- 1Laboratório de Citogenética de Peixes, Departamento de Genética e Evolução, Universidade Federal de São Carlos (UFSCar), São Carlos, Brazil
- 2Laboratory of Fish Genetics, Institute of Animal Physiology and Genetics, Czech Academy of Sciences, Liběchov, Czechia
- 3Secretaria de Estado de Educação de Mato Grosso – SEDUC-MT, Cuiabá, Brazil
- 4Museu de Zoologia da Universidade de São Paulo, (MZUSP), São Paulo, Brazil
- 5Institute of Human Genetics, University Hospital Jena, Jena, Germany
- 6Laboratório de Genética Animal, Instituto Nacional de Pesquisas da Amazônia, Coordenação de Biodiversidade, Manaus, Brazil
Although fishes have traditionally been the subject of comparative evolutionary studies, few reports have concentrated on the application of multipronged modern molecular cytogenetic techniques (such as comparative genomic hybridization = CGH and whole chromosome painting = WCP) to analyze deeper the karyotype evolution of specific groups, especially the historically neglected small-sized ones. Representatives of the family Lebiasinidae (Characiformes) are a notable example, where only a few cytogenetic investigations have been conducted thus far. Here, we aim to elucidate the evolutionary processes behind the karyotype differentiation of Pyrrhulina species on a finer-scale cytogenetic level. To achieve this, we applied C-banding, repetitive DNA mapping, CGH and WCP in Pyrrhulina semifasciata and P. brevis. Our results showed 2n = 42 in both sexes of P. brevis, while the difference in 2n between male and female in P. semifasciata (♂41/♀42) stands out due to the presence of a multiple X1X2Y sex chromosome system, until now undetected in this family. As a remarkable common feature, multiple 18S and 5S rDNA sites are present, with an occasional synteny or tandem-repeat amplification. Male-vs.-female CGH experiments in P. semifasciata highlighted the accumulation of male-enriched repetitive sequences in the pericentromeric region of the Y chromosome. Inter-specific CGH experiments evidenced a divergence between both species’ genomes based on the presence of several species-specific signals, highlighting their inner genomic diversity. WCP with the P. semifasciata-derived Y (PSEMI-Y) probe painted not only the entire metacentric Y chromosome in males but also the X1 and X2 chromosomes in both male and female chromosomes of P. semifasciata. In the cross-species experiments, the PSEMI-Y probe painted four acrocentric chromosomes in both males and females of the other tested Pyrrhulina species. In summary, our results show that both intra- and interchromosomal rearrangements together with the dynamics of repetitive DNA significantly contributed to the karyotype divergence among Pyrrhulina species, possibly promoted by specific populational and ecological traits and accompanied in one species by the origin of neo-sex chromosomes. The present results suggest how particular evolutionary scenarios found in fish species can help to clarify several issues related to genome organization and the karyotype evolution of vertebrates in general.
Introduction
South American miniature freshwater fishes cover, by definition, the species that do not exceed 26 mm in the standard length, yet most of them reach the maturity with a length of 20 mm (Weitzman and Vari, 1988). Such a small size limited or hampered especially cytogenetic investigations in these fishes over years. It is also the case of the Lebiasinidae family (Characiformes), whose representatives generally range from 16 to 70 mm in length. They are mainly distributed in the isolated streams of Central America (Panama and Costa Rica) and in almost all South American countries, except for Chile (Weitzman and Weitzman, 2003). Lebiasinidae branches to two subfamilies: Lebiasininae and Pyrrhulininae, which comprise seven genera and 77 recognized species (Froese and Pauly, 2018). Lebiasininae is formed by three genera: Lebiasina (18 recognized species), Piabucina (nine species), and a monotypic Derhamia (Weitzman and Weitzman, 2003; Froese and Pauly, 2018). Pyrrhulininae is considerably more diverse group on the species level (Netto-Ferreira and Marinho, 2013), encompassing four genera: Nannostomus (20 species), Pyrrhulina (18 species), Copella (6 species), and Copeina (2 species) (Weitzman and Weitzman, 2003; Froese and Pauly, 2018). Fishes from this subfamily experienced gradual decrease in the body size during their evolution, resulting in many miniaturized taxa (Netto-Ferreira and Marinho, 2013).
Lebiasinidae was formerly considered to be phylogenetically related to Erythrinidae, Ctenoluciidae, and Hepsetidae, due to sharing of some particular morphological similarities (Buckup, 1998). Nonetheless, more recent robust molecular phylogenetic analyses indicated that Erythrinidae and Hepsetidae are in fact not closely related to Lebiasinidae; instead, the close relationship between Lebiasinidae and Ctenoluciidae was demonstrated (Arcila et al., 2017; Arcila et al., 2018). However, while providing significant advances to this issue, these relationships still require complementary studies for the comprehensive understanding of the evolutionary history among its evolutionary lineages. In this context, conventional and molecular cytogenetic studies have brought valuable contributions to clarify the evolutionary relationships among phylogenetically related fish lineages (reviewed in Cioffi et al., 2018). However, like many other Neotropical fish groups with many representatives of small to miniature body size, the Lebiasinidae family was subject of only limited cytogenetic effort conducted thus far. The very small size of its species, especially the Pyrrhulininae ones, pose a significant challenge as it is notoriously difficult to obtain satisfactory chromosomal preparations, and therefore, most of the available data are limited only to the description of the haploid and/or diploid chromosome numbers (n/2n) in some species, with particularly 2n ranging from 22 in N. unifasciatus to 46 in N. trifasciatus (Scheel, 1973; Oliveira et al., 1991; Arai, 2011). However, a recent study employing the combined conventional and molecular cytogenetic approach in the two Pyrrhulina species (P. australis and Pyrrhulina aff. australis) has been conducted. Despite the fact that both species have been found to share the same 2n (40), without any karyotype differentiation between the sexes, interspecific CGH experiments were convincing enough to demonstrate some degree of genomic divergence, as inferred from a range of non-overlapping species-specific signals (Moraes et al., 2017).
In recent years, modern molecular cytogenetic techniques including whole chromosome painting (WCP) and comparative genomic hybridization (CGH) have been effective in broadening our understanding of the genome evolution and organization in fishes, allowing us to gain more detailed insights into a number of evolutionary issues. Specifically, both techniques have been used for the investigation of genomic divergence among related species (Nagamachi et al., 2010; Symonová et al., 2013; Moraes et al., 2017; Sember et al., 2018b) and to track the origin and evolution of sex chromosomes (Phillips et al., 2001; Henning et al., 2011; Cioffi et al., 2013; Freitas et al., 2018; Oliveira et al., 2018).
The present work aims to extend our understanding of the chromosomal evolutionary processes within the Lebiasinidae family, particularly by a deep investigation of the evolutionary relationships within the Pyrrhulina genus. For this, we applied C-banding, repetitive DNA mapping, CGH, and WCP in two species of Pyrrhulina – P. semifasciata and P. brevis. Our results strongly indicated the presence of a multiple X1X2Y sex chromosome system in P. semifasciata, which clearly emerged from a relatively recent centric fusion event with the signs of emerging male-specific region around the fusion point. In addition, the data obtained also highlight the high chromosomal dynamics within the investigated Pyrrhulina species, probably driven by the small population sizes and/or certain ecological properties of these small fishes.
Materials and Methods
Animals
The number and sex of individuals investigated, collection sites, and the respective deposit numbers are presented in Figure 1 and Table 1. The individuals were collected with the authorization of the Brazilian environmental agency ICMBIO/SISBIO (license no. 48628-2) and SISGEN (A96FF09). All species were properly identified by morphological criteria, and specimens were deposited in the fish collections of the Museu de Zoologia da Universidade de São Paulo (MZUSP) under the voucher numbers (119077, 119079, 123073, 123077, and 123080). The experiments followed ethical and anesthesia conducts and were approved by the Ethics Committee on Animal Experimentation of the Universidade Federal de São Carlos (process number CEUA 1853260315).
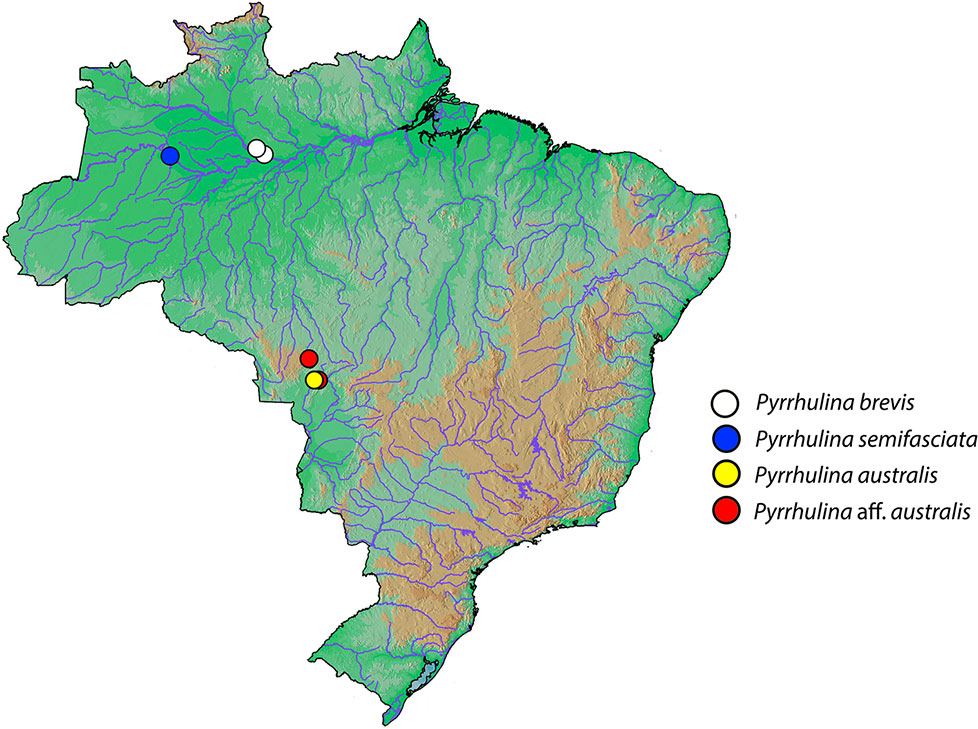
Figure 1 Brazilian map showing the collection sites of Pyrrhulina brevis (white circle), Pyrrhulina semifasciata (blue circles), Pyrrhulina australis (yellow circle), and Pyrrhulina aff. australis (red circles). The last two species were cytogenetically investigated in Moraes et al. (2017).
