- 1Pediatric Hematology/Oncology, Fondazione Istituto di Ricovero e Cura a Carattere Scientifico Policlinico San Matteo, Pavia, Italy
- 2Cell Factory, Fondazione Istituto di Ricovero e Cura a Carattere Scientifico Policlinico San Matteo, Pavia, Italy
- 3Immunohematology and Transfusion Service, Fondazione Istituto di Ricovero e Cura a Carattere Scientifico Policlinico San Matteo, Pavia, Italy
- 4Virology Service, Fondazione Istituto di Ricovero e Cura a Carattere Scientifico Policlinico San Matteo, University of Pavia, Pavia, Italy
Post-transplant lymphoproliferative disorders (PTLDs) are life-threatening complications of iatrogenic immune impairment after allogeneic hematopoietic stem cell transplantation (HSCT). In the pediatric setting, the majority of PTLDs are related to the Epstein–Barr virus (EBV) infection, and present as B-cell lymphoproliferations. Although considered rare events, PTLDs have been increasingly observed with the widening application of HSCT from alternative sources, including cord blood and HLA-haploidentical stem cell grafts, and the use of novel agents for the prevention and treatment of rejection and graft-vs.-host disease. The higher frequency initially paralleled a poor outcome, due to limited therapeutic options, and scarcity of controlled trials in a rare disease context. In the last 2 decades, insight into the relationship between EBV and the immune system, and advances in early diagnosis, monitoring and treatment have changed the approach to the management of PTLDs after HSCT, and significantly ameliorated the prognosis. In this review, we summarize literature on the impact of combined viro-immunologic assessment on PTLD management, describe the various strategies for PTLD prevention and preemptive/curative treatment, and discuss the potential of novel immune-based therapies in the containment of this malignant complication.
Introduction
Post-transplant lymphoproliferative disorders (PTLD) are heterogeneous lymphoproliferative diseases that stem from the unchecked proliferation of neoplastic lymphoid or plasmacytic cells in the setting of immunosuppression after transplantation (1–3).
PTLD in the hematopoietic stem cell transplantation (HSCT) setting are almost exclusively related to Epstein-Barr virus (EBV) infection; they usually develop between 3 and 6 months post-transplant, when virus-specific T cell immunity has not yet reconstituted, and are generally of donor origin. Although recipient-derived PTLDs have been described, they occur mainly in patients with poor graft reconstitution.
This review outlines our current understanding of the interplay between the virus and the immune system in the pathogenesis of these disorders after HSCT, and how our knowledge has improved current approaches to the management of PTLD in this clinical setting.
Interactions Between EBV And the Host
EBV is a human γ-herpesvirus that infects more than 90% of the individuals worldwide (4–6). The virus enters the organism through the oropharyngeal route, and, in healthy subjects, causes a self-limiting primary infection. In normal, seropositive individuals, virus neutralizing antibodies control the spread of infectious virus particles and EBV-specific, HLA class I restricted, CD8+ cytotoxic T lymphocytes (CTL) specific for the early lytic cycle proteins kill cells entering the lytic cycle before they are able to release infectious virus particles (7).
The virus is B lymphotropic, and persists in resting memory B cells for the lifetime of the host in a non-pathogenic state that is invisible to the immune response (8). Initially, EBV infects naïve B cells in tonsillar lymphoepithelium, driving their activation through the expression of nine latent proteins (EBV nuclear antigens, EBNAs 1, 2, 3a, 3b, 3c, and LP and membrane antigens LMP 1, 2a and 2b), two small non-translated RNAs and about 40 microRNAs that constitute the EBV growth program (9). CTL directed to EBV latent cycle antigens prevent the outgrowth of cells latently infected with the virus (7).
Thence, the virus biology parallels that of normal mature B lymphocytes. EBV-infected naïve B-cells migrate to germinal centers in lymph nodes, lymphoid tissue present in mucosa, or the spleen. In germinal centers, normal B-cells undergo activation-induced somatic hypermutation and class switch recombination of the antigen-binding variable region of immunoglobulin genes. Within the germinal center, EBV-positive B cells shift to a more restricted virus transcription program, the default program (EBNA1, LMP1, and LMP2a expression), that helps rescue them into the memory compartment where the virus persists (6). Expression of viral proteins provides EBV-infected naïve B-cells with a selective advantage in the germinal center, and stimulates maturation into memory B-cells, which are the presumed reservoir of EBV (10).
Memory cells latently infected with EBV in the peripheral blood are in the latency program, and do not express any of the known latent proteins, unless they undergo division, in which case they express EBNA1, essential for the maintenance of the viral episome in dividing cells (8, 9). The frequency of infected memory B cells in a healthy carrier is stable over time, although it varies among different individuals, and has been calculated to be around 0.5 × 106, with only 1% residing in the peripheral blood (10). The virus is no longer pathogenic to the host, as the genes that drive cellular proliferation and may lead to neoplastic disease are no longer expressed. Likewise, the virus is safe from immune surveillance, as immunogenic viral protein expression, which serves as a target for the immune system is absent.
Interplay Between EBV and the Immune System: Pathogenesis of PTLD After HSCT
EBV is considered an oncogenic virus, because of its association with tumors. EBV has latent proteins that can drive cellular proliferation, at least in B lymphocytes, such as LMP1 and LMP2, and these likely play a causative role in tumor development through inappropriate or deregulated gene expression (4, 8). HSCT recipients have impaired T-cell mediated immunity due to the pre-transplant conditioning regimen, immunosuppressive agents for prophylaxis of graft-vs.-host disease (GVHD) and GVHD itself (11–16). The reduced numbers of EBV-specific CTLs facilitate uninhibited growth of EBV-infected cells (17, 18). However, only a small number of EBV-positive patients develop PTLD after HSCT or other conditions of immunosuppression (11), and advanced PTLD is an oligoclonal rather than polyclonal disease, suggesting that other rare events contribute to the pathogenesis of the disorder. Thus, in order to have PTLD development, the growth program must be erroneously expressed in a B cell that cannot exit the cell cycle, and immunosuppression must prevent the elimination of these rare cells. At this stage, the disease may still be controlled by intervening on the immune status. Indeed, PTLD patients in early stages of disease may regress in response to the reduction of immunosuppression (19, 20) or after donor lymphocyte (DLI) (21) or EBV-specific CTL infusion (18, 22), strongly pointing to the essential role played by the underlying state of immunosuppression. However, in the absence of T cell immunity, such as it is often observed after T-cell depleted HSCT, proliferating cells acquire additional genetic or epigenetic damage, and these new cell clones may become unresponsive to immune surveillance (23).
A similar pathogenetic mechanism may be hypothesized in the rare cases of EBV-positive PTLD of T-cell origin. It has been postulated that some T cells may express CD21, the EBV receptor on B cells, and thus may allow viral entry (24).
Risk Factors FOR EBV-PTLD After HSCT
Development of PTLD after HSCT is mainly associated with T-cell depletion of the graft before transplantation and the type/duration of immunosuppression employed to prevent and treat graft-vs.-host disease, and the degree of mismatch between recipient and donor (1, 2, 25–28). Consequently, PTLD is more often observed in T-cell depleted HSCT from haploidentical donors.
Among ex-vivo approaches, elective T cell depletion methods are associated with a greater increase in PTLD risk (26), as donor EBV-targeted cytotoxic T cells are removed from the inoculum, thus compromising specific immune surveillance. However, the use of lymphocyte depletion strategies that target both T and B cells, such as in vitro alemtuzumab (26) or combined depletion of αβ T-cell and CD19 B-cells (29), have a lower risk of PTLD, by delaying potential EBV-infected B cell proliferation until recovery of functional T cell immunity. This observation supports the concept that an imbalance between EBV-infected B cells and EBV-specific T cells favors neoplastic outgrowth of EBV-positive B cells. Likewise, in vivo depletion of T-cells using antithymocyte globulin (ATG) is associated with a higher risk of developing PTLD than the use of broad lymphocyte-targeting alemtuzumab monoclonal antibody (Mab) (26, 30, 31). Rabbit ATG was suggested to be more likely to cause profound lymphodepletion than horse ATG (32). However, a recent study in the setting of pediatric and adult haploidentical HSCT show comparable rates of EBV DNAemia and PTLD (33). The effect of ATG seems dose-dependent, as high-dose ATG had a 2.3-fold higher risk of PTLD than low-dose (30, 34).
The degree of HLA matching between donor and recipient correlates with the development of PTLD. HSCT from a HLA-mismatched donor has been observed to be associated with a higher risk of PTLD than the use of a HLA-identical donor (26, 27). Although a certain degree of mismatch between recipient and donor may impair EBV antigen recognition by HLA-restricted donor T cells, the risk associated with HLA mismatch is mainly due to the in vitro and/or in vivo T-cell depletion strategies employed in mismatched transplants to facilitate engraftment and prevent GVHD: the combination of different depletion approaches results in additive risk (26).
