- 1Wuxi People’s Hospital Affiliated to Nanjing Medical University, Wuxi Children’s Hospital, Wuxi, China
- 2Department of Digestive, Affiliated Children’s Hospital of Jiangnan University, Wuxi, China
Ulcerative colitis (UC) has become a worldwide public health problem, and the prevalence of the disease among children has been increasing. The pathogenesis of UC has not been elucidated, but dysbiosis of the gut microbiota is considered the main cause of chronic intestinal inflammation. This review focuses on the therapeutic effects of probiotics on UC and the potential mechanisms involved. In animal studies, probiotics have been shown to alleviate symptoms of UC, including weight loss, diarrhea, blood in the stool, and a shortened colon length, while also restoring intestinal microecological homeostasis, improving gut barrier function, modulating the intestinal immune response, and attenuating intestinal inflammation, thereby providing theoretical support for the development of probiotic-based microbial products as an adjunctive therapy for UC. However, the efficacy of probiotics is influenced by factors such as the bacterial strain, dose, and form. Hence, the mechanisms of action need to be investigated further. Relevant clinical trials are currently lacking, so the extension of animal experimental findings to clinical application requires a longer period of consideration for validation.
1. Introduction
UC is a type of inflammatory bowel disease (IBD) that manifests as non-specific chronic inflammation of the colonic mucosa with alternating cycles of remission and exacerbation. The annual incidence of UC has risen in recent years, notably among children (Kuenzig et al., 2022). The prevalence of childhood UC varies worldwide, with European countries having the highest prevalence (15.0/100,000), and the prevalence in North America is 10.6/100,000 (Sýkora et al., 2018). However, data regarding the disease from developing and underdeveloped countries is scarce. Approximately 25% of patients with UC develop symptoms before the age of 18, and the prevalence in children over the age of 10 is clearly increasing. One report showed that the age 10–17 subgroup of children made the highest contribution to the increased prevalence (Ye et al., 2020). This points to an increased burden of UC on the health care system as well as patients and caregivers in the future. Abdominal pain, diarrhea, bloody stools, weight loss, and a decrease in bone density and muscle strength are characteristics of UC. All of these symptoms can impede the growth and development of children (Agrawal et al., 2021). Moreover, repeated hospitalizations and disruptions in learning and life can cause psychological changes such as irritability and even depression, which are major obstacles to healthy development (Reed et al., 2021). Eventually, approximately 15% of patients with UC will require surgery within 20 years of diagnosis (Eisenstein, 2018). Additionally, the younger the age at diagnosis, the worse the prognosis and the greater the likelihood of colonic resection and colon cancer, which significantly increase the health risks of children with UC (Agrawal et al., 2021). Therefore, interventions for UC in children are warranted.
The pathogenesis of UC is not entirely known, although it is assumed to be the result of a combination of genetic predisposition, environmental factors, microbial infections, immune system dysregulation, and gut microbiota disruption. This suggests that UC is an immune-related inflammatory disease caused by disturbances in the intestinal environment (Figure 1). Analyzes of the frequency of UC in twins and family members have revealed that being a first-degree relative of an individual with UC is a better predictor of the development of UC than any other environmental factor. Genome-wide association studies revealed 163 single nucleotide polypeptides related to UC and nucleotide binding oligomerization genes (Agrawal et al., 2022; Liu et al., 2023). Environmental factors include smoking, frequent consumption of fast food, antibiotic misuse and abuse, early exposure to antibiotics, and otitis media (Agrawal et al., 2021). Recently, an increasing number of studies have shown dysbiosis of the gut microbiota as the central mechanism in the development of UC (Mizoguchi et al., 2020; Metwaly et al., 2022). A study reporting that pre-existing signs of the imbalance of gut microbiota can be noticed prior to the active phase supports this theory (Kumar and Kumar, 2022). Clostridium difficile causes a decrease in beneficial bacteria, a loss of immune function, and increased intestinal permeability. In addition, some studies have shown that transplanting fecal microbiota restores gut ecology, providing a new treatment strategy for UC (Costello et al., 2019; Kelly and Ananthakrishnan, 2019). Lack of protection from beneficial bacteria along with chronic exposure to harmful bacteria causes homeostatic dysbiosis and immunological disturbance, sustaining intestinal inflammation (Oka and Sartor, 2020). Increased numbers of pathogenic microorganisms damage the intestinal epithelial mucus layer, and pathogens that destroy the mucus layer impair the intestinal barrier. After barrier rupture, antigens penetrate the intestinal epithelium, activating downstream mechanistic target of rapamycin (mTOR) via the toll-like receptor (TLR)4 and phosphatidylinositol 3 kinase/protein kinase B (PI3K/AKT) signaling pathways and leading to the production of inflammatory factors, including tumor necrosis factor (TNF)-α, interleukin (IL)-6, and IL-1β (Zhou et al., 2018). In response to antigenic (mostly bacterial) activation, T cells develop into T helper (Th)2, Th9, and Th17 cells, which produce IL-13, IL-9, and IL-17, respectively (Neurath, 2019). Additionally, innate lymphoid cells in the intestine are activated, which then produce TNF-α, interferon (INF)-γ, IL-4, IL-5, IL-17, and IL-22 (Schulz-Kuhnt et al., 2021). Increased levels of inflammatory factors promote neutrophil migration and cell permeability, aggravating the already defective barrier function (Martini et al., 2017), which may also be linked to increased nuclear factor kappa-B (NF-κB) transcription (Peng et al., 2021). Disturbances in the gut microbiota in UC are mostly manifested by a loss of diversity and dominance of Firmicutes and Bacteroidetes, as well as an increase in the number of Desulfovibrio subspecies (Vich Vila et al., 2018; Kumar and Kumar, 2022). This aberrant colony structure reduces levels of short-chain fatty acids (SCFAs), an essential metabolite of bacteria, leading to defective B cell maturation and differentiation as well as lower levels of regulatory cells (Treg), further weakening the mucosal defense (Lavelle and Sokol, 2020). Moreover, bacteria take advantage of the dysbiosis, further perpetuating the vicious cycle. Previous research has revealed complex relationships between inflammatory substances and immune cells, and scientists may uncover new mechanisms of action that are currently unknown.
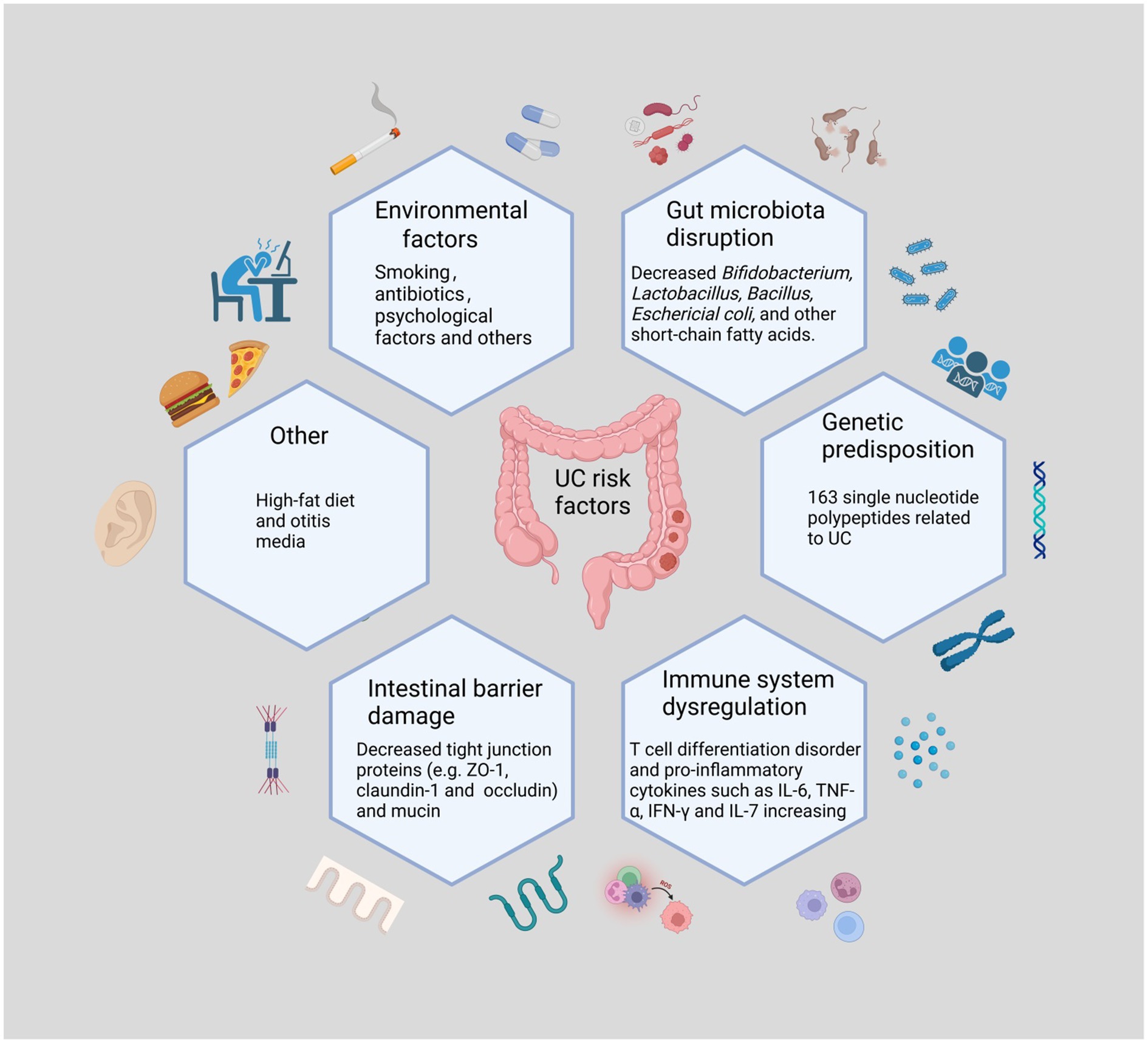
Figure 1. The Main Causes of Ulcerative Colitis. UC may occur as a result of the interaction of the imbalance of gut microbiota, immune disorders, genetic susceptibility, and other factors with external stimuli. The loss of beneficial intestinal flora and the increase of harmful flora disrupt the intestinal environment. The decrease of intestinal epithelial intercellular tight junction proteins as well as mucins also causes damage to the intestinal barrier. Psychological factors and poor lifestyle habits are also risk factors for the development of UC. Various harmful factors trigger intestinal immune disorders and release large amounts of pro-inflammatory factors, causing intestinal inflammation.
UC is prone to recurrence after remission. Since there is no cure for UC, treatments are aimed at alleviating symptoms, maintaining remission and improving quality of life. Mainly, treatments can be divided into pharmaceutical and surgical based on the European Crohn’s and Colitis Organization’s treatment recommendations. Biologics, 5-aminosalicylic acid (5-ASA), immunosuppressive drugs, anti-integrin and anti-interleukin antibodies, and topical or systemic steroid hormones are the mainstays of pharmacological therapy (Turner et al., 2018a,b; Raine et al., 2022). Although 5-ASA induces and sustains remission of UC, oral 5-ASA can cause side effects such as stomach discomfort, diarrhea, and nasopharyngitis (Cochrane Gut Group et al., 2020). Additionally, UC is a chronic inflammatory disease that affects patients with impaired gut barrier function. The long-term administration of corticosteroids carries a high risk of complications, such as cataract, glaucoma, and adrenal insufficiency (Valdes and Sobrin, 2020). Biologics are frequently administered to patients who are refractory to previous pharmacological therapies or who are unable to endure severe side effects (Segal et al., 2021). Immunosuppressive drugs and biologics are also advised for individuals with extraintestinal symptoms (Feuerstein et al., 2020; Baumgart and Le Berre, 2021). An increasing number of patients are opting for the early use of immunosuppressive or biological drugs, or both, to increase the chances of remission (Chapman et al., 2020). When administered intermittently, acute infusion reactions occur in 5–10% of cases, and long-term use of immunosuppressive agents can cause side effects such as pancreatitis, leukopenia, nausea, and allergic reactions, as well as increase the risk of infection, heart failure, and cancer (Lemaitre et al., 2017; Singh et al., 2021). Among the biologics, the Food and Drug Administration (FDA) has approved infliximab and adalimumab for pediatric patients with UC, while ustekinumab can only be used in adult patients. Meanwhile, vedolizumab and golimumab are still being studied for use in children (Hyams et al., 2022). Acute, severe, or refractory UC all necessitate surgical intervention such as colorectal resection, fistulae, or flap advancement (Spinelli et al., 2022). Surgical removal of the water-absorbing colorectum can result in severe diarrhea and cause postoperative complications such as intestinal adhesions and obstruction, anastomotic fistula, and abdominal infection. Meanwhile, early surgery in children can affect their growth and development and reduce their quality of life. Although there are several medications available for UC, many patients are ineffective or develop secondary failure during treatment. Due to the above reasons, safe and effective treatments for UC remain limited. Recently, treatment with probiotics has been proposed to regulate the gut microbiome in UC. The gut microbiota plays an essential role in the maintenance of host homeostasis and immunomodulation. Disruptions of inter-species function in gut microbiota contribute to the leading cause of inflammatory bowel disease (Zhang et al., 2022), and UC was demonstrated to be strongly associated with microbial dysbiosis (Kobayashi et al., 2020). The mechanisms of action of probiotics for UC have been extensively researched recently. Hence, probiotics present a potential therapy for pediatric patients with UC due to their safety, low cost, and ease of administration.
Given the pathophysiological importance of the gut microbiome in UC, the hypothesis that altering the gut microbiota may be a viable way to treat UC is gaining attention (Paramsothy et al., 2017). Based on a cross-sectional study, among patients with a high disease activity index (DAI), frequent defecation, severe abdominal pain, and a history of related surgery, the frequency of probiotics being prescribed increased, indicating that probiotics are now being recognized in the treatment of UC (Kim and Cheon, 2022). Although the current evidence base supporting the use of probiotics in patients with UC is thin, probiotics are often widely used as adjunctive therapy and are often recommended by physicians, and they are generally considered to be safe (Abraham and Quigley, 2017). A 2020 Cochrane Review that included 14 studies indicated that probiotics can induce clinical remission during the active period and prevent recurrence in UC patients (Cochrane IBD Group et al., 2020). Additionally, a meta-analysis indicated that VSL#3 resulted in the most significant improvement in UC, followed by Lactobacillus and E. coli (Shen et al., 2014). However, probiotics have not yet demonstrated a meaningful benefit in maintaining clinical remission in patients with UC (Iheozor-Ejiofor et al., 2020). In children, an analysis of three trials revealed that the combination of Lactobacillus with VSL#3 (composed of four different strains of Lactobacillus spp., three strains of Bifidobacterium spp., and a sole strain of Streptococcus spp.) probiotics had significant effects in children with UC (Ganji-Arjenaki and Rafieian-Kopaei, 2018). Another previous study showed that the addition of VSL#3 to regular treatment markedly reduced relapse rates compared with placebo (21.4% vs. 73.3%) when delivered within a year of induction of remission in 29 children with UC (Miele et al., 2009). Unfortunately, there is a lack of clinical studies on the therapeutic effects of probiotics in UC, with most studies being at the animal experimental stage, and there are no compliance randomized controlled trials of probiotics in children with UC; therefore, recommendations for the use of probiotics are not available (Su et al., 2020). Probiotics like Bifidobacterium and Lactobacillus are recommended as adjuvant therapy in China for adults with mild-to-moderate UC to maintain remission. The European Society for Parenteral Enteral Nutrition also affirms the induction of remission by specific strains of bacteria in patients with mild to moderate UC (Bischoff et al., 2022). Probiotics are highly tolerated and cause few side effects; therefore, it is worth promoting them as a potentially novel treatment alternative for patients with UC (Cochrane IBD Group et al., 2020; Selvamani et al., 2022). Meanwhile, caution should be exercised when applying the results of animal experiments to the treatment of children with UC.
2. Therapeutic effects of probiotics on UC
The International Scientific Association of Probiotics and Prebiotics panel defined probiotics in 2014 as “live microorganisms which when administered in adequate amounts confer a health benefit on the host” (Hill et al., 2014). With a better understanding of the function of the gut microbiota, various probiotics, such as Bifidobacterium, Lactobacillus, and Bacillus, have been proven to be useful to the human body. They colonize the gut and correct the aberrant bacterial composition of the host by increasing the number of symbiotic bacteria (Wieërs et al., 2019). In various investigations, it was shown that probiotics, particularly Bifidobacterium and Lactobacillus, significantly decreased as the gut microflora in IBD patients became more aberrant (Van der Waal et al., 2019). Pathological signs of UC primarily include epithelial destruction of the colon. Probiotics improve intestinal barrier integrity by metabolizing SCFAs, tryptophan, and other chemicals to increase the production of mucin and tight junction (TJ) proteins in intestinal epithelial cells (Cochrane IBD Group et al., 2020; Lavelle and Sokol, 2020). Recent evidence suggests that probiotics can regulate intestinal immunity, prevent excessive activation of intestinal immune cells, reduce levels of pro-inflammatory factors such as IL-6, INF-γ, TNF-α, and IL-1β, increase levels of anti-inflammatory factors IL-10 and TGF-β, and inhibit the expression of the NF-κB signaling pathway to improve intestinal inflammation (Blacher et al., 2017; Popov et al., 2021). Several modern single-strain probiotics have shown promising results in animal models. A mixed probiotic called VSL#3, which is a combination of eight beneficial bacteria strains and is frequently used in clinical studies, is effective in treating UC in children and adults (Miele et al., 2009; Lee et al., 2012; Oliva et al., 2012). However, some clinical findings imply that probiotics have no significant impact on the maintenance of UC remission (Wildt et al., 2011). Furthermore, while probiotics are effective in preventing the development of acute storage pouchitis and the recurrence of chronic storage pouchitis in adults, research on children with storage pouchitis is lacking. There are different viewpoints on the use of probiotics in the treatment of pediatric UC; some guidelines claim that probiotics are not required, whereas others propose using VSL#3 or Escherichia coli Nissle1917 as adjuvant therapy to improve symptoms in pediatric patients with UC (Cheng et al., 2021). Undeniably, different probiotic strains have value in the treatment of UC, but their efficacy and safety require in-depth research due to a paucity of large-scale case–control studies and data on long-term clinical efficacy. In animal model experiments, the application of probiotics had multiple improvements in the symptoms of colitis in mice (Figure 2). Therefore, this review collected animal studies (Tables 1, 2) on the treatment of UC by a variety of potentially beneficial bacteria over the last 5 years, providing a reference for the clinical use of probiotics for the treatment of UC.
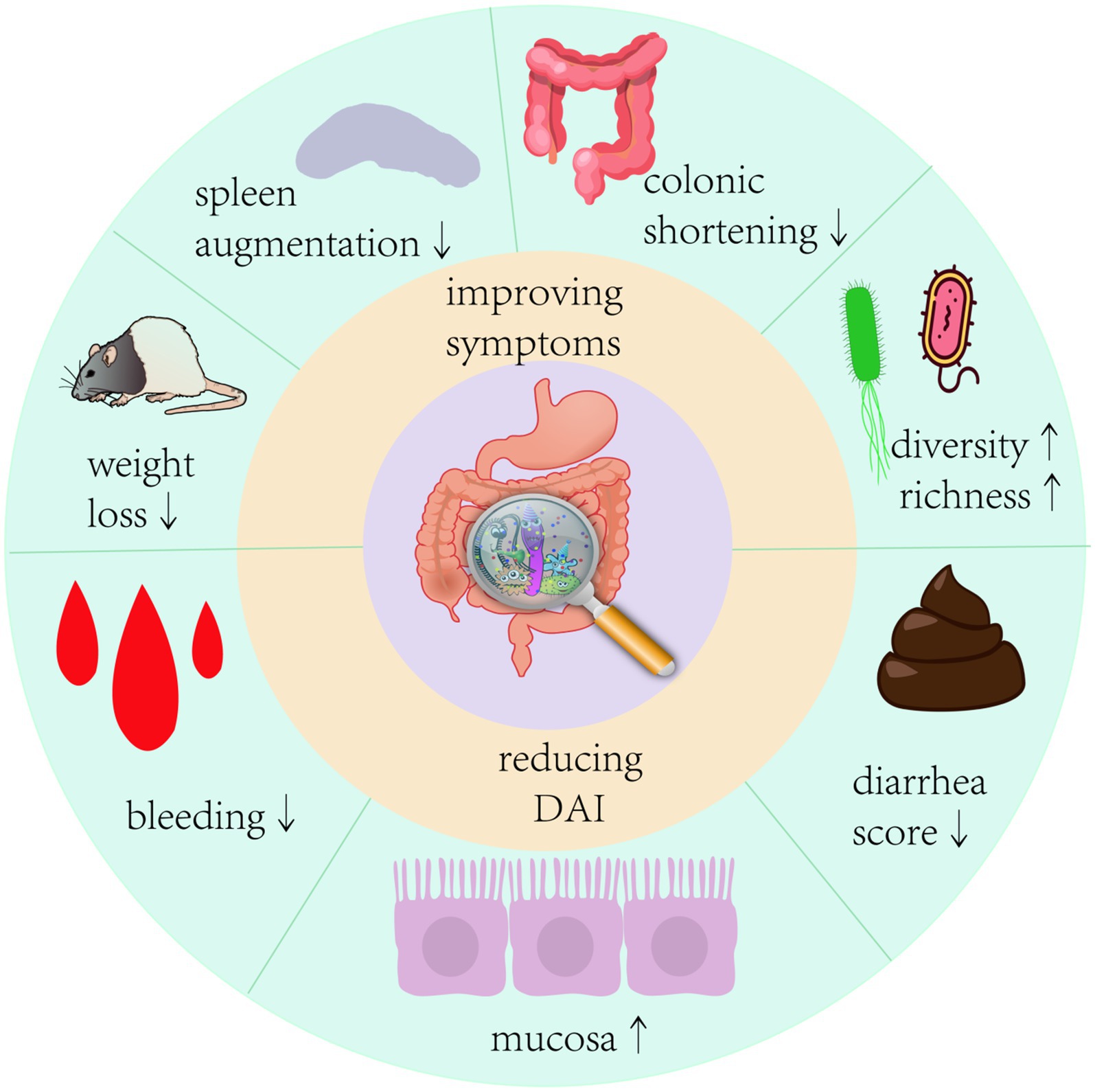
Figure 2. Therapeutic effects of probiotics on colitis in mice Probiotic intervention given to mice with colitis model showed significant improvements in colonic shortening, mucosal damage, weight loss, a decrease in disease activity index, and a significant improvement in the structure of the intestinal flora of the mice.
