- Department of Medicine, Division of Infectious Diseases, Icahn School of Medicine at Mount Sinai, New York, NY, United States
Approximately 100,000 mother-to-child transmission (MTCT) events of HIV via human milk feeding occur each year. However, only about 15% of infants milk-fed by untreated HIV+ mothers become infected, suggesting a protective effect of the milk itself. Infants ingest 105-108 maternal leukocytes daily via milk, which remain functional beyond ingestion. Such function may be elicited by maternal milk antibody (Ab). Though IgA is dominant in milk, most HIV-specific milk Abs are of the IgG subclass, highlighting the importance of investigating the function of each IgG isotype in the milk context. Though Ab effector function mediated by the constant (Fc) domain via interaction with Fc Receptors (FcRs), such as Ab-dependent cellular phagocytosis (ADCP), are critical in protecting against HIV infection, ADCP is largely unexplored as it relates to mitigation of MTCT. Presently we report the ADCP activity of milk leukocytes against HIV particles and immune complexes (ICs), using 57 unique samples from 34 women, elicited by IgG1/2/3/4 of monoclonal (m)Ab 246-D. Granulocyte ADCP of HIV was most potent compared to other phagocytes when elicited by IgG1/3/4. IgG1/3 activated granulocytes similarly, exhibiting 1.6x-4.4x greater activity compared to IgG2/4, and a preference for virus compared to ICs. Notably, CD16- monocyte ADCP of a given target were unaffected by isotype, and CD16+ monocytes were poorly stimulated by IgG1. IgG2/4 elicited potent IC ADCP, and in terms of total leukocyte IC ADCP, IgG4 and IgG3 exhibited similar function, with IgG4 eliciting 1.6x-2.1x greater activity compared to IgG1/IgG2, and CD16+ monocytes most stimulated by IgG2. These data contribute to a more comprehensive understanding of Fc-mediated functionality of milk leukocytes, which is critical in order to develop therapeutic approaches to eliminating this route of MTCT, including mucosal administration of mAbs and/or a maternal vaccination aimed to elicit a potent milk Ab response.
Background
It is evident that although human milk is a vehicle for Mother-to-child transmission (MTCT) of HIV, its virus-killing properties are significant, and unless access to clean water and appropriate infant formula is reliable, the World Health Organization does not recommend cessation of breastfeeding for HIV-infected mothers (1, 2). Milk-derived cytokines, defensins, mucins, erythropoietin, long-chain polyunsaturated fatty acids, oligosaccharides, and lactoferrin have been found to inhibit HIV in vitro, with some being directly correlated with reduced transmission in vivo. Importantly, HIV-specific antibodies (Abs) in milk have been correlated with reduced MTCT, but what remains largely unexplored is the contribution of the cellular fraction of human milk (3–12).
Human milk is comprised of maternal cells that are >90% viable (13). Cell composition is impacted strongly by stage of lactation, health status of mother and infant, and individual variation, which remains poorly understood (13–16). Given that human milk contains ~102–105 leukocytes/mL, it can be estimated that breastfed infants ingest ∼105–108 maternal leukocytes daily (17). Various in vivo studies have demonstrated that maternal leukocytes provide critical immunity to the infant and are functional well beyond these sites of initial ingestion (17–23). All maternally-derived cells ingested by the infant have the potential to perform immune functions alongside or to compensate for the infant’s own leukocytes (24). This is likely in conjunction with other components, such as maternal Abs.
Many Abs that are unable to prevent viral entry per se are capable of binding to the viral surface or to viral proteins on the surface of infected cells to facilitate a variety of anti-viral activities that ultimately contribute to viral clearance. These functions are mediated by the ‘constant’ region of the immunoglobulin molecule – the crystallizable fragment (Fc) – via interactions with Fc receptors (FcRs) found on virtually all innate immune cells (25). Human milk contains all classes of immunoglobulins, with IgA being dominant (~90% of total Ab); however, most studies have found all HIV-specific Ab in the milk of lactating HIV-infected women to be IgG, a finding which extends to non-human primates (NHPs) and highlights the importance of investigating the function of the various IgG isotypes in milk (3, 26–31). Fc-mediated activity of human milk IgG has been associated with reduced risk of MTCT of HIV, and follow-up analyses of RV144 - the only clinical HIV vaccine trial to show significant efficacy, and various other immune correlate analyses have demonstrated that Fc-mediated Ab effector functions such as Ab-dependent cellular phagocytosis (ADCP) are critical in protecting against HIV infection (32–36). Importantly, FcR allelic variants, particularly for FcγRIIa (implicated in IgG-mediated ADCP against HIV-1), have been shown to be related to disease progression, risk of infection, and/or MTCT of HIV, and it has been shown that IgG from HIV-infected controllers exhibits superior ADCP activity compared to IgG from viremic non-controllers, further highlighting the likely important role of ADCP in mitigating HIV replication (37, 38).
Neutrophils, monocytes, macrophages, and dendritic cells (DCs) are the “professional” phagocytes; these cells have been identified or are purported to be found in human milk (13, 39). We have undertaken a study to assess the ADCP capacity of human milk leukocytes against HIV. Our previous pilot study confirmed milk leukocytes as capable of ADCP of HIV Env gp120-coated microbeads, and demonstrated that most activity was granulocyte-driven (40, 41). In the present study we report our analysis of the ADCP activity of milk leukocytes in 57 unique samples from 34 women, elicited by recombinant IgG1/2/3/4 of monoclonal (m)Ab 246-D, a weakly-neutralizing Env gp41 (cluster 1)-specific Ab - the type of Ab commonly elicited by natural HIV infection.
Materials and Methods
Participant Information
Participants were recruited using social media and informed consent was obtained in accordance with the ethical and institutional board approval under the guidance and authorization of Mount Sinai’s Program for the Protection of Human Subjects (PPHS) using an IRB-approved protocol for obtaining human milk samples (STUDY-17-01089). Individuals were eligible to have their milk samples included in this analysis if they were lactating, feeling well, and living in New York City. Participants were excluded if they had any acute or chronic health conditions affecting the immune system. Milk was expressed using manual or electric pumps in participants’ homes just prior to sample pickup using clean containers. Milk was used for ADCP experiments within 4 hours of expression.
Recombinant Ab Production
mAbs 246-D and 3865 were originally isolated from human peripheral blood mononuclear cells (42, 43), and were expressed recombinantly in 293T cells for this study as human IgG1, IgG2, IgG3, IgG4 using IgG plasmids (In vivogen) by conventional cloning techniques as previously described (44, 45).
GFP-HIV Target Virus
The infectious molecular clone (HIV-GFP11056) used for these experiments carries a Gag-interdomain green fluorescent protein (GFP), and these GFP viruses were originally described by Dr. Benjamin Chen who generously supplied the virus in the present study (46). Virus was produced by standard transfection of 293T cells, sucrose-gradient purified, and quantified by p24 ELISA as previously described (46).
ADCP Assay
This primary milk cell assay was adapted from Ackerman et al. (47) and has been described previously using latex beads (41). Milk samples were centrifuged at 600g for 15 min, cell pellet was washed 2x with Hank’s balanced salt solution (HBSS) without calcium and magnesium with centrifugation at 350g for 10 min. Care was taken to gently resuspend cell pellets to avoid granulocyte activation and apoptosis. For virus ADCP, 50ul of 300ng/mL (by p24 quantification) GFP-HIV was aliquoted in 96-well plates. Titrated (200ug/ml – 12.5ug/ml) mAb in 50ul was incubated with virus for 2hrs at 37°C. Fifty thousand milk cells were added per well and plate was incubated as above. For IC ADCP, 100ug/mL anti-human IgG(Fc) (Life Technologies) was added to virus-Ab complexes and incubated for 30 min at 37°C prior to the addition of cells as described in (48). Control experiments to distinguish between virions bound to the cell-surface and those phagocytosed by cells were performed by pre-incubating milk cells with 10ug/mL of the actin inhibitor Cytochalasin-D (Sigma) and/or 50ug/mL of FcR blocking agent FcBlock (BD). After incubation, cells were washed and incubated in Accutase cell detachment solution for 10 min at room temperature to gently remove any surface-bound virus while preserving epitopes for flow cytometry analysis [Innovative Cell Technologies; as described in (48)]. Cells were then washed and stained. For all CD45+ leukocytes, as well as for each of the phagocyte subsets, ADCP activity was measured as the percent of GFP+ cells of that cell type. ADCP scores for total CD45+ leukocytes were calculated as [(MFI of GFP+ cells) x (% GFP+ cells of the CD45+ population)], and for each phagocyte subset were calculated as [(MFI of GFP+ cells) x (% of total CD45+ cells in the GFP+ population)], in order to provide a relative assessment of each cell population’s overall phagocytic contribution within each unique sample. Experiments for each milk sample were performed in duplicate. Scores at each mAb dilution were plotted using GraphPad Prism and Area under the Curve (AUC) values were calculated for each experiment. AUC scores were normalized by subtracting those obtained for each cell type using the irrelevant anthrax mAb 3865 of the same class. Two-tailed Mann-Whitney tests were used to compare data. Correlation analyses were performed using Spearman’s test.
