with a 27% and 98% reduction in UIA risk, respectively. Each SD increase in N-acetylornithine levels suggested an association with a 77% increase in RIA risk.
Sensitivity analysis
Cochran's Q test showed no heterogeneity between the relevant SNPs for each serum metabolite (all P > 0.05, Table 1). Additionally, the MR-Egger regression analysis did not reveal any potential for directional pleiotropy, except for the effect of 1-arachidonoylglycerophosphocholine on aSAH (P = 0.028, Table 2). Because there was not a sufficient number of IVs to assess theobromine, it was not possible to test for potential horizontal pleiotropy using MR-PRESSO and global testing methods, and the remaining metabolites showed no horizontal pleiotropy (P > 0.05, Supplementary Table 4). We performed leave-one-out analyses and identified strong effects of the SNP rs174535 on the causal associations of 1-arachidonoylglycerophosphocholine with IA and aSAH. The leave-one-out analysis indicated that other metabolites did not differ significantly in their estimated causal effects on IA, aSAH, and UIA. This finding suggests that no single independent variable was driving the identified causal associations (Supplementary Figure S1). Therefore, we excluded the MR analyses results of 1-arachidonoylglycerophosphocholine on IA and aSAH and those of ADSGEGDFXAEGGGVR on aSAH.
Discussion
We employed available GWAS data and conducted a two-sample MR analysis to examine the causal associations between blood metabolites and IAs. Potential confounding factors were thoroughly addressed through a comprehensive sensitivity analysis, thereby enhancing the reliability and validity of the findings. Notably, this study represents a pioneering attempt to integrate metabolomic and genomic approaches to investigate the causal relationships between blood metabolites and IAs.
In a study of IAs, Tomoki Hashimoto and colleagues highlighted the promising therapeutic implications of dietary daidzein and its metabolite, equol, in averting the occurrence of IAs and the associated SAHs (23). Additionally, Duan et al. conducted a pseudotargeted metabolomics investigation, systematically elucidating the metabolic profiles and associated pathways involved in the progression of IAs. These findings suggest that blood metabolites may be a potential target for preventing and treating IAs.
The findings of our study reveal significant causal associations between nine specific metabolites and IA. Notably, our analysis highlights the potential involvement of mannose and theobromine, primarily known for their anti-inflammatory properties, in the intricate processes underlying the initiation and progression of IA. These results provide valuable insights into the pathogenesis of this condition and suggest the potential therapeutic relevance of targeting these metabolites in the management of IAs (7).
A substantial body of existing research has consistently demonstrated an association between inflammation and the occurrence and progression of aneurysms (24–27). Experimental studies utilizing macrophage chemotactic protein 1 (MCP1) knockout mice have provided compelling evidence supporting the pivotal role of MCP1 in the initiation of aneurysms. Notably, these studies have shown a significant reduction in macrophage infiltration within high-flow-exposed cerebral arteries of MCP1 knockout mice, resulting in a more than 50% decrease in the initiation and formation of IAs (28). The pivotal role of macrophage infiltration in the initiation of IAs has been consistently supported by multiple studies utilizing various experimental approaches. These studies have employed variations of the classic model of induced IA formation and strategies such as clodronate-induced macrophage depletion (29–31) as well as manipulation of macrophage activation through peroxisome proliferator-activated receptor gamma (PPARγ) modulation (32).
Smooth muscle cells within the IA wall contain receptors for various growth factors secreted by macrophages, including transforming growth factor-β and platelet-derived growth factor-B. These growth factors act as stimulators for smooth muscle cell matrix synthesis and proliferation (33). The continuous activation of macrophages, leading to the secretion of proteases, coupled with their ability to stimulate smooth muscle cells may provide an explanation for the progressive expansion of the aneurysm wall. Following initiation, macrophage activation within the aneurysm wall is further enhanced through an autocrine feedback loop. This loop involves the production of prostaglandin E2 by cyclooxygenase 2 (COX2), which subsequently activates the transcription factor nuclear factor kappa B (NF-κB) in other macrophages. Consequently, the expression of both MCP1 and COX2 is increased in these cells (34, 35). This process induces the recruitment of additional macrophages to the aneurysm wall, thereby triggering NF-κB activation within these cells. Moreover, a comprehensive analysis of gene expression at the genome-wide level in both ruptured and unruptured human IA walls revealed upregulation of multiple genes regulated by NF-κB, specifically in ruptured IA walls. These findings strongly support the implication of macrophage-induced NF-κB activation in the remodeling of established human IAs, similar to that observed in experimental models of induced IA initiation and formation (34, 36, 37).