2.1. Effects of Bifidobacterium spp. on UC
The genus Bifidobacterium belongs to the gram-positive bacteria of the phylum Actinobacteria and is one of the first microorganisms to colonize the intestine (O’Callaghan and Van Sinderen, 2016). Bifidobacterium is also immunotolerant in humans and not subject to rejection (Yao et al., 2021). Hence, several species of this genus constitute the main group of probiotics (Fontana et al., 2013; Mianzhi and Shah, 2017). Exopolysaccharides (EPS), SCFAs, and conjugated linoleic acid (CLA), which are produced during metabolism, act on intestinal epithelial cells and play a role in regulating intestinal homeostasis (Hughes et al., 2017) by improving intestinal barrier function and modulating the intestinal mucosal immune system and inflammatory response (Dodd et al., 2017). Numerous animal studies have shown that Bifidobacterium can increase the population of the dominant flora, which is absent in UC, and ameliorate symptoms of dextran sulfate sodium (DSS)-induced colitis. Furthermore, conventional medications mixed with probiotics can enhance therapeutic efficacy and improve the remission rate in UC. However, because each Bifidobacterium strain attenuates the inflammatory response differently and responds differently to cytokines (Choi et al., 2022), we believe that the function of probiotics should be subdivided based on strain characteristics. When considering the status of patients to achieve the optimal therapeutic effect, the appropriate probiotic should be selected.
2.1.1. Bifidobacterium longum alleviates oxidative stress
Bifidobacterium longum is one of the most prevalent bacteria in the intestine. The main metabolites of B. longum are SCFAs and CLA (Albert et al., 2019), which alleviate colonic inflammation by increasing antioxidant activity and regulating the production of reactive oxygen species, leading to reduced oxidative stress (Yao et al., 2021). Numerous animal studies have shown that B. longum can improve experimental colitis. B. longum strains YS108R, 51A, and LC67 can attenuate DSS and 2,4,6-trinitrobenzene sulfonic acid (TNBS)-induced colonic damage by strengthening the mucosal barrier and regulating the composition of the gut microbiota (Jang et al., 2018; Yan et al., 2019; Abrantes et al., 2020; Yan et al., 2020; Bi et al., 2022). Furthermore, mice in the B. longum CCFM681 intervention group outperformed those in the CCFM760 and CCFM642 groups in terms of the improvement in mucosal barrier function and reduction of mucosal barrier levels, with the results of the analysis of bacterial products revealing that the improvements were related to the amount of CLA produced by B. longum; higher CLA levels were associated with greater colitis remission (Chen et al., 2021). In cell experiments, B. longum strains CCFM752, CCFM1149, CCFM10, and LTBL16 attenuated intestinal inflammatory responses by increasing intracellular catalase activity, lowering NADPH oxidase activation, and improving intracellular antioxidant capacity (Huang et al., 2020; Yan et al., 2020; Wang et al., 2021; Yao et al., 2021). Notably, ESP produced by B. longum 35,624 induced the secretion of relatively low levels of cytokines from human dendritic cells and attenuated the accumulation of IL-17 in the intestine (Schiavi et al., 2016). Similarly, B. longum YS108R produces ropy exopolysaccharides that suppress the immunological response, reducing intestinal inflammation (Yan et al., 2020). Additionally, Chen (Chen et al., 2019) investigated the effects of similar doses (4 × 109 CFU/dose) of B. longum HB5502 and VSL#3 on colitis in mice. The study found that both the two strains relieved colonic inflammation, reduced serum inflammatory factors, and increased tight ligin expression, demonstrating that there is no significant difference in the results between a single strain of B. longum HB5502 and a mixed probiotic containing B. longum. However, whether this suggests that B. longum plays a major role in probiotics requires further investigation. Furthermore, another selenium-rich strain of B. longum, namely DD98, ameliorates UC-induced selenium deficiency by producing selenoproteins, which also possess substantial anti-inflammatory and immunomodulatory activities to improve DSS-induced intestinal inflammation in mice (Hu et al., 2022). Additionally, B. longum strains NK173, NK151, and NK175 alleviate stress fatigue, depression, and symptoms of UC by regulating the expression ratio of pro- and anti-inflammatory cytokines and gut microbiota byproducts (e.g., lipopolysaccharide, LPS) (Yoo et al., 2022), making them potential probiotics for the treatment of patients with UC who also have neuropsychiatric disorders. Meanwhile, the combination of B. longum CECT 7894 and infliximab improved the therapeutic effect of both drugs, with greater improvement of symptoms in the B. longum group compared to the infliximab-only group (Xiao et al., 2022). A prior clinical trial found that combining B. longum trisporus with mesalazine improved the therapeutic effect of drugs used for UC (Jiang et al., 2020), inferring that trisporus could be a safe and convenient supplementary treatment option for patients who are resistant to traditional medications. Overall, B. longum may be a useful adjuvant biologic drug in the treatment of UC (Li et al., 2023).
2.1.2. Bifidobacterium breve enhances the intestinal mucosal barrier and reduces levels of inflammatory factors
Bifidobacterium breve is a non-budding, non-motile, gram-positive, specialist anaerobic bacteria. Like B. longum, B. breve can lower the intestinal inflammatory response via EPS. Moreover, the improvement was greater with B. breve H4-2 than with H9-3, which may be related to the higher production of EPS by H4-2 (Niu et al., 2022). Similarly, B. breve strains CCFM683 and BJCP1M6B reduced the levels of TNF-α and IL-6, significantly increased the levels of mucin-2 (MUC2) and cupped cells, upregulated the expression of TJ proteins, and improved DSS-induced epithelial cell apoptosis; in contrast, neither B. breve strains FHLJDQ3M5 nor M2CF22M7 showed these effects (Chen et al., 2021). B. breve YH68 reduced the population of C. difficile and toxin levels in feces in an animal study, and B. breve CCFM1025 improved symptoms of major depression by modulating tryptophan metabolism in a randomized controlled trial (Yang et al., 2022), providing another therapeutic option for patients with UC with concomitant symptoms of depression. However, the role of B. breve in maintaining UC remission was not significant, and there was no significant difference in relapse-free survival between the B. breve and placebo groups after 48 weeks of oral administration of fermented yogurt containing primarily B. breve (Matsuoka et al., 2018). The results contradict those of a prior animal study, and the difference may be related to the probiotic dose, delivery route, and probiotic production process. However, this does not rule out the beneficial effects of B. breve for the treatment of UC.
2.1.3. Bifidobacterium animalis subsp. lactis has potential as a supplement for the treatment of UC due to its anti-inflammatory properties
Bifidobacterium lactis is a gram-positive anaerobic bacterium found in the intestines of most animals. B. lactis adheres to the epithelial mucosa in vast numbers and is an important component of a healthy gut microbiota (Masco et al., 2004). Regarding the efficacy of B. lactis on UC, mice with DSS- or TNBS-induced colitis treated with B. lactis strains A6, BB12, and 5,764 showed significant improvements in intestinal barrier function and immunomodulation (Chae et al., 2018; Hrdý et al., 2020; Wang et al., 2022). Furthermore, B. lactis exerts its anti-inflammatory properties through the activation of peripheral blood mononuclear cells and increasing forkhead box P3 (FOXP3) gene expression to increase TGF-β levels (Shakurnia et al., 2019). Notably, B. lactis BL-99 not only reduced intestinal inflammation in UC but also alleviated colitis-related lung injury by altering SCFA production and inflammatory cell ratios (Nan et al., 2023). Preliminary evidence suggests that B. lactis is another potentialz option for reducing inflammation in UC as well as improving extraintestinal manifestations in patients with UC.
2.1.4. Bifidobacterium bifidum supplementation is effective in treating UC
Bifidobacterium bifidum is the dominant gram-positive anaerobic bacterium in the human intestine and plays a significant role in the prevention of gastrointestinal dysfunction (Andresen et al., 2020). Animal experiments have suggested that the role of B. bifidum FJSWX19M5 in improving TNBS-induced chronic colitis is achieved through repairing gut barrier damage and enhancing Tregs (Qu et al., 2023). B. bifidum strains FL-276.1 and FL-228.1 improve immune function by activating the aryl hydrocarbon receptor (AHR) (Cui et al., 2022). Additionally, improvement in the symptoms of colitis brought about by B. bifidum B1628 was accompanied by obvious gut microbiota remodulation (Feng et al., 2022). Furthermore, B. bifidum BGN4-SK alleviated DSS-induced colitis by producing antioxidant enzymes and reducing pro-inflammatory cytokine production. However, B. bifidum BGN4-pBESIL10 had little effect on IL-10 production and the improvement of colitis (Kang et al., 2022). Interestingly, B. bifidum improved the TJ barrier function in a strain-specific manner, with B. bifidum BB1 demonstrating the greatest improvement (Al-Sadi et al., 2021a). Furthermore, heat-inactivated and lysozyme-treated B. bifidum strains FJSWX19M5 and BGN4 also improved symptoms of colitis, but the degree of improvement was not similar to that of live bacteria. However, lysozyme-treated B. bifidum BGN4 maintained the intestinal mucosal barrier function better than live bacteria (Lee et al., 2022; Qu et al., 2023), which may be related to the stronger adhesion of the inactivated strain. In summary, B. bifidum is a potent probiotic that can improve colitis in a variety of ways, including by regulating gut microbiota, improving intestinal barrier function, and modulating immunity; however, the effects of B. bifidum are affected by the type of strain and organism. Nevertheless, B. bifidum is a promising probiotic that should undergo further investigation for its specific therapeutic properties.
2.1.5. Bifidobacterium adolescentis treats UC by balancing the gut microbiota
As a gram-positive anaerobic bacterium, B. adolescentis is the dominant bacterium in the intestinal tract of young people and plays an important role in the treatment of constipation, anxiety, depression, and colitis (Jang et al., 2019). Current research shows that B. adolescentis Reuter 1963 can reverse dysbiosis of the gut microbiota caused by peptidoglycan recognition protein 3 deficiency (Ghadimi et al., 2021). Oral administration of B. adolescentis ATCC15703 relieves symptoms of chronic colitis in mice by regulating the immune response, making it a potential probiotic (Fan et al., 2021). B. adolescentis IF1-03 with high-molecular-weight EPSs can activate dendritic cells or macrophages, and both rely on the toll-like receptor 2-ERK/p38 MAPK signaling cascade to skew Treg/Th17 cells to protect mice from DSS-induced colitis (Yu et al., 2019). B. adolescentis has an obvious antioxidant effect, but the SOD level in the intestinal tract of model mice was not measured in the above experiments. Hence, the effects of antioxidants on improving colitis could not be evaluated. Future studies should further explore whether B. adolescentis has other properties that may improve UC.
2.1.6. Bifidobacterium infantis alleviates symptoms of UC by reducing the inflam-matory response
Bifidobacterium infantis is the dominant bacteria in breastfed infants; thus, it has a high abundance in infancy (Mattarelli et al., 2008). It is acid- and bile-resistant, is strongly adhesive, does not destroy mucus, is non-invasive, and does not harm the intestinal mucosa (Ewaschuk et al., 2008). B. infantis FJSYZ1M3 can remarkably reduce the DAI, limit weight loss and colonic shortening via alteration of the gut microbiota, maintain the integrity of the intestinal barrier, and modulate levels of inflammatory cytokines (Li et al., 2023). B. infantis also enhances the development of cluster of differentiation (CD) 4+ T cells into Tregs and also increases the expression of IL-10 and TGF-β1, subsequently reducing the inflammatory response in the gut (Zhou et al., 2022). Additionally, co-administration of B. infantis ATCC 15697 with xylooligosaccharide demonstrated additional efficacy in protecting against colonic damage due to DSS-induced colitis in mice (Sheng et al., 2020). Moreover, B. infantis can enhance the development of the host immune system and maintain elevated IgA and IgG titers in infancy and at 2 years (Huda et al., 2019). Significantly lower levels of pro-inflammatory cytokines on postnatal days 40 and 60 in EVC001-fed infants have been reported, providing a new strategy to improve intestinal inflammation during developmental stages (Henrick et al., 2019). B. infantis also exerts anti-inflammatory effects through various pathways. However, the effectiveness of B. infantis in the treatment of UC in children remains unclear. B. infantis is abundant in the infant gut and may play a major role in inhibiting the development of UC in this population; hence, its effects need to be further explored.
2.2. Effects of Lactobacillus spp. on UC
Lactobacillus is a genus of gram-positive microbes in fermented foods that colonize the human digestive tract in vast numbers; they are also the first probiotics (De Melo Pereira et al., 2018). Lactobacillus is distinguished by its capacity to convert glucose, lactose, and galactose into lactic acid, which reduces the intestinal pH, and produce bacteriocins, hydrogen peroxide, and diacetyl to inhibit the growth of other bacteria. Lactobacillus has been extensively studied, and its use in the treatment of UC has been recognized (Garbacz, 2022). SCFAs, bacteriocins, and EPS of Lactobacillus can exert powerful immunomodulatory effects, and its cell wall can attenuate inflammation and oxidative interference and enhance antioxidant defense (Chorawala et al., 2021). Additionally, Lactobacillus can increase levels of catalase (CAT) and superoxide dismutase (SOD) while decreasing those of reactive oxygen species (ROS) at the genetic level (Jin et al., 2020), thus reducing intestinal oxidative stress. Lactobacillus is the most extensively utilized genus of probiotic products, and it has been successfully used clinically in the adjuvant treatment of patients with UC.
2.2.1. Lactobacillus plantarum has strain specificity in the treatment of UC
Lactobacillus plantarum is the most common flora in the gut and is a parthenogenic anaerobic bacterium found in vegetables and fermented fruit juices. L. plantarum can supplement human vitamin B by synthesizing folic acid, increasing the stability and absorption of B1, B6, and B12 in the intestine, and secreting antibacterial active peptides to inhibit the growth of many gram-positive bacteria (Yin et al., 2018). L. plantarum is a probiotic used in the treatment of UC; in animal studies, oral administration of several L. plantarum strains, including AR17-1, Q7, Y44, L15, 12, N13, CCFM8610, CAU1055, HNU082, ZS62, ZDY2013, LC27, 06CC2, CBT LP3, and MTCC 5690, had a preventive effect on the development of UC (Jang et al., 2018; Wang et al., 2018, 2021, 2022; Zhou et al., 2018; Choi et al., 2019; Pradhan et al., 2019; Kim et al., 2020; Liu et al., 2020; Tanaka et al., 2020; Yu et al., 2020; Gao et al., 2021; Hao et al., 2021; Pan et al., 2021; Wu et al., 2022a,b). Additionally, a high concentration (1 × 109 CFU/mL) of L. plantarum strains YS3 and CQPC06 had superior effects compared to a low concentration (1 × 108 CFU/mL) of the same strains (Zhang et al., 2018; Shi et al., 2020), indicating that the effect of probiotics may be dose-dependent. Studies have shown that the efficacy of L. plantarum is strain-specific (Liu et al., 2022). For example, L. plantarum strains CAU1055, which has a strong CLA synthesis ability; AR326, which has strong adhesion; and CCFM242, which is rich in zinc, better relieve symptoms of colitis than other strains (Choi et al., 2019; Wang et al., 2019; Zhai et al., 2019). Meanwhile, L. plantarum strains NCIMB8826 and LM0419, which are unable to synthesize bacteriocins, showed no protective effect on mice with TNB-induced colitis (Yin et al., 2018). In addition, EPSs plays an important role in improving the intestinal barrier and gut microbiota. For example, L. plantarum NCU116 EPSs regulate colonic epithelial regeneration, and L. plantarum YW11 enhances the amount of SCFAs. However, the relevant mechanisms remain obscure but may relate to stimulating the signal transducer and activator of transcription 3 (STAT3) signaling pathway (Yunyun Jiang, 2018; Zhou et al., 2021; Liu et al., 2022). These characteristics may provide guidance in determining the beneficial strains that may be used to produce probiotics. In addition to differences between strains, different forms of the same strain have different efficacy in treating colitis. Studies have found that the AB-1 and SS-128 strains with the autoinducer-2 defect can reduce colon inflammation more significantly than wild-type strains (Qian et al., 2022). Additionally, intervention with L. plantarum strains C2 (IMAU10216) and C3 (IMAU70095) 21 days before the induction of colitis in an animal model resulted in less severe symptoms, indicating a preventive effect of L. plantarum on colitis development (Khan et al., 2022). L. plantarum has been extensively studied at the phenotypic, molecular, and genetic levels, and the results have proven that L. plantarum is a powerful and promising probiotic. Its usefulness should be confirmed by future clinical trials.
2.2.2. Lactobacillus rhamnosus can restore the gut microbiota, improve gut barrier function, and decrease levels of pro-inflammatory cytokines
Although L. rhamnosus was discovered only in 1983, it has been the most studied and thoroughly researched Lactobacillus among all probiotics due to its various roles in the adjuvant treatment of intestinal diseases. L. rhamnosus strains CY12, ZFM231, M9, MTCC-5897, LDTM 7511, SHA113, and L. rhamnosus GG (LGG) restore the gut microbiota, improve gut barrier function, and improve DSS-induced colitis by downregulating LPS-induced inflammatory cytokines (Son et al., 2019; Fatmawati et al., 2020; Jia et al., 2020; Yeo et al., 2020; Kaur et al., 2021; Pang et al., 2021; Xu et al., 2021; Wan et al., 2022; Zheng et al., 2022). It has been demonstrated that the EPS produced by L. rhamnosus plays a significant role in biofilm formation, and as a result, it may successfully repair the injured intestinal mucosa (Capurso, 2019). L. rhamnosus FBB81 improves hydrogen peroxide-induced inflammation by enhancing the intestinal epithelial barrier in Caco-2 cells (Fatmawati et al., 2020). A study showed that L. rhamnosus HM0539 inhibited the distal NF-κB signaling pathway via TLR4 to attenuate the LPS-induced inflammatory response, with a concentration of 108 CFU/mL having the most significant effect (Li et al., 2020). Additionally, combinations of L. rhamnosus with other agents have also been studied. Samat Kozhakhmetov et al. (Kozhakhmetov et al., 2022) mixed L. rhamnosus with food-grade horse milk and administered the combination to mice with DSS-induced colitis, resulting in a significant improvement in UC symptoms. Similarly, L. rhamnosus 1.0320 with inulin and LGG combined with targose significantly improved symptoms of colitis in mice (Son et al., 2019; Liu et al., 2020). Inulin and tagatose are prebiotics that promote the growth of probiotic bacteria in the intestine. The results suggest that the adherence and dose of probiotics influence the digestive system. Given that the disturbances of the gut microbiota in UC are manifested by the alteration of the quantity and structure of multiple bacteria, simultaneous administration of multiple probiotics may be more effective in restoring the balance of the gut microbiota than administration of a single strain. In clinical trials, 20 patients with UC were given oral LGG for 1 week, resulting in increased concentrations of LGG in the colon, demonstrating the adhesion properties of LGG (Pagnini et al., 2018). However, the observation period in that study was only 1 week; the gut microbiota may not have changed significantly during that period. Additionally, the observed increase in the number of bacteria may have been caused by the oral administration of LGG. Another clinical trial found that 4 weeks of oral administration of a probiotic complex (based on L. rhamnosus NCIMB 30174) resulted in a significant decrease in fecal calprotectin levels and a significant improvement in clinical symptoms in patients with UC (Bjarnason et al., 2019). A previous randomized controlled trial demonstrated the long-term effects and safety of LGG in patients with UC (ZOCCO et al., 2006). These findings demonstrate that various L. rhamnosus strains, all of which improve UC, have been verified in human in vivo investigations and are now recommended probiotics in clinical practice. In the last 5 years, L. rhamnosus has been an effective treatment for UC in animal experiments; however, clinical trials in humans are relatively lacking and outdated. Therefore, future research needs to explore other strains by using data of strains with excellent laboratory efficacy in clinical trials.
2.2.3. Lactobacillus acidophilus has potential for treating UC due to its anti-inflammatory and gut barrier-protective properties
Lactobacillus acidophilus is a gram-positive bacterium that not only produces significant amounts of lactic acid to limit the growth of other dangerous bacteria but is also bile-tolerant, making it a candidate strain for probiotics (Usman and Hosono, 1999). Animal studies have provided the foundation for the treatment of UC by L. acidophilus, including ATCC 4356, BIO5768, KBL402, KBL409, and XY27 (Hu et al., 2020; Kim et al., 2021; Hrdý et al., 2022; Li et al., 2022), which all play an effective role in improving gut microbiota, maintaining the intestinal barrier, and lowering levels of inflammatory factors. L. acidophilus evidently increased levels of SCFAs, inhibited the NLRP3 inflammasome, and facilitated autophagy to improve UC. Notably, live L. acidophilus produces better effects on UC rats than heat-inactivated L. acidophilus (Li et al., 2022). However, the improvement in UC symptoms is strain-specific. L. acidophilus strains NCFM and FAHWH11L56 improved DSS-induced colitis and increased levels of IL-10 and IL-17 in the colon by altering the CCL2/CCR2 and CCL3/CCR1 axes. The potential alleviating effect of L. acidophilus NCFM, CCFM137, and FAHWH11L56 on colitis may be related to the fact that they have an intact gene cluster for the synthesis of EPS (Huang et al., 2022). However, L. acidophilus CCFM137 had no therapeutic effects. In contrast, L. acidophilus FGSYC48L79 aggravates colitis by increasing the population of harmful bacteria in the gut (Li et al., 2022). Additionally, L. acidophilus can extend the lifespan of mice treated with DSS and reduce the severity of colitis by activating M2 macrophages in peritoneal cavity cells and Th2 and Treg cells in splenocytes. Notably, L. acidophilus improves UC by modulating the gut microbiota composition and amino acid and oligosaccharide metabolic pathways. The combination with 5-ASA did not affect the pharmacokinetics of L. acidophilus, providing strong evidence for its safety (Li et al., 2022). Overall, specific strains of L. acidophilus may potentially be used to improve UC. Furthermore, different strains of L. acidophilus have varying degrees of effectiveness, which may guide the production of probiotic formulations. The focus of future research should shift from animal studies to human trials to provide new therapy choices for juvenile UC.
