Telomerase Reverse Transcriptase Polymorphism rs2736100: A Balancing Act between Cancer and Non-Cancer Disease, a Meta-Analysis
- 1Interstitial Lung Diseases Center of Excellence, Department of Pulmonology, St Antonius Hospital, Nieuwegein, Netherlands
- 2Interstitial Lung Diseases Center of Excellence, Department of Pathology, St Antonius Hospital, Nieuwegein, Netherlands
- 3Division of Heart and Lung, University Medical Center Utrecht, Utrecht, Netherlands
The enzyme telomerase reverse transcriptase (TERT) is essential for telomere maintenance. In replicating cells, maintenance of telomere length is important for the preservation of vital genetic information and prevention of genomic instability. A common genetic variant in TERT, rs2736100 C/A, is associated with both telomere length and multiple diseases. Carriage of the C allele is associated with longer telomere length, while carriage of the A allele is associated with shorter telomere length. Furthermore, some diseases have a positive association with the C and some with the A allele. In this study, meta-analyses were performed for two groups of diseases, cancerous diseases, e.g., lung cancer and non-cancerous diseases, e.g., pulmonary fibrosis, using data from genome-wide association studies and case-control studies. In the meta-analysis it was found that cancer positively associated with the C allele (pooled OR 1.16 [95% CI 1.09–1.23]) and non-cancerous diseases negatively associated with the C allele (pooled OR 0.81 [95% CI 0.65–0.99]). This observation illustrates that the ambiguous role of telomere maintenance in disease hinges, at least in part, on a single locus in telomerase genes. The dual role of this single nucleotide polymorphism also emphasizes that therapeutic agents aimed at influencing telomere maintenance should be used with caution.
Introduction
Telomere biology is emerging as a significant factor in an increasing number of diseases (1–4). Studies have found disease associations with both abnormal telomere length and with genetic variants are related to telomere biology (5–7). Telomeres are non-coding tandem repeats spatially organized by specialized proteins that maintain stability of the chromosome ends (8–10). Furthermore, telomeres serve as a buffer against the shortening of chromosomes, thereby preventing the loss of vital genetic information (11). To maintain replicative potential, telomeres can be elongated by the ribonucleoprotein telomerase (12, 13). Telomerase consists of a catalytic protein component, encoded by the gene telomerase reverse transcriptase (TERT), and a RNA template, encoded by telomerase RNA component.
Over the course of the human lifespan, the average length of telomeres can be disproportionally influenced by a number of factors, leading to a broad spectrum of diseases. Genetic variation in telomere maintenance genes has been shown to either accelerate or prohibit telomere shortening. Genomic mutations in the coding regions of telomerase genes are primarily found in degenerative diseases like dyskeratosis congenita, aplastic anemia, and idiopathic pulmonary fibrosis (IPF) (14–16). These mutations generally lead to a decrease in telomerase activity (17) and shorter telomeres in mutation carriers who develop fatal disease due to organ failure. Mutations in the coding regions of telomerase genes are very rare in cancer, however, somatic mutations in the promotor region of the TERT gene has been reported in the context of several cancer types (18–20). These mutations generally lead to an increase in telomerase activity, which corroborates the observation of high levels of telomerase activity in cancer cells (21). Telomerase activation and the subsequent telomere elongation lead to the immortalization of cells and prevent fatal instability of the chromosomes, opening up the possibility of unrestricted cell proliferation (21, 22).
However, Telomere biology is an ambiguous factor in cancer pathology (23–25). In healthy individuals it is thought that the restricted transcription of telomerase and the resulting limited number of cell divisions present a barrier to unlimited replication of somatic cells, thus preventing cancer (26, 27). However, other research suggests that telomere shortening can lead to chromosomal instability in the form of chromosome fusion, genomic copy addition, deletion, and mutation, which in turn can lead to tumor initiation (22, 28–31). This duplicity is apparent in humans, where both long and short telomere length of white blood cells has been associated with different cancers (32–36). As short telomeres can lead to damaged chromosomes, it is proposed that long telomeres postpone senescence, thereby increasing the risk for cells to acquire genetic abnormalities that facilitate tumorigenesis (35, 37, 38).
Besides rare mutations in telomerase genes, common genetic variation in these genes has also been associated with disease. A well-studied example is the single nucleotide polymorphism (SNP) rs2736100 in the TERT gene (5p15.33). Interestingly, the first report on a disease association to this SNP, was to the non-cancerous disease IPF (39). An IPF susceptibility odds ratio (OR) of 1.82 [95% CI: 1.47–2.22] was found for the A allele of this SNP. The second report on this SNP showed an association between lung cancer and the C allele of this SNP (40). Later studies have shown an association between the A allele and shorter blood cell telomere length, while it follows that the C allele is associated with longer telomeres (41, 42). This duality in disease association of the rs2736100 alleles might reflect a fundamentally different role of telomere biology in cancerous diseases as opposed to non-cancerous diseases. Such a dichotomy would underline that therapeutic agents influencing telomere length or telomerase activity should be used with caution, as both (too) long and short telomeres could lead to disease.
The aim of this study is to conduct a systematic review and meta-analysis of disease association studies with TERT SNP rs2736100 and to gain insight in the balancing act between telomere maintenance and disease predisposition.
Materials and Methods
Study Selection
The electronic databases PubMed1 and Embase2 were queried for studies on TERT SNP rs2736100 by using “rs2736100” as search input (Figure 1). Initially 92 studies were found. After selecting for papers pertaining to the subject of these review 57 studies remained. Of these, 49 studies described associations between TERT SNP rs2736100 and cancer, and 8 studies described associations between this SNP and non-cancer disease. Another 28 studies were added through references found in the original studies. Excluded were reviews and meta-analyses, and studies from which no OR data was available or could be calculated. Furthermore, studies were excluded that did not provide definite data on which allele was associated with risk for the investigated disease. Finally, 85 studies were included of which 77 described association with cancer and 8 with non-cancer and the TERT SNP, respectively.
Eligibility Criteria
Included in this meta-analysis were case-control and genome-wide association studies assessing the association between TERT SNP rs2736100 and disease. These studies were furthermore included when the associated allele and the used inheritance model were clearly derivable from the study. Results of meta-analyses were excluded; however, these studies were searched for eligible studies to be included in the present study. This lead to further inclusion of studies that did not find significant associations between rs2736100 and disease, thereby preventing major publication bias. Finally, systematic reviews, abstracts, non-english studies and studies investigating rs2736100 not in the context of disease were excluded from the meta-analysis.
Data Extraction
The following data was derived from each study: first author, year of publication, number of cases and controls, associated disease (cancer and non-cancer), and OR and 95% confidence interval (95% CI). Furthermore, the used inheritance model was checked as well as in which allele specifically was associated with the studied disease. When this information was not provided it was derived from the provided genotype data when possible.
Bias Evaluation
(Publication) bias was evaluated by visual inspection of Doi plots, as well as calculates the Luis Furuya-Kanamori (LFK) index, which provides a statistic for the amount of bias in a Doi plot (No asymmetry: LFK index within ±1, minor asymmetry: LFK index exceeds ±1 but within ±2, major asymmetry: LFK index exceeds ±2).
Statistical Analysis
rs2736100 associations and (publication) bias were both analyzed using meta-analysis software MetaXL 4.0 (EpiGear International, Sunrise Beach, Australia). Due to the large number of different diseases that were included in this study, a high level of variation in study outcome, heterogeneity, was expected. The meta-analysis was, therefore, performed using the inverse variance heterogeneity method, to determine the pooled result and heterogeneity (43). Many studies do not provide genotype data. Therefore, we performed meta-analysis on the OR and 95% CI for allelic association.
