Multidisciplinary Scientific Cruise to the Northern Mid-Atlantic Ridge and Azores Archipelago
- 1Marine Geology Dv., Geological Survey of Spain IGME, Madrid, Spain
- 2Portuguese Task Group for the Extension of the Continental Shelf EMEPC, Paço de Arcos, Portugal
- 3Instituto Volcanológico de Canarias INVOLCAN, Santa Cruz de Tenerife, Spain
- 4IMAR Instituto do Mar, Universidade dos Açores, Horta, Portugal
- 5Okeanos Research Center, Universidade dos Açores, Horta, Portugal
- 6Hydrographic Institute of the Navy IHM, Cádiz, Spain
- 7Department of Mineralogy and Petrology, Complutense University of Madrid UCM, Madrid, Spain
- 8GeoBioTec, Aveiro University, Aveiro, Portugal
- 9Department of Geobiology, Georg-August University Göttingen, Göttingen, Germany
- 10MARE – Marine and Environmental Sciences Center, Coimbra, Portugal
- 11Institute of Earth Sciences, Department of Geosciences, Évora University, Evora, Portugal
This work presents the preliminary result of the multidisciplinary cruise EXPLOSEA2 surveying the northern Mid-Atlantic Ridge and Azores Archipelago from 46° 30′ N to 38° 30′ N aboard the R/V Sarmiento de Gamboa and ROV Luso over 54 days (June 11 to July 27, 2019). In this cruise report, we detail the geophysical, hydrographic, geological, oceanographic, ecological, and microbiological data acquired and a brief of main findings. The cruise addressed the exploration and comprehensive characterization of venting sites, including the water column, the sediments and rocks that host the hydrothermal activity, and the associated mineralizations, biology, and microbiology. Deep hydrothermal chimneys and massive sulfide deposits (up 3,000 m in depth) within the Moytirra hydrothermal active field were identified on slopes that had not been explored previously. Another striking finding made during the EXPLOSEA2 cruise was the field of carbonate chimneys named the “Magallanes-Elcano” field, a potentially relict ultramafic-hosted hydrothermal site sourced by abiotic methane. This field is related to a serpentinite and gabbro rock outcropping on a dome-shaped massif named the “Iberian Massif.” An outstanding finding of the EXPLOSEA2 survey was the identification of the first garden of soft corals growing after active submarine eruptions were reported in the Azores Archipelago composed by a high density of soft corals the suborder Alcyoniina at the summit and flanks of a recent volcanic cone at 160 m water depth developed during the 1957–1958 eruption of Capelinhos. Several cold-water coral habitats formed by colonial scleractinians (e.g., Lophelia pertusa and Madrepora oculata), coral gardens composed of mixed assemblages of black corals (Leiopathes sp.), and octocorals and dense aggregations of the glass sponge Pheronema carpenteri that may be classified as vulnerable marine ecosystems (VMEs) have been discovered during the EXPLOSEA2 cruise along the northern Mid-Atlantic Ridge. This work reveals the importance of multidisciplinary surveys to the knowledge of deep-sea environments.
Introduction
The high-resolution mapping of the seabed and habitat of the entire North Atlantic Ocean is a challenge that must be overcome to augment our present knowledge of the Atlantic seabed. It is being addressed by the Atlantic Seabed Mapping International Working Group (ASMIWG) as part of the Trilateral Galway Statement agreement between the United States, European Union, and Canada for the Atlantic Ocean Research Alliance (AORA1).
The northern Mid-Atlantic Ridge (MAR) has been recently proposed by the International Seabed Authority (ISBA) as a priority area for the development of a regional environmental management plan (REMP). REMPs have been interpreted as essential tools because they provide regional-specific information that facilitates the decision-making for present exploration and future exploitation of mineral resources in order to ensure the effective protection of the marine environment according to Article 145 of the United Nations Convention on the Law of the Sea (UNCLOS). In preparing the North Atlantic REMP, the procedure should involve the use of an extensive multidisciplinary scientific dataset including information such as high-resolution bathymetry mapping, habitat types, biodiversity, active vents, and distribution of massive sulfide mineralizations as essential tools for ensuring the effective protection of the marine environment.
More than 11 high-temperature vent fields have been confirmed in the axial valley of the MAR between the equator and the Azores Triple Junction at ∼39° N (Interridge Vents Database ver. 3.42; Beaulieu et al., 2013; Baker, 2017). In addition, several deep-water mantle plumes inferred from 3He/4He and CH4 anomalies, reported along the northern MAR between 36° N and 40° N, were investigated along the MAR (Jean-Baptiste et al., 2008). However, between the South Azores Fault at ∼ 38° 20′ N and the Charlie-Gibbs Fracture Zone at 52° 25′ N, only two active hydrothermal vents with associated mineral deposits have been reported: (1) The Moytirra field at ∼ 45° N, a deep-sea, high-temperature vent field with active black smokers discovered on the terraces of the axial valley (Wheeler et al., 2013); and (2) the Luso hydrothermal vent, a shallow low-temperature hydrothermal field, which was recently discovered at the Azores latitude and is associated with the Faial transform fault crossing the MAR3.
The EXPLOSEA2 project4 aims to explore submarine hydrothermal fields and associated mineralizations and geobio-systems along distinct morpho-tectonic settings at the active Bransfield Ridge (Pacific Margin, Antarctica) and slow-spreading ridges at the northern MAR. The objective of the EXPLOSEA2 cruise (Figure 1A) was the exploration and multidisciplinary research of active hydrothermal vents, magmatic activity, and/or potential sources of serpentinization along the northern MAR between 37° 30′ N and 45° N and the Azores Triple Junction between the North American, Eurasian, and Nubian lithospheric plates at 39° N (Figure 1A; Full map and GIS data can be downloaded at http://www.igme.es/explosea/dataset.html).