Chromosome Preparation and Analysis of Constitutive Heterochromatin
Mitotic chromosomes were obtained from kidney cells by the protocol described in Bertollo et al. (2015). Visualization of the amount and distribution of constitutive heterochromatin was done by C-banding according to Sumner (1972).
Preparation of FISH Probes Derived From Repetitive Sequences
The 5S rDNA probe included 120 base pairs (bp) of the 5S rDNA gene coding region and the 200 bp long non-transcribed spacer (NTS) (Pendás et al., 1994). The 18S rDNA probe corresponded to a 1,400-bp-long segment of the 18S rDNA coding region (Cioffi et al., 2009). The 18S and 5S rDNA probes were directly labeled with the Nick-Translation Mix (Roche, Mannheim, Germany) – 18S rDNA with Spectrum Green-dUTP and 5S rDNA with Spectrum Orange-dUTP (both Vysis, Downers Grove, USA), according to the manufacturer’s instructions. (CA)15 and (GA)15 microsatellite probes were directly labeled with Cy3 during the synthesis according to Kubát et al. (2008).
Fluorescence In Situ Hybridization (FISH) for Repetitive DNA Mapping
Fluorescence in situ hybridization (FISH) was performed under high-stringency conditions as described in Yano et al. (2017a). Briefly, the chromosome preparations were incubated with RNase A (40 μg/ml) for 1.5 h at 37°C. After denaturation of the chromosomal DNA in 70% formamide/2x SSC at 70°C, slides were dehydrated in an ethanol series (70, 85 and 100%), 2 min each. 20 µl of the hybridization mixture (100 ng of each probe, 50% deionized formamide and 10% dextran sulfate) were dropped on the slides, and the hybridization was performed for 14 h at 37°C in a moist chamber containing 2x SSC (pH = 7.0). The post-hybridization wash was carried out with 1x SSC for 5 min at 42°C. Finally, the chromosomes were counterstained with DAPI (1.2 µg/ml) and mounted in antifade solution (Vector, Burlingame, CA, USA).
Preparation of Probes for Comparative Genomic Hybridization (CGH)
The gDNAs of males and females of P. semifasciata and P. brevis were extracted from liver tissue by a standard phenol-chloroform-isoamyl alcohol method (Sambrook and Russell, 2001). Two different experimental designs were used for this study. In the first set of experiments, we focused on intraspecific comparisons. In this case, male and female gDNAs of P. semifasciata and P. brevis were labeled and hybridized against the chromosomal background of males from P. semifasciata and P. brevis, respectively. Male gDNAs were labeled with digoxigenin-11-dUTP using DIG-Nick Translation Mix (Roche, Mannheim, Germany), while female gDNAs were labeled with biotin-16-dUTP using BIO-Nick Translation Mix (Roche, Mannheim, Germany). The final hybridization mixture for each slide contained 500 ng of each male- and female-derived labeled gDNA and 25 μg of unlabeled female-derived C0t-1 DNA (to block the shared repetitive sequences; prepared according to Zwick et al., 1997), dissolved in 20 μl of the hybridization buffer (50% formamide, 2× SSC, 10% SDS, 10% dextran sulfate and Denhardt´s buffer, pH 7.0). In the second set of experiments, we focused on the interspecific genomic comparisons; hence, we co-hybridized 500 ng of male-derived gDNA of P. semifasciata (labeled with digoxigenin-11-dUTP) with 500 ng of male-derived gDNA of P. brevis (labeled with biotin-16-dUTP) on the chromosomal background of both species. In this case, the final probe cocktail for each slide contained also 15 μg of female-derived C0t-1 DNA from P. semifasciata and 15 μg of female-derived C0t-1 DNA from P. brevis.
FISH Used for CGH
CGH experiments were performed according to Symonová et al. (2015). Briefly, the slides were aged for 1–2 h at 60°C, followed by a treatment with RNase A (200 µg/ml; 90 min at 37°C in a wet chamber) and with pepsin (50 µg/ml; 3 min at 37°C). Chromosomes were denatured in 75% formamide in 2xSSC at 74°C for 3 min, while the probes were denatured at 86°C for 6 min, chilled on ice (10 min) and then applied on the slides. Hybridization was done for 3 days in a humid chamber (37°C). Subsequently, non-specific hybridization was removed by a stringent washing at 44°C, twice in 50% formamide/2xSSC (10 min each) and three times in 1xSSC (7 min each), and then rinsed in 2xSSC at room temperature. The hybridization signals were detected with Anti-Digoxigenin-Rhodamin (Roche, Mannheim, Germany) diluted in 0.5% bovine serum albumin (BSA) in PBS, and avidin-FITC (Sigma, St. Louis, MO, USA) diluted in PBS containing 10% normal goat serum (NGS). Four final washes were performed at 44°C in 4xSSC/0.1% Tween, 7 min each. Finally, the chromosomes were counterstained with DAPI (1.2 µg/ml) and mounted in an antifade solution (Vector, Burlingame, CA, USA).
Chromosome Microdissection, Probe Preparation, and Labeling
Twenty copies of the Y chromosome from P. semifasciata (hereafter designated as PSEMI-Y) were manually microdissected using the glass needles, under an inverted microscope (Zeiss Axiovert 135). The chromosomes were amplified by degenerate oligonucleotide primed-PCR (DOP-PCR), following the protocol described in Yang et al. (2009). Then, 1 μl of the primary amplification product was used as a template DNA for a secondary labeling DOP-PCR with Spectrum Orange-dUTP (Vysis, Downers Grove, USA) in 30 cycles, following Yang and Graphodatsky (2009). The final probe mixture for one slide contained 500 ng of the PSEMI-Y probe and 30µg of C0t-1 DNA isolated from P. semifasciata female genome.
FISH Used for Whole Chromosome Painting
Chromosomal preparations of males and females of P. semifasciata, P. brevis, and two other Pyrrhulina species (P. australis and P.yrrhulina aff. australis) were used for Zoo-FISH experiments with the PSEMI-Y probe. The hybridization procedures followed Yano et al. (2017a). Hybridization was performed for 48 h at 37°C in a moist chamber. The post-hybridization wash was carried out with 1xSSC for 5 min at 65°C, and in 4xSSC/Tween (RT), and the chromosomes were mounted with DAPI (1.2 µg/ml) in antifade as described above.
Microscopy and Image Processing
At least 30 metaphase spreads per individual were analyzed to confirm the 2n, karyotype structure and the FISH results. Images were captured using an Olympus BX50 epifluorescence microscope (Olympus Corporation, Ishikawa, Japan) with the CoolSNAP system software and the images were processed using Image Pro Plus 4.1 Software (Media Cybernetics, Silver Spring, MD, USA). Final images were optimized and arranged using Adobe Photoshop, version 7.0. Chromosomes were classified as metacentric (m), submetacentric (sm), subtelocentric (st), or acrocentric (a), according to their arm ratios (Levan et al., 1964).
Results
Karyotype Analysis and Heterochromatin Distribution
The karyotype of P. semifasciata was composed of 2n = 41, 1m + 4st + 36a in males, and 2n = 42, 4st + 38a in females (Figure 2). However, all P. brevis individuals displayed 2n = 42 and the karyotype composed of 2sm + 4st + 36a, both in males and females (Figure 3). The distribution of constitutive heterochromatin was restricted to the centromeric and telomeric regions of several chromosomes in both species, but the intensity of C-bands was more pronounced in P. semifasciata. P. brevis further displayed conspicuous interstitial C-bands, which were absent in P. semifasciata (Figures 2 and 3).