The incidence of PTLD with regard to the different types of donors ranges from 1% in HSCT from matched related donors to 4% for matched unrelated and 11% for mismatched unrelated donors (20). Among different stem cell sources, cord blood was associated with a greater risk of PTLD (30), due to low numbers and naiveté of infused T cells that likely delay early immune reconstitution, although there is no evidence of delayed virus-specific immune recovery in pediatric CBT recipients beyond the first 100 days post-transplant (35). Moreover, there is in vitro evidence that CB lymphocytes may mediate a sizeable immune response directed against autologous EBV-infected cells, exerted by both NK cells and CD4+ T lymphocytes (36). The development of graft engineering strategies and pharmacologic GVHD prevention protocols, together with optimal conditioning regimens, have significantly ameliorated the outcomes of haploidentical HSCT, and this progress has led to a widespread use of the procedure (18, 29, 37–46). Interestingly, despite the high degree of mismatch and the procedures employed to facilitate engraftment and prevent GVHD, the incidence of PTLD with the newest platforms for haplo-HSCT, either T-cell and B-cell depleted (29, 43) or T-cell repleted with post-transplant cyclophosphamide (PTCy) (44–46), are unexpectedly low. In the case of PTCy, the incidence is <3% (47), possibly due to the destruction of EBV-infected B cells, together with an immune reconstitution that is hypothesized to be faster than that observed after the use of ATG (48).
Among other risk factors relevant for pediatric HSCT recipients, a higher incidence of PTLD has been observed in recipients of allogeneic HSCT conditioned with a reduced-intensity regimen (49), and the development of acute or chronic GVHD (20, 26, 27, 30), due to a delay in the reconstitution of functional specific immunity. Finally, EBV serology mismatch, in particular EBV-seronegative patients receiving grafts from seropositive donors, are also at increased risk for PTLD development (25, 27).
Some studies have suggested that significant factors could be combined within a prognostic model. Three single-center risk factor scoring systems have been published, but their use in common clinical practice is limited and needs to be validated (25, 27, 30).
Epidemiology, Clinical Presentation and Diagnosis
EBV-PTLD is a severe complication that occurs in 1–3.5% of HSCT recipients (20, 50), although incidence rates may exceed 10% in patients with established risk factors (2, 20, 27, 28, 50). An expansion in the indications for HSCT from alternative donors, including haploidentical family members, and the use of novel T-cell depletion strategies, together with improved diagnostic protocols, have led to the observation of an increased incidence of PTLD in the last 2 decades (20). However, greater awareness of the disorder has fueled studies that have addressed PTLD preemptive/preventive strategies, and facilitated patient management.
Patients with PTLD after HSCT generally present with fever, lymphadenopathy, tonsil enlargement or discrete organ lesions, although the disease may manifest as a systemic process that mimics fulminant sepsis syndrome (2, 28). Primary central nervous system (CNS) localization of PTLD is rare, and generally burdened with a dismal prognosis (20), partly due to the challenges associated with limited drug penetration across the blood-brain barrier. In order to overcome this peculiar feature, intrathecal drug delivery has been proposed (51).
The diagnosis of EBV-PTLD is based on symptoms and/or signs consistent with PTLD, together with the quantitative determination of EBV-DNAemia or detection of EBV in a specimen from the involved tissue (1, 2), and imaging studies, such as computed tomography (CT) or positron emission tomography CT (PET-CT). Definitive diagnosis of EBV-PTLD requires biopsy of sites suspected for EBV disease and histological examination. EBV detection requires identification of viral antigens or in situ hybridization for the EBER transcripts. The histological WHO 2016 classification includes six morphological types of PTLD: plasmacytic hyperplasia, infectious mononucleosis-like, florid follicular hyperplasia, polymorphic, monomorphic (B-cell or T-/NK-cell types), and classical Hodgkin lymphoma PTLD (52).
EBV-PTLD may be diagnosed at the probable or proven level (53). Probable EBV disease is defined as the presence of symptoms and/or signs of lymphoproliferative disease in the absence of tissue biopsy, but without other documented causes, together with significant EBV DNAemia, measured in any blood specimen. Diagnosis of proven EBV-PTLD requires detection of EBV nucleic acids or EBV-encoded proteins in a tissue sample.
Early Identification of Patients at Risk of PTLD
The development of EBV-PTLD after HSCT represents a life threatening event; mortality is still relevant, at 30 and 40% of diagnosed cases (20, 54). The onset of PTLD is preceded by a pre-clinical phase denoted by increased EBV DNA levels in the peripheral blood. Indeed, it has been demonstrated that, irrespective of baseline characteristics, the post-transplant monitoring of peripheral EBV viral load after HSCT is effective in predicting risk of EBV-PTLD (18, 55–61).
Thus, according to international guidelines, prospective monitoring of EBV DNA should be started within the first month after HSCT, and continued on a weekly basis at least until the fourth post-transplant month (1). The frequency and duration of EBV DNAemia screening should be based on the risk profile of the transplanted patients (62).
EBV DNA analysis is an indispensable tool for early diagnosis and the application of preemptive strategies to avoid progression of early-stage PTLD to oligoclonal/monoclonal disease (18, 63). However, even with the available data there is not a defined EBV-DNA threshold for prompt initiation of preemptive therapy (1), as EBV PCR assays are not standardized (63), and evidence has been obtained in cohorts with heterogeneous clinical characteristics using different peripheral blood specimens. Thresholds for assays using mononuclear cells, plasma, or whole blood in the reported studies range from 1,000 to 40.000 copies/ml according to the source, and data on the best specimen source are inconclusive (1, 59–61, 64, 65). Moreover, probable/proven PTLD has been described in a proportion of patients with EBV DNA levels below commonly adopted intervention thresholds (66, 67). Thus, it seems rational to adopt validated center-specific cut-off values, tailored on the specific cohort characteristics, and employ the rate of EBV DNA level increase, that is an indicator of EBV-infected B cells, as a predictor of when to start preemptive interventions. Regarding peripheral blood specimen choice, a recent study including 121 pediatric and adult HSCT recipients evaluated the kinetics of EBV DNA, assessed with a molecular method approved by regulatory agencies, in paired whole blood and plasma samples during episodes of post-transplant EBV infection, and found that plasma had a low sensitivity for identifying PTLD, thus suggesting a preferential use for whole blood in the post-transplant management of infection (64). Some studies indicated that plasma measurement may be useful in the follow-up after treatment, but these studies included high numbers of solid organ transplant recipients, and data are yet not conclusive (60).
EBV DNA analysis is not a precise predictor of PTLD development, and tailoring screening on the basis of a whole cohort is not always practical, feasible, or successful. As the other central factor determining progression to PTLD is the lack of a protective immune response, it seems reasonable to associate DNAemia screening with analysis of immune reconstitution. This approach has been used successfully for other viral infections in HSCT or solid organ transplant patients (13, 68–75), and has been proposed by some groups also in the setting of EBV infection and PTLD after HSCT (18, 76–82). Although studies are largely descriptive and based on the use of different technologies, the results suggest that numbers and function of virus-specific T cells inversely correlate with viral DNA levels and risk of disease, whereby strong cellular immune responses are associated with containment of viral replication or EBV-infected B cell outgrowth. The key obstacles to the introduction of EBV-specific T cell quantification into clinical practice is the definition of reliable cutoffs for clinical decision making for the different assays, and the absence of controlled interventional clinical trials.
Prevention of PTLD After HSCT
There are two possible approaches for prevention of EBV-PTLD after HSCT: prophylaxis and pre-emptive therapy (53, 83, 84). Prophylaxis of EBV disease includes any intervention applied to an asymptomatic patient to prevent EBV DNAemia. Pre-emptive therapy includes any intervention given to a patient with EBV DNAemia to prevent EBV disease.
Prophylaxis
In the setting of HSCT, there are two strategies to prevent EBV DNAemia. The first is based on interventions on the graft or the patient prior to HSCT, in order to decrease the risk of EBV-infected B cell outgrowth. As we have already seen, in the case of T-cell depleted HSCT, the use of in vitro or in vivo methods that deplete B cells as well as T cells reduce the risk of PTLD by temporarily removing the EBV reservoir and potential EBV-transformed B lymphoblasts, at least until functional immune reconstitution is achieved (28, 29, 43, 44, 47). If no T-cell depletion is employed, but the risk of PTLD is high due to the use of ATG and/or the presence of HLA mismatches between donor and recipient, peri-transplant B-cell depletion by rituximab may be considered (85). The efficacy of peritransplant rituximab was suggested by observations in adult patients receiving anti-CD20 monoclonal antibody close to HSCT, as part of their treatment for B cell malignancies (85), and was tested in a study from the European Group for Blood and Marrow Transplantation (EBMT) as part of the conditioning regimen for pediatric and adult patients with severe aplastic anemia (86). Based on these studies, peritransplant rituximab has been employed in pediatric recipients of αβ T-cell/B-cell depleted haploidentical HSCT, and the combination of in vitro and in vivo B cell depletion succeeded in counteracting the risk of PTLD given by T-cell depletion, ATG and HLA mismatch (29, 87). Relevantly, rituximab role in controlling acute and chronic GVHD may also favorably impact PTLD development (29, 85–87).