Mannose, an aldohexose sugar, is abundantly present in various fruits and vegetables, including cranberries, peaches, eggplants, and green beans. Blood concentrations of mannose typically range from 20 to 80 μM, and dietary supplementation can increase levels to over 900 μM, all without observable adverse effects (38). This finding underscores the safety profile of mannose as a naturally occurring sugar. Currently, mannose is widely marketed as a dietary supplement for urinary tract infection management because of its ability to hinder bacterial adherence to the urinary tract (39). Furthermore, recent investigations have highlighted the potential antitumor properties of mannose and its ability to mitigate inflammation in inflammatory bowel disease (40, 41). Nevertheless, the therapeutic potential of mannose in the context of IA remains uncertain and requires further investigation.
Recent research has elucidated the therapeutic effects of mannose in alleviating colitis through its actions on intestinal epithelial cells and macrophages. While the metabolic shift toward increased glycolysis is a well-documented feature of inflammatory macrophages, the precise proinflammatory mechanisms associated with glycolysis remain poorly characterized. Prior research has demonstrated that inhibiting glycolysis can suppress the transcription of inflammatory cytokine genes by restricting the activation of upstream transcription factors, including NF-κB (42, 43). Additionally, evidence supports the notion that mannose exerts a transcription-independent inhibitory effect on tumor necrosis factor (TNF)-α production, which coincides with a reduction in intracellular glycerol-3-phosphate levels induced by mannose. As a result, glyceraldehyde-3-phosphate dehydrogenase is released from glycerol-3-phosphate to bind to TNF-α mRNA (41). The underlying hypothesis of our study posits that mannose acts as an anti-inflammatory agent in the context of IAs, potentially through a similar mechanism. There is no direct evidence that mannose is associated with a reduced risk of IA formation and rupture. However, one study previously found that, in the plasma and aneurysmal tissue of abdominal aortic aneurysm patients, in contrast to CD68 (+) mannose receptor (+) macrophages, CD68 (+) mannose receptor (–) macrophages may contribute to the development of oxidative stress, which is associated with abdominal aortic aneurysm formation in humans (44, 45). This finding provides evidence for a mechanism of action by which mannose attenuates oxidative stress injury by binding to macrophages. Interestingly, oxidative stress injury plays a key role in the development and rupture of IAs (46, 47), and this antioxidant mechanism of mannose may likewise play a similar role in the development and rupture of IAs. Consequently, our MR investigation results revealed a positive association between mannose and a decreased risk of both UIAs and ruptured IAs, signifying its clinical significance. Considering the limited understanding of mannose in the pathophysiological mechanisms of IAs, the identification of mannose as a modulator holds profound implications.
Theobromine, chemically known as 3,7-dimethylxanthine, is a methylxanthine alkaloid found primarily in cocoa beans and dark chocolate and belongs to the same class of plant-derived alkaloids as caffeine and theophylline (48). Extensive research has established that theobromine possesses the capability to impede the adipogenic differentiation process in primary adipose-derived 3T3-L1 mouse cells through suppression of adipogenesis-related factors (49, 50). The interaction between adipocytes and macrophages plays a pivotal role in the inflammatory response in vivo (51). Theobromine demonstrated a significant concentration-dependent suppression of lipid accumulation, which was attributed to its ability to decrease the expression of key adipogenic regulators, namely PPARγ and CCAAT/enhancer-binding protein α. Additionally, theobromine exerted antiadipogenic effects by inhibiting AR1 signaling (49, 50). Previous investigations have also provided evidence of the capacity of theobromine to impede the differentiation of preadipocytes into mature adipocytes and reduce the secretion of proinflammatory cytokines, such as MCP1 and interleukin-1β, in the supernatants derived from the interaction between mature adipocytes and macrophages (52). These findings suggest that theobromine has the potential to attenuate proinflammatory compound release, albeit to a partial extent. These findings are in accordance with the outcomes of our MR analysis, indicating a potential role of theobromine in modulating the inflammatory response associated with the development and progression of IAs through inhibition of adipocyte-macrophage interactions. However, the precise underlying mechanism by which theobromine exerts its protective effects against IAs remains unclear and requires further elucidation in future investigations.