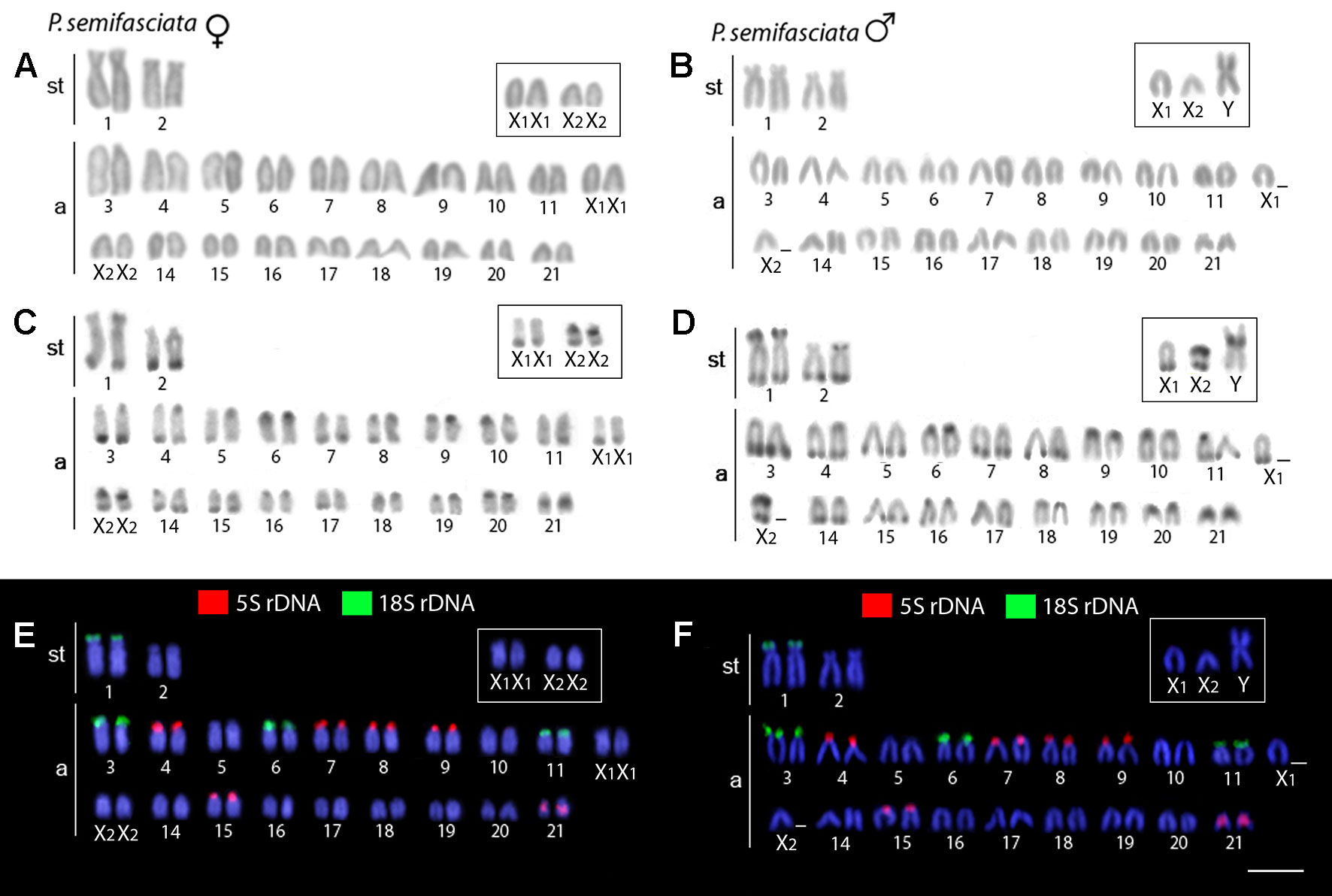
Figure 2 Karyotypes of Pyrrhulina semifasciata (female and male) arranged from chromosomes after different cytogenetic procedures. Giemsa staining in female (A) and male (B), C-banding in female (C) and male (D), dual-color FISH with 18S (green) and 5S (red) rDNA probes in female (E) and male (F). Chromosomes are counterstained with DAPI (blue). Bar = 5 µm.
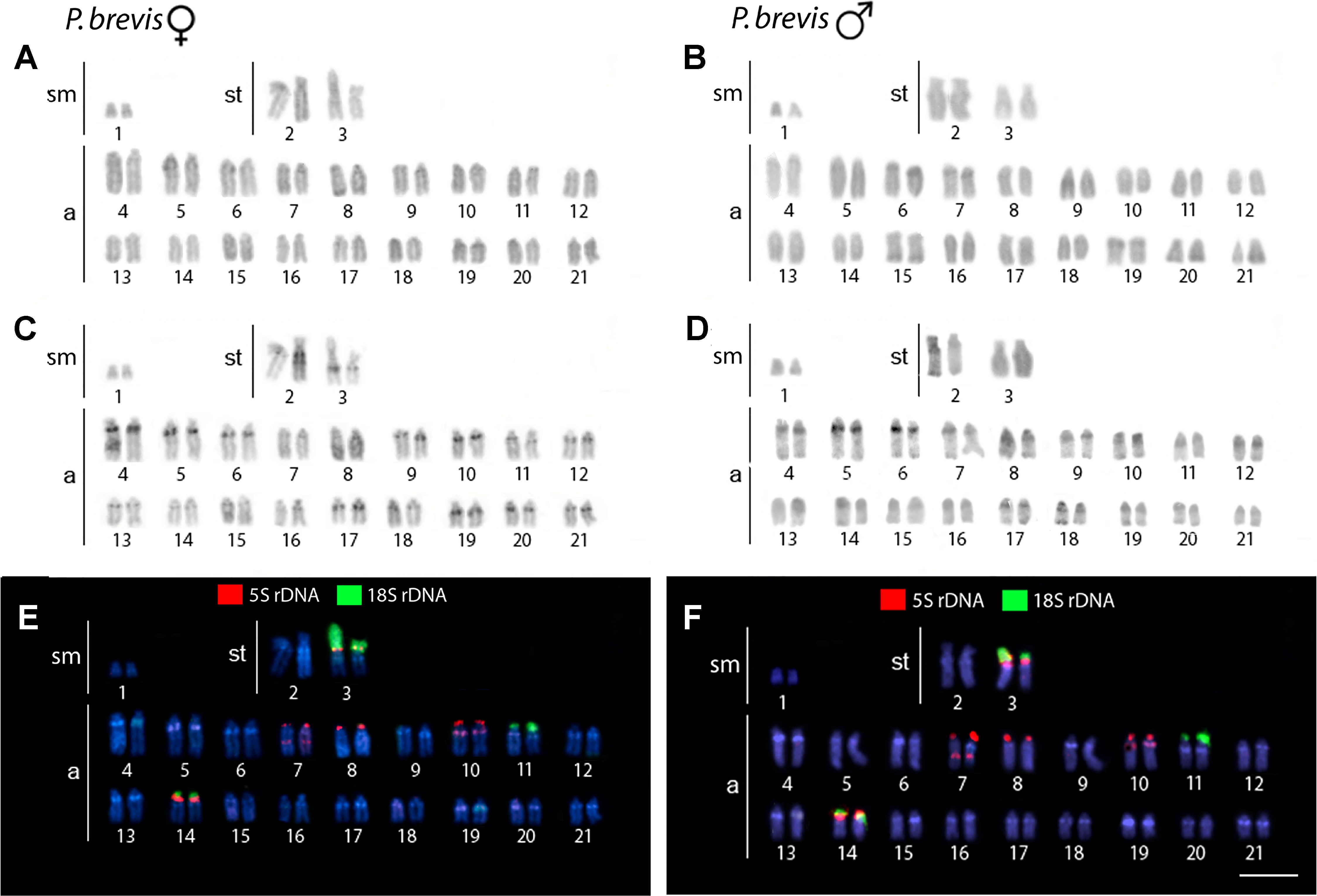
Figure 3 Karyotypes of Pyrrhulina brevis (female and male) arranged from chromosomes after different cytogenetic protocols. Giemsa staining in female (A) and male (B), C-banding in female (C) and male (D), dual-color FISH with 18S (green) and 5S (red) rDNA probes in female (E) and male (F). Chromosomes are counterstained with DAPI (blue). Bar = 5 µm.
Chromosomal Mapping of Repetitive DNA Markers
Dual-color FISH with 5S and 18S rDNA probes revealed that the investigated species differ notably by their patterns of distribution for both multigene families, yet they share a presence of multiple sites for both ribosomal clusters. In P. semifasciata, the 18S rDNA cistrons were found to cover short (p) arms of the largest (st) chromosome pair in the karyotype as well as the p-arms of three acrocentric pairs (nos. 3, 6, and 11), while 5S rDNA signals occupied the p-arms of five acrocentric pairs (nos. 4, 7, 8, 9, and 15), with yet another sixth acrocentric pair (no. 21) bearing an interstitial 5S cluster (Figures 2 and 4). In contrast to P. semifasciata, where none of the rDNA signals occured in synteny or even in the adjacent regions, two out of three pairs of 18S-bearing chromosomes in P. brevis (st pair no. 3 and a pairs nos. 11 and 14) bore also an adjacent 5S rDNA site on their p-arms (pairs nos. 3 and 14). At the same time, pair no. 3 exhibited a remarkable 18S rDNA site amplification accompanied by an extensive size heteromorphism between homologs (Figures 3 and 4). Besides chromosome pairs nos. 3 and 14, there were another three acrocentric pairs bearing 5S rDNA tandem repeats (pairs nos. 7, 8, and 10). Interestingly, pairs 7 and 10 encompassed double 5S rDNA sites—one occupying p-arms and the second being placed interstitially on the long (q) arms on both chromosome pairs (Figures 3 and 4).