2.2.4. Lactobacillus reuteri is effective in treating pediatric UC
Lactobacillus reuteri is a probiotic found in all vertebrate and mammalian intestines and is one of the few microbial species known to live in the human stomach. L. reuteri strongly adheres to the intestinal mucosa and is the only strain that can efficiently eliminate Helicobacter pylori (Saviano et al., 2021; Liang et al., 2022). L. reuteri also plays a key role in the treatment of colitis. L. reuteri ATCC PTA 4659 improved colitis clinically and morphologically in mice (Liu et al., 2022). L. reuteri NK33 and NK99 not only significantly attenuated symptoms of colitis but also prevented the occurrence and development of anxiety and depression in mice (Jang et al., 2019). Additionally, pretreatment with L. reuteri strains 4,659 (human origin) and R2LC (murine origin) thickened the intestinal mucus and improved DSS-induced colitis in mice (Ahl et al., 2016). Meanwhile, L. reuteri R28 showed better colonization than L. plantarum AR17-1 (Ahl et al., 2016). L. reuteri DSM 17938 increased levels of tryptophan metabolites and purine nucleoside adenosine in neonatal mice as well as increased their tolerance to inflammatory stimuli (Liu et al., 2019). However, the mice in that experiment did not have colitis. Hence, it is unclear whether a reduction in inflammation can prevent the development of UC. L. reuteri 1 enhances intestinal epithelial barrier function and lowers the inflammatory response induced by Enterotoxigenic Escherichia coli K88 via suppressing the myosin light-chain kinase signaling pathway in IPEC-J2 cells (Gao et al., 2022). Moreover, L. reuteri FN041 improves dyslipidemia and repairs mucosal-barrier damage caused by a high-fat diet, and it can also affect the diurnal variation of the gut microbiota (Li et al., 2019). Notably, perinatal mice supplemented with a combination of L. reuteri and L. johnsonii reduce the incidence of colitis in the neonatal period (Krishna et al., 2022), implying the preventive effect of L. reuteri on UC and providing a foundation for the prevention of UC through oral probiotics in children with risk factors. Previous studies have confirmed that L. reuteri has multifaceted effects on improving the symptoms of colitis. Further clinical research on L. reuteri is needed to explore its use in the treatment of children with UC.
2.2.5. Lactobacillus gasseri treats UC by modulating the immune response and gut microbiota
Lactobacillus gasseri tolerates low pH and bile salt environments and is strongly adherent, which provides the foundation for its successful colonization of the human intestine. This results in a variety of benefits via antimicrobial activity, bacteriocin production, and immunomodulation by innate and adaptive systems (Selle and Klaenhammer, 2013). L. gasseri 4 M13 fermented with protein and galactose regulates the systemic inflammatory response and improves the intestinal epithelial barrier (Jeong et al., 2022). L. gasseri G098 modulates host immunity and the gut microbiome to improve colitis symptoms in mice (Zhang et al., 2022). L. gasseri NK109 improves gut dysbiosis and alleviates symptoms of both colitis and depression (Yun et al., 2020). L. gasseri RW2014 modulates the metabolism of bile acid and the composition of the gut microbiota (Li et al., 2022). Furthermore, co-administration of L. gasseri KBL697 and infliximab has a synergistic effect on treating colitis in mice by decreasing levels of pro-inflammatory cytokines (Han et al., 2022). L. gasseri M1 increases levels of SCFAs to repair intestinal barrier damage caused by DSS in mice (Cheng et al., 2023). Moreover, L. gasseri can inhibit the expression of the TNF-α-converting enzyme in host cells to suppress the release of TNF and IL-6 (Gebremariam et al., 2019). However, the precise function of L. gasseri is mostly unknown and requires further research. Nevertheless, L. gasseri has anti-inflammatory properties that improve gut health. However, there is limited evidence that L. gasseri is effective in the treatment of pediatric UC.
2.2.6. Lactobacillus paracacei maintains and improves intestinal barrier function
Lactobacillus paracacei is a gram-positive parthenogenic anaerobic bacterium with high acid and bile salt tolerance that can enhance immunity. L. paracacei NTU101 strengthens antioxidant capabilities to protect mice from DSS-induced colitis (Chen et al., 2019). L. paracacei R3 significantly attenuates pathological damage and symptoms of colitis by regulating Th17/Treg cell balance (Huang et al., 2021). Additionally, L. paracacei-derived extracellular vesicles also reduce the expression of pro-inflammatory cytokines by augmenting the pathway of endoplasmic reticulum stress (Choi et al., 2020). Meanwhile, L. paracacei BD5115 promotes the proliferation of intestinal epithelial cells (Qiao et al., 2022). Notably, neonatal administration of L. paracacei N1115 prevents intestinal inflammation in adulthood in mice (Xun et al., 2022). A randomized controlled clinical trial has shown that oral administration of L. paracacei CBA L74-fermented formula could enhance the immune system, microbiota, and metabolome maturation in infants (Roggero et al., 2020) and that L. paracacei BD5115 may help repair intestinal damage. In summary, L. paracacei exhibits protective effects on the intestine, especially in maintaining the stability of the intestinal barrier. However, more studies are needed to clarify whether L. paracacei can improve symptoms of UC by improving intestinal barrier function.
2.2.7. Lactobacillus johnsonii treats colitis by decreasing the level of pro-inflammatory cytokines
According to the FDA, L. johnsonii is a naturally occurring strain in the human digestive tract. L. johnsonii prevents colonic shortening and spleen augmentation and attenuates colonic hyperplasia by reducing levels of inflammatory factors in mouse colitis models (Zhang et al., 2021). L. johnsonii improved experimental colitis by promoting the conversion of native macrophages into CD206+ macrophages and releasing IL-10 via the TLR1/2-STAT3 pathway (Jia et al., 2022). Moreover, L. johnsonii repairs the TJ of Caco-2 cells damaged by hydrogen peroxide and enhances barrier function and integrity (Bai et al., 2022). In a piglet Salmonella model with diarrhea, L. johnsonii L531 improved enteritis by removing damaged mitochondria (Xia et al., 2020). Taken together, L. johnsonii has significant potential for treating UC due to its anti-inflammatory properties. However, there is scarce evidence related to the treatment of UC in children using L. johnsonii; hence, further research is needed to confirm its efficacy.
2.2.8. Lactobacillus kefiranofaciens improves intestinal homeostasis in piglets
Lactobacillus kefiranofens is a gram-positive parthenogenic anaerobic bacterium that generates EPS derived from Kefir grains. The precise categorization of L. kefiranofens is unknown (Chen et al., 2017). However, L. kefiranofens ZW18 modulates the gut microbiota (Zhao et al., 2022). Additionally, L. kefiranofens JKSP109 alleviates inflammation, prevents colorectal carcinogenesis, and reduces the DAI in mice with colitis (Zhao et al., 2022). Furthermore, L. kefiranofens BS15 enhances intestinal immunity and the gut microbiota, resulting in an improved diarrhea index in piglets (Xin et al., 2020). Some studies showed that environmental factors, such as heat, cold, acid, and bile salts, could affect the activity of L. kefiranofens; hence, encapsulation of L. kefiranofens must be performed to preserve its beneficial effects (Wang et al., 2015; Chen et al., 2017). Although L. kefiranofens can potentially improve intestinal homeostasis and is a potential probiotic, future studies need to explore its role in maintaining intestinal homeostasis.
2.2.9. Lactobacillus helveticus enriches the gut microbiota and increases levels of anti-inflammatory cytokines
Lactobacillus helveticus is a gram-positive bacterium with high proteolytic activity. Its ability to synthesize EPS can reduce the number of harmful bacteria such as Clostridium perfringens and increase the number of parabacteria to enhance the ability of symbiotic bacteria in the intestine to produce SFAs (Wang et al., 2022). L. helveticus KLDS 1.8701 has antibacterial, antioxidant, and immunomodulatory capacity in DSS-induced colitis mouse models (Shi et al., 2021). L. helveticus ASCC 511, an intestinal commensal high in guanine (L-citrulline), dramatically ameliorates DAI, reduces colonic tissue damage, and reduces levels of pro-inflammatory markers in mice (Ho et al., 2022). Additionally, oral intake of L. helveticus NS8 significantly inhibits the activation of NF-κB and upregulates IL-10 (Rong et al., 2019). Although current research on L. helveticus does not focus on its use for the treatment of UC, its potential as a probiotic should be explored further given its important function in altering the gut microbiota and increasing levels of anti-inflammatory cytokines.
2.2.10. Lactobacillus fermentum improves intestinal inflammation and alleviates symptoms of UC
Lactobacillus fermentum is a gram-positive bacterium with significant acid-producing capacity. L. fermentum also has high acid tolerance and can inhibit pathogens by producing antimicrobial peptides. In humans, L. fermentum may potentially improve metabolic and immune diseases because it can significantly improve the gut microbiota (Molina-Tijeras et al., 2021). Several strains may improve DSS-induced colitis in mice. L. fermentum MTCC 5689, KBL374, KBL375, and a hybrid strain containing L. fermentum L930BB all had positive effects on the remission of colitis without causing any serious side effects in mice (Paveljšek et al., 2018; Pradhan et al., 2019). L. fermentum F-B9-1 protects the intestinal barrier and has shown anti-inflammatory potential by alleviating DSS-induced experimental UC in mice, and the EPS from the bacterium act as active components to inhibit inflammation by reducing the levels of IL-1β and IL-6 (Pradhan et al., 2019). L. fermentum HFY-06 ameliorates the pathological damage induced by DSS by balancing the ratio of anti- and pro-inflammatory cytokines (Liu et al., 2022). Similarly, L. fermentum ZS-40 has anti-inflammatory effects (Chen et al., 2021). It is probable that after establishing efficacy in human clinical trials, it can be safely used as a biological therapy to improve inflammation in UC.
2.2.11. Lactobacillus coryniformis has antioxidant properties
Lactobacillus coryniformis is a gram-positive bacterium that is primarily found in the respiratory and reproductive systems of humans. L. coryniformis can be used in human food (Lara-Villoslada et al., 2007); surprisingly, it is frequently utilized as a vaccine adjuvant. L. coryniformis MXJ32 improves intestinal barrier function, enhances beneficial gut microbiota, and reduces levels of pro-inflammatory factors; however, it has not yet been tested on colitis models (Wang et al., 2022). Meanwhile, L. coryniformis NA-3-derived EPS has the ability to scavenge free radicals and is therefore expected to be used as an antioxidant in the treatment of patients with ulcerative colitis (Xu et al., 2020). The specific function of L. coryniformis remains unknown and requires additional exploration. Although L. coryniformis has anti-inflammatory effects and improves gut health, evidence that L. coryniformis is effective in the treatment of UC disease is limited.
2.2.12. Lactobacillus curvatus has anti-inflammatory effects
Lactobacillus curvatus is a gram-positive bacterium found primarily in the urinary tract and has high antioxidant capability. EPS-producing L. curvatus strains can prevent the formation of Salmonella enterica serovar Eteritidis biofilm and effectively limit the colonization of pathogenic bacteria (Redondo et al., 2017). In animal investigations, blood levels of IL-6, TNF-R1, TNF-R2, and TNF-α were considerably reduced in mice administered with L. curvatus BYB3, which improved DSS-induced intestinal inflammation (Wang et al., 2022). Meanwhile, L. curvatus GH5L demonstrates antioxidant effects (Düz et al., 2020). Current studies suggest that L. curvatus has anti-inflammatory and antioxidant properties, but there is no evidence that it can be used to improve symptoms of UC. Because L. curvatus is not abundant in the intestine, more animal and clinical trials are needed to determine how effective oral L. curvatus is in treating UC and whether it has harmful effects.
2.2.13. Lactobacillus delbrueckii improves the intestinal barrier and regulates immunity
Lactobacillus delbrueckii is a gram-positive bacterium that has received little attention as an adjuct for the treatment of diseases. However, L. elbrueckii reduces LPS-induced damage of the intestinal epithelium in piglets and improves mucosal barrier function (Düz et al., 2020). Furthermore, in mice with colitis, L. elbrueckii improved symptoms aggravated by alcohol (Cannon et al., 2022). However, whether this is attributed to the ability of L. elbrueckii to counteract the effects of alcohol or the influence of other actions is uncertain. Oral administration of L. elbrueckii can improve intestinal integrity by strengthening the intestinal structure and TJ while increasing antioxidant activity via the TLR-nuclear factor erythroid 2-related factor 2 signaling pathway in piglets (Cannon et al., 2022). L. elbrueckii also improves gut immunity in suckling piglets by activating dendritic cells (Peng et al., 2022). Additionally, L. elbrueckii modulates immunity through different mechanisms while also having anti-inflammatory effects. Unfortunately, the therapeutic effect of L. elbrueckii on UC remains unclear. L. elbrueckii may be a natural probiotic that inhibits the development of UC.
2.3. Effects of other probiotics on UC
Bacillus spp. is found in all living species and the environment, and it has considerable applications in agriculture, industry, medicine, and health. Bacillus spp. is resistant to external damage and suited for gastrointestinal digestion, storage, and survival. Bacillus amyloliquefaciens improves symptoms of TNBS-induced colitis in mice by attenuating the expression of pro-inflammatory cytokines (Khalifa et al., 2022). Bacillus cereus enhances intestinal barrier function and modulates the gut microbiota to improve symptoms in mice with colitis (Sheng et al., 2021). Bacillus smithii XY1 has anti-inflammatory properties that can attenuate the inflammatory response (Huang et al., 2021). Bacillus subtilis improves colitis in experimental UC mice by maintaining the integrity of the intestinal barrier and suppressing inflammatory responses (Chung et al., 2021; Zhang et al., 2021). Previous studies have demonstrated the therapeutic effects of Bacillus spp. in mice with colitis. Bacillus is a potential candidate probiotic for the treatment of UC, but more clinical trials are needed to support its use for UC.
Pediococcus is mainly found in fermented plant materials and pickled vegetables. Pediococcus pentosaceus showed protective effects on the intestinal tract. Pediococcus pentosaceus belongs to the genus Pediococcus, which can ferment glucose to produce lactic acid. Pediococcus pentosaceus CECT 8330 regulates immunity and the gut microbiota to improve DSS-induced colitis in mice (Dong et al., 2022). Pediococcus pentosaceus LI05 modulates immunological profiles, the gut microbiota, and SCFA levels in mice (Bian et al., 2020). Oral administration of Pediococcus pentosaceus SMM914 can activate the Nrf2-Keap1 antioxidant signaling pathway to increase antioxidant capacity in piglets (Bian et al., 2020). Pediococcus pentosaceus improves symptoms of colitis in animal experiments and could be a candidate probiotic for the treatment of pediatric UC. However, the specific functions of other strains of Pediococcus in UC remain unclear and require further investigation.
Escherichia coli Nissle1917 (EcN) belongs to the non-pathogenic gram-negative bacteria of the Enterobacteriaceae family (Schultz, 2008). Currently, EcN has been used for the treatment of UC with considerable efficacy. A randomized controlled trial verified that 5-ASA combined with EcN can improve the quality of life of patients with UC and induce colonoscopic remission (Park et al., 2022). EcN can repair and maintain the integrity of the intestinal epithelium, regulate the host immune response, and modulate the gut microbiota (Schlee et al., 2007). The role of EcN in the treatment of UC is well established, and future research should focus on the use of bioengineering to improve the stability of gene expression and enhance the therapeutic efficacy of EcN.
2.4. Effects of multi-strain probiotics on UC
Apart from a single strain, we also discuss the effectiveness of simultaneous combinations of probiotics, including two or more strains. The mixed preparation of B. longum Bif10 and B. breve Bif11 increased anti-inflammatory marker levels and SCFAs, ameliorating symptoms of colitis in mice (Sharma et al., 2023). In addition, a mixture of L. rhamnosus NCIMB 30174, L. plantarum NCIMB 30173, L. acidophilus NCIMB 30175, and Enterococcus faecium NCIMB 30176 decreased intestinal inflammation and reduced fecal calprotectin in adults with UC (Bjarnason et al., 2019). A mixture of L. johnsonii IDCC9203, L. plantarum IDCC3501, and B. lactis IDCC4301 reduced intestinal histological damage, such as loss of goblet cells, immune cell infiltration in the mucosa, and submucosal and crypt destruction (Je et al., 2018). Moreover, mixed lactobacilli had superior anti-inflammatory effects compared with single-strain treatments (Shi et al., 2021). The probiotic product Bifico, composed of B. longum, L. acidophilus, and Enterococcus faecalis, increased the expression of TJ proteins and the number of Tregs (Zhang et al., 2018). Additionally, oral Bifico enhanced the efficacy of mesalazine and maintained the UC in remission (Fan et al., 2019). Other related mixed microbial preparations have also achieved positive effects in animal tests (Li et al., 2022). Furthermore, mixed probiotics can significantly improve the quality of life of patients (Kamarli Altun et al., 2022). Therefore, complex probiotics may potentially be used to relieve symptoms of UC. However, the effectiveness of combined probiotics should be interpreted cautiously. In an animal experiment, consumption of Bifico might exacerbate the damage of colonic tissue when the mucosal barrier is impaired (Zhang et al., 2018). Therefore, we believe that blended probiotics are not appropriate for individuals with UC with severe symptoms and intestinal mucosal tissue destruction. Moreover, the effects of probiotics depend on both strains and dose; therefore, the proportion of each strain in mixed probiotics and the dose of probiotics can influence their effects, which is a problem that needs to be solved in the future.
The above extensive animal studies have explored the role of potential probiotics in the treatment of UC, providing us with additional therapeutic options. However, to clarify whether these probiotics can treat pediatric UC, the findings from animal studies need to be applied to clinical trials, which is the direction of our future exploration. Due to the poor controllability and low follow-up rate of pediatric UC patients, clinical trials on probiotics for pediatric UC have been scarce. As shown in Table 3, we found only three relevant clinical studies about children with UC and added the clinical studies in adults with UC, hoping to provide useful reference data for the role of probiotics in the treatment of pediatric UC. Based on previous clinical studies in adults, the probiotic most commonly used in clinical studies is currently VSL#3 (a probiotic including eight strains: Lactobacillus casei, L. plantarum, L. acidophilus, L. delbrueckii subsp. bulgaricus, Bifidobacterium longum, B. breve, B. infantis, and Streptococcus salivarius subsp). Supplementation of conventional therapy with oral VSL#3 can induce and maintain remission in active UC by modulating the gut microbiota structure and improving the intestinal mucosal barrier. The increased remission rate and significantly lower relapse rate at follow-up within the period of VSL#3 administration in both adult and child patients with UC demonstrate the effective therapeutic effect of probiotics. In addition, the combination of Bifidobacterium trivium capsules with mesalazine significantly reduced DAI and clinical symptom scores in patients with UC while decreasing the level of inflammatory factors and increasing the level of IL-10, which has an inhibitory effect on inflammation (Agraib et al., 2022). In a study in which patients with UC received probiotic therapy for 2 years, it was noted that long-term administration of probiotics could replace glucocorticoids in the treatment of mild to moderate UC (Palumbo et al., 2016). In addition to oral administration, probiotic rectal administration using enemas can also achieve relief of UC symptoms (Matthes et al., 2010; D’Incà et al., 2011). However, it is not clear which mode of administration provides the best effect. Some patients with UC undergo total proctocolectomy with ileal pouch anal anastomosis (IPAA); pouchitis is a common postoperative complication, and there are many studies showing the beneficial role of probiotics in the prevention and treatment of pouchitis (Veereman-Wauters, 2003; Gionchetti et al., 2007; Pronio et al., 2008). The application of probiotics (La-5) and Bifidobacterium (Bb-12) after IPAA can increase the number of intestinal Lactobacillus and Bifidobacterium in patients, reduce involuntary bowel movements, abdominal cramps, and endoscopic scores (Laake et al., 2005), and it has also been shown that VSL#3 can improve joint pain in UC patients with extraintestinal manifestations (Karimi, O., et al., 2005). During all trials, no probiotic-related side effects were reported in patients, demonstrating the good safety profile of probiotics. It is evident from these clinical studies that probiotics have good adjuvant therapeutic effects and are more effective in inducing mild to moderate UC. The positive results of adult studies are inspiring and informative for pediatric studies and bring hope to pediatric patients with UC. However, children are not the epitome of adults, and caution should be exercised when drawing on adult-related research results. Additionally, many of the current trials are pilot studies; to further investigate the role of probiotics in the treatment of UC in children, more large samples and high-quality randomized controlled trials deserve to be investigated.
3. Potential mechanisms of probiotics in the treatment of UC
As shown in Figure 3, the mechanism of probiotics for UC treatment is three-fold: regulating gut microbiota homeostasis, improving intestinal barrier function, and modulating the intestinal immune response. The three are interrelated and interact with each other to promote the remission of UC in terms of pathology and symptoms. Additionally, probiotics’ unique characteristics depend on the strain level; therefore, we list detailed information on the mechanism of action of different strains in Tables 4, 5.
