- 1Biomedical Research Center (BRC), QU Health, Qatar University, Doha, Qatar
- 2Department of Basic Medical Sciences, College of Medicine, QU Health, Qatar University, Doha, Qatar
- 3College of Health Sciences, QU Health, Qatar University, Doha, Qatar
Background: The cross-protective nature of Bacillus Calmette-Guerin (BCG) vaccine against SARS-CoV-2 virus was previously suggested, however its effect in COVID-19 patients with type 2 diabetes (T2D) and the underlying metabolic pathways has not been addressed. This study aims to investigate the difference in the metabolomic patterns of type 2 diabetic patients with BCG vaccination showing different severity levels of COVID-19 infection.
Methods: Sixty-seven COVID-19 patients were categorized into diabetic and non-diabetic individuals who had been previously vaccinated or not with BCG vaccination. Targeted metabolomics were performed from serum samples from all patients using tandem mass spectrometry. Statistical analysis included multivariate and univariate models.
Results: Data suggested that while BCG vaccination may provide protection for individuals who do not have diabetes, it appears to be linked to more severe COVID-19 symptoms in T2D patients (p = 0.02). Comparing the metabolic signature of BCG vaccinated T2D individuals to non-vaccinated counterparts revealed that amino acid (sarcosine), cholesterol esters (CE 20:0, 20:1, 22:2), carboxylic acid (Aconitic acid) were enriched in BCG vaccinated T2D patients, whereas spermidine, glycosylceramides (Hex3Cer(d18:1_22:0), Hex2Cer(d18:1/22:0), HexCer(d18:1/26:1), Hex2Cer(d18:1/24:0), HexCer(d18:1/22:0) were higher in BCG vaccinated non- T2D patients. Furthermore, data indicated a decrease in sarcosine synthesis from glycine and choline and increase in spermidine synthesis in the BCG vaccinated cohort in T2D and non-T2D groups, respectively.
Conclusion: This pilot study suggests increased severity of COVID-19 in BCG vaccinated T2D patients, which was marked by decreased sarcosine synthesis, perhaps via lower sarcosine-mediated removal of viral antigens.
1 Introduction
Severe acute respiratory syndrome coronavirus 2 (SARS-CoV-2) emerged as a novel human pathogen that led to world’s leading pandemic in the year 2019 (1). Bacille Calmette-Guerin (BCG) vaccination at infancy was suggested to alleviate the severe impact of the pandemic in some countries (2).
BCG vaccine was developed by Albert Calmette and Camile Guérin to protect against Mycobacterium tuberculosis infection, otherwise called Tuberculosis (TB). By procuring the attenuated strain of the bacteria Mycobacterium bovis, followed by several clinical trials, BCG vaccination has proved to be dependable (3). Much later into its introduction, it was reported that BCG was not only effective against Mycobacterium tuberculosis but also protective against several other infections in various studies (4, 5). BCG administration has shown a 45% reduction in mortality rate in West Africa (6) and decreased child mortality in Sweden (7) possibly due to its non-specific protective nature. These pilot studies suggested that BCG vaccine was responsible for lower incidence of neonatal sepsis and pulmonary infections that decreased infant mortality (8). Other examples include protection against yellow fever virus (9), HPV (human papilloma virus) (10), HSV (herpes simplex virus) (11), RSV (respiratory syncytial virus) (12) and Influenza A (H1N1) (13, 14). Automatously, the cross-protective effect of BCG vaccination can be delineated, partially, by training innate immunity based on temporary epigenetic reprogramming of macrophages, which enables them to produce more inflammatory cytokines, triggering powerful immunological responses (15). This innate immunity training mechanism was explained several years ago (9). Furthermore, the BCG vaccine also offers protection against immunological disorders including type 1 diabetes and multiple sclerosis (16–18). The mechanics underlying these numerous advantages are still being actively researched by scientists.
The controversy over whether the BCG vaccine can impact the immune response against the virus or other unrelated diseases was reignited in 2019 with the onset of the severe acute respiratory syndrome coronavirus (SARS-CoV-2) pandemic (15). The BCG vaccine is the only vaccine licensed against TB and due to the above observations regarding its cross-protectivity, it is proposed that many low- and lower-middle-income nations would probably have the infrastructure and medical staff necessary to distribute the novel BCG-based COVID-19 vaccine on a large-scale. This shows that BCG may be able to get over any remaining obstacles to vaccine adoption in nations with weak health-care systems and potentially catastrophic coronavirus consequences (14, 19). Although the effect of BCG against COVID-19 infection remains controversial due to minimal number of published clinical trials (2).
Currently, the impact of BCG vaccinations seems to be largely hypothetical or non-specific in decreasing the severity of COVID-19 infection. Our previous study suggested a difference in the metabolic signature of Type 2 diabetic patients with COVID-19 infection compared to non-diabetic population (20). In general, diabetic patients are at a greater risk of disease progression when infected with SARS-CoV-2. Compared to SARS-CoV-2-infected non-diabetic people, diabetics have a higher rate of hospital admission, severe pneumonia, and worse mortality rate (21, 22). A meta-analysis revealed that diabetes raises the probability of severity by 2.3 times and the risk of the COVID-19-related death by 2.5 times, making it a main contributing factor to the poor prognosis in COVID-19 (23). In this context, the effect of BCG vaccination should be evaluated in diabetic patients with COVID-19. Since an altered metabolic profile is evident in COVID-19 patients, there might be altered expression of disease in diabetic patients with respect to BCG vaccination. The objective of this study is to investigate the difference in the metabolic patterns of type 2 diabetic patients with BCG vaccination showing different levels of COVID-19 infection and identify the underlying metabolic pathways.
2 Methods
2.1 Study design
The study included 67 patients diagnosed with SARS-CoV-2 infection at Hamad Medical Corporation (HMC, Doha) between the period of June 2020 and March 2021. Participants were included if they were between 35 and 65 years of age, had a positive SARS-CoV-2 PCR test result (with a CT value <30), and were residents of Qatar. Upper respiratory tract specimens, specifically throat and nasopharyngeal swabs, were collected and tested for SARS-CoV-2 using the TaqPath COVID-19 Combo Kit (Thermo Fisher Scientific, Waltham, Massachusetts) or Cobas SARS-CoV-2 Test (Roche Diagnostics, Rotkreuz, Switzerland). The study recruited all consenting patients from Hammad Medical Corporation. Protocols were approved by the Institutional Review Boards (IRBs) of HMC (MRC-01-20-145) and Qatar University (QU-IRB 1289-EA/20). The patients were clinically identified as symptomatic, mild, moderate and severe based on WHO’s COVID-19 guidelines (24). For analysis, the cohort were categorized into Type 2 diabetic (T2D) and non-diabetic (non-T2D) and on the basis of BCG vaccination status as: BCG+/T2D (n=26), BCG-/T2D (n=8), BCG+/non-T2D (n=23), BCG-/non-T2D (n=10) and further dichotomized as mild and moderate-severe cases. The moderate and severe cases were grouped to differentiate RT PCR positive cases with no clinical findings (mild) from those with respiratory symptoms, pulmonary involvement (moderate), and SpO2 <94% on room air (severe). Figure 1 shows the categorization of study participants in each of the groups. Blood samples were drawn during the diagnosis, before quarantine, or hospitalization. Patients exhibiting moderate to severe disease symptoms were given inpatient treatment. Two patients in the severe group died due to respiratory failure. Data regarding the clinical parameters were retrieved from the hospital’s healthcare system following patients consent, which includes Body Mass Index (BMI), SARS-CoV-2 viral load, complete blood count (CBC), and specific blood tests.
