- 1Grupo Mosquitos Vetores: Endossimbiontes e Interação Patógeno-Vetor, Instituto René Rachou, Fiocruz, Belo Horizonte, Brazil
- 2Grupo Entomologia Médica, Laboratório de Coleções Zoológicas, Instituto Butantan, São Paulo, Brazil
Arthropod-borne viruses have a significant impact on public health worldwide, and their (re) emergence put aside the importance of other circulating arboviruses. Therefore, this scoping review aims to identify and characterize the literature produced in recent years, focusing on aspects of two arboviruses: Mayaro virus and Oropouche orthobunyavirus. The Mayaro and Oropouche viruses were isolated for the first time in Trinidad and Tobago in 1954 and 1955, respectively, and have more recently caused numerous outbreaks. In addition, they have been incriminated as candidate diseases for human epidemics. These viruses have been drawing the attention of public health authorities worldwide following recent outbreaks. To determine the global epidemiological profile of these viruses, we used the Dimensions Database, which contains more than 100 million publications. In general, we identified 327 studies published from 1957 to 2020 for Mayaro virus, and 152 studies published from 1961 to 2020 for Oropouche orthobunyavirus. Interestingly, we observed that Mayaro and Oropouche had a significant increase in the number of publications in recent years. Thus, this comprehensive review will be helpful to guide future research based on the identified knowledge gaps.
Introduction
New pathogens, vectors, and diseases are constantly discovered; which is therefore applied the concept of “emerging disease” (1, 2). In this context, the arthropod-borne viruses (arboviruses) stand out due to their explosive global distribution, transmission capacity, close relationship with changes in the environment, and human behavior (3). However, even though arboviruses infect millions of humans and other animals and cause great economic impact on public health worldwide, most remain neglected. These viruses correspond to an extensive group of viral vector borne infections, for example the Dengue virus (DENV), Zika virus (ZIKV), and Chikungunya virus (CHIKV) viruses that are globally spreading (4). The (re)emergence of these “already known” pathogens put aside the importance of other lesser known circulating arboviruses, such as Mayaro virus (MAYV) and Oropouche orthobunyavirus (OROV). The first one was isolated in Trinidad and Tobago (1) and quickly spread outside its original geographical range because of its vector’s vast distribution and competence. In addition, this virus has drawn the attention of public health authorities worldwide following recent outbreaks in the Americas (5).
Lastly, OROV is a poorly studied pathogen responsible for some outbreaks since the early 1960s, representing a public health burden to Brazil, Panama, and Peru (6). Therefore, this review aims to summarize the state of the entomo-epidemiological literature about these two arboviruses, gathering the information published in recent years, focusing on the virus and vector dispersion, symptoms, and clinical issues.
Methods
Search and Data Management Process
The present review was performed on the Dimensions Database (Digital Science and Research Solutions Inc.), which contains more than 100 million publications available to consult. Our research has been composed of two Phases: I) The first group of documents was accessed using the following inclusion factor criteria/keywords: ’Mayaro Virus’ in the title and abstract + Publication Year: 1957-2020; and ’Oropouche Virus’ in the title and abstract + Publication Year: 1961-2020. In addition, all documents published were considered; Open and Closed Access; Publication Type: Article, Preprint, Chapter/Thesis, and Proceeding.
Thus, the search results composed a database in electronic spreadsheets (Google Sheets) related to each keyword and organized by Title, DOI, Type and Year of Publication, Authors, and Abstract. Following that, the results were analyzed, and the second and final phase was subsequently applied. II) In this phase, we performed a filtered analysis considering the following exclusion criteria: Any data not covered by the date range of MAYV (1957–2020) and OROV (1961–2020); any study that was not in the English language; any document that was not a Scientific Article (except Letter to Editor, Editorial, Note, Short Communication, Etc.); any duplicated file; any article which did not present at least one piece of information about viral biology, epidemiology (virus/vector distribution), clinical manifestations and disease prevention/treatment in its abstract.
Results
The scope of this review included studies focused on different aspects of two arboviruses: MAYV and OROV. As a result of Phase I, a constant, although low, number of publications were observed for MAYV between 1957 and 2009, with no more than five documents published per year. Subsequently, the numbers of reports fluctuate until 2016, followed by an exponential upward curve that peaked in 2019, with more than 60 documents (Figure 1). Those papers were mainly published in open access platforms, with emphasis on articles (n= 295), followed by preprints (n= 17), chapters (n= 10), proceedings (n= 5) and edited books (n= 1) (Figure 2).
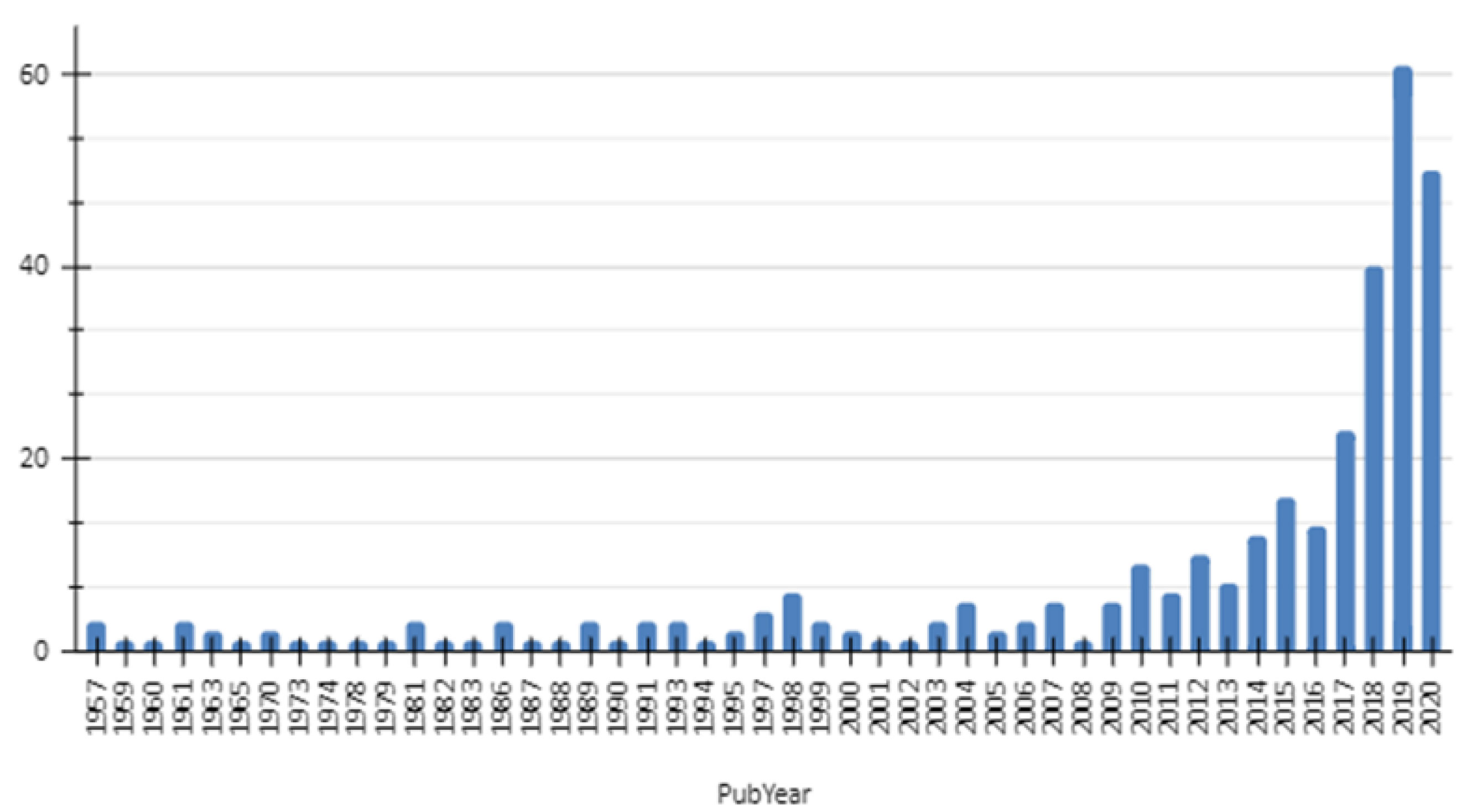
Figure 1 Graphical representation of the number of documents published about MAYV by year from 1957 to 2020 resulted from a search performed at Dimensions.io Database and exported on Jan 15, 2021.