An increased risk of IA has been observed in association with elevated androsterone sulfate levels. Androsterone sulfate is a predominant 5-α-reduced androgen metabolite present in serum. Previous investigations have reported markedly increased concentrations of androsterone sulfate in individuals with hyperthyroidism and women exhibiting hirsutism (53, 54). Aberrant endocrine-mediated overproduction of androsterone sulfate has been hypothesized to disrupt the delicate equilibrium between androgens and other hormones, such as estrogen, compromising the protective effects of estrogen against aneurysms. Consequently, the assessment of androsterone sulfate levels holds promise as a potential diagnostic marker, while targeting 5-α-reductase as a therapeutic approach may offer therapeutic benefits for IA management.
Our study identified a suggestive association between the metabolites 7-alpha-hydroxy-3-oxo-4-cholestenoate and N-acetylornithine and UIAs. Nonetheless, the precise underlying mechanisms through which these metabolites contribute to the pathogenesis and progression of unruptured aneurysms will require further investigations of the specific roles and molecular pathways involved.
Limitations
This study has several limitations. First, it is possible that genetic proxies for blood metabolites may affect the risk of IA through alternate mechanisms, thus challenging the assumptions of MR. Second, the study relied mostly on GWAS summary data from individuals of European ancestry, with limited representation from other racial groups, which may have introduced biases in the findings. Third, although some of the blood metabolites have suggestive causal effects on IA, they may have an underlying non-linear relationship with IA, which may not be resolved in MR studies based on summary-level GWAS data. Finally, the study did not account for the higher incidence of IAs in women than in men, which might affect the results. Future research may benefit from conducting sex-specific MR analyses to address this limitation.
Conclusion
The results of this study provide evidence for the potential causal effects of four metabolites on IA, two metabolites on aSAH, and three metabolites on UIA, warranting further investigation into the specific roles of blood metabolites in the development of IA.
Data availability statement
The original contributions presented in the study are included in the article/Supplementary material, further inquiries can be directed to the corresponding authors.
Ethics statement
Ethical review and approval was not required for the study on human participants in accordance with the local legislation and institutional requirements. Written informed consent from the patients/participants or patients/participants' legal guardian/next of kin was not required to participate in this study in accordance with the national legislation and the institutional requirements.
Author contributions
JJ: Writing – original draft. SG: Writing – original draft. DW: Writing – review & editing. XC: Writing – review & editing. YT: Formal analysis, Writing – review & editing. JL: Software, Writing – review & editing. WY: Software, Writing – review & editing. TC: Formal analysis, Writing – review & editing. SY: Data curation, Writing – review & editing. HG: Writing – review & editing. YL: Writing – review & editing.
Funding
The author(s) declare financial support was received for the research, authorship, and/or publication of this article. This work was supported by Beijing Natural Science Foundation (grant no. M22007), the National Natural Science Foundation of China (grant nos. 82271319 and 82171289), and the Natural Science Foundation of Hebei Province of China (grant no. F2020202053).
Acknowledgments
We thank Lisa Kreiner, PhD, from Liwen Bianji, (Edanz) (www.liwenbianji.cn), for editing the English text of a draft of this manuscript.
Conflict of interest
The authors declare that the research was conducted in the absence of any commercial or financial relationships that could be construed as a potential conflict of interest.
Publisher's note
All claims expressed in this article are solely those of the authors and do not necessarily represent those of their affiliated organizations, or those of the publisher, the editors and the reviewers. Any product that may be evaluated in this article, or claim that may be made by its manufacturer, is not guaranteed or endorsed by the publisher.