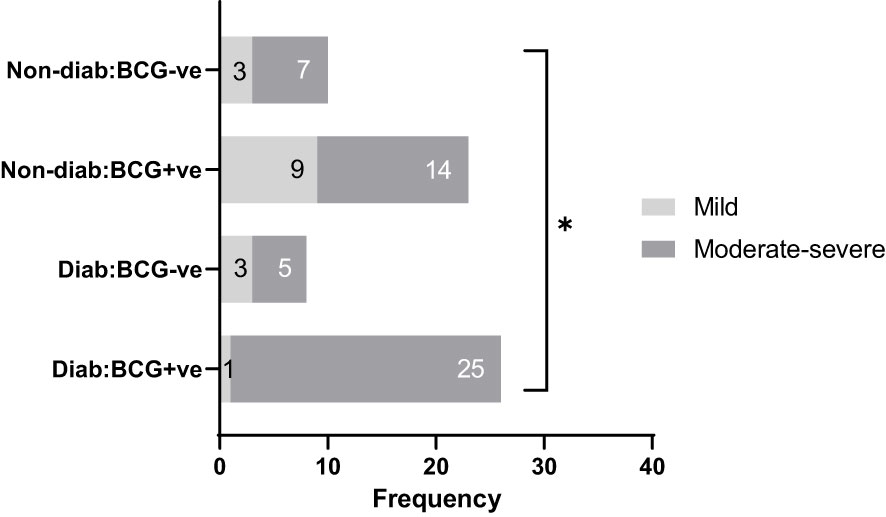
Figure 1 Study participants classified by disease severity (mild and moderate-severe), diabetes status (diabetic, non-diabetic), and BCG vaccination status (positive, negative). * signifies p-value <0.05.
2.2 Metabolomics
Using the Biocrates MxP® Quant 500 Kit (Biocrates, Innsbruck, Austria), assessed by tandem mass spectrometry at the Fraunhofer Institute for Toxicology and Experimental Medicine, targeted metabolomics was performed on serum samples obtained from all subjects between 24 and 48 h after diagnosis. As part of the MetIDQ™ MetaboINDICATOR™ module created especially for MxP® Quant 500 kit data, 630 metabolites were evaluated. Additionally, 202 metabolite-indicators were created from combinations of metabolite measurements that encapsulate significant biological processes, for example indicators of enzymatic activity (substrate/product metabolite ratio) and sum of functionally or structurally similar metabolites. Quantification of lipids and small molecules were performed using Flow injection Analysis Tandem Mass Spectrometry (FIA-MS/MS) and liquid chromatography-tandem mass spectrometry (LC-MS/MS) respectively, using 5500 QTRAP® instrument triple quadrupole mass spectrometer (AB Sciex, Darmstadt, Germany). The “_” signifies that the fatty acid residue locations (sn-1/sn-2/sn-3) for the listed triacylglycerols are unknown. Potential isomers are described by the manufacturer: https://biocrates.com/wp-content/uploads/2020/02/Biocrates_Q500_isomers_isobars.pdf.
2.3 Statistical analysis
The metabolite measurements were log transformed and orthogonal partial least squares discriminant analysis (OPLS-DA) was performed using SIMCA (v.16). Linear regression model was run separately for T2D and non-T2D groups using RStudio version 4.2.1 with metabolites depicted as y-variable against BCG vaccination status while correcting for age and gender to deal with the imbalanced data. The nominal p-values were corrected for multiple testing using the false discovery rate (FDR) method. Metabolite classes were defined by Biocrates and those with more than three numbers were selected for functional enrichment analysis, which was conducted on list of metabolites ordered by p-value from the linear regression models using one-way Wilcoxon sum of ranks test and adjusted for multiple testing using FDR method. Metabolism indicators were excluded in functional enrichment analysis.
3 Results
3.1 Clinical characteristics of participants
As depicted in Table 1, COVID-19 patients with different severity levels (categorized as mild, moderate-severe) were classified according to their previous BCG vaccination and diabetes mellitus statuses. There was a significant difference between the 4 groups in terms of COVID-19 severity (p <0.05). Out of 34 diabetic patients, individuals with BCG vaccination (25, 96.15%) had higher moderate-severe cases than non-BCG group (5, 62.5%) (p < 0.05). On the other hand, in the non-diabetic population (n = 33) there was no significant difference between BCG vaccinated groups (14, 61%) and its non-vaccinated counterpart (7, 70%) with regard to COVID-19 severity. The comorbidities associated with diabetes and COVID-19 (hypertension, COPD, cardiovascular disease, cerebrovascular disease, and asthma) are depicted in Table 1 which shows no significant difference in their proportions among the study populations. A higher incidence of dyslipidemia was observed among diabetic individuals compared to non-diabetic individuals (p 0.0046). However, further investigation revealed no significant difference in the prevalence of dyslipidemia between BCG-positive and BCG-negative diabetic individuals (p 0.409).
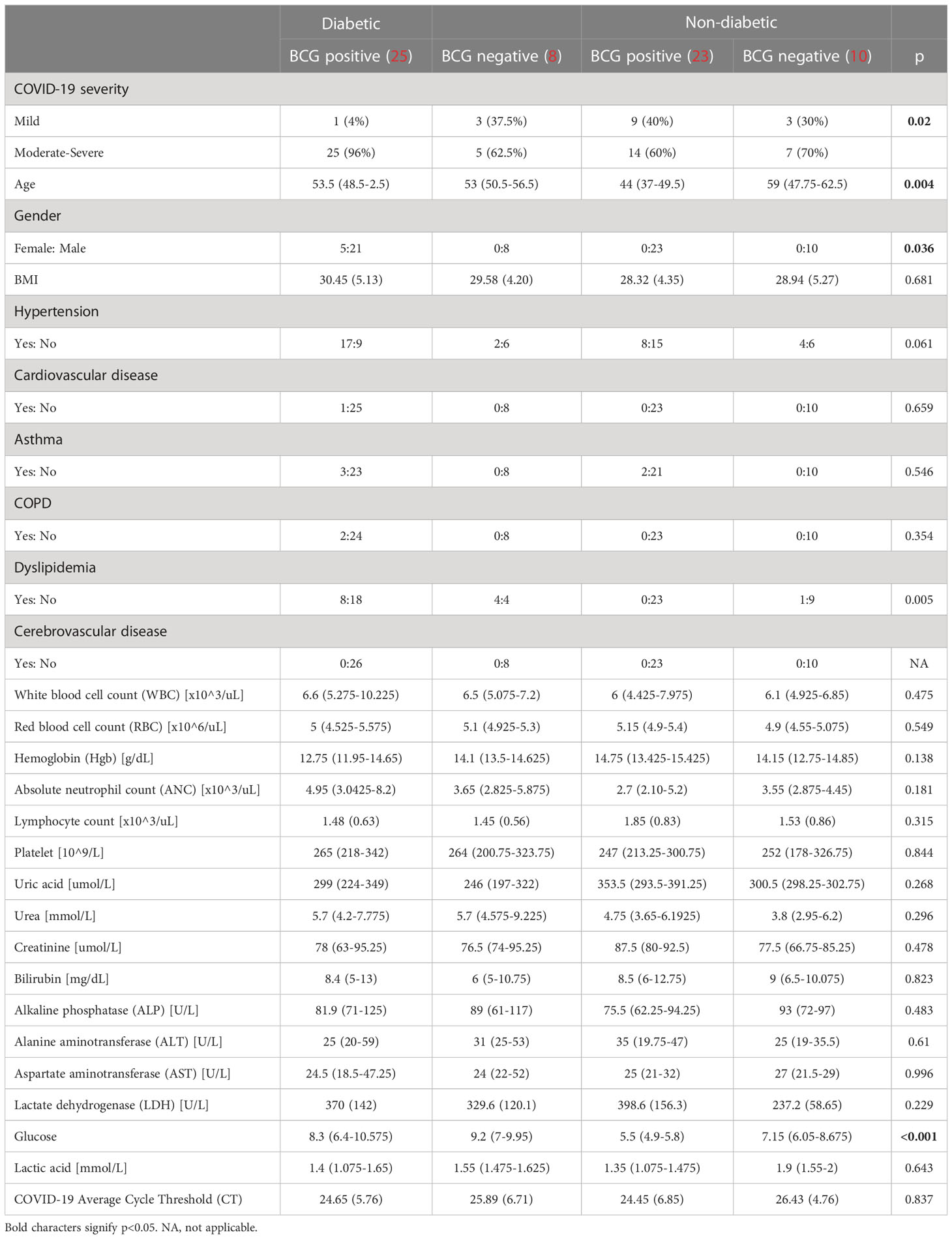
Table 1 Demographic characteristics of study participants categorized by diabetic status and BCG vaccination (positive and negative).