Gating Strategy
Analysis was performed using an LSR Fortessa. Cells were stained as directed by the manufacturer for CD45, CD3, CD14, CD16, CD20, CD56, and MHC-II (HLA-DR, DP, DQ) (BD) (39, 49, 50). Initial gating and viability dye (BD) was used to exclude doublets and dead cells. A side scatter (SSC) vs. CD45 plot was then used to identify CD45+ leukocytes. FSC/SSC was used to identify monocytes followed by gating to exclude CD3+ T cells, CD20+ B cells, and CD56+ NK cells. MHC-II/CD14/CD16 staining was employed to differentiate classical monocytes (CD14+CD16−) from activated monocytes/macrophages (CD14+CD16+ and CD14dimCD16+) (50, 51). DCs were identified by selecting from the FSC/SSC plot to include both lymphocytes and monocytes, followed by gating on MHC-II+ cells after exclusion of CD3+ T lymphocytes, CD14+ monocytes, CD20+ B lymphocytes, and CD56+ NK cells. Granulocytes were identified based on the FSC/SSC plot and further confirmed by exclusion of other cell markers as above, and positive CD16 stain. Gating strategy is shown in Figure 1.
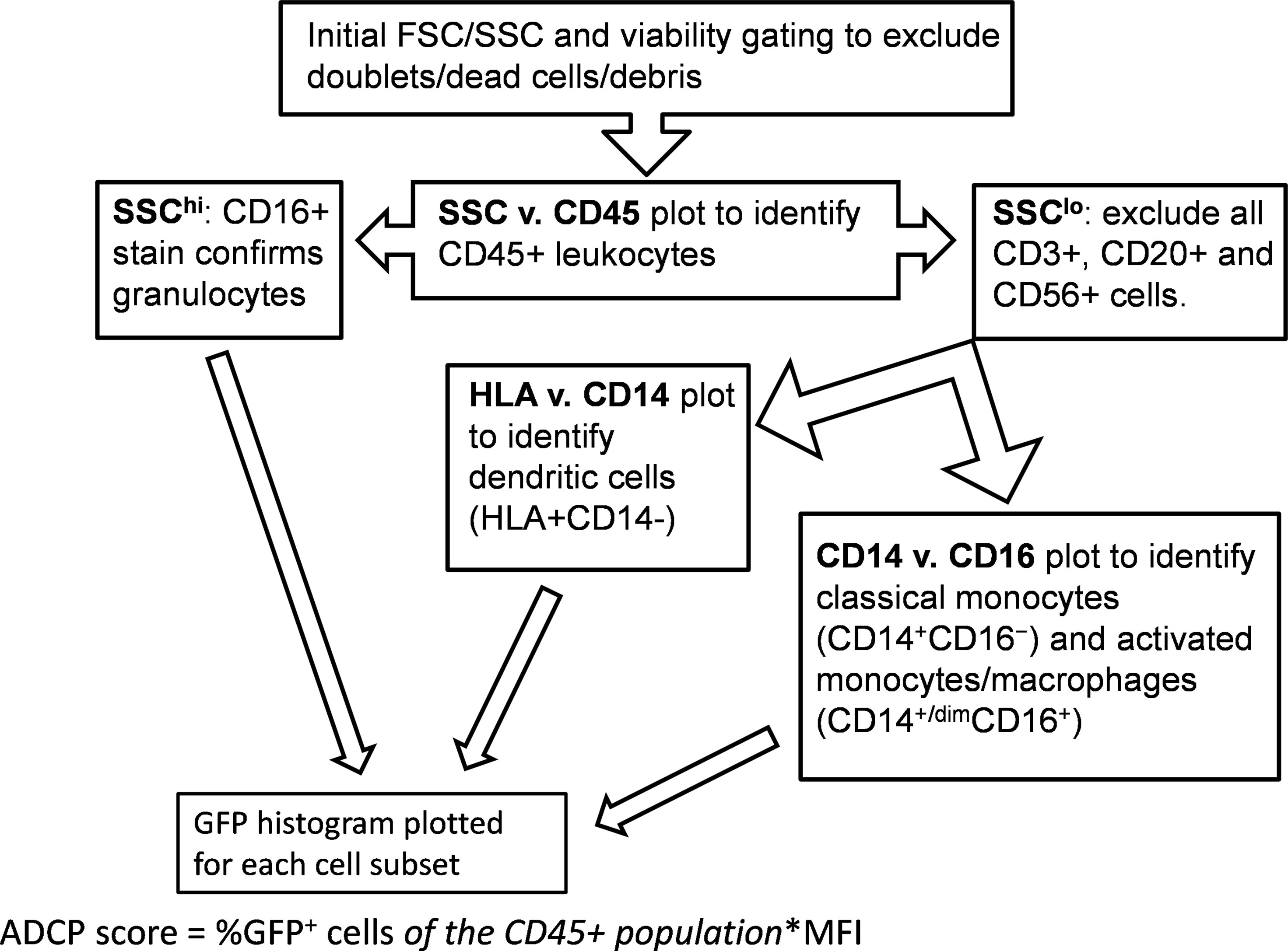
Figure 1 Gating strategy. Flow cytometry was performed using an LSR Fortessa (BD) with initial gating on FACSDiva software. Analytical gating was performed using FCS Express.
Results
Sample Data
Fifty-seven unique milk samples were obtained from 34 different women who were between 1 – 24 months postpartum at the time of milk collection (mean, 8.1 months), yielding 42mL – 250mL of milk per sample [mean, 88.4mL (Figures 2A, B)]. Samples contained between 12,054 and 707,143 total cells/mL of milk (mean, 69,291 cells/mL; Figure 2C), which included 0.02% - 33% CD45+ leukocytes (mean, 6.83%; Figure 2D). On average, classical monocytes (SSCintCD45+CD3-CD20-CD56-CD16-CD14+) and granulocytes (SSChiCD45+CD16+) accounted for similar proportions of the CD45+ leukocytes (15.1% and 11.8%, respectively), while the proportion of activated monocytes/macrophages (SSCintCD45+CD3-CD20-CD56-CD16+CD14+/dim) was significantly lower compared to the classical monocytes (mean, 6.03%; p<0.0001), and the proportion of dendritic cells (DCs; SSClo/intCD45+CD3-CD20-CD56-CD16+/-D14-HLA+) was lowest of all subsets identified – significantly lower than all other subsets (mean, 3.18%; p< 0.0001; Figure 2E).