Results
Non-Cancerous Diseases
A meta-analysis was performed to analyze the association of TERT SNP rs2736100 with a group of cancer diseases and with a group of non-cancerous diseases. Table 1 shows all included studies. For the non-cancer group, 8 studies were found with diagnoses of pulmonary fibrosis and coronary heart disease among others. Each of these eight studies showed either a negative association between the C allele and disease or a non-significant result. Figure 2 shows a forest plot of a meta-analysis of these studies using the inverse variance heterogeneity model (43). The OR in this figure is an effect measure for the association with the C allele of rs2736100 in a co-dominant model. The pooled negative association with disease for the C allele was significant with an OR ratio of 0.81 [95% CI: 0.65–0.99]. Presence of the C allele is protective for non-cancerous diseases.
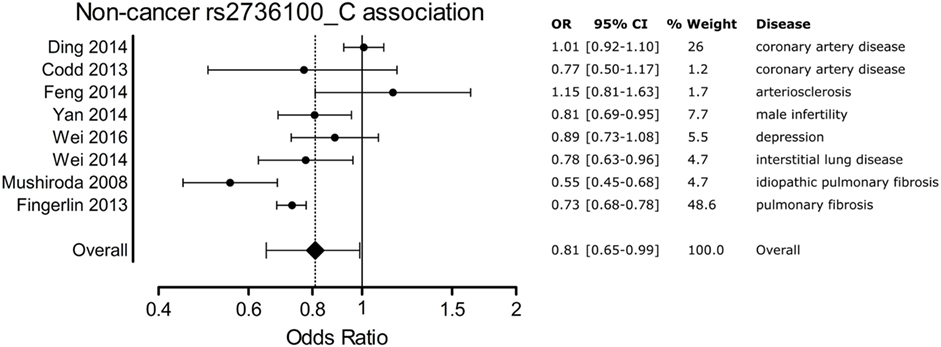
Figure 2. Meta-analysis of non-cancer association to rs2736100 allele C. OR, odds ratio; 95% CI, 95% confidence interval.
Association with Cancer
Meta-analysis for associations with cancer included 77 studies. These studies included a variety of cancers of which the majority (n = 46) involved studies on lung cancer (n = 33) and glioma (n = 13). The majority of studies reported a positive association with the C allele of rs2736100. However, four of the included studies reported a negative association with the C allele, these included two studies on testicular cancer, one on colorectal cancer and one on pancreatic cancer (50, 76, 109). In the meta-analysis of cancer studies, the pooled effect size was significant with a pooled OR of 1.16 [95% CI: 1.09–1.23] (Figure 3) and shows that the C allele is a risk allele for cancer.
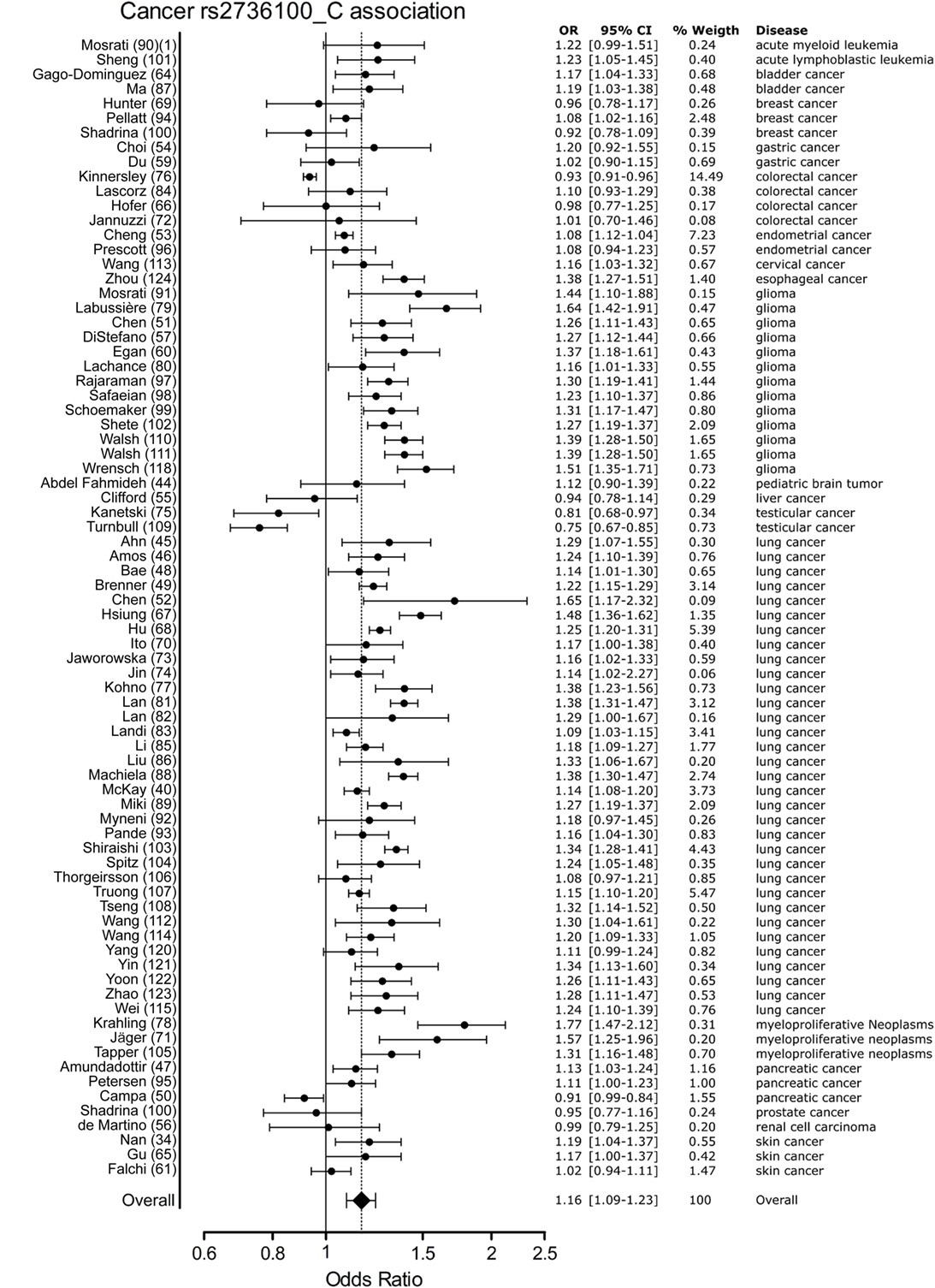
Figure 3. Meta-analysis of cancer association to rs2736100 allele C. OR, odds ratio; 95% CI, 95% confidence interval.
Figure 4 shows the significant pooled ORs for the meta-analysis of cancer and non-cancer diseases.
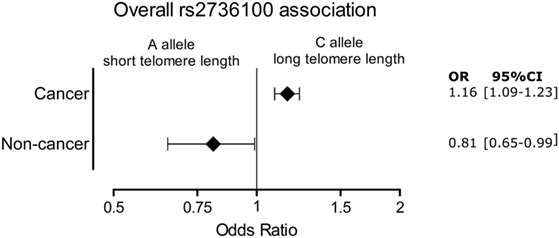
Figure 4. Overall association of the cancer and age-related group of diseases with telomerase reverse transcriptase rs2736100 allele C. OR, odds ratio; 95% CI, 95% confidence interval.