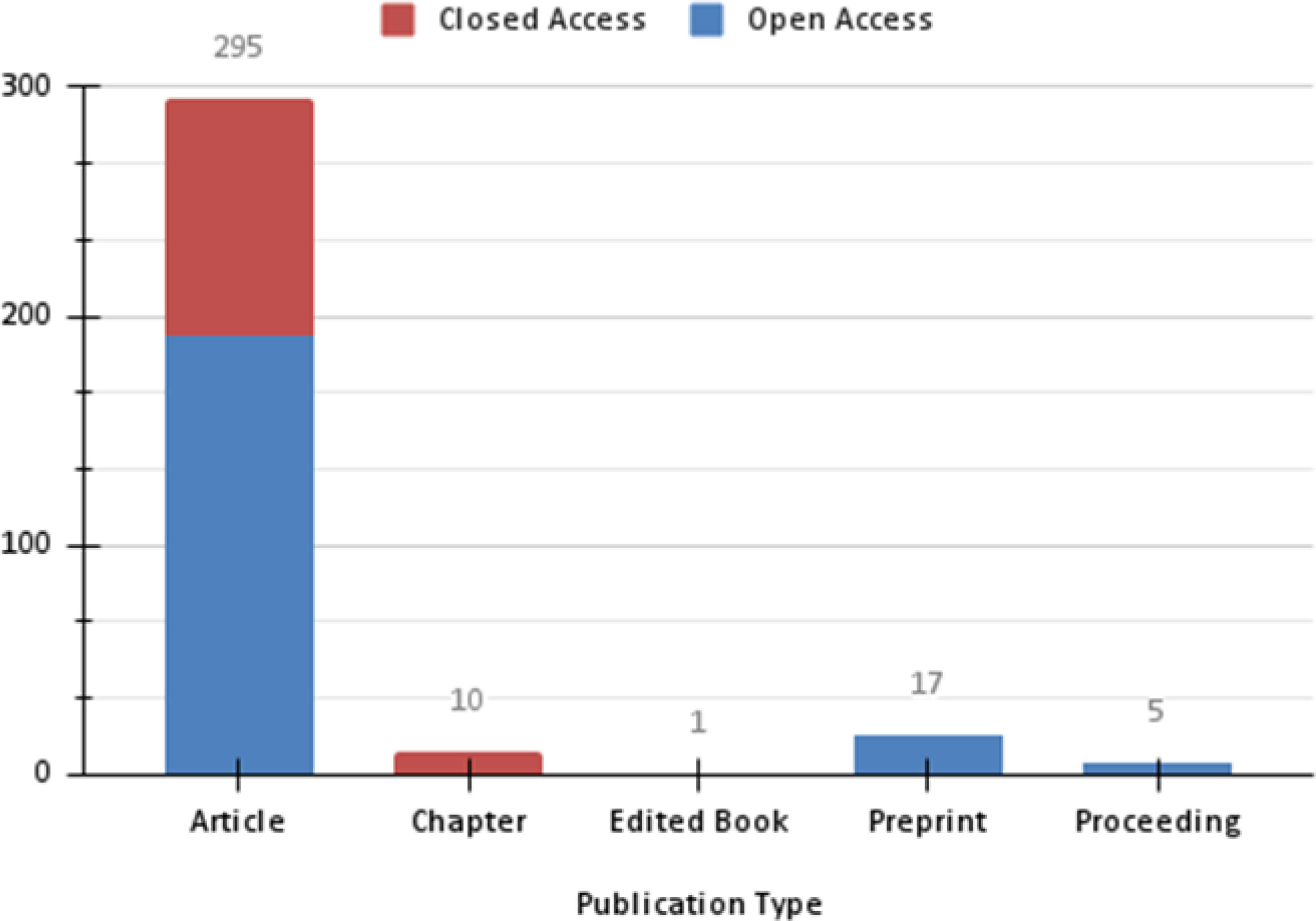
Figure 2 Graphical representation of the number of documents published about MAYV by type and access platform resulted from a search performed at Dimensions.io Database and exported on Jan 15, 2021.
The lowest number of documents was observed for OROV, rarely exceeding five documents per year from 1961 to 2014. From 2015, the number of documents rose significantly, reaching a peak in 2017 with 16 documents (Figure 3). Among those reports, we retrieved articles (n= 134), chapters (n= 12), preprint (n= 4) and proceedings (n= 2) (Figure 4).
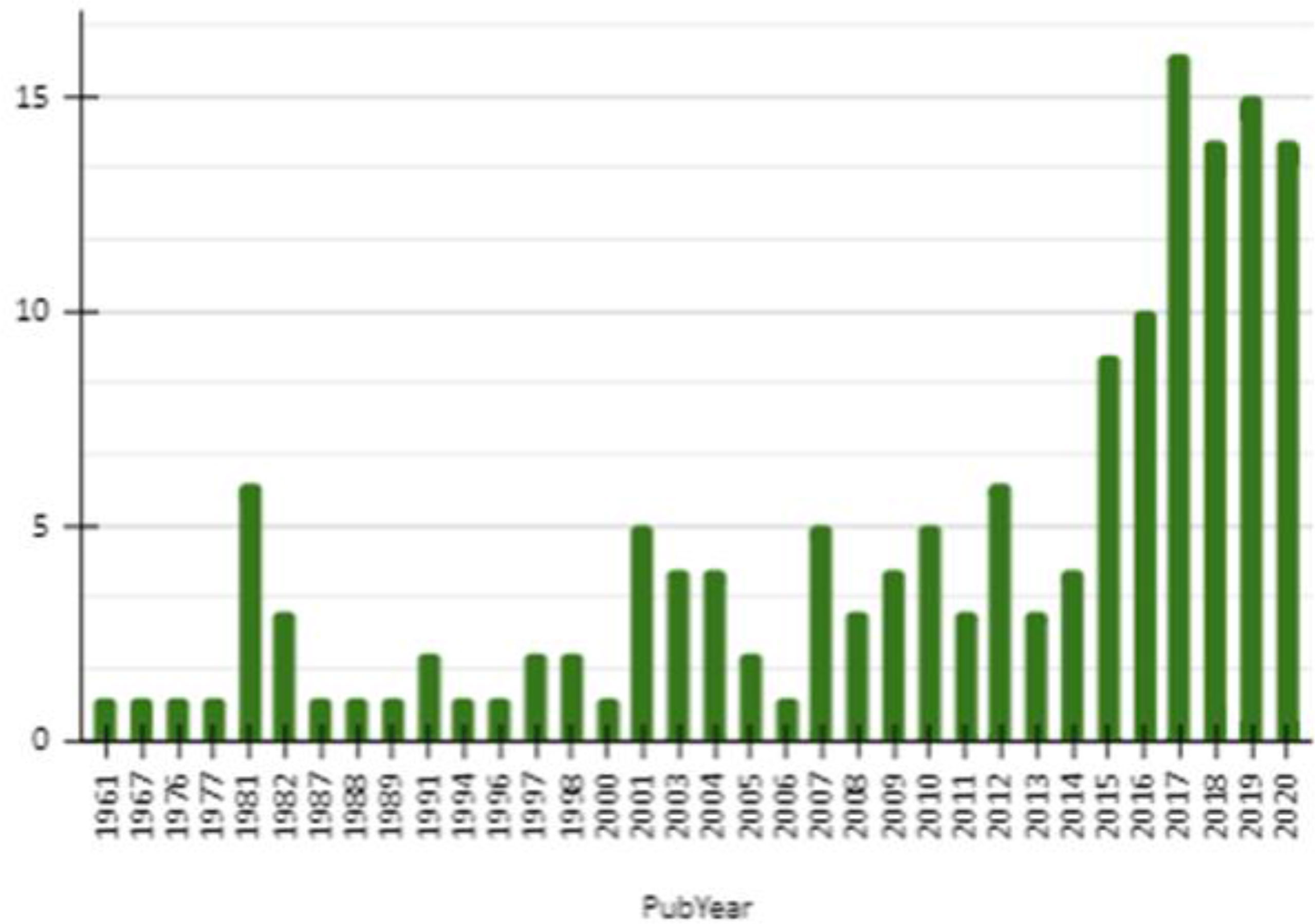
Figure 3 Graphical representation of the number of documents published about OROV by year from 1961 to 2020 resulted from a search performed at Dimensions.io Database and exported on Jan 15, 2021.