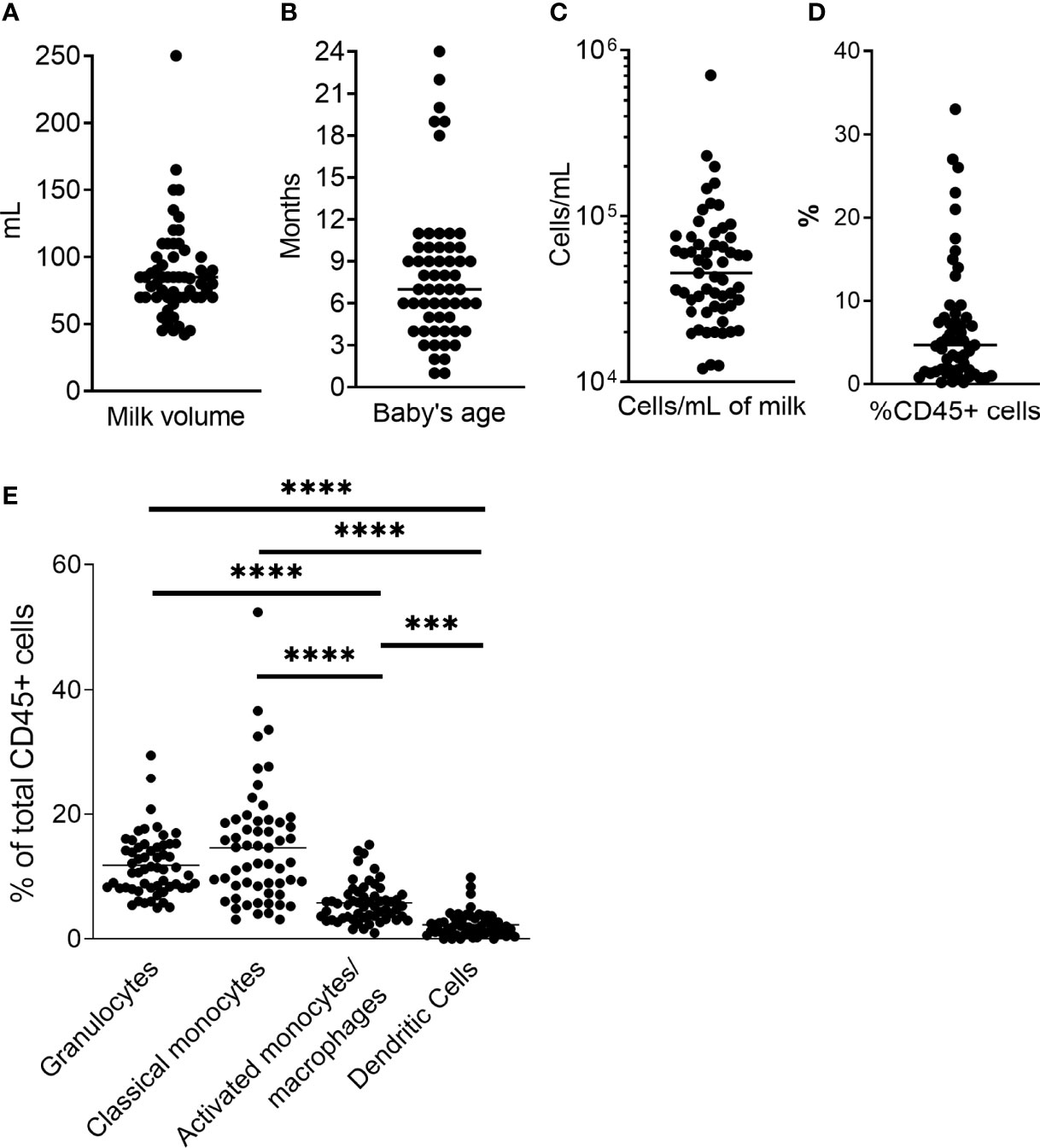
Figure 2 Sample data. (A) Milk volume collected for each sample. Milk was expressed just prior to sample pickup and used within 4h. (B) Baby’s age/time post-partum of study participant. (C) Total cell concentration for each sample. Milk was centrifuged at 600g for 15 min, and cell pellet washed with HBSS at 350g. Cells were counted, and stained as directed (BD). (D) Percent CD45+ cells of total cell population as determined by flow cytometry. (E) Phagocyte subset populations expressed as percent of total CD45+ cells. CD45+ cells and phagocyte subsets were identified as described in Figure 1. Two-tailed Students’ t-tests were used to compare phagocyte populations. ***0.0005 ≥ p > 0.0001. ****p = < 0.0001.
ADCP Activity of 246-D-Opsonized Virus and ICs as Compared by Effector Cell Type
Total CD45+ leukocytes as well as each phagocyte subset were assessed for their ADCP capacity against the GFP-HIV target virus opsonized with titrated mAb 246-D. Phagocytosis is characterized by reliance on remodeling of the actin cytoskeleton; importantly, blocking experiments with Cytochalasin-D, an inhibitor of this process, demonstrated that ADCP activity was actin dependent, with residual activity below that of the 3865 mAb control (Figure 3). Similarly, pre-incubation of cells with FcBlock indicated activity was FcR-mediated. ADCP was performed using milk cells isolated from 11-19 unique samples for each IgG isotype tested.
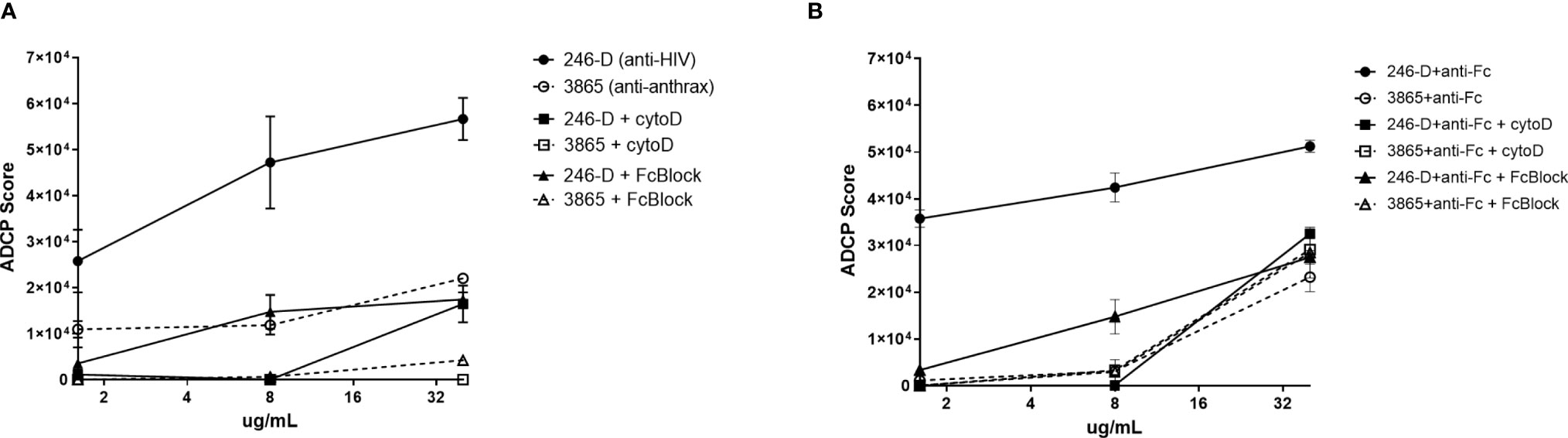
Figure 3 ADCP activity measured using milk phagocytes is actin and FcR dependent. 300ng/mL GFP-HIV per well was incubated with titrated IgG1 mAb for 2hrs at 37°C. Meanwhile, 50,000 milk cells per well in a separate plate were prepared, with certain wells pre-incubated with 10ug/mL of the actin inhibitor Cytochalasin-D (Sigma) or 50ug/mL of FcR blocking agent FcBlock (BD) for 30min at 37°C. (A) Virus ADCP; (B) Immune complex ADCP: 100ug/mL anti-human IgG(Fc) was added to virus-Ab complexes and incubated for 30 min at 37°C prior to the addition of cells. Plated cells were washed and incubated with virus/mAb complexes. Plates were washed and incubated in Accutase cell detachment solution, then washed and stained. ADCP activity was measured as the percent of GFP+ cells, with ADCP scores calculated as [(MFI of GFP-positive cells) x (% of GFP+ cells of CD45+ population)]. Data shown was measured for CD45+ population. Mean values of duplicate experiments are shown with SEM.
IgG1-Elicited ADCP
IgG1-246D-elicited granulocyte ADCP of HIV-GFP was the most dominant, and this activity was significantly greater than that of all other phagocyte subsets (6.7x – 57x greater activity; p<0.0001; Figure 4A). Activated and classical monocyte ADCP were of similar potencies, wherein both monocyte types exhibited significantly more potent virus ADCP activity compared to DCs (6.7x – 8.4x greater activity; p=0.001 and p=0.009, respectively; Figure 4A). Similarly, granulocyte ADCP of the larger anti-Fc-complexed HIV-GFP immune complexes (ICs) was the most potent (1.7x – 13x greater activity; p=0.005 and p<0.0001, respectively), although this activity was not significantly greater than that of the activated monocytes in this case (Figure 4A). In fact, against these larger targets, activated monocyte activity was significantly increased compared to that of the classical monocytes and the DCs (3.2x – 7.6x greater activity; p=0.023 and 0.0004, respectively), while classical monocyte and DC activities were similar (Figure 4A). When comparing ADCP potency against opsonized virus vs. IC for each cell type, only granulocytes exhibited a significant preference, for the smaller, ‘free’ virus (1.6x greater activity; p=0.024).
