- 1Department of Veterinary Population Medicine, College of Veterinary Medicine, University of Minnesota–Twin Cities, St. Paul, MN, United States
- 2Department of Biology, College of Arts and Sciences, University of Nebraska Omaha, Omaha, NE, United States
- 3Departamento de Patología Animal, Facultad de Veterinaria, Universidad de Zaragoza, Zaragoza, Spain
- 4Koret School of Veterinary Medicine, The Robert H. Smith Faculty of Agriculture, Food and Environment, The Hebrew University of Jerusalem, Jerusalem, Israel
- 5Health Sciences Library, University of Minnesota–Twin Cities, Minneapolis, MN, United States
- 6Department of Veterinary and Biomedical Sciences, College of Veterinary Medicine, University of Minnesota–Twin Cities, St. Paul, MN, United States
- 7School of Public Health, University of Minnesota–Twin Cities, Minneapolis, MN, United States
- 8VISAVET Health Surveillance Centre, Universidad Complutense Madrid, Madrid, Spain
- 9Department of Animal Health, Facultad de Veterinaria, Universidad Complutense Madrid, Madrid, Spain
Third generation cephalosporins and carbapenems are considered critically important antimicrobials in human medicine. Food animals such as swine can act as reservoirs of antimicrobial resistance (AMR) genes/bacteria resistant to these antimicrobial classes, and potential dissemination of AMR genes or resistant bacteria from pigs to humans is an ongoing public health threat. The objectives of this systematic review and meta-analysis were to: (1) estimate global proportion and animal-level prevalence of swine E. coli phenotypically resistant to third generation cephalosporins (3GCs) and carbapenems at a country level; and (2) measure abundances and global distribution of the genetic mechanisms that confer resistance to these antimicrobial classes in these E. coli isolates. Articles from four databases (CAB Abstracts, PubMed/MEDLINE, PubAg, and Web of Science) were screened to extract relevant data. Overall, proportion of E. coli resistant to 3GCs was lower in Australia, Europe, and North America compared to Asian countries. Globally, <5% of all E. coli were carbapenem-resistant. Fecal carriage rates (animal-level prevalence) were consistently manifold higher as compared to pooled proportion of resistance in E. coli isolates. blaCTX–M were the most common 3GC resistance genes globally, with the exception of North America where blaCMY were the predominant 3GC resistance genes. There was not a single dominant blaCTX–M gene subtype globally and several blaCTX–M subtypes were dominant depending on the continent. A wide variety of carbapenem-resistance genes (blaNDM–, VIM–, IMP–, OXA–48, and KPC–) were identified to be circulating in pig populations globally, albeit at very-low frequencies. However, great statistical heterogeneity and a critical lack of metadata hinders the true estimation of prevalence of phenotypic and genotypic resistance to these antimicrobials. Comparatively frequent occurrence of 3GC resistance and emergence of carbapenem resistance in certain countries underline the urgent need for improved AMR surveillance in swine production systems in these countries.
Introduction
Third generation cephalosporins (3GCs) such as ceftriaxone and cefotaxime are used to treat Gram-negative infections in humans (Iwamoto et al., 2017) and are considered critically important antimicrobials in human medicine according to the World Health Organization., 2019. Consequently, resistance to these antimicrobials has received increased attention in molecular epidemiological studies in bacteria retrieved from humans (Manges et al., 2019). Third generation cephalosporins are also used in food animal production including swine for treating respiratory disease complex (Hayer et al., 2020b; Sun et al., 2020). Although some of these antimicrobials such as ceftiofur are only used in food animal medicine, their use can exert selective pressure favoring resistance to cephalosporins used in human medicine (Cantón and Ruiz-Garbajosa, 2011). Hence, there is a need to monitor the prevalence of resistance to higher generation cephalosporins in swine production in order to monitor the role of swine production systems as a reservoir of potentially zoonotic bacteria resistant to these antimicrobials. Resistance to 3GCs is mediated by extended spectrum beta-lactamases (ESBLs, commonly coded by blaSHV– and CTX–M– family of genes) and ampC (commonly coded by blaCMY family of genes) (Pfeifer et al., 2010). These genes can be plasmid-borne and plasmids aid in the widespread dissemination of these genes horizontally or across different bacterial species as well as mammalian hosts (Hayer et al., 2020a).
The global spread of pathogens resistant to third and fourth generation cephalosporins has led to an increased consumption of last resort antimicrobials in human medicine such as carbapenems (Woodford et al., 2014). Carbapenemases are β-lactamase enzymes capable of hydrolyzing not only carbapenems but also penicillins and 3GCs, and genes encoding these enzymes (like blaVIM–, IMP–, OXA–48, NDM–, and GES–) can also be transmitted between different bacterial species via plasmids (Giske et al., 2009; Nordmann et al., 2012). Although carbapenems are not licensed for use in food animal production in any country (Woodford et al., 2014), the use of licensed higher generation cephalosporins such as ceftiofur in animals can lead to co-selection of carbapenem resistance (Mollenkopf et al., 2017).
In order to make a reliable assessment of the possible role of antimicrobial usage in animals on the emergence of antimicrobial resistance (AMR) and its impact on human health, knowledge on the occurrence of AMR in different animal production systems is needed. The aim of this systematic review was (1) to evaluate available information on the frequency and types of phenotypic resistance to 3GCs and carbapenems in swine Escherichia coli, and (2) to assess the spread of select bla genes conferring these resistance phenotypes in this bacterial species. E. coli was selected as an indicator bacterial species because of its widespread prevalence across multiple animal species, ease of isolation and propensity to develop resistance against antimicrobials reviewed in this study (Tadesse et al., 2012) and has been identified as the most relevant resistant bacterial pathogen in swine populations (Nielsen et al., 2021).
Methods
Literature Search
Four databases, PubAg, Web of Science, PubMed, and CAB Abstracts, were screened using different search strings (Supplementary File 1) on 13 April 2017 and were updated on 18 October 2021. Search strings were designed to identify publications on cephalosporin, colistin, quinolone, and carbapenem resistance in swine. Data on fluoroquinolone and colistin resistance was extracted to conduct a separate systematic review (Hayer et al., 2022).
Records were retrieved, and duplicate articles were removed using Zotero version 5.0.96.3. In addition to the database searches, reports containing information on AMR in E. coli from swine were retrieved from the websites of national and international agencies coordinating surveillance programs for AMR (EFSA, Europe; RESAPATH, France; DANMAP, Denmark; MARAN, Netherlands; NORMVET, Norway; SWEDRES, Sweden; FINRES, Finland; NARMS, United States; and CIPARS, Canada).
Retrieved records were first screened for inclusion if there was a mention of AMR, E. coli or Enterobacteriaceae, and livestock in title and/or abstract. The selected full-length articles were then downloaded and data was collected from articles if there was data on occurrence of phenotypic AMR against 3GCs or carbapenems in E. coli collected from pigs. Articles were excluded if:
• The data was based on in vivo or in vitro trials that carried out experiments other than measuring phenotypic or genotypic AMR in E. coli circulating in swine populations. For example, if an animal trial involved feeding antimicrobials to pigs and then studying occurrence of AMR, then that study was excluded. Similarly, studies that included experimental induction of AMR in E. coli strains in vitro were excluded.
• Articles were written in a language other than English
• Articles were providing a review, a commentary or data on just antimicrobial usage
• Data was not provided for E. coli specifically
• Bacteria were not isolated from pigs
• Data was based on a single farm/outbreak, with the exception of articles that presented research based on multiple farms but were able to find an isolate of interest from a single farm
• Article described a single isolate that carried a gene of our interest but did not provide the number of isolates tested (lack of denominator)
• A metagenomic approach was taken
• Total number of isolates tested was not provided.
Data Extraction
A predesigned datasheet was developed using Microsoft Excel 2013 for collecting data such as publication year, country and year of bacterial isolation, health status of pigs, phenotypic resistance tested, microbiological methods employed for AMR determination, criteria for classifying bacteria as resistant/non-resistant, total number of isolates tested and number of isolates resistant against each antimicrobial and whether information on sampling design, antimicrobial use and husbandry was provided. Extracted phenotypic and genotypic data are provided in Supplementary File 2.
Meta-Analyses of Literature Review
Arguably, the biggest issue in aggregating AMR data across studies was the use of different breakpoints to classify bacteria as “resistant” in these studies. We harmonized the AMR prevalence data in a multi-step manner. First, for those studies where MIC distributions were available, the number of resistant bacteria were re-calculated as if the current CLSI breakpoints (CLSI, 2021) were used for defining resistance. If MIC distributions were not available or disk diffusion method was used for estimating resistance, the number of resistant isolates in a particular study was multiplied by a harmonizing factor. These harmonization factors were calculated using the following formulae (Van Boeckel et al., 2019):
1. For dilution methods – number of isolates in the reference EUCAST distribution with MIC ≥ current CLSI breakpoint divided by number of isolates in reference EUCAST distribution with MIC ≥ applied breakpoint in the study from which data were extracted.
2. For diffusion methods – number of isolates in reference EUCAST distribution with zone of inhibition ≤ current CLSI breakpoint divided by number of isolates in reference EUCAST distribution with zone of inhibition ≤ applied breakpoint in the study from which data were extracted.
MIC or disc diffusion reference distributions were downloaded from EUCAST (2021). Breakpoints were determined for each article based on the performance methodology (e.g., CLSI-M100 series) document provided in the bibliography. If this information was not provided, it was assumed that authors used CLSI breakpoints for interpretation as these are the most commonly used guidelines to differentiate resistant bacteria globally (Van Boeckel et al., 2019).
Considering that isolates collected from healthy pigs were likely to have different phenotypic resistance profiles compared to isolates from diseased pigs, pooled prevalences of resistance were estimated separately for these health categories at a country level. Studies that reported AMR levels in mixed bacterial populations isolated from both healthy and diseased pigs were not included in the meta-analyses, but the results from these studies are available in Supplementary File 2. Studies that did not provide data on health status were assumed to have been conducted on healthy pigs because the authors compared their results to those from other healthy pig populations in the discussion section and these isolates were not based on diagnostic submissions or collected during outbreak investigations. Removing data from such articles did not impact the pooled prevalence estimates (data not shown).
Another issue with pooling resistance data was the detection of resistance to different 3GCs and carbapenems across studies. To address this issue, we selected three 3GC resistances as markers: ceftiofur, cefotaxime, and ceftriaxone resistance. At least one of these cephalosporin resistances was estimated in all studies except one, and for studies estimating resistance to more than one of these 3GCs, the correlation between the proportions of isolates resistant to these 3GCs was high (correlation coefficients > 0.9). For those studies providing data on more than one of these three cephalosporin resistances (e.g., both ceftiofur and cefotaxime resistance measured on same isolates), we used the highest number of isolates estimated to be resistant after harmonization for any of these 3GCs in a study for downstream analyses. For example, if the number of ceftiofur and cefotaxime resistant isolates after harmonization were 30 and 35, respectively in a study, then we used 35 as the number of isolates resistant to 3GCs for statistical analysis. In addition, when available, ceftazidime resistance was analyzed separately from the other 3GCs because the genetic basis of ceftazidime resistance and ceftiofur, cefotaxime, or ceftriaxone resistance are different (Hiki et al., 2017). Similarly, if a study provided data on multiple carbapenem resistances (e.g., doripenem and ertapenem resistance for same set of isolates in the same article), the highest number of isolates after harmonization for any of these carbapenems were used as a proxy for number of isolates resistant to carbapenem on a per article basis.
The pooled proportions of 3GC, ceftazidime and carbapenem resistances were calculated at a country-level by transforming the proportions of resistant isolates using the Freeman–Tukey double arcsine transformation method (Miller, 1978), followed by a random effects model using inverse method and back-transforming to get the final pooled proportion estimates (in percent) across studies using library meta version 5.1-0 (Schwarzer, 2021). Statistical heterogeneity (I2) was retrieved from the output of this model and pooled estimates at a country-level were considered to be heterogenous and homogenous if p-values were <0.05 and >0.05, respectively. Choropleth maps were created to graph the pooled proportions of 3GC and ceftazidime resistances at a global scale using QGis version 3.20.3.
Similarly, pooled fecal carriage rates of 3GC and carbapenem resistance were also estimated and mapped. Fecal carriage rate is defined as the number of fecal samples collected from individual animals that test positive for carrying E. coli with specific resistance phenotype after screening on selective media. Herd level prevalence was not estimated and are not presented in the results section but raw data to do so has been provided in Supplementary File 2. Finally, choropleth maps for estimates for “sampling index” were also generated. For this study, sampling index was defined as the number of unique isolates tested for phenotypic 3GC, ceftazidime or carbapenem resistance divided by the number of specific pig population at a country level. For ease of interpretation, this index was generated per 1,000 pigs. Country-level pig population data (as of 2018) was downloaded from FAO (Food and Agriculture Organisation, 2022).
Genomic Meta-Analyses
Data on genotypic prevalence of 3GC and carbapenem resistance was also extracted from the articles selected above and is available in Supplementary File 2. Pooled proportions of ESBL/ampC genes in E. coli isolated on selective media or having unique resistance phenotypes (i.e., carbapenem resistant, ESBL/ampC producers, and 3GC resistant) were estimated as described above.
