- 1The Central Laboratory of Birth Defects Prevention and Control, Ningbo Women and Children’s Hospital, Ningbo, Zhejiang, China
- 2Neonatal Screening Center, Ningbo Women and Children’s Hospital, Ningbo, Zhejiang, China
Background: Balanced translocation (BT) carriers can produce imbalanced gametes and experience recurrent spontaneous abortions (RSAs) and even give birth to a child with complex chromosomal disorders. Here, we report a cryptic BT, t(5; 6) (p15.31; p25.1), in the proband’s grandmother, which caused unbalanced chromosomal rearrangements and various anomalies in the two subsequent generations. We also provide a thorough overview of the application of optical genome mapping (OGM) to identify chromosomal structural variants (SVs).
Methods: Trio-based whole exome sequencing (Trio-WES) was conducted to explore the genetic basis of the phenotype of the proband and her mother. High-resolution karyotype analysis and OGM detection were performed on the proband’s grandparents to trace the origin of the unbalanced rearrangements between chromosomes 5 and 6. A PubMed search was conducted with the following keywords: “OGM” and “SVs.” Then, relevant studies were collected and systematically reviewed.
Results: The proband and her mother presented with various anomalies, whereas the grandmother was healthy but had a history of four abnormal pregnancies. Trio-WES revealed a heterozygous duplication on the terminal region of chromosome 5p and a heterozygous deletion on the proximal end of chromosome 6p in the proband and her mother. High-resolution karyotype analysis revealed no aberrant karyotypes in either grandparent, whereas OGM detection revealed a cryptic BT, t(5; 6)(p15.31; p25.1), in the proband’s grandmother. An overwhelming majority of research publications have verified the clinical utility of OGM in detecting SVs.
Conclusion: The results of this study revealed that the unbalanced chromosomal rearrangements and many anomalies observed in multiple members of the family were attributable to the cryptic BT carried by the proband’s grandmother. This study supports that OGM has a unique advantage for detecting cryptic BTs, and can be used as a first-tier genetic test for the etiological diagnosis of infertility, RSAs, and other complex genetic disorders.
1 Introduction
Balanced translocations (BTs) are formed by the random de novo breakage and rejoining of two or more chromosomes and are classified as chromosomal structural rearrangements (Wang et al., 2020). It occurs in approximately 0.2% of the general population and 2.2% of patients with a history of recurrent spontaneous abortions (RSAs) (Jacobs et al., 1992; Ogilvie et al., 2001; Wilch and Morton, 2018). BT carriers can produce unbalanced gametes, resulting in recurrent implantation failure, early abortion, or fetal death (Chantot-Bastaraud et al., 2008; Hofherr et al., 2011; Wilch and Morton, 2018). Occasionally, a living fetus with an unbalanced chromosomal rearrangement may exhibit complex chromosome diseases. Keify et al. reported two novel familial BTs, t(8; 11)(p23; q21), and t(6; 16)(q26; p12), which are implicated in RSAs (Keify et al., 2012). Soltani et al. conducted cytogenetic studies on 608 couples with RSAs in northeastern Iran and found 25 BT cases (approximately 2.05%) (Soltani et al., 2021). Wan et al. have recently reported two rare Chinese Han cases of RSAs associated with chromosomal BTs (Wan et al., 2021).
Traditional karyotyping (KT) is the standard-of-care (SOC) genetic testing for large chromosome rearrangements; however, its relatively low resolution of 5–10 Mb G banding limits its use in identifying copy number variations (CNVs) and cryptic structural variants (SVs) (Trask, 2002; Wapner et al., 2012). Fluorescence in situ hybridization (FISH) is another efficient SOC approach that can precisely detect target SVs depending on the corresponding probes. However, it is not applicable for accurate analysis at the whole-genome level (Carpenter, 2001; Bates, 2011). Recently, next-generation sequencing (NGS) technologies, including whole-genome sequencing, have been applied to map chromosome breakpoints; however, this application sometimes may present technical difficulties in determining the accurate breakpoints of BTs (Liang et al., 2017; Nilsson et al., 2017; Hu et al., 2019).
More recently, optical genome mapping (OGM) technology, which is based on the analysis of ultra-high molecular weight (UHMW) DNA, allows the precise detection of chromosomal SV abnormalities. OGM can be used to directly label, straighten, linearize, and unfold each DNA molecule through very fine capillary electrophoresis, in which the segment of a complicated SV is easily spanned and mapped. Different from SOC testing, OGM exhibits a unique ability to detect nearly all classes of clinically significant SVs per sample, including aneuploidy, CNVs (deletions/duplications), balanced/unbalanced translocations, inversion, insertion, ring chromosomes, and the absence of heterozygosity (Sun et al., 2018; Mantere et al., 2021). A systematic literature review has summarized the clinical applications and performance evaluation of OGM in constitutional disorders and hematologic neoplasms. Furthermore, multiple studies have validated a nearly 100% concordance between OGM and SOC methods in different clinical settings.
In the present study, we uncovered a cryptic BT, t(5; 6)(p15.31; p25.1), in the proband’s grandmother through OGM analysis. This translocation was believed to underlie the unbalanced rearrangements between chromosomes 5 and 6 in the proband and her mother. Based on data from literature reviews, OGM can be used as a first-tier genetic test for detecting cryptic BTs.
1.1 Case description
The proband was an 8-year-old girl with multiple anomalies, including glaucoma, corneal opacities, deafness, tooth deformity, abnormal tongue, hypoglycemia, foot varus, and aberrant hip joint. She also presented with intellectual disability due to loss of language function and lack of response to normal communication, as well as a lag in growth, with initial standing at 3 years old and independent walking at 7 years old. The proband’s mother also showed several similar deformities but generally had milder phenotypes, including occasional nystagmus, pectus carinatum, low verbal skills, and intelligence. Notably, the proband’s grandmother was healthy but had experienced four abnormal pregnancies. Her first child died a month after birth, and the following three pregnancies were spontaneously aborted. The proband’s father, grandfather, and other grandparents showed no abnormalities. A pedigree chart of the family is shown in Figure 1A. The proband underwent eye and orthopedic surgeries prior to genetic testing. In addition, self-care rehabilitation was routinely conducted, but only slight symptomatic improvement was observed. The proband’s mother did not receive any therapeutic intervention. Written informed consent was obtained from all participants, and this study was approved by the ethics board of Ningbo Women and Children’s Hospital (No. EC 2020-048). This study conformed to the provisions of the Declaration of Helsinki as revised in 2013.