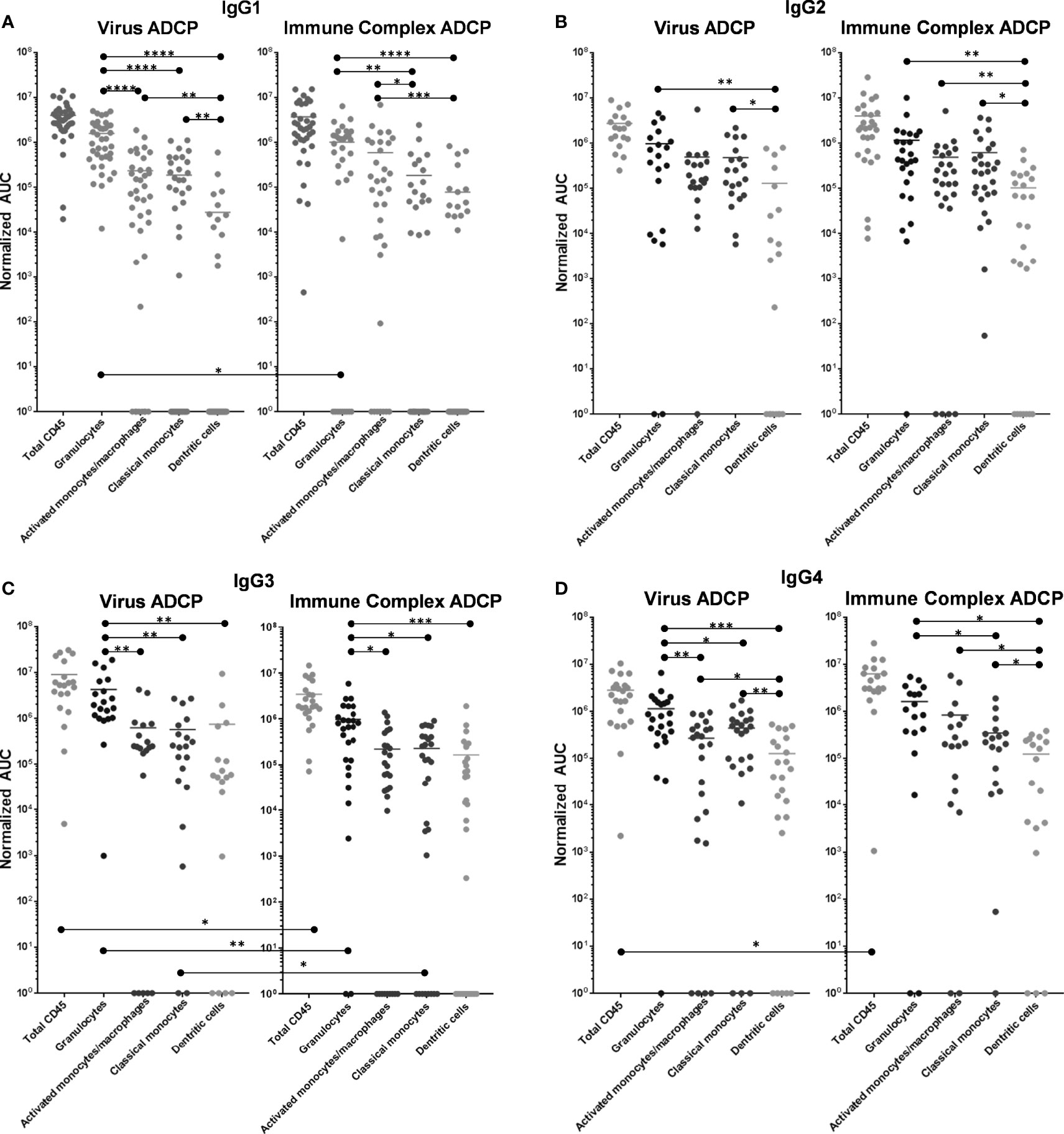
Figure 4 ADCP activity of 246-D-opsonized virus and ICs as compared by effector cell type. (A) IgG1 data. (B) IgG2 data. (C) IgG3 data. (D) IgG4 data. ADCP was performed as described above. ADCP scores at each mAb dilution were plotted using GraphPad Prism and Area under the Curve (AUC) values were calculated for each experiment. AUC scores were normalized by subtracting those obtained for each cell type using the irrelevant anthrax mAb 3865 of the same class. Each data point represents cells from a unique milk sample. Mean AUC are shown. Two-tailed Mann-Whitney tests were used to compare data. ****p < 0.0001; ***0.0009 > p > 0.0001; **0.009 > p > 0.001; *0.05 > p > 0.01.
IgG2-Elicited ADCP
As IgG2, 246D elicited granulocyte ADCP of HIV-GFP significantly more potently than DCs (7.5x greater activity; p=0.0076; Figure 4B), but not compared to any other cell type. Activated and classical monocyte ADCP were of similar potencies, though classical monocytes also exhibited significantly more potent virus ADCP activity compared to DCs (3.7x greater activity; p=0.0125; Figure 4B). Granulocyte ADCP of the HIV ICs was again only significantly greater than that of the DCs (11.1x greater activity; p=0.0058; Figure 4B). Classical and activated monocyte ADCP was similar, while both cell subsets exhibited more potent ADCP than DCs (4.7 – 6x greater activity; p=0.0046 – 0.029; Figure 4B). When comparing ADCP potency against virus vs. IC for each cell type, no significant differences were found.
IgG3-Elicited ADCP
As IgG3, 246D elicited significantly greater granulocyte ADCP of the virus particles compared to all other cell types (5.7x – 6.8x greater activity; p=0.0023 – 0.014; Figure 4C). All other phagocyte subsets exhibited similar activities. Granulocytes also exhibited significantly greater ADCP compared to all other cell types against the HIV-ICs when elicited by IgG3 (4.1x – 5.9x greater activity; p=0.0006 – 0.011; Figure 4C). As observed for the virus targets, all other phagocyte subsets exhibited similar activities. Notably, total CD45+, granulocyte, and classical monocyte ADCP activity was significantly greater against the virus targets compared to the ICs (2.5x – 4.4x greater activity; p=0.0096 – 0.0225).
IgG4-Elicited ADCP
As IgG4, 246D elicited significantly greater granulocyte ADCP compared to all other cell types (2.6x – 9x greater activity; p=0.0008 – 0.022; Figure 4D). Additionally, both monocyte subsets exhibited greater ADCP activity compared to the DCs (2.1x – 3.5x greater activity; p=0.001 – 0.026; Figure 4D). Against the HIV-ICs, granulocytes exhibited significantly greater ADCP than classical monocytes and DCs (4.6x – 13x greater activity; p=0.0003 – 0.0019; Figure 4D), while both monocyte subsets exhibited greater activity compared to DCs (2.8x – 6.7x greater activity; p=0.031 – 0.032; Figure 4D). Total CD45+ ADCP activity was significantly greater against the ICs when elicited by IgG4 compared to the virus targets (2.4x greater activity; p=0.0096 – 0.0225).
Additionally, given the general potency of granulocyte ADCP observed, the proportion of granulocytes for each sample studied was plotted against the total CD45+ ADCP scores for both the virus and IC ADCP assays, and grouped by IgG isotype used, in order to assess if the amount of granulocytes was related to a sample’s ADCP activity. No significant correlation was found for any of these datasets (Supplementary Figure 1).
ADCP Activity as Compared by IgG Isotype
Virus ADCP
When including all CD45+ leukocytes, it was evident that 246-D as IgG3 elicited ADCP of virions significantly better than as IgG2 or IgG4 (3.2x – 3.3x greater activity; p=0.017 – 0.026; Figure 5A). In terms of granulocyte ADCP, IgG1 activity was significantly greater than IgG2 activity (1.6x greater activity, p=0.030), while similar to total CD45+ leukocytes, IgG3 elicited granulocyte virion ADCP more strongly than IgG2 or IgG4 (3.7x – 4.4x greater activity; p=0.0013 – 0.0029; Figure 5A). No significant differences in ADCP activity was found for activated monocytes/macrophages when comparing IgG isotypes. In contrast, classical monocyte ADCP was elicited more strongly by IgG2, IgG3, and IgG4 compared to IgG1 (2.4x – 3.1x greater activity, p=0.0051 – 0.0297; Figure 5A). Similarly, dendritic cell ADCP was also elicited more strongly by IgG2, IgG3, and IgG4 compared to IgG1 (4.6x - 26.7x greater activity; p=0.0336 - p<0.0001; Figure 5A).