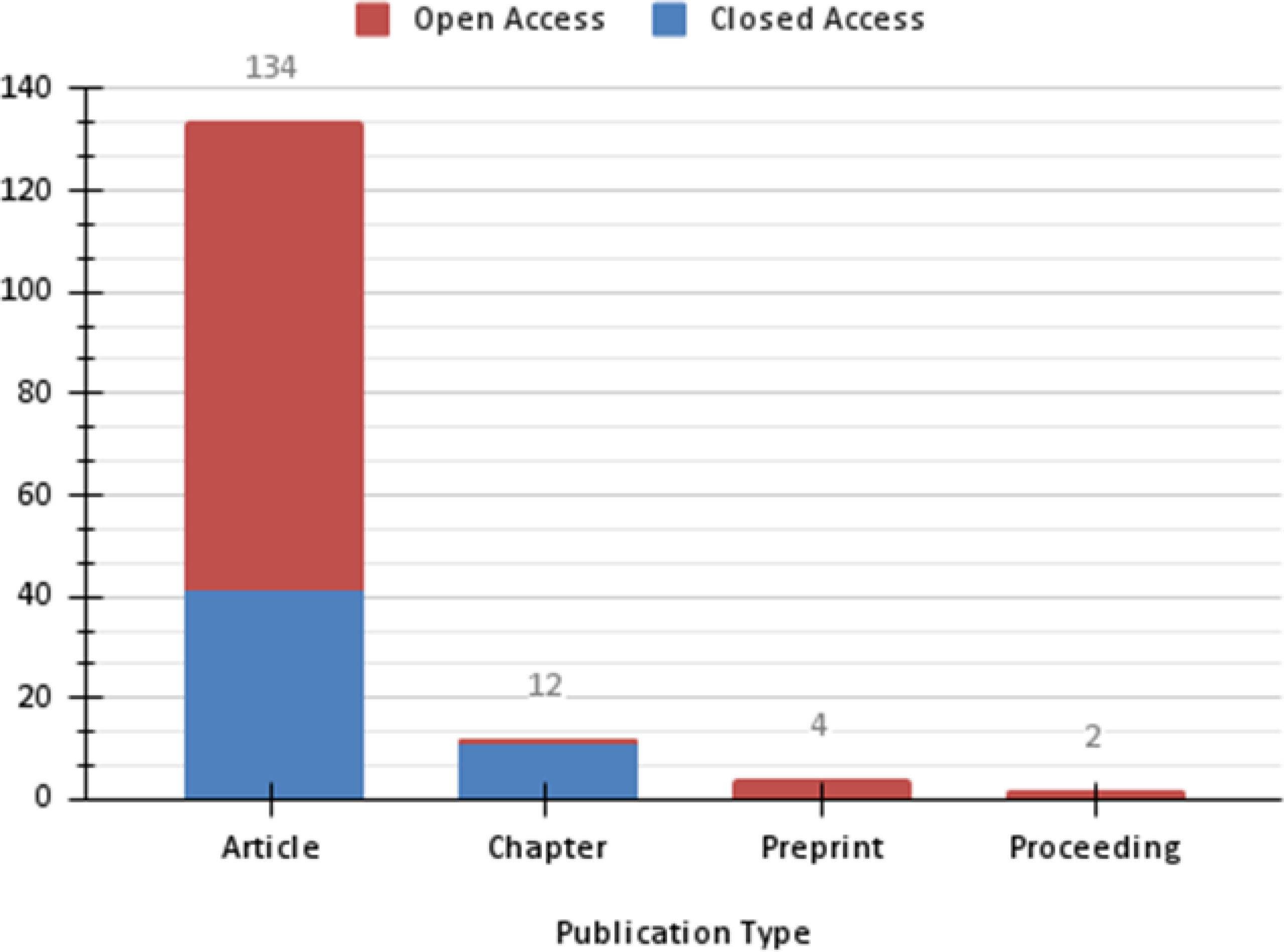
Figure 4 Graphical representation of the number of documents published about OROV by type and access platform resulted from a search performed at Dimensions.io Database and exported on Jan 15, 2021.
As a result of Phase II (Table 1), after applying all of the predefined filters, we obtained a total of 168, and 82 articles for MAYV and OROV, respectively. Despite the lower number for filtered OROV data, the scientific content was rich, which provided an interesting discussion. Curiously, despite OROV first being isolated in 1955, the first article was published only six years later. Below, we present our review of the compiled data.
Mayaro Virus
Virus Information
Jordi Casals and Whitman described MAYV as a representative of the Alphavirus genus, belonging to the Togaviridae family (1, 7, 8), and it has been isolated in the 1950s by Charles Anderson from rural workers in Trinidad Island. It has three identified genotypes (D, L, and N), and the virus comprises a simple RNA strand with positive polarity, with a genome sequence of approximately 11.5 kilobases (kb).
In general, the life cycle of MAYV is poorly known and needs further study. However, some aspects of its life cycle are like several arthritogenic alphaviruses. The cycle starts with the fusion of the viral envelope and the target cells’ plasma membrane via specific receptors on their surface. After that, the virus invades the cell and reaches the cytoplasm simultaneously when the pH of the endosome decreases (9).
Subsequently, the basic virus structure is disassembled, and the genomic RNA is released and translated into nonstructural proteins, which generate messenger RNA and various structural proteins (5). Finally, it generates the nucleocapsid, and its glycoproteins, combined with the plasma membrane, lead to the release of new viral particles outside the cell (5). The released viral particles can then infect new cells and start their cycle again.
In addition, the MAYV presents remarkable genetic plasticity due to the high rate of mutation, which increases the likelihood of adaptation to new hosts, both vertebrates and invertebrates (10).
Vectors and Reservoir Hosts
The primary vectors of MAYV are mosquitoes belonging to the genus Haemagogus (11, 12), while the secondary vectors may vary between different genus like Culex sp., Sabethes sp., Psorophora sp, Coquillettidia sp. and Aedes spp (8, 13). Furthermore, in a laboratory-controlled environment, studies show that mosquitoes such as Ae. aegypti, Ae. albopictus, Anopheles spp. and Cx. quinquefasciatus can also harbor and transmit MAYV (14–18). Considering the potential of those mosquitoes playing a role as vectors for MAYV, the importance of this virus in urban areas is increasing.
The MAYV seropositivity in different vertebrates such as arboreal mammals, birds, and even alligators, has already been demonstrated. Reports of this range of potential hosts indicates replication and circulation of the virus, which is likely using them as reservoirs (19). In addition, birds, marsupials, and rodents combined with primary and secondary vectors further increase the chances of spreading the virus (20–22).
Geographic Distribution
After the first report, it was suggested that MAYV could be restricted to forest areas in the tropical region of South and Central America. However, over the years, many countries such as French Guiana, Bolivia, Peru, Suriname, Trinidad, Tobago, Argentina, Colombia, Caribbean, Venezuela, and Brazil have reported cases of MAYV (1, 23–25). Furthermore, in 2015, the virus was disclosed in an outbreak in Venezuela. Recently, the presence of the virus was recorded in two new Brazilian states: São Paulo and Rio de Janeiro (26), indicating that the virus continues to spread. In the wild cycle, MAYV is kept in a similar way as the sylvatic cycle of YFV, being transmitted mainly by mosquitoes of the genus Haemagogus (12). However, it is believed that MAYV can also be transmitted by secondary vectors, since the isolation of MAYV from other Culicidae species such as Aedes, Culex, Psorophora, Coquillettidia, Sabethes, and Haemagogus spp. has been reported (11, 13, 27).
Regarding the MAYV genotypes, until now, three genotypes (D, L, and N) have already been identified. The genotype D has a wide geographical distribution, occurring in Brazil, and other countries such as French Guiana, Bolivia, Peru, Surinam, Trinidad, Tobago, Argentina, Colombia, and Venezuela (28–30). On the other hand, the L genotype has a more limited distribution and has being described in Haiti and Brazil (27, 29). Lastly, the N genotype was first reported in 2015 in Venezuela (28).
The relevance of MAYV as a human pathogen and its potential for emergence in urban areas is of great concern, for example, Lorenz et al. (31), highlighted that environmental variations, mainly due to global warming, may contribute in the coming years to expand the distribution of MAYV. Furthermore, population growth, demographics, and other factors combined with the vectorial competence of different species of mosquitoes suggest that MAYV infection can reach an even larger scale in a short time, along with other arboviruses.
MAYV has already been registered in different parts of the world in isolated cases or small outbreaks, which indicates that the virus continues to spread. In this context, a significant number of published articles have arisen since this virus obtained greater visibility, both in the media and the scientific environment. Another factor contributing to the spread of the virus may be related to negligence towards this disease and its suspected and confirmed vectors, such as mosquito Ae. albopictus. In the last years, this species has been highlighted in studies which presents its ability to play a role as a potential bridge between the sylvatic and urban cycles of several arboviruses (32–35).