Potential bias from publication selection and other sources was evaluated using Doi plots and quantified by the LFK index (Figure 5). Figure 5A shows the Doi plot for the cancer meta-analysis. A LFK index of 109 was found from indication minor influence from publication bias or bias from other sources. Figure 5B shows the Doi plot for non-cancer diseases.
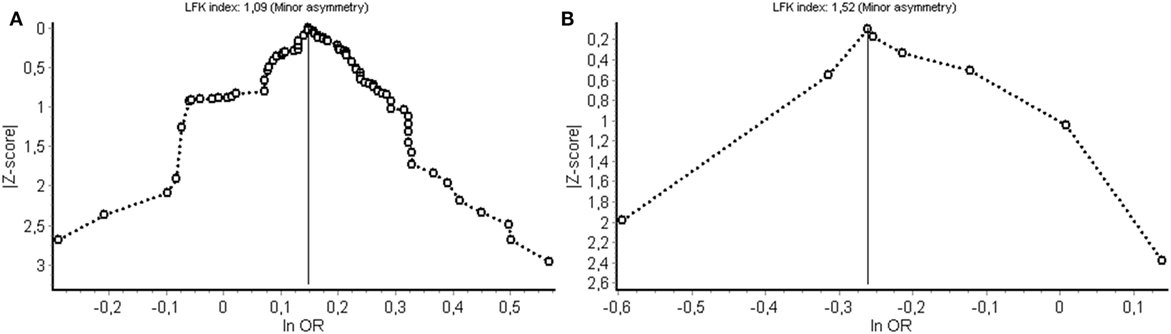
Figure 5. Evaluation of (publication) bias for the cancer (A) and non-cancer (B) meta-analysis. LFK index, Luis Furuya-Kanamori index; OR, odds ratio.
Discussion
The results of the meta-analyses illustrate the duality of telomere biology in disease predisposition. Pooled analysis showed that non-cancerous diseases, such as pulmonary fibrosis and coronary artery disease, associate positively with the telomerase A allele that is linked to shorter telomeres. Pooled analysis of cancer studies, however, showed an association with the opposite allele, the C allele that is linked to longer telomere length. This is supported by a recent study that showed an association of genetically increased telomere length and cancer, while the opposite protected against non-cancerous diseases (125). This two-sided association suggests opposite roles of telomere length in cancerous and non-cancerous diseases.
The TERT SNP rs2736100 has robustly been associated with telomere length in healthy controls (41, 42). Studies showed that presence of the A allele is associated with shorter telomere length. This is in congruence with observations made in IPF, cardiovascular disease, and male infertility, where patients have been shown to have relatively short leukocyte telomeres (126–128). This SNP could, therefore, be part of the genetic background that increases susceptibility to these diseases in combination with other cellular and environmental factors that might cause increased cellular turnover (129). On the other hand, the C allele, which is associated with most cancer types, is associated with longer telomere length in health (42, 101). How the rs2736100 SNP influences telomere length is presently not understood. The SNP is located in intron 2 in TERT and was suggested to influence telomerase activity or to be in strong linkage with a functional variant in TERT (83, 101).
The dual associations of the SNP in this study would support the hypothesis that telomere maintenance is at an intersection between cancer- and premature-aging (130, 131). Cancer and aging share many molecular pathways, including telomere maintenance pathways. And while aging is associated with a progressive decrease in telomere length, cancer is characterized by immortalization of the cell often through telomerase activation (21, 132). Short telomeres accelerate aging through cell senescence, but long telomeres postpone senescence, which in turn, facilitates survival of cells with acquired oncogenic DNA alterations and thereby promotes tumorigenesis (35, 37, 38). The meta-analysis of cancer studies showed a pooled positive correlation with the TERT allele that is known to cause longer telomeres. This suggests that the majority of patients develop cancer due to a.o. the presence of long telomeres in tumor initiating cells. On the other hand, it is also well understood that critically short telomeres lead to chromosomal instability, which can cause tumorigenesis (32, 33, 36). Although the results of the meta-analysis suggest this if not the cause in the majority of cases, four cancer studies were included that originally reported an association with the TERT allele for short telomeres. For colorectal cancer there is general agreement that short telomere length increases tumor initiation by causing chromosomal instability (133). This would account for the association of colorectal cancer with the A allele of rs2736100 that is also associated with short telomere length (76). Testicular cancer is also associated with the A allele (109). Telomerase activity is restricted in most tissues, one exception being germ cells (21). It is assumed that in highly proliferative tissues, a genetic factor decreasing telomerase activity may cause chromosomal instability leading to cancer.
Most non-cancer diseases showed an association with the A allele of rs2736100 SNP. Regarding telomere biology, disease susceptibility for pulmonary fibrosis is opposite to that of lung cancer, while both diseases are highly associated with smoking behavior. In case of short telomeres, smoking may cause increased senescence with subsequent pulmonary fibrosis, while in case of long telomeres; smoking may cause DNA damage in cells with sustained proliferative capacity. For coronary artery disease no significant allelic association has been found for rs2736100. But reports have shown a significant effect of this variant when analyzed in combination with other risk loci or when analyzed in a dominant model (41, 58, 62). However, conflicting data were found for the direction of the association, which could have been due to the ethnic background of the population. Codd et al. reported an association between coronary artery disease and the A allele in a Caucasian cohort. Both Feng et al. and Ding et al. study Asian populations found no association or an association with the C allele (58, 62).
A limitation is that although an exhaustive literature search was performed, studies could have been overlooked. Some studies were excluded because of missing genotype data and some described an association without reporting the associated allele. Furthermore, it should be emphasized that the pathogenesis for most of the diseases mentioned in this study is not fully known and is suggested to be complex, involving both genetic and environmental factors. Another limitation is the discrepancy in the number of studies found for cancer vs non-cancer. This results in a strong influence of interstitial lung disease on the pooled OR. Finally, (publication) bias was evaluated for both the cancer and non-cancer study (Figure 5). For both, meta-analyses asymmetry was minor and the corresponding bias introduced by study selection and other sources is, therefore, also considered to be of minor influence on the pooled result.
The ambiguous effects of telomere maintenance do pose a great challenge for the development of therapeutic agents, for instance putative anti-aging therapeutics. Therapeutic agents aimed at increasing telomere length should be used with caution (25, 134). Telomerase activating agents used in the context of degenerative or aging-related diseases could facilitate tumorigenesis or lead to proliferation of untargeted tissues (131, 135). On the other hand, in relation to cancer, telomerase inhibiting agents are attractive candidates, but could lead to accelerated tissue degeneration or negatively affect stem cell function and immune response as this requires increased telomerase activity to sustain a high level of cell proliferation (135, 136). To develop disease-specific treatment, future research should be aimed at further understanding of optimal telomere length per specific tissues during life, and at the ability to target specific cell-types or tissues very accurately.
Conclusion
Meta-analyses showed that the TERT SNP rs2736100 C allele is positively associated with multiple cancerous diseases, while the A allele is positively associated with predisposition to non-cancerous (degenerative) diseases. Because the SNP is known for its influence on telomere length, this result illustrates that optimal telomere maintenance balances between increasing the risk for cancer or for non-cancerous diseases. This underlines the caution that should be taken when developing therapies that influence telomere length.
Author Contributions
RS and CM contributed to the study concept and design, data analysis and interpretation, writing of the manuscript and final approval; had full access to all the data in the study and takes responsibility for the integrity of the data and the accuracy of the data analysis. MO and JG contributed to the study concept and design, writing of the manuscript and final approval; had full access to all the data in the study and takes responsibility for the integrity of the data and the accuracy of the data analysis.
Conflict of Interest Statement
The authors declare that the research was conducted in the absence of any commercial or financial relationships that could be construed as a potential conflict of interest.