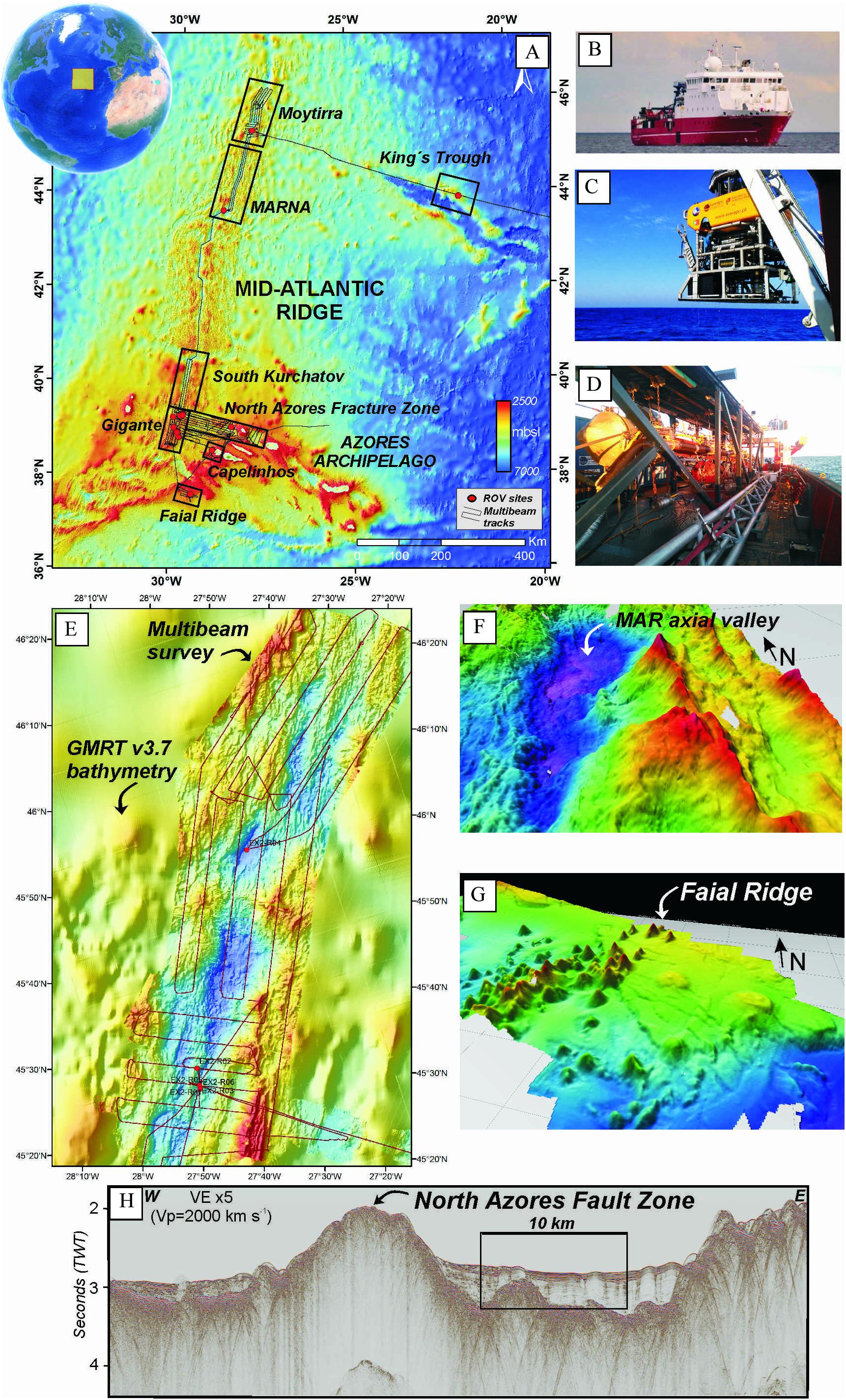
Figure 1. (A) Areas investigated during the EXPLOSEA2 cruise in the northern Mid-Atlantic Ridge; (B) R/V Sarmiento de Gamboa; (C) ROV Luso; (D) Multichannel seismic equipment; (E) Multibeam bathymetry mosaic along the MAR axial valley obtained during EXPLOSEA2 cruise and overlapped to Global Multi-resolution Topography (GMRT) data (Ryan et al., 2009); (F) 3d view of the MAR axial valley in the vicinity of the vicinity of the Moytirra hydrothermal vent site; (G) 3d view of the bathymetry of the Faial Ridge showing numerous volcanic cones; (H) Multichannel seismic profile crossing the North Azores Fault Zone.