Clinical Aspects
To date, few studies are available on MAYV infection, and those which are provide a favorable general clinical aspect. However, inflammation and joint pain can persist for a long time, limiting or incapacitating the infected person. In general, the symptoms are very similar to those of other arboviruses; for this reason, MAYV is often misdiagnosed (8, 19, 23, 24, 36, 37). In humans, MAYV is considered an accidental infection and presents a greater risk for individuals who developed activities inside or close to forests. MAYV infection is not fatal, and its symptoms resemble those of DENV and CHIKV, causing an acute and self-limiting febrile illness with a short viremic period.
When in the viremic phase, the infected individual may have a viral amount of RNA (detected by RT-qPCR) between 2.7×102 and 1.9×105. For CHIKV (virus close-related to MAYV), symptomatic individuals present between 1.3×101 and 2.9×108 pfu/mL. Individuals infected with MAYV and/or CHIKV, presenting the described titers, may be potential disseminators of the virus (27, 38, 39).
The acute phase of the disease lasts for 3-5 days and includes fever, headache, eye pain, rash, myocarditis, hemorrhagic and neurological manifestations (37, 40, 41). Arthralgia and myalgia account for 50–89% and 75% in infected patients, respectively. The arthralgia can be accompanied by joint swelling, which is the main symptom in severe infection that occasionally persists for several months, incapacitating the patient (24, 42).
In addition, severe cases can present encephalitis (inflammation in the brain), although the disease is self-limiting in most cases, with the symptoms disappearing within a week (23, 37). Since 2011, the Brazilian Ministry of Health has included MAYV as a compulsory notification disease within the National Health System (Ministério da Saúde, Portaria N°104, de 25 de Janeiro de 2011).
Diagnostic and Treatment
Neutralization and reverse-transcription polymerase chain reaction (RT-qPCR) tests are now available in most laboratories and can help to confirm MAYV infection (43). Molecular studies have advanced towards the detection of MAYV in plasma and urine samples with promising results, being a potential tool for diagnosis of patients with MAYV (44). Currently, the absence of a specific test for diagnoses contributes to the underreported number of cases. Furthermore, since the diagnosis is mainly based on symptoms, the infection can be easily misdiagnosed with other arboviruses, such as CHIKV, DENV, and ZIKV infections, due to the possible co-circulation of these viruses in the same area (10, 23, 30).
In this sense, we must consider other factors that limit MAYV diagnosis, as the possibility of cross-reaction, in particular with CHIKV and O’nyong-nyong virus specific antibodies and the high prevalence of asymptomatic cases, thus underestimating the number of cases (25, 40, 45). In addition, it is important to mention that cross-reactivity for both MAYV with ZIKV and CHIKV has already been reported in Zambia (46). Furthermore, to date, there is no vaccine or specific therapy for this illness, although there are studies working towards vaccine development (47, 48).
Oropouche orthobunyavirus
Virus Information
OROV is a member of the Peribunyaviridae family, Orthobunyavirus genus, and is part of the Simbu serogroup. OROV is an emerging virus that causes a Dengue-like illness known as Oropouche fever. It is an enveloped virus with a tri-segmented single-stranded negative-sense RNA genome, typical of the Orthobunyaviruses (49). The large segment (L) encodes the viral RNA-dependent RNA polymerase. The medium segment (M) encodes a polyprotein originating the envelope glycoproteins and the nonstructural protein (NS). Additionally, the small segment (S) encodes the nucleocapsid protein that protects viral RNA from degradation (49, 50).
In this context, OROV NSm and NSs proteins have started to be exploited by the generation of OROV recombinant viruses (51–54). In addition, Aquino et al. (55), described the complete nucleotide sequence of the OROV L RNA segment. OROV can interchange segments of RNA with other strains through genetic reassortment, which is common in viruses with segmented genomes, allowing the incorporation of genes and development of novel virulent viral strains (36, 56), such as Iquitos Virus (36) and Jatobal (57). These reassorted strains together with the “original strain” are the only zoonotic virus of the Simbu serogroup present in South America. The genome of these reassortment viruses contains an L and S segment from OROV and an M segment from an unknown virus. Since the M segment encodes for viral attachment and entry proteins, it could potentially change the virus tropism and expand the host range of OROV (58).
Studies on the molecular biology of the OROV small RNA segment have suggested its monophyletic origin and the existence of at least three genotypes (I, II, and III) (57). In addition, Vasconcelos et al. (59) suggested that OROV emerged in Brazil ≈223 years ago. Furthermore, based on the N gene analysis, these authors suggested that genotype I was responsible for the emergence of all other genotypes and virus dispersal, besides the observed distinct patterns of OROV strain clustered according to each RNA segment (59). However, their results suggest that each has a different evolutionary history and that the classification in genotypes must consider each particularity.
Vectors and Reservoir Hosts
Studies have suggested that South and Central America OROV has two distinct transmission cycles: sylvatic and urban. In the first, the transmission occurs between its insect vectors and wild host such as birds and mammals like Proechimys sp., Bradypus tridactulus, Allouatta caraya, and Callithrix penicillata (50, 60, 61). During this cycle, the virus is transmitted by vector culicids such as Aedes serratus and Coquillettidia venezuelensis, from which OROV has been isolated in the Brazilian Amazon region and Trinidad, respectively (62–64, 66).
In contrast, the urban cycle involves humans as the only vertebrate hosts, excluding domestic animals (66), and hitherto uncertain mosquito species. In this cycle, the role of Culicoides paraensis (Goeldi) as a main vector has been supported by experimental and epidemiological data (50, 63). Besides that, anthropogenic actions on forest areas and the presence of C. paraensis in urban areas can facilitate the vector’s contact with humans (67–71).
Additionally, laboratory settings have shown the susceptibility of culicids species such as Aedes scapularis, Aedes albopictus, Culex fatigans, and Psorophora ferox to OROV (58). Mendonça et al. (72) recently showed that OROV is restricted by the midgut barrier of Ae. aegypti, Ae. albopictus, and Cx. quinquefasciatus in a laboratory-controlled environment. However, the authors highlight that if this restriction is overcome, OROV could be efficiently transmitted to vertebrate hosts. In this context, besides Cx quinquefasciatus having been frequently detected with OROV during outbreaks (73), to date, it appears to have a low efficiency as a vector for OROV.
The link between the sylvatic and urban cycles is probably due to humans entering the forests, becoming infected, and returning to urbanized areas (63). Additionally, it is essential to mention that wild and domestic birds have tested positive for OROV antibodies during outbreaks, but their importance to OROV spread remains to be determined (58).
Geographic Distribution
OROV is an endemic virus which was isolated in 1955 in Trinidad and Tobago from a pool of Coquillettidia venezuelensis. Simultaneously, antibodies against OROV were detected in a 24-year-old male field worker and non-human primate. All of these discoveries were in 1955, with data from a village called Vega de Oropouche on the Oropouche river from which the virus acquired its name (64, 65). This virus has caused several outbreaks, mainly in Brazil, the Amazon region, Panama, and the Caribbean (50, 52, 68, 73, 74).
Other South American countries also faced the presence of OROV, such as Ecuador (Guayaquil) (10, 75); Peru (Martin, Santa Clara, Cajamarca, Cusco, Yurimaguas, Madre de Dios, Iquitos - Loreto, Bagazan-Pachiza, Huánuco, Leoncio Prado, Puerto Maldonado (62, 76–79), Panama (Chame/San Miguelito, Bejuco, Chilibre), Venezuela, Argentina and Bolivia (64, 73, 78, 79). Given the current situation of OROV, it is possible to note the silent expansion of this virus.
Clinical Aspects
It is estimated that 63% of OROV infections are symptomatic (36, 56, 67). The most common clinical symptoms reported among the patients with OROV infections are acute febrile, headache, myalgia, arthralgia, malaise, photophobia, exanthema, polyuria, anorexia, dizziness, chills, joint pain (64), retro-ocular pain, hypoxia (79), muscle pain and skin rash. In addition, many infections develop into meningitis or encephalitis (69), as well as spontaneous bleeding (in rare cases: petechiae, epistaxis and gingival bleeding) and aseptic meningitis (66).
Most of these clinical symptoms are observed in around 60% of patients resembling classical arboviral infection and highlighting the importance of laboratory diagnostic tests (65), being challenging to diagnose and distinguish from other co-occurring infectious diseases (80).
In addition, it was not uncommon to find studies reporting the identification of OROV in patients with suspected other viral infections, as well as co-infections with DENV, ZIKV and CHIKV (77). Simultaneous co-infection with two or three different viruses alters the immune response but does not imply more clinical severe symptomatology than single viral infections (81). Specifically, in the Peruvian region, OROV infections and co-infections were more frequent in May, June, and July, corresponding to the region’s dry season (77).