Footnotes
References
1. Armanios M, Blackburn EH. The telomere syndromes. Nat Rev Genet (2012) 13:693–704. doi:10.1038/nrg3246
2. Calado RT, Young NS. Telomere diseases. N Engl J Med (2009) 361:2353–65. doi:10.1056/NEJMra0903373
3. Carroll KA, Ly H. Telomere dysfunction in human diseases: the long and short of it! Int J Clin Exp Pathol (2009) 2:528–43.
4. Kong CM, Lee XW, Wang X. Telomere shortening in human diseases. FEBS J (2013) 280:3180–93. doi:10.1111/febs.12326
5. Barrett JH, Iles MM, Dunning AM, Pooley KA. Telomere length and common disease: study design and analytical challenges. Hum Genet (2015) 134:679–89. doi:10.1007/s00439-015-1563-4
6. Savage SA, Bertuch AA. The genetics and clinical manifestations of telomere biology disorders. Genet Med (2010) 12:753–64. doi:10.1097/GIM.0b013e3181f415b5
7. Trudeau MA, Wong JMY. Genetic variations in telomere maintenance, with implications on tissue renewal capacity and chronic disease pathologies. Curr Pharmacogenomics Person Med (2010) 8:7–24. doi:10.2174/1875692111008010007
8. Autexier C, Lue NF. The structure and function of telomerase reverse transcriptase. Annu Rev Biochem (2006) 75:493–517. doi:10.1146/annurev.biochem.75.103004.142412
9. de Lange T. Shelterin: the protein complex that shapes and safeguards human telomeres. Genes Dev (2005) 19:2100–10. doi:10.1101/gad.1346005
10. Diotti R, Loayza D. Shelterin complex and associated factors at human telomeres. Nucleus (2011) 2:119–35. doi:10.4161/nucl.2.2.15135
11. Allsopp RC, Vaziri H, Patterson C, Goldstein S, Younglai EV, Futcher AB, et al. Telomere length predicts replicative capacity of human fibroblasts. Proc Natl Acad Sci USA (1992) 89:10114–8. doi:10.1073/pnas.89.21.10114
12. Liu L, Lai S, Andrews LG, Tollefsbol TO. Genetic and epigenetic modulation of telomerase activity in development and disease. Gene (2004) 340:1–10. doi:10.1016/j.gene.2004.06.011
13. Wyatt HDM, West SC, Beattie TL. InTERTpreting telomerase structure and function. Nucleic Acids Res (2010) 38:5609–22. doi:10.1093/nar/gkq370
14. Armanios MY, Chen JJ-L, Cogan JD, Alder JK, Ingersoll RG, Markin C, et al. Telomerase mutations in families with idiopathic pulmonary fibrosis. N Engl J Med (2007) 356:1317–26. doi:10.1056/NEJMoa066157
15. Knight SW, Heiss NS, Vulliamy TJ, Greschner S, Stavrides G, Pai GS, et al. X-linked dyskeratosis congenita is predominantly caused by missense mutations in the DKC1 gene. Am J Hum Genet (1999) 65:50–8. doi:10.1086/302446
16. Yamaguchi H, Calado RT, Ly H, Kajigaya S, Baerlocher GM, Chanock SJ, et al. Mutations in TERT, the gene for telomerase reverse transcriptase, in aplastic anemia. N Engl J Med (2005) 352:1413–24. doi:10.1056/NEJMoa042980
17. Armanios M. Telomerase and idiopathic pulmonary fibrosis. Mutat Res (2012) 730:52–8. doi:10.1016/j.mrfmmm.2011.10.013
18. Huang FW, Hodis E, Xu MJ, Kryukov GV, Chin L, Garraway LA. Highly recurrent TERT promoter mutations in human melanoma. Science (2013) 339:957–9. doi:10.1126/science.1229259
19. Liu X, Bishop J, Shan Y, Pai S, Liu D, Murugan AK, et al. Highly prevalent TERT promoter mutations in aggressive thyroid cancers. Endocr Relat Cancer (2013) 20:603–10. doi:10.1530/ERC-13-0210
20. Vinagre J, Pinto V, Celestino R, Reis M, Populo H, Boaventura P, et al. Telomerase promoter mutations in cancer: an emerging molecular biomarker? Virchows Arch (2014) 465:119–33. doi:10.1007/s00428-014-1608-4
21. Kim NW, Piatyszek MA, Prowse KR, Harley CB, West MD, Ho PL, et al. Specific association of human telomerase activity with immortal cells and cancer. Science (1994) 266:2011–5. doi:10.1126/science.7605428
22. Ding Z, Wu C-J, Jaskelioff M, Ivanova E, Kost-Alimova M, Protopopov A, et al. Telomerase reactivation following telomere dysfunction yields murine prostate tumors with bone metastases. Cell (2012) 148:896–907. doi:10.1016/j.cell.2012.01.039
23. DePinho RA, Polyak K. Cancer chromosomes in crisis. Nat Genet (2004) 36:932–4. doi:10.1038/ng0904-932
24. Stanley SE, Armanios M. The short and long telomere syndromes: paired paradigms for molecular medicine. Curr Opin Genet Dev (2015) 33:1–9. doi:10.1016/j.gde.2015.06.004
25. Xu L, Li S, Stohr BA. The role of telomere biology in cancer. Annu Rev Pathol (2013) 8:49–78. doi:10.1146/annurev-pathol-020712-164030
26. Cosme-Blanco W, Shen M-F, Lazar AJF, Pathak S, Lozano G, Multani AS, et al. Telomere dysfunction suppresses spontaneous tumorigenesis in vivo by initiating p53-dependent cellular senescence. EMBO Rep (2007) 8:497–503. doi:10.1038/sj.embor.7400937
27. Greenberg RA, Chin L, Femino A, Lee KH, Gottlieb GJ, Singer RH, et al. Short dysfunctional telomeres impair tumorigenesis in the INK4a(delta2/3) cancer-prone mouse. Cell (1999) 97:515–25. doi:10.1016/S0092-8674(00)80761-8
28. Blackburn EH. Switching and signaling at the telomere. Cell (2001) 106:661–73. doi:10.1016/S0092-8674(01)00492-5
29. Hu J, Hwang SS, Liesa M, Gan B, Sahin E, Jaskelioff M, et al. Antitelomerase therapy provokes ALT and mitochondrial adaptive mechanisms in cancer. Cell (2012) 148:651–63. doi:10.1016/j.cell.2011.12.028