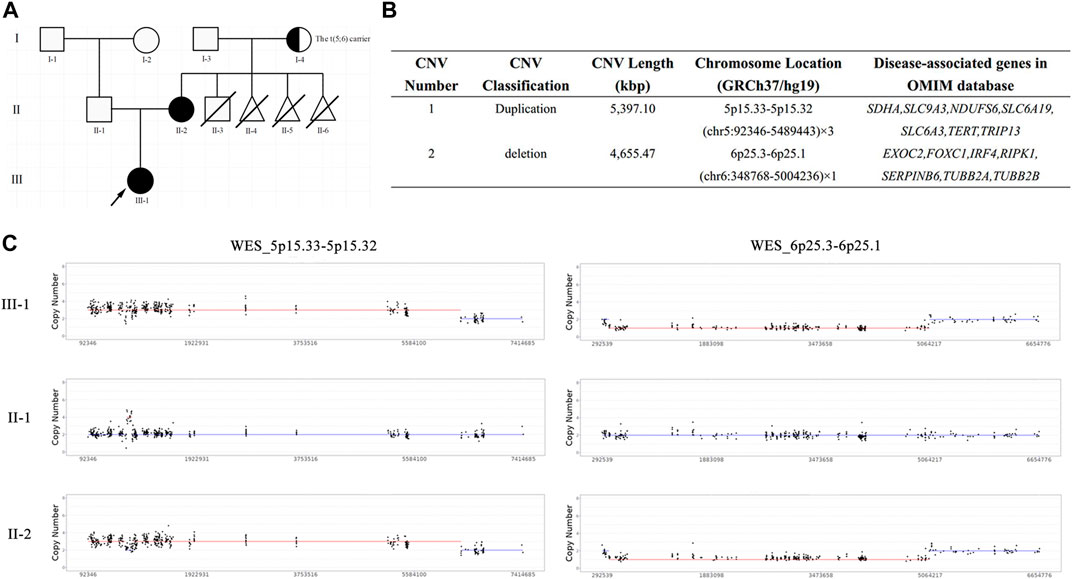
FIGURE 1. The pedigree chart and results of trio-WES. (A) The pedigree chart of this family: black represents the patients, arrow represents the proband, half-blackened circle represents the carrier of BT, oblique line represents the died; (B) The quantified CNVs of trio-WES for the proband and her mother; (C) The copy number of WES_5p15.33-5p15.32 and WES_6p25.3-6p25.1 in this family: III-1 represents the proband, II-1 represents the proband’s father, II-2 represents the proband’s mother.
2 Methods
2.1 Trio-WES
Genomic DNA was isolated from the peripheral blood of the proband and her parents using a QIAamp DNA Blood Mini Kit (Qiagen, Valencia, CA, United States) in accordance with the manufacturer’s protocol. Trio-WES was performed using the HiSeq 2,500 sequencing platform (Illumina, San Diego, CA, United States). Raw fastq data (including CNVs) were filtered using fastp (v0.23.1) and annotated based on the human reference genome (hg19). BAM files were generated by re-ordering using Burrows–Wheeler Aligner (0.7.17-r1194-dirty). The Genome Analysis Toolkit (GATK, v4.20) was used to obtain single nucleotide polymorphisms (SNP) and insertions and deletions, and ANNOVAR and VEP were carried out for variation annotations. Pathogenicity classification and interpretation of sequence variants were performed in accordance with the guidelines of the American College of Medical Genetics and Genomics (ACMG). Sanger sequencing was performed to confirm the identified variants. CNVs were obtained using the eXome Hidden Markov model, and copy number was estimated using Lattice-Aligned Mixture Models (clamms). The pathogenicity classification of the identified CNVs was consistent with the consensus recommendations of ACMG and ClinGen in 2021 (Riggs et al., 2021).
2.2 High-resolution karyotype analysis
The Chromed CK cell synchronization kit (Shanghai Lechen Biotechnology Co. Ltd., Item No: CM-SK-80), which included high-resolution reagent solutions A (Fudr) and B (Thymidine), was used in this experiment. High-resolution karyotyping was performed in accordance with the manufacturer’s instructions. Briefly, 2 mL of anticoagulated peripheral blood (heparin) was collected from the proband’s grandparents. T lymphocytes in the peripheral blood were first stimulated into childish lymphocytes by plant lectins (PHA). After 72 h of cell culture, Solution A was added. After 16–18 h, the cells were treated with solution B. This process was maintained for 3–5 h, after which the cells were harvested using colchicine. After the experimental procedures of preparation, baking, drying, and sealing, the automatic scanning system GSL120 (Leica Biosystems Richmond, Inc., 5,205 Route 12 P. 0. Box 528 Richmond, Illinois 60071, United States) was used to automatically capture and record karyotypes. The karyotyping results were consistent with the International Nomenclature System for Human Cytogenetics (ISCN 2020).
2.3 OGM
OGM experiments were performed in accordance with the manufacturer’s instructions. Briefly, 2 mL of anticoagulant peripheral blood (EDTA) was collected from the proband’s grandparents. UHMW gDNA was extracted using the Blood and Cell DNA Isolation Kit v2 (Bionano Genomics, San Diego, CA, United States). Then, UHMW gDNA molecules were labeled with the DLS (Direct Label and Stain) DNA Labeling Kit (Bionano Genomics, San Diego, CA, United States of America). The DNA was quantified using a Qubit dsDNA Assay BR Kit with a Qubit 3.0 Fluorometer (Thermo Fisher Scientific). The labeled UHMW gDNA was loaded onto a Saphyr chip for linearization and imaged using a Saphyr instrument (Bionano Genomics, San Diego, CA, United States). Bionano Access v1.7 software was used for bioinformatics analysis, which included de novo assembly and comparison with a reference genome map (hg19).