3.2 Metabolic signature of BCG vaccination in diabetic and non-diabetic groups with COVID-19 infection
Orthogonal partial least squares discriminant analysis (OPLS-DA) was conducted to capture the metabolic profile of BCG positive vs BCG negative with different levels of COVID-19 severity in diabetic and non-diabetic individuals. Data revealed a clear separation between BCG positive and BCG negative in diabetic patients (R2Y = 0.69) (Figure 2A), and in non-diabetic patients (R2Y = 0.45) (Figure 2B). The corresponding loadings plot for each OPLS-DA models revealed lower abundance of cholesterols esters/glycosylceramides in BCG-positive diabetic (Figure 2C)/non-diabetic (Figure 2D) patients, respectively.
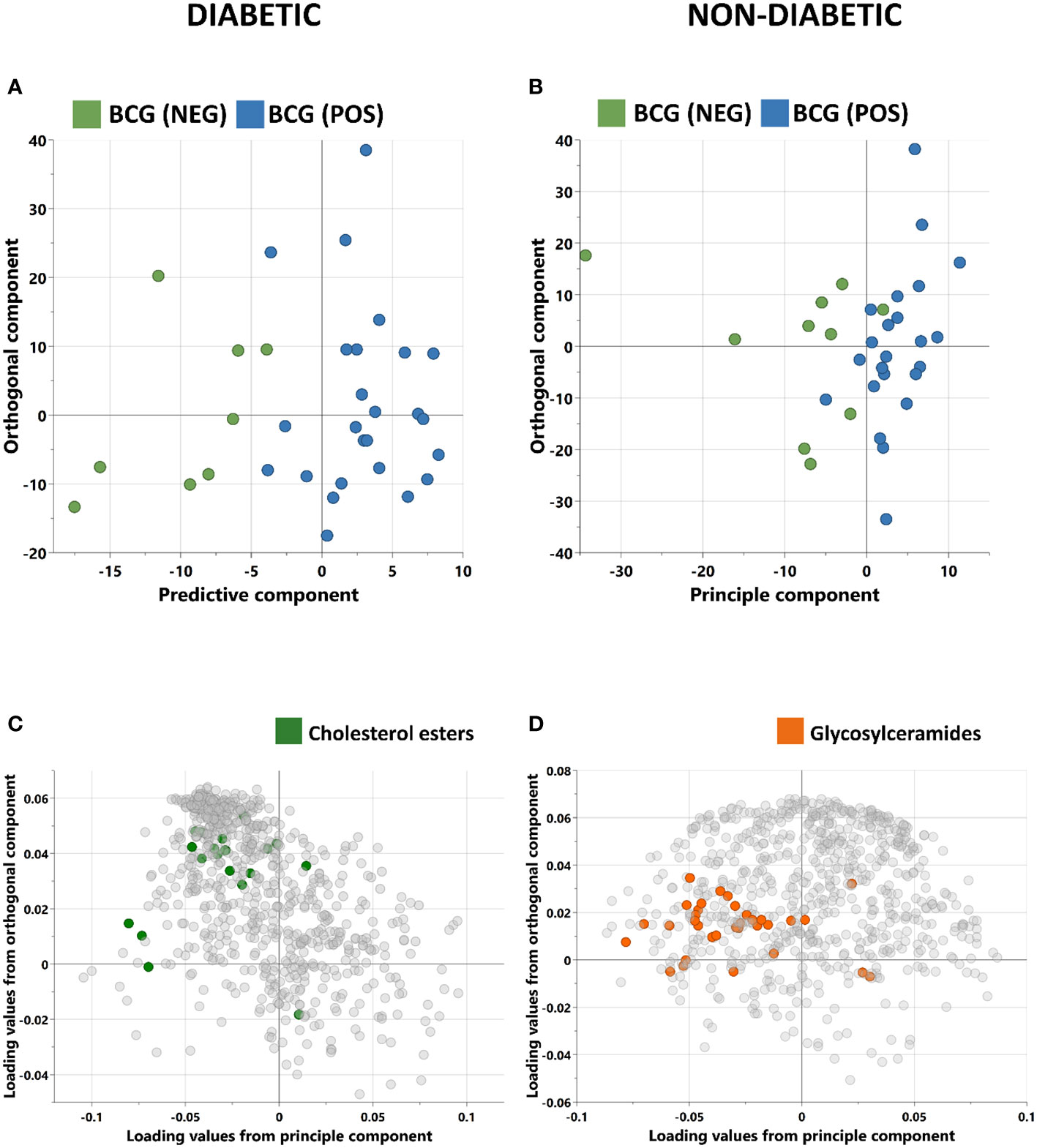
Figure 2 Score plots of OPLS-DA models discriminating between BCG vaccinated (BCG-pos) and non-vaccinated (BCG-neg) patients with COVID-19 infection. The analysis was performed separately for (A) Diabetic individuals (B) non-diabetic individuals. OPLS-DA identified one predictive and one orthogonal component for each model. (C, D) are the respective loading plots for diabetic and non-diabetic COVID-19 patients with highlighted enriched pathways.
3.3 Metabolites associated with BCG vaccination in T2D patients
Targeted metabolomics of serum samples from 34 diabetic patients was employed to elucidate the metabolites that differentiated between BCG-positive and BCG-negative individuals with COVID-19. Linear regression analysis provided several nominally significant (p < 0.01) metabolic changes between the two groups in diabetic individuals (Table 2). The altered metabolic profile involved decreased level of sarcosine, metabolic indicators related to sarcosine, cholesterol esters (CE 20:0, 20:1, 22:2) and increased arachidonic acid, acylcarnitine (C12) in the BCG-positive diabetic group. Figures 3 shows examples of significantly different levels of these metabolites. Enrichment analysis revealed changes in cholesterol esters pathway in BCG positive patients compared to BCG negative patients at the nominal level of significance (p < 0.01).