In order to extract more data on the genomic basis of resistance to these antimicrobials, we downloaded information on genomes of 6,167 E. coli isolates of swine origin from Enterobase webserver (Zhou et al., 2020) (last accessed on 24 July 2021) and analyzed these genomes. The advantage of this data over the PCR data extracted from the literature search is that information regarding several genomic characteristics can be obtained at the isolate level. However, the disadvantage of this dataset is a lack of critical metadata such as microbiological methods employed, health status of the animal, etc.
Short read sequences of these isolates were downloaded from NCBI database based on their accession numbers from Enterobase (Zhou et al., 2020). Raw reads were checked for quality control using FastQC version 0.11.9 (Andrews, 2021) and subsequently trimmed using Trimmomatic version 0.39 (settings: sliding window mode; number of bases to average across = 4; average quality required = 20) (Bolger et al., 2014). Trimmed reads were then assembled using Shovill version 1.1.0 (default settings) (Seemann, 2021b). When short reads were not available for download, assembled genomes were directly downloaded from the Enterobase webserver. Genomes were characterized for virulence genes (Virulence Finder) (Malberg Tetzschner et al., 2020), resistance genes (ResFinder) (Bortolaia et al., 2020), chromosomal mutations that can confer resistance (PointFinder) (Zankari et al., 2017) and plasmid replicons (Plasmidfinder) (Carattoli et al., 2014) using Abricate version 1.0.0 (Seemann, 2021a). All databases were last updated on 24 July 2021. Isolates carrying ESBL/ampC and carbapenemase genes were identified and described in the sections below. However, the number of the isolates carrying carbapenemase resistance genes were too few to do the analyses described in the following paragraphs.
Relative abundance of ESBL/ampC genes were estimated as the ratio of isolates carrying a particular bla gene family (e.g., blaCMY) divided by total number of isolates carrying blaCMY, blaCTX–M and/or blaSHV. Furthermore, relative abundance of subclasses of blaCTX–M genes were estimated as the ratio of isolates carrying a particular blaCTX–M subclass gene family (e.g., blaCTX–M–15) divided by total number of isolates carrying blaCTX–M genes. Relative abundances of ESBL/ampC genes (blaCMY, blaCTX–M, and blaSHV) and blaCTX–M subclasses at a global and continent level were plotted using library ggplot2 version 3.3.5 (Wickham et al., 2021).
Random Forest models were built to characterize genetic features (virulence factors, plasmid replicons, chromosomal mutations, acquired resistance genes, and plasmid replicon types) that could predict the presence of blaCMY and blaCTX–M genes. Two models were built separately for blaCMY and blaCTX–M genes (dependent variables). Models were not built for blaSHV isolates as they were too few in numbers to make reasonable models that can be generalized at a local or global scale. Only one of the perfectly correlated genetic features (r > 0.99) was retained for further analysis using library caret version 6.0-90 (Kuhn et al., 2021). The dataset was divided into training (65% of the dataset) and testing (35% of the dataset) data based on stratified random sampling in order to retain the same proportion of results for the dependent variables in these split datasets (presence of blaCMY or blaCTX–M depending on the model) (library caTools version 1.18.2) (Tuszynski, 2021). These models were first built for imbalanced training datasets using quantile-classifier algorithm (O’Brien and Ishwaran, 2019) in library RandomForestSRC (version 2.14.0) with the following settings: mtry = 25, number of trees = 12,000, split rule = AUC, block size = 100. Model performances were evaluated using geometric means. Training models were used to identify the top variables (i.e., variables of importance) that can predict blaCMY or blaCTX–M genes in testing data using default settings (library RandomForestSRC version 2.14.0) (Ishwaran and Kogalur, 2021). The top 10 variables of importance were extracted and plotted using library ggplot2 version 3.3.5 (Wickham et al., 2021). Unadjusted odds ratios were also estimated for the top 10 variables of importance to estimate an effect size and directionality and for ease of interpretation.
Results
Description of Articles Included
The stepwise process followed for article selection is presented in Figure 1. Ultimately, data from 394 articles that fulfilled the inclusion criteria was extracted and evaluated in this review. Description of these articles is presented in Table 1. The majority of the articles contained data from European (196 articles; 49.7%) or Asian (125 articles; 31.7%) countries. The studies included were published from 1998 to 2021, although there was a clear increase in the number of articles published after 2010 (70.1% of all articles). EUCAST epidemiological cut-offs (n = 83) and CLSI breakpoints (n = 174) were used to classify isolates as susceptible/resistant in 222 articles. The majority of the articles utilizing EUCAST epidemiological cut-offs were from European countries, whereas CLSI breakpoints were predominantly used in studies from other countries. Overall, data on resistance against 3GCs or carbapenem in E. coli of swine origin from 61 countries were available.
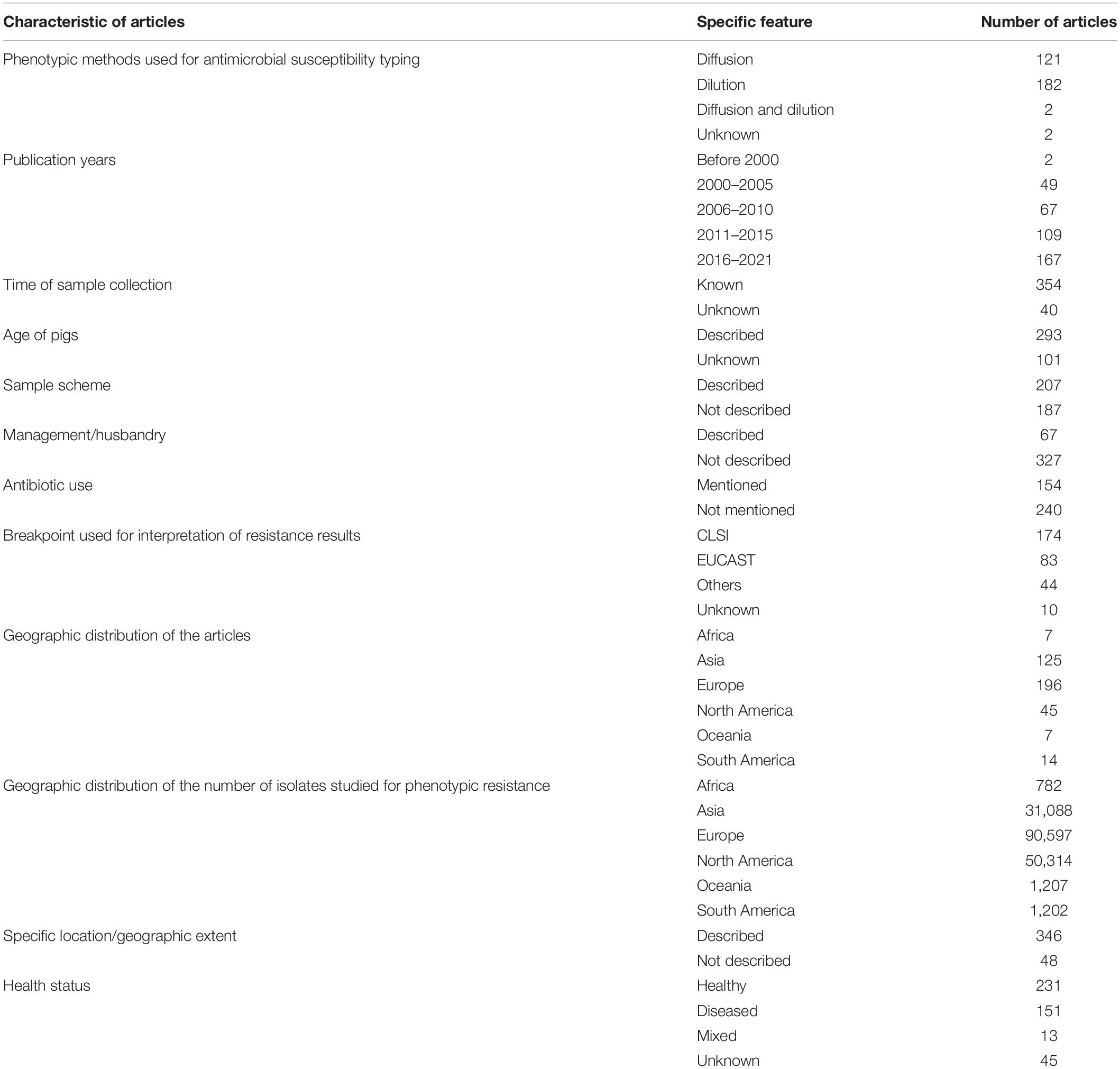
Table 1. General characteristics of the data reported in the articles selected reporting antimicrobial resistance data for cephalosporins and carbapenems in swine E. coli isolates (n = 394 articles).
Information on health characteristics of the pigs, time of sample collection and specific geographic location was available in 88.8, 89.8, and 87.8% of the articles, respectively. However, information regarding the age of pigs, sampling scheme employed for isolate collection, husbandry characteristics and previous antibiotic use was only available in 74.4, 52.5, 17.0, and 39.1% of the articles, respectively. These figures on reporting characteristics further decrease if surveillance reports are not considered. For example, if we only include data published in journal articles, then only 19.4% of the studies reported previous antibiotic use. Although we did not analyze this formally, there were very few studies that provided data regarding the clinical history, tissues collected and strain information of the E. coli collected from diseased pigs.
There was a clear disparity between number of isolates characterized phenotypically for 3GC, ceftazidime or carbapenem resistance across continents (Table 1). Overall, there were 175,190 isolates evaluated for phenotypic resistance globally, of which 51.7 and 28.7% of the isolates were collected from Europe and North America, respectively. After factoring in the pig populations by calculating sampling indices, this disparity was even more evident (Figure 2 and Supplementary File 2). Out of 15 countries with the highest sampling indices, 13 were European and rest of the countries were Canada and Grenada. On the other hand, 13 out of 15 countries with lowest sampling indices were Asian (6), African (4), or South American (3) and the rest of the countries were Cuba and Serbia. The exact number of isolates per study are provide in Supplementary File 2.
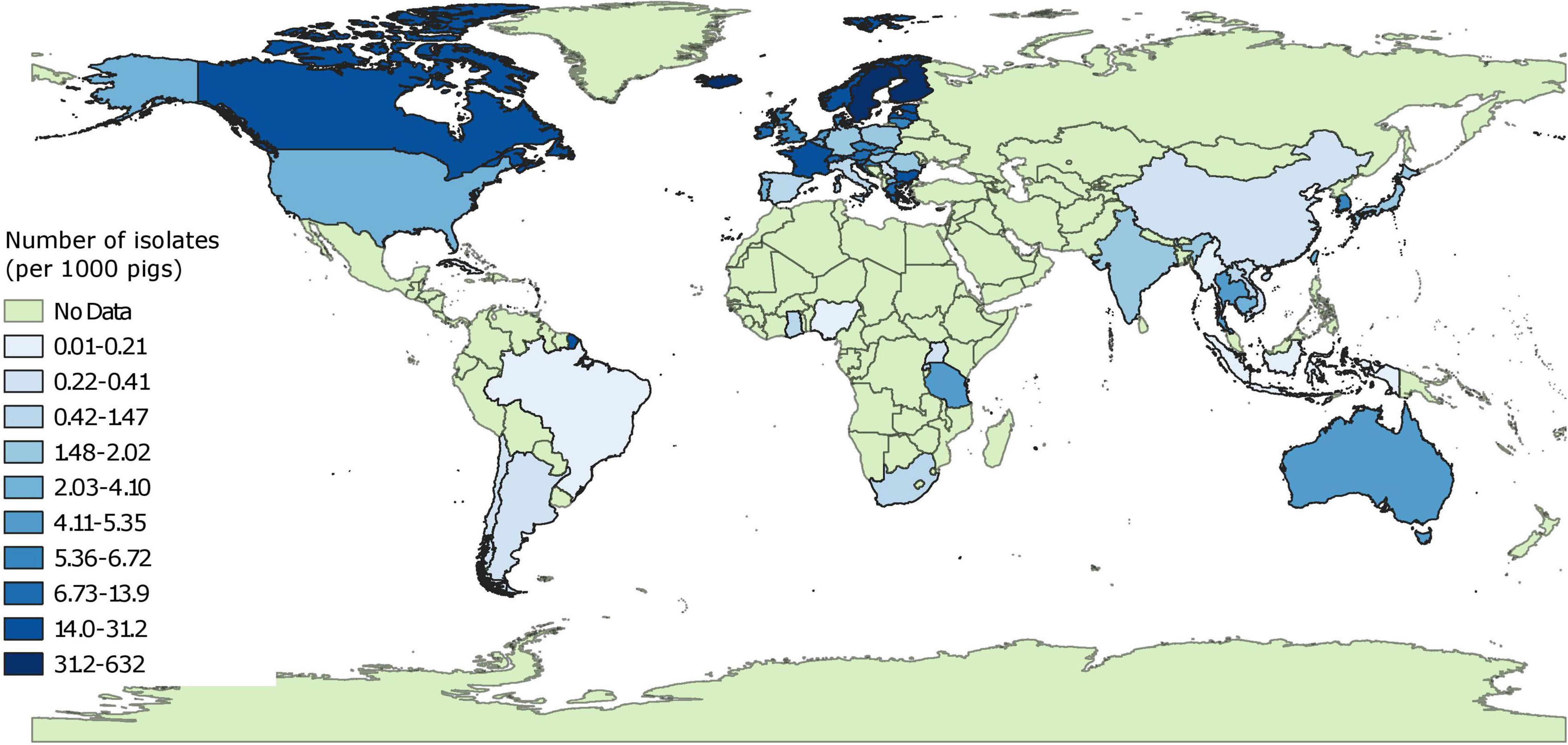
Figure 2. Global distribution of sampling indices (number of isolates characterized for phenotypic resistance per 1,000 pigs). “No data” implies that there were no isolates of interest reported and does not mean that there were no pigs in particular countries.