The cruise EXPLOSEA2 was developed aboard the R/V Sarmiento de Gamboa (Figure 1B) over 54 days (June 11 to July 27, 2019). The survey explored a total of eight areas of interest (Figure 1A): (A) Kings Through, an aborted ridge to the east of the MAR; four segments of the axial valley: (B1) MAR axial valley, between 46° 30′ N and 45° N including the Moytirra hydrothermal field; (B2) MAR axial valley within the Azores High Seas Marine Protected Area (MARNA) between 45° N and 43° 30′ N; (B3) MAR segment south of Kurchatov Transform Fault, between 40° 30′ N and 39° 30′ N; and (B4) MAR axial valley south of the North Azores Fracture Zone (NAFZ) named Gigante, between 39° 25′ N and 38° 30′ N, and three areas within the Azores Plateau: (C1) the NAFZ, the westernmost edge of the Terceira Rift, the basin west of the Graciosa Island and its connection with the MAR at ∼39° 20′ N, termed the Azores triple junction (Miranda et al., 2014); (C2) the offshore extension of the Capelinhos volcanic area (eruption active in 1957–1958), west of the Faial Island; and (C3) the Faial Ridge, southwest of the Faial Island (∼37° 40′ N), an area named “Fried Eggs” (Dias et al., 2009), characterized by numerous volcanic edifices. The cruise addressed the exploration and comprehensive characterization of venting sites including the water column, the sediments and rocks that host the hydrothermal activity, and the associated mineralizations, biology, and microbiology.
Materials and Methods
Geophysics
The geophysical survey was designed to obtain: (1) full bathymetric and backscatter coverage of the study areas for understanding the morpho-tectonic characteristics of the MAR; (2) multichannel seismic profiles to define the tectonic and sedimentary evolution of the MAR segments and a succession of basins in the WSW–ENE Terceira Rift, the westernmost part of the Eurasian–Nubian plate boundary; (3) chirp sub-bottom profiler data to characterize recent processes of the sub-seafloor; and (4) gravimeter data to characterize the ridge segments and potential hydrothermal systems.
Multibeam Bathymetry
Multibeam bathymetry data (Figures 1E–G) were acquired using an Atlas DS 1 × 1 multibeam echosounder (MBES) operating at a frequency between 14 kHz and 16 kHz. The Atlas MBES, which has an angular coverage of 140 degrees, allows a maximum of 360 beams per ping with an angular distance of 1°. At average water depths of 1,700 m, the real Atlas MBES coverage was four times the water depth. Resolution was 0.2% of the water depth. We used a frequency of 15 kHz, a power of 236 dB, a pulse length of 30 ms, a gain of –132 dB, and average beams of 313 per ping. The Atlas MBES collected bathymetry and backscatter, together with water column data. We used the NAVIPAC (EIVA) navigation system to design bathymetric lines and to visualize 3D models of the multibeam bathymetry data in real time. For positioning, we used a GPS system with an estimated error below 2 m. Surveys were conducted over the working areas (Moytirra, MARNA, South Kurchatov, Gigante, NAFZ, Capelinhos, and Faial Ridge) at average water depths of 150–3,000 m and along the transits between them.
MBES data were processed using CARIS HIPS&SIPSTM software. The MBES bathymetry data were used to generate digital terrain models (DTMs) at spatial resolutions of 40 and 60 m (Figure 1E shows MBES data overlapped to Global Multi-Resolution Topography GMRT v3.7 data). Different products such as slope angle maps were generated in order to design the ROV tracks. The multi-resolution DTMs were also used to generate regional sun-shaded image renders, perspective views, and to extract margin-wide bathymetric profiles using FledermausTM software to interpret the submarine landscapes (Figures 1F,G). Sound velocity profiles (SVPs) used for correction of the MBES data consisted of synthetic profiles derived from the CTDs data and XBT (expendable bathythermograph) probes T5 that were compared with the profiles from the WOA13 sound speed database. Single-beam bathymetric tracks were also collected using an EA600 echosounder in order to calibrate the gravimeter data. No further processing was applied to this data.
Sub-Bottom Profiler
Chirp sub-bottom profiles were acquired using a PARASOUND DS Sub-bottom profiler by Teledyne RESON GmBH. The parasound echosounder operates with a primary high frequency (PHF) of 18.6 kHz and a secondary low frequency (SLF) of 4 kHz triggering in chirp mode with a pulse length of 7.5 ms, a source level of 238 dB, and a frequency shift of 3,500–1,700 Hz. The vertical resolution of the PARASOUND DS is 15 cm. We used the software PARASTORE for visualization, processing, and storage the sub-bottom profiler data. We recorded a window of 500–400 m for the SLF records and 1,000 m for the PHF records. SLFs were filtered with a low band pass filter of 6 kHz. The two frequencies, PHF and SLF, were recorded separately and were used to detect plumes along the water column and to study the shallow subsurface sediments respectively.
2D High-Resolution Seismic Profiling
The 2D high-resolution seismic reflection profiles (Figure 1H) were acquired in order to detect fluid pathways in the sedimentary register. The seismic source was composed of an array of 9 GI Guns II with a total capacity of 910 cubic inches (14.9 L) (Figure 1D). The receiving system used was a digital GEOEEL streamer composed of 56 channels and 7 active sections of 50 m length each with a total length of 513.4 m. The recording length of the seismic sections was 9 s at a sample rate of 1 ms. We use Triggerfish System software as the seismic navigation system sourced by two different GPSs. The distance between shots was 25 m in the first leg and 18.75 m in the second leg (multiples of 6.25). Seismic data were processed aboard using Radexpro (DECO) software for quality control.
Mitigation Plan for Mammals and Turtles
In accordance with the existing law within the Portuguese Exclusive Economic Zone, the Azorean Regional Government (GRA) requested the presence of a marine mammal observer (MMO) on board the research vessel Sarmiento de Gamboa to implement mitigation measures (Joint Nature Conservation Committee [JNCC], 2017) during seismic acquisitions using airguns. Standardized recording spreadsheets were used to log effort, airgun activity, and sightings of marine mammals and turtles. Agreed-upon mitigation measures for the survey included soft-start procedures, where the power of the airguns is slowly increased over 20 minutes, pre-shooting watches prior to all airgun activity, and delays in activating the airguns if marine mammals are spotted within the 500 m of mitigation zone. An additional mitigation measure implemented was a “turtle pause,” where the airguns were shut down to eight shots if turtles approached at 100 m of the source in order to minimize possible disturbance or physical harm to the animal. The survey was conducted in full compliance with the Joint Nature Conservation Committee [JNCC] (2017) mitigation plan according the MMO report.