In the Amazon region, OROV infections are challenging to distinguish from undifferentiated febrile illness associated with group C Orthobunyaviruses or Candiru complex. Furthermore, the symptoms present no differences even in patients with co-infections between different arboviruses (82), and they can reappear one or two weeks after recovery in about 60% of the patients. Additionally, there are reports of patients that showed hemorrhagic symptoms or neurological complications associated with OROV infection (50, 67, 77).
Although fatal cases have not been reported, OROV is known for causing dramatic human epidemics (83). In addition, it is essential to mention that the segmented nature of the OROV genome enhances the likelihood of genome reassortment events. This phenomenon has been associated, in some cases, with a significant increase in disease severity to other viruses (84).
Diagnostic and Treatment
The epidemiological surveillance of OROV is based on detecting the virus genome in the plasma/sera of acutely infected patients or by specific IgM serology during convalescence (50, 55, 57, 64, 85). However, despite genome detection by PCR or RT-qPCR being considered a high-quality detection test, the cost is still high. As an alternative, recent studies have shown arbovirus detection using other body fluids, such as saliva and urine. For example, Naveca et al. (83) found a higher viral load in urine, as suggested by the lower Ct value observed in this specimen, in comparison with results from saliva or plasma.
There is no specific treatment for OROV, only primarily supportive care like prevention and treatment. Adhikari et al. (86) showed novel candidates for epitope-based peptide vaccine design against OROV. Furthermore, they predicted the 3D structure of the most antigenic polyprotein and inhibitor-binding sites for the docking simulation study using various bioinformatics tools.
Conclusion
Infectious diseases have a considerable impact on human, animal, and plant health, causing significant morbimortality and placing a costly burden on global economies. Moreover, arboviruses have become critical threats worldwide due to rapid climatic and geographical changes in urban and peri-urban areas (87). In addition, in many cases, the precarious health conditions favor the reproduction of vectors and, consequently, viral amplification and transmission.
MAYV infection in humans has mainly been reported in rural areas. However, the virus has been registered in urban areas in recent years, making the disease more evident. Given the circumstances and their new worldwide records, it is crucial to monitor the evolution of MAYV since this virus can establish an epidemic scenario, as happened with other viruses such as ZIKV and CHIKV. Currently, the literature reports that Ae. aegypti mosquitoes are susceptible to MAYV infection and are potential candidates for the urban life cycle. However, it is essential to emphasize the role of Ae. albopictus; this vector can serve as a secondary vector in an outbreak or as a bridge vector between the MAYV or another arbovirus.
Although the MAYV has been neglected for many years and its biology remains poorly understood and largely inferred from related alphaviruses, studies have progressed and for the first time in Latin America, researchers have managed to elucidate the complete molecular structure of MAYV (88). Another critical point is the lack of studies on vaccine development, antiviral treatment, and specific diagnostic tests since the symptoms can be easily confused with other arboviruses.
OROV fever is an emerging and endemic zoonotic disease from some South and Central American areas, with an acute and self-limiting febrile condition. It has long been a neglected disease even though it has infected many people (more than half a million). Although OROV represents a public health concern to some countries, this virus is still poorly studied, and one of the factors that may contribute to the absence of studies on OROV is an underreporting, since its symptomatology can be easily confounded with other febrile illnesses.
The spread of OROV is related to environmental factors, including climate, fauna, and flora changes. For example, Romero Alvarez et al. (62) reported an OROV outbreak in southeastern Peru, where a loss of vegetation was observed at the outbreak site. Indeed, environmental changes, climate and socioeconomic factors could shortly cause the translocation of OROV, as has already been seen for other pathogens. Consistent with climate change, in the case of Culicoides, rising temperatures favor vector spread (89). Like the other viruses mentioned here, there is no vaccine for OROV besides supportive treatment.
The emergence and distribution of vector-borne diseases is a consequence of the disturbance of the ecological balance, which is observed worldwide and to minimize this disturbance, vector control/arbovirus methodologies have emerged in recent years. Although the vector control programs are the best way to reduce vector and the mosquito-borne diseases, the results have not been satisfactory as well as the use of chemical control, which is the most commonly used method in recent times.
On the other hand, some new techniques for urban vector have called attention, for example, the use of the sterile insect technique, which involves the rearing and release of thousands of male mosquitoes carrying a lethal gene for its offspring. The other alternative control method involves the release of Wolbachia-carrying mosquitoes for blocking vector-borne pathogens. Studies showed that the introduction of Wolbachia into Ae. aegypti populations was effective in reducing the incidence of more than 60% of DENV and CHIKV and it is important to emphasize that this is a natural and self-sustaining technique (90–92).
Finally, this review intended to alert public health authorities worldwide to the importance of studies around viruses and their interactions with different vectors, improved methods for the differential diagnosis of arboviruses, mainly to MAYV and OROV, which are diseases that are commonly confused in epidemic outbreaks with other arboviruses. Another essential point that must be prioritized is the integration of different vector control methods, besides the development of vaccines.
Data Availability Statement
The original contributions presented in the study are included in the article/supplementary material. Further inquiries can be directed to the corresponding author.
Author Contributions
TP, FV, and JS contributed to the draft manuscript’s conception, design, and writing. LM supervised the research and edited the final version of the manuscript. All authors have read and agreed to the published version of the manuscript.
Conflict of Interest
The authors declare that the research was conducted in the absence of any commercial or financial relationships that could be construed as a potential conflict of interest.
Publisher’s Note
All claims expressed in this article are solely those of the authors and do not necessarily represent those of their affiliated organizations, or those of the publisher, the editors and the reviewers. Any product that may be evaluated in this article, or claim that may be made by its manufacturer, is not guaranteed or endorsed by the publisher.
Acknowledgments
We wish to thank the Institute René Rachou, World Mosquito Program, CNPq and FAPEMIG APQ-02760-17.
References
1. Anderson CR, Downs WG, Wattley GH, Ahin NW, Reese AA. Mayaro Virus: A New Human Disease Agent. Am J Trop Med Hyg (1957) 6(6):1012–6. doi: 10.4269/ajtmh.1957.6.1012
2. Mcarthur DB. Emerging Infectious Diseases. Nurs Clin NA (2019) 54(2):297–311. doi: 10.1016/j.cnur.2019.02.006
3. Lima-Camara TN. Emerging Arboviruses and Public Health Challenges in Brazil. Rev Saúde Pública (2016) 50:1–7. doi: 10.1590/S1518-8787.2016050006791
4. Jones R, Kulkarni MA, Davidson TMV. Arbovirus Vectors of Epidemiological Concern in the Americas: A Scoping Review of Entomological Studies on Zika, Dengue and Chikungunya Virus Vectors. PloS One (2020) 15(2):e0220753. doi: 10.1371/journal.pone.0220753
5. Diagne C. Mayaro Virus Pathogenesis and Transmission Mechanisms. Pathogens (2020) 9:1–23. doi: 10.3390/pathogens9090738
6. Gutierrez B, Wise EL, Pullan ST, Logue CH, Bowden TA, Escalera-Zamudio M, et al. Evolutionary Dynamics of Oropouche Virus in South America. J Virol (2020) 94(5):e01127-19. doi: 10.1128/JVI.01127-19
7. Casals J, Whitman A. Mayaro Virus: A New Human Disease Agent. Relationship to Other Arbor Viruses. Am J Trop Med Hyg (1957) 6(6):1004–11. doi: 10.4269/ajtmh.1957.6.1004
8. Azevedo RS, Silva EV, Carvalho VL, Rodrigues SG, Nunes-Neto JP, Monteiro H, et al. Mayaro Fever Virus, Brazilian Amazon. Emerg Infect Dis (2009) 15(11):1830–2. doi: 10.3201/eid1511.090461
9. Zhang R, Kim AS, Fox JM, Nair S, Basore K, Klimstra WB, et al. Mxra8 is a Receptor for Multiple Arthritogenic Alphaviruses. Nature (2018) 557(7706):570–4. doi: 10.1038/s41586-018-0121-3
10. Forshey BM, Guevara C, Laguna-Torres VA, Cespedes M, Vargas J, Gianella A, et al. Arboviral Etiologies of Acute Febrile Illnesses in Western South America, 2000-2007. PloS Negl Trop Dis (2010) 4(8):e787. doi: 10.1371/journal.pntd.0000787
11. Muñoz M, Navarro JC. Virus Mayaro: Un Arbovirus Reemergente En Venezuela Y Latinoamérica. Biomedica (2012) 32(2):286–302. doi: 10.7705/biomedica.v32i2.647
12. Llagonne-Barets M, Icard V, Leparc-Goffart I, Prat C, Perpoint T, André P, et al. A Case of Mayaro Virus Infection Imported From French Guiana. J Clin Virol (2016) 77(12):66–8. doi: 10.1016/j.jcv.2016.02.013
13. Serra OP, Cardoso BF, Ribeiro ALM, Santos FALD, Slhessarenko RD. Mayaro Virus and Dengue Virus 1 and 4 Natural Infection in Culicids From Cuiabá, State of Mato Grosso, Brazil. Mem do Inst Oswaldo Cruz (2016) 111(1):20–9. doi: 10.1590/0074-02760150270
14. Smith GC, Francy DB. Laboratory Studies of a Brazilian Strain of Aedes Albopictus as a Potential Vector of Mayaro and Oropouche Viruses. J Am Mosq Control Assoc (1991) 7(1):89–93.