Pooled Proportion Estimates of Third Generation Cephalosporin Resistance in Isolates From Healthy Pigs
Pooled proportion estimates of 3GC resistance in E. coli from healthy pigs at the country level are mapped in Figure 3 and provided in Supplementary File 3. Briefly, 3GC resistance was very low (0–1%) and low (1–2%) in isolates collected from 24 and 8 European countries, respectively, with isolates from Portugal having highest proportion of this resistance (2%). These estimates were statistically homogenous for data from 26 out of the 32 European countries. Similarly, the pooled proportion of 3GC resistance ranged between very low and low in countries from North America (Grenada, 0%; Canada, 1%; and United States, 3%) and Oceania (Australia, 2%). In South America, the pooled proportion estimates were also very low to low (Chile, 0%; Argentina, 0%; and Brazil, 8%). However, the estimates from North American, South American, and Oceanic countries were either based on one data-point or were statistically heterogenous, with the exception of pooled proportion of 3GC resistance in Argentina, which was statistically homogenous.
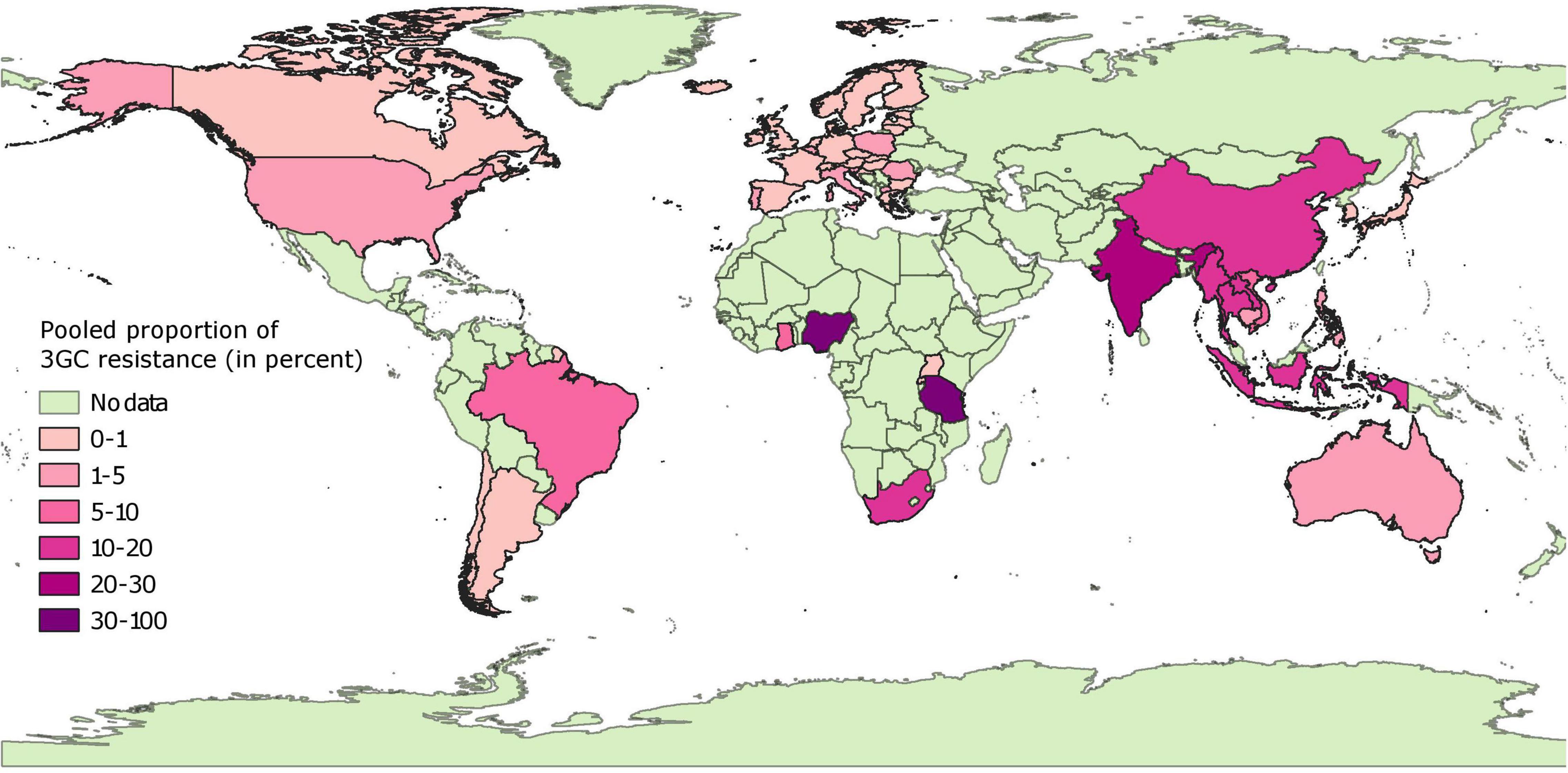
Figure 3. Global distribution of pooled proportion estimates of third generation cephalosporin resistance in E. coli isolates collected from healthy pigs. “No data” implies that there were no isolates of interest reported and does not mean that there were no pigs in particular countries.
In contrast to the results above, the pooled proportion estimates from African and Asian countries were highly variable. Very low (<1%) pooled proportion estimates of 3GC resistance were estimated for Japan, South Korea, Rwanda, and Uganda. Low percentage of isolates were resistant to 3GCs in four countries (Cambodia, 2%; Philippines, 5%; Vietnam, 8%; and Ghana, 7%). In contrast, for other countries estimates of pooled proportion of 3GC resistance ranged between moderate (Laos, 12%; Indonesia, 12%; China, 16%; Myanmar, 18%; Thailand, 20%; and South Africa, 19%), high (India, 24% and Tanzania, 37%), and extremely high (Nigeria, 91%). These estimates were either statistically heterogeneous or based on a single data-point, except for Japan, Laos, and Uganda.
Pooled Proportion Estimates of Third Generation Cephalosporin Resistance in Isolates From Diseased Pigs
Data on isolates from diseased pigs was available from 30 countries. Pooled proportion estimates of 3GC resistances at the country level are mapped in Figure 4 and provided in Supplementary File 3. In general, the pooled proportion estimates of 3GC resistance were higher in isolates from diseased pigs compared to healthy pigs. In Europe, North America, and Australia, pooled proportion in these subsets of isolates ranged between very low (<1% in 8 countries) and low (1–6% in 10 countries), with higher (moderate) pooled proportion estimated from Spain (14%), Austria (14%), and United States (19%). In Asian and South American countries, pooled proportion ranged from low (between 1 and 4% in South Korea, Brazil, Vietnam, and Japan) to high (between 24 and 47% in Argentina, India, China, Thailand, and Taiwan). No estimates from diseased pigs in African countries were available. The estimates were statistically homogenous for 10 of the 30 countries (8 European countries, Australia, and Taiwan).
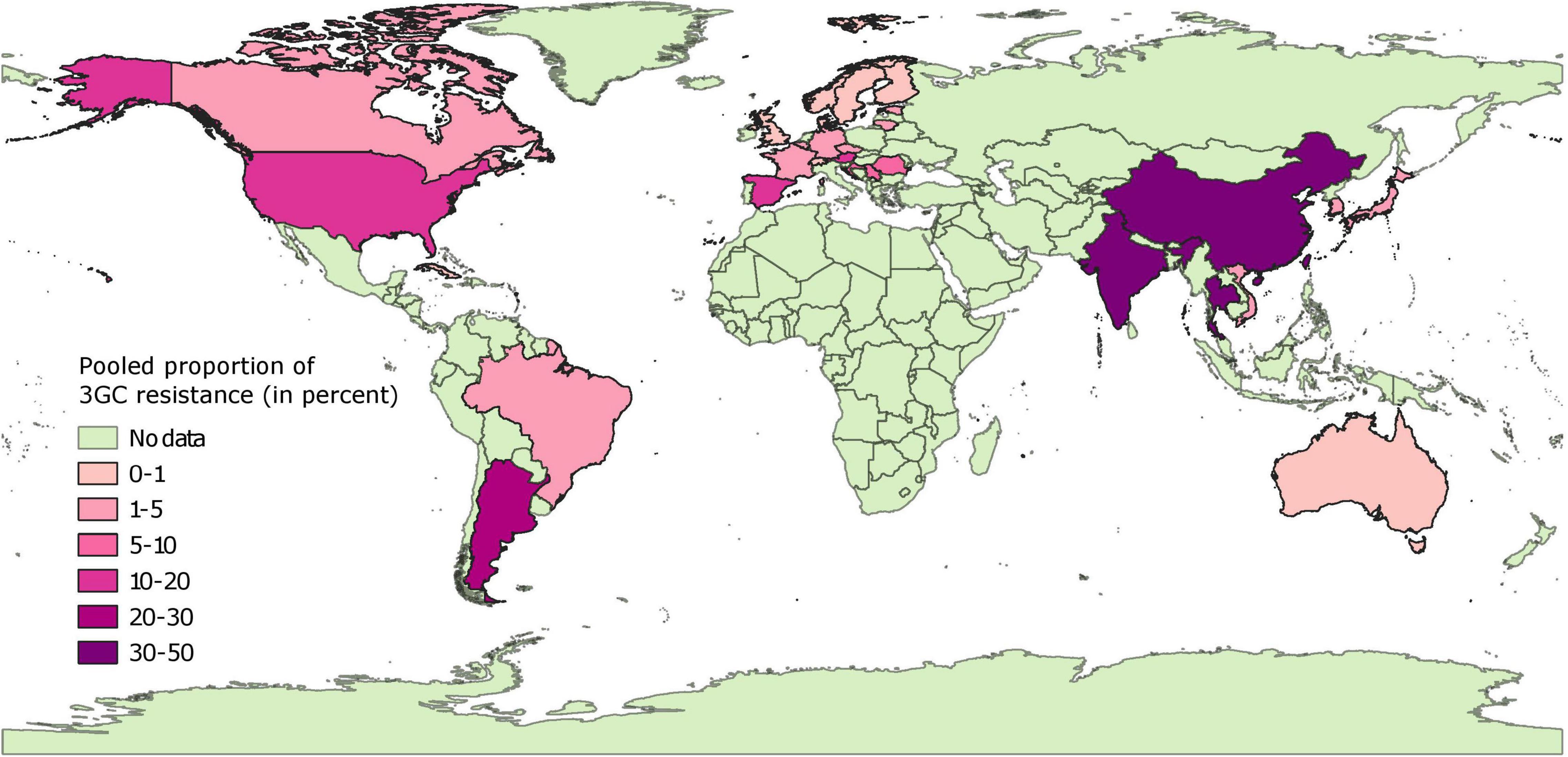
Figure 4. Global distribution of pooled proportion estimates of third generation cephalosporin resistance in E. coli isolates collected from diseased pigs. “No data” implies that there were no isolates of interest reported and does not mean that there were no pigs in particular countries.
Pooled Proportion Estimates of Ceftazidime Resistance
Detailed pooled proportion estimates of ceftazidime resistance are presented in Supplementary File 3. Estimates of resistance to ceftazidime largely mirrored the estimates for frequency of 3GC resistances. Based on the data from 46 countries, the correlation between proportions of ceftazidime and 3GC resistance in isolates from healthy pigs were strong (r = 0.98, Pearson correlation). However, the pooled proportion estimates of 3GC resistance were 6.54 (95% CI: 2.57–10.5) times higher as compared to ceftazidime resistance in isolates collected from healthy animals. There was a moderate correlation between proportion estimates of 3GC and ceftazidime resistance in isolates from diseased pigs (r = 0.58, 10 countries, Pearson correlation), and proportion estimates of 3GC resistance were 1.93 times higher (95% CI: 0.07–3.79) than those of ceftazidime.
Pooled Proportion Estimates of Carbapenem Resistance
Data on carbapenem resistance in isolates from healthy and diseased pigs was available from 47 countries (32 European, 7 Asian, 5 African, and 3 North American) and 9 countries (2 European, 6 Asian, 1 Australia), respectively. Proportions of carbapenem resistance in isolates from healthy pigs were estimated to be zero in 38 of these countries (Table 2). Pooled proportion of carbapenem resistance was 0% in isolates from healthy pigs from the European countries, except Portugal (0.1%). In Asia, these proportions ranged from 0% in Cambodia, Japan, and Laos, to 0.8–3.6% in China, Philippines, Thailand, and Vietnam. Estimates were highly variable in African countries ranging from 0% (Uganda and Rwanda), low (2% South Africa and 5 Tanzania) to 100% [Ghana, on a study performed on 43 isolates (Larbi et al., 2021)].