Regarding the use of ATG to prevent rejection and GVHD, given that the increased risk of PTLD is dose-dependent, in pediatric allogeneic HSCT a lower therapeutic dose may be administered. Indeed, a recent multicenter randomized trial has shown that 15 vs. 30 mg/kg rabbit ATG was equally effective in preventing acute and chronic GVHD, but was associated with lower relapse and non-relapse mortality in pediatric patients receiving UD HSCT for hematologic malignancies (88). An alternative GVHD prophylaxis, that may have a direct impact on PTLD development due to its anti-tumor activity, is the use of mTOR inhibitors (89, 90).
Post-transplant prophylactic administration of agents, such as antiviral drugs, rituximab and EBV-CTLs has been proposed. Treatment of latent EBV with antivirals has been unsuccessful, as latently infected B cells do not express the EBV thymidine kinase enzyme (1, 84).
In a large retrospective study, prophylactic post-transplant rituximab significantly reduced the risk of EBV DNAemia (91); however, no statistically significant impact on PTLD incidence, treatment-related mortality, and overall survival in comparison to a pre-emptive approach was shown. Post-transplant rituximab is associated with cytopenias (92) and delayed B cell recovery with an increased risk of infections (93), that seem less evident with peritransplant use. Thus, prophylactic rituximab post-HSCT ought to be employed with caution (1). The use of prophylactic EBV-CTLs, pioneered by Rooney et al. in high-risk, pediatric unrelated-donor HSCT recipients, has been highly successful, and devoid of side effects (22, 94) (Table 1). None of the 101 patients who received CTLs as prophylaxis developed PTLD compared with 11.5% of controls. As the donors were EBV-seropositive, even in the absence of circulating EBV one may hypothesize that the efficacy of this treatment was due to stimulation by EBV present in patient tissues or donor B cells, or just cross-stimulation of low-affinity T cells present in the infused product by other antigens. Current use of EBV-CTLs is, however, limited to a few selected centers.
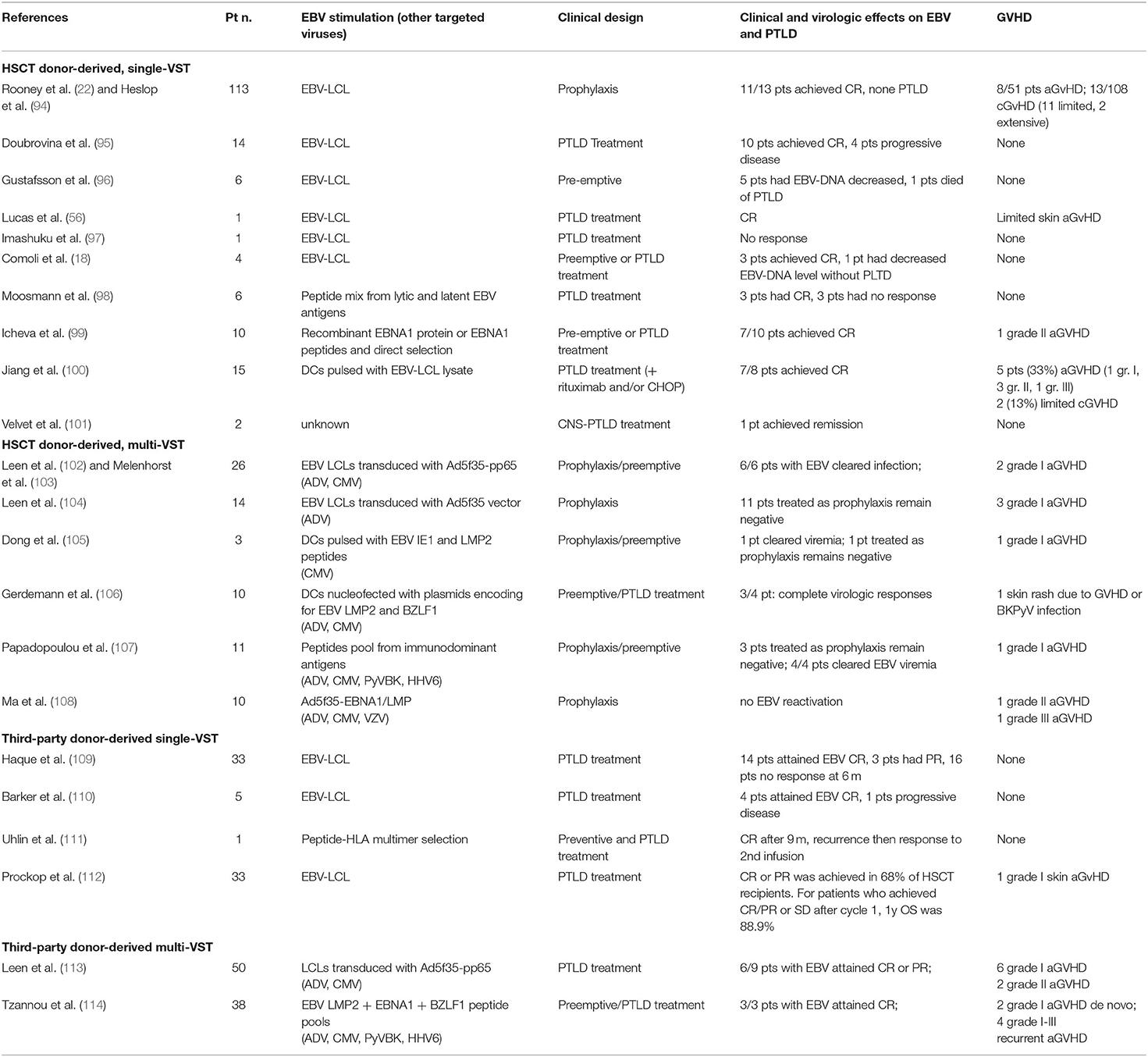
Table 1. Results of published trials using EBV-specific T cells to prevent or treat EBV infection and PTLD.
Preemptive Therapy
The mainstay of pre-emptive therapy for EBV PTLD after HSCT is anti-CD20 antibody rituximab, given at increase in EBV-DNA load especially in patients lacking T-cell reconstitution (18, 63, 86, 115), due to its acceptable toxicity and widespread availability (1, 84). A retrospective study reviewed the results of more than 300 patients described in reported case series, and found that successful prevention of PTLD was observed in almost 90% of treated patients (84). Pre-emptive rituximab is employed at the dose of 375 mg/m2, once weekly until EBV DNAemia is found negative. Dose number should be assessed on the basis of EBV DNAemia monitoring and on the patient's specific immune recovery, but 1–4 doses are generally sufficient. However, it has been shown that, in certain circumstances, clearance of EBV DNA from peripheral blood may not reflect a long-lasting response (67). Limitations of this approach are selection for CD20-negative clones (18), and the fact that rituximab acts on the EBV reservoir, rather than on restoration of the cellular immune response to EBV, which is central to the long-term control of EBV mediated B-cell proliferation (18, 72).
Among the strategies that boost specific immune reconstitution and immune surveillance, reduction of maintenance immunosuppression (IS), whenever feasible in the absence of GVHD, should be employed in association with anti-CD20 Mab therapy (1). Data on IS reduction employed alone are too limited to derive any useful indications (19). The use of donor EBV-specific T cells in unrelated donor (UD) or haplo-HSCT in a pre-emptive approach has been very successful (18, 96, 116–119), with long-lasting EBV viral load clearance in more than 90% of patients, and responses observed also in patients with increased viral load after rituximab treatment (18) (Table 1). In HSCT recipients, EBV-CTL therapy enhances virus-specific immune responses, and allows establishment of a memory T cell response, observed for as long as 9 years after T cell administration (117). No major toxicity was observed (118), and the reported rate of new-onset GVHD was around 1% (119). When the donor is not available, or is EBV-seronegative, or to increase access to T cell treatment, the use of third-party CTLs has been advocated (109). The first reported study used banked EBV-specific CTLs to treat PTLD after solid organ or HSCT, matching by low resolution HLA typing and screening for absence of alloreactivity, obtaining 50% responses in established PTLD and no GVHD development (109). Since then, a number of studies have further explored this option and refined matching criteria by evaluating activity against viral epitopes through the shared HLA allele (110–114, 119) (Table 1). A recent study treated 33 HSCT recipients with third-party CTLs, obtaining a 68% remission rate, with a 89% overall survival. Interestingly, patients in progression after the first cycle benefited from a change in CTL donor (9% survival after repeated cycles with same donor CTLs vs. 60% survival after donor switch) (112).