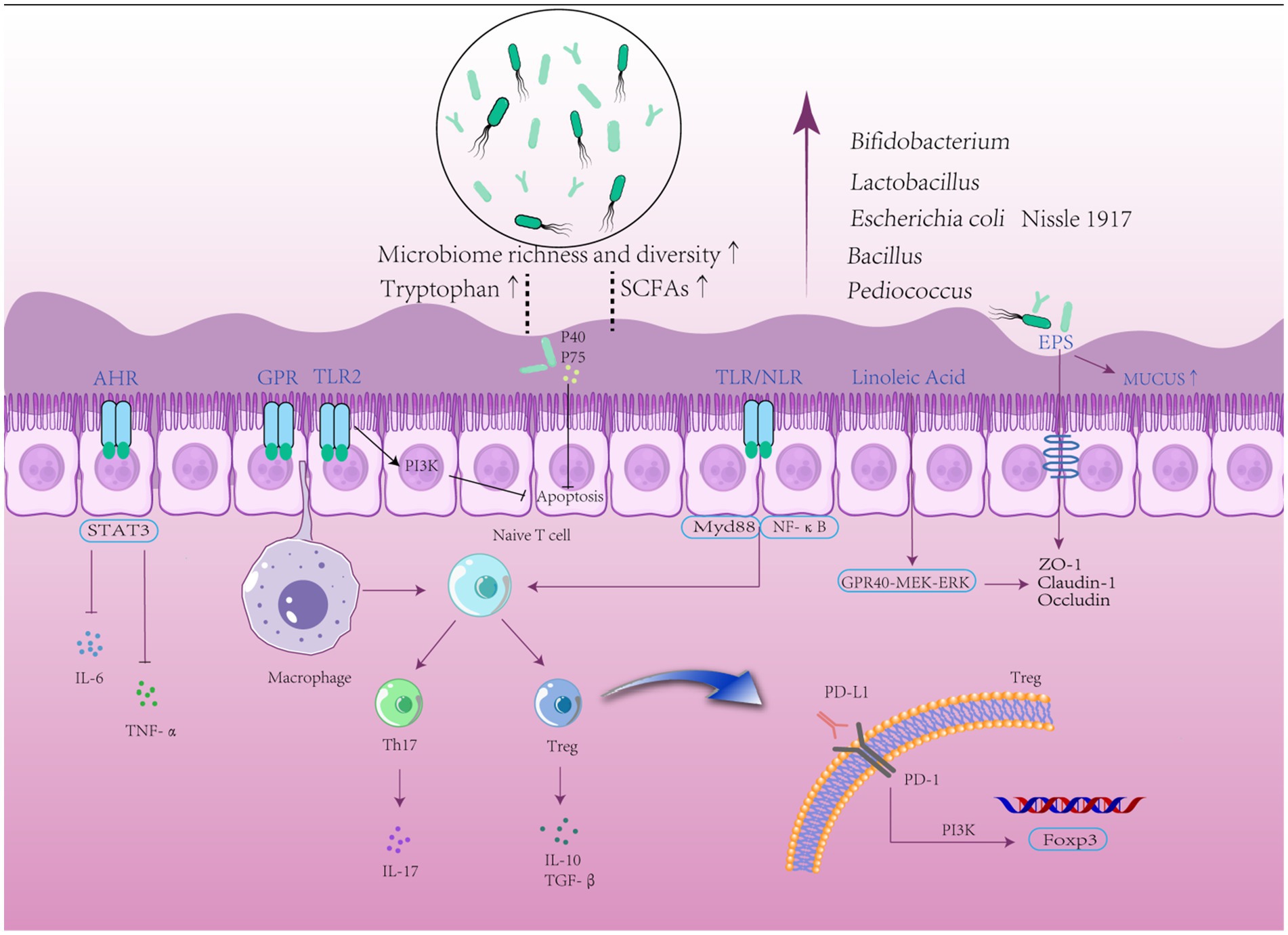
Figure 3. Protective mechanisms of probiotics. Probiotics can maintain gut microbiota homeostasis by increasing the number of beneficial bacteria, regulating T cell differentiation to control inflammatory responses and immune disorders, and maintaining barrier function by regulating signaling pathways to upregulate the expression of tight junction proteins.
3.1. Probiotics regulate the gut microbiota and increase its diversity
Probiotics can directly increase the relative abundance of Firmicutes while decreasing the relative abundance of Bacteroides (Cao et al., 2018) to maintain the population of the dominant flora. Furthermore, probiotics can improve adhesion via surface adhesins and surface-expressed fibrinogen, as well as express microbial-associated molecular patterns that interact with pathogenic bacteria for the intestinal epithelial receptor and limit pathogenic bacterial colonization of the gut (Arena et al., 2017). Meanwhile, probiotic supplements hinder the growth of harmful bacteria by consuming metabolic substrates and competing for nutrients. Additionally, probiotics increase the abundance of beneficial bacteria by secreting bacteriocins (Ortiz-Rivera et al., 2017), which can increase the concentration of acetic, lactic, and propionic acids and lower intestinal pH, increase the permeability of the outer membrane of gram-negative bacteria, and directly or indirectly inhibit the growth of pathogenic bacteria (Stavropoulou and Bezirtzoglou, 2020; Ma et al., 2022). Therefore, supplementation with probiotics can effectively maintain homeostasis of the gut microbiota by increasing the abundance of the dominant bacteria.
3.2. Probiotics enhance intestinal barrier function
It was proposed that probiotics improve intestinal barrier function via several mechanisms, including increased mucus secretion and upregulation of TJ proteins such as claudin-1, occludin, and ZO-1 via upregulation of MUC2, MUC3, and MUC1 expression in colonic epithelial cells (Kaur et al., 2021). Probiotic-secreted adhesive molecules, such as lipopolysaccharide, polysaccharide A, and peptidoglycan, can stimulate intestinal epithelial cell thickening and increase MUC2 expression. Butyric acid promotes epithelial cell differentiation and mucin synthesis and secretion (Wrzosek et al., 2013). Additionally, L. reuteri can promote germinal center-like B cell differentiation to increase the induction and production of PD-1-T follicular helper cell-dependent IgA (Liu et al., 2021). IgA-coated bacteria improved the mucosal barrier better due to their capacity for easy interaction with the host intestine, such as L. jensenii and L. reutri (Sun et al., 2019; Qi et al., 2021). Furthermore, L. reutri can adhere to the surface of Caco-2 or HT29 cells to activate the NF-κB signaling cascade, subsequently upregulating IgA expression and neutralizing bacterial toxins, reducing microbial immunogenicity, and binding to pathogenic microbial surface antigens to prevent the invasion of pathogenic bacteria. B.bifidum BB1 improves intestinal epithelial barrier function by connecting to TLR-2 receptors and activating the p38 kinase pathway, which increases TJ expression (Al-Sadi et al., 2021a,b). Similarly, L. plantarum NCU116 increases TJ expression by promoting signaling sensors and activators of transcription 3 (STAT3) binding to the promoters of occludin and ZO-1 via EPS (Zhou et al., 2018). L. fermentum L930BB and B. animalis IM386 are involved in actin cell regulation via the activation of protein kinase C and GTPases as well as phosphatidylinositol-4,5-bisphosphate 3-kinase (PI3K) via TLR-2 receptor/Akt upregulation of the anti-apoptotic pathway. The actin backbone reorganization and the reduction of apoptosis both contribute to the reconstruction of intestinal epithelial cells (Paveljšek et al., 2018). Furthermore, B. bifidum increases Caco-2 cell monolayer epithelial resistance, decreases glucose endocytosis, and diminishes LPS-induced colonic damage (Cui et al., 2022). Moreover, L. plantarum metabolizes linoleic acid to produce 10-hydroxycis-12-octadecenoic acid, which modulates the GPR40-MEK–ERK pathway and suppresses occludin, ZO-1, and claudin-1 downregulation-induced enhanced intestinal permeability (Miyamoto et al., 2015). L. plantarum 12 can prevent colonic damage in mice by increasing colonic TJ protein levels through the suppression of the proliferation of cell nuclear antigen PCNA and enhancement of pro-apoptotic Bax. Furthermore, two soluble proteins (p40 and p75) from L GG were suggested to improve intestinal epithelial homeostasis by preventing cytokine-induced epithelial cell death (Yan et al., 2007). Recent research has found that the probiotic metabolite indolepropionic acid can improve intestinal barrier integrity by interacting directly with the pregnane X receptor 4 (Collins and Patterson, 2020). Therefore, upregulation of TJ expression by probiotics via multiple signaling pathways is the primary mechanism for improving intestinal barrier function.
3.3. Probiotics regulate the intestinal immune response
Probiotics can modify immune cells and immunological factors. Meanwhile, probiotics, as innate immune organisms or bacteriophage components, bind to pattern recognition receptors (PRR) on the intestinal mucosa to activate immune cells. L. plantarum CBT LP3 exerts anti-inflammatory effects by increasing the number of Tregs, DCs, and Th2 cells while also stimulating the release of anti-inflammatory cytokines such as IL-10 and TGF-β (Kim et al., 2020). B. adolescentis EPS activates macrophages via the TLR2-ERK/p38 MAPK signaling cascade, and the macrophage-regulated Treg/Th17 axis suppresses the immunological response (Yu et al., 2019). EPS, proteins, and lipophosphates from L. acidophilus LA1 cell walls inhibit NF-κB activation in human HT-29 cells and prevent the release of downstream pro-inflammatory factors (Chen et al., 2013). Immunosuppressive mechanisms are required for intestinal homeostasis, which can be achieved by increasing IL-18 secretion by IECs and the number of Tregs and IL-10-producing T cells. For example, the surface-associated EPS (exopolysaccharide) of B. longum 35,624 stimulates the differentiation of naive CD4+ cells into Tregs, which govern effector T cells and exert immunosuppressive effects (Schiavi et al., 2016). LPS-induced NF-κB activation in BV-2 cells can be inhibited by L. reuteri NK33 and B. adolescentis NK98. Furthermore, probiotic metabolites, particularly SCFAs, can modulate intestinal immune factors, with butyrate activating G protein-coupled receptor 109A signaling to promote Treg differentiation (Couto et al., 2020) and inhibit TLR4/NF-κB signaling pathway-induced pro-inflammatory gene expression (Ghadimi et al., 2020; Zheng et al., 2022). Tryptophan metabolism produces indole compounds that bind to the aromatic hydrocarbon receptor (AHR) and inhibit the production of IFN-γ, IL-6, IL-12, TNF-α, IL-7, and Th17 (Blacher et al., 2017; Chen et al., 2021; Liu et al., 2022), while L. plantarum KLDS 1.0386, a strain with high tryptophan metabolizing activity, produces indole-3-acetic acid that further upregulates AHR mRNA expression (Gu et al., 2021; Yang et al., 2021), activating the IL-22/STAT3 signaling pathway and inhibiting inflammatory cytokines production (Shi et al., 2020). Both Lactobacillus and Bifidobacterium strains can drastically lower adherent-invasive Escherichia coli LF82 viability inside macrophages and dendritic cells, decreasing the release of polarizing cytokines linked to the IL-23/Th17 axis (Leccese et al., 2020). NLRP3 components are highly expressed in mice and patients with colitis, and the probiotic MSP inhibits NLRP3 to reduce inflammatory factor production by inhibiting NLRP3-mediated caspase-1 activation (Liu and Wang, 2021; Alkushi et al., 2022). L. acidophilus also reduces colonic inflammation through this pathway (Li et al., 2022). In a homeostatic state, metabolites and commensal flora can be recognized by a variety of PRRs, including NOD-like receptors and TLRs, and systematic reviews have shown that the interaction of these receptors is involved in the treatment of UC (Impellizzeri et al., 2015; Kogut et al., 2020). L. plantarum inhibits IL-6 and IL-1 secretion to reduce inflammation and improve immunological control by downregulating the expression of JAK, TIRAP, IRAK4, NEMO, and RIP genes in the NF-κB pathway (Aghamohammad et al., 2022; Qian et al., 2022). The LGG effector protein HM0539 of L. rhamnosus regulates distal NF-κB activation by lowering TLR4 activation and blocking MyD88 transactivation (Li et al., 2020). B. infantis increases T cell-to-Treg conversion by amplifying programmed cell death 1 (PD-1), PD ligand (PD-L1), and the nuclear transcription factor FOXP3, although the mechanism by which PD-1 and PD-L1 are amplified is unknown. Furthermore, it has been proposed that L. acidophilus suppresses colitis by modulating immune cells as well as IL-10, thus selectively interfering with endoplasmic reticulum stress. However, it is unclear how probiotics and IL-10 affect endoplasmic reticulum stress, and more research is needed to determine the exact mechanisms of L. acidophilus.
4. Discussion
The number of studies on the gut microbiota has increased during the last two decades, and scientists are gradually revealing the hidden interactions between the gut microbiota and host. Because the pathophysiology of UC is intrinsically linked to dysbiosis of the gut microbiota, employing probiotics as a therapeutic intervention for UC is justified. Animal trials have demonstrated that probiotics can induce UC remission. The two most dominant genera in the digestive tract, Bifidobacterium and Lactobacillus, have proven their efficacy in the treatment of UC and are the key constituents in probiotic products currently on the market. Probiotic administration in mice with colitis achieved positive results in terms of symptom relief, tissue improvement, reduction of cellular inflammatory markers, and enhanced expression of tight junction proteins.
Probiotics can be utilized as carriers for genetic engineering and can be altered through genetic engineering. This change can improve the stability of probiotic effects, which may enhance their therapeutic benefits. For example, EcN has been genetically modified to increase the expression of catalase, superoxide dismutase, and endothelial inhibitors (Bi et al., 2022; Zhou et al., 2022), improving its antioxidant effects. The combination of genetic engineering and probiotics for the treatment of UC is widely employed and has a promising future in terms of boosting probiotic efficacy. It is also important to consider the substance of probiotics. Some researchers have employed unique materials as coatings for oral probiotics to preserve their biological activity in the gastrointestinal tract and considerably improve their therapeutic impact (Mauras et al., 2018; Dosoky et al., 2020; Zhou et al., 2022). Fecal transplantation technology is gaining attention in the field of gut microbiota, with two randomized control trials, a meta-analysis, and a review reporting that fecal transplantation (Imdad et al., 2018; Costello et al., 2019; Ooijevaar et al., 2019; Haifer et al., 2022) significantly relieved symptoms of UC, demonstrating the importance of improving the gut microbiota in the treatment of UC. However, fecal transplantation technology is demanding and not routinely performed in clinical practice. Thus, oral probiotic products are still primarily used to maintain the balance of the gut microbiota. Animal experiments have provided substantial evidence regarding the effectiveness of probiotics. However, probiotics take time to colonize the intestine and exert their biological effects (Gao et al., 2019). Hence, hematological therapy and surgery are still preferred for severe and acute UC with serious complications (Kaur et al., 2020). The clinical use of medicines in conjunction with probiotics results in better induction of remission (Tan et al., 2022). The European Society for Parenteral and Enteral Nutrition published the Guidelines for Clinical Nutrition Management of Inflammatory Bowel Disease, which confirm the remission-inducing effect of specific probiotic strains in patients with mild to moderate UC, with the use of Lactobacillus reuteri and VSL#3 being recommended (Forbes et al., 2019). In adults with UC, Bifidobacterium bifidum powder/capsules, Lactobacillus and Bifidobacterium triplex tablets, and Bacillus subtilis dibacterium enteric-coated capsules are recommended in China. Although there have been no reports of major adverse effects from probiotics, they should be administered with caution in patients with an injured gastrointestinal tract. In clinical studies on patients with UC, the probiotics used, dose and length of treatment regimens, inclusion criteria, and study objectives varied, resulting in convincing evidence regarding the benefits of probiotics. As the pathophysiology of UC and tolerances to probiotics in animals and humans vary, more clinical trials are required to determine the optimal use of probiotics.
5. Conclusion
Probiotics are symbiotic organisms that colonize the human gut. The gut microbiota has been widely studied as a critical factor in the occurrence and progression of UC, and probiotics have demonstrated their efficacy in the treatment of experimental UC in mice primarily by correcting gut microbiota dysbiosis, enhancing intestinal mucosa and barrier function, regulating intestinal immune function, and reducing intestinal inflammation. Animal research, however, cannot substitute for clinical trials, and a significant number of high-quality randomized controlled trials are required to establish the efficacy and safety of probiotics. Simultaneously, probiotics have few and mild adverse effects and have a favorable biosafety index. As such, probiotics may be used as an adjuvant treatment of UC. Therefore, clinical trials based on the results of animal experiments are worth exploring, which is the goal of our future research. However, high-quality studies on the efficacy of probiotics in UC with children are extremely limited: there are only two randomized controlled trials and one pilot study in UC. Thus, while it is undoubtedly possible to infer conclusions from evidence acquired in adults, as briefly outlined below, caution must be exercised. Probiotics are now used mostly as adjuvant therapy for UC, and if used as primary therapy, it is necessary to evaluate if the GI environment reduces and inactivates them. Considering the diverse areas of colonization of particular strains and the fact that the primary lesions of UC occur in the colorectum, it is worth investigating if specific strain enemas may be increased to accomplish the effect of boosting effectiveness. For future clinical studies, in addition to clarifying the mechanism of action and dose and duration of treatment, the extent to which probiotics mitigate the adverse effects of existing treatment regimens and the synergistic effects with existing treatment regimens should be explored. For pediatric patients, the low compliance rate and the high rate of missed visits are the main barriers to conducting clinical studies. Moreover, as children are in the growth and development stage, they should be very cautious about the medication of the disease, and the treatment of UC in children with probiotics alone is difficult to achieve.
Author contributions
QL: conceptualization and funding acquisition. CH, WH, and XW: methodology. CH, WH, XW, and RZ: formal analysis. CH and WH: writing—original draft preparation. CH and WH have contributed equally to this work and share first authorship. RZ and QL: writing—review and editing. XW, RZ, and QL: supervision. All authors have read and agreed to the published version of the manuscript.
Funding
This research was funded by the Wuxi Medical Innovation Team (Grant No. CXTD2021011).
Acknowledgments
We would like to thank all team members for their contributions to this article, Editage (www.editage.cn) for English language editing and Biorender (app.biorender.com) for the drawing materials.
Conflict of interest
The authors declare that the research was conducted in the absence of any commercial or financial relationships that could be construed as a potential conflict of interest.
Publisher’s note
All claims expressed in this article are solely those of the authors and do not necessarily represent those of their affiliated organizations, or those of the publisher, the editors and the reviewers. Any product that may be evaluated in this article, or claim that may be made by its manufacturer, is not guaranteed or endorsed by the publisher.