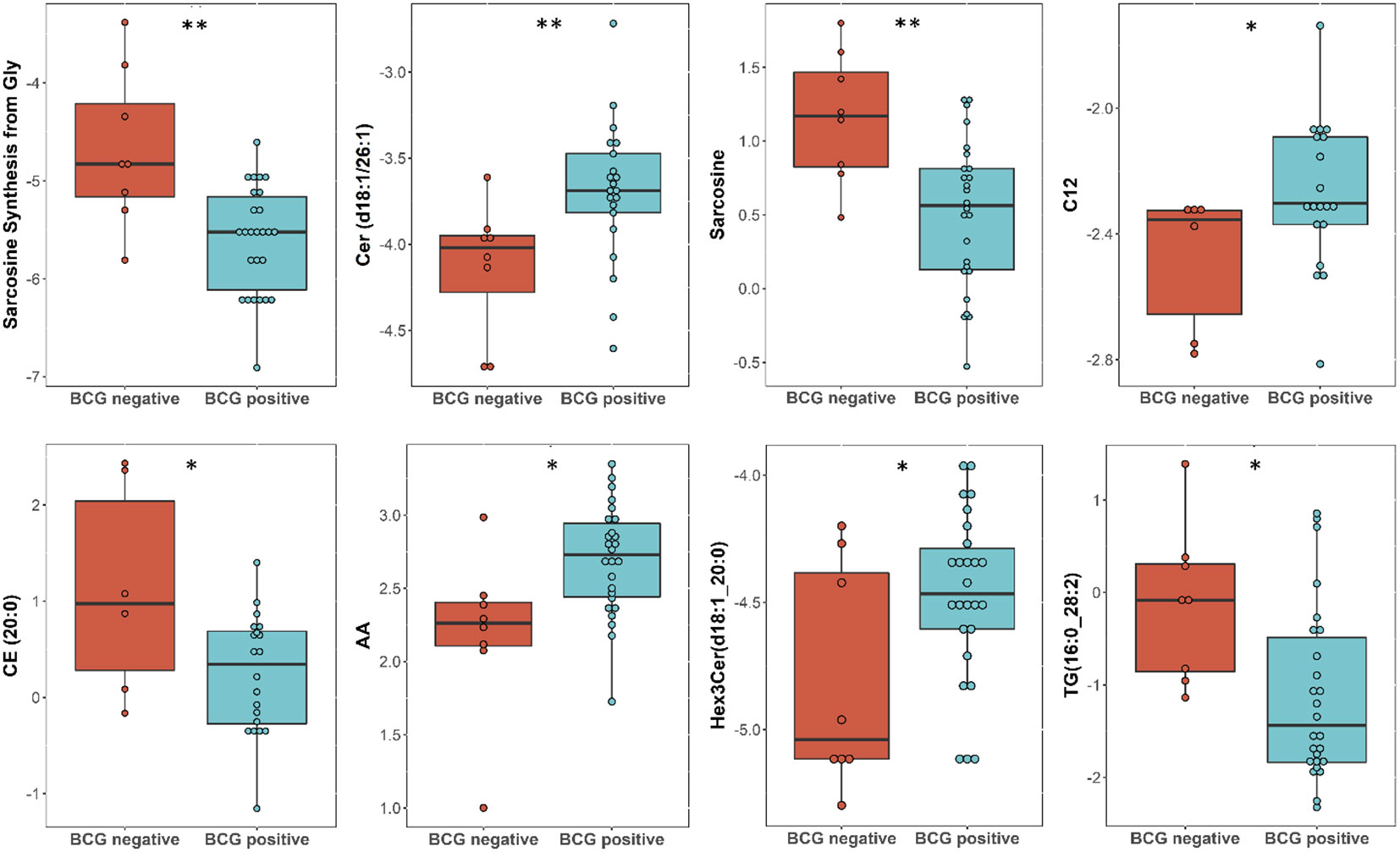
Figure 3 Metabolites associated with BCG vaccination in T2D patients with different levels of COVID-19 severity. **/* signifies <0.01/<0.05 p value.
3.4 Metabolites associated with BCG vaccination in non-T2D patients
Linear regression revealed several nominally (≤0.001) significant changes between the vaccinated and non-vaccinated groups (Table 3). These alterations include the difference in the levels of various glycosylceramides, triacylglycerols, amino acid related metabolites such as cystine, HCys, alpha-aminobutyric acid (AABA), and Betaine. Figure 4 shows examples of the top nominally significant levels of these based on vaccination status in non-diabetic patients. Enrichment analysis revealed changes in the glycosylceramide pathway in BCG vaccinated individuals compared to non-vaccinated counterpart (p = 0.01). Other significant pathways include amino acid related metabolites (p = 0.015).
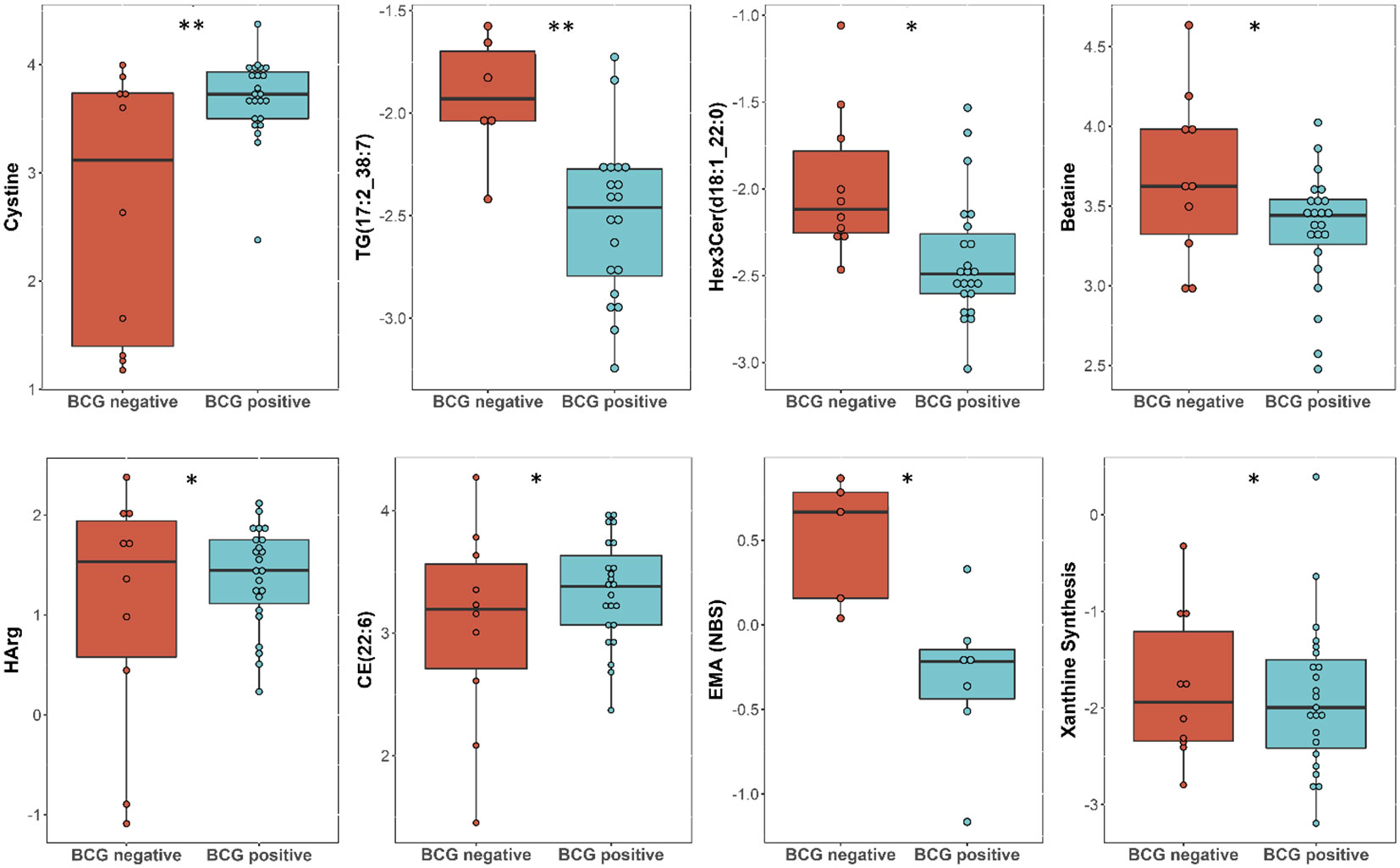
Figure 4 Metabolites associated with BCG vaccination in non-T2D patients with different levels of COVID-19 severity. **/* signifies <0.01/<0.05 p value.