Conclusions
Prognosis of EBV PTLD after HSCT is still suboptimal. Because of the relatively low incidence of this complication, and its particular situation related to the post-HSCT period, there is limited evidence on the best treatment strategy for established disease failing first line treatment.
Thus, therapeutic strategies with high efficacy and minimal toxic effects for HSCT patients at high risk of PTLD are a clinical need. Knowledge on the interplay between the virus and the host immune system (120) has allowed the design of tailored management approaches, based on longitudinal combined virological and immunological testing, and the development of novel cellular therapeutic agents burdened with little toxicity, and therefore suitable for employment in pre-emptive therapeutic strategies. Limitations to the pre-emptive approach are related to the difficulty in establishing viral load cut-off values for the start and discontinuation of therapeutic interventions, and standardized and cellular immunity assays with validated thresholds, together with limited availability of cellular therapies. These hurdles may be overcome by a general effort in standardization, which has already begun, and by local management. So far, the use of EBV-specific T cells has been limited to the few academic centers with infrastructure resources to produce advanced cellular therapies. Recently, excellent cell therapy clinical results, together with the development of new methodologies to obtain rapid manufacture of third-party T cells, have fuelled considerable interest from the Pharmaceutical industry to bring to the market third-party cellular therapies including EBV CTLs. Further efforts are required to design the most appropriate clinical trials to rapidly identify efficient combinatorial approaches, and to invent new and sustainable reimbursement modalities for novel therapies.
Author Contributions
FC, SB, AP, JB, LS, AM, TM, PZ, CP, FB, MZ, and PC all participated in writing the manuscript. FC, SB, MZ, and PC co-edited the final version of the manuscript. All authors have read and approved the final manuscript.
Funding
This work was supported by grants from the Fondazione Regionale per la Ricerca Biomedica (PC); Fondazione Istituto di Ricovero e Cura a Carattere Scientifico (IRCCS) Policlinico San Matteo [Ricerca Corrente 08069113 and 08069119 (PC); Ricerca Corrente 08045818 (MZ); Ricerca Corrente 08071519 (SB)].
Conflict of Interest
The authors declare that the research was conducted in the absence of any commercial or financial relationships that could be construed as a potential conflict of interest.
Abbreviations
PTLD, Post-transplant lymphoproliferative disorders; HSCT, hematopoietic stem cell transplantation; EBV, Epstein-Barr virus; CTL, Cytotoxic T lymphocytes; a/cGVHD, acute/chronic graft-vs.-host disease; ATG, antithymocyte globulin; Mab, monoclonal antibody; CBT, cord blood transplantation; PTCy, post-transplant cyclophosphamide; PCR, polymerase chain reaction; IS, immunosuppression; UD, unrelated donor.
References
1. Styczynski J, van der Velden W, Fox CP, Engelhard D, de la Camara R, Cordonnier C, et al. Management of epstein-barr virus infections and post-transplant lymphoproliferative disorders in patients after allogeneic hematopoietic stem cell transplantation: sixth European conference on infections in leukemia (ECIL-6) guidelines. Haematologica. (2016) 101:803–11. doi: 10.3324/haematol.2016.144428
2. Dierickx D, Habermann TM. Post-transplantation lymphoproliferative disorders in adults. N Engl J Med. (2018) 378:549–62. doi: 10.1056/NEJMra1702693
3. Dharnidharka VR, Webster AC, Martinez OM, Preiksaitis JK, Leblond V, Choquet S. Post-transplant lymphoproliferative disorders. Nat Rev Dis Primers. (2016) 2:15088. doi: 10.1038/nrdp.2015.88
4. Cohen JI. Epstein–barr virus infection. N Engl J Med. (2000) 343:481–92. doi: 10.1056/NEJM200008173430707
5. Young LS, Rickinson AB. Epstein–barr virus: 40 years on. Nat Rev Cancer. (2004) 4:757–68. doi: 10.1038/nrc1452
6. Kieff E, Rickinson AB. Epstein-barr virus and its replication. In: Knipe DM, Howley PM, editors. Fields Virology. Philadelphia, PA: Lippincott Williams & Wilkins (2007).
7. Rickinson AB, Moss DJ. Human cytotoxic T lymphocyte responses to epstein-barr virus infection. Annu Rev Immunol. (1997) 15:405–31. doi: 10.1146/annurev.immunol.15.1.405
8. Thorley-Lawson DA, Gross A. Persistence of the epstein-barr virus and the origins of associated lymphomas. N Engl J Med. (2004) 350:1328–37. doi: 10.1056/NEJMra032015
9. Babcock GJ, Hochberg D, Thorley-Lawson AD. The expression pattern of epstein-barr virus latent genes in vivo is dependent upon the differentiation stage of the infected B cell. Immunity. (2000) 13:497–506. doi: 10.1016/S1074-7613(00)00049-2
10. Thorley-Lawson DA. EBV persistence-introducing the virus. Curr Top Microbiol Immunol. (2015) 390:151–209. doi: 10.1007/978-3-319-22822-8_8
11. Parkman R, Weinberg KI. Immunological reconstitution following bone marrow transplantation. Immunol Rev. (1997) 157:73–8. doi: 10.1111/j.1600-065X.1997.tb00975.x
12. Small TN, Papadopoulos EB, Boulad F, Black P, Castro-Malaspina H, Childs BH, et al. Comparison of immune reconstitution after unrelated and related T-cell-depleted bone marrow transplantation: effect of patient age and donor leukocyte infusions. Blood. (1999) 93:467–80. doi: 10.1182/blood.V93.2.467
13. Lilleri D, Gerna G, Fornara C, Chiesa A, Comolli G, Zecca M, et al. Human cytomegalovirus-specific T cell reconstitution in young patients receiving T cell-depleted, allogeneic hematopoietic stem cell transplantation. J Infect Dis. (2009) 199:829–36. doi: 10.1086/597123
14. Bartelink IH, Belitser SV, Knibbe CA, Danhof M, de Pagter AJ, Egberts TC, et al. Immune reconstitution kinetics as an early predictor for mortality using various hematopoietic stem cell sources in children. Biol Blood Marrow Transplant. (2013) 19:305–13. doi: 10.1016/j.bbmt.2012.10.010
15. Toubert A, Glauzy S, Douay C, Clave E. Thymus and immune reconstitution after allogeneic hematopoietic stem cell transplantation in humans: never say never again. Tissue Antigens. (2012) 79:83–9. doi: 10.1111/j.1399-0039.2011.01820.x
16. Bondanza A, Ruggeri L, Noviello M, Eikema DJ, Bonini C, Chabannon C, et al. Beneficial role of CD8+ T-cell reconstitution after HLA-haploidentical stem cell transplantation for high-risk acute leukaemias: results from a clinico-biological EBMT registry study mostly in the T-cell-depleted setting. Bone Marrow Transplant. (2019) 54:867–76. doi: 10.1038/s41409-018-0351-x
17. Lucas KG, Small TN, Heller G, Dupont B, O'Reilly RJ. The development of cellular immunity to epstein-barr virus after allogeneic bone marrow transplantation. Blood. (1996) 87:2594–603. doi: 10.1182/blood.V87.6.2594.bloodjournal8762594
18. Comoli P, Basso S, Zecca M, Pagliara D, Baldanti F, Bernardo ME, et al. Preemptive therapy of EBV-related lymphoproliferative disease after pediatric haploidentical stem cell transplantation. Am J Transplant. (2007) 7:1648–55. doi: 10.1111/j.1600-6143.2007.01823.x
19. Cesaro S, Pegoraro A, Tridello G, Calore E, Pillon M, Varotto S, et al. A prospective study on modulation of immunosuppression for epstein-barr virus reactivation in pediatric patients who underwent unrelated hematopoietic stem-cell transplantation. Transplantation. (2010) 89:1533–40. doi: 10.1097/TP.0b013e3181dd6c0a
20. Styczynski J, Gil L, Tridello G, Ljungman P, Donnelly JP, van der Velden W, et al. Response to rituximab-based therapy and risk factor analysis in epstein barr virus-related lymphoproliferative disorder after hematopoietic stem cell transplant in children and adults: a study from the infectious diseases working party of the European group for blood and marrow transplantation. Clin Infect Dis. (2013) 57:794–802. doi: 10.1093/cid/cit391
21. Papadopoulos EB, Ladanyi M, Emanuel D, Mackinnon S, Boulad F, Matthew H, et al. Infusions of donor leukocytes as treatment of epstein–barr virus associated lymphoproliferative disorders complicating allogeneic marrow transplantation. N Engl J Med. (1994) 330:1185–91. doi: 10.1056/NEJM199404283301703
22. Rooney CM, Smith CA, Ng CY, Loftin S, Li C, Krance RA, et al. Use of gene-modified virus-specific T lymphocytes to control epstein–barr-virusrelated lymphoproliferation. Lancet. (1995) 345:9–12. doi: 10.1016/S0140-6736(95)91150-2
23. Capello D, Rossi D, Gaidano G. Post-transplant lymphoproliferative disorders: molecular basis of disease histogenesis and pathogenesis. Hematol Oncol. (2005) 23:61–7. doi: 10.1002/hon.751
24. Fischer E, Delibrias C, Kazatchkine MD. Expression of CR2 (the C3dg/EBV receptor, CD21) on normal human peripheral blood T lymphocytes. J Immunol. (1991) 146:865–9.