Supplementary material
The Supplementary Material for this article can be found online at: https://www.frontiersin.org/articles/10.3389/fneur.2023.1268138/full#supplementary-material
References
1. Etminan N, Rinkel GJ. Unruptured intracranial aneurysms: development, rupture and preventive management. Nat Rev Neurol. (2016) 12:699–713. doi: 10.1038/nrneurol.2016.150
2. Brisman JL, Song JK, Newell DW. Cerebral aneurysms. N Engl J Med. (2006) 355:928–39. doi: 10.1056/NEJMra052760
3. Nieuwkamp DJ, Setz LE, Algra A, Linn FHH, de Rooij NK, Rinkel GJE. Changes in case fatality of aneurysmal subarachnoid haemorrhage over time, according to age, sex, and region: a meta-analysis. Lancet Neurol. (2009) 8:635–42. doi: 10.1016/S1474-4422(09)70126-7
4. Chen Y, Wright N, Guo Y, Turnbull I, Kartsonaki C, Yang L, et al. Mortality and recurrent vascular events after first incident stroke: a 9-year community-based study of 0·5 million Chinese adults. Lancet Glob Health. (2020) 8:e580–e90.
5. van Gijn J, Kerr RS, Rinkel GJE. Subarachnoid haemorrhage. Lancet. (2007) 369:306–18. doi: 10.1016/S0140-6736(07)60153-6
6. Gieger C, Geistlinger L, Altmaier E, Hrabé de., Angelis M, Kronenberg F, Meitinger T, et al. Genetics meets metabolomics: a genome-wide association study of metabolite profiles in human serum. PLoS Genet. (2008) 4:e1000282. doi: 10.1371/journal.pgen.1000282
7. Sun K, Zhang X, Li X, Li X, Su S, Luo Y, et al. Plasma metabolic signatures for intracranial aneurysm and its rupture identified by pseudotargeted metabolomics. Clin Chim Acta. (2023) 538:36–45. doi: 10.1016/j.cca.2022.11.002
8. Missori P, de Sousa DA, Ambrosone A, Currà A, Paolini S, Incarbone G, et al. Cotinine levels influence the risk of rupture of brain aneurysms. Acta Neurol Scand. (2022) 146:562–7. doi: 10.1111/ane.13679
9. Smith GD, Ebrahim S. ‘Mendelian randomization': can genetic epidemiology contribute to understanding environmental determinants of disease? Int J Epidemiol. (2003) 32:1. doi: 10.1093/ije/dyg070
10. Davies NM, Holmes MV, Davey Smith G. Reading Mendelian randomisation studies: a guide, glossary, and checklist for clinicians. BMJ. (2018) 362:k601. doi: 10.1136/bmj.k601
11. Davey Smith G, Hemani G. Mendelian randomization: genetic anchors for causal inference in epidemiological studies. Hum Mol Genet. (2014) 23:R89–98. doi: 10.1093/hmg/ddu328
12. Tan J-S, Liu N-N, Guo T-T, Hu S, Hua L. Genetically predicted obesity and risk of deep vein thrombosis. Thromb Res. (2021) 207:16–24. doi: 10.1016/j.thromres.2021.08.026
13. Wang W, Tan J-S, Wang J, Xu W, Bai L, Jin Y, et al. Genetically predicted waist circumference and risk of atrial fibrillation. Chin Med J (Engl). (2023). doi: 10.1097/CM9.0000000000002775
14. Shin S-Y, Fauman EB, Petersen A-K, Krumsiek J, Santos R, Huang J, et al. An atlas of genetic influences on human blood metabolites. Nat Genet. (2014) 46:543–50. doi: 10.1038/ng.2982
15. Bakker MK, van der Spek RAA, van Rheenen W, Morel S, Bourcier R, Hostettler IC, et al. Genome-wide association study of intracranial aneurysms identifies 17 risk loci and genetic overlap with clinical risk factors. Nat Genet. (2020) 52:1303–13. doi: 10.1038/s41588-020-00725-7
16. Skrivankova VW, Richmond RC, Woolf BAR, Yarmolinsky J, Davies NM, Swanson SA, et al. Strengthening the reporting of observational studies in epidemiology using mendelian randomization: the STROBE-MR statement. JAMA. (2021) 326:1614–21. doi: 10.1001/jama.2021.18236