3.5 Spearman’s correlation between clinical traits and significant metabolites in diabetic COVID-19 patients.
Correlation of clinical measurements of diabetic BCG-positive individuals and significantly altered metabolites using Spearman’s test revealed negative association of aconitic acid (p<0.01) and positive correlation of arachidonic acid (p<0.01) with SARS-CoV-2 infection average RT-PCR cycle threshold (CT). Arachidonic acid also shows negative association with hemoglobin and red blood cells (RBC) levels (p<0.05). The amino acid sarcosine shows positive correlation with bilirubin (p<0.05), lactate dehydrogenase (p<0.05), and average CT (p=ns). Data are summarized in Figure 5.
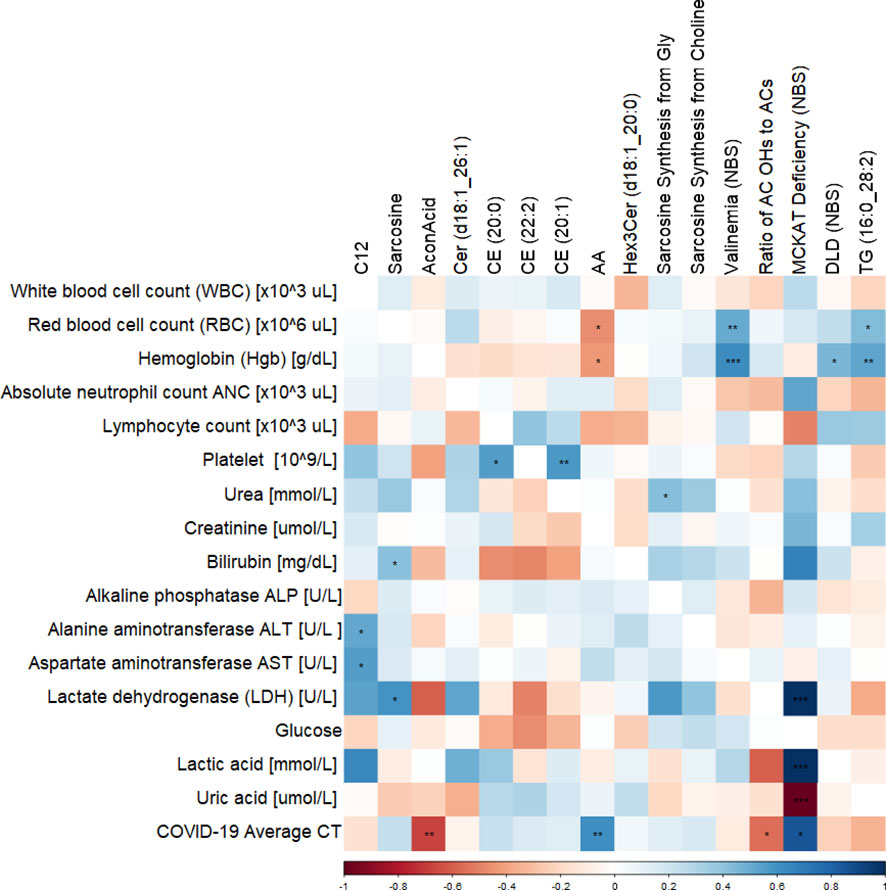
Figure 5 Correlation matrix showing the Spearman’s association between the significant metabolites and clinical traits in BCG-positive diabetic individuals. ***/**/* signifies <0.001/<0.01/<0.05 p value.
4 Discussion
When a virus infects a human body, such as SARS-CoV-2, both the host and the virus influence each other’s metabolism. In fact, host cells support viral survival and reproduction, and as a result, undergo metabolic reprogramming (26). This metabolic alterations can affect the host’s immune response, resulting in a wide spectrum of outcomes, from asymptomatic illness to life-threatening respiratory distress syndrome, and death (25). Additionally, severity of the illness and mortality was closely associated with pre-existing comorbidities such as cardiovascular disease, hypertension, diabetes etc. (27, 28). During the early stages of vaccine development for COVID-19, it was reported that countries with neonatal BCG vaccination policy had fewer cases and lower mortality rate than the countries without BCG (29, 30), sparking interest in the cross-protective nature of BCG vaccine. Since then, several studies have only hypothesized on the safety of BCG for COVID-19 (2, 14, 15, 31).
BCG vaccine is suggested as a prophylactic adjuvant to decrease the severity of COVID-19 when no other agents are available (14). In order to understand if the BCG vaccine can help in reducing the sickness in high-risk group such as the T2D individuals, this study aimed to compare the effect of BCG vaccination status and investigate the metabolic signature associated with BCG vaccination in diabetic vs non-diabetic COVID-19 patients.
In this retrospective pilot study of 67 COVID-19 patients, BCG vaccinated T2D patients (25, 96%) presented with higher severity than BCG-negative T2D (5, 62.5%) patients. This study aimed to elucidate the potential metabolic differences between BCG positive T2D patients with COVID-19 and its BCG-negative counterpart, which could provide an insight into the underlying molecular mechanisms responsible for increased risk of disease progression. The discovery of these pathways would offer more information about the efficacy of BCG vaccination for COVID-19 disease management in T2D individuals.
In this study, targeted metabolomics of serum samples from T2D COVID-19 patients with different levels of disease severity has revealed alterations in amino acid sarcosine, cholesterol esters, and ceramides in BCG-positive group. Functional enrichment analysis revealed changes in cholesterol esters is associated with BCG vaccination in diabetic individuals with COVID-19 (Figure 2C, Table 3). Lower level of sarcosine, metabolic indicators (sarcosine/glycine and sarcosine/choline ratios) was observed in the BCG positive diabetic group compared to BCG-negative counterpart. Sarcosine is an amino acid that is crucial in COVID-19 pathology as it is responsible for boosting antigen-presenting cell activity (32) and autophagy (33), the body’s process of eliminating damaged cells and their immunostimulatory waste. In the course of COVID-19, autophagy functions as a protective catabolic process and is essential for the antiviral response via the direct removal of virus, the display of viral antigens, and the suppression of excessive inflammation (34). Furthermore, our data revealed that arachidonic acid (AA) was elevated in BCG-positive diabetic population. AA is highly potent antiviral agent that renders enveloped viruses, such as SARS-CoV-2 inactive by suppressing viral replication (35). Previous studies have also shown that AA pathway up-regulated due to BCG vaccination (36). Other COVID-19 specific ceramides-related metabolite Cer(d18:1/26:1) (37) was found to be increased in BCG positive in this study.