ROV Observations and Sampling
Seafloor observations and sampling were carried out using the ROV Luso (Figure 1C), which was equipped with a high-definition video camera (1920 × 1080 resolution) (O in Supplementary Figure 1) a 10-megapixel still camera (N in Supplementary Figure 1), two robotic manipulators for the recovery of biological/geological samples (H,L, and P in Supplementary Figure 1), CTD (C in Supplementary Figure 1) (E in Supplementary Figure 1), CO2 and CH4 sensors (C in Supplementary Figure 1) and 4 Niskin bottles (2.5 L) for water samples (G1 and G2 in Supplementary Figure 1), and a laser scaling device, with two green lines, distancing approximately 61 cm (A in Supplementary Figure 1). CTD measures temperature (°C), specific conductivity (mS/cm), dissolved oxygen (mg/l), pH, Eh (mV), Turbidity (FTU), and chlorophyll (μg/l). The ROV possessed cores for sediment sampling (K in Supplementary Figure 1), a suction sampler device with five individual chambers for sediment, and some macro- and microbio samples (F1 and F2 in Supplementary Figure 1). A drawer is also installed for larger samples (rocks and large biology samples) (H in Supplementary Figure 1) (I1 and I2 in Supplementary Figure 1) and some sensitive samples. We used a transponder installed in the ROV for submarine positioning of the track dives, which operates at a frequency of 21–31 kHz at water depths ranging between 1,000 and 4,000 m.
Tracks of the ROV were planned using previous MBES. ROV navigation was tracked using Olex software. ROV surveys were controlled in the ROV pilot/copilot container (J in Supplementary Figure 1) and in the laboratory by means of a high-resolution monitor.
Benthic Organisms and Geological Samples
During the ROV Luso dives, real-time video annotation of the observed geology and biology was carried out using the Ocean Floor Observation Protocol (OFOP) software. Afterward, each dive was finalized with a report containing the general characterization of the diving area and the most important geological and biological aspects and samples of the dive. Biological samples were photographed with a scale, labeled, and preserved. Epibionts on geological samples were scraped, photographed, labeled, and preserved as well. All taxa were identified to the lowest possible level, taking into account the expertise of the biologists on board. Collected specimens were labeled with a unique code and photographed with an Olympus camera mounted on the binocular lens. Most of the macroinvertebrates such as Crustacea, Echinodermata, Polychaeta, Bryozoa, and Hydrozoa were preserved in ethanol 96% for further taxonomic identifications in the laboratory. Larger specimens of sponges and corals were frozen at –80°C with some subsamples also taken and preserved in formaldehyde 10% and/or ethanol 96% for morphological/taxonomic and genetic studies. For biology purposes (micromolluscs), the sediment was preserved in ethanol 96%.
All geological rock samples were photographed, labeled, carefully washed with fresh water, and cut aboard using a rock saw to select the best fragments for the different mineralogical, geochemical, and isotopic analysis. Part of the rocks were dried in an oven (max. temp. 48°C) and stored in sealed bags to prevent further oxidation. Other rocks were directly stored at –25°C after recovery. General descriptions of geological samples include their color, structural, textural, and mineralogical features, which were observed on board using binocular lens.
Hydrography
Water Column Measurements
Stations for detection of hydrothermal plumes within the water column were selected following previous geochemical anomalies reported along the northern MAR (Aballea et al., 1998; Jean-Baptiste et al., 2008). Water column measurements and water sampling were performed with a CTD-Rosette (Supplementary Figure 2). The parameters measured with the integrated sensors were temperature, electric conductivity, salinity, turbidity, dissolved oxygen, photosynthetically active radiation, fluorescence, and depth. Oceanographic Rosette is equipped with 24 × 12 L Niskin bottles for water sampling at different depths.
Water and Gas Geochemistry
Fifty subsamples of 1 L were taken on board with the CTD-Rosette and Niskin bottles (ROV) for different analyses at different depths. Sampling was performed for seawater chemistry (major ions, trace elements, metals and oxygen, and hydrogen isotopes) and dissolved gases (chemical and isotopic composition) analyses. All seawater samples were filtered in situ, stored in polyethylene bottles, acidified with HNO3, and refrigerated at 4°C during transport. Redox potentials (ORP) and pH values of the water contained in the Niskin and Rosette bottles were measured. The probes used were properly calibrated on site against supplied calibration standard solutions, pH (7.01, 9.18, and 10.01) and ORP (240 and 220 mV). Concentrations of S2–, Fe(II), and the total Fe were measured with a spectrophotometer, and alkalinity was determined by digital titration with H2SO4 1.6 N.
Dataset Outcomes
A total of 6,250 km of multibeam bathymetry, chirp sub-bottom profiler and gravimeter data were collected in the targeted areas and transits (Figure 1). This dataset allowed us to characterize the physiography, morphostructure, and major tectonic structures of the MAR and the North Azores Transform Fault. In addition, 730 km of seismic multichannel profiles were acquired focusing on the main morpho-tectonic zones, for example, the MAR Axial Valley, the Faial Ridge or the NAFZ, where a series of aligned deep basins extend eastward of the MAR.