17. Xie J, Huang H, Liu Z, Li Y, Yu C, Xu L, et al. The associations between modifiable risk factors and nonalcoholic fatty liver disease: a comprehensive Mendelian randomization study. Hepatology. (2023) 77:949–64. doi: 10.1002/hep.32728
18. Burgess S, Dudbridge F, Thompson SG. Combining information on multiple instrumental variables in Mendelian randomization: comparison of allele score and summarized data methods. Stat Med. (2016) 35:1880–906. doi: 10.1002/sim.6835
19. Pierce BL, Ahsan H, Vanderweele TJ. Power and instrument strength requirements for Mendelian randomization studies using multiple genetic variants. Int J Epidemiol. (2011) 40:740–52. doi: 10.1093/ije/dyq151
20. Burgess S, Butterworth A, Thompson SG. Mendelian randomization analysis with multiple genetic variants using summarized data. Genet Epidemiol. (2013) 37:658–65. doi: 10.1002/gepi.21758
21. Sedgwick P. Multiple hypothesis testing and Bonferroni's correction. BMJ. (2014) 349:g6284. doi: 10.1136/bmj.g6284
22. Verbanck M, Chen C-Y, Neale B, Do R. Detection of widespread horizontal pleiotropy in causal relationships inferred from Mendelian randomization between complex traits and diseases. Nat Genet. (2018) 50:693–8. doi: 10.1038/s41588-018-0099-7
23. Yokosuka K, Rutledge C, Kamio Y, Kuwabara A, Sato H, Rahmani R, et al. Roles of phytoestrogen in the pathophysiology of intracranial aneurysm. Stroke. (2021) 52:2661–70. doi: 10.1161/STROKEAHA.120.032042
24. Frösen J, Cebral J, Robertson AM, Aoki T. Flow-induced, inflammation-mediated arterial wall remodeling in the formation and progression of intracranial aneurysms. Neurosurg Focus. (2019) 47:E21. doi: 10.3171/2019.5.FOCUS19234
25. Jin J, Duan J, Du L, Xing W, Peng X, Zhao Q. Inflammation and immune cell abnormalities in intracranial aneurysm subarachnoid hemorrhage (SAH): relevant signaling pathways and therapeutic strategies. Front Immunol. (2022) 13:1027756. doi: 10.3389/fimmu.2022.1027756
26. Signorelli F, Sela S, Gesualdo L, Chevrel S, Tollet F, Pailler-Mattei C, et al. Hemodynamic stress, inflammation, and intracranial aneurysm development and rupture: a systematic review. World Neurosurg. (2018) 115:234–44. doi: 10.1016/j.wneu.2018.04.143
27. Texakalidis P, Sweid A, Mouchtouris N, Peterson EC, Sioka C, Rangel-Castilla L, et al. Aneurysm formation, growth, and rupture: the biology and physics of cerebral aneurysms. World Neurosurg. (2019) 130:277–84. doi: 10.1016/j.wneu.2019.07.093
28. Aoki T, Kataoka H, Ishibashi R, Nozaki K, Egashira K, Hashimoto N. Impact of monocyte chemoattractant protein-1 deficiency on cerebral aneurysm formation. Stroke. (2009) 40:942–51. doi: 10.1161/STROKEAHA.108.532556
29. Kanematsu Y, Kanematsu M, Kurihara C, Tada Y, Tsou T-L, van Rooijen N, et al. Critical roles of macrophages in the formation of intracranial aneurysm. Stroke. (2011) 42:173–8. doi: 10.1161/STROKEAHA.110.590976
30. Koseki H, Miyata H, Shimo S, Ohno N, Mifune K, Shimano K, et al. Two diverse hemodynamic forces, a mechanical stretch and a high wall shear stress, determine intracranial aneurysm formation. Transl Stroke Res. (2020) 11:80–92. doi: 10.1007/s12975-019-0690-y
31. Tutino VM, Mandelbaum M, Choi H, Pope LC, Siddiqui A, Kolega J, et al. Aneurysmal remodeling in the circle of Willis after carotid occlusion in an experimental model. J Cereb Blood Flow Metab. (2014) 34:415–24. doi: 10.1038/jcbfm.2013.209