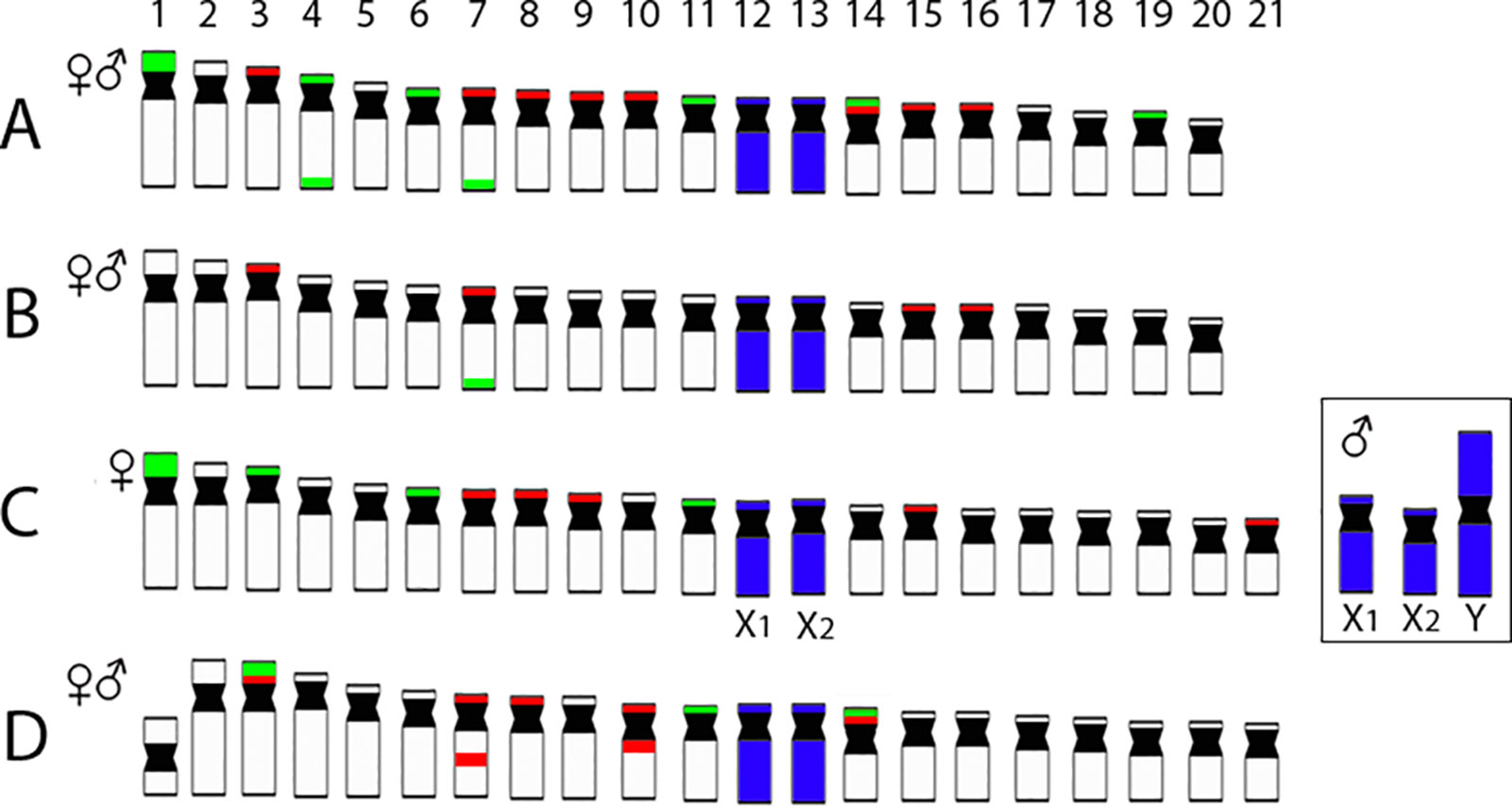
Figure 4 Representative idiograms of Pyrrhulina species showing the distribution of 18S (green) and 5S rDNA (red) sites on the chromosomes of P. australis(A), Pyrrhulina aff. australis(B) (based on our previous study; Moraes et al. (2017), and P. semifasciata(C) and P. brevis(D) (this study). Dark blue indicates the chromosomes painted with the PSEMI-Y probe. Bar = 5 µm.
The chromosomal mapping of the microsatellite motif (CA)15 showed a prominent clustering in the telomeric sites of all chromosomes, especially on q-arms, while few distinct interstitial accumulations were also apparent, especially in P. brevis. On the other hand, (GA)15 motif displayed more scattered distribution along the chromosome complement of both species, though a strong preference for telomeric regions can be also inferred for this motif (Figure 5).
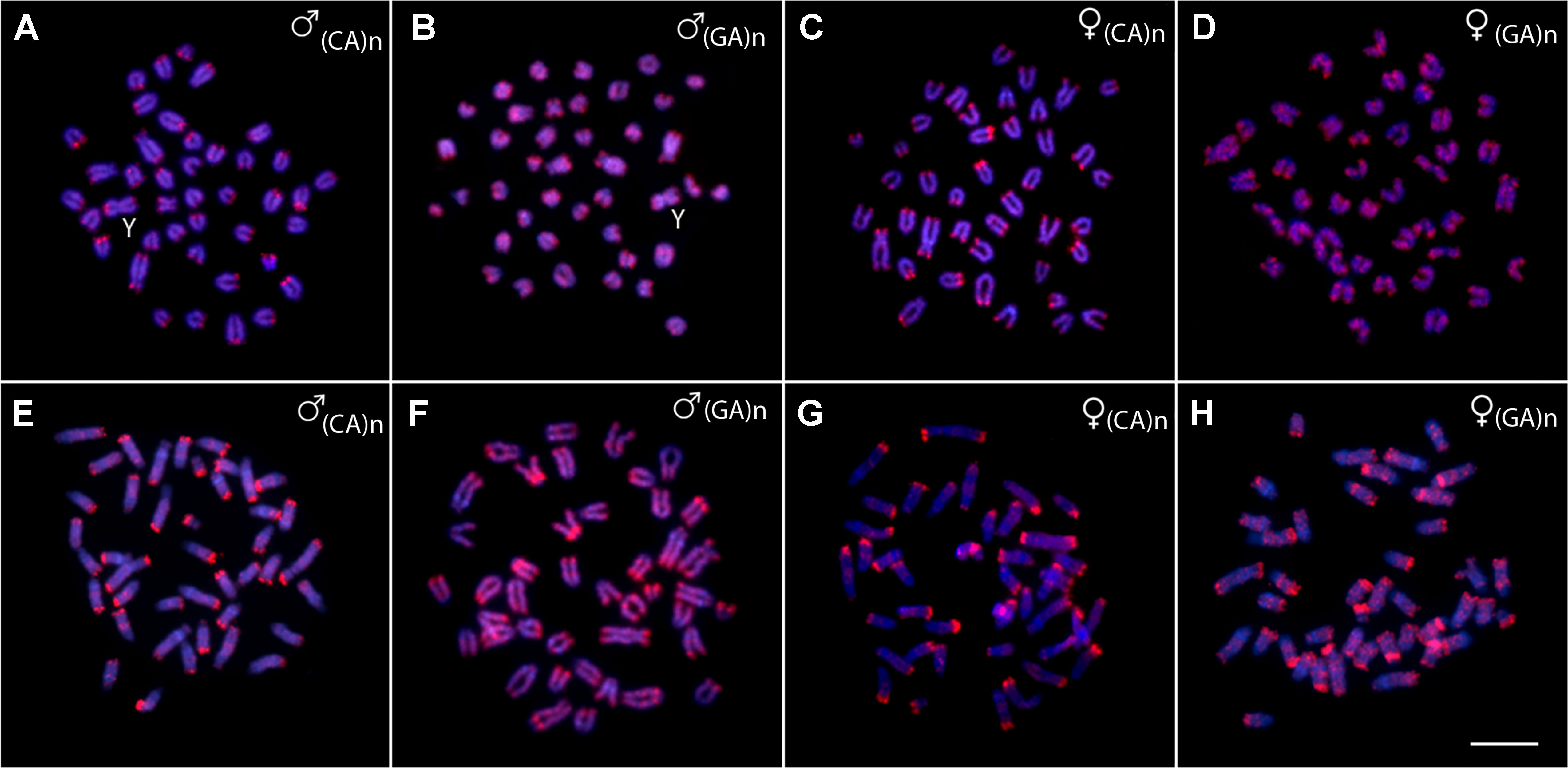
Figure 5 Metaphases from males and females of Pyrrhulina semifasciata(A-D) and Pyrrhulina brevis(E-H) hybridized with the microsatellite probes (CA)15 and (GA)15, showing the general distribution pattern of these repetitive DNAs on the chromosomes. Bar = 5 µm.
Detection of the Male-Specific Region and Interspecific Genomic Divergence by CGH
The intraspecific genomic hybridization between males and females of P. semifasciata revealed a strong binding preference for the male-derived probe to the pericentromeric region of the neo-Y chromosome, while the female-derived probe produced only a weak hybridization signal in this segment (Figures 6A–D). The intraspecific genomic hybridization between males and females of P. brevis did not show clustering of sex-specific sequences on any chromosome (Figures 6E–H).
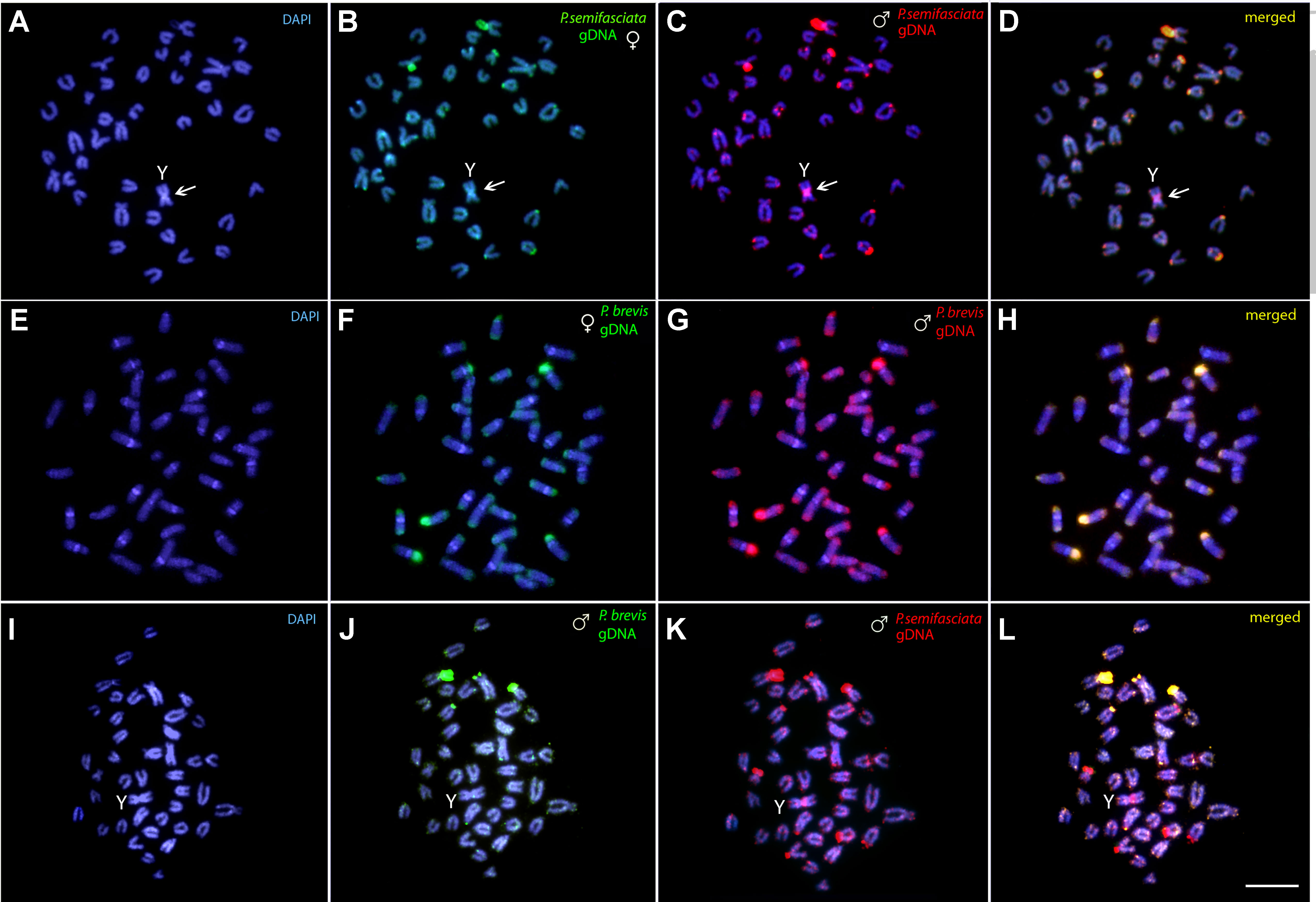
Figure 6 Comparative genomic hybridization (CGH) for intra- and interspecific comparisons. (A–D) Male- and female-derived genomic probes from P. semifasciata mapped against the male chromosomes of P. semifasciata. (E–H) Male- and female-derived genomic probes from P. brevis mapped against the male chromosomes of P. brevis. (I–L) Male-derived genomic probes from both P. semifasciata and P. brevis hybridized together onto male chromosomes of P. semifasciata. The common genomic regions of both compared karyomorphs are depicted in yellow and the arrows indicate the male-specific region located on the Y chromosome of P. semifasciata. Bar = 5 µm.