30. McClintock B. The stability of broken ends of chromosomes in Zea mays. Genetics (1941) 26:234–82.
31. Palm W, de Lange T. How shelterin protects mammalian telomeres. Annu Rev Genet (2008) 42:301–34. doi:10.1146/annurev.genet.41.110306.130350
32. Gu J, Chen M, Shete S, Amos CI, Kamat A, Ye Y, et al. A genome-wide association study identifies a locus on chromosome 14q21 as a predictor of leukocyte telomere length and as a marker of susceptibility for bladder cancer. Cancer Prev Res (Phila) (2011) 4:514–21. doi:10.1158/1940-6207.CAPR-11-0063
33. Ma H, Zhou Z, Wei S, Liu Z, Pooley KA, Dunning AM, et al. Shortened telomere length is associated with increased risk of cancer: a meta-analysis. PLoS One (2011) 6:e20466. doi:10.1371/journal.pone.0020466
34. Nan H, Du M, De Vivo I, Manson JE, Liu S, McTiernan A, et al. Shorter telomeres associate with a reduced risk of melanoma development. Cancer Res (2011) 71:6758–63. doi:10.1158/0008-5472.CAN-11-1988
35. Shen M, Cawthon R, Rothman N, Weinstein SJ, Virtamo J, Hosgood HD III, et al. A prospective study of telomere length measured by monochrome multiplex quantitative PCR and risk of lung cancer. Lung Cancer (2011) 73:133–7. doi:10.1016/j.lungcan.2010.11.009
36. Willeit P, Willeit J, Kloss-Brandstatter A, Kronenberg F, Kiechl S. Fifteen-year follow-up of association between telomere length and incident cancer and cancer mortality. JAMA (2011) 306:42–4. doi:10.1001/jama.2011.901
37. Bull CF, Mayrhofer G, O’Callaghan NJ, Au AY, Pickett HA, Low GKM, et al. Folate deficiency induces dysfunctional long and short telomeres; both states are associated with hypomethylation and DNA damage in human WIL2-NS cells. Cancer Prev Res (Phila) (2014) 7:128–38. doi:10.1158/1940-6207.CAPR-13-0264
38. Halaschek-Wiener J, Vulto I, Fornika D, Collins J, Connors JM, Le ND, et al. Reduced telomere length variation in healthy oldest old. Mech Ageing Dev (2008) 129:638–41. doi:10.1016/j.mad.2008.07.004
39. Mushiroda T, Wattanapokayakit S, Takahashi A, Nukiwa T, Kudoh S, Ogura T, et al. A genome-wide association study identifies an association of a common variant in TERT with susceptibility to idiopathic pulmonary fibrosis. J Med Genet (2008) 45:654–6. doi:10.1136/jmg.2008.057356
40. McKay JD, Hung RJ, Gaborieau V, Boffetta P, Chabrier A, Byrnes G, et al. Lung cancer susceptibility locus at 5p15.33. Nat Genet (2008) 40:1404–6. doi:10.1038/ng.254
41. Codd V, Nelson CP, Albrecht E, Mangino M, Deelen J, Buxton JL, et al. Identification of seven loci affecting mean telomere length and their association with disease. Nat Genet (2013) 45:e1–2. doi:10.1038/ng.2528
42. Iles MM, Bishop DT, Taylor JC, Hayward NK, Brossard M, Cust AE, et al. The effect on melanoma risk of genes previously associated with telomere length. J Natl Cancer Inst (2014) 106:dju267. doi:10.1093/jnci/dju267
43. Doi SAR, Barendregt JJ, Khan S, Thalib L, Williams GM. Advances in the meta-analysis of heterogeneous clinical trials I: the inverse variance heterogeneity model. Contemp Clin Trials (2015) 45:130–8. doi:10.1016/j.cct.2015.05.009
44. Adel Fahmideh M, Lavebratt C, Schuz J, Roosli M, Tynes T, Grotzer MA, et al. CCDC26, CDKN2BAS, RTEL1 and TERT polymorphisms in pediatric brain tumor susceptibility. Carcinogenesis (2015) 36:876–82. doi:10.1093/carcin/bgv074
45. Ahn M-J, Won H-H, Lee J, Lee S-T, Sun J-M, Park YH, et al. The 18p11.22 locus is associated with never smoker non-small cell lung cancer susceptibility in Korean populations. Hum Genet (2012) 131:365–72. doi:10.1007/s00439-011-1080-z
46. Amos CI, Wu X, Broderick P, Gorlov IP, Gu J, Eisen T, et al. Genome-wide association scan of tag SNPs identifies a susceptibility locus for lung cancer at 15q25.1. Nat Genet (2008) 40:616–22. doi:10.1038/ng.109
47. Amundadottir L, Kraft P, Stolzenberg-Solomon RZ, Fuchs CS, Petersen GM, Arslan AA, et al. Genome-wide association study identifies variants in the ABO locus associated with susceptibility to pancreatic cancer. Nat Genet (2009) 41:986–90. doi:10.1038/ng.429
48. Bae EY, Lee SY, Kang BK, Lee EJ, Choi YY, Kang H-G, et al. Replication of results of genome-wide association studies on lung cancer susceptibility loci in a Korean population. Respirology (2012) 17:699–706. doi:10.1111/j.1440-1843.2012.02165.x
49. Brenner DR, Brennan P, Boffetta P, Amos CI, Spitz MR, Chen C, et al. Hierarchical modeling identifies novel lung cancer susceptibility variants in inflammation pathways among 10,140 cases and 11,012 controls. Hum Genet (2013) 132:579–89. doi:10.1007/s00439-013-1270-y
50. Campa D, Rizzato C, Stolzenberg-Solomon R, Pacetti P, Vodicka P, Cleary SP, et al. TERT gene harbors multiple variants associated with pancreatic cancer susceptibility. Int J Cancer (2015) 137:2175–83. doi:10.1002/ijc.29590
51. Chen H, Chen Y, Zhao Y, Fan W, Zhou K, Liu Y, et al. Association of sequence variants on chromosomes 20, 11, and 5 (20q13.33, 11q23.3, and 5p15.33) with glioma susceptibility in a Chinese population. Am J Epidemiol (2011) 173:915–22. doi:10.1093/aje/kwq457
52. Chen XF, Cai S, Chen QG, Ni ZH, Tang JH, Xu DW, et al. Multiple variants of TERT and CLPTM1L constitute risk factors for lung adenocarcinoma. Genet Mol Res (2012) 11:370–8. doi:10.4238/2012.February.16.2
53. Cheng THT, Thompson D, Painter J, O’Mara T, Gorman M, Martin L, et al. Meta-analysis of genome-wide association studies identifies common susceptibility polymorphisms for colorectal and endometrial cancer near SH2B3 and TSHZ1. Sci Rep (2015) 5:17369. doi:10.1038/srep17369
54. Choi BJ, Yoon JH, Kim O, Choi WS, Nam SW, Lee JY, et al. Influence of the hTERT rs2736100 polymorphism on telomere length in gastric cancer. World J Gastroenterol (2015) 21:9328–36. doi:10.3748/wjg.v21.i31.9328
55. Clifford RJ, Zhang J, Meerzaman DM, Lyu M-S, Hu Y, Cultraro CM, et al. Genetic variations at loci involved in the immune response are risk factors for hepatocellular carcinoma. Hepatology (2010) 52:2034–43. doi:10.1002/hep.23943