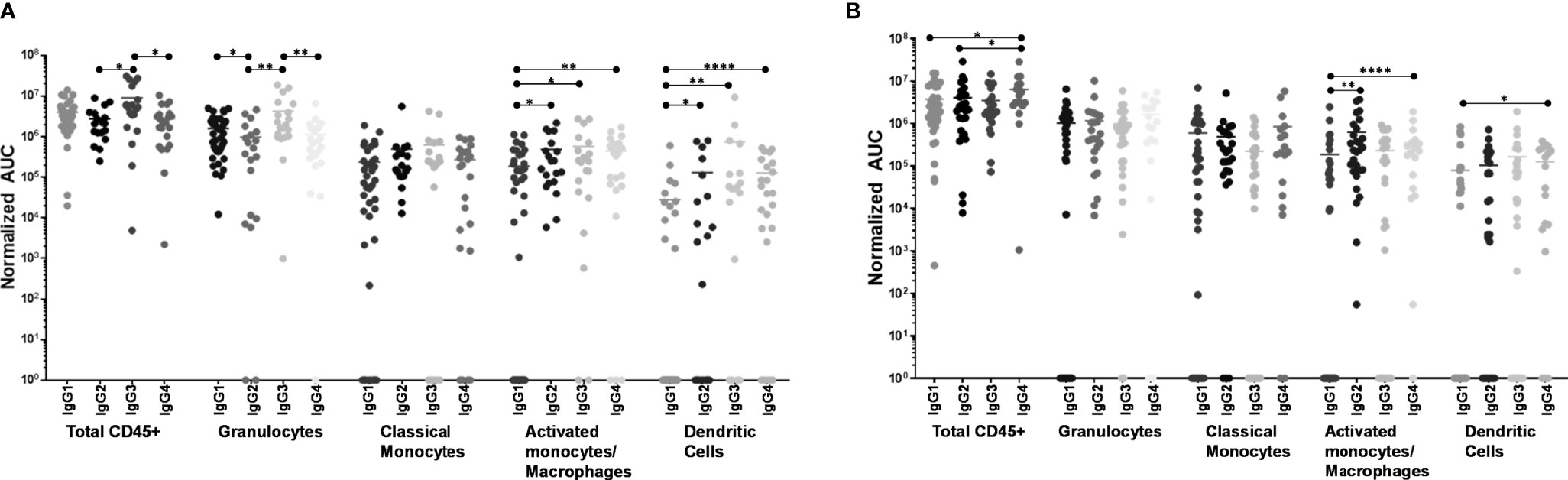
Figure 5 ADCP activity of each phagocyte subset as compared by IgG isotype. (A) Virus ADCP. (B) Immune complex (IC) ADCP. Experiments and analyses were performed as in Figure 4. ****p < 0.0001; **0.009 > p > 0.001; *0.05 > p > 0.01.
IC ADCP
Measuring total CD45+ cells, IgG4 was the most potent at eliciting IC ADCP compared to IgG1 and IgG2 (1.6x – 2.1x greater activity; p=0.0176 – 0.0399; Figure 5B). Granulocytes and activated monocytes/macrophages exhibited no significant differences in ADCP activity when comparing the IgG isotypes. Classical monocyte IC ADCP was elicited more strongly by IgG4 and IgG2 compared to IgG1 (1.9x and 3.4x greater activity; p=0.0035 and p<0.0001, respectively; Figure 5B). Lastly, it was also apparent that IgG4 elicited more potent IC ADCP by dendritic cells compared to IgG1 (1.6x greater activity; p=0.0121; Figure 5B).
Discussion
Each phagocyte subset expresses a unique FcγR repertoire that includes FcγRI, FcγRIIa/b/c, and/or FcγRIIIa/b (52). As well, FcR expression and function of phagocytes can vary by their tissue localization; for example, HIV-1-specific ADCP activity of colon-resident macrophages is ~10-fold lower compared to those found in the cervix, possibly due to comparably low expression levels of FcγRI and FcγRIII (53). Most FcγRs have a measurable affinity for all IgG isotypes, though selectivity for a given isotype certainly exists; moreover, most FcRs mediate cytoplasmic signaling, including FcγRIIa/FcγRIIc, which possess immunoreceptor tyrosine-based activation motif (ITAM) domains, and FcγRI/FcγRIIIa that signal via a γ2 chain containing an ITAM domain (33, 54). Binding to most FcγRs requires the Ab to be at least dimerized, i.e., part of an immune complex, and in this form, affinity experiments have shown that both IgG1 and IgG3 bind all FcγRs comparably, with only a slightly better binding profile exhibited by IgG3 (54). Even FcγRI, which can bind IgG monomers with high affinity, requires receptor clustering and cross-linking to signal effectively (55). Though a weakly-neutralizing gp41-specific Ab was used for these experiments because this is the type of Ab commonly elicited by HIV infection, most HIV Abs can elicit ADCP and it would be expected that (broadly) neutralizing Abs would yield similar results.
In the present study, granulocytes were the most potently induced phagocyte against the virus target for most IgG isotypes, consistent with our previous findings using bead-based targets (41). However, in terms of total milk leukocyte ADCP, the proportion of granulocytes in a given sample was not found to significantly influence activity. IgG2 did not appear to elicit granulocyte ADCP more potently than monocyte ADCP, possibly due to the apparent lack of binding affinity of IgG2 for FcγRIIIb, which is dominantly expressed on neutrophils, and for FcγRI, which neutrophils express when overstimulated (54, 56). Granulocyte dominance was less apparent for the IC targets, likely due to the extensive crosslinking of Fc-FcR interactions leading to similar levels of ADCP across the cell types tested, with the exception of IgG3, which induced highly potent ADCP by granulocytes of ICs compared to other cell types. Otherwise, IgG1/2/4 in this case more similarly stimulated granulocytes and activated monocytes/macrophages, or even classical monocytes in the case of IgG2. Overall, dendritic cells exhibited the lowest relative activity in terms of both virus and IC targets, though this would be expected given these cells comprise the smallest fraction of phagocytes analyzed.
The ADCP scores presented herein are a relative assessment of each cell population’s overall phagocytic contribution within each unique sample, as the scores take into account the percent of total CD45+ leukocytes each phagocyte subset comprises. The ADCP activity measured here is therefore a biologically applicable indication of the most relevant cells and isotypes to target for further studies that make use of these data. However, when directly comparing the ADCP activity induced by each IgG isotype for a given cell type, the absolute phagocytic potency can be better examined. In this case, it was apparent that examining all CD45+ leukocytes together, IgG3 and IgG1 elicited similar activity against the virus target, which is likely a reflection of the potent activity of granulocytes whose dominant FcγRIIIb expression limited IgG2 and IgG4-elicited ADCP activity due to the low affinity of this interaction even when measured in cell-surface, cross-linked binding studies (54). Classical CD14+CD16- milk monocytes were the least impacted by isotype, suggesting the high levels of Ab used in these experiments to opsonize the virus targets facilitated cross-liking of FcγRIIa to such an extent that differential affinities for each isotype was essentially irrelevant. Indeed, even moderate crosslinking of Ab acting on FcγRIIa has been shown to minimize any binding differences between the ‘high affinity’ IgG1/IgG3 and ‘low affinity’ IgG2/IgG4 (54). Though primary blood monocytes express high levels of FcγRI, which exhibits very poor IgG2 binding even when cross-linked, these data suggest that FcγRIIa activity accounted for this difference, and/or that milk monocyte FcγRI expression may be relatively low (57). IgG isotype also seemed to have minimal impact on activated monocyte/macrophage and DC ADCP; however, these cell types were most poorly stimulated by IgG1. Reasons for this are not clear, though it is possible that combined with the very limited expression of FcγRI expression on these cells (particularly DCs), IgG1 induction of ADCP may have exhausted the FcγRs and/or overstimulated the inhibitory FcγRIIb. Notably, in vivo, the high-affinity FcγRI may be consistently bound by monomeric IgG; as such, the lower affinity FcγRs are responsible for most observed Fc-mediated activities (58).
Overall, IC ADCP appeared to be best mediated by IgG4. This was most apparent for DCs, as well as for activated monocytes/macrophages, which also exhibited potent IgG2 activity. However, granulocyte activity against ICs appeared to be unaffected by IgG isotype. Granulocytes exhibited more potent ADCP of the smaller virus targets when elicited by the high affinity IgG1 and IgG3, but no difference in terms of IgG2 or IgG4, again likely a reflection of FcγRIIIb binding preferences. The significant IC crosslinking and/or target size appeared to overcome the typical lack of FcγRIIIb-IgG2/4 affinity, though it may be that the observed activity was triggered strongly by granulocyte FcγRIIa despite the lower expression levels expected on these cells.