The interspecific CGH experiments performed to compare the genomes of P. semifasciata and P. brevis on the level of repetitive DNA divergence yielded a range of non-overlapping species-specific signals as a consequence of their specific evolutionary history. Preferential hybridization of the P. brevis-derived probe to the terminal regions of some chromosomes highly likely overlaps with the rDNA sites (Figures 6I–L).
WCP With a PSEMI-Y Probe
The WCP experiments with the PSEMI-Y probe prepared from the neo-Y chromosome of P. semifasciata (Figure 7A) entirely painted four chromosomes (named X1 and X2, two homologs of each) in females and three elements (named X1, X2, and neo-Y chromosome) in males of P. semifasciata, confirming the occurrence of a multiple X1X2Y sex chromosome system in this species (Figures 7B, C). In the cross-species experiments, the PSEMI-Y probe painted two independent chromosome pairs in both males and females of P. brevis, P. australis, and P. aff. australis (Figures 7D–F).
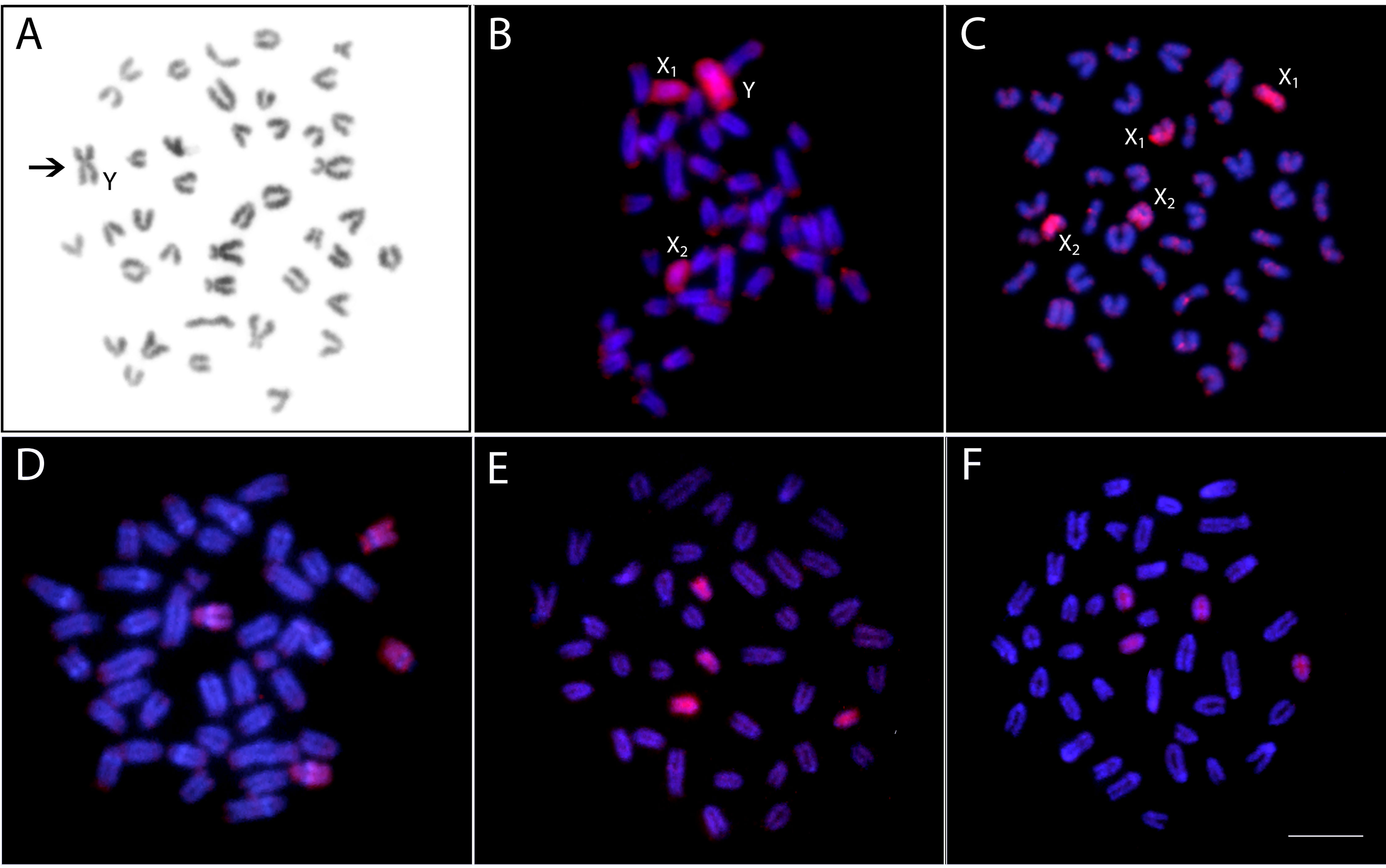
Figure 7 Zoo-FISH with the PSEMI-Y painting probe derived from the Y chromosome (arrow) of P. semifasciata(A) hybridized on the metaphase plates of P. semifasciata male (B), P. semifasciata female (C), P. brevis male (D), P. australis male (E), and Pyrrhulina aff. australis male (F). Bar = 5 µm.
Discussion
Karyotype and Repetitive DNA Patterns in the Genus Pyrrhulina
In many fish groups with taxa of the small-sized body, the lack of cytogenetic data impairs the knowledge about the chromosomal relationships and it prevents to make any meaningful inferences about the impact of chromosome dynamics on their evolutionary history (Liu et al., 2012). The present study brings new insights into the karyotype dynamics of two Pyrrhulina species (P. brevis and P. semifasciata) using conventional and molecular cytogenetic procedures. The karyotype analyses showed the predominance of acrocentric chromosomes in both species, thus documenting a common pattern found in all other studied species from the Lebiasinidae family (Oliveira et al., 1991; Arai, 2011; Moraes et al., 2017). In addition, the observed 2n (41 or 42) fits the conserved 2n found in Pyrrhulina species to date, as it ranges from 40 to 42 chromosomes (Oliveira et al., 1991; Arai, 2011; Moraes et al., 2017). Nonetheless, the difference in 2n between male and female in P. semifasciata (♂41/♀42) stands out due to the presence of multiple X1X2Y sex chromosome system, until now unique for this genus. Based solely on the Giemsa-stained karyotypes, an apparent Robertsonian (Rb) translocation gave rise to the largest metacentric Y sex chromosome in the male karyotype. Karyotypes of both analyzed species are otherwise very similar, being composed of 4st + 38a in P. semifasciata females and 2sm + 4st + 36a in both sexes of P. brevis, as well as in other two Pyrrhulina species (P. australis and P. aff. australis), whose karyotypes were revised by us recently, both presenting 4st + 38a (Moraes et al., 2017). This scenario thus points to the involvement of structural chromosome rearrangements such as pericentric inversions in the differentiation of Pyrrhulina karyotypes.
Reciprocal interspecific CGH patterns encountered in P. semifasciata and P. brevis showed that a certain degree of the genome divergence is apparent between both genomes despite close evolutionary relationships between these congeners – on the level of repetitive DNA distribution, manifested by a presence of certain species-specific CGH signals. In addition, such divergent evolutionary features are also supported by the patterns of C-banding and repetitive DNA mapping, in which an advanced stage of sequence divergence is observed, except for the bright signal, corresponding to C-positive heterochromatic/NOR sites (Figure 6).
In fact, the presence of interstitial C-bands differentiates P. brevis from P. semifasciata, in addition to a pool of repetitive elements in such regions that are not shared between these two species, as evidenced by CGH experiments. On the contrary, interstitial C-bands represent a shared trait between P. brevis and P. aff. australis (Moraes et al., 2017) and their presence supports our view about the probable action of intrachromosomal rearrangements of the peri/pericentromeric inversion type in these genomes. Interestingly, a conspicuous polymorphic block of constitutive heterochromatin found previously on the chromosome pair no. 5 in both males and females of P. aff. australis is not present in the species analyzed herein.