Pooled proportion estimates of carbapenem resistance were also 0% based on data from isolates from diseased pigs in Australia, Austria, Japan, Switzerland, and Taiwan (Table 2). In addition, a multi-country study in Europe estimated this proportion at 0.1% in isolates from diseased pigs in six countries (Germany, Belgium, United Kingdom, Denmark, Italy, and Netherlands) (Pulss et al., 2017). In this study, resistant isolates were isolated only from Germany and Italy. In China, India, and South Korea, this prevalence ranged between 0.6 and 4.62%, whereas in Thailand this prevalence was 59.1% based on a single study (Pokhrel et al., 2018; Table 2).
Pooled Fecal Carriage Rates (Animal-Level Prevalence) of Third Generation Cephalosporin Resistance
Data on pooled fecal carriage rates of 3GC resistance was available from 26 countries (Figure 5 and Supplementary File 3). Fecal carriage rates were lower in European countries as compared to most of the non-European countries. In general, fecal carriage rates were 433 times higher than the pooled proportion estimates. In Europe, pooled fecal carriage rates ranged were <10% in Finland, France, and Norway, between 10 and 20% in Switzerland, Denmark, Belgium, Poland, and Netherlands and between 20 and 45% in Latvia, United Kingdom, Portugal, Austria, Spain, and Germany. In Asia, pooled fecal carriage rates ranged between 5 and 22% in Japan, South Korea, and Taiwan, whereas this rate ranged between 55 and 80% in samples from Vietnam, China, Lebanon, and India. These rates ranged between 44 and 80% in other countries (Cuba, Rwanda, Argentina, Uruguay, and United States), with the exception of Brazil (3%). It should be noted that data from 17 of these 26 countries was based on a single study, and pooled estimates were statistically heterogenous for all but 3 countries (Finland, Japan, and Poland). Still, there was a moderate correlation (r = 0.53, Pearson correlation) between pooled proportion estimates and fecal carriage rates of 3GC resistance. Fecal carriage rate of carbapenem resistance was found to be zero in 6,791 fecal samples collected from Norway, Sweden, Denmark, Finland, and Switzerland. Pooled fecal carriage rate of carbapenem resistance was estimated to be 1.36% from China and India.
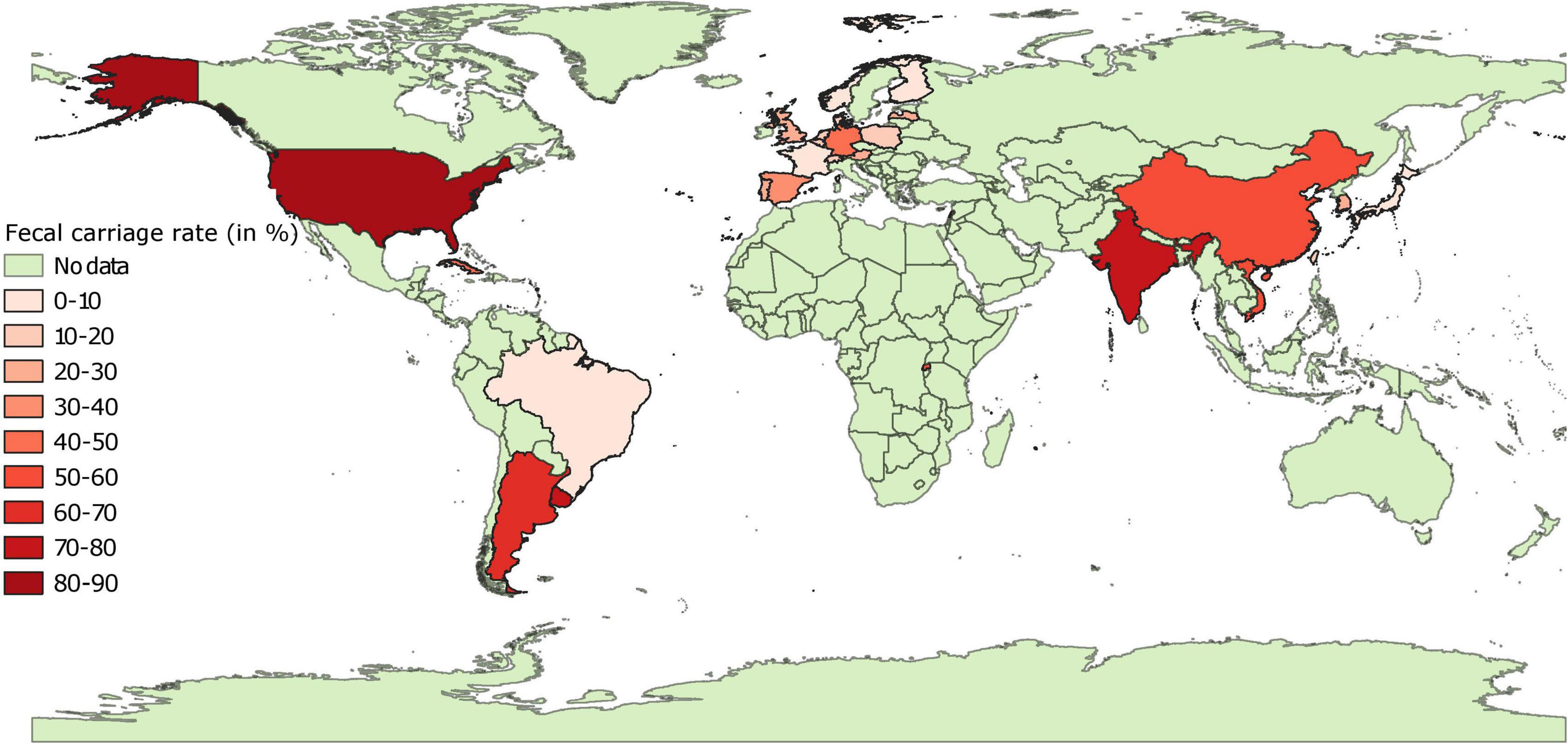
Figure 5. Global distribution of fecal carriage rates (animal-level prevalence) of third generation cephalosporin resistant E. coli isolates in pigs. “No data” implies that there were no isolates of interest reported and does not mean that there were no pigs in particular countries.
The biggest confounding factor in estimation of accurate fecal carriage rates was the use of different antibiotics and concentrations thereof in the media used for isolating 3GC or carbapenem E. coli. Of the 55 articles reporting animal-level or herd-level prevalence of 3GC resistance, 16 employed plates that were commercially available for 3GC detection and rest (39) added various 3GCs to routinely used lab media for 3GC detection (Supplementary File 2). Out of these 39 studies, 32 and 5 used cefotaxime and ceftriaxone at the concentration of 1–4 mg/L to supplement media, respectively. Rest of the studies used cefoxitin and ceftiofur (Supplementary File 2). Similarly, of the 14 articles that provided estimates of carbapenem resistance at herd- or animal-level, 9 used commercial plates and 5 supplemented routine laboratory media with ertapenem, meropenem and imipenem (Supplementary File 2).
Relative Abundance and Genomic Characteristics of Extended Spectrum Beta-Lactamase/ampC and Carbapenemase Genes Based on Literature Search
Most of the studies focused on identifying genes of interest after selecting isolates on a media containing carbapenems or 3GCs or after identifying individual isolates as ESBL/ampC-producers. We have provided the data on isolates collected without aforementioned selection procedures in the Supplementary File 2, but we will only discuss data on those isolates that were identified based on selection procedures in the rest of this section.
blaCTX–M were the dominant genes in ESBL/ampC or 3GC-resistant E. coli isolates in Asia and Europe and were present in 84.3% (95% CI: 76.3–91.1%) and 65.3% (95% CI: 55.1–74.9%) of such isolates in Asia and Europe, respectively (Figure 6A). blaSHV genes were present in 3.42% (95% CI: 0.08–9.55%) and 3.36% (95% CI: 1.09–6.44%) of ESBL/ampC or 3GC-resistant E. coli in Asia and Europe, respectively. blaCMY genes were present in only 3.58% (95% CI: 0.19–9.41%) and 1.99% (95% CI: 0.55–3.99%) of such isolates in Asia and Europe, respectively (Figure 6A). In contrast, blaCMY was the most prevalent gene group in 3GC-resistant E. coli in North America and these genes were present in 73.1% (95% CI: 41.9–95.4%) of the isolates; whereas blaCTX–M and blaSHV were present in 22.6% (95% CI: 0–65.7%) and 0% (95% CI: 0–1.41%) of such isolates, respectively (Figure 6A).
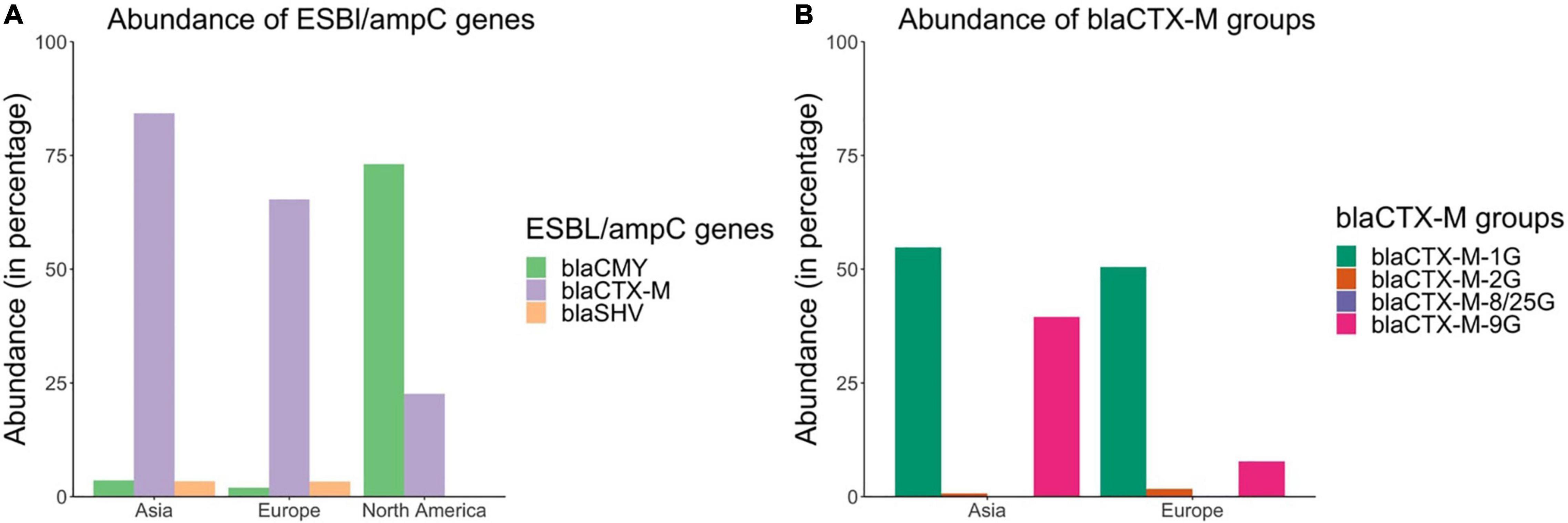
Figure 6. Abundances of (A) blaCTX–M, blaCMY, and blaSHV genes and (B) blaCTX–M groups in ESBL/ampC or 3rd generation cephalosporin resistant E. coli isolates based on the literature review.
There were also differences in the distribution of blaCTX–M groups across different continents (Figure 6B). For example, in Europe blaCTX–M–1G (consisting primarily of blaCTX–M–1, –15, –55) was the predominant blaCTX–M group and was present in 50.5% (95% CI: 40.2–60.7%) of the ESBL/ampC or 3GC-resistant E. coli isolates; whereas in Asia, both blaCTX–M–1G (54.8%, 95% CI: 44.3–65.2%) and blaCTX–M–9G (consisting of blaCTX–M–14, –27, and –65) (39.5%, 95% CI: 29.7–49.5%) were the predominant groups.
The frequency of carbapenem resistance genes in carbapenem-resistant and/or ESBL-E. coli isolates are presented in Table 3. In brief, a wide variety of carbapenem resistance bla genes were found to be circulating in pig populations in Asia and Europe. These included blaNDM–1, NDM–5, VIM–1, KPC–2, and OXA–48 in several Asian countries (China, India, and South Korea) (Table 3). blaVIM–1 and blaOXA–181 were present in pig populations in Germany and Italy (as part of a pan-European study) (Table 3). Carbapenem resistance genes were found to be circulating in E. coli isolates collected between 2001 and 2011 in South Korea and Germany (Table 3), hinting that these genes have been present in pig populations globally since a long time.