32. Shimada K, Furukawa H, Wada K, Korai M, Wei Y, Tada Y, et al. Protective role of peroxisome proliferator-activated receptor-γ in the development of intracranial aneurysm rupture. Stroke. (2015) 46:1664–72. doi: 10.1161/STROKEAHA.114.007722
33. Frösen J, Piippo A, Paetau A, Kangasniemi M, Niemelä M, Hernesniemi J, et al. Growth factor receptor expression and remodeling of saccular cerebral artery aneurysm walls: implications for biological therapy preventing rupture. Neurosurgery. (2006) 58:3. doi: 10.1227/01.NEU.0000197332.55054.C8
34. Aoki T, Fr?sen J, Fukuda M, Bando K, Shioi G, Tsuji K, et al. Prostaglandin E2-EP2-NF-κB signaling in macrophages as a potential therapeutic target for intracranial aneurysms. Sci Signal. (2017) 10:6037. doi: 10.1126/scisignal.aah6037
35. Aoki T, Nishimura M, Matsuoka T, Yamamoto K, Furuyashiki T, Kataoka H, et al. PGE(2) -EP(2) signalling in endothelium is activated by haemodynamic stress and induces cerebral aneurysm through an amplifying loop via NF-κB. Br J Pharmacol. (2011) 163:1237–49. doi: 10.1111/j.1476-5381.2011.01358.x
36. Aoki T, Kataoka H, Shimamura M, Nakagami H, Wakayama K, Moriwaki T, et al. NF-kappaB is a key mediator of cerebral aneurysm formation. Circulation. (2007) 116:2830–40. doi: 10.1161/CIRCULATIONAHA.107.728303
37. Kurki MI, Häkkinen S-K, Frösen J, Tulamo R, von und zu Fraunberg M, Wong G, et al. Upregulated signaling pathways in ruptured human saccular intracranial aneurysm wall: an emerging regulative role of Toll-like receptor signaling and nuclear factor-κB, hypoxia-inducible factor-1A, and ETS transcription factors. Neurosurgery. (2011) 68:1667–75. doi: 10.1227/NEU.0b013e318210f001
38. Sharma V, Ichikawa M, Freeze HH. Mannose metabolism: more than meets the eye. Biochem Biophys Res Commun. (2014) 453:220–8. doi: 10.1016/j.bbrc.2014.06.021
39. Ala-Jaakkola R, Laitila A, Ouwehand AC, Lehtoranta L. Role of D-mannose in urinary tract infections - a narrative review. Nutr J. (2022) 21:18. doi: 10.1186/s12937-022-00769-x
40. Gonzalez PS, O'Prey J, Cardaci S, Barthet VJA, Sakamaki J-I, Beaumatin F, et al. Mannose impairs tumour growth and enhances chemotherapy. Nature. (2018) 563:719–23. doi: 10.1038/s41586-018-0729-3
41. Xiao P, Hu Z, Lang J, Pan T, Mertens RT, Zhang H, et al. Mannose metabolism normalizes gut homeostasis by blocking the TNF-α-mediated proinflammatory circuit. Cell Mol Immunol. (2023) 20:119–30. doi: 10.1038/s41423-022-00955-1
42. Shen Y, Kapfhamer D, Minnella AM, Kim J-E, Won SJ, Chen Y, et al. Bioenergetic state regulates innate inflammatory responses through the transcriptional co-repressor CtBP. Nat Commun. (2017) 8:624. doi: 10.1038/s41467-017-00707-0
43. Moreno-Fernandez ME, Giles DA, Oates JR, Chan CC, Damen MSMA, Doll JR, et al. PKM2-dependent metabolic skewing of hepatic Th17 cells regulates pathogenesis of non-alcoholic fatty liver disease. Cell Metab. (2021) 33:18. doi: 10.1016/j.cmet.2021.04.018
44. Boytard L, Spear R, Chinetti-Gbaguidi G, Acosta-Martin AE, Vanhoutte J, Lamblin N, et al. Role of proinflammatory CD68(+) mannose receptor(-) macrophages in peroxiredoxin-1 expression and in abdominal aortic aneurysms in humans. Arterioscler Thromb Vasc Biol. (2013) 33:431–8. doi: 10.1161/ATVBAHA.112.300663
45. McCormick ML, Gavrila D, Weintraub NL. Role of oxidative stress in the pathogenesis of abdominal aortic aneurysms. Arterioscler Thromb Vasc Biol. (2007) 27:461–9. doi: 10.1161/01.ATV.0000257552.94483.14