References
Abraham, B. P., and Quigley, E. M. M. (2017). Probiotics in inflammatory bowel disease. Gastroenterol. Clin. N. Am. 46, 769–782. doi: 10.1016/j.gtc.2017.08.003
Abrantes, F. A., Nascimento, B. B., Andrade, M. E. R., de Barros, P. A. V., Cartelle, C. T., Martins, F. S., et al. (2020). Treatment with Bifidobacterium longum 5(1A) attenuates intestinal damage and inflammatory response in experimental colitis. Benef Microbes 11, 47–57. doi: 10.3920/BM2019.0098
Aghamohammad, S., Sepehr, A., Miri, S. T., Najafi, S., Pourshafie, M. R., and Rohani, M. (2022). Anti-inflammatory and immunomodulatory effects of Lactobacillus spp. as a preservative and therapeutic agent for IBD control. Immun Inflamm Dis 10:e635. doi: 10.1002/iid3.635
Agraib, L. M., Yamani, M. I., Tayyem, R., Abu-Sneineh, A. T., and Rayyan, Y. M. (2022). Probiotic supplementation induces remission and changes in the immunoglobulins and inflammatory response in active ulcerative colitis patients: a pilot, randomized, double-blind, placebo-controlled study. Clin Nutr ESPEN 51, 83–91. doi: 10.1016/j.clnesp.2022.08.020
Agrawal, M., Allin, K. H., Petralia, F., Colombel, J. F., and Jess, T. (2022). Multiomics to elucidate inflammatory bowel disease risk factors and pathways. Nat. Rev. Gastroenterol. Hepatol. 19, 399–409. doi: 10.1038/s41575-022-00593-y
Agrawal, M., Sabino, J., Frias-Gomes, C., Hillenbrand, C. M., Soudant, C., Axelrad, J. E., et al. (2021). Early life exposures and the risk of inflammatory bowel disease: systematic review and meta-analyses. EClinicalMedicine 36:100884. doi: 10.1016/j.eclinm.2021.100884
Agrawal, M., Spencer, E. A., Colombel, J. F., and Ungaro, R. C. (2021). Approach to the Management of Recently Diagnosed Inflammatory Bowel Disease Patients: a User’s guide for adult and pediatric gastroenterologists. Gastroenterology 161, 47–65. doi: 10.1053/j.gastro.2021.04.063
Ahl, D., Liu, H., Schreiber, O., Roos, S., Phillipson, M., and Holm, L. (2016). Lactobacillus reuteri increases mucus thickness and ameliorates dextran sulphate sodium-induced colitis in mice. Acta Physiol (Oxf.) 217, 300–310. doi: 10.1111/apha.12695
Ahmed, J., Reddy, B. S., Mølbak, L., Leser, T. D., and MacFie, J. (2013). Impact of probiotics on colonic microflora in patients with colitis: a prospective double blind randomised crossover study. Int. J. Surg. 11, 1131–1136. doi: 10.1016/j.ijsu.2013.08.019
Albert, K., Rani, A., and Sela, D. A. (2019). Comparative Pangenomics of the mammalian gut commensal Bifidobacterium longum. Microorganisms 8:7. doi: 10.3390/microorganisms8010007
Alkushi, A. G., Elazab, S. T., Abdelfattah-Hassan, A., Mahfouz, H., Salem, G. A., Sheraiba, N. I., et al. (2022). Multi-strain-probiotic-loaded nanoparticles reduced Colon inflammation and orchestrated the expressions of tight junction, NLRP3 Inflammasome and Caspase-1 genes in DSS-induced colitis model. Pharmaceutics 14:1183. doi: 10.3390/pharmaceutics14061183
Al-Sadi, R., Dharmaprakash, V., Nighot, P., Guo, S., Nighot, M., Do, T., et al. (2021a). Bifidobacterium bifidum enhances the intestinal epithelial tight junction barrier and protects against intestinal inflammation by targeting the toll-like Receptor-2 pathway in an NF-κB-independent manner. Int. J. Mol. Sci. 22:8070. doi: 10.3390/ijms22158070
Al-Sadi, R., Nighot, P., Nighot, M., Haque, M., Rawat, M., and Ma, T. Y. (2021b). Lactobacillus acidophilus induces a strain-specific and toll-like receptor 2-dependent enhancement of intestinal epithelial tight junction barrier and protection against intestinal inflammation. Am. J. Pathol. 191, 872–884. doi: 10.1016/j.ajpath.2021.02.003
Andresen, V., Gschossmann, J., and Layer, P. (2020). Heat-inactivated Bifidobacterium bifidum MIMBb75 (SYN-HI-001) in the treatment of irritable bowel syndrome: a multicentre, randomised, double-blind, placebo-controlled clinical trial. Lancet Gastroenterol. Hepatol. 5, 658–666. doi: 10.1016/S2468-1253(20)30056-X
Arena, M. P., Capozzi, V., Spano, G., and Fiocco, D. (2017). The potential of lactic acid bacteria to colonize biotic and abiotic surfaces and the investigation of their interactions and mechanisms. Appl. Microbiol. Biotechnol. 101, 2641–2657. doi: 10.1007/s00253-017-8182-z
Bai, Y., Lyu, M., Fukunaga, M., Watanabe, S., Iwatani, S., Miyanaga, K., et al. (2022). Lactobacillus johnsonii enhances the gut barrier integrity via the interaction between GAPDH and the mouse tight junction protein JAM-2. Food Funct. 13, 11021–11033. doi: 10.1039/D2FO00886F
Baumgart, D. C., and Le Berre, C. (2021). Newer biologic and small-molecule therapies for inflammatory bowel disease. N. Engl. J. Med. 385, 1302–1315. doi: 10.1056/NEJMra1907607
Bi, Z., Cui, E., Yao, Y., Chang, X., Wang, X., Zhang, Y., et al. (2022). Recombinant Bifidobacterium longum carrying Endostatin protein alleviates dextran sodium sulfate-induced colitis and Colon Cancer in rats. Front. Microbiol. 13:927277. doi: 10.3389/fmicb.2022.927277
Bian, X., Yang, L., Wu, W., Lv, L., Jiang, X., Wang, Q., et al. (2020). Pediococcus pentosaceus LI05 alleviates DSS-induced colitis by modulating immunological profiles, the gut microbiota and short-chain fatty acid levels in a mouse model. Microb. Biotechnol. 13, 1228–1244. doi: 10.1111/1751-7915.13583
Bibiloni, R., Fedorak, R. N., Tannock, G. W., Madsen, K. L., Gionchetti, P., Campieri, M., et al. (2005). VSL#3 probiotic-mixture induces remission in patients with active ulcerative colitis. Am. J. Gastroenterol. 100, 1539–1546. doi: 10.1111/j.1572-0241.2005.41794.x
Bischoff, S. C., Escher, J., Hébuterne, X., Kłęk, S., Krznaric, Z., Schneider, S., et al. (2022). ESPEN guideline: clinical nutrition in inflammatory bowel disease. Nutr. Hosp. 39, 678–703. doi: 10.20960/nh.03857
Bjarnason, I., Sission, G., and Hayee, B. (2019). A randomised, double-blind, placebo-controlled trial of a multi-strain probiotic in patients with asymptomatic ulcerative colitis and Crohn’s disease. Inflammopharmacology 27, 465–473. doi: 10.1007/s10787-019-00595-4
Blacher, E., Levy, M., Tatirovsky, E., and Elinav, E. (2017). Microbiome-modulated metabolites at the Interface of host immunity. J. Immunol. 198, 572–580. doi: 10.4049/jimmunol.1601247
Cannon, A. R., Shim, E. H., Kuprys, P. V., and Choudhry, M. A. (2022). IL-22 and Lactobacillus delbrueckii mitigate alcohol-induced exacerbation of DSS-induced colitis. J. Leukoc. Biol. 112, 1471–1484. doi: 10.1002/JLB.4A0122-068R
Cao, G., Wang, K., Li, Z., Tao, F., Xu, Y., Lan, J., et al. (2018). Bacillus amyloliquefaciens ameliorates dextran sulfate sodium-induced colitis by improving gut microbial Dysbiosis in mice model. Front. Microbiol. 9:3260. doi: 10.3389/fmicb.2018.03260
Capurso, L. (2019). Thirty years of Lactobacillus rhamnosus GG: a review. J. Clin. Gastroenterol. 53, S1–s41. doi: 10.1097/MCG.0000000000001170
Chae, J. M., Heo, W., Cho, H. T., Lee, D. H., Kim, J. H., Rhee, M. S., et al. (2018). Effects of orally-administered Bifidobacterium animalis subsp. lactis strain BB12 on dextran sodium sulfate-induced colitis in mice. J. Microbiol. Biotechnol. 28, 1800–1805. doi: 10.4014/jmb.1805.05072
Chapman, T. P., Gomes, C. F., Louis, E., Colombel, J. F., and Satsangi, J. (2020). De-escalation of immunomodulator and biological therapy in inflammatory bowel disease. Lancet Gastroenterol. Hepatol. 5, 63–79. doi: 10.1016/S2468-1253(19)30186-4
Chapman, T. M., Plosker, G. L., and Figgitt, D. P. (2007). Spotlight on VSL#3 probiotic mixture in chronic inflammatory bowel diseases. BioDrugs 21, 61–63. doi: 10.2165/00063030-200721010-00007
Chen, Y., Chen, H., Ding, J., Stanton, C., Ross, R. P., Zhao, J., et al. (2021). Bifidobacterium longum ameliorates dextran sulfate sodium-induced colitis by producing conjugated linoleic acid, protecting intestinal mechanical barrier, restoring unbalanced gut microbiota, and regulating the toll-like Receptor-4/nuclear factor-κB signaling pathway. J. Agric. Food Chem. 69, 14593–14608. doi: 10.1021/acs.jafc.1c06176
Chen, X., Fu, Y., Wang, L., Qian, W., Zheng, F., and Hou, X. (2019). Bifidobacterium longum and VSL#3(®) amelioration of TNBS-induced colitis associated with reduced HMGB1 and epithelial barrier impairment. Dev. Comp. Immunol. 92, 77–86. doi: 10.1016/j.dci.2018.09.006
Chen, C. L., Hsu, P. Y., and Pan, T. M. (2019). Therapeutic effects of Lactobacillus paracasei subsp. paracasei NTU 101 powder on dextran sulfate sodium-induced colitis in mice. J. Food Drug Anal. 27, 83–92. doi: 10.1016/j.jfda.2018.05.004
Chen, Y., Jin, Y., Stanton, C., Paul Ross, R., Zhao, J., Zhang, H., et al. (2021). Alleviation effects of Bifidobacterium breve on DSS-induced colitis depends on intestinal tract barrier maintenance and gut microbiota modulation. Eur. J. Nutr. 60, 369–387. doi: 10.1007/s00394-020-02252-x
Chen, K., Liang, N., Luo, X., and Zhang, T. C. (2013). Lactobacillus acidophilus strain suppresses the transcription of proinflammatory-related factors in human HT-29 cells. J. Microbiol. Biotechnol. 23, 64–68. doi: 10.4014/jmb.1208.04067
Chen, M. J., Tang, H. Y., and Chiang, M. L. (2017). Effects of heat, cold, acid and bile salt adaptations on the stress tolerance and protein expression of kefir-isolated probiotic Lactobacillus kefiranofaciens M1. Food Microbiol. 66, 20–27. doi: 10.1016/j.fm.2017.03.020
Chen, Z., Yi, L., Pan, Y., Long, X., Mu, J., Yi, R., et al. (2021). Lactobacillus fermentum ZS40 ameliorates inflammation in mice with ulcerative colitis induced by dextran sulfate sodium. Front. Pharmacol. 12:700217. doi: 10.3389/fphar.2021.700217
Cheng, S., Li, H., Huang, Y., Su, Y., Li, Y., Jia, A., et al. (2023). Lactobacillus gasseri JM1 isolated from infant feces alleviates colitis in mice via protecting the intestinal barrier. Nutrients 15:139. doi: 10.3390/nu15010139
Cheng, H., Ma, Z., Yu, B., Liu, X., Tian, C., Zhong, X., et al. (2021). Quality assessment of clinical guidelines on probiotics therapy in children with IBD using the AGREE II instrument. J. Clin. Pharm. Ther. 46, 1155–1165. doi: 10.1111/jcpt.13422
Choi, S. H., Lee, S. H., Kim, M. G., Lee, H. J., and Kim, G. B. (2019). Lactobacillus plantarum CAU1055 ameliorates inflammation in lipopolysaccharide-induced RAW264.7 cells and a dextran sulfate sodium-induced colitis animal model. J. Dairy Sci. 102, 6718–6725. doi: 10.3168/jds.2018-16197
Choi, J. H., Moon, C. M., Shin, T. S., Kim, E. K., McDowell, A., Jo, M. K., et al. (2020). Lactobacillus paracasei-derived extracellular vesicles attenuate the intestinal inflammatory response by augmenting the endoplasmic reticulum stress pathway. Exp. Mol. Med. 52, 423–437. doi: 10.1038/s12276-019-0359-3
Choi, Y. J., Shin, S. H., and Shin, H. S. (2022). Immunomodulatory effects of Bifidobacterium spp. and use of Bifidobacterium breve and Bifidobacterium longum on acute diarrhea in children. J. Microbiol. Biotechnol. 32, 1186–1194. doi: 10.4014/jmb.2206.06023
Chorawala, M. R., Chauhan, S., Patel, R., and Shah, G. (2021). Cell Wall contents of probiotics (Lactobacillus species) protect against lipopolysaccharide (LPS)-induced murine colitis by limiting Immuno-inflammation and oxidative stress. Probiotics Antimicrob Proteins 13, 1005–1017. doi: 10.1007/s12602-020-09738-4
Chung, K. S., Shin, J. S., Lee, J. H., Park, S. E., Han, H. S., Rhee, Y. K., et al. (2021). Protective effect of exopolysaccharide fraction from Bacillus subtilis against dextran sulfate sodium-induced colitis through maintenance of intestinal barrier and suppression of inflammatory responses. Int. J. Biol. Macromol. 178, 363–372. doi: 10.1016/j.ijbiomac.2021.02.186
Cochrane Gut Group Murray, A., Nguyen, T. M., Parker, C. E., Feagan, B. G., and MacDonald, J. K. (2020). Oral 5-aminosalicylic acid for maintenance of remission in ulcerative colitis. Cochrane Database Syst. Rev. 2020:Cd000544. doi: 10.1002/14651858.CD000544.pub5
Cochrane IBD Group Kaur, L., Gordon, M., Baines, P. A., Iheozor-Ejiofor, Z., Sinopoulou, V., et al. (2020). Probiotics for induction of remission in ulcerative colitis. Cochrane Database Syst. Rev. 3:Cd005573. doi: 10.1002/14651858.CD005573.pub3
Collins, S. L., and Patterson, A. D. (2020). The gut microbiome: an orchestrator of xenobiotic metabolism. Acta Pharm. Sin. B 10, 19–32. doi: 10.1016/j.apsb.2019.12.001
Costello, S. P., Hughes, P. A., Waters, O., Bryant, R. V., Vincent, A. D., Blatchford, P., et al. (2019). Effect of fecal microbiota transplantation on 8-week remission in patients with ulcerative colitis: a randomized clinical trial. JAMA 321, 156–164. doi: 10.1001/jama.2018.20046
Couto, M. R., Gonçalves, P., Magro, F., and Martel, F. (2020). Microbiota-derived butyrate regulates intestinal inflammation: focus on inflammatory bowel disease. Pharmacol. Res. 159:104947. doi: 10.1016/j.phrs.2020.104947
Cui, H. H., Chen, C. L., Wang, J. D., Yang, Y. J., Cun, Y., Wu, J. B., et al. (2004). Effects of probiotic on intestinal mucosa of patients with ulcerative colitis. World J. Gastroenterol. 10, 1521–1525. doi: 10.3748/wjg.v10.i10.1521
Cui, Q. Y., Tian, X. Y., Liang, X., Zhang, Z., Wang, R., Zhou, Y., et al. (2022). Bifidobacterium bifidum relieved DSS-induced colitis in mice potentially by activating the aryl hydrocarbon receptor. Food Funct. 13, 5115–5123. doi: 10.1039/D1FO04219J
D’Incà, R., Barollo, M., Scarpa, M., Grillo, A. R., Brun, P., Vettorato, M. G., et al. (2011). Rectal administration of Lactobacillus casei DG modifies flora composition and toll-like receptor expression in colonic mucosa of patients with mild ulcerative colitis. Dig. Dis. Sci. 56, 1178–1187. doi: 10.1007/s10620-010-1384-1
De Melo Pereira, G. V., de Oliveira Coelho, B., Magalhães Júnior, A. I., Thomaz-Soccol, V., and Soccol, C. R. (2018). How to select a probiotic? A review and update of methods and criteria. Biotechnol. Adv. 36, 2060–2076. doi: 10.1016/j.biotechadv.2018.09.003
Dodd, D., Spitzer, M. H., van Treuren, W., Merrill, B. D., Hryckowian, A. J., Higginbottom, S. K., et al. (2017). A gut bacterial pathway metabolizes aromatic amino acids into nine circulating metabolites. Nature 551, 648–652. doi: 10.1038/nature24661
Dong, F., Xiao, F., Li, X., Li, Y., Wang, X., Yu, G., et al. (2022). Pediococcus pentosaceus CECT 8330 protects DSS-induced colitis and regulates the intestinal microbiota and immune responses in mice. J. Transl. Med. 20:33. doi: 10.1186/s12967-022-03235-8
Dosoky, N. S., May-Zhang, L. S., and Davies, S. S. (2020). Engineering the gut microbiota to treat chronic diseases. Appl. Microbiol. Biotechnol. 104, 7657–7671. doi: 10.1007/s00253-020-10771-0
Düz, M., Doğan, Y. N., and Doğan, I. (2020). Antioxidant activitiy of Lactobacillus plantarum, Lactobacillus sake and Lactobacillus curvatus strains isolated from fermented Turkish Sucuk. An. Acad. Bras. Cienc. 92:e20200105. doi: 10.1590/0001-3765202020200105
Eisenstein, M. (2018). Ulcerative colitis: towards remission. Nature 563:S33. doi: 10.1038/d41586-018-07276-2
Ewaschuk, J. B., Diaz, H., Meddings, L., Diederichs, B., Dmytrash, A., Backer, J., et al. (2008). Secreted bioactive factors from Bifidobacterium infantis enhance epithelial cell barrier function. Am. J. Physiol. Gastrointest. Liver Physiol. 295, G1025–G1034. doi: 10.1152/ajpgi.90227.2008
Fan, H., Du, J., Liu, X., Zheng, W. W., Zhuang, Z. H., Wang, C. D., et al. (2019). Effects of pentasa-combined probiotics on the microflora structure and prognosis of patients with inflammatory bowel disease. Turk J Gastroenterol 30, 680–685. doi: 10.5152/tjg.2019.18426
Fan, L., Qi, Y., Qu, S., Chen, X., Li, A., Hendi, M., et al. (2021). B. adolescentis ameliorates chronic colitis by regulating Treg/Th2 response and gut microbiota remodeling. Gut Microbes 13, 1–17. doi: 10.1080/19490976.2020.1826746
Fatmawati, N. N. D., Gotoh, K., Mayura, I. P. B., Nocianitri, K. A., Suwardana, G. N. R., Komalasari, N. L. G. Y., et al. (2020). Enhancement of intestinal epithelial barrier function by Weissella confusa F213 and Lactobacillus rhamnosus FBB81 probiotic candidates in an in vitro model of hydrogen peroxide-induced inflammatory bowel disease. BMC. Res. Notes 13:489. doi: 10.1186/s13104-020-05338-1
Feng, C., Zhang, W., Zhang, T., He, Q., Kwok, L. Y., Tan, Y., et al. (2022). Heat-killed Bifidobacterium bifidum B1628 may alleviate dextran sulfate sodium-induced colitis in mice, and the anti-inflammatory effect is associated with gut microbiota modulation. Nutrients 14:5233. doi: 10.3390/nu14245233
Feuerstein, J. D., Isaacs, K. L., Schneider, Y., Siddique, S. M., Falck-Ytter, Y., Singh, S., et al. (2020). AGA clinical practice guidelines on the Management of Moderate to severe ulcerative colitis. Gastroenterology 158, 1450–1461. doi: 10.1053/j.gastro.2020.01.006
Fontana, L., Bermudez-Brito, M., Plaza-Diaz, J., Muñoz-Quezada, S., and Gil, A. (2013). Sources, isolation, characterisation and evaluation of probiotics. Br. J. Nutr. 109, S35–S50. doi: 10.1017/S0007114512004011
Forbes, A., Escher, J., Hébuterne, X., Kłęk, S., Krznaric, Z., Schneider, S., et al. (2019). Corrigendum to “ESPEN guideline: clinical nutrition in inflammatory bowel disease” [clinical nutrition 36 (2017) 321-347]. Clin. Nutr. 38:1486. doi: 10.1016/j.clnu.2019.01.017
Furrie, E., Macfarlane, S., Kennedy, A., Cummings, J. H., Walsh, S. V., O’Neil, D. A., et al. (2005). Synbiotic therapy (Bifidobacterium longum/synergy 1) initiates resolution of inflammation in patients with active ulcerative colitis: a randomised controlled pilot trial. Gut 54, 242–249. doi: 10.1136/gut.2004.044834
Ganji-Arjenaki, M., and Rafieian-Kopaei, M. (2018). Probiotics are a good choice in remission of inflammatory bowel diseases: a meta analysis and systematic review. J. Cell. Physiol. 233, 2091–2103. doi: 10.1002/jcp.25911
Gao, J., Cao, S., Xiao, H., Hu, S., Yao, K., Huang, K., et al. (2022). Lactobacillus reuteri 1 enhances intestinal epithelial barrier function and alleviates the inflammatory response induced by enterotoxigenic Escherichia coli K88 via suppressing the MLCK signaling pathway in IPEC-J2 cells. Front. Immunol. 13:897395. doi: 10.3389/fimmu.2022.897395
Gao, Y., Liu, Y., Ma, F., Sun, M., Song, Y., Xu, D., et al. (2021). Lactobacillus plantarum Y44 alleviates oxidative stress by regulating gut microbiota and colonic barrier function in Balb/C mice with subcutaneous d-galactose injection. Food Funct. 12, 373–386. doi: 10.1039/D0FO02794D
Gao, R., Zhang, X., Huang, L., Shen, R., and Qin, H. (2019). Gut microbiota alteration after long-term consumption of probiotics in the elderly. Probiotics Antimicrob Proteins 11, 655–666. doi: 10.1007/s12602-018-9403-1
Garbacz, K. (2022). Anticancer activity of lactic acid bacteria. Semin. Cancer Biol. 86, 356–366. doi: 10.1016/j.semcancer.2021.12.013
Gebremariam, H. G., Qazi, K. R., Somiah, T., Pathak, S. K., Sjölinder, H., Sverremark Ekström, E., et al. (2019). Lactobacillus gasseri suppresses the production of proinflammatory cytokines in Helicobacter pylori-infected macrophages by inhibiting the expression of ADAM17. Front. Immunol. 10:2326. doi: 10.3389/fimmu.2019.02326
Ghadimi, D., de Vrese, M., Ebsen, M., Röcken, C., Olaf Frahm, S., Zahlten, J., et al. (2021). Study on the additive protective effect of PGLYRP3 and Bifidobacterium adolescentis Reuter 1963 on severity of DSS-induced colitis in Pglyrp3 knockout (Pglyrp3 −/−) and wild-type (WT) mice. Immunobiology 226:152028. doi: 10.1016/j.imbio.2020.152028
Ghadimi, D., Yoness Hassan, M. F., Fölster-Holst, R., Röcken, C., Ebsen, M., de Vrese, M., et al. (2020). Regulation of hepcidin/iron-signalling pathway interactions by commensal bifidobateria plays an important role for the inhibition of metaflammation-related biomarkers. Immunobiology 225:151874. doi: 10.1016/j.imbio.2019.11.009
Gionchetti, P., Rizzello, F., Morselli, C., Poggioli, G., Tambasco, R., Calabrese, C., et al. (2007). High-dose probiotics for the treatment of active pouchitis. Dis. Colon Rectum 50, 2075–2084. doi: 10.1007/s10350-007-9068-4