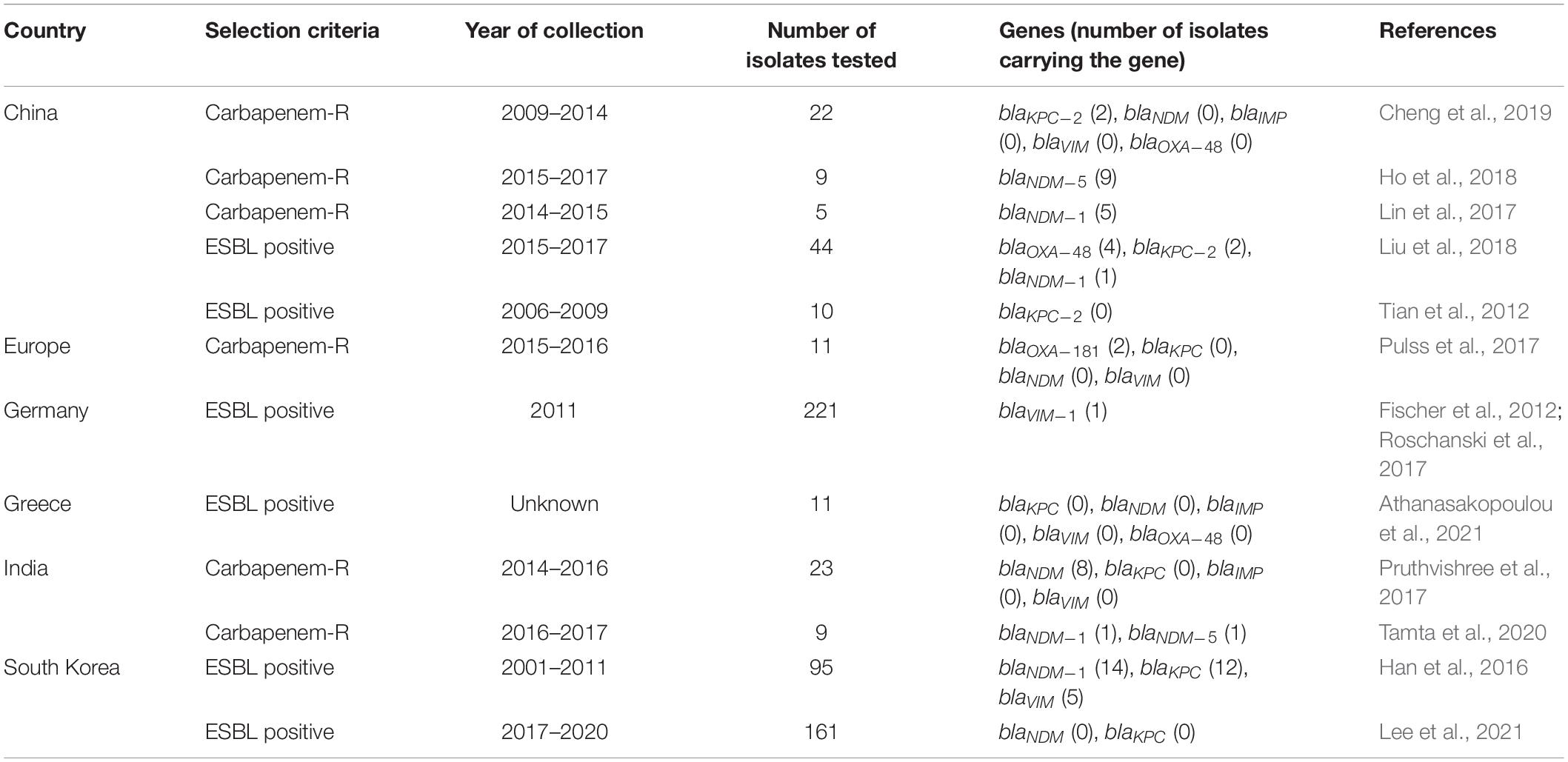
Table 3. Distribution of carbapenem resistant genes in carbapenem-resistant or ESBL-E. coli isolates in pigs based on literature review.
Relative Abundance and Genomic Characteristics of Extended Spectrum Beta-Lactamase/ampC and Carbapenemase Genes
Overall, there were 1,223 genomes out of the 6,167 sequences analyzed that carried ESBL/ampC genes (either blaCTX–M, blaCMY, or blaSHV genes) globally. Details of these genomes are available in Supplementary File 4. blaCTX–M genes were more prevalent compared to blaCMY and blaSHV genes in ESBL/ampC isolates from Asia (89.0% blaCTX–M, 10.5% blaCMY, and 1.61% blaSHV) and Europe (77.1% blaCTX–M, 17.9% blaCMY, and 6.04% blaSHV) (Figure 7A). In contrast, blaCMY were the most prevalent genes in ESBL/ampC isolates in North America (77.9% blaCMY, 21.3% blaCTX–M, and 1.15% blaSHV) (Figure 7A).
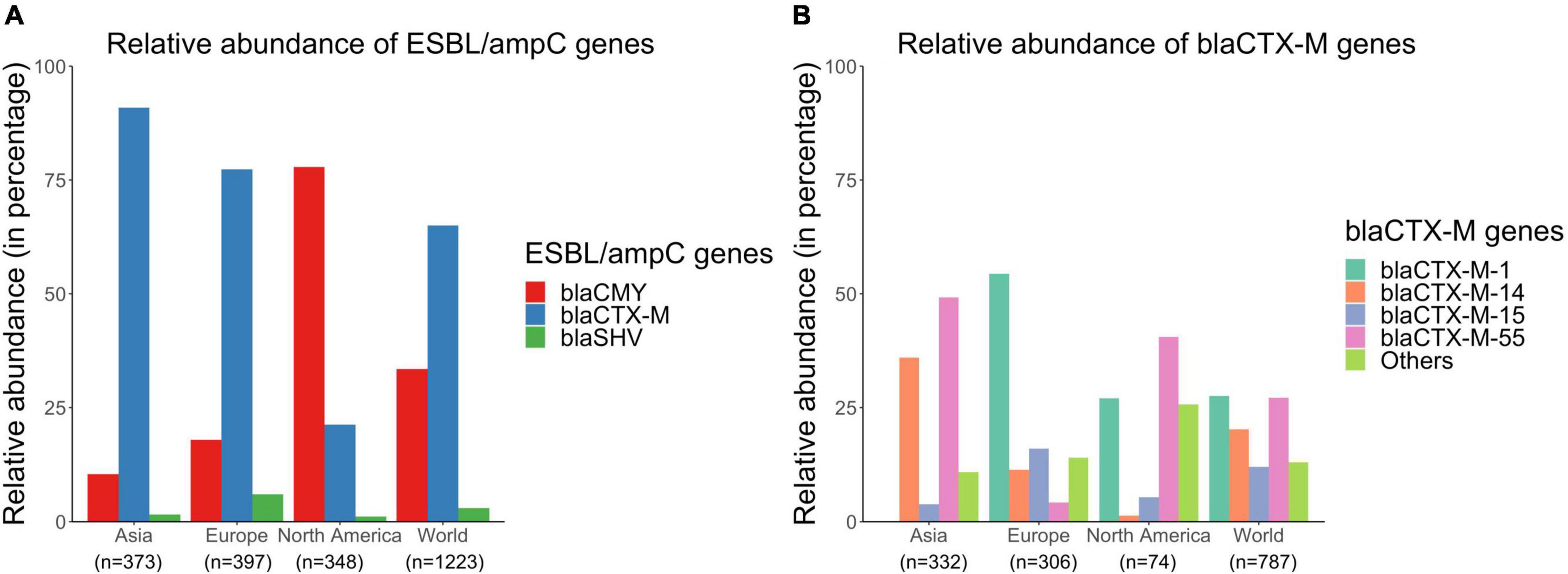
Figure 7. Relative abundances of (A) blaCTX–M, blaCMY, and blaSHV genes and (B) blaCTX–M subtypes in publicly available E. coli genomes of swine origin.
Furthermore, there were several different subtypes of blaCTX–M genes distributed globally in the sampled pig populations. blaCTX–M–1, blaCTX–M–55, and blaCTX–M–14 were the most prevalent blaCTX–M genes present in 27.8, 27.5, and 20.8% of blaCTX–M carrying isolates, respectively (Figure 7B). However, the distribution patterns of these blaCTX–M subtypes varied across different continents (Figure 7B). For example, 40.5 and 50.3% of blaCTX–M-positive isolates carried blaCTX–M–55 genes in North America and Asia, respectively, whereas only 4.25% of blaCTX–M-positive isolates carried this gene in Europe (Figure 7B). Similarly, distributions of blaCTX–M–1, –14, and –15 genes also varied across continents (Figure 7B).
Only 22 of the E. coli genomes carried a carbapenemase gene. The most common type of carbapenemase gene was blaNDM–5, that was present in 13 Chinese isolates. Other carbapenemase genes were blaNDM–1 (China, n = 3), blaIMP–27 (Canada, United States, n = 3), blaIMP–38 (China, n = 1), blaVIM–5 (Belgium, n = 1) and blaGES–5 (Germany, n = 1). Since carbapenemase genes were so rare, we were not able to generalize their genetic characteristics and global distribution, but this information can be extracted from the Supplemental File 4.
Characteristics of Isolates Carrying blaCMY and blaCTX–M Genes
Extended spectrum beta-lactamase/ampC isolates belonged to 319 unique sequence types (STs). Top five genetic characteristics of these isolates are presented in Table 4. Nearly 40% of the ESBL/ampC isolates belonged to clonal complex (CC) 10 (21.9%, including ST10 and ST744), CC23 (10.1%, including ST88 and ST410), and CC155 (7.3%, including ST58); and 75% of ESBL/ampC isolates were either phylotype A (43%) or B1 (32%).
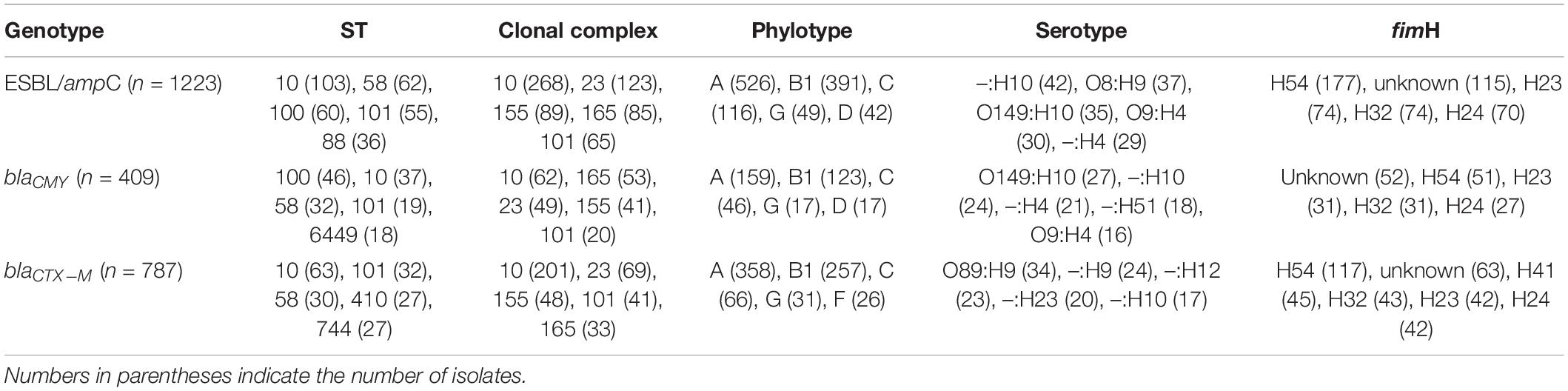
Table 4. Top characteristics of publicly available E. coli genomes of swine origin carrying chromosomal mutations in ESBL/ampC genes.
The results of random forest models are displayed in Figure 8. Specific plasmid replicon types (IncI1-alpha, IncC, and IncI2-delta), virulence factors (iro operon) and genes that can confer resistance to aminoglycosides [aph(3″)-Ib] and florfenicol (floR) were the most common predictors of presence of blaCMY gene in these E. coli genomes. Four genetic determinants of (fluoro)quinolone resistance (qnrS1, gyrA-S83L, parC-S80I, and gyrA-D87N) were among the top 10 predictors of blaCTX–M genes in these genomes, with other AMR markers [sul2, floR, aac (3)-II, and mph(A)], plasmid replicon (IncI11-alpha) and virulence factor (clpV/tssH) being the other top 10 predictors of blaCTX–M presence in isolates in swine populations.
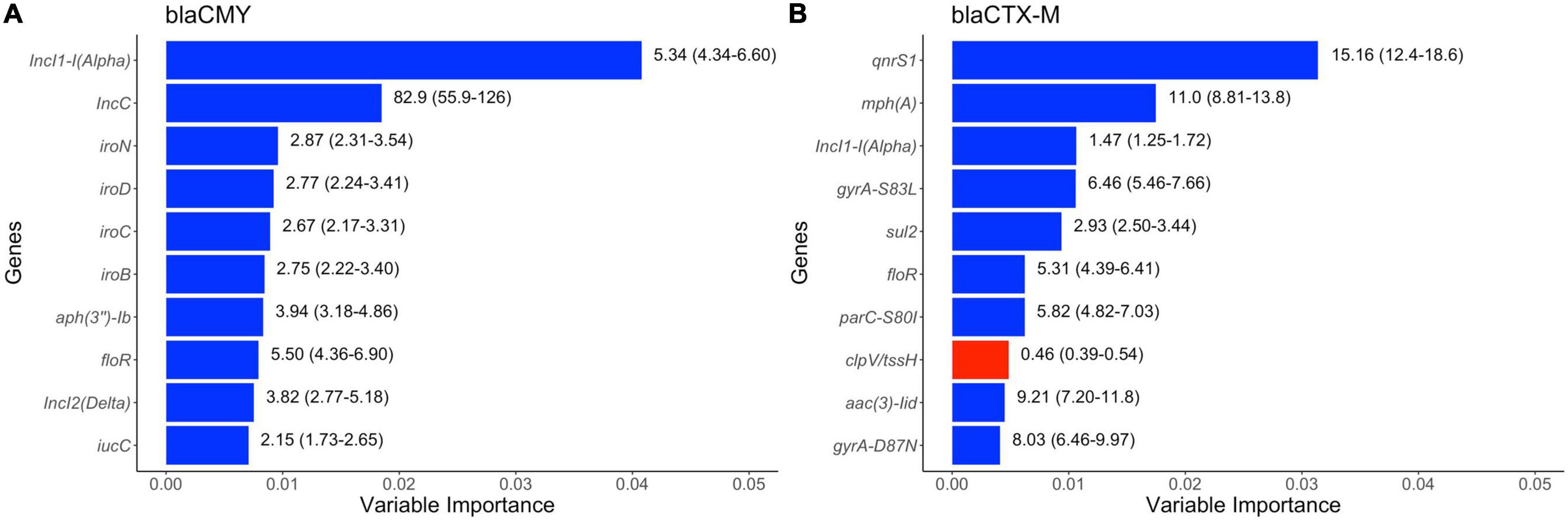
Figure 8. Top ten variables of importance predictive of (A) blaCMY and of (B) blaCTX–M genes in publicly available E. coli genomes of swine origin. Variable importance was estimated using Random Forest models. The numbers on the right side of the bars represent unadjusted odds ratio (95% confidence values). “Blue” and “red” colored bars represent positive (odds ratio > 1) and negative (odds ratio < 1) association between dependent and independent variables.