Targets for ROV dives were identified with the high-resolution multibeam bathymetry mapping of each area. Eighteen targets were previously investigated for the detection of hydrothermal plumes in the water column using CTD probes with water-sampling carousels. Once the targets were selected, a total of 20 dives were carried out with the ROV Luso to collect images and biological and geological samples (Figures 2, 3) from a wide range of morphotectonic settings along the MAR axial valley (hydrothermal vent fields, axial volcanoes, peripheral subcircular plateaus, massifs, or seamounts related to transform fault zones) and Azores Plateau (Capelinhos volcanic area west of Faial Island, Faial Ridge, and Graciosa Basin along the NAFZ) at different depths. A total of 294 samples were collected with the ROV Luso at water depths between 650 and 3,000-m (Supplementary Figure 1): 179 rock samples, 77 biological samples, 22 water samples, and 16 sediment samples. More than 10,000 Gb of underwater high-resolution videos, representing 154 h, and 34,100 HD pictures were obtained from the diverse deep-sea habitats of the northern MAR north of Azores.
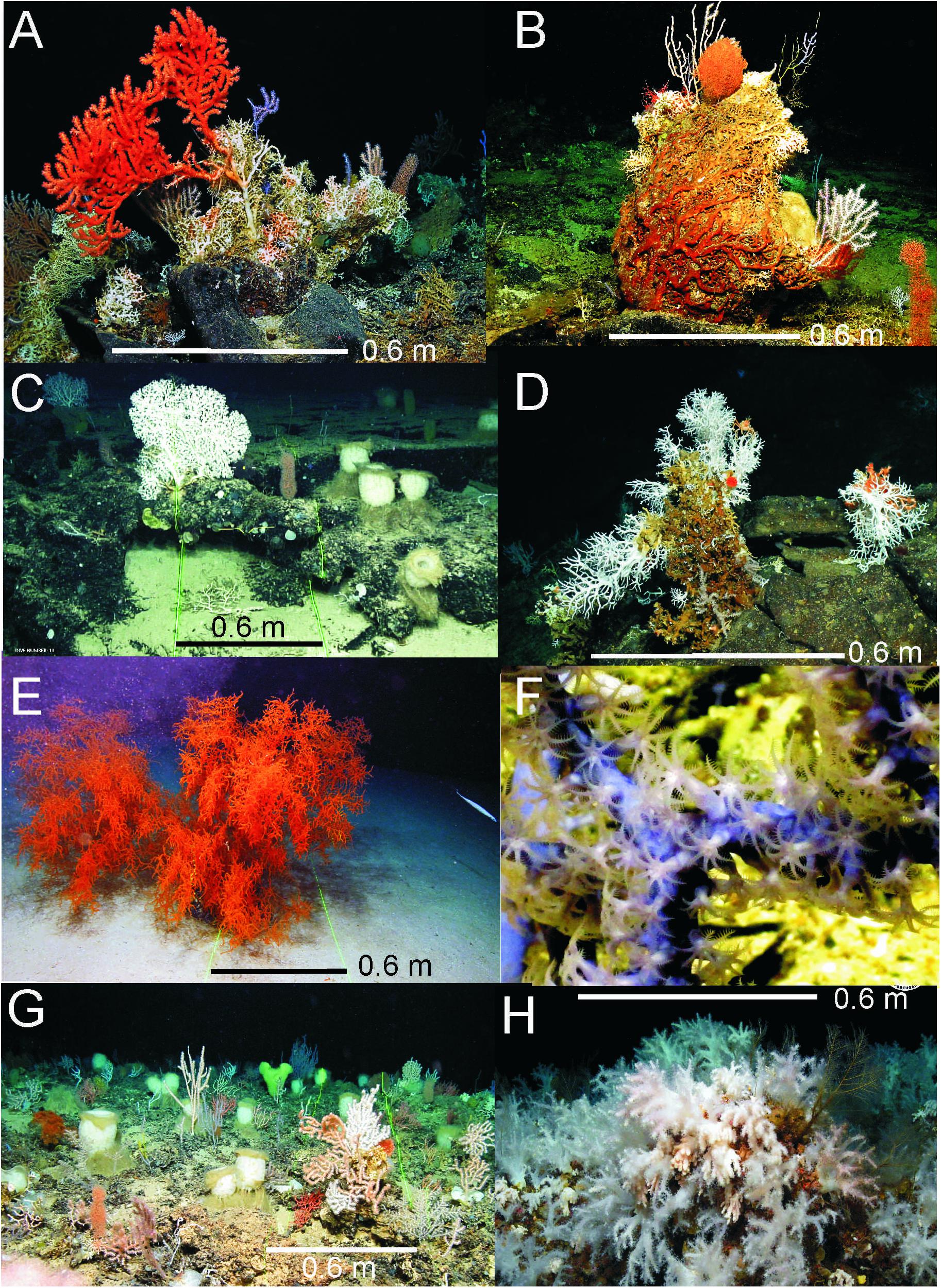
Figure 2. Images of benthic communities identified during the EXPLOSEA2 cruise: (A) Pillow lavas colonized by different species of octocorals and the scleractinians Madrepora oculata and Lophelia pertusa, recently synonymized to Desmophyllum pertusum (Addamo et al., 2016) at 1135 m depth; (B) Several species of octocorals and scleractinians growing over the calcareous skeleton of dead coral at 1155 m depth; (C) Mixed assemblage of octocorals and the glass sponge Pheronema carpenteri at 1226 m depth; (D) Hydrocorals and solitary scleractinian corals (Caryophyllia sp.) growing on pillow lavas at 935 m depth; (E) Large colony of the black coral Leiopathes sp. at 2295 m depth; (F) Unidentified purple octocoral; (G) Mixed coral garden with several species of octocorals, scleractinians (Lophelia pertusa, Madrepora oculata), black corals (Leiopathes sp.) and glass sponges at 1220 m depth; (H) Soft coral garden discovered on a volcanic cone of the Capelinhos volcano, Faial, Azores at 126 m depth.