56. de Martino M, Taus C, Lucca I, Hofbauer SL, Haitel A, Shariat SF, et al. Association of human telomerase reverse transcriptase gene polymorphisms, serum levels, and telomere length with renal cell carcinoma risk and pathology. Mol Carcinog (2015) 55(10):1458–66. doi:10.1002/mc.22388
57. Di Stefano AL, Enciso-Mora V, Marie Y, Desestret V, Labussiere M, Boisselier B, et al. Association between glioma susceptibility loci and tumour pathology defines specific molecular etiologies. Neuro Oncol (2013) 15:542–7. doi:10.1093/neuonc/nos284
58. Ding H, Yan F, Zhou L-L, Ji X-H, Gu X-N, Tang Z-W, et al. Association between previously identified loci affecting telomere length and coronary heart disease (CHD) in Han Chinese population. Clin Interv Aging (2014) 9:857–61. doi:10.2147/CIA.S60760
59. Du J, Zhu X, Xie C, Dai N, Gu Y, Zhu M, et al. Telomere length, genetic variants and gastric cancer risk in a Chinese population. Carcinogenesis (2015) 36:963–70. doi:10.1093/carcin/bgv075
60. Egan KM, Thompson RC, Nabors LB, Olson JJ, Brat DJ, Larocca RV, et al. Cancer susceptibility variants and the risk of adult glioma in a US case-control study. J Neurooncol (2011) 104:535–42. doi:10.1007/s11060-010-0506-0
61. Falchi M, Bataille V, Hayward NK, Duffy DL, Bishop JAN, Pastinen T, et al. Genome-wide association study identifies variants at 9p21 and 22q13 associated with development of cutaneous nevi. Nat Genet (2009) 41:915–9. doi:10.1038/ng.410
62. Feng L, Nian S-Y, Zhang J. The GG genotype of telomerase reverse transcriptase at genetic locus rs2736100 is associated with human atherosclerosis risk in the Han Chinese population. PLoS One (2014) 9:e85719. doi:10.1371/journal.pone.0085719
63. Fingerlin TE, Murphy E, Zhang W, Peljto AL, Brown KK, Steele MP, et al. Genome-wide association study identifies multiple susceptibility loci for pulmonary fibrosis. Nat Genet (2013) 45:613–20. doi:10.1038/ng.2609
64. Gago-Dominguez M, Jiang X, Conti DV, Castelao JE, Stern MC, Cortessis VK, et al. Genetic variations on chromosomes 5p15 and 15q25 and bladder cancer risk: findings from the Los Angeles-Shanghai bladder case-control study. Carcinogenesis (2011) 32:197–202. doi:10.1093/carcin/bgq233
65. Gu Y, Yu C, Miao L, Wang L, Xu C, Xue W, et al. Telomere length, genetic variants and risk of squamous cell carcinoma of the head and neck in Southeast Chinese. Sci Rep (2016) 6:20675. doi:10.1038/srep20675
66. Hofer P, Baierl A, Bernhart K, Leeb G, Mach K, Micksche M, et al. Association of genetic variants of human telomerase with colorectal polyps and colorectal cancer risk. Mol Carcinog (2012) 51:E176–82. doi:10.1002/mc.21911
67. Hsiung CA, Lan Q, Hong YC, Chen C-J, Dean Hosgood H, Chang I-S, et al. The 5p15.33 locus is associated with risk of lung adenocarcinoma in never-smoking females in Asia. PLoS Genet (2010) 6:e1001051. doi:10.1371/journal.pgen.1001051
68. Hu Z, Wu C, Shi Y, Guo H, Zhao X, Yin Z, et al. A genome-wide association study identifies two new lung cancer susceptibility loci at 13q12.12 and 22q12.2 in Han Chinese. Nat Genet (2011) 43:792–6. doi:10.1038/ng.875
69. Hunter DJ, Kraft P, Jacobs KB, Cox DG, Yeager M, Hankinson SE, et al. A genome-wide association study identifies alleles in FGFR2 associated with risk of sporadic postmenopausal breast cancer. Nat Genet (2007) 39:870–4. doi:10.1038/ng2075
70. Ito H, McKay JD, Hosono S, Hida T, Yatabe Y, Mitsudomi T, et al. Association between a genome-wide association study-identified locus and the risk of lung cancer in Japanese population. J Thorac Oncol (2012) 7:790–8. doi:10.1097/JTO.0b013e3182475028
71. Jäger R, Harutyunyan AS, Rumi E, Pietra D, Berg T, Olcaydu D, et al. Common germline variation at the TERT locus contributes to familial clustering of myeloproliferative neoplasms. Am J Hematol (2014) 89(12):1107–10. doi:10.1002/ajh.23842
72. Jannuzzi AT, Karaman E, Oztas E, Yanar HT, Ozhan G. Telomerase reverse transcriptase (TERT) gene variations and susceptibility of colorectal cancer. Genet Test Mol Biomarkers (2015) 19:692–7. doi:10.1089/gtmb.2015.0150
73. Jaworowska E, Trubicka J, Lener MR, Masojc B, Zlowocka-Perlowska E, McKay JD, et al. Smoking related cancers and loci at chromosomes 15q25, 5p15, 6p22.1 and 6p21.33 in the Polish population. PLoS One (2011) 6:e25057. doi:10.1371/journal.pone.0025057
74. Jin EC, Kang H-G, Jin SJ, Yi YC, Min JK, Jong SK, et al. Polymorphisms in telomere maintenance genes and risk of lung cancer. Cancer Epidemiol Biomarkers Prev (2009) 18:2773–81. doi:10.1158/1055-9965.EPI-09-0323
75. Kanetsky PA, Mitra N, Vardhanabhuti S, Vaughn DJ, Li M, Ciosek SL, et al. A second independent locus within DMRT1 is associated with testicular germ cell tumor susceptibility. Hum Mol Genet (2011) 20:3109–17. doi:10.1093/hmg/ddr207
76. Kinnersley B, Migliorini G, Broderick P, Whiffin N, Dobbins SE, Casey G, et al. The TERT variant rs2736100 is associated with colorectal cancer risk. Br J Cancer (2012) 107:1001–8. doi:10.1038/bjc.2012.329
77. Kohno T, Kunitoh H, Mimaki S, Shiraishi K, Kuchiba A, Yamamoto S, et al. Contribution of the TP53, OGG1, CHRNA3, and HLA-DQA1 genes to the risk for lung squamous cell carcinoma. J Thorac Oncol (2011) 6:813–7. doi:10.1097/JTO.0b013e3181ee80ef
78. Krahling T, Balassa K, Kiss KP, Bors A, Batai A, Halm G, et al. Co-occurrence of myeloproliferative neoplasms and solid tumors is attributed to a synergism between cytoreductive therapy and the common TERT Polymorphism rs2736100. Cancer Epidemiol Biomarkers Prev (2016) 25:98–104. doi:10.1158/1055-9965.EPI-15-0805
79. Labussière M, Di Stefano AL, Gleize V, Boisselier B, Giry M, Mangesius S, et al. TERT promoter mutations in gliomas, genetic associations and clinico-pathological correlations. Br J Cancer (2014) 111:2024–32. doi:10.1038/bjc.2014.538
80. Lachance DH, Yang P, Johnson DR, Decker PA, Kollmeyer TM, McCoy LS, et al. Associations of high-grade glioma with glioma risk alleles and histories of allergy and smoking. Am J Epidemiol (2011) 174:574–81. doi:10.1093/aje/kwr124