It was apparent from these data that with extensive crosslinking of larger ICs, isotype of the effector IgG becomes considerably less relevant in the context of gp41-mediated ADCP. In fact, isotypes less classically associated with effector function, particularly IgG4, appear to function well in this context. Given the overall low numbers of DCs, they likely do not have a major impact on ADCP activity in milk, though they certainly can perform this function and likely contribute to viral clearance. As also observed in our previous study using gp120-coated bead targets, granulocytes are potent mediators of ADCP in milk, and one major finding based on the present data is that granulocytes – predominantly neutrophils - should be targeted therapeutically to mitigate MTCT of HIV via breastfeeding. As granulocytes express the FcγRs described above as well as relatively high levels of FcαR, these cells could be targeted by IgG or IgA. Depending on the therapeutic approach, be it mucosal administration of mAbs to elicit powerful enhancement of the natural passive immunity of HIV+ mothers’ milk, or a therapeutic maternal vaccine aimed to elicit a potent milk Ab response, it is evident that granulocyte preferences, in particular, for IgG isotype in terms of elicitation of ADCP should be considered. Should IgG3 be used therapeutically, these data suggest that granulocytes will be potently activated, though very high viral loads and/or very high mAb doses that might generate large ICs might reduce the potency of this effect, and careful titration experiments would have to be done in vivo, also taking into account the short half-life of this isotype (25). A therapeutic vaccine designed to elicit HIV-specific sIgA in may also be a powerful approach to reducing or eliminating MTCT via breastfeeding, and this subclass should not be dismissed, as it has been shown previously that HIV-specific milk (s)IgA titers were positively associated with reduced MTCT, and monomeric, dimeric, and sIgA can mediate potent FcαR-mediated function, including ADCP, against HIV and other pathogens (3, 59–62). Notably, Simian HIV (SHIV) vaccine regimens in Rhesus macaques have successfully induced specific IgA in milk, particularly those including an intramuscular (IM) prime + intranasal (IN) boost, and even more so if followed by an additional combined IN/IM boost (63). However, this IgA was largely monomeric, suggesting B cells secreting these Abs did not traffic to milk via the entero-mammary route (64). Follow-up study found this IgA insufficient to protect breastfeeding infant macaques from oral SHIV acquisition (64). In humans, vaccines that target the GALT may be ideal for enriching milk with HIV-specific sIgA. Studies on this topic must continue, and further exploration into the utility of Rhesus macaques as a model for human milk sIgA elicitation is needed, as the entero-mammary link in this species has not been adequately verified.
The data presented herein also suggest that IgG1 may not be an ideal choice for such therapeutics to fully harness the ADCP potential of milk monocytes, while high doses of IgG4 in a high viral load context may induce ADCP by all milk phagocytes. As IgG4 induction tends to occur after repeated and prolonged immunogen exposure, its ADCP functionality may become relevant should frequent maternal vaccine boosters be used (65). Importantly, though most HIV-specific Abs in milk have been found to be IgG, the dominant Ab class in milk is sIgA, and the Fc-mediated function of (s)IgA must be better understood, as it may be relevant therapeutically. As well, the present data have highlighted that the milk leukocyte FcR expression profiles should be further examined in subsequent studies. Given the substantial gap in present knowledge of the potential contribution of ADCP activity by milk phagocytes to prevention of MTCT of HIV, it is critical to develop a multidimensional, comprehensive understanding of ADCP by these relevant primary cells.
Data Availability Statement
The raw data supporting the conclusions of this article will be made available by the authors, without undue reservation.
Ethics Statement
Participants were recruited using social media and informed consent was obtained in accordance with the ethical and institutional board approval under the guidance and authorization of Mount Sinai’s Program for the Protection of Human Subjects (PPHS) using an IRB-approved protocol for obtaining human milk samples (STUDY-17-01089). The patients/participants provided their written informed consent to participate in this study.
Author Contributions
AF performed all experiments and reviewed manuscript. XL contributed to experiments and reviewed manuscript. SZ-P contributed to experiment planning and data analysis and reviewed manuscript. RP planned all experiments, analyzed all data, acquired funding, and wrote the manuscript. All authors contributed to the article and approved the submitted version.
Funding
This work was supported by the NIH/NICHD (grant #R21 HD095772) and the Icahn School of Medicine at Mount Sinai.
Conflict of Interest
The authors declare that the research was conducted in the absence of any commercial or financial relationships that could be construed as a potential conflict of interest.
Publisher’s Note
All claims expressed in this article are solely those of the authors and do not necessarily represent those of their affiliated organizations, or those of the publisher, the editors and the reviewers. Any product that may be evaluated in this article, or claim that may be made by its manufacturer, is not guaranteed or endorsed by the publisher.
Acknowledgments
We are indebted to our milk donors. HIV-GFP was generously gifted from the Benjamin Chen lab.
Supplementary Material
The Supplementary Material for this article can be found online at: https://www.frontiersin.org/articles/10.3389/fimmu.2022.831767/full#supplementary-material
Supplementary Figure 1 | Proportion of granulocytes in a milk sample is not related to total ADCP activity of CD45+ leukocytes. Percent granulocytes of total cells and of CD45+ cells for each milk sample analyzed were plotted against the total CD45+ leukocyte ADCP scores for virus ADCP or IC ADCP assays, grouped by IgG isotype. Datasets were assessed for correlation by nonparametric Spearman test. No significant correlations were found.
References
1. World Health Organization Guideline: Updates on HIV and Infant Feeding: The Duration of Breastfeeding, and Support from Health Services to Improve Feeding Practices Among Mothers Living with HIV. World Health Organization Guidelines Approved by the Guidelines Review Committee. Geneva: World Health Organization (2016).
2. Fowler MG, Lampe MA, Jamieson DJ, Kourtis AP, Rogers MF. Reducing the Risk of Mother-to-Child Human Immunodeficiency Virus Transmission: Past Successes, Current Progress and Challenges, and Future Directions. Am J Obstet Gynecol (2007) 197:S3–9. doi: 10.1016/j.ajog.2007.06.048
3. Pollara J, McGuire E, Fouda GG, Rountree W, Eudailey J, Overman RG, et al. Association of HIV-1 Envelope-Specific Breast Milk IgA Responses With Reduced Risk of Postnatal Mother-To-Child Transmission of HIV-1. J Virol (2015) 89:9952–61. doi: 10.1128/JVI.01560-15
4. Arsenault JE, Webb AL, Koulinska IN, Aboud S, Fawzi WW, Villamor E. Association Between Breast Milk Erythropoietin and Reduced Risk of Mother-to-Child Transmission of HIV. J Infect Dis (2010) 202:370–3. doi: 10.1086/653706
5. Villamor E, Koulinska IN, Furtado J, Baylin A, Aboud S, Manji K, et al. Long-Chain N-6 Polyunsaturated Fatty Acids in Breast Milk Decrease the Risk of HIV Transmission Through Breastfeeding. Am J Clin Nutr (2007) 86:682–9. doi: 10.1093/ajcn/86.3.682
6. Walter J, Ghosh MK, Kuhn L, Semrau K, Sinkala M, Kankasa C, et al. High Concentrations of Interleukin 15 in Breast Milk Are Associated With Protection Against Postnatal HIV Transmission. J Infect Dis (2009) 200:1498–502. doi: 10.1086/644603
7. Kuhn L, Trabattoni D, Kankasa C, Semrau K, Kasonde P, Lissoni F, et al. Alpha-Defensins in the Prevention of HIV Transmission Among Breastfed Infants. J Acquir Immune Defic Syndr (2005) 39:138–42.