The non-T2D (general population) counterpart showed no statistical significant difference between BCG vaccinated (60%) and non-BCG vaccinated (70%) groups with COVID-19 severity, which was consistent with clinical trial in this study (31). However, when closely looked at the metabolic profile difference between the study groups, alterations in glycosylceramides, amino acid related metabolites and triacylglycerols were observed in the BCG vaccinated group. Functional enrichment analyses showed glycosylceramides enriched in the BCG vaccinated group (Figure 2D, Table 3). Glycosylceramides (Hex3Cer(d18:1_22:0), Hex2Cer(d18:1/22:0), HexCer(d18:1/26:1), Hex2Cer(d18:1/24:0), HexCer(d18:1/22:0)) were lower in the BCG vaccinated non-diabetic population, as previously suggested to be lower in severe COVID-19 patients (37). Higher levels of glycerophospholipids (Lyso PC a 26:0, lyso PC a 26:1) and triacylglycerols shown here are also consistent with previous studies that showed critically ill patients having increased levels of these metabolites (37, 38). The results in this study are on par with the published metabolic profile of severe COVID-19 patients. However, interestingly, increased level of spermidine and metabolic indicator spermidine synthesis from polyamine putrescine are seen in BCG vaccinated individuals. Spermidine has cardio-protective (39), anti-inflammatory and antioxidant properties (40, 41), which may be mediated via autophagy (42, 43). Indeed, studies have suggested the therapeutic effects of spermidine in COVID-19 affected individuals (44, 45). Additionally, viral replication blocks the conversion of putrescine to spermidine, preventing autophagy and slowing the hosts’ immune response (45). In this study, the increase of spermidine and spermidine conversion from putrescine suggests a conceivably positive effect of BCG in non-diabetic individuals as polyamine metabolism is altered by BCG vaccination (36), possibly due to innate immunity training mechanism.
Spearman’s correlation of clinical parameters and metabolites altered in BCG vaccinated group among diabetic individuals shows negative correlation between AA and SARS-CoV-2 average CT, hemoglobin and RBC measurements. As previously mentioned, higher value of AA is associated with suppressing viral infection, perhaps explaining the positive association between AA and average CT and lower viral load, although the negative association with hemoglobin and RBC levels could indicate increased oxidative stress exerted by high levels of AA in the BCG vaccinated patients (46). Sarcosine shows a positive association with average CT, although not significant, which indicates lower viral load with higher level of sarcosine. It also has a positive correlation with lactate dehydrogenase (LDH), a biomarker of COVID-19 severity and mortality (47). The latter association requires further investigation.
In both diabetic and non-diabetic patients, most of the metabolites identified in the BCG vaccinated cohort were also identified with COVID-19 patients in general population. Sarcosine, AA, and spermidine metabolites and their altered levels in the experimental groups suggest a difference in the efficacy of BCG vaccination for the management of diabetic COVID-19 patients (Figure 6). It must still be determined whether these alterations are mediators or consequences of the BCG vaccination.
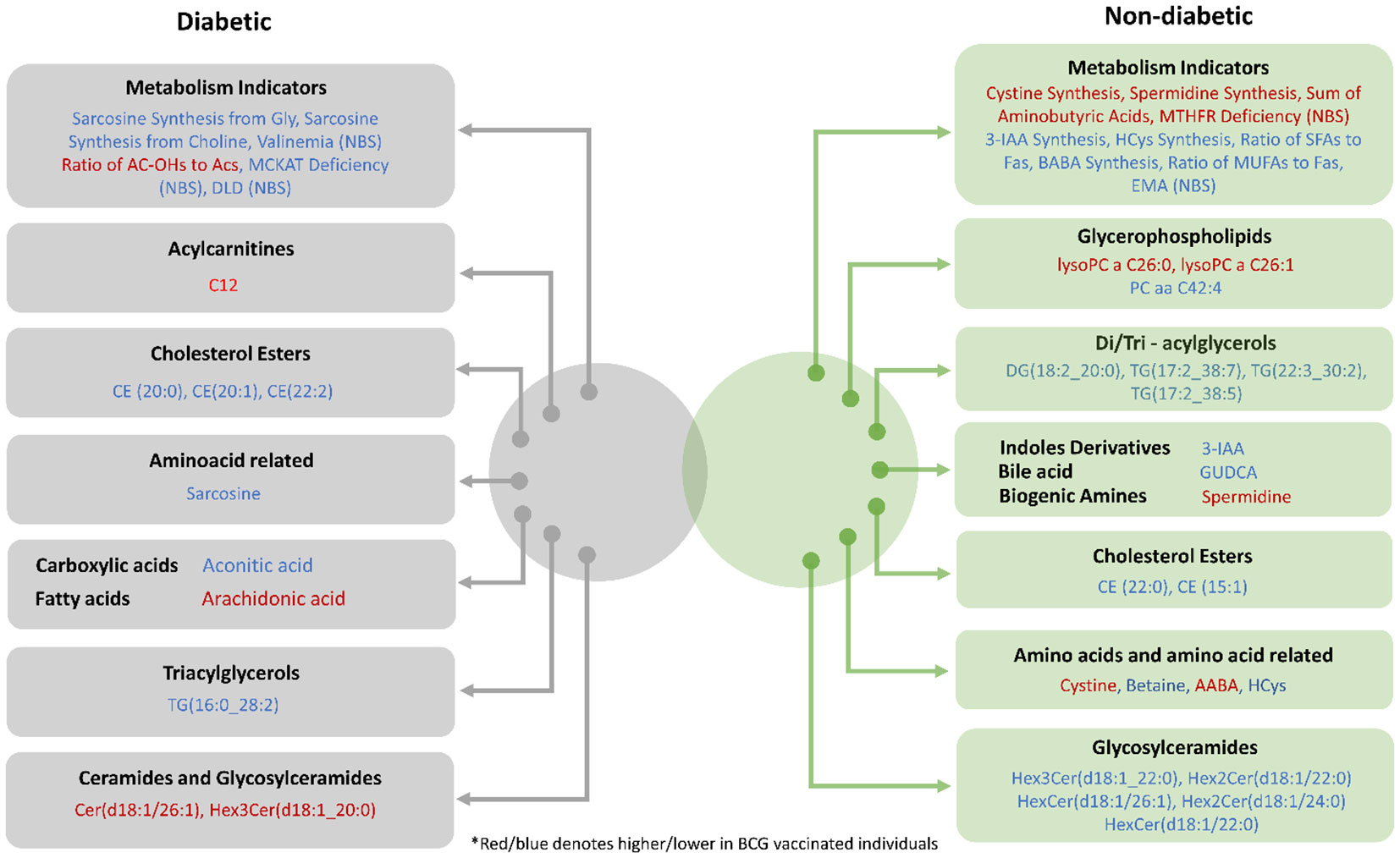
Figure 6 Venn diagram providing a snapshot of the BCG associated metabolites from diabetic and non-diabetic individuals with varying levels of COVID-19 severity.
5 Study limitations
There are several drawbacks in this study including the low number of participants in control groups (BCG negative) and the retrospective nature of the study, which has restricted the information required for interpreting the data from a pathological perspective. Additionally, the study cohort were predominantly males (62 males and 5 females), further studies are required with gender matched subjects to conclude that the findings reflect the metabolic profile of the whole general population. It is possible that other factors and comorbidities with T2DM, conditions such as tobacco usage, COPD, and pneumonia may have contributed to the results seen in the study population. Additionally, the duration between the BCG re-vaccination (if applicable) and SARS-CoV-2 infection was not corrected for in the analysis. Further research is required on a large-scale population which includes information on the patient medication history, comorbidities, smoking habits, and respiratory illness in order to determine the mechanistic involvement of the specific compounds/metabolites mentioned in this study. Nevertheless, to the best of our knowledge, no other study has highlighted the likelihood of negative effect of BCG in T2D individuals with COVID-19.
6 Conclusions
To conclude, our data indicates a possible change in the metabolic profile of diabetic patients with previous BCG vaccination compared to non-vaccinated patients. Our data highlights a possibility that BCG vaccination may have detrimental effect on the diabetic population with regard to COVID-19 severity.
Data availability statement
The raw data supporting the conclusions of this article will be made available by the authors, without undue reservation.
Ethics statement
The studies involving human participants were reviewed and approved by Institutional Review Boards (IRBs) of HMC (MRC-01-20-145) and Qatar University (QU-IRB 1289-EA/20). The patients/participants provided their written informed consent to participate in this study.