2.4 Literature review
A PubMed search was performed using the following keywords: “OGM” and “SVs”. Studies concerning the clinical application of OGM for detecting chromosomal SVs in human samples were downloaded and systematically reviewed. Detailed information, including the aim of clinical research, results and clinical efficacy evaluation, publication date, and PMID, is summarized in Table 1.
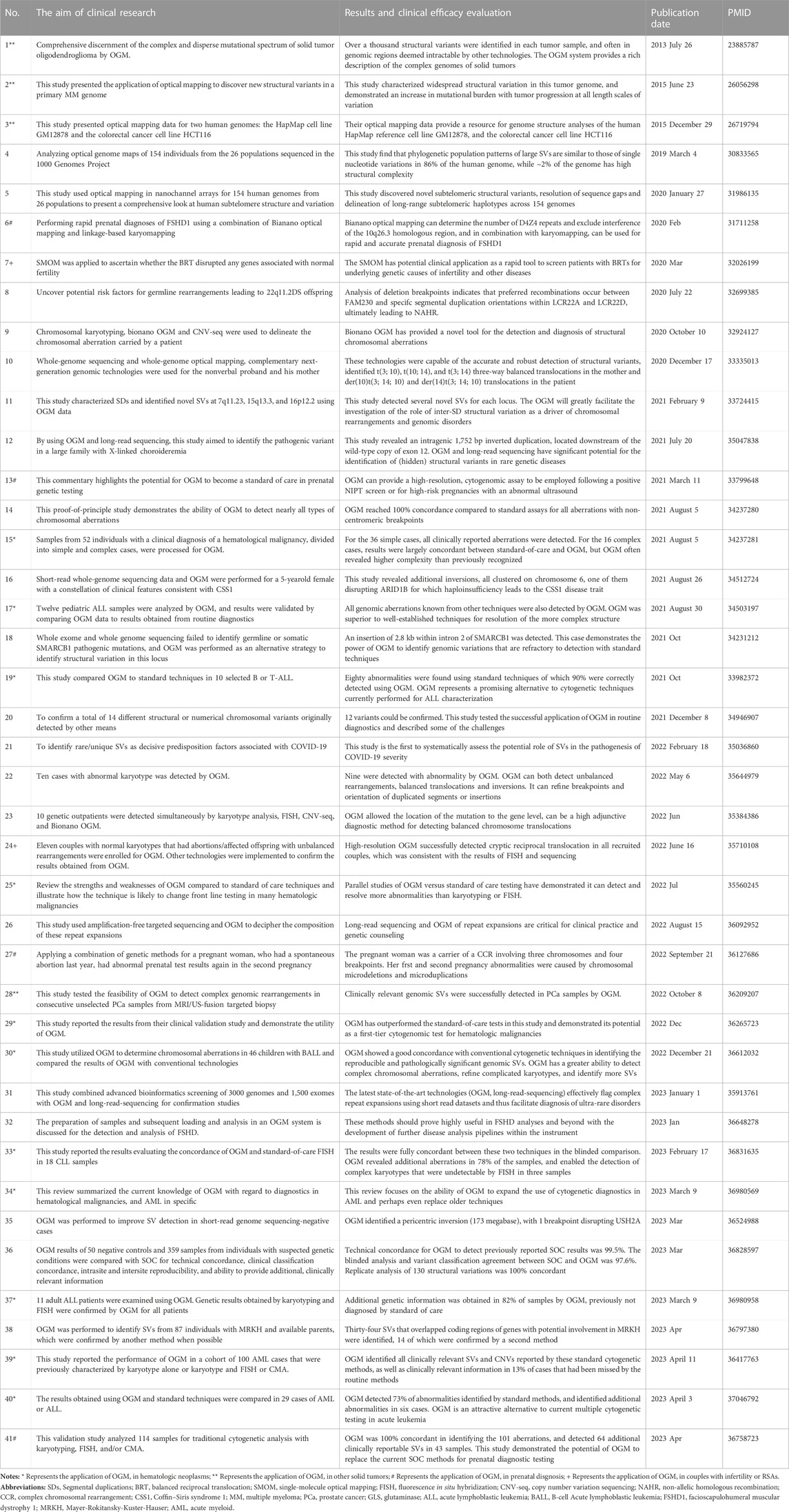
TABLE 1. The systematical overview of OGM application and clinical utility for detecting chromosomal SVs.
3 Results
3.1 Unbalanced rearrangements between chromosomes 5 and 6 were discovered in proband and her mother
To unveil the genetic basis of the phenotype and ascertain the clinical diagnosis, we first performed trio-WES on the proband and her parents. The proband carried a heterozygous duplication of 5,397.10 Kbp in the 5p15.33–5p15.32 region of chromosome 5 and a heterozygous deletion of 4,655.47 Kbp in the 6p25.3–6p25.1 region of chromosome 6 (Figures 1B, C), both of which were inherited from her mother. These unbalanced rearrangements were not detected in the father of the proband (Figure 1C). A duplication in chromosome 5, which is a non-polymorphic variant, was not reported in the DGV database. The DECIPHER database described a similar case with clinical phenotypes including cerebral hemorrhage, hearing impairment, neurodevelopmental delay, and strabismus (Patient ID: 339222). In accordance with the ACMG guidelines, the proband’s duplication was classified as having unknown clinical significance.
The deletion on chromosome 6 contained FOXC1 with a haploinsufficiency score of 3. Heterozygous mutation in FOXC1 could lead to Anterior segment dysgenesis 3 (MIM number: 601631) and Axenfeld–Rieger syndrome type 3 (RIEG3, MIM number: 602482). ASGD and ASMD are heterogeneous developmental disorders that affect the anterior segment of the eye, including the cornea, iris, lens, trabecular meshwork, and Schlemm’s canal. The clinical features of ASGD include iris hypoplasia, enlarged or reduced corneal diameter, corneal vascularization and opacity, posterior embryotoxin, corectopia, polycoria, abnormal iridocorneal angle, ectopia lentis, and anterior synechiae between the iris and posterior corneal surface (Cheong et al., 2016). The clinical phenotypes of RIEG3 are highly heterogeneous and include zygomatic planar process, ocular hypertelorism, sensorineural deafness, glaucoma, eyeball protrusion, posterior embryonic ring, denture, microdentin, vermium hypoplasia, atrial septal defect, patent ductus arteriosus, iris hypoplasia, pupil dislocation, concave nasal ridge, and midface retroretraction (Gould et al., 1997; Cunningham et al., 1998). The deletion in the 6p25.3–6p25.1 region, which is a non-polymorphic variant, was not included in the DGV database. Conversely, the DECIPHER database enrolled a crowd of cases presenting with multiple anomalies and carrying similar pathogenic/likely pathogenic CNVs (Patient ID:308608, 332098, 401710, etc.). Combined with the proband’s clinical symptoms, the deletion in the 6p25.3–6p25.1 region was classified as pathogenic.