25. Sundin M, Le Blanc K, Ringden O, Barkholt L, Omazic B, Lergin C, et al. The role of HLA mismatch, splenectomy and recipient epstein-barr virus seronegativity as risk factors in post-transplant lymphoproliferative disorder following allogeneic hematopoietic stem cell transplantation. Haematologica. (2006) 91:1059–67.
26. Landgren O, Gilbert ES, Rizzo JD, Socie G, Banks PM, Sobocinski KA, et al. Risk factors for lymphoproliferative disorders after allogeneic hematopoietic cell transplantation. Blood. (2009) 113:4992–5001. doi: 10.1182/blood-2008-09-178046
27. Uhlin M, Wikell H, Sundin M, Blennow O, Maeurer M, Ringden O, et al. Risk factors for epstein-barr virus-related post-transplant lymphoproliferative disease after allogeneic hematopoietic stem cell transplantation. Haematologica. (2014) 2:346–52. doi: 10.3324/haematol.2013.087338
28. Al Hamed R, Bazarbachi AH, Mohty M. Epstein-barr virus-related post-transplant lymphoproliferative disease (EBV-PTLD) in the setting of allogeneic stem cell transplantation: a comprehensive review from pathogenesis to forthcoming treatment modalities. Bone Marrow Transplant. (2020) 55:25–39. doi: 10.1038/s41409-019-0548-7
29. Locatelli F, Merli P, Pagliara D, Li Pira G, Falco M, Pende D, et al. Outcome of children with acute leukemia given HLA-haploidentical HSCT after αβ T-cell and B-cell depletion. Blood. (2017) 130:677–85. doi: 10.1182/blood-2017-04-779769
30. Fujimoto A, Hiramoto N, Yamasaki S, Inamoto Y, Uchida N, Maeda T, et al. Risk factors and predictive scoring system for post-transplant lymphoproliferative disorder after hematopoietic stem cell transplantation. Biol Blood Marrow Transplant. (2019) 25:1441–9. doi: 10.1016/j.bbmt.2019.02.016
31. Hoegh-Petersen M, Goodyear D, Geddes MN, Liu S, Ugarte-Torres A, Liu Y, et al. High incidence of posttransplant lymphoproliferative disorder after antithymocyte globulin-based conditioning and ineffective prediction by day 28 EBV-specific T lymphocyte counts. Bone Marrow Transplant. (2011) 46:1104–12. doi: 10.1038/bmt.2010.272
32. Scheinberg P, Nunez O, Weinstein B, Scheinberg P, Biancotto A, Wu CO, et al. Horse versus rabbit antithymocyte globulin in acquired aplastic anemia. N Engl J Med. (2011) 365:430–8. doi: 10.1056/NEJMoa1103975
33. Zhou L, Gao ZY, Lu DP. Comparison of ATG-thymoglobulin with ATG-Fresenius for epstein-barr virus infections and graft-versus-host-disease in patients with hematological malignances after haploidentical hematopoietic stem cell transplantation: a single-center experience. Ann Hematol. (2020) 99:1389–400. doi: 10.1007/s00277-020-04014-5
34. Mensen A, Na IK, Hafer R, Li Pira G, Falco M, Pende D, et al. Comparison of different rabbit ATG preparation effects on early lymphocyte subset recovery after allogeneic HSCT and its association with EBV-mediated PTLD. J Cancer Res Clin Oncol. (2014) 140:1971–80. doi: 10.1007/s00432-014-1742-z
35. Szabolcs P, Cairo MS. Unrelated umbilical cord blood transplantation and immune reconstitution. Semin Hematol. (2010) 4:22–36. doi: 10.1053/j.seminhematol.2009.10.009
36. Moretta A, Comoli P, Montagna D, Gasparoni A, Percivalle E, Carena I, et al. High frequency of epstein–barr virus (EBV) lymphoblastoid cell line-reactive lymphocytes in cord blood: evaluation of cytolytic activity and IL-2 production. Clin Exp Immunol. (1997) 107:312–20. doi: 10.1111/j.1365-2249.1997.258-ce1131.x
37. Aversa F, Tabilio A, Velardi A, Cunningham I, Terenzi A, Falzetti F, et al. Treatment of high-risk acute leukemia with T-cell-depleted stem cells from related donors with one fully mismatched HLA haplotype. N Engl J Med. (1998) 339:1186–93. doi: 10.1056/NEJM199810223391702
38. Buckley RH, Schiff SE, Schiff RI, Markert L, Williams LW, Roberts JL, et al. Hematopoietic stem-cell transplantation for the treatment of severe combined immunodeficiency. N Engl J Med. (1998) 340:508–16. doi: 10.1056/NEJM199902183400703
39. Marks DI, Khattry N, Cummins M, Goulden N, Green A, Harvey J, et al. Haploidentical stemcell transplantation for children with acute leukaemia. Br J Haematol. (2006) 134:196–201. doi: 10.1111/j.1365-2141.2006.06140.x
40. Handgretinger R, Schumm M, Lang P, Greil J, Reiter A, Bader P, et al. Transplantation of megadoses of purified haploidentical stem cells. Ann N Y Acad Sci. (1999) 872:351–61. doi: 10.1111/j.1749-6632.1999.tb08479.x
41. Chen X, Hale GA, Barfield R, Benaim E, Leung WH, Knowles J, et al. Rapid immune reconstitution after a reduced-intensity conditioning regimen and a CD3-depleted haploidentical stem cell graft for paediatric refractory haematological malignancies. Br J Haematol. (2006) 135:524–32. doi: 10.1111/j.1365-2141.2006.06330.x
42. Klingebiel T, Cornish J, Labopin M, Locatelli F, Darbyshire P, Handgretinger R, et al. Results and factors influencing outcome after fully haploidentical hematopoietic stem cell transplantation in children with very high-risk acute lymphoblastic leukemia: impact of center size: an analysis on behalf of the acute leukemia and pediatric disease working parties of the European blood and marrow transplant group. Blood. (2010) 115:3437–46. doi: 10.1182/blood-2009-03-207001
43. Bertaina A, Zecca M, Buldini B, Sacchi N, Algeri M, Saglio F, et al. Unrelated donor vs HLA-haploidentical α/β T-cell- and B-cell-depleted HSCT in children with acute leukemia. Blood. (2018) 132:2594–607. doi: 10.1182/blood-2018-07-861575
44. Luznik L, O'Donnell PV, Symons HJ, Chen AR, Leffell MS, Zahurak M, et al. HLA-haploidentical bone marrow transplantation for hematologic malignancies using nonmyeloablative conditioning and high-dose, posttransplantation cyclophosphamide. Biol Blood Marrow Transplant. (2008) 14:641–50. doi: 10.1016/j.bbmt.2008.03.005
45. Berger M, Lanino E, Cesaro S, Zecca M, Vassallo E, Faraci M, et al. Feasibility and outcome of haploidentical hematopoietic stem cell transplantation with post-transplant high-dose cyclophosphamide for children and adolescents with hematologic malignancies: an AIEOP-GITMO retrospective multicenter study. Biol Blood Marrow Transplant. (2016) 22:902–9. doi: 10.1016/j.bbmt.2016.02.002
46. Jaiswal SR, Chakrabarti A, Chatterjee S, Bhargava S, Ray K, O'Donnell P, et al. Haploidentical peripheral blood stem cell transplantation with post-transplantation cyclophosphamide in children with advanced acute leukemia with fludarabine-, busulfan-, and melphalan-based conditioning. Biol Blood Marrow Transplant. (2016) 22:499–504. doi: 10.1016/j.bbmt.2015.11.010
47. Kanakry JA, Kasamon YL, Bolanos-Meade J, Borrello IM, Brodsky RA, Fuchs EJ, et al. Absence of post-transplantation lymphoproliferative disorder after allogeneic blood or marrow transplantation using post-transplantation cyclophosphamide as graft-versus-host disease prophylaxis. Biol Blood Marrow Transplant. (2013) 19:1514–7. doi: 10.1016/j.bbmt.2013.07.013
48. Retière C, Willem C, Guillaume T, Vié H, Gautreau-Rolland L, Scotet E, et al. Impact on early outcomes and immune reconstitution of high-dose post-transplant cyclophosphamide vs anti-thymocyte globulin after reduced intensity conditioning peripheral blood stem cell allogeneic transplantation. Oncotarget. (2018) 9:11451–64. doi: 10.18632/oncotarget.24328
49. Cohen JM, Cooper N, Chakrabarti S, Thomson K, Samarasinghe S, Cubitt D, et al. EBV-related disease following haematopoietic stem cell transplantation with reduced intensity conditioning. Leuk Lymphoma. (2007) 48:256–69. doi: 10.1080/10428190601059837
50. Curtis RE, Travis LB, Rowlings PA, Socie G, Kingma DW, Banks PM, et al. Risk of lymphoproliferative disorders after bone marrow transplantation: a multi-institutional study. Blood. (1999) 94:2208–16.