15. Diop F, Alout H, Diagne CT, Bengue M, Baronti C, Hamel R, et al. Differential Susceptibility and Innate Immune Response of Aedes Aegypti and Aedes Albopictus to the Haitian Strain of the Mayaro Virus. Viruses (2019) 11(10):924. doi: 10.3390/v11100924
16. Pereira TN, Carvalho FD, De Mendonça SF, Rocha MN, Moreira LA. Vector Competence of Aedes Aegypti, Aedes Albopictus, and Culex Quinquefasciatus Mosquitoes for Mayaro Virus. PloS Negl Trop Dis (2020) 14(4):e0007518. doi: 10.1371/journal.pntd.0007518
17. Brustolin M, Pujhari S, Henderson CA, Rasgon JL. Anopheles Mosquitoes may Drive Invasion and Transmission of Mayaro Virus Across Geographically Diverse Regions. PloS Negl Trop Dis (2018) 12(11):e0006895. doi: 10.1371/journal.pntd.0006895
18. Dieme C, Ciota AT, Kramer LD. Transmission Potential of Mayaro Virus by Aedes Albopictus, and Anopheles Quadrimaculatus From the USA. Parasit Vectors (2020) 13(1):1–6. doi: 10.1186/s13071-020-04478-4
19. Zuchi N, Da Silva Heinen LB, Dos Santos MAM, Pereira FC, Slhessarenko RD. Molecular Detection of Mayaro Virus During a Dengue Outbreak in the State of Mato Grosso, Central-West Brazil. Mem do Inst Oswaldo Cruz (2014) 109(06):820–3. doi: 10.1590/0074-0276140108
20. Weaver SC, Reisen WK. Present and Future Arboviral Threats. Antiviral Res (2010) 85(2):328–45. doi: 10.1016/j.antiviral.2009.10.008
21. Gomes FA, Jansen AM, Machado Z, Pena J, Alves BF, Fumagalli J, et al. Serological Evidence of Arboviruses and Coccidia Infecting Horses in the Amazonian Region of Brazil. Plos One (2019) 14(12):e0225895. doi: 10.1016/j.antiviral.2009.10.008
22. Mota MTO, Vedovello D, Estofolete C, Malossi CD, Araújo JP Jr., Nogueira ML. Complete Genome Sequence of Mayaro Virus Imported From the Amazon Basin to Sao Paulo State, Brazil. Genome Announc (2015) 3(6):e01341–15. doi: 10.1128/genomeA.01341-15
23. Mourão MP, Bastos M, de Figueiredo RP, Gimaque JB, Galusso E, Kramer VM, et al. Mayaro Fever in the City of Manaus, Brazil, 2007-2008. Vector Borne Zoonotic Dis (Larchmont NY) (2012) 12(1):42–6. doi: 10.1089/vbz.2011.0669
24. Halsey ES, Siles C, Guevara C, Vilcarromero S, Jhonston EJ, Ramal C, et al. Mayaro Virus Infection, Amazon Basin Region, Peru, 2010-2013. Emerg Infect Dis (2013) 19(11):1839–42. doi: 10.3201/eid1911.130777
25. Strauss JH, Strauss EG. The Alphaviruses: Gene Expression, Replication, and Evolution. Microbiol Rev (1994) 58(3):491–562. doi: 10.1128/mr.58.3.491-562.1994
26. Lima WG, Pereira RS, da Cruz Nizer WS, Brito J, Godói IP, Cardoso VN, et al. Rate of Exposure to Mayaro Virus (MAYV) in Brazil Between 1955 and 2018: A Systematic Review and Meta-Analysis. Arch Virol (2021) 166(2):347–61. doi: 10.1007/s00705-020-04889-9
27. Long KC, Ziegler SA, Thangamani S, Hausser NL, Kochel TJ, Higgs S, et al. Experimental Transmission of Mayaro Virus by Aedes Aegypti. Am J Trop Med Hyg (2011) 85(4):750–7. doi: 10.4269/ajtmh.2011.11-0359
28. Auguste AJ, Liria J, Forrester NL, Giambalvo D, Moncada M, Long KC, et al. Evolutionary and Ecological Characterization of Mayaro Virus Strains Isolated During an Outbreak, Venezuela, 2010. Emerg Infect Dis (2015) 21(10):1742–50. doi: 10.3201/eid2110.141660
29. Powers AM, Aguilar PV, Chandler LJ, Brault AC, Meakins TA, Watts D, et al. Genetic Relationships Among Mayaro and Una Viruses Suggest Distinct Patterns of Transmission. Am J Trop Med Hyg (2006) 75(3):461–9. doi: 10.4269/ajtmh.2006.75.461
30. Lednicky J, De Rochars VM, Elbadry M, Loeb J, Telisma T, Chavannes S, et al. Mayaro Virus in Child With Acute Febrile Illness, Haiti, 2015. Emerg Infect Dis (2016) 22(11):2000–2. doi: 10.3201/eid2211.161015
31. Lorenz C, Ribeiro AF, Chiaravalloti-neto F. Acta Tropica Mayaro Virus Distribution in South America. Acta Trop (2019) 198(July):105093. doi: 10.1016/j.actatropica.2019.105093
32. Zahouli JB, Utzinger J, Adja MA, Müller P, Malone D, Tano Y, et al. Oviposition Ecology and Species Composition of Aedes Spp. And Aedes Aegypti Dynamics in Variously Urbanized Settings in Arbovirus Foci in Southeastern Côte D’ivoire. Parasit Vectors (2016) 9(1):1–14. doi: 10.1186/s13071-016-1778-9
33. Zahouli JBZ, Koudou BG, Müller P, Malone D, Tano Y, Utzinger J. (2017). Urbanization Is a Main Driver for the Larval Ecology of Aedes Mosquitoes in Arbovirus-Endemic Settings in South-Eastern Côte d’Ivoire. PLoS Negl Trop Dis 11(7):e0005751. doi: 10.1371/journal.pntd.0005751
34. Gomes FA, Jansen AM, Machado RZ, Jesus Pena HF, Fumagalli MJ, Silva A, et al. Serological Evidence of Arboviruses and Coccidia Infecting Horses in the Amazonian Region of Brazil. PloS One (2019) 14(12):e0225895. doi: 10.1371/journal.pone.0225895
35. Couto-Lima D, Madec Y, Bersot MI, Campos SS, de Albuquerque Motta M, Dos Santos FB, et al. Potential Risk of Re-Emergence of Urban Transmission of Yellow Fever Virus in Brazil Facilitated by Competent Aedes Populations. Sci Rep (2017) 7(1):1–12. doi: 10.1038/s41598-017-05186-3
36. Aguilar PV, Ampuero JS. Mayaro Virus Infection, Amazon Basin Region, Peru, 2010-2013. Emerg Infect Dis (2013) 19(11):1839–42. doi: 10.3201/eid1911.130777
37. Slegers CA, Keuter M, Günther S, Schmidt-Chanasit J, van der Ven AJ, de Mast Q. Persisting Arthralgia Due to Mayaro Virus Infection in a Traveler From Brazil: Is There a Risk for Attendants to the 2014 FIFA World Cup? J Clin Virol Off Publ Pan Am Soc Clin Virol (2014) 60(3):317–9. doi: 10.1016/j.jcv.2014.04.020
38. Appassakij H, Khuntikij P, Kemapunmanus M, Wutthanarungsan R, Silpapojakul K. Viremic Profiles in Asymptomatic and Symptomatic Chikungunya Fever: A Blood Transfusion Threat? Transfusion (2013) 53(10 Pt 2):2567–74. doi: 10.1111/j.1537-2995.2012.03960
39. Coimbra TL, Santos CL, Suzuki A, Petrella SM, Bisordi I, Nagamori AH, et al. Mayaro Virus: Imported Cases of Human Infection in São Paulo State, Brazil. Rev do Inst Med Trop Sao Paulo (2007) 49(4):221–4. doi: 10.1590/s0036-46652007000400005
40. Mackay IM, Scientist S, Arden KE. Mayaro Virus: A Forest Virus Primed for a Trip to the City? Forensic and Scientific Services SC. Microbes Infect (2016) 18(12):724–34. doi: 10.1016/j.micinf.2016.10.007 ISSN 1286-4579.