Author contributions
NA, MEl designed and performed the data analysis. NA wrote the manuscript. MEl supervised and designed the study and edited the manuscript. FC, HY, AA-T, AA, and MEm helped in the sampling and collection of patients information. All authors contributed to the article and approved the submitted version.
Funding
This research was funded by the Qatar National Research Fund, Grant Number NPRP11S-1212-170092.
Acknowledgments
APCs were paid through QUCG-BRC-22/23-578.
Conflict of interest
The authors declare that the research was conducted in the absence of any commercial or financial relationships that could be construed as a potential conflict of interest.
Publisher’s note
All claims expressed in this article are solely those of the authors and do not necessarily represent those of their affiliated organizations, or those of the publisher, the editors and the reviewers. Any product that may be evaluated in this article, or claim that may be made by its manufacturer, is not guaranteed or endorsed by the publisher.
Supplementary material
The Supplementary Material for this article can be found online at: https://www.frontiersin.org/articles/10.3389/fimmu.2023.1146443/full#supplementary-material
References
1. WHO. Report of the WHO-China joint mission on coronavirus disease 2019 (COVID-19): World health organisation (2020). Available at: https://bmjopen.bmj.com/lookup/google-scholar?link_type=googlescholar&gs_type=article&q_txt=.+Report+of+the+WHO-China+joint+mission+on+coronavirus+disease+2019+(COVID-19).+World+Health+Organisation%2C+2020%3A+40.
2. Gong W, Mao Y, Li Y, Qi Y. BCG Vaccination: A potential tool against COVID-19 and COVID-19-like black swan incidents. Int Immunopharmacol (2022) 108:108870. doi: 10.1016/j.intimp.2022.108870
4. Parmar K, Siddiqui A, Nugent K. Bacillus calmette-guerin vaccine and nonspecific immunity. Am J Med Sci (2021) 361(6):683–9. doi: 10.1016/j.amjms.2021.03.003
5. Covián C, Fernández-Fierro A, Retamal-Díaz A, Díaz FE, Vasquez AE, Lay MK, et al. BCG-Induced cross-protection and development of trained immunity: Implication for vaccine design. Front Immunol (2019) 10. doi: 10.3389/fimmu.2019.02806
6. Kristensen I, Fine P, Aaby P, Jensen H. Routine vaccinations and child survival: follow up study in Guinea-Bissau, West Africa commentary: an unexpected finding that needs confirmation or rejection. BMJ (2000) 321(7274):1435. doi: 10.1136/bmj.321.7274.1435
7. Schaltz-Buchholzer F, Sørensen MK, Benn CS, Aaby P. The introduction of BCG vaccination to neonates in Northern Sweden, 1927–31: Re-analysis of historical data to understand the lower mortality among BCG-vaccinated children. Vaccine (2022) 40(11):1516–24. doi: 10.1016/j.vaccine.2021.06.006
8. Garly M-L, Martins CL, Balé C, Baldé MA, Hedegaard KL, Gustafson P, et al. BCG Scar and positive tuberculin reaction associated with reduced child mortality in West Africa: A non-specific beneficial effect of BCG? Vaccine (2003) 21(21):2782–90. doi: 10.1016/S0264-410X(03)00181-6
9. Arts RJW, Moorlag S, Novakovic B, Li Y, Wang SY, Oosting M, et al. BCG Vaccination protects against experimental viral infection in humans through the induction of cytokines associated with trained immunity. Cell Host Microbe (2018) 23(1):89–100.e5. doi: 10.1016/j.chom.2017.12.010
10. Daulatabad D, Pandhi D, Singal A. BCG Vaccine for immunotherapy in warts: is it really safe in a tuberculosis endemic area? Dermatol Ther (2016) 29(3):168–72. doi: 10.1111/dth.12336
11. Hippmann G, Wekkeli M, Rosenkranz AR, Jarisch R, Götz M. Nonspecific immune stimulation with BCG in herpes simplex recidivans. follow-up 5 to 10 years after BCG vaccination. Wien Klin Wochenschr (1992) 104(7):200–4.
12. Stensballe LG, Nante E, Jensen IP, Kofoed PE, Poulsen A, Jensen H, et al. Acute lower respiratory tract infections and respiratory syncytial virus in infants in Guinea-Bissau: a beneficial effect of BCG vaccination for girls community based case-control study. Vaccine (2005) 23(10):1251–7. doi: 10.1016/j.vaccine.2004.09.006
13. Leentjens J, Kox M, Stokman R, Gerretsen J, Diavatopoulos DA, van Crevel R, et al. BCG Vaccination enhances the immunogenicity of subsequent influenza vaccination in healthy volunteers: A randomized, placebo-controlled pilot study. J Infect Dis (2015) 212(12):1930–8. doi: 10.1093/infdis/jiv332
14. Gonzalez-Perez M, Sanchez-Tarjuelo R, Shor B, Nistal-Villan E, Ochando J. The BCG vaccine for COVID-19: First verdict and future directions. Front Immunol (2021) 12. doi: 10.3389/fimmu.2021.632478
15. Kulesza J, Kulesza E, Koziński P, Karpik W, Broncel M, Fol M. BCG And SARS-CoV-2-What have we learned? Vaccines (Basel). (2022) 10(10). doi: 10.3390/vaccines10101641
16. Faustman DL, Wang L, Okubo Y, Burger D, Ban L, Man G, et al. Proof-of-concept, randomized, controlled clinical trial of bacillus-Calmette-Guerin for treatment of long-term type 1 diabetes. PloS One (2012) 7(8):e41756. doi: 10.1371/journal.pone.0041756
17. Paolillo A, Buzzi MG, Giugni E, Sabatini U, Bastianello S, Pozzilli C, et al. The effect of bacille calmette-guérin on the evolution of new enhancing lesions to hypointense T1 lesions in relapsing remitting MS. J Neurol (2003) 250(2):247–8. doi: 10.1007/s00415-003-0967-6
18. Ristori G, Buzzi MG, Sabatini U, Giugni E, Bastianello S, Viselli F, et al. Use of bacille calmette-guèrin (BCG) in multiple sclerosis. Neurology (1999) 53(7):1588–9. doi: 10.1212/WNL.53.7.1588
19. Faustman DL, Lee A, Hostetter ER, Aristarkhova A, Ng NC, Shpilsky GF, et al. Multiple BCG vaccinations for the prevention of COVID-19 and other infectious diseases in type 1 diabetes. Cell Rep Med (2022) 3(9):100728. doi: 10.1016/j.xcrm.2022.100728
20. Elrayess MA, Cyprian FS, Abdallah AM, Emara MM, Diboun I, Anwardeen N, et al. Metabolic signatures of type 2 diabetes mellitus and hypertension in COVID-19 patients with different disease severity. Front Med (Lausanne). (2021) 8:788687. doi: 10.3389/fmed.2021.788687
21. Yang X, Yu Y, Xu J, Shu H, Ja X, Liu H, et al. Clinical course and outcomes of critically ill patients with SARS-CoV-2 pneumonia in wuhan, China: a single-centered, retrospective, observational study. Lancet Respir Med (2020) 8(5):475–81. doi: 10.1016/S2213-2600(20)30079-5
22. Guan W-j, Ni Z-y, Hu Y, Liang W-h, Ou C-q, He J-x, et al. Clinical characteristics of coronavirus disease 2019 in China. New Engl J Med (2020) 382(18):1708–20. doi: 10.1056/NEJMoa2002032
23. de Almeida-Pititto B, Dualib PM, Zajdenverg L, Dantas JR, de Souza FD, Rodacki M, et al. Severity and mortality of COVID 19 in patients with diabetes, hypertension and cardiovascular disease: a meta-analysis. Diabetol Metab Syndrome. (2020) 12(1):75. doi: 10.1186/s13098-020-00586-4
24. WHO. Living guidance for clinical management of COVID-19 (2021). Available at: https://apps.who.int/iris/bitstream/handle/10665/349321/WHO-2019-nCoV-clinical-2021.2-eng.pdf.