81. Lan Q, Hsiung CA, Matsuo K, Hong Y-C, Seow A, Wang Z, et al. Genome-wide association analysis identifies new lung cancer susceptibility loci in never-smoking women in Asia. Nat Genet (2012) 44:1330–5. doi:10.1038/ng.2456
82. Lan Q, Cawthon R, Gao Y, Hu W, Hosgood IIIHD, Barone-Adesi F, et al. Longer telomere length in peripheral white blood cells is associated with risk of lung cancer and the rs2736100 (CLPTM1L-TERT) polymorphism in a prospective cohort study among women in China. PLoS ONE (2013) 8(3):e59230. doi:10.1371/journal.pone.0059230
83. Landi MT, Chatterjee N, Yu K, Goldin LR, Goldstein AM, Rotunno M, et al. A genome-wide association study of lung cancer identifies a region of chromosome 5p15 associated with risk for adenocarcinoma. Am J Hum Genet (2009) 85:679–91. doi:10.1016/j.ajhg.2009.09.012
84. Lascorz J, Forsti A, Chen B, Buch S, Steinke V, Rahner N, et al. Genome-wide association study for colorectal cancer identifies risk polymorphisms in German familial cases and implicates MAPK signalling pathways in disease susceptibility. Carcinogenesis (2010) 31:1612–9. doi:10.1093/carcin/bgq146
85. Li H, Yang L, Zhao X, Wang J, Qian J, Chen H, et al. Prediction of lung cancer risk in a Chinese population using a multifactorial genetic model. BMC Med Genet (2012) 13:118. doi:10.1186/1471-2350-13-118
86. Liu SG, Ma L, Cen QH, Huang JS, Zhang JX, Zhang JJ. Association of genetic polymorphisms in TERT-CLPTM1L with lung cancer in a Chinese population. Genet Mol Res (2015) 14:4469–76. doi:10.4238/2015.May.4.4
87. Ma Z, Hu Q, Chen Z, Tao S, Macnamara L, Kim S-T, et al. Systematic evaluation of bladder cancer risk-associated single-nucleotide polymorphisms in a Chinese population. Mol Carcinog (2013) 52:916–21. doi:10.1002/mc.21932
88. Machiela MJ, Hsiung CA, Shu X-O, Seow WJ, Wang Z, Matsuo K, et al. Genetic variants associated with longer telomere length are associated with increased lung cancer risk among never-smoking women in Asia: a report from the female lung cancer consortium in Asia. Int J Cancer (2015) 137:311–9. doi:10.1002/ijc.29393
89. Miki D, Kubo M, Takahashi A, Yoon K-A, Kim J, Lee GK, et al. Variation in TP63 is associated with lung adenocarcinoma susceptibility in Japanese and Korean populations. Nat Genet (2010) 42:893–6. doi:10.1038/ng.667
90. Mosrati MA, Malmstrom A, Lysiak M, Krysztofiak A, Hallbeck M, Milos P, et al. TERT promoter mutations and polymorphisms as prognostic factors in primary glioblastoma. Oncotarget (2015) 6:16663–73. doi:10.18632/oncotarget.4389
91. Mosrati MA, Willander K, Falk IJ, Hermanson M, Hoglund M, Stockelberg D, et al. Association between TERT promoter polymorphisms and acute myeloid leukemia risk and prognosis. Oncotarget (2015) 6:25109–20. doi:10.18632/oncotarget.4668
92. Myneni AA, Chang S-C, Niu R, Liu L, Ochs-Balcom HM, Li Y, et al. Genetic polymorphisms of TERT and CLPTM1L and risk of lung cancer-A case-control study in a Chinese population. Lung Cancer (2013) 80:131–7. doi:10.1016/j.lungcan.2013.01.021
93. Pande M, Spitz MR, Wu X, Gorlov IP, Chen WV, Amos CI. Novel genetic variants in the chromosome 5p15.33 region associate with lung cancer risk. Carcinogenesis (2011) 32:1493–9. doi:10.1093/carcin/bgr136
94. Pellatt AJ, Wolff RK, Torres-Mejia G, John EM, Herrick JS, Lundgreen A, et al. Telomere length, telomere-related genes, and breast cancer risk: The breast cancer health disparities study. Genes Chromosomes Cancer (2013) 52:595–609. doi:10.1002/gcc.22056
95. Petersen GM, Amundadottir L, Fuchs CS, Kraft P, Stolzenberg-Solomon RZ, Jacobs KB, et al. A genome-wide association study identifies pancreatic cancer susceptibility loci on chromosomes 13q22.1, 1q32.1 and 5p15.33. Nat Genet (2010) 42:224–8. doi:10.1038/ng.522
96. Prescott J, McGrath M, Lee I-M, Buring JE, De Vivo I. Telomere length and genetic analyses in population-based studies of endometrial cancer risk. Cancer (2010) 116:4275–82. doi:10.1002/cncr.25328
97. Rajaraman P, Melin BS, Wang Z, McKean-Cowdin R, Michaud DS, Wang SS, et al. Genome-wide association study of glioma and meta-analysis. Hum Genet (2012) 131:1877–88. doi:10.1007/s00439-012-1212-0
98. Safaeian M, Rajaraman P, Hartge P, Yeager M, Linet M, Butler MA, et al. Joint effects between five identified risk variants, allergy, and autoimmune conditions on glioma risk. Cancer Causes Control (2013) 24:1885–91. doi:10.1007/s10552-013-0244-7
99. Schoemaker MJ, Robertson L, Wigertz A, Jones ME, Hosking FJ, Feychting M, et al. Interaction between 5 genetic variants and allergy in glioma risk. Am J Epidemiol (2010) 171:1165–73. doi:10.1093/aje/kwq075
100. Shadrina AS, Boyarskikh UA, Oskina NA, Sinkina TV, Lazarev AF, Petrova VD, et al. TERT polymorphisms rs2853669 and rs7726159 influence on prostate cancer risk in Russian population. Tumour Biol (2015) 36:841–7. doi:10.1007/s13277-014-2688-0
101. Sheng X, Tong N, Tao G, Luo D, Wang M, Fang Y, et al. TERT polymorphisms modify the risk of acute lymphoblastic leukemia in Chinese children. Carcinogenesis (2013) 34:228–35. doi:10.1093/carcin/bgs325
102. Shete S, Hosking FJ, Robertson LB, Dobbins SE, Sanson M, Malmer B, et al. Genome-wide association study identifies five susceptibility loci for glioma. Nat Genet (2009) 41:899–904. doi:10.1038/ng.407
103. Shiraishi K, Kunitoh H, Daigo Y, Takahashi A, Goto K, Sakamoto H, et al. A genome-wide association study identifies two new susceptibility loci for lung adenocarcinoma in the Japanese population. Nat Genet (2012) 44:900–3. doi:10.1038/ng.2353
104. Spitz MR, Amos CI, Land S, Wu X, Dong Q, Wenzlaff AS, et al. Role of selected genetic variants in lung cancer risk in African Americans. J Thorac Oncol (2013) 8:391–7. doi:10.1097/JTO.0b013e318283da29
105. Tapper W, Jones AV, Kralovics R, Harutyunyan AS, Zoi K, Leung W, et al. Genetic variation at MECOM, TERT, JAK2 and HBS1L-MYB predisposes to myeloproliferative neoplasms. Nat Commun (2015) 6.