41. Acosta-Ampudia Y, Monsalve DM, Rodríguez Y, Pacheco Y, Anaya JM, Ramírez-Santana C. Mayaro: An Emerging Viral Threat? Emerg Microbes Infect (2018) 7(1):163. doi: 10.1038/s41426-018-0163-5
42. Zacks MA, Paessler S. Encephalitic Alphaviruses. Vet Microbiol (2010) 140(3-4):281–6. doi: 10.1016/j.vetmic.2009.08.023
43. Neumayr A, Gabriel M, Fritz J, Günther S, Hatz C, Schmidt-Chanasit J, et al. Mayaro Virus Infection in Traveler Returning From Amazon Basin, Northern Peru. Emerg Infect Dis (2012) 18(4):695–6. doi: 10.3201/eid1804.111717
44. Waggoner JJ, Rojas A, Mohamed-Hadley A, de Guillén YA, Pinsky BA. Real-Time RT-PCR for Mayaro Virus Detection in Plasma and Urine. J Clin Virol (2018) 1–4. doi: 10.1016/j.jcv.2017.11.006
45. Fischer C, Bozza F, Merino Merino XJ, Pedroso C, de Oliveira Filho EF, Moreira-Soto A, et al. Robustness of Serologic Investigations for Chikungunya and Mayaro Viruses Following Coemergence. mSphere (2020) 5(1):e00915–19. doi: 10.1128/mSphere.00915-19
46. Chisenga CC, Bosomprah S, Musukuma K, Mubanga C, Chilyabanyama ON, Velu RM, et al. Sero-Prevalence of Arthropod-Borne Viral Infections Among Lukanga Swamp Residents in Zambia. PloS One (2020) 15(7):e0235322. doi: 10.1371/journal.pone.0235322
47. Choi H, Kudchodkar SB, Reuschel EL, Asija K, Borole P, Ho M, et al. Protective Immunity by an Engineered DNA Vaccine for Mayaro Virus. PloS Negl Trop Dis (2019) 13(2):e0007042. doi: 10.1371/journal.pntd.0007042
48. Weise WJ, Hermance ME, Forrester N, Adams AP, Langsjoen R, Gorchakov R, et al. A Novel Live-Attenuated Vaccine Candidate for Mayaro Fever. PloS Negl Trop Dis (2014) 8(8):e2969. doi: 10.1371/journal.pntd.0002969
49. Hart TJ, Kohl A, Elliott RM. Role of the NSs Protein in the Zoonotic Capacity of Orthobunyaviruses. Zoonoses Public Health (2009) 56(6-7):285–96. doi: 10.1111/j.1863-2378.2008.01166.x
50. Travassos da Rosa JF, de Souza WM, Pinheiro FP, Figueiredo ML, Cardoso JF, Acrani GO, et al. Oropouche Virus: Clinical, Epidemiological, and Molecular Aspects of a Neglected Orthobunyavirus. Am J Trop Med Hyg (2017) 96(5):1019–30. doi: 10.4269/ajtmh.16-0672
51. Elliott RM. Orthobunyaviruses: Recent Genetic and Structural Insights. Nat Rev Microbiol (2014) 12(10):673–85. doi: 10.1038/nrmicro3332
52. Tilston-Lunel NL, Acrani GO, Randall RE, Elliott RM. Generation of Recombinant Oropouche Viruses Lacking the Nonstructural Protein NSm or NSs. J Virol (2015) 90(5):2616–27. doi: 10.1128/JVI.02849-15
53. Bowden TA, Bitto D, McLees A, Yeromonahos C, Elliott RM, Huiskonen JT. Orthobunyavirus Ultrastructure and the Curious Tripodal Glycoprotein Spike. PloS Pathog (2013) 9(5):e1003374. doi: 10.1371/journal.ppat.1003374
54. Hellert J, Aebischer A, Wernike K, Haouz A, Brocchi E, Reiche S, et al. Orthobunyavirus Spike Architecture and Recognition by Neutralizing Antibodies. Nat Commun (2019) 10(1):879. doi: 10.1038/s41467-019-08832-8
55. Moreli ML, Aquino VH, Cruz AC, Figueiredo LT. Diagnosis of Oropouche Virus Infection by RT-Nested-PCR. J Med Virol (2002) 66(1):139–42. doi: 10.1002/jmv.2122
56. Ladner JT, Savji N, Lofts L, Travassos da Rosa A, Wiley MR, Gestole MC, et al. Genomic and Phylogenetic Characterization of Viruses Included in the Manzanilla and Oropouche Species Complexes of the Genus Orthobunyavirus, Family Bunyaviridae. J Gen Virol (2014) 95(Pt 5):1055–66. doi: 10.1099/vir.0.061309-0
57. Saeed MF, Wang H, Suderman M, Beasley DW, Travassos da Rosa A, Li L, et al. Jatobal Virus is a Reassortant Containing the Small RNA of Oropouche Virus. Virus Res (2001) 77(1):25–30. doi: 10.1016/s0168-1702(01)00262-3
58. Tilston-Lunel NL, Shi X, Elliott RM, Acrani GO. The Potential for Reassortment Between Oropouche and Schmallenberg Orthobunyaviruses. Viruses (2017) 9(8):220. doi: 10.3390/v9080220
59. Vasconcelos HB, Nunes MR, Casseb LM, Carvalho VL, Pinto da Silva EV, Silva M, et al. Molecular Epidemiology of Oropouche Virus, Brazil. Emerg Infect Dis (2011) 17(5):800–6. doi: 10.3201/eid1705.101333
60. Nunes MR, Martins LC, Rodrigues SG, Chiang JO, Azevedo R, da Rosa AP, et al. Oropouche Virus Isolation, Southeast Brazil. Emerg Infect Dis (2005) 11(10):1610–3. doi: 10.3201/eid1110.050464
61. Casseb AR, Brito TC, Rogéria M, Chiang JO, Martins LC, Patroca S, et al. Prevalence of Antibodies to Equine Alphaviruses in the State of Pará, Brazil. Arq do Inst Biol (2016) 83. doi: 10.1590/1808-1657000202014
62. Romero-Alvarez D, Escobar LE. Vegetation Loss and the 2016 Oropouche Fever Outbreak in Peru. Mem do Inst Oswaldo Cruz (2017) 112(4):292–8. doi: 10.1590/0074-02760160415
63. Sakkas H, Bozidis P, Franks A, Papadopoulou C. Oropouche Fever: A Review. Viruses (2018) 10(4):175. doi: 10.3390/v10040175
64. Anderson CR, Spence L, Downs WG, Aitken TH. Oropouche Virus: A New Human Disease Agent From Trinidad, West Indies. Am J Trop Med Hyg (1961) 10:574–8. doi: 10.4269/ajtmh.1961.10.574
65. Romero-Alvarez D, Escobar LE. Oropouche Fever, an Emergent Disease From the Americas. Microbes Infect (2018) 20(3):135–46. doi: 10.1016/j.micinf.2017.11.013
66. Pinheiro FP, Hoch AL, Gomes ML, Roberts DR. Oropouche Virus. IV. Laboratory Transmission by Culicoides Paraensis. Am J Trop Med Hyg (1981) 30(1):172–6. doi: 10.4269/ajtmh.1981.30.172
67. Linley JR, Hoch AL, Pinheiro FP. Biting Midges (Diptera: Ceratopogonidae) and Human Health. J Med Entomol (1983) 20(4):347–64. doi: 10.1093/jmedent/20.4.347
68. Mellor PS, Boorman J, Baylis M. Culicoides Biting Midges: Their Role as Arbovirus Vectors. Annu Rev Entomol (2000) 45:307–40. doi: 10.1146/annurev.ento.45.1.307
69. Roberts DR, Hoch AL, Dixon KE, Llewellyn CH. Oropouche Virus. III. Entomological Observations from Three Epidemics in Para, Brazil, 1975. Am J Trop Med Hyg (1981) 30(1):165–71. doi: 10.4269/ajtmh.1981.30.165
70. Souza Bastos M, Figueiredo LTM, Naveca FG, Monte RL, Lessa N, de Figueiredo RMP, et al. (2012). Identification of Oropouche Orthobunyavirus in the Cerebrospinal Fluid of Three Patients in the Amazonas, Brazil. Am J Trop Med Hyg 86(4):732. doi: 10.4269/ajtmh.2012.11-0485
71. Mercer DR, Castillo-Pizango MJ. Changes in Relative Species Compositions of Biting Midges (Diptera: Ceratopogonidae) and an Outbreak of Oropouche Virus in Iquitos, Peru. J Med Entomol (2005) 42(4):554–8. doi: 10.1093/jmedent/42.4.554