46. Laaksamo E, Tulamo R, Liiman A, Baumann M, Friedlander RM, Hernesniemi J, et al. Oxidative stress is associated with cell death, wall degradation, and increased risk of rupture of the intracranial aneurysm wall. Neurosurgery. (2013) 72:109–17. doi: 10.1227/NEU.0b013e3182770e8c
47. Sheinberg DL, McCarthy DJ, Elwardany O, Bryant J-P, Luther E, Chen SH, et al. Endothelial dysfunction in cerebral aneurysms. Neurosurg Focus. (2019) 47:E3. doi: 10.3171/2019.4.FOCUS19221
48. Martínez-Pinilla E, Oñatibia-Astibia A, Franco R. The relevance of theobromine for the beneficial effects of cocoa consumption. Front Pharmacol. (2015) 6:30. doi: 10.3389/fphar.2015.00030
49. Mitani T, Watanabe S, Yoshioka Y, Katayama S, Nakamura S, Ashida H. Theobromine suppresses adipogenesis through enhancement of CCAAT-enhancer-binding protein β degradation by adenosine receptor A1. Biochim Biophys Acta Mol Cell Res. (2017) 1864:2438–48. doi: 10.1016/j.bbamcr.2017.09.017
50. Jang YJ, Koo HJ, Sohn E-H, Kang SC, Rhee D-K, Pyo S. Theobromine inhibits differentiation of 3T3-L1 cells during the early stage of adipogenesis via AMPK and MAPK signaling pathways. Food Funct. (2015) 6:2365–74. doi: 10.1039/C5FO00397K
51. Cho U, Kim B, Kim S, Han Y, Song YS. Pro-inflammatory M1 macrophage enhances metastatic potential of ovarian cancer cells through NF-κB activation. Mol Carcinog. (2018) 57:235–42. doi: 10.1002/mc.22750
52. Fuggetta MP, Zonfrillo M, Villivà C, Bonmassar E, Ravagnan G. Inflammatory microenvironment and adipogenic differentiation in obesity: the inhibitory effect of theobromine in a model of human obesity in vitro. Mediators Inflamm. (2019) 2019:1515621. doi: 10.1155/2019/1515621
53. Rosenfeld RS, Kream J, Hellman L. Androsterone sulfate concentrations in plasma in hypo- and hyperthyroidism. J Clin Endocrinol Metab. (1976) 42:464–7. doi: 10.1210/jcem-42-3-464
Keywords: intracranial aneurysm, blood metabolites, Mendelian randomization, risk factors, single-nucleotide polymorphisms
Citation: Jiang J, Gui S, Wei D, Chen X, Tang Y, Lv J, You W, Chen T, Yang S, Ge H and Li Y (2023) Causal relationships between human blood metabolites and intracranial aneurysm and aneurysmal subarachnoid hemorrhage: a Mendelian randomization study. Front. Neurol. 14:1268138. doi: 10.3389/fneur.2023.1268138
Received: 28 July 2023; Accepted: 27 November 2023;
Published: 14 December 2023.
Edited by:
Jiang-Shan Tan, Chinese Academy of Medical Sciences and Peking Union Medical College, ChinaReviewed by:
Linshuoshuo Lyu, Yale University, United StatesXin Feng, Southern Medical University, China
Yang Zhao, Peking University, China
Copyright © 2023 Jiang, Gui, Wei, Chen, Tang, Lv, You, Chen, Yang, Ge and Li. This is an open-access article distributed under the terms of the Creative Commons Attribution License (CC BY). The use, distribution or reproduction in other forums is permitted, provided the original author(s) and the copyright owner(s) are credited and that the original publication in this journal is cited, in accordance with accepted academic practice. No use, distribution or reproduction is permitted which does not comply with these terms.
*Correspondence: Huijian Ge, 474025129@qq.com; Youxiang Li, liyouxiang@mail.ccmu.edu.cn
†These authors have contributed equally to this work and share first authorship