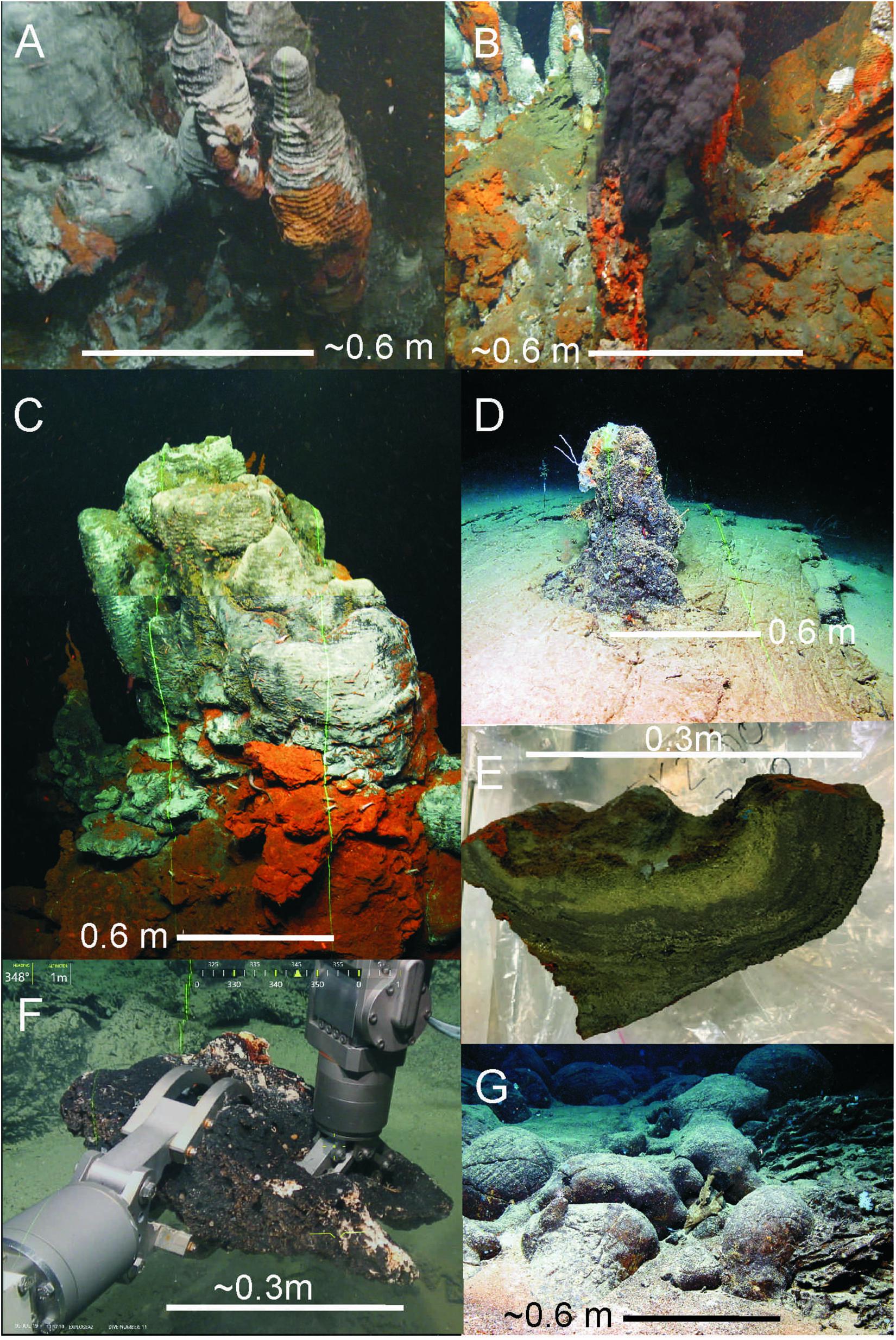
Figure 3. Geological settings identified on the MAR axial valley: (A) Sulfide-anhydrite chimneys; (B) Active black smokers; (C) Large hydrothermal sulfide chimneys topped by anhydrite and Fe-oxyhydroxides; (D) Carbonate chimneys probably associated with abiotic methane oxidation of serpentinites; (E) Massive Sulfide chimney section; (F) Large carbonate chimney collected between the two mechanic arms of the ROV Luso; (G) Lava toothpaste-like flowing downslope the flank of mid-axial ridge volcanoes. Laser scale represents 0.6 m.
The first ROV dives were carried out at the Moytirra field, where it was possible to observe the active vents and to investigate the deepest vents and the slopes that support the previously unexplored hydrothermal field (Supplementary Figure 2). An intense water column study was also conducted in this deep active hydrothermal venting system. We identified hydrothermal plumes in the water column characterized by increases in the turbidity up to + 0.15 NTU and by the reduction of the beam transmission up to –1.2% floating at two levels, 2,200–2,300 m and 2,650–2,800 m in depth (Supplementary Figure 2). Twenty-three water samples along four stations were collected to analyze major ions, trace elements, metals, oxygen, and hydrogen isotopes as well as the chemical (He, H2, CO2, CH4, N2, and O2) and isotopic (δ13C-CO2 and 3He/4He) compositions of the dissolved gases within the hydrothermal plume, some of them directly from the smoking chimneys. The ROV dive sampled venting chimneys, hydrothermally altered volcanic substrates, and broken fragments of chimneys and mounds from the active part of Moytirra field.