8. Bode L, Kuhn L, Kim HY, Hsiao L, Nissan C, Sinkala M, et al. Human Milk Oligosaccharide Concentration and Risk of Postnatal Transmission of HIV Through Breastfeeding. Am J Clin Nutr (2012) 96:831–9. doi: 10.3945/ajcn.112.039503
9. Groot F, Geijtenbeek TB, Sanders RW, Baldwin CE, Sanchez-Hernandez M, Floris R, et al. Lactoferrin Prevents Dendritic Cell-Mediated Human Immunodeficiency Virus Type 1 Transmission by Blocking the DC-SIGN–gp120 Interaction. J Virol (2005) 79:3009–15. doi: 10.1128/JVI.79.5.3009-3015.2005
10. Habte HH, de Beer C, Lotz ZE, Tyler MG, Kahn D, Mall AS. Inhibition of Human Immunodeficiency Virus Type 1 Activity by Purified Human Breast Milk Mucin (MUC1) in an Inhibition Assay. Neonatology (2008) 93:162–70. doi: 10.1159/000108414
11. Wahl A, Swanson MD, Nochi T, Olesen R, Denton PW, Chateau M, et al. Human Breast Milk and Antiretrovirals Dramatically Reduce Oral HIV-1 Transmission in BLT Humanized Mice. PloS Pathog (2012) 8:e1002732. doi: 10.1371/journal.ppat.1002732
12. Wahl A, Baker C, Spagnuolo RA, Stamper LW, Fouda GG, Permar SR, et al. Breast Milk of HIV-Positive Mothers Has Potent and Species-Specific In Vivo HIV-Inhibitory Activity. J Virol (2015) 89:10868–78. doi: 10.1128/JVI.01702-15
13. Hassiotou F, Geddes DT, Hartmann PE. Cells in Human Milk: State of the Science. J Hum Lact (2013) 29:171–82. doi: 10.1177/0890334413477242
14. Lonnerdal B. Nutritional and Physiologic Significance of Human Milk Proteins. Am J Clin Nutr (2003) 77:1537S–43S. doi: 10.1093/ajcn/77.6.1537S
15. Butte NF, Garza C, Stuff JE, Smith EO, Nichols BL. Effect of Maternal Diet and Body Composition on Lactational Performance. Am J Clin Nutr (1984) 39:296–306. doi: 10.1093/ajcn/39.2.296
16. Dewey KG, Finley DA, Lonnerdal B. Breast Milk Volume and Composition During Late Lactation (7-20 Months). J Pediatr Gastroenterol Nutr (1984) 3:713–20. doi: 10.1097/00005176-198411000-00014
17. Hassiotou F, Geddes DT. Immune Cell-Mediated Protection of the Mammary Gland and the Infant During Breastfeeding. Adv Nutr (2015) 6:267–75. doi: 10.3945/an.114.007377
18. Hanson LA. The Mother-Offspring Dyad and the Immune System. Acta Paediatr (2000) 89:252–8. doi: 10.1111/j.1651-2227.2000.tb01325.x
19. Wirt DP, Adkins LT, Palkowetz KH, Schmalstieg FC, Goldman AS. Activated and Memory T Lymphocytes in Human Milk. Cytometry (1992) 13:282–90. doi: 10.1002/cyto.990130310
20. Jain L, Vidyasagar D, Xanthou M, Ghai V, Shimada S, Blend M. In Vivo Distribution of Human Milk Leucocytes After Ingestion by Newborn Baboons. Arch Dis Child (1989) 64:930–3. doi: 10.1136/adc.64.7_Spec_No.930
21. Zhou L, Yoshimura Y, Huang Y, Suzuki R, Yokoyama M, Okabe M, et al. Two Independent Pathways of Maternal Cell Transmission to Offspring: Through Placenta During Pregnancy and by Breast-Feeding After Birth. Immunology (2000) 101:570–80. doi: 10.1046/j.1365-2567.2000.00144.x
22. Tuboly S, Bernath S. Intestinal Absorption of Colostral Lymphoid Cells in Newborn Animals. Adv Exp Med Biol (2002) 503:107–14. doi: 10.1007/978-1-4615-0559-4_12
23. Cabinian A, Sinsimer D, Tang M, Zumba O, Mehta H, Toma A, et al. Transfer of Maternal Immune Cells by Breastfeeding: Maternal Cytotoxic T Lymphocytes Present in Breast Milk Localize in the Peyer's Patches of the Nursed Infant. PloS One (2016) 11:e0156762. doi: 10.1371/journal.pone.0156762
24. Filias A, Theodorou GL, Mouzopoulou S, Varvarigou AA, Mantagos S, Karakantza M. Phagocytic Ability of Neutrophils and Monocytes in Neonates. BMC Pediatr (2011) 11:29. doi: 10.1186/1471-2431-11-29
26. Sacha CR, Vandergrift N, Jeffries TL Jr., McGuire E, Fouda GG, Liebl B, et al. Restricted Isotype, Distinct Variable Gene Usage, and High Rate of Gp120 Specificity of HIV-1 Envelope-Specific B Cells in Colostrum Compared With Those in Blood of HIV-1-Infected, Lactating African Women. Mucosal Immunol (2015) 8:316–26. doi: 10.1038/mi.2014.69
27. Friedman J, Alam SM, Shen X, Xia SM, Stewart S, Anasti K, et al. Isolation of HIV-1-Neutralizing Mucosal Monoclonal Antibodies From Human Colostrum. PloS One (2012) 7:e37648. doi: 10.1371/journal.pone.0037648
28. Tuaillon E, Valea D, Becquart P, Al Tabaa Y, Meda N, Bollore K, et al. Human Milk-Derived B Cells: A Highly Activated Switched Memory Cell Population Primed to Secrete Antibodies. J Immunol (2009) 182:7155–62. doi: 10.4049/jimmunol.0803107
29. Tay MZ, Kunz EL, Deal A, Zhang L, Seaton KE, Rountree W, et al. Rare Detection of Antiviral Functions of Polyclonal IgA Isolated From Plasma and Breast Milk Compartments in Women Chronically Infected With HIV-1. J Virol (2019) 93(7):e02084–18. doi: 10.1128/JVI.02084-18
30. Permar SR, Wilks AB, Ehlinger EP, Kang HH, Mahlokozera T, Coffey RT, et al. Limited Contribution of Mucosal IgA to Simian Immunodeficiency Virus (SIV)-Specific Neutralizing Antibody Response and Virus Envelope Evolution in Breast Milk of SIV-Infected, Lactating Rhesus Monkeys. J Virol (2010) 84:8209–18. doi: 10.1128/JVI.00656-10
31. Weaver LT, Arthur HM, Bunn JE, Thomas JE. Human Milk IgA Concentrations During the First Year of Lactation. Arch Dis Child (1998) 78:235–9. doi: 10.1136/adc.78.3.235
32. Mabuka J, Nduati R, Odem-Davis K, Peterson D, Overbaugh J. HIV-Specific Antibodies Capable of ADCC Are Common in Breastmilk and Are Associated With Reduced Risk of Transmission in Women With High Viral Loads. PloS Pathog (2012) 8:e1002739. doi: 10.1371/journal.ppat.1002739
33. Tay MZ, Wiehe K, Pollara J. Antibody-Dependent Cellular Phagocytosis in Antiviral Immune Responses. Front Immunol (2019) 10:332. doi: 10.3389/fimmu.2019.00332
34. Yates NL, Liao HX, Fong Y, deCamp A, Vandergrift NA, Williams WT, et al. Vaccine-Induced Env V1-V2 IgG3 Correlates With Lower HIV-1 Infection Risk and Declines Soon After Vaccination. Sci Transl Med (2014) 6:228ra39. doi: 10.1126/scitranslmed.3007730
35. Chung AW, Ghebremichael M, Robinson H, Brown E, Choi I, Lane S, et al. Polyfunctional Fc-Effector Profiles Mediated by IgG Subclass Selection Distinguish RV144 and VAX003 Vaccines. Sci Transl Med (2014) 6:228ra38. doi: 10.1126/scitranslmed.3007736
36. Rerks-Ngarm S, Pitisuttithum P, Nitayaphan S, Kaewkungwal J, Chiu J, Paris R, et al. Vaccination With ALVAC and AIDSVAX to Prevent HIV-1 Infection in Thailand. N Engl J Med (2009) 361:2209–20. doi: 10.1056/NEJMoa0908492
37. Forthal DN, Landucci G, Bream J, Jacobson LP, Phan TB, Montoya B. FcgammaRIIa Genotype Predicts Progression of HIV Infection. J Immunol (2007) 179:7916–23. doi: 10.4049/jimmunol.179.11.7916
38. Ackerman ME, Dugast AS, McAndrew EG, Tsoukas S, Licht AF, Irvine DJ, et al. Enhanced Phagocytic Activity of HIV-Specific Antibodies Correlates With Natural Production of Immunoglobulins With Skewed Affinity for FcgammaR2a and FcgammaR2b. J Virol (2013) 87:5468–76. doi: 10.1128/JVI.03403-12
39. Trend S, de Jong E, Lloyd ML, Kok CH, Richmond P, Doherty DA, et al. Leukocyte Populations in Human Preterm and Term Breast Milk Identified by Multicolour Flow Cytometry. PloS One (2015) 10:e0135580. doi: 10.1371/journal.pone.0135580