Gu, Z., Pei, W., Shen, Y., Wang, L., Zhu, J., Zhang, Y., et al. (2021). Akkermansia muciniphila and its outer protein Amuc_1100 regulates tryptophan metabolism in colitis. Food Funct. 12, 10184–10195. doi: 10.1039/D1FO02172A
Haifer, C., Paramsothy, S., Kaakoush, N. O., Saikal, A., Ghaly, S., Yang, T., et al. (2022). Lyophilised oral faecal microbiota transplantation for ulcerative colitis (LOTUS): a randomised, double-blind, placebo-controlled trial. Lancet Gastroenterol. Hepatol. 7, 141–151. doi: 10.1016/S2468-1253(21)00400-3
Han, S. K., Kim, J. K., Joo, M. K., Lee, K. E., Han, S. W., and Kim, D. H. (2020). Lactobacillus reuteri NK33 and Bifidobacterium adolescentis NK98 alleviate Escherichia coli-induced depression and gut Dysbiosis in mice. J. Microbiol. Biotechnol. 30, 1222–1226. doi: 10.4014/jmb.2002.02058
Han, D. H., Kim, W. K., Lee, C., Park, S. J., Lee, K., Jang, S. J., et al. (2022). Co-administration of Lactobacillus gasseri KBL697 and tumor necrosis factor-alpha inhibitor infliximab improves colitis in mice. Sci. Rep. 12:9640. doi: 10.1038/s41598-022-13753-6
Hao, H., Zhang, X., Tong, L., Liu, Q., Liang, X., Bu, Y., et al. (2021). Effect of extracellular vesicles derived from Lactobacillus plantarum Q7 on gut microbiota and ulcerative colitis in mice. Front. Immunol. 12:777147. doi: 10.3389/fimmu.2021.777147
Hegazy, S. K., and El-Bedewy, M. M. (2010). Effect of probiotics on pro-inflammatory cytokines and NF-kappaB activation in ulcerative colitis. World J. Gastroenterol. 16, 4145–4151. doi: 10.3748/wjg.v16.i33.4145
Henrick, B. M., Chew, S., Casaburi, G., Brown, H. K., Frese, S. A., Zhou, Y., et al. (2019). Colonization by B. infantis EVC001 modulates enteric inflammation in exclusively breastfed infants. Pediatr. Res. 86, 749–757. doi: 10.1038/s41390-019-0533-2
Hill, C., Guarner, F., Reid, G., Gibson, G. R., Merenstein, D. J., Pot, B., et al. (2014). Expert consensus document. The international scientific Association for Probiotics and Prebiotics consensus statement on the scope and appropriate use of the term probiotic. Nat. Rev. Gastroenterol. Hepatol. 11, 506–514. doi: 10.1038/nrgastro.2014.66
Ho, S. W., el-Nezami, H., Corke, H., Ho, C. S., and Shah, N. P. (2022). L-citrulline enriched fermented milk with Lactobacillus helveticus attenuates dextran sulfate sodium (DSS) induced colitis in mice. J. Nutr. Biochem. 99:108858. doi: 10.1016/j.jnutbio.2021.108858
Hrdý, J., Alard, J., Couturier-Maillard, A., Boulard, O., Boutillier, D., Delacre, M., et al. (2020). Lactobacillus reuteri 5454 and Bifidobacterium animalis ssp. lactis 5764 improve colitis while differentially impacting dendritic cells maturation and antimicrobial responses. Sci. Rep. 10:5345. doi: 10.1038/s41598-020-62161-1
Hrdý, J., Couturier-Maillard, A., Boutillier, D., Lapadatescu, C., Blanc, P., Procházka, J., et al. (2022). Oral supplementation with selected Lactobacillus acidophilus triggers IL-17-dependent innate defense response, activation of innate lymphoid cells type 3 and improves colitis. Sci. Rep. 12:17591. doi: 10.1038/s41598-022-21643-0
Hu, T., Fan, Y., Long, X., Pan, Y., Mu, J., Tan, F., et al. (2021). Protective effect of Lactobacillus plantarum YS3 on dextran sulfate sodium-induced colitis in C57BL/6J mice. J. Food Biochem. 45:e13632. doi: 10.1111/jfbc.13632
Hu, Y., Jin, X., Gao, F., Lin, T., Zhu, H., Hou, X., et al. (2022). Selenium-enriched Bifidobacterium longum DD98 effectively ameliorates dextran sulfate sodium-induced ulcerative colitis in mice. Front. Microbiol. 13:955112. doi: 10.3389/fmicb.2022.955112
Hu, T., Wang, H., Xiang, C., Mu, J., and Zhao, X. (2020). Preventive effect of Lactobacillus acidophilus XY27 on DSS-induced ulcerative colitis in mice. Drug Des. Devel. Ther. 14, 5645–5657. doi: 10.2147/DDDT.S284422
Huang, X., Ai, F., Ji, C., Tu, P., Gao, Y., Wu, Y., et al. (2021). A rapid screening method of candidate probiotics for inflammatory bowel diseases and the anti-inflammatory effect of the selected strain Bacillus smithii XY1. Front. Microbiol. 12:760385. doi: 10.3389/fmicb.2021.760385
Huang, Z., Gong, L., Jin, Y., Stanton, C., Ross, R. P., Zhao, J., et al. (2022). Different effects of different Lactobacillus acidophilus strains on DSS-induced colitis. Int. J. Mol. Sci. 23:14841. doi: 10.3390/ijms232314841
Huang, G., Pan, H., Zhu, Z., and Li, Q. (2020). The complete genome sequence of Bifidobacterium longum LTBL16, a potential probiotic strain from healthy centenarians with strong antioxidant activity. Genomics 112, 769–773. doi: 10.1016/j.ygeno.2019.05.015
Huang, J., Yang, Z., Li, Y., Chai, X., Liang, Y., Lin, B., et al. (2021). Lactobacillus paracasei R3 protects against dextran sulfate sodium (DSS)-induced colitis in mice via regulating Th17/Treg cell balance. J. Transl. Med. 19:356. doi: 10.1186/s12967-021-02943-x
Huda, M. N., Ahmad, S. M., Alam, M. J., Khanam, A., Kalanetra, K. M., Taft, D. H., et al. (2019). Bifidobacterium abundance in early infancy and vaccine response at 2 years of age. Pediatrics 143:e20181489. doi: 10.1542/peds.2018-1489
Hughes, K. R., Harnisch, L. C., Alcon-Giner, C., Mitra, S., Wright, C. J., Ketskemety, J., et al. (2017). Bifidobacterium breve reduces apoptotic epithelial cell shedding in an exopolysaccharide and MyD88-dependent manner. Open Biol. 7:160155. doi: 10.1098/rsob.160155
Huynh, H. Q., deBruyn, J., Guan, L., Diaz, H., Li, M., Girgis, S., et al. (2009). Probiotic preparation VSL#3 induces remission in children with mild to moderate acute ulcerative colitis: a pilot study. Inflamm. Bowel Dis. 15, 760–768. doi: 10.1002/ibd.20816
Hyams, J. S., Turner, D., Cohen, S. A., Szakos, E., Kowalska-Duplaga, K., Ruemmele, F., et al. (2022). Pharmacokinetics, safety and efficacy of intravenous Vedolizumab in Paediatric patients with ulcerative colitis or Crohn’s disease: results from the phase 2 HUBBLE study. J. Crohns Colitis 16, 1243–1254. doi: 10.1093/ecco-jcc/jjac036
Iheozor-Ejiofor, Z., Kaur, L., Gordon, M., Baines, P. A., Sinopoulou, V., and Akobeng, A. K. (2020). Probiotics for maintenance of remission in ulcerative colitis. Cochrane Database Syst. Rev. 3:Cd007443. doi: 10.1002/14651858.CD007443.pub3
Imdad, A., Nicholson, M. R., Tanner-Smith, E. E., Zackular, J. P., Gomez-Duarte, O. G., Beaulieu, D. B., et al. (2018). Fecal transplantation for treatment of inflammatory bowel disease. Cochrane Database Syst. Rev. 2018:Cd012774. doi: 10.1002/14651858.CD012774.pub2
Impellizzeri, D., Ahmad, A., di Paola, R., Campolo, M., Navarra, M., Esposito, E., et al. (2015). Role of toll like receptor 4 signaling pathway in the secondary damage induced by experimental spinal cord injury. Immunobiology 220, 1039–1049. doi: 10.1016/j.imbio.2015.05.013
In Kim, H. I., Kim, J. K., Kim, J. Y., Jang, S. E., Han, M. J., and Kim, D. H. (2019). Lactobacillus plantarum LC27 and Bifidobacterium longum LC67 simultaneously alleviate high-fat diet-induced colitis, endotoxemia, liver steatosis, and obesity in mice. Nutr. Res. 67, 78–89. doi: 10.1016/j.nutres.2019.03.008
Ishikawa, H., Matsumoto, S., Ohashi, Y., Imaoka, A., Setoyama, H., Umesaki, Y., et al. (2011). Beneficial effects of probiotic bifidobacterium and galacto-oligosaccharide in patients with ulcerative colitis: a randomized controlled study. Digestion 84, 128–133. doi: 10.1159/000322977
Jang, S. E., Jeong, J. J., Kim, J. K., Han, M. J., and Kim, D. H. (2018). Simultaneous Amelioratation of colitis and liver injury in mice by Bifidobacterium longum LC67 and Lactobacillus plantarum LC27. Sci. Rep. 8:7500. doi: 10.1038/s41598-018-25775-0
Jang, H. M., Lee, K. E., and Kim, D. H. (2019). The preventive and curative effects of Lactobacillus reuteri NK33 and Bifidobacterium adolescentis NK98 on immobilization stress-induced anxiety/depression and colitis in mice. Nutrients 11:819. doi: 10.3390/nu11040819
Je, I. G., Lee, D. G., Jeong, D. G., Hong, D., Yoon, J. M., Moon, J. S., et al. (2018). The probiotic, ID-JPL934, attenuates dextran sulfate sodium-induced colitis in mice through inhibition of proinflammatory cytokines expression. J. Med. Food 21, 858–865. doi: 10.1089/jmf.2017.4152
Jeong, Y. J., Kim, D. H., and Lee, K. W. (2022). Homeostasis effects of fermented Maillard reaction products by Lactobacillus gasseri 4M13 in dextran sulfate sodium-induced colitis mice. J. Sci. Food Agric. 102, 434–444. doi: 10.1002/jsfa.11374
Jia, D. J., Wang, Q. W., Hu, Y. Y., He, J. M., Ge, Q. W., Qi, Y. D., et al. (2022). Lactobacillus johnsonii alleviates colitis by TLR1/2-STAT3 mediated CD206(+) macrophages(IL-10) activation. Gut Microbes 14:2145843. doi: 10.1080/19490976.2022.2145843
Jia, L., Wu, R., Han, N., Fu, J., Luo, Z., Guo, L., et al. (2020). Porphyromonas gingivalis and Lactobacillus rhamnosus GG regulate the Th17/Treg balance in colitis via TLR4 and TLR2. Clin Transl Immunology 9:e1213. doi: 10.1002/cti2.1213
Jiang, X. E., Yang, S. M., Zhou, X. J., and Zhang, Y. (2020). Effects of mesalazine combined with bifid triple viable on intestinal flora, immunoglobulin and levels of cal, MMP-9, and MPO in feces of patients with ulcerative colitis. Eur. Rev. Med. Pharmacol. Sci. 24, 935–942. doi: 10.26355/eurrev_202001_20079
Jin, X., He, Y., Liu, Z., Zhou, Y., Chen, X., Wang, G., et al. (2020). Lactic acid bacteria exhibit similar antioxidant capacities in Caenorhabditis elegans- and Campylobacter jejuni-infected mice. RSC Adv. 10, 3329–3342. doi: 10.1039/C9RA06105C
Kamarli Altun, H., Akal Yildiz, E., and Akin, M. (2022). Impact of Synbiotic therapy on the quality of life in patients with mild-to-moderately active ulcerative colitis. J. Gastrointestin. Liver Dis. 31, 417–423. doi: 10.15403/jgld-4345
Kang, S., Lin, Z., Xu, Y., Park, M., Ji, G. E., Johnston, T. V., et al. (2022). A recombinant Bifidobacterium bifidum BGN4 strain expressing the streptococcal superoxide dismutase gene ameliorates inflammatory bowel disease. Microb. Cell Factories 21:113. doi: 10.1186/s12934-022-01840-2
Karimi, O., Pena, A. S., and Van Bodegraven, A. A. (2005). Probiotics (VSL#3) in arthralgia in patients with ulcerative colitis and Crohn’s disease: a pilot study. Drugs Today (Barc.) 41, 453–459. doi: 10.1358/dot.2005.41.7.917341
Kato, K., Mizuno, S., Umesaki, Y., Ishii, Y., Sugitani, M., Imaoka, A., et al. (2004). Randomized placebo-controlled trial assessing the effect of bifidobacteria-fermented milk on active ulcerative colitis. Aliment. Pharmacol. Ther. 20, 1133–1141. doi: 10.1111/j.1365-2036.2004.02268.x
Kaur, M., Dalal, R. L., Shaffer, S., Schwartz, D. A., and Rubin, D. T. (2020). Inpatient Management of Inflammatory Bowel Disease-Related Complications. Clin. Gastroenterol. Hepatol. 18, 1346–1355. doi: 10.1016/j.cgh.2019.12.040
Kaur, H., Gupta, T., Kapila, S., and Kapila, R. (2021). Protective effects of potential probiotic Lactobacillus rhamnosus (MTCC-5897) fermented whey on reinforcement of intestinal epithelial barrier function in a colitis-induced murine model. Food Funct. 12, 6102–6116. doi: 10.1039/D0FO02641G
Kelly, C. R., and Ananthakrishnan, A. N. (2019). Manipulating the microbiome with fecal transplantation to treat ulcerative colitis. JAMA 321, 151–152. doi: 10.1001/jama.2018.20397
Khalifa, A., Sheikh, A., and Ibrahim, H. I. M. (2022). Bacillus amyloliquefaciens enriched camel Milk attenuated colitis symptoms in mice model. Nutrients 14:1967. doi: 10.3390/nu14091967
Khan, I., Wei, J., Li, A., Liu, Z., Yang, P., Jing, Y., et al. (2022). Lactobacillus plantarum strains attenuated DSS-induced colitis in mice by modulating the gut microbiota and immune response. Int. Microbiol. 25, 587–603. doi: 10.1007/s10123-022-00243-y
Kim, J. K., and Cheon, J. H. (2022). Factors associated with the prescription of probiotics in patients with inflammatory bowel disease: a cross-sectional study. J Yeungnam Med Sci 40, 37–48. doi: 10.12701/jyms.2022.00031
Kim, W. K., Han, D. H., Jang, Y. J., Park, S. J., Jang, S. J., Lee, G., et al. (2021). Alleviation of DSS-induced colitis via Lactobacillus acidophilus treatment in mice. Food Funct. 12, 340–350. doi: 10.1039/D0FO01724H
Kim, D. H., Kim, S., Ahn, J. B., et al. (2020). Lactobacillus plantarum CBT LP3 ameliorates colitis via modulating T cells in mice. Int. J. Med. Microbiol. 310:151391. doi: 10.1016/j.ijmm.2020.151391
Kobayashi, T., Siegmund, B., le Berre, C., Wei, S. C., Ferrante, M., Shen, B., et al. (2020). Ulcerative colitis. Nat. Rev. Dis. Primers. 6:74. doi: 10.1038/s41572-020-0205-x
Kogut, M. H., Lee, A., and Santin, E. (2020). Microbiome and pathogen interaction with the immune system. Poult. Sci. 99, 1906–1913. doi: 10.1016/j.psj.2019.12.011
Kozhakhmetov, S., Babenko, D., Kozhakhmetova, S., Tuyakova, A., Nurgaziyev, M., Nurgozhina, A., et al. (2022). Therapeutic potential of metabolites from Lactobacillus rhamnosus and Mare’s Milk in the treatment of Dysbiosis. Biomed. Res. Int. 2022, 1–8. doi: 10.1155/2022/3851478
Krag, A., Israelsen, H., von Ryberg, B., Andersen, K. K., and Bendtsen, F. (2012). Safety and efficacy of Profermin® to induce remission in ulcerative colitis. World J. Gastroenterol. 18, 1773–1780. doi: 10.3748/wjg.v18.i15.1773
Krishna, M., Engevik, M., Queliza, K., Britto, S., Shah, R., Ruan, W., et al. (2022). Maternal Lactobacillus reuteri supplementation shifts the intestinal microbiome in mice and provides protection from experimental colitis in female offspring. FASEB Bioadv 4, 109–120. doi: 10.1096/fba.2021-00078
Kruis, W., Fric, P., Pokrotnieks, J., Lukás, M., Fixa, B., Kascák, M., et al. (2004). Maintaining remission of ulcerative colitis with the probiotic Escherichia coli Nissle 1917 is as effective as with standard mesalazine. Gut 53, 1617–1623. doi: 10.1136/gut.2003.037747
Kuenzig, M. E., Fung, S. G., Marderfeld, L., Mak, J. W. Y., Kaplan, G. G., Ng, S. C., et al. (2022). Twenty-first century trends in the global epidemiology of pediatric-onset inflammatory bowel disease: systematic review. Gastroenterology 162, 1147–1159.e4. doi: 10.1053/j.gastro.2021.12.282
Kumar, S., and Kumar, A. (2022). Microbial pathogenesis in inflammatory bowel diseases. Microb. Pathog. 163:105383. doi: 10.1016/j.micpath.2021.105383
Laake, K. O., Bjørneklett, A., Aamodt, G., Aabakken, L., Jacobsen, M., Bakka, A., et al. (2005). Outcome of four weeks’ intervention with probiotics on symptoms and endoscopic appearance after surgical reconstruction with a J-configurated ileal-pouch-anal-anastomosis in ulcerative colitis. Scand. J. Gastroenterol. 40, 43–51. doi: 10.1080/00365520410009339
Lara-Villoslada, F., Sierra, S., Martín, R., Delgado, S., Rodríguez, J. M., Olivares, M., et al. (2007). Safety assessment of two probiotic strains, Lactobacillus coryniformis CECT5711 and Lactobacillus gasseri CECT5714. J. Appl. Microbiol. 103, 175–184. doi: 10.1111/j.1365-2672.2006.03225.x
Lavelle, A., and Sokol, H. (2020). Gut microbiota-derived metabolites as key actors in inflammatory bowel disease. Nat. Rev. Gastroenterol. Hepatol. 17, 223–237. doi: 10.1038/s41575-019-0258-z
Leccese, G., Bibi, A., Mazza, S., Facciotti, F., Caprioli, F., Landini, P., et al. (2020). Probiotic Lactobacillus and Bifidobacterium strains counteract adherent-invasive Escherichia coli (AIEC) virulence and hamper IL-23/Th17 Axis in ulcerative colitis, but not in Crohn’s disease. Cells 9:1824. doi: 10.3390/cells9081824
Lee, S. Y., Lee, B. H., Park, J. H., Park, M. S., Ji, G. E., and Sung, M. K. (2022). Bifidobacterium bifidum BGN4 paraprobiotic supplementation alleviates experimental colitis by maintaining gut barrier and suppressing nuclear factor kappa B activation signaling molecules. J. Med. Food 25, 146–157. doi: 10.1089/jmf.2021.K.0150
Lee, J. H., Moon, G., Kwon, H. J., Jung, W. J., Seo, P. J., Baec, T. Y., et al. (2012). Effect of a probiotic preparation (VSL#3) in patients with mild to moderate ulcerative colitis. Korean J. Gastroenterol. 60, 94–101. doi: 10.4166/kjg.2012.60.2.94
Lemaitre, M., Kirchgesner, J., Rudnichi, A., Carrat, F., Zureik, M., Carbonnel, F., et al. (2017). Association between use of Thiopurines or tumor necrosis factor antagonists alone or in combination and risk of lymphoma in patients with inflammatory bowel disease. JAMA 318, 1679–1686. doi: 10.1001/jama.2017.16071
Li, P., Chen, G., Zhang, J., Pei, C., Chen, Y., Gong, J., et al. (2022). Live Lactobacillus acidophilus alleviates ulcerative colitis via the SCFAs/mitophagy/NLRP3 inflammasome axis. Food Funct. 13, 2985–2997. doi: 10.1039/D1FO03360C
Li, M., Ding, J., Stanton, C., Ross, R. P., Zhao, J., Yang, B., et al. (2023). Bifidobacterium longum subsp. infantis FJSYZ1M3 ameliorates DSS-induced colitis by maintaining the intestinal barrier, regulating inflammatory cytokines, and modifying gut microbiota. Food Funct. 14, 354–368. doi: 10.1039/D2FO03263E
Li, Z., Ma, S., Wang, X., et al. (2022). Pharmacokinetic and gut microbiota analyses revealed the effect of Lactobacillus acidophilus on the metabolism of Olsalazine in ulcerative colitis rats. Eur. J. Pharm. Sci. 175:106235. doi: 10.1016/j.ejps.2022.106235
Li, S., Qi, C., Zhu, H., Yu, R., Xie, C., Peng, Y., et al. (2019). Lactobacillus reuteri improves gut barrier function and affects diurnal variation of the gut microbiota in mice fed a high-fat diet. Food Funct. 10, 4705–4715. doi: 10.1039/C9FO00417C
Li, X., Xiao, Y., Huang, Y., Song, L., Li, M., and Ren, Z. (2022). Lactobacillus gasseri RW2014 ameliorates hyperlipidemia by modulating bile acid metabolism and gut microbiota composition in rats. Nutrients 14:4945. doi: 10.3390/nu14234945
Li, Y., Yang, S., Lun, J., Gao, J., Gao, X., Gong, Z., et al. (2020). Inhibitory effects of the Lactobacillus rhamnosus GG effector protein HM0539 on inflammatory response through the TLR4/MyD88/NF-кB Axis. Front. Immunol. 11:551449. doi: 10.3389/fimmu.2020.551449
Li, Z., Zhang, S., Xu, L., Fang, X., Wan, Y., Yu, D., et al. (2022). A tetrapeptide from maize combined with probiotics exerted strong anti-inflammatory effects and modulated gut microbiota in DSS-induced colitis mice. Food Funct. 13, 12602–12618. doi: 10.1039/D2FO02678C
Liang, B., Yuan, Y., Peng, X. J., Liu, X. L., Hu, X. K., and Xing, D. M. (2022). Current and future perspectives for Helicobacter pylori treatment and management: from antibiotics to probiotics. Front. Cell. Infect. Microbiol. 12:1042070. doi: 10.3389/fcimb.2022.1042070
Liu, H. Y., Giraud, A., Seignez, C., Ahl, D., Guo, F., Sedin, J., et al. (2021). Distinct B cell subsets in Peyer’s patches convey probiotic effects by Limosilactobacillus reuteri. Microbiome 9:198. doi: 10.1186/s40168-021-01128-4
Liu, H. Y., Gu, F., Zhu, C., Yuan, L., Zhu, C., Zhu, M., et al. (2022). Epithelial heat shock proteins mediate the protective effects of Limosilactobacillus reuteri in dextran sulfate sodium-induced colitis. Front. Immunol. 13:865982. doi: 10.3389/fimmu.2022.865982
Liu, Z., Liu, R., Gao, H., Jung, S., Gao, X., Sun, R., et al. (2023). Genetic architecture of the inflammatory bowel diseases across east Asian and European ancestries. Nat. Genet. 55, 796–806. doi: 10.1038/s41588-023-01384-0
Liu, Z., Liu, F., Wang, W., Sun, C., Gao, D., Ma, J., et al. (2020). Study of the alleviation effects of a combination of Lactobacillus rhamnosus and inulin on mice with colitis. Food Funct. 11, 3823–3837. doi: 10.1039/C9FO02992C
Liu, Y., Liu, Q., Zhao, J., Zhang, H., Zhai, Q., and Chen, W. (2022). Strain-specific regulative effects of Lactobacillus plantarum on intestinal barrier dysfunction are associated with their capsular polysaccharides. Int. J. Biol. Macromol. 222, 1343–1352. doi: 10.1016/j.ijbiomac.2022.09.136
Liu, Y., Sheng, Y., Pan, Q., Xue, Y., Yu, L., Tian, F., et al. (2020). Identification of the key physiological characteristics of Lactobacillus plantarum strains for ulcerative colitis alleviation. Food Funct. 11, 1279–1291. doi: 10.1039/C9FO02935D