Our hypothesis about the involvement of peri- or paracentric inversions in the karyotype differentiation of P. semifasciata and P. brevis is further strengthened by the patterns of rDNA distribution. More specifically, the presence of two 5S rDNA sites on the same specific chromosomes in P. brevis might indicate that a portion of an original 5S rDNA cluster might have been shifted by inversion to a different location, resulting in a secondary site, similarly to what has been proposed in other (not only) fish groups (Fernandes et al., 2017; Sember et al., 2018a). Nonetheless, bearing in mind that i) the region between doubled 5S rDNA sites encompasses the centromere (thus favorizing pericentric inversions as the underlying mechanism of rDNA mobility) and that, ii) both studied species exhibit very similar karyotypes, an alternative explanation operating with the spreading of 5S rDNA sites through (retro-) transposition is equally probable, especially when taking into account previously reported association of Rex3 non-LTR retrotransposon with amplified 5S rDNA loci in P. australis and P. aff. australis (Moraes et al., 2017). Taken together, Pyrrhulina species deviate from the prevalent patterns of rDNA distribution in fish genomes, where the most of species often bear a single pair of 5S and/or 45S rDNA sites (Gornung, 2013; Sochorová et al., 2018). Nevertheless, multiple rDNA loci are not uncommon in fishes and they might eventually point to elevated genome dynamics, possibly associated with an ongoing interspecific divergence or with the fast fixation due to genetic drift in small populations (Symonová et al., 2013; Sember et al., 2015; Symonová and Howell, 2018). Similar syntenic association of both rDNA classes, as revealed in P. brevis, is repeatedly emerging across the teleost phylogeny, being likely rather a by-product of sub-chromosomal dynamics, though bearing potentially some significance with respect to spatial gene co-expression in interphase nuclei, organized into active and inactive domains (Cavalli and Misteli, 2013; Fraser et al., 2015).
Microsatellites are also repetitive elements useful for analyzing the biodiversity and evolutionary processes among fishes (reviewed in Cioffi et al., 2012a). In fact, clustering of microsatellites might help to trace the level of sub-chromosomal dynamics (Basset et al., 2006) and it might also provide important insights into the processes of sex chromosome differentiation (e.g., Kubát et al., 2008; Pokorná et al., 2011; Kejnovský et al., 2013; Poltronieri et al., 2014). In this study, the (CA)15 and (GA)15 microsatellite motifs showed similar distributional patterns among P. semifasciata and P. brevis and this holds true also for other species of the Pyrrhulina genus already analyzed (Moraes et al., 2017). More specifically, in all four species, the accumulation of (CA)15 motif appeared to be almost exclusively telomere-specific, while (GA)15 showed rather a dispersed distribution throughout the analyzed chromosome complements, in addition to a higher affinity for telomeric regions. From this data, it might be inferred that microsatellite motifs utilized herein are not resolute for tracking the sub-chromosomal dynamics in Pyrrhulina, as their distribution seems to be largely conserved within the analyzed species. Furthermore, they do not show any significant sex chromosome-specific accumulations.
In summary, cytogenetic data accumulated for Pyrrhulina species (Moraes et al., 2017, this study) point on largely conserved karyotype macrostructure, yet evidencing extensive dynamics on the sub-chromosomal level, i.e., divergence in the accumulation of certain repetitive DNA classes and highly probable presence of genome-specific repeats. The sub-chromosomal dynamics might be likely facilitated by divergent evolutionary histories of Pyrrhulina species and by their common endemic status (Netto-Ferreira and Marinho, 2013).
Origin and Differentiation of the X1X2Y Sex Chromosome System in Pyrrhulina semifasciata
Although the different 2n present in males (41) and females (42) could also indicate the occurrence of an X0 sex system, our CGH and particularly WCP results confirmed the occurrence of a multiple X1X2Y sex chromosome system in P. semifasciata. Though fishes possess an amazing variety of sex determination and differentiation mechanisms (Devlin and Nagahama, 2002; Herpin and Schartl, 2015; Schartl et al., 2016; Guiguen et al., 2019), sex chromosomes have been described only in about 5% of cytogenetically analyzed species (based on Arai, 2011). It is, however, increasingly apparent that this information is skewed by frequent presence of homomorphic (i.e., cytogenetically unrecognizable) fish gonosomes, which goes hand in hand with their relative evolutionary “youth” and predisposition to frequent sex chromosome turnovers in closely related species or even within species (Kitano and Peichel, 2012; Pennell et al., 2015; Gamble, 2016; Schartl et al., 2016). In spite of that, at least eight sex chromosome systems (XY and ZW and their variations) are known to occur in certain fish species, fully represented also in Neotropical ichthyofauna (Cioffi et al., 2012b; Cioffi et al., 2017). Among them, X1X2Y gonosomes represent the most frequent multiple sex chromosome system (Kitano and Peichel, 2012; Pennell et al., 2015). In most of the fish taxa with X1X2Y sex chromosomes, centric or tandem fusions are hypothesized to be the underlying mechanism of their origin, giving rise to a large neo-Y chromosome. Examples of this scenario can be found in several fish species, such as Harttia punctata (Loricariidae) (Blanco et al., 2014), Eigenmannia trilineata (Sternopygidae) (Fernandes et al., 2010), Achirus achirus (Achiridae) (Bitencourt et al., 2016), Erythrinus erythrinus and Hoplias malabaricus (Erythrinidae) (Bertollo et al., 2004; Cioffi et al., 2013), and Gymnotus pantanal (Gymnotidae) (Margarido et al., 2007), among others. Based on available data, it seems likely that chromosome rearrangements are often the fully sufficient mechanism to establish the recombination arrest in different fish neo/multiple sex chromosomes, without need for additional repetitive DNA and heterochromatin accumulation (Almeida-Toledo and Foresti, 2001; Oliveira et al., 2008; Fernandes et al., 2010; Cioffi et al., 2011a; Soares et al., 2014; Cardoso et al., 2015; Sember et al., 2015; Bitencourt et al., 2016; Sember et al., 2018b). This scenario sharply contrasts with several examples in animal or plant kingdom, where massive repetitive DNA accumulations are observed on nascent neo-sex chromosomes (e.g., Mariotti et al., 2009; Bachtrog, 2013).
Bearing in mind its general principle, CGH method might represent a useful tool also for delimitation and gross molecular characterization of sex-specific regions on sex chromosomes and, in many cases, it was also sensitive enough to reveal morphologically homomorphic sex chromosomes (Traut et al., 1999; Symonová et al., 2015; Montiel et al., 2017; Yano et al., 2017b; Freitas et al., 2018; Oliveira et al., 2018; Sember et al., 2018b; Zrzavá et al., 2018). In this study, CGH revealed a notable bias in the accumulation of male-specific or male-enriched repetitive DNA in the pericentromeric region of the Y chromosome, when compared to the intensity of female probe hybridization in the same region. We suppose that this pattern might reflect the incipient stage of differentiation inside the male-specific region, similarly to what has been supposed for karyomorphs C and F of the wolf fish H. malabaricus (Freitas et al., 2018; Oliveira et al., 2018; Sember et al., 2018b). It is of interest to note that the region in question encompasses the area around the fusion point on Y. As the recombination in the rearranged region might be significantly reduced or abolished due to sterical constraints, this region gradually accumulates sequence divergence (Faria and Navarro, 2010; Guerrero and Kirkpatrick, 2014). One of the consequences might be a selective advantage, especially if the rearrangement brings into close proximity two (or more) loci whose maintained linkage disequilibrium is favorable to contribute to local adaptation and/or perhaps to speciation (Kawakami et al., 2011) or to resolve genomic conflict (through the linkage of sexually antagonistic genes to male-specific region) (Charlesworth et al., 2005; van Doorn and Kirkpatrick, 2010). The evidence is currently mounting for such scenarios, especially in conjunction with emerging neo-sex chromosomes (Kitano et al., 2009; Yasukochi et al., 2011; Nguyen et al., 2013; Smith et al., 2016; Bracewell et al., 2017). It would be therefore interesting to further investigate, whether the formation of X1X2Y sex chromosomes was a selected event providing an advantage for a species or whether a genetic drift, highly likely acting in small isolated P. semifasciata populations, drove fast fixation of this sex chromosome system randomly just in this species (Charlesworth and Wall, 1999). Importantly, analogous male-vs.-female CGH experiments in other Pyrrhulina species failed to show any sex-specific region (data not shown).
Lastly, we employed WCP with the PSEMI-Y probe in order to evaluate our hypotheses about the origin of the sex chromosome system present in P. semifasciata and to map the orthologous regions in other Pyrrhulina species in an attempt to predict potential homomorphic sex chromosomes in these species. Indeed, this approach facilitated many times a finer-scale survey of fish sex chromosomes with a common (Machado et al., 2011; Parise-Maltempi et al., 2013; Pansonato-Alves et al., 2014; Scacchetti et al., 2015; Yano et al., 2017b; Barros et al., 2018) or independent (Reed et al., 1995; Phillips et al., 2001; Henning et al., 2008; Henning et al., 2011; Cioffi et al., 2011b; Cioffi et al., 2013; Oliveira et al., 2018) origin within the frame of certain family, genus, or species/species complex. Here, WCP with the PSEMI-Y probe applied back against its own chromosome complement painted not only the entire metacentric Y chromosome in males but also the entire acrocentric X1 and X2 chromosomes in both male and female karyotypes. In the cross-species experiments, the PSEMI-Y probe marked four acrocentric chromosomes in both males and females of the other tested Pyrrhulina species. These results not only strongly support the proposed origin via centric fusion between two non-homologous acrocentric chromosomes, but also that this event might have been fixed in P. semifasciata relatively recently, as WCP revealed preservation of all orthologous chromosomes in related Pyrrhulina species without apparent major divergence or rearrangements. As CGH results did not show clustering of sex-specific sequences on any chromosome of the P. brevis complement, it remains to be investigated, whether any of four PSEMI-Y labeled chromosomes represent cryptic (homomorphic) sex chromosomes with a sex-specific region being under resolution limit of the CGH method, or whether sex chromosomes are not present at all in this species and the sex determination is governed by other means (Herpin and Schartl, 2015; Guiguen et al., 2019).