Discussion
The systematic literature review performed documented that the percent proportion estimates of phenotypic 3GC resistance in swine E. coli were variable depending on the countries and regions, as well as the source (healthy or diseased animals) from where an isolate was sampled. In general, the frequencies of 3GC resistance were low in isolates from Australia and European and North American countries. Some of these countries have imposed policies restricting the use of antimicrobials in animal production, including cephalosporins (Agerso and Aarestrup, 2013; Abraham et al., 2015; Callens et al., 2018), and this might have played a role in lower occurrence of 3GC resistance in generic E. coli isolates in pigs. Indeed, Chantziaras et al. (2014) found a high correlation between antimicrobial use and resistance using data available at a national level from seven European countries (Chantziaras et al., 2014). In contrast, the proportions of 3GC resistance were strikingly high in E. coli isolates recovered from swine in Asian countries. Asian countries have been known for higher rates of antimicrobial usage in animal production compared to other regions of the world (Van Boeckel et al., 2015). Most Asian countries however have yet to institute structured programs to monitor antimicrobial usage and surveillance of AMR (Founou et al., 2016). The results from our systematic review further emphasize the critical and immediate need to establish policy changes in Asian countries, especially regarding monitoring the use of critical antimicrobials such as 3GCs in food animals, specifically swine production. Comparing maps of sampling indices and pooled proportions of 3GC resistance, it is evident that the sampling efforts are inversely proportionate to the frequency of 3GC resistance and countries with lower number of isolates have higher proportions of 3GC resistance.
The results of this analysis also strongly suggest that a low proportion of 3GC resistance does not imply that the fecal carriage rate (i.e., prevalence at animal-level) is also low. Although fecal carriage rates are not as often discussed as isolate-level frequencies of AMR, these rates can provide valuable epidemiological and microbiological information pertinent to AMR surveillance. Fecal carriage rate is an additional important metric for measuring the extent of spread of AMR in animal populations. For example, in nationwide surveillance conducted by Swedish and Norwegian researchers between 2011 and 2019, the frequency of 3GC resistance seems to be stable and extremely rare but the fecal carriage rates are seemingly on a rise (from 10 and 5% in 2011 to 19 and 12% in 2019 from fecal samples collected in Norway and Sweden, respectively) (NORM/NORM-VET, 2012, 2020; SVARM, 2012; SWEDRES-SVARM., 2020). Secondly, screening of samples using selective screening can aid in early discovery of rarer but critical mechanisms of AMR and bacterial isolates carrying these genes. For example, in the same studies, Swedish and Norwegian researchers discovered that the increase in fecal carriage rates was due to mutations in promoter regions of chromosomal ampC genes rather than transferable ESBl/ampC genes. This discovery would not have been possible if the scientists had relied on the rather small number of 3GC resistant isolates collected during non-selective surveillance. It should be noted that different media and concentrations of antimicrobials were used for estimating fecal carriage rates in the studies we extracted data from and these differences can hinder the estimation of true prevalence of cephalosporin and carbapenem resistance (Duggett et al., 2020; Jacob et al., 2020).
Although the phenotypic occurrence of carbapenem resistance in swine populations was found to be very low, the significance of occasional studies reporting higher values should not be understated. The widespread dissemination of carbapenem resistance is a relatively recent phenomena as evidenced by the post-2010 changes in the extent and prevalence of carbapenem resistance in bacterial populations in European countries (Albiger et al., 2015). Considering the mechanistic similarities in propagation and dissemination of extended spectrum cephalosporin and carbapenem resistance (such as plasmid mediated carriage of genetic elements, broad range substrate specificity, broad bacterial host range, etc.), carbapenem resistance could become established in bacterial populations as has been the case with resistance against extended spectrum cephalosporin in E. coli from swine. Some studies on pigs already indicate that carbapenem resistance might be highly prevalent in certain countries such as Thailand and Ghana (Pokhrel et al., 2018; Dawangpa et al., 2021; Larbi et al., 2021). Also, fecal carriage rate of greater than 1% in pigs from China and India should be of significant concern. Thus, there is an active need for assessing the risk of food animals and food products, such as swine products, as a source of carbapenem resistance (Madec et al., 2017; Kock et al., 2018).
The global pattern of relative gene distribution of blaCTX–M subclasses in pigs differed from that in humans. In humans, blaCTX–M–14 and blaCTX–M–15 are the most prevalent of blaCTX–M genes, with blaCTX–M–27 identified as an emerging subclass (Bevan et al., 2017) which is in contrast to our results, where blaCTX–M–1 and blaCTX–M–55 were the most prevalent blaCTX–M genes. This difference can be attributed to the evolutionary success of pathogenic ST131 isolates which also carry blaCTX–M–14, –15, and –27 genes in humans (Nicolas-Chanoine et al., 2014; Matsumura et al., 2015), whereas in pigs, E. coli ST 131 isolates are rarely isolated (Reid et al., 2019; Flament-Simon et al., 2020; Hayer et al., 2020a), and blaCTX–M genes were present on multiple ST types with the most common being ST10.
blaCMY genes were the most common ESBL/ampC genes in North America. However, there has been a rise in blaCTX–M genes, which had not been reported in swine populations since the beginning of 2010s (Roach and Wallinga, 2013). Future studies should focus on whether there is a further turnover of ESBL/ampC genes in North America and if there is any impact of these changes on swine and human health. Finally, the genomic dataset currently available might not be representative of the distribution of the bla genes that can confer carbapenem resistance. Several studies have reported the presence of blaVIM–1, –IMP–1, –KPC, –NDM–5, and –OXA–181 in pig populations across the world (Tang et al., 2011; Fischer et al., 2012; Han et al., 2016; Pulss et al., 2017; Roschanski et al., 2017). Clearly, there is a paucity of genomic data when it comes to carbapenem resistance in pig populations. This is a major knowledge gap considering the importance of carbapenem resistance and the fact that it could only take a certain “right” combination of E. coli ST type, resistance gene(s) and plasmid to unleash the next highly resistant, virulent, pandemic E. coli clone, as has been the case with the hyper-virulent, fluoroquinolone and cephalosporin resistant ST131 clones (Stoesser et al., 2016).
The most noteworthy result from Random Forest models was a strong association of blaCTX–M genes with both plasmid-mediated and chromosomal-mediated mechanisms of fluoroquinolone resistance. Co-resistance against multiple drug classes makes the problem of AMR in bacteria even more complex, as use of one class of antimicrobials can provide positive selection pressure to maintain resistance against other antimicrobial classes (Holmes et al., 2016). Fluoroquinolones are also considered as critically important antimicrobials in human medicine (World Health Organization., 2019) and are also used in food animals in some countries for treatment purposes (Römer et al., 2017; Ahmad et al., 2021). Co-resistance to fluoroquinolones and 3GCs can hence be considered both a human and animal health threat. Previous studies have noted an increased co-resistance between these two antimicrobials at a regional or national level (Balkhed et al., 2013; Bai et al., 2015; Falgenhauer et al., 2016; Pires et al., 2021) and our results confirm that this co-resistance is widespread across multiple countries and can be considered to be a global phenomenon.
This study aimed at providing a comprehensive review on the global frequency of distribution of 3GC and carbapenem resistance in swine E. coli populations. However, there are several limitations that should be kept in mind while interpreting the results from this analysis. First, limiting articles to English-only might have excluded data from certain countries, thus introducing a publication language bias. A high number of articles did not report if the pigs were administered antimicrobials for growth promotion, disease prevention, therapy, or control. Antimicrobial use is arguably the most-often cited risk factor in selection of AMR and it has been shown that isolates from farms that do not use antimicrobials are less likely to be resistant as compared to conventional farms (Osterberg et al., 2016). Similarly, husbandry factors such as level of intensification has been correlated with the extent of AMR in developing countries (Strom et al., 2018; Lunha et al., 2020). The vast majority of the studies did not report which sampling scheme was employed during isolate selection, making the direct comparison between countries with and without surveillance programs difficult. Indeed, results from countries without a surveillance program were more likely to be statistically heterogeneous than countries with an active surveillance system with a well-designed sampling scheme. Some of the studies included were point prevalence surveys and data from such surveys might not be representative of the situation in the country as a whole. Finally, we did not include temporality in the meta-analyses and we were not able to incorporate impact of country-level policy changes regarding antimicrobial usage in our models because of data scarcity from several nations. We were not able to assess the effect of time on estimates of pooled proportions of AMR because data at a country-disease level was too scarce to analyze this effect and pooling AMR estimates from different time-points could have contributed to statistical heterogeneity in some cases.
There are several limitations of the genomic meta-analysis as well. Several plasmid-level genetic features can be investigated further to confirm the results of the random forest models. For example, we identified an association between blaCTX–M genes and sul2, floR, and mph(A). These genes have been often associated with other genetic elements such as integrons and insertion sequences which aid in transmission and co-persistence of multiple AMR genes. mph(A) have been found to be associated with class I integrons in isolates carrying ESBL genes (Hastak et al., 2020; Cameron et al., 2021). Insertion sequences have and continue to play a massive role in mobilization and evolution of bla genes (Cantón and Coque, 2006). Constructing the genetic contexts of bla genes from the contigs of the bacterial genomes can help in identifying these mobile genetic elements and associated AMR genes and verifying the results of random forest models.
Despite the above limitations, we believe that we have achieved the aim of being comprehensive while acknowledging and correcting certain biases. We are also sharing the phenotypic and genomic data in Supplementary Files 2, 4 should other researchers be interested in evaluating the results with a different approach.
Conclusion
This review summarizes the frequency and distribution of phenotypic and genotypic 3GC and carbapenem resistance and associated AMR genes in E. coli isolates collected from swine worldwide. We observed a large variability in proportions of E. coli resistant to 3GCs and distribution of associated gene markers in swine E. coli. Carbapenem resistance remains low but should be continuously monitored. We also discussed the strengths and weaknesses of the current review in order to give the full context of this complex issue to the readers. This review is useful in summarizing the continually evolving problem of AMR in swine production and reinforces the need to review current country and global policies.
Author Contributions
SH and JA conceived the review. SH and AN selected the databases and search strings. SH, AC-H, and EP retrieved the manuscripts and collected the data. SH, AC-H, EP, AN, EE, JB, TJ, AP, and JA contributed in data analysis and writing and editing manuscript. All authors contributed to the article and approved the submitted version.
Funding
This work was funded by the USDA National Institute of Food and Agriculture (Animal Health Formula Fund project MIN-62-091), Swine Disease Eradication Center fund, and the Rapid Agricultural Response Fund (RARF) at the University of Minnesota.
Conflict of Interest
The authors declare that the research was conducted in the absence of any commercial or financial relationships that could be construed as a potential conflict of interest.
Publisher’s Note
All claims expressed in this article are solely those of the authors and do not necessarily represent those of their affiliated organizations, or those of the publisher, the editors and the reviewers. Any product that may be evaluated in this article, or claim that may be made by its manufacturer, is not guaranteed or endorsed by the publisher.
Supplementary Material
The Supplementary Material for this article can be found online at: https://www.frontiersin.org/articles/10.3389/fmicb.2022.853810/full#supplementary-material
Supplementary File 1 | Database search strings.
Supplementary File 2 | Raw data collected from articles.
Supplementary File 3 | Detailed pooled proportion estimates of phenotypic 3GC and ceftazidime resistance.
Supplementary File 4 | Genotypic data including metadata from Enterobase, virulence genes, plasmid replicon types and resistance genes.