3.2 Cryptic BT t(5; 6) was revealed in the proband’s grandmother
High-resolution karyotype analysis was performed to trace the origin of the unbalanced rearrangements between chromosomes 5 and 6 in the proband’s grandparents. However, the grandmother and grandfather both exhibited normal karyotypes (Figures 2A, B). Given that OGM has a 10,000-fold higher resolution than karyotyping, the grandparents’ peripheral blood was further subjected to OGM. As expected, no abnormalities were found in the grandfather (Figure 2D), whereas a cryptic BT between chromosomes 5 and 6 was uncovered in the grandmother (Figure 2C), which may explain the 5.4 Mb 5p terminal duplication and 4.7 Mb 6p terminal deletion in the proband and her mother. According to the breakpoints on the chromosomes, chromosome 5 was separated into Chr5-A (5 pter-5p15.31, 6.301 M) and Chr5-B (5p15.31-5 qter, 174.599 M), and chromosome 6 was separated into Chr6-A (6 pter-6p25.1, 5.025 M) and Chr6-B (6p25.1-6 qter, 166.075 M) (Figure 3A). The derived chromosome 5 comprised Chr6-A and Chr5-B, and the derived chromosome 6 comprised Chr5-A and Chr6-B (Figure 3B).
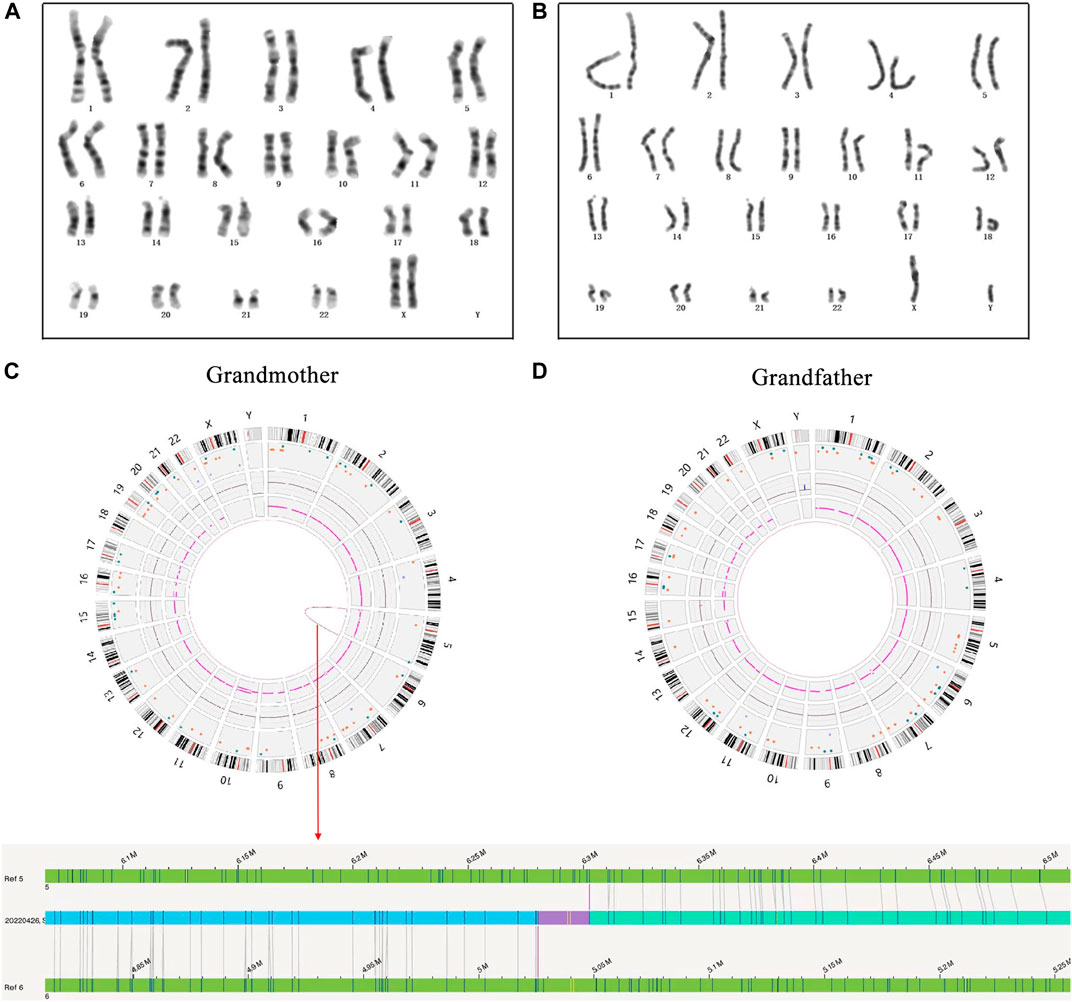
FIGURE 2. The results of high-resolution karyotype analysis and OGM analysis for the proband’s grandparents. (A) The description of grandmother’s karyotype was shown as 46,XX; (B) The description of grandfather’s karyotype was shown as 46,XY; (C) A cryptic BT between chromosomes 5 and 6 was uncovered in the grandmother by OGM: red arrow represents OGM analysis result; (D) No abnormity was found in the grandfather by OGM.