Several habitats formed by cold-water corals and sponges that may be classified as vulnerable marine ecosystems have been discovered during the EXPLOSEA2 cruise along the northern MAR and Azores Plateau. These habitats provide structural complexity to the seafloor that is used by numerous associated fauna and therefore have an important role in enhancing deep-sea biodiversity. This is exemplified by a dense aggregation of sponges (Pheronema carpenteri) and diverse coral gardens composed of octocorals, black corals (Leiopathes sp.), scleractinians (Madrepora oculata and Lophelia pertusa), and glass sponges found at a 1,200-m depth (Figure 2G).
Discussion
An outstanding finding of the EXPLOSEA2 survey has been the identification of the first garden of soft corals reported in the Azores Archipelago (Figure 2H). This habitat, composed of a high density of soft corals of the suborder Alcyoniina, was imaged and sampled at the summit and flanks of volcanic cones at 160-m water depth. This submarine volcanic cone showed evidence of hydrothermal activity at their bottom; this can be associated with the eruption of Capelinhos, west of Faial Island, which occurred in 1957–1958. This type of habitat, one composed of a high abundance of soft corals and that lacks carbonate skeletons, may represent the first stages of colonization by octocorals above an acidic low-temperature hydrothermal environment with continuous degasification emissions of CO2 after the cessation of magmatic activity. The evolution of this habitat, colonized in only 50 years, may be useful as a comparison for other recent eruptions that developed in the Macaronesia, as, for example, with the one that occurred on the El Hierro Island, Canary Islands, in 2011–2012 (Somoza et al., 2017).
Another striking finding made during the EXPLOSEA2 cruise was the field of carbonate chimneys (Figure 3D) named the “Magallanes-Elcano” field, a potentially relict ultramafic-hosted hydrothermal site sourced by abiotic methane. This field is related to serpentinite and gabbro rock outcroppings on a dome-shaped massif named the “Iberian Massif.” We suggest that this Massif resulted from uplift tectonics that exhumed mantle rocks at the Azores triple junction (Miranda et al., 2014), forming where the northern and southern branches of the MAR and the Terceira Rift meet at a single point.
Conclusion
The multidisciplinary studies carried out in the EXPLOSEA2 cruise have allowed for the study of several unexplored segments of the northern MAR. Three outstanding findings can be highlighted: (1) an intensive exploration of the deepest parts of the Moytirra field, (2) the discovery of the Capelinhos soft coral garden, and (3) the Magallanes-Elcano field of carbonate chimneys at the Azores Triple Joint point. The three findings can greatly contribute to improve seabed mapping and the knowledge of the geological and hydrochemical processes developed in these hydrothermal submarine systems as well as their close links to microbial and biological activity and mineralization processes. This represents a new step for the knowledge and protection of the marine environment in the North Atlantic, especially of highly vulnerable habitats.
Data Availability Statement
The raw data supporting the conclusions of this article will be made available by the authors, without undue reservation. GIS data (multibeam and multichannel seismic tracks, CTDs and ROV stations) can be downloaded from the EXPLOSEA website at: http://www.igme.es/explosea/dataset.html.
Author Contributions
LS: chief scientist of the cruise, writing, and overall organization. TM: co-chief (Geophysics) and writing. FG, CD, LR, and PM: petrology description and writing. AC, AA, RB, BR, and MS: ROV pilots and writing. MA-R: gas geochemistry and writing. MA, MC-S, and IT: biological sampling and writing. IB: GIS system and dataset. JC, CC, and SM: multibeam processing and imaging. EL-P and ES: water geochemistry and writing. BR-T: microbiology and writing. CV: hydrography and writing. All authors contributed to the article and approved the submitted version.
Funding
The EXPLOSEA2 cruise was funded by the Spanish Ministry for Science and Innovation as part of the project EXPLOSEA (grant CTM201675947-R). This study also benefits from the Atlantic Seabed Mapping International Working Group (ASMIWG) as part of the Atlantic Ocean Research Alliance Coordination and Support Action (AORA-CSA). This study is a contribution to the EMODNET-Geology project (EASME/EMFF/2018/1.3.1.8-Lot 1/SI2.811048) and the European project H2020 GeoERA-MINDeSEA (Grant Agreement No. 731166, project GeoE.171.001). MC-S was supported by the European Union’s Horizon 2020 research and innovation program under grant agreement no. 678760 (ATLAS). CV is supported by the Fundação para a Ciência e a Tecnologia (FCT) under the Ph.D. fellowship (SFRH/BD/129683/2017), FunAzores -ACORES 01-0145-FEDER-000123 grant, FCT strategic project UID/05634/2020 and SponGES project, European Union’s Horizon 2020 research and innovation programme under grant agreement No. 679849.
Conflict of Interest
The authors declare that the research was conducted in the absence of any commercial or financial relationships that could be construed as a potential conflict of interest.
Acknowledgments
We are grateful to the captain, officers, and the crew of the R/V Sarmiento de Gamboa for their dedication during the EXPLOSEA2 cruise. Special thanks to the technicians of the UTM (CSIC), including acoustic, seismic mechanics, and computers, for their dedication and professionalism during the cruise. We also thank Manuel C. Fernandes, marine mammal observer (MMO), for his seismic works dedication and recommendations, which were strictly followed during the cruise. The EXPLOSEA2 cruise benefits from the Scientific Agreement between Spain and Portugal to share oceanographic vessels and ROV. Thanks to the Azorean Regional Government (GRA) for their facilities and support to investigate marine protected areas.