25. Mussap M, Fanos V. Could metabolomics drive the fate of COVID-19 pandemic? a narrative review on lights and shadows. Clin Chem Lab Med (CCLM). (2021) 59(12):1891–905. doi: 10.1515/cclm-2021-0414
26. Thaker SK, Ch’ng J, Christofk HR. Viral hijacking of cellular metabolism. BMC Biol (2019) 17(1):59. doi: 10.1186/s12915-019-0678-9
27. Shi Q, Zhang X, Jiang F, Zhang X, Hu N, Bimu C, et al. Clinical characteristics and risk factors for mortality of COVID-19 patients with diabetes in wuhan, China: A two-center, retrospective study. Diabetes Care (2020) 43(7):1382–91. doi: 10.2337/dc20-0598
28. McGurnaghan SJ, Weir A, Bishop J, Kennedy S, Blackbourn LAK, McAllister DA, et al. Risks of and risk factors for COVID-19 disease in people with diabetes: a cohort study of the total population of Scotland. Lancet Diabetes Endocrinol (2021) 9(2):82–93. doi: 10.1016/S2213-8587(20)30405-8
29. Miller A, Reandelar MJ, Fasciglione K, Roumenova V, Li Y, Otazu GH. Correlation between universal BCG vaccination policy and reduced mortality for COVID-19. medRxiv (2020) 2020:03.24.20042937. doi: 10.1101/2020.03.24.20042937
30. Bagheri N, Montazeri H. On BCG vaccine protection from COVID-19: A review. SN Compr Clin Med (2021) 3(6):1261–71. doi: 10.1007/s42399-021-00835-1
31. Upton CM, van Wijk RC, Mockeliunas L, Simonsson USH, McHarry K, van den Hoogen G, et al. Safety and efficacy of BCG re-vaccination in relation to COVID-19 morbidity in healthcare workers: A double-blind, randomised, controlled, phase 3 trial. eClinicalMedicine (2022) 48. doi: 10.1016/j.eclinm.2022.101414
32. Dastmalchi F, Karachi A, Yang C, Azari H, Sayour EJ, Dechkovskaia A, et al. Sarcosine promotes trafficking of dendritic cells and improves efficacy of anti-tumor dendritic cell vaccines via CXC chemokine family signaling. J Immunother Cancer. (2019) 7(1):321. doi: 10.1186/s40425-019-0809-4
33. Walters RO, Arias E, Diaz A, Burgos ES, Guan F, Tiano S, et al. Sarcosine is uniquely modulated by aging and dietary restriction in rodents and humans. Cell Rep (2018) 25(3):663–76.e6. doi: 10.1016/j.celrep.2018.09.065
34. Carmona-Gutierrez D, Bauer MA, Zimmermann A, Kainz K, Hofer SJ, Kroemer G, et al. Digesting the crisis: autophagy and coronaviruses. Microb Cell (2020) 7(5):119–28. doi: 10.15698/mic2020.05.715
35. Hoxha M. What about COVID-19 and arachidonic acid pathway? Eur J Clin Pharmacol (2020) 76(11):1501–4. doi: 10.1007/s00228-020-02941-w
36. Diray-Arce J, Angelidou A, Jensen KJ, Conti MG, Kelly RS, Pettengill MA, et al. Bacille calmette-guérin vaccine reprograms human neonatal lipid metabolism in vivo and in vitro. Cell Rep (2022) 39(5):110772. doi: 10.1016/j.celrep.2022.110772
37. Caterino M, Gelzo M, Sol S, Fedele R, Annunziata A, Calabrese C, et al. Dysregulation of lipid metabolism and pathological inflammation in patients with COVID-19. Sci Rep (2021) 11(1):2941. doi: 10.1038/s41598-021-82426-7
38. Herrera-Van Oostdam AS, Castañeda-Delgado JE, Oropeza-Valdez JJ, Borrego JC, Monárrez-Espino J, Zheng J, et al. Immunometabolic signatures predict risk of progression to sepsis in COVID-19. PloS One (2021) 16(8):e0256784. doi: 10.1371/journal.pone.0256784
39. Tong D, Hill JA. Spermidine promotes cardioprotective autophagy. Circ Res (2017) 120(8):1229–31. doi: 10.1161/CIRCRESAHA.117.310603
40. Choi YH, Park HY. Anti-inflammatory effects of spermidine in lipopolysaccharide-stimulated BV2 microglial cells. J Biomed Science. (2012) 19(1):31. doi: 10.1186/1423-0127-19-31
41. Lagishetty CV, Naik SR. Polyamines: Potential anti-inflammatory agents and their possible mechanism of action. Indian J Pharmacol (2008) 40(3):121–5.
42. Yang Y, Chen S, Zhang Y, Lin X, Song Y, Xue Z, et al. Induction of autophagy by spermidine is neuroprotective via inhibition of caspase 3-mediated beclin 1 cleavage. Cell Death Dis (2017) 8(4):e2738. doi: 10.1038/cddis.2017.161
43. Madeo F, Eisenberg T, Pietrocola F, Kroemer G. Spermidine in health and disease. Science (2018) 359(6374):eaan2788. doi: 10.1126/science.aan2788
44. Khan N, Chen X, Geiger JD. Possible therapeutic use of natural compounds against COVID-19. J Cell Signal (2021) 2(1):63–79.
45. Gassen NC, Papies J, Bajaj T, Dethloff F, Emanuel J, Weckmann K, et al. Analysis of SARS-CoV-2-controlled autophagy reveals spermidine, MK-2206, and niclosamide as putative antiviral therapeutics. bioRxiv (2020) 2020:04.15.997254. doi: 10.1101/2020.04.15.997254
46. Yuan T, Cong Y, Meng J, Qian H, Ye W, Sun WS, et al. Arachidonic acid causes hidden blood loss-like red blood cell damage through oxidative stress reactions. J Surg Res (2017) 211:14–20. doi: 10.1016/j.jss.2016.11.060
Keywords: COVID - 19, SARS – CoV – 2, diabete mellitus, BCG vaccination, sarcosine, metabolomics, arachidonic acid (AA)
Citation: Anwardeen NR, Cyprian FS, Yassine HM, Al-Thani AA, Abdallah AM, Emara MM and Elrayess MA (2023) The retrospective study of the metabolic patterns of BCG-vaccination in type-2 diabetic individuals in COVID-19 infection. Front. Immunol. 14:1146443. doi: 10.3389/fimmu.2023.1146443
Received: 17 January 2023; Accepted: 27 March 2023;
Published: 05 April 2023.
Edited by:
Mahbuba Rahman, McMaster University, CanadaReviewed by:
Rizwan Qureshi, Hamad bin Khalifa University, QatarDivya Jyothi Madipally, Moderna Inc., United States
Mohammad Safiqul Islam, Noakhali Science and Technology University, Bangladesh
Copyright © 2023 Anwardeen, Cyprian, Yassine, Al-Thani, Abdallah, Emara and Elrayess. This is an open-access article distributed under the terms of the Creative Commons Attribution License (CC BY). The use, distribution or reproduction in other forums is permitted, provided the original author(s) and the copyright owner(s) are credited and that the original publication in this journal is cited, in accordance with accepted academic practice. No use, distribution or reproduction is permitted which does not comply with these terms.
*Correspondence: Mohamed A. Elrayess, m.elrayess@qu.edu.qa