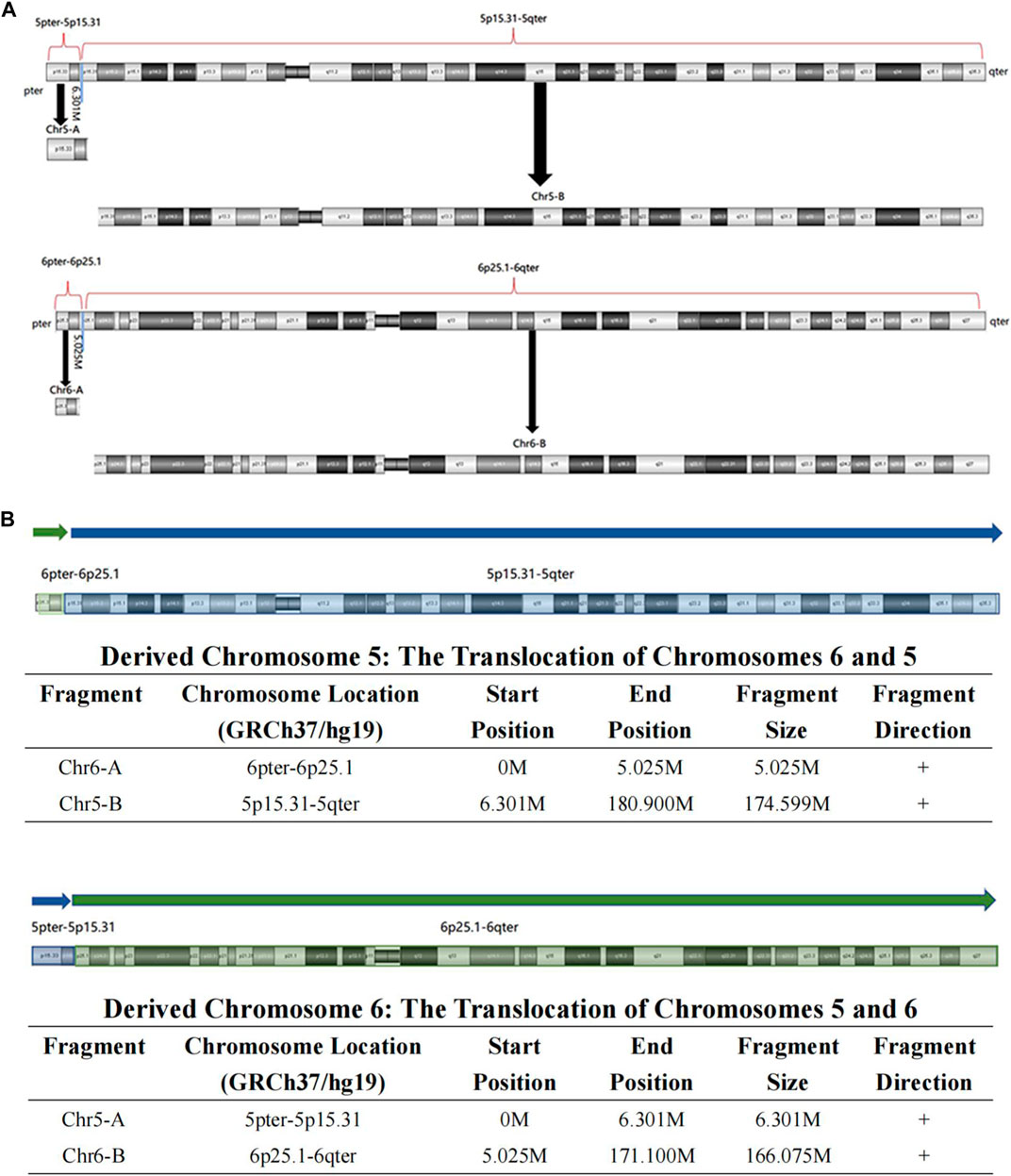
FIGURE 3. The visual BT results of OGM in the grandmother. (A) According to the breakpoint, the whole chromosome 5 was separated into Chr5-A and Chr5-B, and the whole chromosome 6 was separated into Chr6-A and Chr6-B; (B) The derived chromosome 5 comprises Chr6-A (6pter-6p25.1, 5.025 M) and Chr5-B (5p15.31-5qter, 174.599 M), and the derived chromosome 6 comprises Chr5-A (5pter-5p15.31, 6.301 M) and Chr6-B (6p25.1-6qter, 166.075 M).
3.3 Literature review of OGM application and clinical utility for detecting chromosomal SVs
Most studies have validated a 100% clinical concordance of OGM with traditional cytogenetic analysis and identified additional cryptic variations that remain beyond the purview of current technologies (Table 1). OGM has been extensively used to characterize SVs for the diagnosis of hematological neoplasms. The study by Luhmann et al. (2021) indicated that 12 pediatric acute lymphoblastic leukemia samples were analyzed using OGM, and all genomic aberrations identified by other techniques were also detected using OGM. Their results showed that OGM was superior to well-established techniques for the resolution of complex structures and had a higher sensitivity for the detection of copy number alterations (Luhmann et al., 2021). Sahajpal & Mondal et al. also demonstrated that OGM outperforms SOC tests and may be a first-tier cytogenomic test for hematologic malignancies (Sahajpal et al., 2022b). Recently, Levy’s group reported in a real-world setting the performance of OGM in a cohort of 100 acute myeloid leukemia (AML) cases that were previously characterized by KT alone or KT and FISH or CMA. Their multicenter study indicates that OGM effectively recovers clinically relevant SVs and CNVs found by SOC methods and reveals an additional 13% of cases that had been missed by the routine methods (Levy et al., 2023). Moreover, the application and clinical utility of OGM have been reported in other solid neoplasms, including multiple myeloma (Gupta et al., 2015), solid tumor oligodendroglioma (Ray et al., 2013), and prostate cancer (Shim et al., 2022).
Aside from its superiority in the precise diagnosis of oncology, OGM also has greater efficiency in detecting postnatal/prenatal constitutional genetic disorders. A previous multi-site assessment of OGM in constitutional postnatal cases indicated that the technical concordance for OGM to detect previously reported SOC results was 99.5%, and the replicate analysis of 130 SVs was 100% concordant (Iqbal et al., 2023). Additionally, high-resolution OGM has been successfully used to detect cryptic reciprocal translocation in 11 couples with normal karyotypes and abortions/affected offspring with unbalanced rearrangements (Zhang et al., 2022). Another study used a combination of OGM and linkage-based karyomapping for the rapid prenatal diagnoses of facioscapulohumeral muscular dystrophy 1 (Zheng et al., 2020). A commentary by Sahajpal et al. in 2021 highlighted the potential of OGM as a standard of care in prenatal genetic testing (Sahajpal et al., 2021). Furthermore, Sahajpal & Jill et al. performed OGM in 52 patients with severe COVID-19 to identify rare/unique SVs as decisive predisposition factors associated with COVID-19. This study is the first to systematically assess the potential role of SVs in the pathogenesis of COVID-19 severity (Sahajpal et al., 2022a). Systematic information on OGM application and clinical utility for detecting chromosomal SVs was collected and summarized in Table 1.