51. Bonney DK, Htwe EE, Turner A, Kelsey A, Shabani A, Hughes S, et al. Sustained response to intrathecal rituximab in EBV associated post-transplant lymphoproliferative disease confined to the central nervous system following haematopoietic stem cell transplant. Pediatr Blood Cancer. (2012) 58:459–61. doi: 10.1002/pbc.23134
52. Swerdlow SH, Campo E, Pileri SA, Harris NL, Stein H, Siebert R, et al. The 2016 revision of the world health organization classification of lymphoid neoplasms. Blood. (2016) 127:2375–90. doi: 10.1182/blood-2016-01-643569
53. Styczynski J, Reusser P, Einsele H, de la Camara R, Cordonnier C, Ward KN, et al. Management of HSV, VZV and EBV infections in patients with hematological malignancies and after SCT: guidelines from the second European conference on infections in leukemia. Bone Marrow Transplant. (2009) 43:757–70. doi: 10.1038/bmt.2008.386
54. Naik S, Riches M, Hari P, Kim S, Chen M, Bachier C, et al. Survival outcomes of allogeneic hematopoietic cell transplants with EBV-positive or EBV-negative post-transplant lymphoproliferative disorder, a CIBMTR study. Transpl Infect Dis. (2019) 21:e13145. doi: 10.1111/tid.13145
55. Rooney CM, Loftin SK, Holladay MS, Brenner MK, Krance RA, Heslop HE. Early identification of epstein-barr virus-associated post-transplantation lymphoproliferative disease. Br J Haematol. (1995) 89:98–103. doi: 10.1111/j.1365-2141.1995.tb08904.x
56. Lucas KG, Burton RL, Zimmerman SE, Wang J, Cornetta KG, Robertson KA, et al. Semiquantitative epstein-barr virus (EBV) polymerase chain reaction for the determination of patients at risk for EBV-induced lymphoproliferative disease after stem cell transplantation. Blood. (1998) 91:3654–61. doi: 10.1182/blood.V91.10.3654.3654_3654_3661
57. van Esser JW, van der Holt B, Meijer E, Niesters HG, Trenschel R, Thijsen SF, et al. Epstein-barr virus (EBV) reactivation is a frequent event after allogeneic stem cell transplantation (SCT) and quantitatively predicts EBV-lymphoproliferative disease following T-cell-depleted SCT. Blood. (2001) 98:972. doi: 10.1182/blood.V98.4.972
58. Baldanti F, Grossi P, Furione M, Simoncini L, Sarasini A, Comoli P, et al. High levels of epstein-barr virus DNA in blood of solid-organ transplant recipients and their value in predicting posttransplant lymphoproliferative disorders. J Clin Microbiol. (2000) 38:613–9. doi: 10.1128/JCM.38.2.613-619.2000
59. Baldanti F, Gatti M, Furione M, Paolucci S, Tinelli C, Comoli P, et al. Kinetics of epstein–barr virus DNA load in different blood compartments of pediatric recipients of T-cell depleted HLA haploidentical stem cell transplantation. J Clin Microbiol. (2008) 46:3672–7. doi: 10.1128/JCM.00913-08
60. Hakim H, Gibson C, Pan J, Srivastava K, Gu Z, Bankowski MJ, et al. Comparison of various blood compartments and reporting units for the detection and quantification of epstein-barr virus in peripheral blood. J Clin Microbiol. (2007) 45:2151–5. doi: 10.1128/JCM.02308-06
61. Ruf S, Behnke-Hall K, Gruhn B, Bauer J, Horn M, Beck J, et al. Comparison of six different specimen types for epstein-barr viral load quantification in peripheral blood of pediatric patients after heart transplantation or after allogeneic hematopoietic stem cell transplantation. J Clin Virol. (2012) 53:186–94. doi: 10.1016/j.jcv.2011.11.010
62. Wareham NE, Mocroft A, Sengeløv H, Da Cunha-Bang C, Gustafsson F, Heilmann C, et al. The value of EBV DNA in early detection of post-transplant lymphoproliferative disorders among solid organ and hematopoietic stem cell transplant recipients. J Cancer Res Clin Oncol. (2018) 144:1569–80. doi: 10.1007/s00432-018-2674-9
63. van Esser JWJ, Niesters HGM, van der Holt B, Meijer E, Osterhaus A, Gratama JW, et al. Prevention of epstein-barr virus-lymphoproliferative disease by molecular monitoring and preemptive rituximab in high risk patients after allogeneic stem cell transplantation. Blood. (2002) 99:4364–9. doi: 10.1182/blood.V99.12.4364
64. Preiksaitis JK, Pang XL, Fox JD, Fenton JM, Caliendo AM, Miller GG. Interlaboratory comparison of epstein-barr virus viral load assays. Am J Transplant. (2009) 9:269–79. doi: 10.1111/j.1600-6143.2008.02514.x
65. Lazzarotto T, Chiereghin A, Piralla A, Piccirilli G, Girello A, Campanini G, et al. Cytomegalovirus and epstein-barr virus DNA kinetics in whole blood and plasma of allogeneic hematopoietic stem cell transplantation recipients. Biol Blood Marrow Transplant. (2018) 24:1699–706. doi: 10.1016/j.bbmt.2018.03.005
66. Fox CP, Burns D, Parker AN, Peggs KS, Harvey CM, Natarajan S, et al. EBV-associated post-transplant lymphoproliferative disorder following in vivo T-cell-depleted allogeneic transplantation: clinical features, viral load correlates and prognostic factors in the rituximab era. Bone Marrow Transplant. (2014) 49:280–6. doi: 10.1038/bmt.2013.170
67. Yang J, Tao Q, Flinn IW, Murray PG, Post LE, Ma H, et al. Characterization of epstein-barr virus-infected B cells in patients with posttransplantation lymphoproliferative disease: disappearance after rituximab therapy does not predict clinical response. Blood. (2000) 96:4055–63. doi: 10.1182/blood.V96.13.4055
68. Lilleri D, Gerna G, Zelini P, Chiesa A, Rognoni V, Mastronuzzi A, et al. Monitoring of human cytomegalovirus and virus-specific T-cell response in young patients receiving allogeneic hematopoietic stem cell transplantation. PLoS ONE. (2012) 7:e41648. doi: 10.1371/journal.pone.0041648
69. Ginevri F, Azzi A, Hirsch HH, Basso S, Fontana I, Cioni M, et al. Prospective monitoring of polyomavirus BK replication and impact of pre-emptive intervention in pediatric kidney recipients. Am J Transplant. (2007) 7:2727. doi: 10.1111/j.1600-6143.2007.01984.x
70. Mattes FM, Vargas A, Kopycinski J, Hainsworth EG, Sweny P, Nebbia G, et al. Functional impairment of cytomegalovirus specific CD8 T cells predicts high-level replication after renal transplantation. Am J Transplant. (2008) 8:990–9. doi: 10.1111/j.1600-6143.2008.02191.x
71. Kumar D, Chernenko S, Moussa G, Cobos I, Manuel O, Preiksaitis J, et al. Cell-mediated immunity to predict cytomegalovirus disease in high-risk solid organ transplant recipients. Am J Transplant. (2009) 9:1214–22. doi: 10.1111/j.1600-6143.2009.02618.x
72. Savoldo B, Rooney CM, Quiros-Tejeira RE, Caldwell Y, Wagner HJ, Lee T, et al. Cellular immunity to epstein-barr virus in liver transplant recipients treated with rituximab for post-transplant lymphoproliferative disease. Am J Transplant. (2005) 5:566–72. doi: 10.1111/j.1600-6143.2004.00693.x
73. Porcu P, Eisenbeis CF, Pelletier RP, Davies EA, Baiocchi RA, Roychowdhury S, et al. Successful treatment of posttransplant lymphoproliferative disorder (PTLD) following renal allografting is associated with sustained CD8 +T-cell restoration. Blood. (2002) 100:2341–8. doi: 10.1182/blood-2002-01-0210
74. Smets F, Latinne D, Bazin H, Reding R, Otte JB, Buts JP, et al. Ratio between epstein-barr viral load and anti-epstein-barr virus specific T-cell response as a predictive marker of posttransplant lymphoproliferative disease. Transplantation. 73, 1603–10. doi: 10.1097/00007890-200205270-00014