72. Mendonça SF, Rocha MN, Ferreira FV, Leite T, Amadou S, Sucupira P, et al. Evaluation of Aedes Aegypti, Aedes Albopictus, and Culex Quinquefasciatus Mosquitoes Competence to Oropouche Virus Infection. Viruses (2021) 13(5):755. doi: 10.3390/v13050755
73. Cardoso BF, Serra OP, Da Silva Heinen LB, Zuchi N, De Souza VC, Naveca FG, et al. Detection of Oropouche Virus Segment s in Patients and in Culex Quinquefasciatus in the State of Mato Grosso, Brazil. Mem Inst Oswaldo Cruz (2015) 110(6):745–54. doi: 10.1590/0074-02760150123
74. Vasconcelos PF, Travassos Da Rosa JF, Guerreiro SC, Dégallier N, Travassos Da Rosa ES, Travassos Da Rosa AP. Primeiro Registro De Epidemias Causadas Pelo Vírus Oropouche Nos Estados do Maranhão E Goiás, Brasil [1st Register of an Epidemic Caused by Oropouche Virus in the States of Maranhão and Goiás, Brazil]. Rev do Inst Med Trop Sao Paulo (1989) 31(4):271–8. doi: 10.1590/s0036-46651989000400011
75. Wise EL, Pullan ST, Márquez S, Paz V, Mosquera JD, Zapata S, et al. Isolation of Oropouche Virus From Febrile Patient, Ecuador. Emerg Infect Dis (2018) 24(5):935–7. doi: 10.3201/eid2405.171569
76. Baisley KJ, Watts DM, Munstermann LE, Wilson ML. Epidemiology of Endemic Oropouche Virus Transmission in Upper Amazonian Peru. Am J Trop Med Hyg (1998) 59(5):710–6. doi: 10.4269/ajtmh.1998.59.710
77. Martins-Luna J, Del Valle-Mendoza J, Silva-Caso W, Sandoval I, Del Valle LJ, Palomares-Reyes C, et al. Oropouche Infection a Neglected Arbovirus in Patients With Acute Febrile Illness From the Peruvian Coast. BMC Res Notes (2020) 13(1):67. doi: 10.1186/s13104-020-4937-1
78. Watts DM, Phillips I, Callahan JD, Griebenow W, Hyams KC, Hayes CG. Oropouche Virus Transmission in the Amazon River Basin of Peru. Am J Trop Med Hyg (1997) 56(2):148–52. doi: 10.4269/ajtmh.1997.56.148
79. Silva-Caso W, Aguilar-Luis MA, Palomares-Reyes C, Mazulis F, Weilg C, Del Valle LJ, et al. First Outbreak of Oropouche Fever Reported in a non-Endemic Western Region of the Peruvian Amazon: Molecular Diagnosis and Clinical Characteristics. Int J Infect Dis IJID Off Publ Int Soc Infect Dis (2019) 83:139–44. doi: 10.1016/j.ijid.2019.04.011
80. Manock SR, Jacobsen KH, de Bravo NB, Russell KL, Negrete M, Olson JG, et al. Etiology of Acute Undifferentiated Febrile Illness in the Amazon Basin of Ecuador. Am J Trop Med Hyg (2009) 81(1):146–51. doi: 10.4269/ajtmh.2009.81.146
81. Vogels CB, Göertz GP, Pijlman GP, Koenraadt CJ. Vector Competence of European Mosquitoes for West Nile Virus. Emerg Microbes Infect (2017) 6(11):e96. doi: 10.1038/emi.2017.82
82. Sánchez-Carbonel J, Tantaléan-Yépez D, Aguilar-Luis MA, Silva-Caso W, Weilg P, Vásquez-Achaya F, et al. Identification of Infection by Chikungunya, Zika, and Dengue in an Area of the Peruvian Coast. Molecular Diagnosis and Clinical Characteristics. BMC Res Notes (2018) 11(1):175. doi: 10.1186/s13104-018-3290-0
83. Naveca FG, Nascimento V, Souza VC, Nunes B, Rodrigues D, Vasconcelos P. Multiplexed Reverse Transcription Real-Time Polymerase Chain Reaction for Simultaneous Detection of Mayaro, Oropouche, and Oropouche-Like Viruses. Mem do Inst Oswaldo Cruz (2017) 112(7):510–3. doi: 10.1590/0074-02760160062
84. Briese T, Bird B, Kapoor V, Nichol ST, Lipkin WI. Batai and Ngari Viruses: M Segment Reassortment and Association With Severe Febrile Disease Outbreaks in East Africa. J Virol (2006) 80(11):5627–30. doi: 10.1128/JVI.02448-05
85. Saxena V, Bolling BG, Wang T. West Nile Virus. Clinics Lab Med (2017) 37(2):243–52. doi: 10.1016/j.cll.2017.01.001
86. Adhikari UK, Tayebi M, Rahman MM. Immunoinformatics Approach for Epitope-Based Peptide Vaccine Design and Active Site Prediction Against Polyprotein of Emerging Oropouche Virus. J Immunol Res (2018) 2018:6718083. doi: 10.1155/2018/6718083
87. Gould E, Pettersson J, Higgs S, Charrel R, de Lamballerie X. Emerging Arboviruses: Why Today? One Health (Amsterdam Netherlands) (2017) 4:1–13. doi: 10.1016/j.onehlt.2017.06.001
88. Ribeiro-Filho HV, Coimbra LD, Cassago A, Rocha R, Guerra J, de Felicio R, et al. Cryo-EM Structure of the Mature and Infective Mayaro Virus at 4.4 Å Resolution Reveals Features of Arthritogenic Alphaviruses. Nat Commun (2021) 12(1):3038. doi: 10.1038/s41467-021-23400-9
89. Purse BV, Carpenter S, Venter GJ, Bellis G, Mullens BA. Bionomics of Temperate and Tropical Culicoides Midges: Knowledge Gaps and Consequences for Transmission of Culicoides-Borne Viruses. Annu Rev Entomol (2015) 60:373–92. doi: 10.1146/annurev-ento-010814-020614
90. Pinto SB, Riback TIS, Sylvestre G, Costa G, Peixoto J, Dias FB, et al. Effectiveness of Wolbachia-Infected Mosquito Deployments in Reducing the Incidence of Dengue and Other Aedes-Borne Diseases in Niterói, Brazil: A Quasi-Experimental Study. medRxiv (2021). doi: 10.1101/2021.01.31.21250726
91. Gesto J, Ribeiro GS, Rocha MN, Dias F, Peixoto J, Carvalho FD, et al. Reduced Competence to Arboviruses Following the Sustainable Invasion of Wolbachia Into Native Aedes Aegypti From Southeastern Brazil. Sci Rep (2021) 11(1):10039. doi: 10.1038/s41598-021-89409-8
Keywords: arbovirus, neglected diseases, arthropod-borne diseases, tropical disease, public health
Citation: Pereira TN, Virginio F, Souza JI and Moreira LA (2021) Emergent Arboviruses: A Review About Mayaro virus and Oropouche orthobunyavirus. Front. Trop. Dis 2:737436. doi: 10.3389/fitd.2021.737436
Received: 07 July 2021; Accepted: 27 September 2021;
Published: 08 November 2021.
Edited by:
Mariana De Niz, Universidade de Lisboa, PortugalReviewed by:
Marcos Antônio Bezerra-Santos, University of Bari Aldo Moro, ItalyClaudia Rückert, University of Nevada, Reno, United States
Copyright © 2021 Pereira, Virginio, Souza and Moreira. This is an open-access article distributed under the terms of the Creative Commons Attribution License (CC BY). The use, distribution or reproduction in other forums is permitted, provided the original author(s) and the copyright owner(s) are credited and that the original publication in this journal is cited, in accordance with accepted academic practice. No use, distribution or reproduction is permitted which does not comply with these terms.
*Correspondence: Luciano Andrade Moreira, luciano.andrade@fiocruz.br
†These authors have contributed equally to this work and share first authorship