4 Discussion
Trio-WES has been widely used as a first-tier tool for the molecular diagnosis of rare genetic diseases. Along with improvements in CNV algorithms using WES data, WES, especially trio-WES, is already widely accepted as a cost-effective diagnostic assay for rare genetic disorders resulting from pathogenic CNV (Zhai et al., 2021). In this case, trio-WES indicated an unbalanced rearrangement between chromosomes 5 and 6 in the proband and her mother. Given the grandmother’s four abnormal pregnancies, we speculated a potential BT in one of the proband’s grandparents.
Conventional KT has a limited resolution in the recognition of small or cryptic SVs. Our case report again demonstrated its limited usage in this regard because the proband’s grandparents had normal results on high-resolution KT analysis. However, the genetic basis of more than half of these patients remains unclear even though multiple tests, including KT, CMA, and NGS, are used in clinical settings. OGM is widely accepted as a novel tool for detecting SVs. Several factors, including cost, high sample requirement, and the absence of a standard data analysis pipeline, limit its clinical application at this stage. Nevertheless, an increasing number of clinical studies have shown that OGM has superior performance in the detection of SVs compared to SOC assays (e.g., KT, FISH, and CMA) (Table 1). In the present study, OGM analysis showed that the grandmother carried a cryptic BT between chromosomes 5 and 6, which revealed the origin of the unbalanced rearrangements in the proband and her mother. The findings of this study particularly emphasized OGM’s unique advantage for detecting cryptic BTs.
We also reviewed the applications and clinical utility of OGM for detecting chromosomal SVs. OGM has been thoroughly evaluated for potential clinical applications in postnatal and prenatal scenarios. A vast majority of studies have demonstrated the utility of OGM in detecting nearly all types of chromosomal aberrations and highlighted its clinical superiority to SOC assays in detecting complex cryptic SVs. In assisted reproduction, OGM has been used to clarify the etiology of infertility, recurrent spontaneous abortion, and abnormal pregnancy histories (Wang et al., 2020; Yang and Hao, 2022; Zhang et al., 2022). This technology not only helps optimize reproductive decisions but also avoids the birth of children with severe genetic diseases. Certainly, if this cryptic BT t(5; 6) of the grandmother in our case report had been uncovered earlier, her repeated aberrant pregnancy history and multiple anomalies of the two subsequent generations could have been effectively avoided through preimplantation genetic testing.
In addition, the methodology and algorithm of OGM have been researched and optimized to facilitate its utility in detecting complex SVs. In 2021, Mukherjee et al. extended the definition of bilabels in the paired de Bruijn graph to the context of optical mapping data and presented the first de Bruijn graph-based method for Rmap assembly. This method has been successfully applied to three genomes: E. coli, human, and climbing perch fish (Anabas Testudineus) (Mukherjee et al., 2021). Other tools, such as OMTools (Leung et al., 2017) and OMSV (Li et al., 2017), have also been developed to assist in optical mapping analysis. With technological developments and growing applications, OGM is expected to enable the assembly of more complete genomes and discovery of novel variations in the near future.
Overall, our case report and literature review support the indispensable role of OGM in identifying genomic cryptic BTs, that SOC tests could not find out. The limitation of this study is the absence of long-term follow-up for this special family and up-to-date literature summary, which may warrant further discussion.
5 Conclusion
In this case report, the combined use of Trio-WES and OGM revealed an unbalanced rearrangement between chromosomes 5 and 6 in the proband and her mother, as well as a cryptic BT t(5; 6)(p15.31; p25.1) in the proband’s grandmother, which clarified the genetic etiology of the grandmother’s abnormal fertility history and multiple anomalies in the two subsequent generations. Our systematic literature review demonstrates the utility of OGM in detecting and diagnosing chromosomal SVs. Collectively, our study summarized that OGM has an inherent advantage for identifying cryptic BTs, especially when combination with trio-WES or other SOC tests, could provide precise etiological diagnosis of couples with infertility, RSAs, or other patients with solid tumors and other complicated diseases.
Data availability statement
The datasets for this article are not publicly available due to concerns regarding participant/patient anonymity. Requests to access the datasets should be directed to the corresponding authors.
Ethics statement
The studies involving humans were approved by the Ningbo Women and Children’s Hospital (No. EC 2020-048). The studies were conducted in accordance with the local legislation and institutional requirements. Written informed consent for participation in this study was provided by the participants’ legal guardians/next of kin. Written informed consent was obtained from the individual(s), and minor(s)’ legal guardian/next of kin, for the publication of any potentially identifiable images or data included in this article.
Author contributions
Conceptualization: HL and QL; Methodology: YuZ, YiZ, and QY; Formal analysis and investigation: MX and JX; Writing-original draft preparation: MX and JX; Writing-review and editing: HL and QL; Funding acquisition: MX and HL; Resources: YuZ and YiZ; Supervision: HL and QL. All authors contributed to the article and approved the submitted version.
Funding
This study was funded by the Zhejiang Provincial Medical and Healthcare Program (Grant No. 2022KY1157), Ningbo public welfare project (Grant Nos 2022S035 and 202002N3150), Innovation Project of Distinguished Medical Team in Ningbo (Grant No. 2022020405).
Acknowledgments
We are grateful to the patient and her family for participation in the study.
Conflict of interest
The authors declare that the research was conducted in the absence of any commercial or financial relationships that could be construed as a potential conflict of interest.
Publisher’s note
All claims expressed in this article are solely those of the authors and do not necessarily represent those of their affiliated organizations, or those of the publisher, the editors and the reviewers. Any product that may be evaluated in this article, or claim that may be made by its manufacturer, is not guaranteed or endorsed by the publisher.