106. Thorgeirsson TE, Geller F, Sulem P, Rafnar T, Wiste A, Magnusson KP, et al. A variant associated with nicotine dependence, lung cancer and peripheral arterial disease. Nature (2008) 452:638–42. doi:10.1038/nature06846
107. Truong T, Hung RJ, Amos CI, Wu X, Bickeboller H, Rosenberger A, et al. Replication of lung cancer susceptibility loci at chromosomes 15q25, 5p15, and 6p21: a pooled analysis from the International Lung Cancer Consortium. J Natl Cancer Inst (2010) 102:959–71. doi:10.1093/jnci/djq178
108. Tseng T-S, Park JY, Zabaleta J, Moody-Thomas S, Sothern MS, Chen T, et al. Role of nicotine dependence on the relationship between variants in the nicotinic receptor genes and risk of lung adenocarcinoma. PLoS One (2014) 9:e107268. doi:10.1371/journal.pone.0107268
109. Turnbull C, Rapley EA, Seal S, Pernet D, Renwick A, Hughes D, et al. Variants near DMRT1, TERT and ATF7IP are associated with testicular germ cell cancer. Nat Genet (2010) 42:604–7. doi:10.1038/ng.607
110. Walsh KM, Anderson E, Hansen HM, Decker PA, Kosel ML, Kollmeyer T, et al. Analysis of 60 reported glioma risk SNPs replicates published GWAS findings but fails to replicate associations from published candidate-gene studies. Genet Epidemiol (2013) 37:222–8. doi:10.1002/gepi.21707
111. Walsh KM, Codd V, Smirnov IV, Rice T, Decker PA, Hansen HM, et al. Variants near TERT and TERC influencing telomere length are associated with high-grade glioma risk. Nat Genet (2014) 46:731–5. doi:10.1038/ng.3004
112. Wang Y, Broderick P, Matakidou A, Eisen T, Houlston RS. Role of 5p15.33 (TERT-CLPTM1L), 6p21.33 and 15q25.1 (CHRNA5-CHRNA3) variation and lung cancer risk in never-smokers. Carcinogenesis (2010) 31:234–8. doi:10.1093/carcin/bgp287
113. Wang S, Wu J, Hu L, Ding C, Kan Y, Shen Y, et al. Common genetic variants in TERT contribute to risk of cervical cancer in a Chinese population. Mol Carcinog (2012) 51:E118–22. doi:10.1002/mc.21872
114. Wang F, Fu P, Pang Y, Liu C, Shao Z, Zhu J, et al. TERT rs2736100T/G polymorphism upregulates interleukin 6 expression in non-small cell lung cancer especially in adenocarcinoma. Tumour Biol (2014) 35:4667–72. doi:10.1007/s13277-014-1611-z
115. Wei R, Li C, Zhang M, Jones-Hall YL, Myers JL, Noth I, et al. Association between MUC5B and TERT polymorphisms and different interstitial lung disease phenotypes. Transl Res (2014) 163:494–502. doi:10.1016/j.trsl.2013.12.006
116. Wei R, DeVilbiss FT, Liu W. Genetic polymorphism, telomere biology and non-small lung cancer risk. J Genet Genomics (2015) 42:549–61. doi:10.1016/j.jgg.2015.08.005
117. Wei YB, Martinsson L, Liu JJ, Forsell Y, Schalling M, Backlund L, et al. hTERT genetic variation in depression. J Affect Disord (2016) 189:62–9. doi:10.1016/j.jad.2015.09.025
118. Wrensch M, Jenkins RB, Chang JS, Yeh R-F, Xiao Y, Decker PA, et al. Variants in the CDKN2B and RTEL1 regions are associated with high-grade glioma susceptibility. Nat Genet (2009) 41:905–8. doi:10.1038/ng.408
119. Yan L, Wu S, Zhang S, Ji G, Gu A. Genetic variants in telomerase reverse transcriptase (TERT) and telomerase-associated protein 1 (TEP1) and the risk of male infertility. Gene (2014) 534:139–43. doi:10.1016/j.gene.2013.11.008
120. Yang G, Li J, Zhang X, Zhao Q, Gong P, Ren B, et al. Eimeria tenella: cloning and characterization of telomerase reverse transcriptase gene. Exp Parasitol (2010) 124:380–5. doi:10.1016/j.exppara.2009.12.004
121. Yin Z, Cui Z, Ren Y, Zhang H, Yan Y, Zhao Y, et al. Genetic polymorphisms of TERT and CLPTM1L, cooking oil fume exposure, and risk of lung cancer: a case-control study in a Chinese non-smoking female population. Med Oncol (2014) 31:114. doi:10.1007/s12032-014-0114-5
122. Yoon K-A, Park JH, Han J, Park S, Lee GK, Han J-Y, et al. A genome-wide association study reveals susceptibility variants for non-small cell lung cancer in the Korean population. Hum Mol Genet (2010) 19:4948–54. doi:10.1093/hmg/ddq421
123. Zhao Z, Li C, Yang L, Zhang X, Zhao X, Song X, et al. Significant association of 5p15.33 (TERT-CLPTM1L genes) with lung cancer in Chinese Han population. Exp Lung Res (2013) 39:91–8. doi:10.3109/01902148.2012.762436
124. Zhou L, Fu G, Wei J, Shi J, Pan W, Ren Y, et al. The identification of two regulatory ESCC susceptibility genetic variants in the. Oncotarget (2016) 7:5495–506. doi:10.18632/oncotarget.6747
125. Haycock PC, Burgess S, Nounu A, Zheng J, Okoli GN, Bowden J, et al. Association between telomere length and risk of cancer and non-neoplastic diseases: a Mendelian Randomization Study. JAMA Oncol (2017) 3:636–51. doi:10.1001/jamaoncol.2016.5945
126. Alder JK, Chen JJ-L, Lancaster L, Danoff S, Su S, Cogan JD, et al. Short telomeres are a risk factor for idiopathic pulmonary fibrosis. Proc Natl Acad Sci USA (2008) 105:13051–6. doi:10.1073/pnas.0804280105
127. Fitzpatrick AL, Kronmal RA, Gardner JP, Psaty BM, Jenny NS, Tracy RP, et al. Leukocyte telomere length and cardiovascular disease in the cardiovascular health study. Am J Epidemiol (2007) 165:14–21. doi:10.1093/aje/kwj346
128. Thilagavathi J, Kumar M, Mishra SS, Venkatesh S, Kumar R, Dada R. Analysis of sperm telomere length in men with idiopathic infertility. Arch Gynecol Obstet (2013) 287:803–7. doi:10.1007/s00404-012-2632-8
129. Armanios M. Telomeres and age-related disease: how telomere biology informs clinical paradigms. J Clin Invest (2013) 123:996–1002. doi:10.1172/JCI66370
130. Bernardes de Jesus B, Blasco MA. Telomerase at the intersection of cancer and aging. Trends Genet (2013) 29:513–20. doi:10.1016/j.tig.2013.06.007
131. Djojosubroto MW, Choi YS, Lee H-W, Rudolph KL. Telomeres and telomerase in aging, regeneration and cancer. Mol Cells (2003) 15:164–75.
132. Aubert G. Telomere dynamics and aging. Prog Mol Biol Transl Sci (2014) 125:89–111. doi:10.1016/B978-0-12-397898-1.00004-9
133. Bertorelle R, Rampazzo E, Pucciarelli S, Nitti D, De Rossi A. Telomeres, telomerase and colorectal cancer. World J Gastroenterol (2014) 20:1940–50. doi:10.3748/wjg.v20.i8.1940
134. Mocellin S, Verdi D, Pooley KA, Landi MT, Egan KM, Baird DM, et al. Telomerase reverse transcriptase locus polymorphisms and cancer risk: a field synopsis and meta-analysis. J Natl Cancer Inst (2012) 104:840–54. doi:10.1093/jnci/djs222
135. Sprouse AA, Steding CE, Herbert B-S. Pharmaceutical regulation of telomerase and its clinical potential. J Cell Mol Med (2012) 16:1–7. doi:10.1111/j.1582-4934.2011.01460.x
Keywords: telomerase, telomere, cancer, single nucleotide polymorphism, degenerative disease
Citation: Snetselaar R, van Oosterhout MFM, Grutters JC and van Moorsel CHM (2018) Telomerase Reverse Transcriptase Polymorphism rs2736100: A Balancing Act between Cancer and Non-Cancer Disease, a Meta-Analysis. Front. Med. 5:41. doi: 10.3389/fmed.2018.00041
Received: 21 December 2017; Accepted: 06 February 2018;
Published: 27 February 2018
Edited by:
Bethany B. Moore, University of Michigan, United StatesReviewed by:
Carlos Flores, Fundación Canaria Rafael Clavijo, SpainYong Huang, University of Chicago, United States
Copyright: © 2018 Snetselaar, van Oosterhout, Grutters and van Moorsel. This is an open-access article distributed under the terms of the Creative Commons Attribution License (CC BY). The use, distribution or reproduction in other forums is permitted, provided the original author(s) and the copyright owner are credited and that the original publication in this journal is cited, in accordance with accepted academic practice. No use, distribution or reproduction is permitted which does not comply with these terms.
*Correspondence: Coline H. M. van Moorsel, c.van.moorsel@antoniusziekenhuis.nl