75. Sester M, Leboeuf C, Schmidt T, Hirsch HH. The “ABC” of virus-specific t cell immunity in solid organ transplantation. Am J Transplant. (2016) 16:1697–706. doi: 10.1111/ajt.13684
76. Marshall NA, Howe JG, Formica R, Krause D, Wagner JE, Berliner N, et al. Rapid reconstitution of epstein–barr virus specific T lymphocytes following allogeneic stem cell transplantation. Blood. (2000) 96:2814–21. doi: 10.1182/blood.V96.8.2814
77. Meij P, van Esser JW, Niesters HG, van Baarle D, Miedema F, Blake N, et al. Impaired recovery of epstein–barr virus (EBV)-specific CD8+ T lymphocytes after partially T-depleted allogeneic stem cell transplantation may identify patients at very high risk for progressive EBV reactivation and lymphoproliferative disease. Blood. (2003) 101:4290–7. doi: 10.1182/blood-2002-10-3001
78. Clave E, Agbalika F, Bajzik V, Peffault de Latour R, Trillard M, Rabian C, et al. Epstein-barr virus (EBV) reactivation in allogeneic stem-cell transplantation: relationship between viral load, EBV-specific T-cell reconstitution and rituximab therapy. Transplantation. (2004) 77:76–84. doi: 10.1097/01.TP.0000093997.83754.2B
79. Annels NE, Kalpoe JS, Bredius RG, Claas EC, Kroes AC, Hislop AD, et al. Management of epstein–barr virus (EBV) reactivation after allogeneic stem cell transplantation by simultaneous analysis of EBV DNA load and EBV-specific T cell reconstitution. Clin Infect Dis. (2006) 42:1743–8. doi: 10.1086/503838
80. D'Aveni M, Aïssi-Rothé L, Venard V, Salmon A, Falenga A, Decot V, et al. The clinical value of concomitant epstein barr virus (EBV)-DNA load and specific immune reconstitution monitoring after allogeneic hematopoietic stem cell transplantation. Transpl Immunol. (2011) 24:224–32. doi: 10.1016/j.trim.2011.03.002
81. Worth A, Conyers R, Cohen J, Jagani M, Chiesa R, Rao K, et al. Pre-emptive rituximab based on viraemia and T cell reconstitution: a highly effective strategy for the prevention of Epstein-Barr virus-associated lymphoproliferative disease following stem cell transplantation. Br J Haematol. (2011) 155:377–85. doi: 10.1111/j.1365-2141.2011.08855.x
82. Chiereghin A, Piccirilli G, Belotti T, Prete A, Bertuzzi C, Gibertoni D, et al. Clinical utility of measuring epstein-barr virus-specific cell-mediated immunity after HSCT in addition to virological monitoring: results from a prospective study. Med Microbiol Immunol. (2019) 208:825–34. doi: 10.1007/s00430-019-00629-2
83. Heslop HE. How I treat EBV lymphoproliferation. Blood. (2009) 114:4002–8. doi: 10.1182/blood-2009-07-143545
84. Styczynski J, Einsele H, Gil L, Ljungman P. Outcome of treatment of epstein-barr virus-related post-transplant lymphoproliferative disorder in hematopoietic stem cell recipients: a comprehensive review of reported cases. Transpl Infect Dis. (2009) 11:383–92. doi: 10.1111/j.1399-3062.2009.00411.x
85. Savani BN, Pohlmann PR, Jagasia M, Chinratanalab W, Kassim A, Engelhardt B, et al. Does peritransplantation use of rituximab reduce the risk of EBV reactivation and PTLPD? Blood. (2009) 113:6263–4. doi: 10.1182/blood-2009-04-213892
86. Bacigalupo A, Locatelli F, Lanino E, Marsh J, Socié G, Maury S, et al. Fludarabine, cyclophosphamide with or without low dose TBI for alternative donor transplants in acquired aplastic anemia (SAA): a report from the EBMT-SAA Working Party. Biol Blood Marrow Transplant. (2009) 15:5. doi: 10.1016/j.bbmt.2008.12.014
87. Shekhovtsova Z, Shelikhova L, Balashov D, Zacharova V, Ilushina M, Voronin K, et al. Control of graft-versus-host disease with rabbit anti-thymocyte globulin, rituximab, and bortezomib in TCRαβ/CD19-depleted graft transplantation for leukemia in children: a single-center retrospective analysis of two GVHD-prophylaxis regimens. Pediatr Transplant. (2020) 24:e13594. doi: 10.1111/petr.13594
88. Locatelli F, Bernardo ME, Bertaina A, Rognoni C, Comoli P, Rovelli A, et al. Efficacy of two different doses of rabbit anti-T-lymphocyte globulin to prevent graft-versus-host disease in children with haematological malignancies transplanted from an unrelated donor: a multicentre, randomised, open-label, phase 3 trial. Lancet Oncol. (2017) 18:1126–36. doi: 10.1016/S1470-2045(17)30417-5
89. Pulsipher MA, Wall DA, Grimley M, Goyal RK, Boucher KM, Hankins P, et al. A phase I/II study of the safety and efficacy of the addition of sirolimus to tacrolimus/methotrexate graft versus host disease prophylaxis after allogeneic haematopoietic cell transplantation in paediatric acute lymphoblastic leukaemia (ALL). Br J Haematol. (2009) 147:691–9. doi: 10.1111/j.1365-2141.2009.07889.x
90. Peccatori J, Forcina A, Clerici D, Crocchiolo R, Vago L, Stanghellini MT, et al. Sirolimus-based graft-versus-host disease prophylaxis promotes the in vivo expansion of regulatory T cells and permits peripheral blood stem cell transplantation from haploidentical donors. Leukemia. (2015) 29:396–405. doi: 10.1038/leu.2014.180
91. Dominietto A, Tedone E, Soracco M, Bruno B, Raiola AM, Van Lint MT, et al. In vivo B-cell depletion with rituximab for alternative donor hemopoietic SCT. Bone Marrow Transplant. (2012) 47:101–6. doi: 10.1038/bmt.2011.28
92. McIver Z, Stephens N, Grim A, Barrett AJ. Rituximab administration within 6 months of T cell-depleted allogeneic SCT is associated with prolonged life-threatening cytopenias. Biol Blood Marrow Transplant. (2010) 16:1549–56. doi: 10.1016/j.bbmt.2010.05.004
93. Petropoulou AD, Porcher R, Peffault de Latour R, Xhaard A, Weisdorf D, Ribaud P, et al. Increased infection rate after preemptive rituximab treatment for epstein-barr virus reactivation after allogeneic hematopoietic stem-cell transplantation. Transplantation. (2012) 94:879–83. doi: 10.1097/TP.0b013e3182664042
94. Heslop HE, Slobod KS, Pule MA, Hale GA, Rousseau A, Smith CA, et al. Long-term outcome of EBV-specific T-cell infusions to prevent or treat EBV-related lymphoproliferative disease in transplant recipients. Blood. (2010) 115:925–35. doi: 10.1182/blood-2009-08-239186
95. Doubrovina E, Oflaz-Sozmen B, Prockop SE, Kernan NA, Abramson S, Teruya-Feldstein J, et al. Adoptive immunotherapy with unselected or EBV-specific T cells for biopsy-proven EBV+ lymphomas after allogeneic hematopoietic cell transplantation. Blood. (2012) 119:2644–56. doi: 10.1182/blood-2011-08-371971
96. Gustafsson A, Levitsky V, Zou JZ, Frisan T, Dalianis T, Ljungman P, et al. Epstein–barr virus (EBV) load in bone marrow transplant recipients at risk to develop posttransplant lymphoproliferative disease: prophylactic infusion of EBVspecific cytotoxic T cells. Blood. (2000) 95:807–14. doi: 10.1182/blood.V95.3.807.003k24_807_814
97. Imashuku S, Goto T, Matsumura T, Naya M, Yamori M, Hojo M, et al. Unsuccessful CTL transfusion in a case of post-BMT epstein-barr virus-associated lymphoproliferative disorder (EBV-LPD). Bone Marrow Transplant. (1997) 20:337–40. doi: 10.1038/sj.bmt.1700883
98. Moosmann A, Bigalke I, Tischer J, Schirrmann L, Kasten J, Tippmer S, et al. Effective and long-term control of EBV PTLD after transfer of peptide-selected T cells. Blood. (2010) 115:2960–70. doi: 10.1182/blood-2009-08-236356