References
Abraham, S., Jordan, D., Wong, H. S., Johnson, J. R., Toleman, M. A., Wakeham, D. L., et al. (2015). First detection of extended-spectrum cephalosporin- and fluoroquinolone-resistant Escherichia coli in Australian food-producing animals. J. Glob. Antimicrob. Resist. 3, 273–277. doi: 10.1016/j.jgar.2015.08.002
Agerso, Y., and Aarestrup, F. M. (2013). Voluntary ban on cephalosporin use in danish pig production has effectively reduced extended-spectrum cephalosporinase-producing Escherichia coli in slaughter pigs. J. Antimicrob. Chemother. 68, 569–572. doi: 10.1093/jac/dks427
Ahmad, S. U., Sun, J., Cheng, F., Li, B., Arbab, S., Zhou, X., et al. (2021). Comparative study on pharmacokinetics of four long-acting injectable formulations of enrofloxacin in pigs. Front. Vet. Sci. 7:604628. doi: 10.3389/fvets.2020.604628
Albiger, B., Glasner, C., Struelens, M. J., Grundmann, H., and Monnet, D. L. (2015). Carbapenemase-producing Enterobacteriaceae in Europe: assessment by national experts from 38 countries, May 2015. Eurosurveillance 20:30062. doi: 10.2807/1560-7917.ES.2015.20.45.30062
Andrews, S. (2021). FastQC. Available onlibe at: https://github.com/s-andrews/FastQC (accessed November 29, 2021).
Athanasakopoulou, Z., Reinicke, M., Diezel, C., Sofia, M., Chatzopoulos, D. C., Braun, S. D., et al. (2021). Antimicrobial resistance genes in ESBL-producing Escherichia coli isolates from animals in Greece. Antibiotics 10:389. doi: 10.3390/antibiotics10040389
Bai, L., Lan, R., Zhang, X., Cui, S., Xu, J., Guo, Y., et al. (2015). Prevalence of Salmonella isolates from chicken and pig slaughterhouses and emergence of ciprofloxacin and cefotaxime co-resistant s. enterica serovar Indiana in Henan, China. PLoS One 10:e0144532. doi: 10.1371/journal.pone.0144532
Balkhed, ÅÖ, Tärnberg, M., Monstein, H.-J., Hällgren, A., Hanberger, H., and Nilsson, L. E. (2013). High frequency of co-resistance in CTX-M-producing Escherichia coli to non-beta-lactam antibiotics, with the exceptions of amikacin, nitrofurantoin, colistin, tigecycline, and fosfomycin, in a county of Sweden. Scand. J. Infect. Dis. 45, 271–278. doi: 10.3109/00365548.2012.734636
Bevan, E. R., Jones, A. M., and Hawkey, P. M. (2017). Global epidemiology ofCTX-Mβ-lactamases: Temporal and geographical shifts in genotype. J. Antimicrob. Chemother. 72, 2145–2155. doi: 10.1093/jac/dkx146
Bolger, A. M., Lohse, M., and Usadel, B. (2014). Trimmomatic: a flexible trimmer for Illumina sequence data. Bioinformatics 30, 2114–2120. doi: 10.1093/bioinformatics/btu170
Bortolaia, V., Kaas, R. S., Ruppe, E., Roberts, M. C., Schwarz, S., Cattoir, V., et al. (2020). ResFinder 4.0 for predictions of phenotypes from genotypes. J. Antimicrob Chemother 75, 3491–3500. doi: 10.1093/jac/dkaa345
Callens, B., Cargnel, M., Sarrazin, S., Dewulf, J., Hoet, B., Vermeersch, K., et al. (2018). Associations between a decreased veterinary antimicrobial use and resistance in commensal Escherichia coli from belgian livestock species (2011–2015). Prev. Vet. Med. 157, 50–58. doi: 10.1016/j.prevetmed.2017.10.013
Cameron, A., Mangat, R., Mostafa, H. H., Taffner, S., Wang, J., Dumyati, G., et al. (2021). Detection of CTX-M-27 β-lactamase genes on two distinct plasmid types in ST38 Escherichia coli from three U.S. states. Antimicrob Agents Chemother 65:e0082521. doi: 10.1128/AAC.00825-21
Cantón, R., and Coque, T. M. (2006). The CTX-M β-lactamase pandemic. Curr. Opin. Microbiol. 9, 466–475. doi: 10.1016/j.mib.2006.08.011
Cantón, R., and Ruiz-Garbajosa, P. (2011). Co-resistance: an opportunity for the bacteria and resistance genes. Curr. Opin. Pharmacol. 11, 477–485. doi: 10.1016/j.coph.2011.07.007
Carattoli, A., Zankari, E., García-Fernández, A., Voldby Larsen, M., Lund, O., Villa, L., et al. (2014). In silico detection and typing of plasmids using plasmidfinder and plasmid multilocus sequence typing. Antimicrob Agents Chemother 58, 3895–3903. doi: 10.1128/AAC.02412-14
Chantziaras, I., Boyen, F., Callens, B., and Dewulf, J. (2014). Correlation between veterinary antimicrobial use and antimicrobial resistance in food-producing animals: a report on seven countries. J. Antimicrob Chemother 69, 827–834. doi: 10.1093/jac/dkt443
Cheng, P., Li, F., Liu, R., Yang, Y., Xiao, T., Ishfaq, M., et al. (2019). Prevalence and molecular epidemiology characteristics of carbapenem-resistant Escherichia coli in Heilongjiang province, China. Infect. Drug Res. 12, 2505–2518. doi: 10.2147/IDR.S208122
CLSI (2021). CLSI M100-ED31:2021 Performance Standards for Antimicrobial Susceptibility Testing, 31st Edn. Wayne, PA: CLSI.
Dawangpa, A., Lertwatcharasarakul, P., Ramasoota, P., Boonsoongnern, A., Ratanavanichrojn, N., Sanguankiat, A., et al. (2021). Genotypic and phenotypic situation of antimicrobial drug resistance of Escherichia coli in water and manure between biogas and non-biogas swine farms in central Thailand. J. Environ. Manag. 279:111659. doi: 10.1016/j.jenvman.2020.111659
Duggett, N., AbuOun, M., Randall, L., Horton, R., Lemma, F., Rogers, J., et al. (2020). The importance of using whole genome sequencing and extended spectrum beta-lactamase selective media when monitoring antimicrobial resistance. Sci. Rep. 10:19880. doi: 10.1038/s41598-020-76877-7
EUCAST (2021). MIC and Zone Distributions and ECOFFs. Available online at: https://www.eucast.org/mic_distributions_and_ecoffs/ (accessed November 29, 2021)
Falgenhauer, L., Imirzalioglu, C., Ghosh, H., Gwozdzinski, K., Schmiedel, J., Gentil, K., et al. (2016). Circulation of clonal populations of fluoroquinolone-resistant CTX-M-15-producing Escherichia coli ST410 in humans and animals in Germany. Int. J. Antimicrob. Agents 47, 457–465. doi: 10.1016/j.ijantimicag.2016.03.019
Fischer, J., Rodriguez, I., Schmoger, S., Friese, A., Roesler, U., Helmuth, R., et al. (2012). Escherichia coli producing VIM-1 carbapenemase isolated on a pig farm. J. Antimicrob. Chemother. 67, 1793–1795. doi: 10.1093/jac/dks108
Flament-Simon, S.-C., de Toro, M., Mora, A., Garcia, V., Garcia-Menino, I., Diaz-Jimenez, D., et al. (2020). Whole Genome Sequencing and Characteristics of mcr-1-harboring plasmids of porcine Escherichia coli Isolates belonging to the high-risk clone O25b:H4-ST131 clade b. Front. Microbiol. 11:387. doi: 10.3389/fmicb.2020.00387
Founou, L. L., Founou, R. C., and Essack, S. Y. (2016). Antibiotic resistance in the food chain: a developing country-perspective. Front. Microbiol. 7:1881. doi: 10.3389/fmicb.2016.01881
Food and Agriculture Organisation (2022). FAOSTAT. Available online at: https://www.fao.org/faostat/en/#home (accessed 25 March, 2022).
Giske, C. G., Sundsfjord, A. S., Kahlmeter, G., Woodford, N., Nordmann, P., Paterson, D. L., et al. (2009). Redefining extended-spectrum β-lactamases: balancing science and clinical need. J. Antimicrob Chemother 63, 1–4. doi: 10.1093/jac/dkn444
Han, J. W., Koh, H. B., and Kim, T. J. (2016). Molecular Characterization of β-Lactamase-Producing Escherichia coli Collected from 2001 to 2011 from Pigs in Korea. Foodborne Pathog. Dis. 13, 68–76. doi: 10.1089/fpd.2015.2017
Hastak, P., Cummins, M. L., Gottlieb, T., Cheong, E., Merlino, J., Myers, G. S. A., et al. (2020). Genomic profiling of Escherichia coli isolates from bacteraemia patients: a 3-year cohort study of isolates collected at a sydney teaching hospital. Microb. Genom. 6:e000371. doi: 10.1099/mgen.0.000371
Hayer, S. S., Casanova-Higes, A., Paladino, E., Elnekave, E., Nault, A., Johnson, T., et al. (2022). Global distribution of fluoroquinolone and colistin resistance and associated resistance markers in Escherichia coli of swine origin –a systematic review and meta-analysis. Front. Microbiol. 13:834793. doi: 10.3389/fmicb.2022.834793
Hayer, S. S., Rovira, A., Olsen, K., Johnson, T. J., Vannucci, F., Rendahl, A., et al. (2020b). Prevalence and time trend analysis of antimicrobial resistance in respiratory bacterial pathogens collected from diseased pigs in USA between 2006–2016. Res. Vet. Sci. 128, 135–144. doi: 10.1016/j.rvsc.2019.11.010
Hayer, S. S., Lim SeungHyun Hong, S., Elnekave, E., Johnson, T., et al. (2020a). Genetic determinants of resistance to extended-spectrum cephalosporin and fluoroquinolone in Escherichia coli isolated from diseased pigs in the united states. mSphere 5, e990–e920. doi: 10.1128/mSphere.00990-20
Hiki, M., Shimizu, Y., Kawanishi, M., Ozawa, M., Abo, H., Kojima, A., et al. (2017). Evaluation of the relationship between the minimum inhibitory concentration of ceftiofur and third-generation cephalosporins in Escherichia coli isolates from food-producing animals. J. Vet. Diagn. Invest. 29, 716–720. doi: 10.1177/1040638717713794
Ho, P.-L., Wang, Y., Liu, M. C.-J., Lai, E. L.-Y., Law, P. Y.-T., Cao, H., et al. (2018). IncX3 epidemic plasmid carrying bla(NDM-5) in Escherichia coli from Swine in multiple geographic areas in China. Antimicrob Agents Chemother 62, e2295–e2217. doi: 10.1128/AAC.02295-17
Holmes, A. H., Moore, L. S. P., Sundsfjord, A., Steinbakk, M., Regmi, S., Karkey, A., et al. (2016). Understanding the mechanisms and drivers of antimicrobial resistance. Lancet 387, 176–187. doi: 10.1016/S0140-6736(15)00473-0
Ishwaran, H., and Kogalur, U. (2021). randomForestSRC-Fast Unified Random Forests for Survival, Regression, and Classification (RF-SRC). Available online at: https://cran.r-project.org/web/packages/randomForestSRC/randomForestSRC.pdf (accessed November 29, 2021).
Iwamoto, M., Reynolds, J., Karp, B. E., Tate, H., Fedorka-Cray, P. J., Plumblee, J. R., et al. (2017). Ceftriaxone-Resistant Nontyphoidal Salmonella from humans, retail meats, and food animals in the united states, 1996–2013. Foodborne Pathog. Dis. 14, 74–83. doi: 10.1089/fpd.2016.2180
Jacob, M. E., Keelara, S., Aidara-Kane, A., Matheu Alvarez, J. R., and Fedorka-Cray, P. J. (2020). Optimizing a screening protocol for potential extended-spectrum β-lactamase Escherichia coli on MacConkey agar for use in a global surveillance program. J. Clin. Microbiol. 58, e1039–e1019. doi: 10.1128/JCM.01039-19
Kock, R., Daniels-Haardt, I., Becker, K., Mellmann, A., Friedrich, A., Mevius, D., et al. (2018). Carbapenem-resistant Enterobacteriaceae in wildlife, food-producing, and companion animals: a systematic review. Clin. Microbiol. Infect. 24, 1241–1250. doi: 10.1016/j.cmi.2018.04.004
Kuhn, M., Wing, J., Weston, S., Williams, A., Keefer, C., Engelhardt, A., et al. (2021). Caret-Classification and Regression Training. Available online at: https://cran.r-project.org/web/packages/caret/caret.pdf (accessed November 29, 2021).