Supplementary Material
The Supplementary Material for this article can be found online at: https://www.frontiersin.org/articles/10.3389/fmars.2020.568035/full#supplementary-material
Footnotes
- ^ https://www.atlanticresource.org/aora/mapping-our-ocean
- ^ https://vents-data.interridge.org/
- ^ https://vents-data.interridge.org/ventfield/luso
- ^ http://www.igme.es/explosea
References
Aballea, M., Radford-Knoerya, J., Apprioub, P., Bougaulta, H., Charloua, J. L., Donvala, J. P., et al. (1998). Manganese distribution in the water column near the Azores Triple Junction along the Mid-Atlantic Ridge and in the Azores domain. Deep Sea Res. I 45, 1319–1338. doi: 10.1016/s0967-0637(98)80012-5
Addamo, A. M., Vertino, A., Stolarski, J., García-Jiménez, R., Taviani, M., and Machordom, A. (2016). Merging scleractinian genera: the overwhelming genetic similarity between solitary Desmophyllum and colonial Lophelia. BMC Evol. Biol. 16:108. doi: 10.1186/s12862-016-0654-8
Baker, E. T. (2017). Exploring the ocean for hydrothermal venting: new techniques, new discoveries, new insights. Ore Geol. Rev. 86, 55–69. doi: 10.1016/j.oregeorev.2017.02.006
Beaulieu, S. E., Baker, E. T., German, C. R., and Maffei, A. (2013). An authoritative global database for active submarine hydrothermal vent fields. Geochem. Geophys. Geosyst. 14, 4892–4905. doi: 10.1002/2013GC004998
Dias, F. C., Lourenco, N., Lobo, A., Santos de Campos, A., and Pinto de Abreu, M. (2009). “Fried Egg”: An Oceanic Impact Crater in the Mid-Atlantic?. San Francisco, CA: American Geophysical Union.
Jean-Baptiste, P., Fourré, E., Dapoigny, A., Charlou, J. L., and Donval, J. P. (2008). Deepwater mantle 3He plumes over the northern Mid-Atlantic Ridge (36° N–40° N) and the azores platform. Geochem. Geophys. Geosyst. 9:GC001765.
Joint Nature Conservation Committee [JNCC] (2017). JNCC Guidelines for Minimising the Risk of Injury to Marine Mammals From Geophysical Surveys. Peterborough: Joint Nature Conservation Committee.
Miranda, J. M., Luis, J. F., Lourenço, N., and Goslin, J. (2014). Distributed deformation close to the Azores Triple “Point”. Mar. Geol. 355, 27–35. doi: 10.1016/j.margeo.2014.05.006
Ryan, W. B. F., Carbotte, S. M., Coplan, J. O., O’Hara, S., Melkonian, A., Arko, R., et al. (2009). Global multi-resolution topography synthesis. Geochem. Geophys. Geosyst. 10:GC002332.
Somoza, L., González, F. J., Barker, S. J., Madureira, P., Medialdea, T., de Ignacio, C., et al. (2017). Evolution of submarine eruptive activity during the 2011 El Hierro event as documented by hydroacoustic images and remotely operated vehicle observations. Geochem. Geophys. Geosyst. 18:GC006733. doi: 10.1002/2016GC006733
Keywords: Mid Atlantic Ridge, hydrothermal vent areas, remote operated vehicle, Cold-water corals, deep-sea habitats, multidisciplinary approach, vulnerable ecological region, Azores archipelago
Citation: Somoza L, Medialdea T, González FJ, Calado A, Afonso A, Albuquerque M, Asensio-Ramos M, Bettencourt R, Blasco I, Candón JA, Carreiro-Silva M, Cid C, De Ignacio C, López-Pamo E, Machancoses S, Ramos B, Ribeiro LP, Rincón-Tomás B, Santofimia E, Souto M, Tojeira I, Viegas C and Madureira P (2020) Multidisciplinary Scientific Cruise to the Northern Mid-Atlantic Ridge and Azores Archipelago. Front. Mar. Sci. 7:568035. doi: 10.3389/fmars.2020.568035
Received: 31 May 2020; Accepted: 30 September 2020;
Published: 04 November 2020.
Edited by:
Daniela Zeppilli, Institut Français de Recherche pour l’Exploitation de la Mer (IFREMER), FranceReviewed by:
Lorenzo Angeletti, Institute of Marine Sciences, National Research Council (CNR), ItalyElisa Baldrighi, Institute for Biological Resources and Marine Biotechnology, National Research Council (CNR), Italy
Copyright © 2020 Somoza, Medialdea, González, Calado, Afonso, Albuquerque, Asensio-Ramos, Bettencourt, Blasco, Candón, Carreiro-Silva, Cid, De Ignacio, López-Pamo, Machancoses, Ramos, Ribeiro, Rincón-Tomás, Santofimia, Souto, Tojeira, Viegas and Madureira. This is an open-access article distributed under the terms of the Creative Commons Attribution License (CC BY). The use, distribution or reproduction in other forums is permitted, provided the original author(s) and the copyright owner(s) are credited and that the original publication in this journal is cited, in accordance with accepted academic practice. No use, distribution or reproduction is permitted which does not comply with these terms.
*Correspondence: Luis Somoza, l.somoza@igme.es