Conclusion
Despite methodological difficulties, sufficient chromosomal preparations were obtained in miniature fishes of the genus Pyrrhulina in the present study. It was possible to demonstrate that chromosomal markers are useful cytotaxonomic tools in characterizing the biodiversity of these fishes, highlighting their evolutionary relationships. Among the obtained results, a discovery of multiple sex chromosome system in our P. semifasciata stands out and its investigation have led us to the following conclusions: i) the neo-Y chromosome arose likely from a centric fusion between two non-homologous acrocentric chromosomes or, possibly, between former (proto) sex chromosomes and an autosomal pair; ii) this event might have been fixed in P. semifasciata relatively recently, as revealed by CGH and WCP; the latter technique revealed all orthologous chromosomes in related Pyrrhulina species without apparent major divergence or rearrangements; iii) formation of neo-Y in P. semifasciata might be driven by genetic drift, while direct selective/adaptive advantage resulting from close association of formerly unlinked genetic content cannot be ruled out; and iv) despite presumably short evolutionary time, CGH revealed considerable accumulation of male-enriched sequences in the pericentromeric region of neo-Y. Whether the origin of multiple sex chromosomes was driven by positive selection or by genetic drift and whether related cryptic sex chromosomes occur in sibling species, remains to be shown. Nonetheless, a nascent male-specific region on Y in P. semifasciata as might be inferred from CGH suggests fast sequence evolution, with the area around the fusion point potentially hosting candidate genes for the sex determination.
The present study further underscores the importance of analyzing data from so-called lower vertebrates such as fishes, as the evolutionary scenarios uncovered in these lineages may provide important clues about the fundamental processes behind the genome organization and the karyotype evolution of vertebrates in general. It may particularly increase our cytogenetic knowledge in so-called higher vertebrates, especially when we take into account that intra- and interchromosomal rearrangements are potent drivers of evolution in Hominoidea, with gibbons of the family Hylobatidae representing the most spectacular example (Weise et al., 2015, Sangpakdee et al., 2016). The same holds true for the dynamics of repetitive DNA, which significantly contributes to karyotype divergence among fishes, but is rarely studied in detail in higher vertebrates (Mrasek et al., 2001; Liehr et al., 2016), despite it might play a relevant role in species’ divergence here as well. Finally, also complex sex-chromosome systems, such as the one described in the present study, hold a great potential to build up the reproductive barriers among different populations of the same species and can be occasionally found also in Hominoidea, as exemplified, e.g., by Trachypithecus cristatus (Xiaobo et al., 2013).
Data Availability
All datasets generated for this study are included in the manuscript.
Ethics Statement
The experiments followed ethical and anesthesia conducts and were approved by the Ethics Committee on Animal Experimentation of the Universidade Federal de São Carlos (Process number CEUA 1853260315).
Author Contributions
RM and AS carried out the cytogenetic analysis and drafted the manuscript. TH, EO, AA-R, and PV helped in the cytogenetic analysis, drafted and revised the manuscript. TL, PR, EF and MM drafted and revised the manuscript. MC and LB coordinated the study, drafted and revised the manuscript. All authors read and approved the final version of the manuscript.
Funding
This study was supported by Conselho Nacional de Desenvolvimento Científico e Tecnológico – CNPq (Proc. nos 401575/2016-0 and 302449/2018-3), Fundação de Amparo à Pesquisa do Estado de São Paulo- FAPESP (Proc. Nos. 2015/26322-0 and 2017/09321-5) and CAPES/Alexander von Humboldt (Proc. No. 88881.136128/2017-01). Further, by the project EXCELLENCE CZ.02.1.01/0.0/0.0/15_003/0000460 OP RDE and with the institutional support RVO: 67985904 (AS, PR) and PPLZ: L200451751 (AS).
Conflict of Interest Statement
The authors declare that the research was conducted in the absence of any commercial or financial relationships that could be construed as a potential conflict of interest.
The handling editor declared a past co-authorship with one of the authors TL.
References
Almeida-Toledo, L. F., Foresti, F. (2001). Morphologically differentiated sex chromosomes in Neotropical freshwater fish. Genetica 111, 91–100. doi: 10.1023/A:1013768104422
Arai, R. (2011). Fish karyotypes: a check list. 1st Edn. Tokyo: Springer. doi: 10.1007/978-4-431-53877-6
Arcila, D., Petry, P., Ortí, G. (2018). Phylogenetic relationships of the family Tarumaniidae (Characiformes) based on nuclear and mitochondrial data. Neotrop. Ichthyol. 16, e180016. doi: 10.1590/1982-0224-20180016
Arcila, D., Orti, G., Vari, R., Armbruster, J. W., Stiassny, M. L. J., Ko, K. D., et al. (2017). Genome-wide interrogation advances resolution of recalcitrant groups in the tree of life. Nat. Ecol. Evol. 1, 20. doi: 10.1038/s41559-016-0020
Bachtrog, D. (2013). Y-chromosome evolution: emerging insights into processes of Y-chromosome degeneration. Nat. Rev. Genet. 14, 113–124. doi: 10.1038/nrg3366
Barros, L. C., Piscor, D., Parise-Maltempi, D., Feldberg, E. (2018). Differentiation and evolution of the W chromosome in the fish species of Megaleporinus (Characiformes, Anostomidae). Sex. Dev. 12, 204–209. doi: 10.1159/000489693
Basset, P., Yannic, G., Yang, F., O’Brien, P. C. M., Graphodatsky, A. S., Ferguson-Smith, M. A. (2006). Chromosome localization of microsatellite markers in the shrews of the Sorex araneus group. Chromosome Res. 14, 253–262. doi: 10.1007/s10577-006-1041-x
Bertollo, L. A. C., Moreira-Filho, O., Cioffi, M. B. (2015). “Direct chromosome preparations from freshwater Teleost fishes,” in Fish cytogenetic techniques: Ray-fin fishes and chondrichthyans. Eds. Ozouf-Costaz, C., Pisano, E., Foresti, E., Almeida-Toledo, L. F. (Boca Raton: CRC Press), 21–26. doi: 10.1201/b18534-4
Bertollo, L. A. C., Oliveira, C., Molina, W. F., Margarido, V. P., Fontes, M. S., Pastori, M. C., et al. (2004). Chromosome evolution in the erythrinid fish, Erythrinus erythrinus (Teleostei: Characiformes). Heredity 93, 228–233. doi: 10.1038/sj.hdy.6800511
Bitencourt, J. A., Sampaio, I., Ramos, R. T. C., Vicari, M. R., Affonso, P. R. A. M. (2016). First report of sex chromosomes in Achiridae (Teleostei: Pleuronectiformes) with inferences about the origin of the multiple X1X1X2X2/X1X2Y system and dispersal of ribosomal genes in Achirus achirus. Zebrafish 14, 90–95. doi: 10.1089/zeb.2016.1333
Blanco, D. R., Vicari, M. R., Lui, R. L., Artoni, R. F., Almeida, M. C., Traldi, J. B., et al. (2014). Origin of the X1X1X2X2/X1X2Y sex chromosome system of Harttia punctata (Siluriformes, Loricariidae) inferred from chromosome painting and FISH with ribosomal DNA markers. Genetica 142, 119–126. doi: 10.1007/s10709-014-9759-4
Bracewell, R. R., Bentz, J. B., Sullivan, B. S., Good, J. M. (2017). Rapid neo-sex chromosome evolution and incipient speciation in a major forest pest. Nat. Commun. 8, 1953. doi: 10.1038/s41467-017-01761-4
Buckup, P. A. (1998). “Relationships of the Characidiinae and phylogeny of characiform fishes (Teleostei: Characiformes),” in Phylogeny and classification of neotropical fishes. Eds. Malabarba, L. R., Reis, R. E., Vari, R. P., Lucena, Z. M. S., Lucena, C. A. S. (Porto Alegre: EDIPUCRS), 123–144.
Cardoso, A. L., Pieczarka, J. C., Nagamachi, C. Y. (2015). X1X1X2X2/X1X2Y sex chromosome systems in the Neotropical Gymnotiformes electric fish of the genus Brachyhypopomus. Genet. Mol. Biol. 38, 213–219. doi: 10.1590/S1415-4757382220140189
Cavalli, G., Misteli, T. (2013). Functional implications of genome topology. Nat. Struct. Mol. Biol. 20, 290–299. doi: 10.1038/nsmb.2474
Charlesworth, B., Wall, J. D. (1999). Inbreeding, heterozygote advantage and the evolution of neo-X and neo-Y sex chromosomes. Proc. R. Soc. Lond. B 266, 51–56. doi: 10.1098/rspb.1999.0603
Charlesworth, D., Charlesworth, B., Marais, G. (2005). Steps in the evolution of heteromorphic sex chromosomes. Heredity 95, 118–128. doi: 10.1038/sj.hdy.6800697
Cioffi, M. B., Camacho, J. P. M., Bertollo, L. A. C. (2011a). Repetitive DNAs and differentiation of sex chromosomes in Neotropical fishes. Cytogenet. Genome Res. 132, 188–194. doi: 10.1159/000321571
Cioffi, M. B., Liehr, T., Trifonov, V., Molina, W. F., Bertollo, L. A. C. (2013). Independent sex chromosome evolution in lower vertebrates: a molecular cytogenetic overview in the Erythrinidae fish family. Cytogenet. Genome Res. 141, 186–194. doi: 10.1159/000354039
Cioffi, M. B., Martins, C., Centofante, L., Jacobina, U., Bertollo, L. A. C. (2009). Chromosomal variability among allopatric populations of erythrinidae fish Hoplias malabaricus: mapping of three classes of repetitive DNAs. Cytogenet. Genome Res. 125, 132–141. doi: 10.1159/000227838
Cioffi, M. B., Molina, W. F., Artoni, R. F., Bertollo, L. A. C. (2012a). “Chromosomes as tools for discovering biodiversity – the case of Erythrinidae fish family,” in Recent trends in cytogenetic studies – Methodologies and applications. Ed. Tirunilai, P. (Rijeka: InTech Publisher), 125–146.