40. Powell RLR, Fox A. Isolation of Leukocytes From Human Breast Milk for Use in an Antibody-Dependent Cellular Phagocytosis Assay of HIV Targets. J Vis Exp (2019) 6(151) :e60149. doi: 10.3791/60149
41. Powell RLR, Fox A, Liu X, Itri V. Phagocytosis of a Model Human Immunodeficiency Virus Target by Human Breast Milk Leukocytes Is Predominantly Granulocyte-Driven When Elicited by Specific Antibody. Breastfeed Med (2019) 14:185–92. doi: 10.1089/bfm.2018.0232
42. Gorny MK, Xu JY, Gianakakos V, Karwowska S, Williams C, Sheppard HW, et al. Production of Site-Selected Neutralizing Human Monoclonal Antibodies Against the Third Variable Domain of the Human Immunodeficiency Virus Type 1 Envelope Glycoprotein. Proc Natl Acad Sci USA (1991) 88:3238–42. doi: 10.1073/pnas.88.8.3238
43. Hioe CE, Wrin T, Seaman MS, Yu X, Wood B, Self S, et al. Anti-V3 Monoclonal Antibodies Display Broad Neutralizing Activities Against Multiple HIV-1 Subtypes. PloS One (2010) 5:e10254. doi: 10.1371/journal.pone.0010254
44. Musich T, Li L, Liu L, Zolla-Pazner S, Robert-Guroff M, Gorny MK. Monoclonal Antibodies Specific for the V2, V3, CD4-Binding Site, and Gp41 of HIV-1 Mediate Phagocytosis in a Dose-Dependent Manner. J Virol (2017) 91(8):e02325–16. doi: 10.1128/JVI.02325-16
45. Tiller T, Meffre E, Yurasov S, Tsuiji M, Nussenzweig MC, Wardemann H. Efficient Generation of Monoclonal Antibodies From Single Human B Cells by Single Cell RT-PCR and Expression Vector Cloning. J Immunol Methods (2008) 329:112–24. doi: 10.1016/j.jim.2007.09.017
46. Hubner W, Chen P, Del Portillo A, Liu Y, Gordon RE, Chen BK. Sequence of Human Immunodeficiency Virus Type 1 (HIV-1) Gag Localization and Oligomerization Monitored With Live Confocal Imaging of a Replication-Competent, Fluorescently Tagged HIV-1. J Virol (2007) 81:12596–607. doi: 10.1128/JVI.01088-07
47. Ackerman ME, Moldt B, Wyatt RT, Dugast AS, McAndrew E, Tsoukas S, et al. A Robust, High-Throughput Assay to Determine the Phagocytic Activity of Clinical Antibody Samples. J Immunol Methods (2011) 366:8–19. doi: 10.1016/j.jim.2010.12.016
48. Gach JS, Bouzin M, Wong MP, Chromikova V, Gorlani A, Yu KT, et al. Human Immunodeficiency Virus Type-1 (HIV-1) Evades Antibody-Dependent Phagocytosis. PloS Pathog (2017) 13:e1006793. doi: 10.1371/journal.ppat.1006793
49. Faucher JL, Lacronique-Gazaille C, Frebet E, Trimoreau F, Donnard M, Bordessoule D, et al. "6 Markers/5 Colors" Extended White Blood Cell Differential by Flow Cytometry. Cytometry A (2007) 71:934–44. doi: 10.1002/cyto.a.20457
50. Autissier P, Soulas C, Burdo TH, Williams KC. Evaluation of a 12-Color Flow Cytometry Panel to Study Lymphocyte, Monocyte, and Dendritic Cell Subsets in Humans. Cytometry A (2010) 77:410–9. doi: 10.1002/cyto.a.20859
51. Zhu H, Sun X, Zhu L, Hu F, Shi L, Li Z, et al. The Expression and Clinical Significance of Different Forms of Mer Receptor Tyrosine Kinase in Systemic Lupus Erythematosus. J Immunol Res (2014) 2014:431896. doi: 10.1155/2014/431896
52. Guilliams M, Bruhns P, Saeys Y, Hammad H, Lambrecht BN. The Function of Fcgamma Receptors in Dendritic Cells and Macrophages. Nat Rev Immunol (2014) 14:94–108. doi: 10.1038/nri3582
53. Sips M, Krykbaeva M, Diefenbach TJ, Ghebremichael M, Bowman BA, Dugast AS, et al. Fc Receptor-Mediated Phagocytosis in Tissues as a Potent Mechanism for Preventive and Therapeutic HIV Vaccine Strategies. Mucosal Immunol (2016) 9(6):1584–95. doi: 10.1038/mi.2016.12
54. Bruhns P, Iannascoli B, England P, Mancardi DA, Fernandez N, Jorieux S, et al. Specificity and Affinity of Human Fcgamma Receptors and Their Polymorphic Variants for Human IgG Subclasses. Blood (2009) 113:3716–25. doi: 10.1182/blood-2008-09-179754
55. Duchemin AM, Ernst LK, Anderson CL. Clustering of the High Affinity Fc Receptor for Immunoglobulin G (Fc Gamma RI) Results in Phosphorylation of Its Associated Gamma-Chain. J Biol Chem (1994) 269:12111–7. doi: 10.1016/S0021-9258(17)32688-1
56. Bournazos S, Gupta A, Ravetch JV. The Role of IgG Fc Receptors in Antibody-Dependent Enhancement. Nat Rev Immunol (2020) 20:633–43. doi: 10.1038/s41577-020-00410-0
57. Tay MZ, Liu P, Williams LD, McRaven MD, Sawant S, Gurley TC, et al. Antibody-Mediated Internalization of Infectious HIV-1 Virions Differs Among Antibody Isotypes and Subclasses. PloS Pathog (2016) 12:e1005817. doi: 10.1371/journal.ppat.1005817
58. Hayes JM, Wormald MR, Rudd PM, Davey GP. Fc Gamma Receptors: Glycobiology and Therapeutic Prospects. J Inflamm Res (2016) 9:209–19. doi: 10.2147/JIR.S121233
59. Duchemin M, Tudor D, Cottignies-Calamarte A, Bomsel M. Antibody-Dependent Cellular Phagocytosis of HIV-1-Infected Cells Is Efficiently Triggered by IgA Targeting HIV-1 Envelope Subunit Gp41. Front Immunol (2020) 11:1141. doi: 10.3389/fimmu.2020.01141
60. Monteiro RC, Van De Winkel JG. IgA Fc Receptors. Annu Rev Immunol (2003) 21:177–204. doi: 10.1146/annurev.immunol.21.120601.141011
61. Franca EL, Morceli G, Fagundes DL, Rudge MV, Calderon Ide M, Honorio-Franca AC. Secretory IgA-Fcalpha Receptor Interaction Modulating Phagocytosis and Microbicidal Activity by Phagocytes in Human Colostrum of Diabetics. APMIS (2011) 119:710–9. doi: 10.1111/j.1600-0463.2011.02789.x
62. Musich T, Demberg T, Morgan IL, Estes JD, Franchini G, Robert-Guroff M. Purification and Functional Characterization of Mucosal IgA From Vaccinated and SIV-Infected Rhesus Macaques. Clin Immunol (2015) 158:127–39. doi: 10.1016/j.clim.2015.03.020
63. Nelson CS, Pollara J, Kunz EL, Jeffries TL Jr, Duffy R, Beck C, et al. Combined HIV-1 Envelope Systemic and Mucosal Immunization of Lactating Rhesus Monkeys Induces a Robust Immunoglobulin A Isotype B Cell Response in Breast Milk. J Virol (2016) 90:4951–65. doi: 10.1128/JVI.00335-16
64. Eudailey JA, Dennis ML, Parker ME, Phillips BL, Huffman TN, Bay CP, et al. Maternal HIV-1 Env Vaccination for Systemic and Breast Milk Immunity To Prevent Oral SHIV Acquisition in Infant Macaques. mSphere (2018) 3(1):e00505–17. doi: 10.1128/mSphere.00505-17
Keywords: human milk, lactation, ADCP, phagocytosis, mother-to-child HIV transmission, HIV, IgG, phagocytes
Citation: Fox A, Liu X, Zolla-Pazner S and Powell RL (2022) Impact of IgG Isotype on the Induction of Antibody-Dependent Cellular Phagocytosis of HIV by Human Milk Leukocytes. Front. Immunol. 13:831767. doi: 10.3389/fimmu.2022.831767
Received: 08 December 2021; Accepted: 04 April 2022;
Published: 03 May 2022.
Edited by:
Justin Pollara, Duke University, United StatesReviewed by:
Stephanie N. Langel, Duke University, United StatesMarjorie Robert-Guroff, National Cancer Institute (NIH), United States
Copyright © 2022 Fox, Liu, Zolla-Pazner and Powell. This is an open-access article distributed under the terms of the Creative Commons Attribution License (CC BY). The use, distribution or reproduction in other forums is permitted, provided the original author(s) and the copyright owner(s) are credited and that the original publication in this journal is cited, in accordance with accepted academic practice. No use, distribution or reproduction is permitted which does not comply with these terms.
*Correspondence: Rebecca L. Powell, rebecca.powell@mssm.edu