Liu, Y., Tian, X., He, B., Hoang, T. K., Taylor, C. M., Blanchard, E., et al. (2019). Lactobacillus reuteri DSM 17938 feeding of healthy newborn mice regulates immune responses while modulating gut microbiota and boosting beneficial metabolites. Am. J. Physiol. Gastrointest. Liver Physiol. 317, G824–g838. doi: 10.1152/ajpgi.00107.2019
Liu, Z., and Wang, H. (2021). Probiotics alleviate inflammatory bowel disease in mice by regulating intestinal microorganisms-bile acid-NLRP3 inflammasome pathway. Acta Biochim. Pol. 68, 687–693. doi: 10.18388/abp.2020_5597
Liu, B., Yang, L., Wu, Y., and Zhao, X. (2022). Protective effect of Limosilactobacillus fermentum HFY06 on dextran sulfate sodium-induced colitis in mice. Front. Microbiol. 13:935792. doi: 10.3389/fmicb.2022.935792
Ma, F., Sun, M., Song, Y., Wang, A., Jiang, S., Qian, F., et al. (2022). Lactiplantibacillus plantarum-12 alleviates inflammation and Colon Cancer symptoms in AOM/DSS-treated mice through modulating the intestinal microbiome and Metabolome. Nutrients 14:1916. doi: 10.3390/nu14091916
Martini, E., Krug, S. M., Siegmund, B., Neurath, M. F., and Becker, C. (2017). Mend your fences: the epithelial barrier and its relationship with mucosal immunity in inflammatory bowel disease. Cell. Mol. Gastroenterol. Hepatol. 4, 33–46. doi: 10.1016/j.jcmgh.2017.03.007
Masco, L., Ventura, M., Zink, R., Huys, G., and Swings, J. (2004). Polyphasic taxonomic analysis of Bifidobacterium animalis and Bifidobacterium lactis reveals relatedness at the subspecies level: reclassification of Bifidobacterium animalis as Bifidobacterium animalis subsp. animalis subsp. nov. and Bifidobacterium lactis as Bifidobacterium animalis subsp. lactis subsp. nov. Int. J. Syst. Evol. Microbiol. 54, 1137–1143. doi: 10.1099/ijs.0.03011-0
Matsuoka, K., Uemura, Y., Kanai, T., Kunisaki, R., Suzuki, Y., Yokoyama, K., et al. (2018). Efficacy of Bifidobacterium breve fermented Milk in maintaining remission of ulcerative colitis. Dig. Dis. Sci. 63, 1910–1919. doi: 10.1007/s10620-018-4946-2
Mattarelli, P., Bonaparte, C., Pot, B., and Biavati, B. (2008). Proposal to reclassify the three biotypes of Bifidobacterium longum as three subspecies: Bifidobacterium longum subsp. longum subsp. nov., Bifidobacterium longum subsp. infantis comb. nov. and Bifidobacterium longum subsp. suis comb. nov. Int. J. Syst. Evol. Microbiol. 58, 767–772. doi: 10.1099/ijs.0.65319-0
Matthes, H., Krummenerl, T., Giensch, M., Wolff, C., and Schulze, J. (2010). Clinical trial: probiotic treatment of acute distal ulcerative colitis with rectally administered Escherichia coli Nissle 1917 (EcN). BMC Complement. Altern. Med. 10:13. doi: 10.1186/1472-6882-10-13
Mauras, A., Chain, F., Faucheux, A., Ruffié, P., Gontier, S., Ryffel, B., et al. (2018). A new Bifidobacteria expression SysTem (BEST) to produce and deliver Interleukin-10 in Bifidobacterium bifidum. Front. Microbiol. 9:3075. doi: 10.3389/fmicb.2018.03075
Metwaly, A., Reitmeier, S., and Haller, D. (2022). Microbiome risk profiles as biomarkers for inflammatory and metabolic disorders. Nat. Rev. Gastroenterol. Hepatol. 19, 383–397. doi: 10.1038/s41575-022-00581-2
Mianzhi, Y., and Shah, N. P. (2017). Contemporary nucleic acid-based molecular techniques for detection, identification, and characterization of Bifidobacterium. Crit. Rev. Food Sci. Nutr. 57, 987–1016. doi: 10.1080/10408398.2015.1023761
Miele, E., Pascarella, F., Giannetti, E., Quaglietta, L., Baldassano, R. N., and Staiano, A. (2009). Effect of a probiotic preparation (VSL#3) on induction and maintenance of remission in children with ulcerative colitis. Am. J. Gastroenterol. 104, 437–443. doi: 10.1038/ajg.2008.118
Mimura, T., Rizzello, F., Helwig, U., Poggioli, G., Schreiber, S., Talbot, I. C., et al. (2004). Once daily high dose probiotic therapy (VSL#3) for maintaining remission in recurrent or refractory pouchitis. Gut 53, 108–114. doi: 10.1136/gut.53.1.108
Miyamoto, J., Mizukure, T., Park, S. B., Kishino, S., Kimura, I., Hirano, K., et al. (2015). A gut microbial metabolite of linoleic acid, 10-hydroxy-cis-12-octadecenoic acid, ameliorates intestinal epithelial barrier impairment partially via GPR40-MEK-ERK pathway. J. Biol. Chem. 290, 2902–2918. doi: 10.1074/jbc.M114.610733
Mizoguchi, E., Low, D., Ezaki, Y., and Okada, T. (2020). Recent updates on the basic mechanisms and pathogenesis of inflammatory bowel diseases in experimental animal models. Intest Res 18, 151–167. doi: 10.5217/ir.2019.09154
Molina-Tijeras, J. A., Diez-Echave, P., Vezza, T., et al. (2021). Lactobacillus fermentum CECT5716 ameliorates high fat diet-induced obesity in mice through modulation of gut microbiota dysbiosis. Pharmacol. Res. 167:105471. doi: 10.1016/j.phrs.2021.105471
Nan, X., Zhao, W., Liu, W. H., Li, Y., Li, N., Hong, Y., et al. (2023). Bifidobacterium animalis subsp. lactis BL-99 ameliorates colitis-related lung injury in mice by modulating short-chain fatty acid production and inflammatory monocytes/macrophages. Food Funct. 14, 1099–1112. doi: 10.1039/D2FO03374G
Neurath, M. F. (2019). Targeting immune cell circuits and trafficking in inflammatory bowel disease. Nat. Immunol. 20, 970–979. doi: 10.1038/s41590-019-0415-0
Niu, M. M., Guo, H. X., Cai, J. W., and Meng, X. C. (2022). Bifidobacterium breve alleviates DSS-induced colitis in mice by maintaining the mucosal and epithelial barriers and modulating gut microbes. Nutrients 14:3671. doi: 10.3390/nu14183671
O’Callaghan, A., and Van Sinderen, D. (2016). Bifidobacteria and their role as members of the human gut microbiota. Front. Microbiol. 7:925. doi: 10.3389/fmicb.2016.00925
Oka, A., and Sartor, R. B. (2020). Microbial-based and microbial-targeted therapies for inflammatory bowel diseases. Dig. Dis. Sci. 65, 757–788. doi: 10.1007/s10620-020-06090-z
Oliva, S., di Nardo, G., Ferrari, F., Mallardo, S., Rossi, P., Patrizi, G., et al. (2012). Randomised clinical trial: the effectiveness of Lactobacillus reuteri ATCC 55730 rectal enema in children with active distal ulcerative colitis. Aliment. Pharmacol. Ther. 35, 327–334. doi: 10.1111/j.1365-2036.2011.04939.x
Ooijevaar, R. E., Terveer, E. M., Verspaget, H. W., Kuijper, E. J., and Keller, J. J. (2019). Clinical application and potential of fecal microbiota transplantation. Annu. Rev. Med. 70, 335–351. doi: 10.1146/annurev-med-111717-122956
Ortiz-Rivera, Y., Sánchez-Vega, R., Gutiérrez-Méndez, N., León-Félix, J., Acosta-Muñiz, C., and Sepulveda, D. R. (2017). Production of reuterin in a fermented milk product by Lactobacillus reuteri: inhibition of pathogens, spoilage microorganisms, and lactic acid bacteria. J. Dairy Sci. 100, 4258–4268. doi: 10.3168/jds.2016-11534
Pagnini, C., Corleto, V. D., Martorelli, M., Lanini, C., D’Ambra, G., Giulio, E. D., et al. (2018). Mucosal adhesion and anti-inflammatory effects of Lactobacillus rhamnosus GG in the human colonic mucosa: a proof-of-concept study. World J. Gastroenterol. 24, 4652–4662. doi: 10.3748/wjg.v24.i41.4652
Palumbo, V. D., Romeo, M., Gammazza, A. M., Carini, F., Damiani, P., Damiano, G., et al. (2016). The long-term effects of probiotics in the therapy of ulcerative colitis: a clinical study. Biomed. Pap. Med. Fac. Univ. Palacky Olomouc Czech Repub. 160, 372–377. doi: 10.5507/bp.2016.044
Pan, Y., Ning, Y., Hu, J., Wang, Z., Chen, X., and Zhao, X. (2021). The preventive effect of Lactobacillus plantarum ZS62 on DSS-induced IBD by regulating oxidative stress and the immune response. Oxidative Med. Cell. Longev. 2021, 1–16. doi: 10.1155/2021/9416794
Pang, B., Jin, H., Liao, N., Li, J., Jiang, C., Shao, D., et al. (2021). Lactobacillus rhamnosus from human breast milk ameliorates ulcerative colitis in mice via gut microbiota modulation. Food Funct. 12, 5171–5186. doi: 10.1039/D0FO03479G
Paramsothy, S., Kamm, M. A., Kaakoush, N. O., Walsh, A. J., van den Bogaerde, J., Samuel, D., et al. (2017). Multidonor intensive faecal microbiota transplantation for active ulcerative colitis: a randomised placebo-controlled trial. Lancet 389, 1218–1228. doi: 10.1016/S0140-6736(17)30182-4
Park, S. K., Kang, S. B., Kim, S. S., Kim, T. O., Cha, J. M., Im, J. P., et al. (2022). Additive effect of probiotics (Mutaflor) on 5-aminosalicylic acid therapy in patients with ulcerative colitis. Korean J. Intern. Med. 37, 949–957. doi: 10.3904/kjim.2021.458
Paveljšek, D., Juvan, P., Košir, R., Rozman, D., Hacin, B., Ivičak-Kocjan, K., et al. (2018). Lactobacillus fermentum L930BB and Bifidobacterium animalis subsp. animalis IM386 initiate signalling pathways involved in intestinal epithelial barrier protection. Benef Microbes 9, 515–525. doi: 10.3920/BM2017.0107
Peng, K. Y., Gu, J. F., Su, S. L., Zhu, Y., Guo, J. M., Qian, D. W., et al. (2021). Salvia miltiorrhiza stems and leaves total phenolic acids combination with tanshinone protect against DSS-induced ulcerative colitis through inhibiting TLR4/PI3K/AKT/mTOR signaling pathway in mice. J. Ethnopharmacol. 264:113052. doi: 10.1016/j.jep.2020.113052
Peng, W., Li, Y. H., Yang, G., Duan, J. L., Yang, L. Y., Chen, L. X., et al. (2022). Oral administration of Lactobacillus delbrueckii enhances intestinal immunity through inducing dendritic cell activation in suckling piglets. Food Funct. 13, 2570–2580. doi: 10.1039/D1FO03864H
Popov, J., Caputi, V., Nandeesha, N., Rodriguez, D. A., and Pai, N. (2021). Microbiota-immune interactions in ulcerative colitis and colitis associated Cancer and emerging microbiota-based therapies. Int. J. Mol. Sci. 22:11365. doi: 10.3390/ijms222111365
Pradhan, D., Singh, R., Tyagi, A., Rashmi, H. M., Batish, V. K., and Grover, S. (2019). Assessing the safety and efficacy of Lactobacillus plantarum MTCC 5690 and Lactobacillus fermentum MTCC 5689 in colitis mouse model. Probiotics Antimicrob Proteins 11, 910–920. doi: 10.1007/s12602-018-9489-5
Pronio, A., Montesani, C., Butteroni, C., Vecchione, S., Mumolo, G., Vestri, A. R., et al. (2008). Probiotic administration in patients with ileal pouch-anal anastomosis for ulcerative colitis is associated with expansion of mucosal regulatory cells. Inflamm. Bowel Dis. 14, 662–668. doi: 10.1002/ibd.20369
Qi, C., Ding, M., Li, S., Zhou, Q., Li, D., Yu, R., et al. (2021). Sex-dependent modulation of immune development in mice by secretory IgA-coated Lactobacillus reuteri isolated from breast milk. J. Dairy Sci. 104, 3863–3875. doi: 10.3168/jds.2020-19437
Qian, Y., Ma, L., Zeng, M., and Liu, Z. (2022). Amelioration of dextran sulfate sodium-induced colitis by autoinducer-2-deficient Lactiplantibacillus plantarum is mediated by anti-inflammatory effects and alleviation of dysbiosis of the gut microbiota. Front. Microbiol. 13:1013586. doi: 10.3389/fmicb.2022.1013586
Qiao, Z., Wang, X., Wang, C., Han, J., Qi, W., Zhang, H., et al. (2022). Lactobacillus paracasei BD5115-derived 2-Hydroxy-3-Methylbutyric acid promotes intestinal epithelial cells proliferation by Upregulating the MYC signaling pathway. Front. Nutr. 9:799053. doi: 10.3389/fnut.2022.799053
Qu, D., Yu, L., Tian, F., Zhang, H., Chen, W., Gu, Z., et al. (2023). Bifidobacterium bifidum FJSWX19M5 alleviated 2,4,6-trinitrobenzene sulfonic acid (TNBS)-induced chronic colitis by mitigating gut barrier injury and increasing regulatory T cells. Food Funct. 14, 181–194. doi: 10.1039/D2FO02659G
Raine, T., Bonovas, S., Burisch, J., Kucharzik, T., Adamina, M., Annese, V., et al. (2022). ECCO guidelines on therapeutics in ulcerative colitis: medical treatment. J. Crohns Colitis 16, 2–17. doi: 10.1093/ecco-jcc/jjab178
Redondo, N., Nova, E., Gheorghe, A., Díaz, L. E., Hernández, A., and Marcos, A. (2017). Erratum to: evaluation of Lactobacillus coryniformis CECT5711 strain as a coadjuvant in a vaccination process: a randomised clinical trial in healthy adults. Nutr. Metab. (Lond.) 14:39. doi: 10.1186/s12986-017-0193-3
Reed, B., Rea, K. E., Claar, R. L., van Tilburg, M. A. L., and Levy, R. L. (2021). Passive coping associations with self-esteem and health-related quality of life in youth with inflammatory bowel disease. Front. Psychol. 12:670902. doi: 10.3389/fpsyg.2021.670902
Roggero, P., Liotto, N., Pozzi, C., Braga, D., Troisi, J., Menis, C., et al. (2020). Analysis of immune, microbiota and metabolome maturation in infants in a clinical trial of Lactobacillus paracasei CBA L74-fermented formula. Nat. Commun. 11:2703. doi: 10.1038/s41467-020-16582-1
Rong, J., Liu, S., Hu, C., and Liu, C. (2019). Single probiotic supplement suppresses colitis-associated colorectal tumorigenesis by modulating inflammatory development and microbial homeostasis. J. Gastroenterol. Hepatol. 34, 1182–1192. doi: 10.1111/jgh.14516
Saviano, A., Brigida, M., Migneco, A., Gunawardena, G., Zanza, C., Candelli, M., et al. (2021). Lactobacillus reuteri DSM 17938 (Limosilactobacillus reuteri) in diarrhea and constipation: two sides of the same coin? Medicina (Kaunas) 57:643. doi: 10.3390/medicina57070643
Schiavi, E., Gleinser, M., Molloy, E., Groeger, D., Frei, R., Ferstl, R., et al. (2016). The surface-associated exopolysaccharide of Bifidobacterium longum 35624 plays an essential role in dampening host Proinflammatory responses and repressing local TH17 responses. Appl. Environ. Microbiol. 82, 7185–7196. doi: 10.1128/AEM.02238-16
Schlee, M., Wehkamp, J., Altenhoefer, A., Oelschlaeger, T. A., Stange, E. F., and Fellermann, K. (2007). Induction of human beta-defensin 2 by the probiotic Escherichia coli Nissle 1917 is mediated through flagellin. Infect. Immun. 75, 2399–2407. doi: 10.1128/IAI.01563-06
Schultz, M. (2008). Clinical use of E. coli Nissle 1917 in inflammatory bowel disease. Inflamm. Bowel Dis. 14, 1012–1018. doi: 10.1002/ibd.20377
Schulz-Kuhnt, A., Neurath, M. F., Wirtz, S., and Atreya, I. (2021). Innate lymphoid cells as regulators of epithelial integrity: therapeutic implications for inflammatory bowel diseases. Front Med (Lausanne) 8:656745. doi: 10.3389/fmed.2021.656745
Segal, J. P., LeBlanc, J. F., and Hart, A. L. (2021). Ulcerative colitis: an update. Clin. Med. (Lond.) 21, 135–139. doi: 10.7861/clinmed.2021-0080
Selle, K., and Klaenhammer, T. R. (2013). Genomic and phenotypic evidence for probiotic influences of Lactobacillus gasseri on human health. FEMS Microbiol. Rev. 37, 915–935. doi: 10.1111/1574-6976.12021
Selvamani, S., Mehta, V., Ali el Enshasy, H., Thevarajoo, S., el Adawi, H., Zeini, I., et al. (2022). Efficacy of probiotics-based interventions as therapy for inflammatory bowel disease: a recent update. Saudi J Biol Sci 29, 3546–3567. doi: 10.1016/j.sjbs.2022.02.044
Shakurnia, A., Sheikhi, A., Mirzapour, M., Baharifar, V., Baharifar, N., Aghamohammadi, N., et al. (2019). Sugarcane molasses enhances TGF-β secretion and FOXP3 gene expression by Bifidobacterium animalis subsp. lactis stimulated PBMCs of ulcerative colitis patients. Complement. Ther. Med. 47:102210. doi: 10.1016/j.ctim.2019.102210
Sharma, S., Bhatia, R., Devi, K., Rawat, A., Singh, S., Bhadada, S. K., et al. (2023). A synbiotic combination of Bifidobacterium longum Bif10 and Bifidobacterium breve Bif11, isomaltooligosaccharides and finger millet arabinoxylan prevents dextran sodium sulphate induced ulcerative colitis in mice. Int. J. Biol. Macromol. 231:123326. doi: 10.1016/j.ijbiomac.2023.123326
Shen, J., Zuo, Z. X., and Mao, A. P. (2014). Effect of probiotics on inducing remission and maintaining therapy in ulcerative colitis, Crohn’s disease, and pouchitis: meta-analysis of randomized controlled trials. Inflamm. Bowel Dis. 20, 21–35. doi: 10.1097/01.MIB.0000437495.30052.be
Sheng, K., He, S., Sun, M., Zhang, G., Kong, X., Wang, J., et al. (2020). Synbiotic supplementation containing Bifidobacterium infantis and xylooligosaccharides alleviates dextran sulfate sodium-induced ulcerative colitis. Food Funct. 11, 3964–3974. doi: 10.1039/D0FO00518E
Sheng, K., Xu, Y., Kong, X., Wang, J., Zha, X., and Wang, Y. (2021). Probiotic Bacillus cereus alleviates dextran sulfate sodium-induced colitis in mice through improvement of the intestinal barrier function, anti-inflammation, and gut microbiota modulation. J. Agric. Food Chem. 69, 14810–14823. doi: 10.1021/acs.jafc.1c03375
Shi, J., du, P., Xie, Q., Wang, N., Li, H., Smith, E. E., et al. (2020). Protective effects of tryptophan-catabolizing Lactobacillus plantarum KLDS 1.0386 against dextran sodium sulfate-induced colitis in mice. Food Funct. 11, 10736–10747. doi: 10.1039/D0FO02622K
Shi, J., Xie, Q., Yue, Y., Chen, Q., Zhao, L., Evivie, S. E., et al. (2021). Gut microbiota modulation and anti-inflammatory properties of mixed lactobacilli in dextran sodium sulfate-induced colitis in mice. Food Funct. 12, 5130–5143. doi: 10.1039/D1FO00317H
Singh, S., Murad, M. H., Fumery, M., Sedano, R., Jairath, V., Panaccione, R., et al. (2021). Comparative efficacy and safety of biologic therapies for moderate-to-severe Crohn’s disease: a systematic review and network meta-analysis. Lancet Gastroenterol. Hepatol. 6, 1002–1014. doi: 10.1016/S2468-1253(21)00312-5
Son, S. J., Koh, J. H., Park, M. R., Ryu, S., Lee, W. J., Yun, B., et al. (2019). Effect of the Lactobacillus rhamnosus strain GG and tagatose as a synbiotic combination in a dextran sulfate sodium-induced colitis murine model. J. Dairy Sci. 102, 2844–2853. doi: 10.3168/jds.2018-15013
Sood, A., Midha, V., Makharia, G. K., Ahuja, V., Singal, D., Goswami, P., et al. (2009). The probiotic preparation, VSL#3 induces remission in patients with mild-to-moderately active ulcerative colitis. Clin Gastroenterol Hepatol 7, 1202–1209. doi: 10.1016/j.cgh.2009.07.016
Spinelli, A., Bonovas, S., Burisch, J., Kucharzik, T., Adamina, M., Annese, V., et al. (2022). ECCO guidelines on therapeutics in ulcerative colitis: surgical treatment. J. Crohns Colitis 16, 179–189. doi: 10.1093/ecco-jcc/jjab177
Stavropoulou, E., and Bezirtzoglou, E. (2020). Probiotics in medicine: a long debate. Front. Immunol. 11:2192. doi: 10.3389/fimmu.2020.02192
Su, G. L., Ko, C. W., Bercik, P., Falck-Ytter, Y., Sultan, S., Weizman, A. V., et al. (2020). AGA clinical practice guidelines on the role of probiotics in the Management of Gastrointestinal Disorders. Gastroenterology 159, 697–705. doi: 10.1053/j.gastro.2020.05.059
Sun, M., Liu, Y., Song, Y., Gao, Y., Zhao, F., Luo, Y., et al. (2020). The ameliorative effect of Lactobacillus plantarum-12 on DSS-induced murine colitis. Food Funct. 11, 5205–5222. doi: 10.1039/D0FO00007H
Sun, J., Qi, C., Zhu, H., Zhou, Q., Xiao, H., le, G., et al. (2019). IgA-targeted Lactobacillus jensenii modulated gut barrier and microbiota in high-fat diet-fed mice. Front. Microbiol. 10:1179. doi: 10.3389/fmicb.2019.01179
Suzuki, A., Mitsuyama, K., Koga, H., Tomiyasu, N., Masuda, J., Takaki, K., et al. (2006). Bifidogenic growth stimulator for the treatment of active ulcerative colitis: a pilot study. Nutrition 22, 76–81. doi: 10.1016/j.nut.2005.04.013
Sýkora, J., Pomahačová, R., Kreslová, M., Cvalínová, D., Štych, P., and Schwarz, J. (2018). Current global trends in the incidence of pediatric-onset inflammatory bowel disease. World J. Gastroenterol. 24, 2741–2763. doi: 10.3748/wjg.v24.i25.2741
Takeda, Y., Nakase, H., Namba, K., Inoue, S., Ueno, S., Uza, N., et al. (2009). Upregulation of T-bet and tight junction molecules by Bifidobactrium longum improves colonic inflammation of ulcerative colitis. Inflamm. Bowel Dis. 15, 1617–1618. doi: 10.1002/ibd.20861
Tamaki, H., Nakase, H., Inoue, S., Kawanami, C., Itani, T., Ohana, M., et al. (2016). Efficacy of probiotic treatment with Bifidobacterium longum 536 for induction of remission in active ulcerative colitis: a randomized, double-blinded, placebo-controlled multicenter trial. Dig. Endosc. 28, 67–74. doi: 10.1111/den.12553
Tan, F., Deng, Y., Guo, J., Zhou, Z., and Luo, H. (2022). Effect of mesalazine combined with probiotics on inflammation and immune function of patients with inflammatory bowel disease. Am. J. Transl. Res. 14, 8234–8242.