99. Icheva V, Kayser S, Wolff D, Tuve S, Kyzirakos C, Bethge W, et al. Adoptive transfer of epstein-barr virus (EBV) nuclear antigen 1-specific t cells as treatment for EBV reactivation and lymphoproliferative disorders after allogeneic stem-cell transplantation. J Clin Oncol. (2013) 31:39–48. doi: 10.1200/JCO.2011.39.8495
100. Jiang X, Xu L, Zhang Y, Huang F, Liu D, Sun J, et al. Rituximab-based treatments followed by adoptive cellular immunotherapy for biopsy-proven EBV-associated post-transplant lymphoproliferative disease in recipients of allogeneic hematopoietic stem cell transplantation. Oncoimmunology. (2016) 5:e1139274. doi: 10.1080/2162402X.2016.1139274
101. Velvet AJJ, Bhutani S, Papachristos S, Dwivedi R, Picton M, Augustine T, et al. A single-center experience of post-transplant lymphomas involving the central nervous system with a review of current literature. Oncotarget. (2019) 10:437–48. doi: 10.18632/oncotarget.26522
102. Leen AM, Myers GD, Sili U, Huls MH, Weiss H, Leung KS, et al. Monoculture-derived T lymphocytes specific for multiple viruses expand and produce clinically relevant effects in immunocompromised individuals. Nat Med. (2006) 12:1160–6. doi: 10.1038/nm1475
103. Melenhorst JJ, Castillo P, Hanley PJ, Keller MD, Krance RA, Margolin J, et al. Graft versus leukemia response without graft-versus-host disease elicited by adoptively transferred multivirus-specific T-cells. Mol Ther. (2015) 23:179–83. doi: 10.1038/mt.2014.192
104. Leen AM, Christin A, Myers GD, Liu H, Cruz CR, Hanley PJ, et al. Cytotoxic T lymphocyte therapy with donor T cells prevents and treats adenovirus and epstein-barr virus infections after haploidentical and matched unrelated stem cell transplantation. Blood. (2009) 114:4283–92. doi: 10.1182/blood-2009-07-232454
105. Dong L, Gao ZY, Chang LJ, Liang Y, Tan XY, Liu JH, et al. Adoptive transfer of cytomegalovirus/epstein-barr virus specific immune effector cells for therapeutic and preventive/preemptive treatment of pediatric allogeneic cell transplant recipients. J Pediatr Hematol Oncol. (2010) 32:e31–7. doi: 10.1097/MPH.0b013e3181bf5e2d
106. Gerdemann U, Katari UL, Papadopoulou A, Keirnan JM, Craddock JA, Liu H, et al. Safety and clinical efficacy of rapidly-generated trivirus-directed T cells as treatment for adenovirus, EBV, and CMV infections after allogeneic hematopoietic stem cell transplant. Mol Ther. (2013) 21:2113–21. doi: 10.1038/mt.2013.151
107. Papadopoulou A, Gerdemann U, Katari UL, Tzannou I, Liu H, Martinez C, et al. Activity of broad-spectrum T cells as treatment for AdV, EBV, CMV, BKV, and HHV6 infections after HSCT. Sci Transl Med. (2014) 6:242ra83. doi: 10.1126/scitranslmed.3008825
108. Ma CK, Blyth E, Clancy L, Simms R, Burgess J, Brown R, et al. Addition of varicella zoster virus-specific T cells to cytomegalovirus, epstein-barr virus and adenovirus tri-specific T cells as adoptive immunotherapy in patients undergoing allogeneic hematopoietic stem cell transplantation. Cytotherapy. (2015) 17:1406–20. doi: 10.1016/j.jcyt.2015.07.005
109. Haque T, Wilkie GM, Jones MM, Higgins CD, Urquhart G, Wingate P, et al. Allogeneic cytotoxic T-cell therapy for EBV-positive posttransplantation lymphoproliferative disease: results of a phase 2 multicenter clinical trial. Blood. (2007) 110:1123–31. doi: 10.1182/blood-2006-12-063008
110. Barker JN, Doubrovina E, Sauter C, Jaroscak JJ, Perales MA, Doubrovin M, et al. Successful treatment of EBV-associated posttransplantation lymphoma after cord blood transplantation using third-party EBV-specific cytotoxic T lymphocytes. Blood. (2010) 116:5045–9. doi: 10.1182/blood-2010-04-281873
111. Uhlin M, Gertow J, Uzunel M, Okas M, Berglund S, Watz E, et al. Rapid salvage treatment with virus-specific T cells for therapy resistant disease. Clin Infect Dis. (2012) 55:1064–73. doi: 10.1093/cid/cis625
112. Prockop S, Doubrovina E, Suser S, Heller G, Barker J, Dahi P, et al. Off-the-shelf EBV-specific T cell immunotherapy for rituximab-refractory EBV-associated lymphoma following transplantation. J Clin Invest. (2020) 130:733–47. doi: 10.1172/JCI121127
113. Leen AM, Bollard CM, Mendizabal AM. Shpall EJ, Szabolcs P, Antin J, et al. Multicenter study of banked third-party virus specificT cells to treat severe viral infections after hematopoietic stem cell transplantation. Blood. (2013) 121:5113–23. doi: 10.1182/blood-2013-02-486324
114. Tzannou I, Papadopoulou A, Naik S, Leung K, Martinez CA, Ramos CA, et al. Off-the-shelf virus-specific T cells to Treat BK virus, human herpesvirus 6, cytomegalovirus, epstein-barr virus, and adenovirus infections after allogeneic hematopoietic stem-cell transplantation. J Clin Oncol. (2017) 35:3547–57. doi: 10.1200/JCO.2017.73.0655
115. Meijer E, Cornelissen JJ. Epstein-barr virus-associated lymphoproliferative disease after allogeneic haematopoietic stem cell transplantation: molecular monitoring and early treatment of high-risk patients. Curr Opin Hematol. (2008) 15:576–85. doi: 10.1097/moh.0b013e328311f438
116. Comoli P, Basso S, Labirio M, Baldanti F, Maccario R, Locatelli F. T cell therapy of epstein-barr virus and adenovirus infections after hemopoietic stem cell transplant. Blood Cells Mol Dis. (2008) 40:68–70. doi: 10.1016/j.bcmd.2007.06.020
117. Heslop HE, Ng CY, Li C, Smith CA, Loftin SK, Krance RA, et al. Long-term restoration of immunity against epstein-barr virus infection by adoptive transfer of gene-modified virus-specific T lymphocytes. Nat Med. (1996) 2:551–5. doi: 10.1038/nm0596-551
118. Bollard CM, Rooney CM, Heslop HE. T-cell therapy in the treatment of post-transplant lymphoproliferative disease. Nat Rev Clin Oncol. (2012) 9:510–9. doi: 10.1038/nrclinonc.2012.111
119. Bollard CM, Heslop HE. T cells for viral infections after allogeneic hematopoietic stem cell transplant. Blood. (2016) 127:3331–40. doi: 10.1182/blood-2016-01-628982
Keywords: epstein-barr virus, T cell immunity, virological monitoring, prophylaxis, preemptive treatment
Citation: Compagno F, Basso S, Panigari A, Bagnarino J, Stoppini L, Maiello A, Mina T, Zelini P, Perotti C, Baldanti F, Zecca M and Comoli P (2020) Management of PTLD After Hematopoietic Stem Cell Transplantation: Immunological Perspectives. Front. Immunol. 11:567020. doi: 10.3389/fimmu.2020.567020
Received: 29 May 2020; Accepted: 18 August 2020;
Published: 16 September 2020.
Edited by:
Kirk R. Schultz, The University of British Columbia, CanadaReviewed by:
Alice Bertaina, Stanford University, United StatesLuiza Guilherme, University of São Paulo, Brazil
Copyright © 2020 Compagno, Basso, Panigari, Bagnarino, Stoppini, Maiello, Mina, Zelini, Perotti, Baldanti, Zecca and Comoli. This is an open-access article distributed under the terms of the Creative Commons Attribution License (CC BY). The use, distribution or reproduction in other forums is permitted, provided the original author(s) and the copyright owner(s) are credited and that the original publication in this journal is cited, in accordance with accepted academic practice. No use, distribution or reproduction is permitted which does not comply with these terms.
*Correspondence: Patrizia Comoli, pcomoli@smatteo.pv.it
†These authors have contributed equally to this work