References
Bates, S. E. (2011). Classical cytogenetics: karyotyping techniques. Methods Mol. Biol. 767, 177–190. doi:10.1007/978-1-61779-201-4_13
Carpenter, N. J. (2001). Molecular cytogenetics. Semin. Pediatr. Neurol. 8 (3), 135–146. doi:10.1053/spen.2001.26447
Chantot-Bastaraud, S., Ravel, C., and Siffroi, J. P. (2008). Underlying karyotype abnormalities in IVF/ICSI patients. Reprod. Biomed. Online. 16 (4), 514–522. doi:10.1016/s1472-6483(10)60458-0
Cheong, S. S., Hentschel, L., Davidson, A. E., Gerrelli, D., Davie, R., Rizzo, R., et al. (2016). Mutations in CPAMD8 cause a unique form of autosomal-recessive anterior segment dysgenesis. Am. J. Hum. Genet. 99 (6), 1338–1352. doi:10.1016/j.ajhg.2016.09.022
Cunningham, E. J., Eliott, D., Miller, N. R., Maumenee, I. H., and Green, W. R. (1998). Familial axenfeld-rieger anomaly, atrial septal defect, and sensorineural hearing loss: A possible new genetic syndrome. Arch. Ophthalmol. 116 (1), 78–82. doi:10.1001/archopht.116.1.78
Gould, D. B., Mears, A. J., Pearce, W. G., and Walter, M. A. (1997). Autosomal dominant Axenfeld-Rieger anomaly maps to 6p25. Am. J. Hum. Genet. 61 (3), 765–768. doi:10.1016/s0002-9297(07)64340-7
Gupta, A., Place, M., Goldstein, S., Sarkar, D., Zhou, S., Potamousis, K., et al. (2015). Single-molecule analysis reveals widespread structural variation in multiple myeloma. Proc. Natl. Acad. Sci. U. S. A. 112 (25), 7689–7694. doi:10.1073/pnas.1418577112
Hofherr, S. E., Wiktor, A. E., Kipp, B. R., Dawson, D. B., and Van Dyke, D. L. (2011). Clinical diagnostic testing for the cytogenetic and molecular causes of male infertility: the mayo clinic experience. J. Assist. Reprod. Genet. 28 (11), 1091–1098. doi:10.1007/s10815-011-9633-6
Hu, L., Liang, F., Cheng, D., Zhang, Z., Yu, G., Zha, J., et al. (2019). Location of balanced chromosome-translocation breakpoints by long-read sequencing on the oxford nanopore platform. Front. Genet. 10, 1313. doi:10.3389/fgene.2019.01313
Iqbal, M. A., Broeckel, U., Levy, B., Skinner, S., Sahajpal, N. S., Rodriguez, V., et al. (2023). Multisite assessment of optical genome mapping for analysis of structural variants in constitutional postnatal cases. J. Mol. Diagn. 25 (3), 175–188. doi:10.1016/j.jmoldx.2022.12.005
Jacobs, P. A., Browne, C., Gregson, N., Joyce, C., and White, H. (1992). Estimates of the frequency of chromosome abnormalities detectable in unselected newborns using moderate levels of banding. J. Med. Genet. 29 (2), 103–108. doi:10.1136/jmg.29.2.103
Keify, F., Zhiyan, N., Mirzaei, F., Tootian, S., Ghazaey, S., and Abbaszadegan, M. R. (2012). Two novel familial balanced translocations t(8;11)(p23;q21) and t(6;16)(q26;p12) implicated in recurrent spontaneous abortion. Arch. Iran. Med. 15 (4), 249–252.
Leung, A. K., Jin, N., Yip, K. Y., and Chan, T. F. (2017). Omtools: A software package for visualizing and processing optical mapping data. J. Artic. Bioinforma. 33 (18), 2933–2935. doi:10.1093/bioinformatics/btx317
Levy, B., Baughn, L. B., Akkari, Y., Chartrand, S., LaBarge, B., Claxton, D., et al. (2023). Optical genome mapping in acute myeloid leukemia: A multicenter evaluation. Blood Adv. 7 (7), 1297–1307. doi:10.1182/bloodadvances.2022007583
Li, L., Leung, A. K., Kwok, T. P., Lai, Y., Pang, I. K., Chung, G. T., et al. (2017). OMSV enables accurate and comprehensive identification of large structural variations from nanochannel-based single-molecule optical maps. Genome Biol. 18 (1), 230. doi:10.1186/s13059-017-1356-2
Liang, D., Wang, Y., Ji, X., Hu, H., Zhang, J., Meng, L., et al. (2017). Clinical application of whole-genome low-coverage next-generation sequencing to detect and characterize balanced chromosomal translocations. Clin. Genet. 91 (4), 605–610. doi:10.1111/cge.12844
Luhmann, J. L., Stelter, M., Wolter, M., Kater, J., Lentes, J., Bergmann, A. K., et al. (2021). The clinical utility of optical genome mapping for the assessment of genomic aberrations in acute lymphoblastic leukemia. J. Artic. Cancers 13 (17), 4388. doi:10.3390/cancers13174388
Mantere, T., Neveling, K., Pebrel-Richard, C., Benoist, M., van der Zande, G., Kater-Baats, E., et al. (2021). Optical genome mapping enables constitutional chromosomal aberration detection. Am. J. Hum. Genet. 108 (8), 1409–1422. doi:10.1016/j.ajhg.2021.05.012
Mukherjee, K., Rossi, M., Salmela, L., and Boucher, C. (2021). Fast and efficient Rmap assembly using the Bi-labelled de Bruijn graph. Algorithms. Mol. Biol. 16 (1), 6. doi:10.1186/s13015-021-00182-9
Nilsson, D., Pettersson, M., Gustavsson, P., Forster, A., Hofmeister, W., Wincent, J., et al. (2017). Whole-genome sequencing of cytogenetically balanced chromosome translocations identifies potentially pathological gene disruptions and highlights the importance of microhomology in the mechanism of formation. Hum. Mutat. 38 (2), 180–192. doi:10.1002/humu.23146
Ogilvie, C. M., Braude, P., and Scriven, P. N. (2001). Successful pregnancy outcomes after preimplantation genetic diagnosis (PGD) for carriers of chromosome translocations. Hum. Fertil. 4 (3), 168–171. doi:10.1080/1464727012000199252