Tanaka, A., Kanmura, S., Morinaga, Y., Kawabata, K., Arima, S., Sasaki, F., et al. (2020). Oral administration of Lactobacillus plantarum 06CC2 prevents experimental colitis in mice via an anti-inflammatory response. Mol. Med. Rep. 21, 1181–1191. doi: 10.3892/mmr.2020.10925
Turner, D., Ruemmele, F. M., Orlanski-Meyer, E., Griffiths, A. M., de Carpi, J. M., Bronsky, J., et al. (2018a). Management of Paediatric Ulcerative Colitis, part 1: ambulatory care-an evidence-based guideline from European Crohn’s and colitis organization and European Society of Paediatric Gastroenterology, Hepatology and nutrition. J. Pediatr. Gastroenterol. Nutr. 67, 257–291. doi: 10.1097/MPG.0000000000002035
Turner, D., Ruemmele, F. M., Orlanski-Meyer, E., Griffiths, A. M., de Carpi, J. M., Bronsky, J., et al. (2018b). Management of paediatric Ulcerative Colitis, part 2: acute severe colitis-an evidence-based consensus guideline from the European Crohn’s and colitis organization and the European Society of Paediatric Gastroenterology, Hepatology and nutrition. J. Pediatr. Gastroenterol. Nutr. 67, 292–310. doi: 10.1097/MPG.0000000000002036
Tursi, A., Brandimarte, G., Papa, A., Giglio, A., Elisei, W., Giorgetti, G. M., et al. (2010). Treatment of relapsing mild-to-moderate ulcerative colitis with the probiotic VSL#3 as adjunctive to a standard pharmaceutical treatment: a double-blind, randomized, placebo-controlled study. Am. J. Gastroenterol. 105, 2218–2227. doi: 10.1038/ajg.2010.218
Usman, U., and Hosono, A. (1999). Bile tolerance, taurocholate deconjugation, and binding of cholesterol by Lactobacillus gasseri strains. J. Dairy Sci. 82, 243–248. doi: 10.3168/jds.S0022-0302(99)75229-X
Valdes, L. M., and Sobrin, L. (2020). Uveitis therapy: the corticosteroid options. Drugs 80, 765–773. doi: 10.1007/s40265-020-01314-y
Van der Waal, M. B., Flach, J., Browne, P. D., Besseling-van der Vaart, I., Claassen, E., van de Burgwal, L. H. M., et al. (2019). Probiotics for improving quality of life in ulcerative colitis: Exploring the patient perspective. PharmaNutrition 7:100139. doi: 10.1016/j.phanu.2018.100139
Veereman-Wauters, G. (2003). Pouchitis prevention with probiotics. J. Pediatr. Gastroenterol. Nutr. 37:636. doi: 10.1097/00005176-200311000-00027
Vich Vila, A., Imhann, F., Collij, V., Jankipersadsing, S. A., Gurry, T., Mujagic, Z., et al. (2018). Gut microbiota composition and functional changes in inflammatory bowel disease and irritable bowel syndrome. Sci. Transl. Med. 10:eaap8914. doi: 10.1126/scitranslmed.aap8914
Wan, C., Qian, W. W., Liu, W., Pi, X., Tang, M. T., Wang, X. L., et al. (2022). Exopolysaccharide from Lactobacillus rhamnosus ZFM231 alleviates DSS-induced colitis in mice by regulating gut microbiota. J. Sci. Food Agric. 102, 7087–7097. doi: 10.1002/jsfa.12070
Wang, G., Chen, Y., Fei, S., Xie, C., Xia, Y., and Ai, L. (2021). Colonisation with endogenous Lactobacillus reuteri R28 and exogenous Lactobacillus plantarum AR17-1 and the effects on intestinal inflammation in mice. Food Funct. 12, 2481–2488. doi: 10.1039/D0FO02624G
Wang, H., Fan, C., Zhao, Z., Zhai, Z., and Hao, Y. (2022). Anti-inflammatory effect of Bifidobacterium animalis subsp. lactis A6 on DSS-induced colitis in mice. J. Appl. Microbiol. 133, 2063–2073. doi: 10.1111/jam.15681
Wang, Y., Fang, Z., Zhai, Q., Cui, S., Zhao, J., Zhang, H., et al. (2021). Supernatants of Bifidobacterium longum and Lactobacillus plantarum strains exhibited Antioxidative effects on A7R5 cells. Microorganisms 9:452. doi: 10.3390/microorganisms9020452
Wang, Y., Guo, Y., Chen, H., Wei, H., and Wan, C. (2018). Potential of Lactobacillus plantarum ZDY2013 and Bifidobacterium bifidum WBIN03 in relieving colitis by gut microbiota, immune, and anti-oxidative stress. Can. J. Microbiol. 64, 327–337. doi: 10.1139/cjm-2017-0716
Wang, S. Y., Ho, Y. F., Chen, Y. P., and Chen, M. J. (2015). Effects of a novel encapsulating technique on the temperature tolerance and anti-colitis activity of the probiotic bacterium Lactobacillus kefiranofaciens M1. Food Microbiol. 46, 494–500. doi: 10.1016/j.fm.2014.09.015
Wang, X., Li, D., Meng, Z., Kim, K., and Oh, S. (2022). Latilactobacillus curvatus BYB3 isolated from Kimchi alleviates dextran sulfate sodium (DSS)-induced colitis in mice by inhibiting IL-6 and TNF-R1 production. J. Microbiol. Biotechnol. 32, 348–354. doi: 10.4014/jmb.2109.09054
Wang, G., Liu, Y., Lu, Z., Yang, Y., Xia, Y., Lai, P. F. H., et al. (2019). The ameliorative effect of a Lactobacillus strain with good adhesion ability against dextran sulfate sodium-induced murine colitis. Food Funct. 10, 397–409. doi: 10.1039/C8FO01453A
Wang, X., Xu, M., Xu, D., Ma, K., Zhang, C., Wang, G., et al. (2022). Structural and prebiotic activity analysis of the polysaccharide produced by Lactobacillus helveticus SNA12. Carbohydr. Polym. 296:119971. doi: 10.1016/j.carbpol.2022.119971
Wang, Z., Yang, L., Tang, H., Zhang, K., Chen, Q., Liu, C., et al. (2022). In vivo evidence of the prevents DSS-induced colitis of Lactiplantibacillus plantarum L15. Front. Microbiol. 13:1028919. doi: 10.3389/fmicb.2022.1028919
Wang, T., Zhang, L., Wang, P., Liu, Y., Wang, G., Shan, Y., et al. (2022). Lactobacillus coryniformis MXJ32 administration ameliorates azoxymethane/dextran sulfate sodium-induced colitis-associated colorectal cancer via reshaping intestinal microenvironment and alleviating inflammatory response. Eur. J. Nutr. 61, 85–99. doi: 10.1007/s00394-021-02627-8
Wieërs, G., Belkhir, L., Enaud, R., Leclercq, S., De Foy, J. M. P., Dequenne, I., et al. (2019). How probiotics affect the microbiota. Front. Cell. Infect. Microbiol. 9:454. doi: 10.3389/fcimb.2019.00454
Wildt, S., Nordgaard, I., Hansen, U., Brockmann, E., and Rumessen, J. J. (2011). A randomised double-blind placebo-controlled trial with Lactobacillus acidophilus La-5 and Bifidobacterium animalis subsp. lactis BB-12 for maintenance of remission in ulcerative colitis. J. Crohns Colitis 5, 115–121. doi: 10.1016/j.crohns.2010.11.004
Wrzosek, L., Miquel, S., Noordine, M. L., Bouet, S., Chevalier-Curt, M. J., Robert, V., et al. (2013). Bacteroides thetaiotaomicron and Faecalibacterium prausnitzii influence the production of mucus glycans and the development of goblet cells in the colonic epithelium of a gnotobiotic model rodent. BMC Biol. 11:61. doi: 10.1186/1741-7007-11-61
Wu, Y., Jha, R., Li, A., Liu, H., Zhang, Z., Zhang, C., et al. (2022b). Probiotics (Lactobacillus plantarum HNU082) supplementation relieves ulcerative colitis by affecting intestinal barrier functions, immunity-related gene expression, gut microbiota, and metabolic pathways in mice. Microbiol Spectr 10:e0165122. doi: 10.1128/spectrum.01651-22
Wu, Y., Li, A., Liu, H., Zhang, Z., Zhang, C., Ma, C., et al. (2022a). Lactobacillus plantarum HNU082 alleviates dextran sulfate sodium-induced ulcerative colitis in mice through regulating gut microbiome. Food Funct. 13, 10171–10185. doi: 10.1039/D2FO02303B
Xia, B., Yu, J., He, T., Liu, X., Su, J., Wang, M., et al. (2020). Lactobacillus johnsonii L531 ameliorates enteritis via elimination of damaged mitochondria and suppression of SQSTM1-dependent mitophagy in a Salmonella infantis model of piglet diarrhea. FASEB J. 34, 2821–2839. doi: 10.1096/fj.201901445RRR
Xiao, F., Dong, F., Li, X., Li, Y., Yu, G., Liu, Z., et al. (2022). Bifidobacterium longum CECT 7894 improves the efficacy of infliximab for DSS-induced colitis via regulating the gut microbiota and bile acid metabolism. Front. Pharmacol. 13:902337. doi: 10.3389/fphar.2022.902337
Xin, J., Zeng, D., Wang, H., Sun, N., Zhao, Y., Dan, Y., et al. (2020). Probiotic Lactobacillus johnsonii BS15 promotes growth performance, intestinal immunity, and gut microbiota in piglets. Probiotics Antimicrob Proteins 12, 184–193. doi: 10.1007/s12602-018-9511-y
Xu, H., Hiraishi, K., Kurahara, L. H., Nakano-Narusawa, Y., Li, X., Hu, Y., et al. (2021). Inhibitory effects of breast Milk-derived Lactobacillus rhamnosus Probio-M9 on colitis-associated carcinogenesis by restoration of the gut microbiota in a mouse model. Nutrients 13:1143. doi: 10.3390/nu13041143
Xu, X., Peng, Q., Zhang, Y., Tian, D., Zhang, P., Huang, Y., et al. (2020). A novel exopolysaccharide produced by Lactobacillus coryniformis NA-3 exhibits antioxidant and biofilm-inhibiting properties in vitro. Food. Nutr. Res. 64:64. doi: 10.29219/fnr.v64.3744
Xun, Y., Yan, F., Zhu, H., Feng, L., Zhang, D., Xue, Y., et al. (2022). Oral administration of Lactobacillus paracasei N1115 on neonatal mice prevents the intestinal inflammation in adulthood. Lett. Appl. Microbiol. 75, 330–337. doi: 10.1111/lam.13729
Yan, F., Cao, H., Cover, T. L., Whitehead, R., Washington, M. K., and Polk, D. B. (2007). Soluble proteins produced by probiotic bacteria regulate intestinal epithelial cell survival and growth. Gastroenterology 132, 562–575. doi: 10.1053/j.gastro.2006.11.022
Yan, S., Yang, B., Ross, R. P., Stanton, C., Zhang, H., Zhao, J., et al. (2020). Bifidobacterium longum subsp. longum YS108R fermented milk alleviates DSS induced colitis via anti-inflammation, mucosal barrier maintenance and gut microbiota modulation. J. Funct. Foods 73:104153. doi: 10.1016/j.jff.2020.104153
Yan, S., Yang, B., Zhao, J., Zhao, J., Stanton, C., Ross, R. P., et al. (2019). A ropy exopolysaccharide producing strain Bifidobacterium longum subsp. longum YS108R alleviates DSS-induced colitis by maintenance of the mucosal barrier and gut microbiota modulation. Food Funct. 10, 1595–1608. doi: 10.1039/C9FO00014C
Yang, C., du, Y., Ren, D., Yang, X., and Zhao, Y. (2021). Gut microbiota-dependent catabolites of tryptophan play a predominant role in the protective effects of turmeric polysaccharides against DSS-induced ulcerative colitis. Food Funct. 12, 9793–9807. doi: 10.1039/D1FO01468D
Yang, J., Meng, L., and Yang, H. (2022). Therapeutic effects of Bifidobacterium breve YH68 in combination with Vancomycin and metronidazole in a primary clostridioides difficile-infected mouse model. Microbiol Spectr 10:e0067222. doi: 10.1128/spectrum.00672-22
Yao, S., Zhao, Z., Wang, W., and Liu, X. (2021). Bifidobacterium longum: protection against inflammatory bowel disease. J Immunol Res 2021:8030297. doi: 10.1155/2021/8030297
Ye, Y., Manne, S., Treem, W. R., and Bennett, D. (2020). Prevalence of inflammatory bowel disease in pediatric and adult populations: recent estimates from large National Databases in the United States, 2007-2016. Inflamm. Bowel Dis. 26, 619–625. doi: 10.1093/ibd/izz182
Yeo, S., Park, H., Seo, E., Kim, J., Kim, B. K., Choi, I. S., et al. (2020). Anti-inflammatory and gut microbiota modulatory effect of Lactobacillus rhamnosus strain LDTM 7511 in a dextran sulfate sodium-induced colitis murine model. Microorganisms 8, 6102–6116. doi: 10.3390/microorganisms8060845
Yılmaz, İ., Dolar, M. E., and Özpınar, H. (2019). Effect of administering kefir on the changes in fecal microbiota and symptoms of inflammatory bowel disease: a randomized controlled trial. Turk J Gastroenterol 30, 242–253. doi: 10.5152/tjg.2018.18227
Yin, X., Heeney, D., Srisengfa, Y., Golomb, B., Griffey, S., and Marco, M. (2018). Bacteriocin biosynthesis contributes to the anti-inflammatory capacities of probiotic Lactobacillus plantarum. Benef Microbes 9, 333–344. doi: 10.3920/BM2017.0096
Yoo, J. W., Shin, Y. J., Ma, X., Son, Y. H., Jang, H. M., Lee, C. K., et al. (2022). The alleviation of gut microbiota-induced depression and colitis in mice by anti-inflammatory probiotics NK151, NK173, and NK175. Nutrients 14:2080. doi: 10.3390/nu14102080
Yu, P., Ke, C., Guo, J., Zhang, X., and Li, B. (2020). Lactobacillus plantarum L15 alleviates colitis by inhibiting LPS-mediated NF-κB activation and ameliorates DSS-induced gut microbiota Dysbiosis. Front. Immunol. 11:575173. doi: 10.3389/fimmu.2020.575173
Yu, R., Zuo, F., Ma, H., and Chen, S. (2019). Exopolysaccharide-producing Bifidobacterium adolescentis strains with similar adhesion property induce differential regulation of inflammatory immune response in Treg/Th17 Axis of DSS-colitis mice. Nutrients 11:782. doi: 10.3390/nu11040782
Yun, S. W., Kim, J. K., Lee, K. E., Oh, Y. J., Choi, H. J., Han, M. J., et al. (2020). A probiotic Lactobacillus gasseri alleviates Escherichia coli-induced cognitive impairment and depression in mice by regulating IL-1β expression and gut microbiota. Nutrients 12:3441. doi: 10.3390/nu12113441
Yunyun Jiang, Z. Y. (2018). A functional and genetic overview of exopolysaccharides produced by Lactobacillus plantarum. Functional Foods 47, 229–240. doi: 10.1016/j.jff.2018.05.060
Zhai, Q., Zhang, Q., Tian, F., Zhao, J., Zhang, H., and Chen, W. (2019). The synergistic effect of Lactobacillus plantarum CCFM242 and zinc on ulcerative colitis through modulating intestinal homeostasis. Food Funct. 10, 6147–6156. doi: 10.1039/C9FO00926D
Zhang, Y., Bhosle, A., Bae, S., McIver, L. J., Pishchany, G., Accorsi, E. K., et al. (2022). Discovery of bioactive microbial gene products in inflammatory bowel disease. Nature 606, 754–760. doi: 10.1038/s41586-022-04648-7
Zhang, Y., Mu, T., Yang, Y., Zhang, J., Ren, F., and Wu, Z. (2021). Lactobacillus johnsonii attenuates Citrobacter rodentium-induced colitis by regulating inflammatory responses and endoplasmic reticulum stress in mice. J. Nutr. 151, 3391–3399. doi: 10.1093/jn/nxab250
Zhang, W. Q., Quan, K. Y., Feng, C. J., Zhang, T., He, Q. W., Kwok, L. Y., et al. (2022). The Lactobacillus gasseri G098 strain mitigates symptoms of DSS-induced inflammatory bowel disease in mice. Nutrients 14:3745. doi: 10.3390/nu14183745
Zhang, X., Tong, Y., Lyu, X., Wang, J., Wang, Y., and Yang, R. (2021). Prevention and alleviation of dextran sulfate sodium salt-induced inflammatory bowel disease in mice with Bacillus subtilis-fermented Milk via inhibition of the inflammatory responses and regulation of the intestinal Flora. Front. Microbiol. 11:622354. doi: 10.3389/fmicb.2020.622354
Zhang, J., Yi, R., Qian, Y., Sun, P., Zhao, X., and Yang, Z. (2018). Lactobacillus plantarum CQPC06 activity prevents dextran sulfate sodium-induced colitis by regulating the IL-8 pathway. J. Food Sci. 83, 2653–2661. doi: 10.1111/1750-3841.14346
Zhang, Y., Zhao, X., Zhu, Y., Ma, J., Ma, H., and Zhang, H. (2018). Probiotic mixture protects dextran sulfate sodium-induced colitis by altering tight junction protein expressions and increasing Tregs. Mediat. Inflamm. 2018, 1–11. doi: 10.1155/2018/9416391
Zhao, J., Wang, Y., Wang, J., Lv, M., Zhou, C., Jia, L., et al. (2022). Lactobacillus kefiranofaciens ZW18 from kefir enhances the anti-tumor effect of anti-programmed cell death 1 (PD-1) immunotherapy by modulating the gut microbiota. Food Funct. 13, 10023–10033. doi: 10.1039/D2FO01747D
Zheng, J., Ahmad, A. A., Yang, Y., Liang, Z., Shen, W., Feng, M., et al. (2022). Lactobacillus rhamnosus CY12 enhances intestinal barrier function by regulating tight junction protein expression, oxidative stress, and inflammation response in lipopolysaccharide-induced Caco-2 cells. Int. J. Mol. Sci. 23:11162. doi: 10.3390/ijms231911162
Zhou, J., Li, M., Chen, Q., Li, X., Chen, L., Dong, Z., et al. (2022). Programmable probiotics modulate inflammation and gut microbiota for inflammatory bowel disease treatment after effective oral delivery. Nat. Commun. 13:3432. doi: 10.1038/s41467-022-31171-0
Zhou, X., Qi, W., Hong, T., Xiong, T., Gong, D., Xie, M., et al. (2018). Exopolysaccharides from Lactobacillus plantarum NCU116 regulate intestinal barrier function via STAT3 signaling pathway. J. Agric. Food Chem. 66, 9719–9727. doi: 10.1021/acs.jafc.8b03340
Zhou, L. Y., Xie, Y., and Li, Y. (2022). Bifidobacterium infantis regulates the programmed cell death 1 pathway and immune response in mice with inflammatory bowel disease. World J. Gastroenterol. 28, 3164–3176. doi: 10.3748/wjg.v28.i26.3164
Zhou, M., Xu, W., Wang, J., Yan, J., Shi, Y., Zhang, C., et al. (2018). Boosting mTOR-dependent autophagy via upstream TLR4-MyD88-MAPK signalling and downstream NF-κB pathway quenches intestinal inflammation and oxidative stress injury. EBioMedicine 35, 345–360. doi: 10.1016/j.ebiom.2018.08.035
Zhou, X., Zhang, D., Qi, W., Hong, T., Xiong, T., Wu, T., et al. (2021). Exopolysaccharides from Lactobacillus plantarum NCU116 facilitate intestinal homeostasis by modulating intestinal epithelial regeneration and microbiota. J. Agric. Food Chem. 69, 7863–7873. doi: 10.1021/acs.jafc.1c01898
Keywords: ulcerative colitis, Bifidobacterium, Lactobacillus, probiotic, treatment, children
Citation: Huang C, Hao W, Wang X, Zhou R and Lin Q (2023) Probiotics for the treatment of ulcerative colitis: a review of experimental research from 2018 to 2022. Front. Microbiol. 14:1211271. doi: 10.3389/fmicb.2023.1211271
Edited by:
Renqiang Yu, Wuxi Maternity and Child Health Care Hospital, ChinaReviewed by:
Zhipeng Gu, South China University of Technology, ChinaYulin Ma, China Agricultural University, China
Kristie Keeney, Lumen Bioscience, Inc., United States
Copyright © 2023 Huang, Hao, Wang, Zhou and Lin. This is an open-access article distributed under the terms of the Creative Commons Attribution License (CC BY). The use, distribution or reproduction in other forums is permitted, provided the original author(s) and the copyright owner(s) are credited and that the original publication in this journal is cited, in accordance with accepted academic practice. No use, distribution or reproduction is permitted which does not comply with these terms.
*Correspondence: Qiong Lin, linqiong76@163.com
†These authors have contributed equally to this work