Larbi, R., Ofori, L., Sylverken, A., Ayim-Akonor, M., and Obiri-Danso, K. (2021). Antimicrobial resistance of Escherichia coli from broilers, pigs, and cattle in the greater kumasi metropolis, Ghana. Int. J. Microbiol. 2021, 1–7. doi: 10.1155/2021/5158185
Lee, S., An, J.-U., Guk, J.-H., Song, H., Yi, S., Kim, W.-H., et al. (2021). Prevalence, characteristics and clonal distribution of extended-spectrum beta-lactamase- and ampc beta-lactamase-producing Escherichia coli following the swine production stages, and potential risks to humans. Front. Microbiol. 12:710747. doi: 10.3389/fmicb.2021.710747
Lin, D., Xie, M., Li, R., Chen, K., Chan, E. W.-C., and Chen, S. (2017). IncFII Conjugative Plasmid-Mediated Transmission of blaNDM-1 Elements among animal-borne Escherichia coli Strains. Antimicrob Agents Chemother 61, e2285–e2216. doi: 10.1128/AAC.02285-16
Liu, X., Liu, H., Wang, L., Peng, Q., Li, Y., Zhou, H., et al. (2018). Molecular characterization of extended-spectrum beta-lactamase-producing multidrug resistant Escherichia coli from swine in Northwest China. Front. Microbiol. 9:1756. doi: 10.3389/fmicb.2018.01756
Lunha, K., Leangapichart, T., Jiwakanon, J., Angkititrakul, S., Sunde, M., Jarhult, J. D., et al. (2020). Antimicrobial resistance in fecal Escherichia coli from humans and pigs at farms at different levels of intensification. Antibiotics 9:662. doi: 10.3390/antibiotics9100662
Madec, J. Y., Haenni, M., Nordmann, P., and Poirel, L. (2017). Extended-spectrum β-lactamase/AmpC- and carbapenemase-producing Enterobacteriaceae in animals: a threat for humans? Clin. Microbiol. Infect. 23, 826–833. doi: 10.1016/j.cmi.2017.01.013
Malberg Tetzschner, A. M., Johnson, J. R., Johnston, B. D., Lund, O., and Scheutz, F. (2020). In silico genotyping of Escherichia coli isolates for extraintestinal virulence genes by use of whole-genome sequencing data. J. Clin. Microbiol. 58, e1269–e1220. doi: 10.1128/JCM.01269-20
Manges, A. R., Geum, H. M., Guo, A., Edens, T. J., Fibke, C. D., and Pitout, J. D. D. (2019). Global extraintestinal pathogenic Escherichia coli (ExPEC) lineages. Clin Microb. Rev. 32, e135–e118. doi: 10.1128/CMR.00135-18
Matsumura, Y., Johnson, J. R., Yamamoto, M., Nagao, M., Tanaka, M., Takakura, S., et al. (2015). CTX-M-27- and CTX-M-14-producing, ciprofloxacin-resistant Escherichia coli of the H30 subclonal group within ST131 drive a Japanese regional ESBL epidemic. J. Antimicrob Chemother 70, 1639–1649. doi: 10.1093/jac/dkv017
Miller, J. J. (1978). The inverse of the freeman –tukey double arcsine transformation. Am. Stat. 32, 138–138. doi: 10.1080/00031305.1978.10479283
Mollenkopf, D. F., Stull, J. W., Mathys, D. A., Bowman, A. S., Feicht, S. M., Grooters, S. V., et al. (2017). Carbapenemase-Producing Enterobacteriaceae recovered from the environment of a swine farrow-to-finish operation in the united states. Antimicrob Agents Chemother 61, 1–9. doi: 10.1128/AAC.01298-16
Nicolas-Chanoine, M.-H., Bertrand, X., and Madec, J.-Y. (2014). Escherichia coli ST131, an intriguing clonal group. Clin. Microbiol. Rev. 27, 543–574. doi: 10.1128/CMR.00125-13
Nielsen, S. S., Bicout, D. J., Calistri, P., Canali, E., Drewe, J. A., Garin-Bastuji, B., et al. (2021). Assessment of animal diseases caused by bacteria resistant to antimicrobials: swine. EFSA J. 19:e07113. doi: 10.2903/j.efsa.2021.7113
Nordmann, P., Dortet, L., and Poirel, L. (2012). Carbapenem resistance in Enterobacteriaceae: here is the storm! Trends Mol. Med. 18, 263–272. doi: 10.1016/j.molmed.2012.03.003
NORM/NORM-VET (2012). Usage of Antimicrobial Agents and Occurrence of Antimicrobial Resistance in Norway (2011). Oslo: Norm/norm-vet.
NORM/NORM-VET (2020). Usage of Antimicrobial Agents and Occurrence of Antimicrobial Resistance in Norway (2019). Oslo: Norm/norm-vet.
O’Brien, R., and Ishwaran, H. (2019). A random forests quantile classifier for class imbalanced data. Pattern Recognit. 90, 232–249. doi: 10.1016/j.patcog.2019.01.036
Osterberg, J., Wingstrand, A., Jensen, A. N., Kerouanton, A., Cibin, V., Barco, L., et al. (2016). Antibiotic resistance in Escherichia coli from pigs in organic and conventional farming in four European countries. PLoS One 11:e0157049. doi: 10.1371/journal.pone.0157049
Pfeifer, Y., Cullik, A., and Witte, W. (2010). Resistance to cephalosporins and carbapenems in gram-negative bacterial pathogens. Int. J. Med. Microbiol. 300, 371–379. doi: 10.1016/j.ijmm.2010.04.005
Pires, J., Huisman, J. S., Bonhoeffer, S., and Van Boeckel, T. P. (2021). Multidrug resistance dynamics in Salmonella in food animals in the united states: an analysis of genomes from public databases. Microb. Spectr. 9:e0049521. doi: 10.1128/Spectrum.00495-21
Pokhrel, N., Kasemsuwan, S., Goutard, F., Viriyarampa, S., and Tulayakul, P. (2018). Antibiotic resistance and extended-spectrum beta -lactamase productivity in clinical Escherichia coli isolates from diarrheal pigs in central Thailand. J. Kasetsart Vet. 28, 1–17.
Pruthvishree, B., Vinodh Kumar, O., Sinha, D., Malik, Y., Dubal, Z., Desingu, P., et al. (2017). Spatial molecular epidemiology of carbapenem-resistant and New Delhi metallo beta-lactamase (blaNDM) -producing Escherichia coli in the piglets of organized farms in India Spatial molecular epidemiology of carbapenem-resistant and New Delhi metallo beta. J. Appl. Microbiol. 122, 1537–1546. doi: 10.1111/jam.13455
Pulss, S., Semmler, T., Prenger-berninghoff, E., Bauerfeind, R., and Ewers, C. (2017). First report of an Escherichia coli strain from swine carrying an OXA-181 carbapenemase and the colistin resistance determinant MCR-1. Int. J. Antimicrob. Agents 50, 232–236. doi: 10.1016/j.ijantimicag.2017.03.014
Reid, C. J., McKinnon, J., and Djordjevic, S. P. (2019). Clonal ST131-H22 Escherichia coli strains from a healthy pig and a human urinary tract infection carry highly similar resistance and virulence plasmids. Microb. Genom. 5:000295. doi: 10.1099/mgen.0.000295
Roach, S., and Wallinga, D. (2013). Commentary on genetic mechanisms of antimicrobial resistance in bacteria from U.S. food animals: ESBLs are here. Front. Microbiol. 4:214. doi: 10.3389/fmicb.2013.00214
Römer, A., Scherz, G., Reupke, S., Meißner, J., Wallmann, J., Kietzmann, M., et al. (2017). Effects of intramuscularly administered enrofloxacin on the susceptibility of commensal intestinal Escherichia coli in pigs (sus scrofa domestica). BMC Vet Res 13:378. doi: 10.1186/s12917-017-1260-8
Roschanski, N., Friese, A., von Salviati-Claudius, C., Hering, J., Kaesbohrer, A., Kreienbrock, L., et al. (2017). Prevalence of carbapenemase producing Enterobacteriaceae isolated from German pig-fattening farms during the years 2011–2013. Vet. Microbiol. 200, 124–129. doi: 10.1016/j.vetmic.2015.11.030
Schwarzer, G. (2021). meta-General Package for Meta-Analysis. Available online at: https://cran.r-project.org/web/packages/meta/meta.pdf (accessed November 29, 2021).
Seemann, T. (2021b). Shovill. Available online at: https://github.com/tseemann/shovill (accessed November 29, 2021).
Seemann, T. (2021a). Abricate. Available online at: https://github.com/tseemann/abricate
Stoesser, N., Sheppard, A. E., Pankhurst, L., De Maio, N., Moore, C. E., Sebra, R., et al. (2016). Evolutionary history of the global emergence of the Escherichia coli epidemic clone ST131. mBio 7, e02162. doi: 10.1128/mBio.02162-15
Strom, G., Boqvist, S., Albihn, A., Fernstrom, L., Djurfeldt, A., Sokerya, S., et al. (2018). Antimicrobials in small-scale urban pig farming in a lower middle-income country –arbitrary use and high resistance levels. Antimicrob Res. Infect. Control. 7:35. doi: 10.1186/s13756-018-0328-y
Sun, D., Mi, K., Hao, H., Xie, S., Chen, D., and Huang, L. (2020). Optimal regimens based on PK/PD cutoff evaluation of ceftiofur against actinobacillus pleuropneumoniae in swine. BMC Vet. Res. 16:366. doi: 10.1186/s12917-020-02589-9
SVARM (2012). Swedish Veterinary Antimicrobial Resistance Monitoring (2011). Uppsala: The National Veterinary Institute (SVA).
SWEDRES-SVARM. (2020). Consumption of Antibiotics and Occurrence Of Antibiotic Resistance In Sweden (2019). Uppsala: National Veterinary Institute.
Tadesse, D. A., Zhao, S. H., Tong, E., Ayers, S., Singh, A., Bartholomew, M. J., et al. (2012). Antimicrobial drug resistance in Escherichia coli from humans and food animals, united states, 1950-2002. Emerg. Infect. Dis. 18, 741–749. doi: 10.3201/eid1805.111153
Tamta, S., Kumar, V. O. R., Pruthvishree, B. S., Karthikeyan, R., Rupner, R. N., Chethan, G. E., et al. (2020). Faecal carriage of extended spectrum beta-lactamase (ESBL) and New Delhi metallo beta-lactamase(NDM) producing Escherichia coli between piglets and pig farmworkers. Comp. Immunol. Microbiol. Infect. Dis. 73:101564. doi: 10.1016/j.cimid.2020.101564
Tang, X., Tan, C., Zhang, X., Zhao, Z., Xia, X., Wu, B., et al. (2011). Antimicrobial resistances of extraintestinal pathogenic Escherichia coli isolates from swine in China. Microb. Pathogen. 50, 207–212. doi: 10.1016/j.micpath.2011.01.004
Tian, G. B., Wang, H. N., Zhang, A. Y., Zhang, Y., Fan, W. Q., Xu, C. W., et al. (2012). Detection of clinically important β-lactamases in commensal Escherichia coli of human and swine origin in western China. J. Med. Microbiol. 61, 233–238. doi: 10.1099/jmm.0.036806-0
Tuszynski, J. (2021). CaTools-Tools: Moving Window Statistics, GIF, Base64, ROC AUC, etc. Available online at: https://cran.r-project.org/web/packages/caTools/caTools.pdf (accessed November 29, 2021).
Van Boeckel, T. P., Brower, C., Gilbert, M., Grenfell, B. T., Levin, S. A., Robinson, T. P., et al. (2015). Global trends in antimicrobial use in food animals. Proc. Natl. Acad. Sci. U.S.A. 112, 5649–5654. doi: 10.1073/pnas.1503141112
Van Boeckel, T. P., Pires, J., Silvester, R., Zhao, C., Song, J., Criscuolo, N. G., et al. (2019). Global trends in antimicrobial resistance in animals in low–and middle-income countries. Science 365, eaaw1944. doi: 10.1126/science.aaw1944
Wickham, H., Chang, W., Henry, L., Pedersen, T., Takahashi, K., Wilke, C., et al. (2021). GGplot2-Create Elegant Data Visualisations Using the Grammar of Graphics. Available online at: https://cran.r-project.org/web/packages/ggplot2/ggplot2.pdf (accessed November 29, 2021).
Woodford, N., Wareham, D. W., Guerra, B., and Teale, C. (2014). Carbapenemase-producing enterobacteriaceae and non-enterobacteriaceae from animals and the environment: An emerging public health risk of our own making? J. Antimicrob Chemother 69, 287–291. doi: 10.1093/jac/dkt392
World Health Organization. (2019). Critically Important Antimicrobials For Human Medicine. 6th Rev. Geneva: World Health Organization.
Zankari, E., Allesøe, R., Joensen, K. G., Cavaco, L. M., Lund, O., and Aarestrup, F. M. (2017). PointFinder: a novel web tool for WGS-based detection of antimicrobial resistance associated with chromosomal point mutations in bacterial pathogens. J. Antimicrob. Chemother. 72, 2764–2768. doi: 10.1093/jac/dkx217
Keywords: antimicrobial resistance, pigs, cephalosporin, carbapenem, ESBL, ampC, Escherichia coli, systematic review
Citation: Hayer SS, Casanova-Higes A, Paladino E, Elnekave E, Nault A, Johnson T, Bender J, Perez A and Alvarez J (2022) Global Distribution of Extended Spectrum Cephalosporin and Carbapenem Resistance and Associated Resistance Markers in Escherichia coli of Swine Origin – A Systematic Review and Meta-Analysis. Front. Microbiol. 13:853810. doi: 10.3389/fmicb.2022.853810
Received: 13 January 2022; Accepted: 20 April 2022;
Published: 10 May 2022.
Edited by:
Karsten Becker, University Medicine Greifswald, GermanyReviewed by:
Robin Koeck, Institut für Hygiene, DRK Kliniken Berlin, GermanyEthan Robert Wyrsch, University of Technology Sydney, Australia
Copyright © 2022 Hayer, Casanova-Higes, Paladino, Elnekave, Nault, Johnson, Bender, Perez and Alvarez. This is an open-access article distributed under the terms of the Creative Commons Attribution License (CC BY). The use, distribution or reproduction in other forums is permitted, provided the original author(s) and the copyright owner(s) are credited and that the original publication in this journal is cited, in accordance with accepted academic practice. No use, distribution or reproduction is permitted which does not comply with these terms.
*Correspondence: Shivdeep Singh Hayer, hayer002@umn.edu