Ray, M., Goldstein, S., Zhou, S., Potamousis, K., Sarkar, D., Newton, M. A., et al. (2013). Discovery of structural alterations in solid tumor oligodendroglioma by single molecule analysis. BMC Genomics 14, 505. doi:10.1186/1471-2164-14-505
Riggs, E. R., Andersen, E. F., Cherry, A. M., Kantarci, S., Kearney, H., Patel, A., et al. (2021). Correction: technical standards for the interpretation and reporting of constitutional copy-number variants: A joint consensus recommendation of the American College of medical genetics and genomics (ACMG) and the clinical genome resource (ClinGen). Genet. Med. 23 (11), 2230. doi:10.1038/s41436-021-01150-9
Sahajpal, N. S., Barseghyan, H., Kolhe, R., Hastie, A., and Chaubey, A. (2021). Optical genome mapping as a next-generation cytogenomic tool for detection of structural and copy number variations for prenatal genomic analyses. Genes 12 (3), 398. doi:10.3390/genes12030398
Sahajpal, N. S., Jill, L. C., Hastie, A., Mondal, A. K., Dehkordi, S. R., van der Made, C. I., et al. (2022a). Optical genome mapping identifies rare structural variations as predisposition factors associated with severe COVID-19. iScience 25 (2), 103760. doi:10.1016/j.isci.2022.103760
Sahajpal, N. S., Mondal, A. K., Tvrdik, T., Hauenstein, J., Shi, H., Deeb, K. K., et al. (2022b). Clinical validation and diagnostic utility of optical genome mapping for enhanced cytogenomic analysis of hematological neoplasms. J. Mol. Diagn. 24 (12), 1279–1291. doi:10.1016/j.jmoldx.2022.09.009
Shim, Y., Lee, J., Seo, J., Park, C. K., Shin, S., Han, H., et al. (2022). Optical genome mapping identifies clinically relevant genomic rearrangements in prostate cancer biopsy sample. Cancer Cell Int. 22 (1), 306. doi:10.1186/s12935-022-02728-2
Soltani, N., Mirzaei, F., and Ayatollahi, H. (2021). Cytogenetic studies of 608 couples with recurrent spontaneous abortions in northeastern Iran. Iran. J. Pathol. 16 (4), 418–425. doi:10.30699/IJP.2021.521514.2554
Sun, Y., Man, J., Wan, Y., Pan, G., Du, L., Li, L., et al. (2018). Targeted next-generation sequencing as a comprehensive test for mendelian diseases: A cohort diagnostic study. Sci. Rep. 8 (1), 11646. doi:10.1038/s41598-018-30151-z
Trask, B. J. (2002). Human cytogenetics: 46 chromosomes, 46 years and counting. Nat. Rev. Genet. 3 (10), 769–778. doi:10.1038/nrg905
Wan, X., Li, L., Liu, Z., Fan, Z., and Yu, L. (2021). Recurrent spontaneous abortion related to balanced translocation of chromosomes: two case reports. J. Med. Case Rep. 15 (1), 270. doi:10.1186/s13256-021-02848-9
Wang, H., Jia, Z., Mao, A., Xu, B., Wang, S., Wang, L., et al. (2020). Analysis of balanced reciprocal translocations in patients with subfertility using single-molecule optical mapping. J. Assist. Reprod. Genet. 37 (3), 509–516. doi:10.1007/s10815-020-01702-z
Wapner, R. J., Martin, C. L., Levy, B., Ballif, B. C., Eng, C. M., Zachary, J. M., et al. (2012). Chromosomal microarray versus karyotyping for prenatal diagnosis. N. Engl. J. Med. 367 (23), 2175–2184. doi:10.1056/NEJMoa1203382
Wilch, E. S., and Morton, C. C. (2018). Historical and clinical perspectives on chromosomal translocations. Adv. Exp. Med. Biol. 1044, 1–14. doi:10.1007/978-981-13-0593-1_1
Yang, Y., and Hao, W. (2022). Identification of a familial complex chromosomal rearrangement by optical genome mapping. J. Artic. Mol. cytogenet. 15 (1), 41. doi:10.1186/s13039-022-00619-9
Zhai, Y., Zhang, Z., Shi, P., Martin, D. M., and Kong, X. (2021). Incorporation of exome-based CNV analysis makes trio-WES a more powerful tool for clinical diagnosis in neurodevelopmental disorders: A retrospective study. Hum. Mutat. 42 (8), 990–1004. doi:10.1002/humu.24222
Zhang, S., Pei, Z., Lei, C., Zhu, S., Deng, K., Zhou, J., et al. (2022). Detection of cryptic balanced chromosomal rearrangements using high-resolution optical genome mapping. J. Med. Genet. 60, 274–284. doi:10.1136/jmedgenet-2022-108553
Keywords: case report, optical genome mapping, cryptic balanced translocation, unbalanced chromosomal rearrangements, multiple anomalies
Citation: Xie M, Xue J, Zhang Y, Zhou Y, Yu Q, Li H and Li Q (2023) Combination of trio-based whole exome sequencing and optical genome mapping reveals a cryptic balanced translocation that causes unbalanced chromosomal rearrangements in a family with multiple anomalies. Front. Genet. 14:1248544. doi: 10.3389/fgene.2023.1248544
Received: 27 June 2023; Accepted: 22 August 2023;
Published: 07 September 2023.
Edited by:
Ammar Husami, Cincinnati Children’s Hospital Medical Center, United StatesReviewed by:
Shuo Zhang, Fudan University, ChinaFortunato Lonardo, Azienda Ospedaliera San Pio di Benevento, Italy
Copyright © 2023 Xie, Xue, Zhang, Zhou, Yu, Li and Li. This is an open-access article distributed under the terms of the Creative Commons Attribution License (CC BY). The use, distribution or reproduction in other forums is permitted, provided the original author(s) and the copyright owner(s) are credited and that the original publication in this journal is cited, in accordance with accepted academic practice. No use, distribution or reproduction is permitted which does not comply with these terms.
*Correspondence: Qiong Li, liqiong1111@163.com; Haibo Li, lihaibo-775@163.com
†These authors have contributed equally to this work and share first authorship