Cioffi, M. B., Moreira-Filho, O., Almeida-Toledo, L. F., Bertollo, L. A. C. (2012b). The contrasting role of heterochromatin in the differentiation of sex chromosomes: an overview from Neotropical fishes. J. Fish Biol. 80, 2125–2139. doi: 10.1111/j.1095-8649.2012.03272.x
Cioffi, M. B., Moreira-Filho, O., Ráb, P., Sember, A., Molina, W. F., Bertollo, L. A. C. (2018). Conventional cytogenetic approaches-useful and indispensable tools in discovering fish biodiversity. Curr. Genet. Med. Rep. 6, 176–186. doi: 10.1007/s40142-018-0148-7
Cioffi, M. B., Sánchez, A., Marchal, J. A., Kosyakova, N., Liehr, T., Trifonov, V., et al. (2011b). Whole chromosome painting reveals independent origin of sex chromosomes in closely related forms of a fish species. Genetica 139, 1065. doi: 10.1007/s10709-011-9610-0
Cioffi, M. D. B., Yano, C. F., Sember, A., Bertollo, L. A. C. (2017). Chromosomal evolution in lower vertebrates: sex chromosomes in neotropical fishes. Genes 8, 258. doi: 10.3390/genes8100258
Devlin, R. H., Nagahama, Y. (2002). Sex determination and sex differentiation in fish: an overview of genetic, physiological, and environmental influences. Aquaculture 208, 191–364. doi: 10.1016/S0044-8486(02)00057-1
Faria, R., Navarro, A. (2010). Chromosomal speciation revisited: rearranging theory with pieces of evidence. Trends Ecol. Evol. 25, 660–669. doi: 10.1016/j.tree.2010.07.008
Fernandes, C. A., Bally, D., Silva, V. F. B., Martins-Santos, I. C. (2010). System of multiple sex chromosomes in Eigenmannia trilineata López & Castello, 1966 (Sternopygidae, Gymnotiformes) from Iguatemi River Basin, MS, Brazil. Cytologia 75, 463–466. doi: 10.1508/cytologia.75.463
Fernandes, C. A., Paiz, L. M., Baumgärtner, L., Margarido, V. P., Vieira, M. M. R. (2017). Comparative cytogenetics of the black ghost knifefish (Gymnotiformes: Apteronotidae): evidence of chromosomal fusion and pericentric inversions in karyotypes of two Apteronotus species. Zebrafish 14, 471–476. doi: 10.1089/zeb.2017.1432
Fraser, J., Williamson, I., Bickmore, W. A., Dostie, J. (2015). An overview of genome organization and how we got there: from FISH to Hi-C. Microbiol. Mol. Biol. Rev. 79, 347–372. doi: 10.1128/MMBR.00006-15
Freitas, N. L., Al-Rikabi, A. B. H., Bertollo, L. A. C., Ezaz, T., Yano, C. F., Oliveira, E. A., et al. (2018). Early stages of XY sex chromosomes differentiation in the fish Hoplias malabaricus (Characiformes, Erythrinidae) revealed by DNA repeats accumulation. Curr. Genomics 18, 01–08. doi: 10.2174/1389202918666170711160528
Froese, R., Pauly, D. (2018). FishBase: World Wide Web electronic publication. Available at: http://www.fishbase.org (Accessed October 25, 2018).
Gamble, T. (2016). Using RAD-seq to recognize sex-specific markers and sex chromosome systems. Mol. Ecol. 25, 2114–2116. doi: 10.1111/mec.13648
Gornung, E. (2013). Twenty years of physical mapping of major ribosomal RNA genes across the teleosts: a review of research. Cytogenet. Genome Res. 141, 90–102. doi: 10.1159/000354832
Guerrero, R. F., Kirkpatrick, M. (2014). Local adaptation and the evolution of chromosome fusions. Evolution 68, 2747–2756. doi: 10.1111/evo.12481
Guiguen, Y., Fostier, A., Herpin, A. (2019). “Sex determination and differentiation in fish: genetic, genomic, and endocrine aspects,” in Sex control in aquaculture. Eds. Wang, H. P., Piferrer, F., Chen, S. L., Shen, Z. G. (New Jersey: John Wiley & Sons Ltd), 35–63. doi: 10.1002/9781119127291.ch2
Henning, F., Moysés, C. B., Calcagnotto, D., Meyer, A., de Almeida-Toledo, L. F. (2011). Independent fusions and recent origins of sex chromosomes in the evolution and diversification of glass knife fishes (Eigenmannia). Heredity 106, 391. doi: 10.1038/hdy.2010.82
Henning, F., Trifonov, V., Ferguson-Smith, M. A., de Almeida-Toledo, L. F. (2008). Non-homologous sex chromosomes in two species of the genus Eigenmannia (Teleostei: Gymnotiformes). Cytogenet. Genome Res. 121, 55–58. doi: 10.1159/000124382
Herpin, A., Schartl, M. (2015). Plasticity of gene-regulatory networks controlling sex-determination: of masters, slaves, usual suspects, newcomers, and usurpators. EMBO Rep. 16, 1260–1274. doi: 10.15252/embr.201540667
Kawakami, T., Butlin, R. K., Cooper, S. J. B. (2011). Chromosomal speciation revisited: modes of diversification in Australian morabine grasshoppers (Vandiemenella, viatica species group). Insects 2, 49–61. doi: 10.3390/insects2010049
Kejnovský, E., Michalovova, M., Steflova, P., Kejnovska, I., Manzano, S., Hobza, R., et al. (2013). Expansion of microsatellites on evolutionary young Y chromosome. PLoS One 8, e45519. doi: 10.1371/journal.pone.0045519
Kitano, J., Peichel, C. L. (2012). Turnover of sex chromosomes and speciation in fishes. Environ. Biol. Fishes 94, 549–558. doi: 10.1007/s10641-011-9853-8
Kitano, J., Ross, J. A., Mori, S., Kume, M., Jones, F. C., Chan, Y. F., et al. (2009). A role for a neo-sex chromosome in stickleback speciation. Nature 461, 1079–1083. doi: 10.1038/nature08441
Kubát, Z., Hobza, R., Vyskot, B., Kejnovský, E. (2008). Microsatellite accumulation in the Y chromosome of Silene latifolia. Genome 51, 350–356. doi: 10.1139/G08-024
Levan, A., Fredga, K., Sandberg, A. A. (1964). Nomenclature for centromeric position on chromosomes. Hereditas 52, 201–220. doi: 10.1111/j.1601-5223.1964.tb01953.x
Liehr, T. (2016). Benign and pathological gain or loss of genetic material - about microscopic and submicroscopic copy number variations (CNVs) in human genetics. Tsitologiia 58, 476–477.
Liu, S., Hui, T. H., Tan, S. L., Hong, Y. (2012). Chromosome evolution and genome miniaturization in minifish. PLoS One 7, e37305. doi: 10.1371/journal.pone.0037305
Machado, T. C., Pansonato-Alves, J. C., Pucci, M. B., Nogaroto, V., Almeida, M. C., Oliveira, C., et al. (2011). Chromosomal painting and ZW sex chromosomes differentiation in Characidium (Characiformes, Crenuchidae). BMC Genet. 12, 65. doi: 10.1186/1471-2156-12-65
Margarido, V. P., Bellafronte, E., Moreira-Filho, O. (2007). Cytogenetic analysis of three sympatric Gymnotus (Gymnotiformes, Gymnotidae) species verifies invasive species in the Upper Paraná River basin, Brazil. J. Fish Biol. 70, 155–164. doi: 10.1111/j.1095-8649.2007.01365.x
Mariotti, B., Manzano, S., Kejnovský, E., Vyskot, B., Jamilena, M. (2009). Accumulation of Y-specific satellite DNAs during the evolution of Rumex acetosa sex chromosomes. Molecular. Genet. Genom. 281, 249–259. doi: 10.1007/s00438-008-0405-7
Montiel, E. E., Badenhorst, D., Tamplin, J., Burke, R. L., Valenzuela, N. (2017). Discovery of the youngest sex chromosomes reveals first case of convergent co-option of ancestral autosomes in turtles. Chromosoma 126, 105–113. doi: 10.1007/s00412-016-0576-7
Moraes, R. L. R., Bertollo, L. A. C., Marinho, M. M. F., Yano, C. F., Hatanaka, T., Barby, F. F., et al. (2017). Evolutionary relationships and cytotaxonomy considerations in the genus Pyrrhulina (Characiformes, Lebiasinidae). Zebrafish 14, 536–546. doi: 10.1089/zeb.2017.1465
Mrasek, K., Heller, A., Rubtsov, N., Trifonov, V., Starke, H., Rocchi, M., et al. (2001). Reconstruction of the female Gorilla gorilla karyotype using 25-color FISH and multicolor banding (MCB). Cytogenet. Cell Genet. 93, 242–248. doi: 10.1159/000056991
Nagamachi, C. Y., Pieczarka, J. C., Milhomem, S. S. R., O’Brien, P. C. M., de Souza, A. C. P., Ferguson-Smith, M. A. (2010). Multiple rearrangements in cryptic species of electric knifefish, Gymnotus carapo (Gymnotidae, Gymnotiformes) revealed by chromosome painting. BMC Genet. 11, 28. doi: 10.1186/1471-2156-11-28
Netto-Ferreira, A. L., Marinho, M. M. F. (2013). New species of Pyrrhulina (Ostariophysi: Characiformes: Lebiasinidae) from the Brazilian Shield, with comments on a putative monophyletic group of species in the genus. Zootaxa 3664, 369–376. doi: 10.11646/zootaxa.3664.3.7
Nguyen, P., Sýkorová, M., Šíchová, J., Kůta, V., Dalíková, M., Čapková, et al. (2013). Neo-sex chromosomes and adaptive potential in tortricid pests. Proc. Natl. Acad. Sci. U.S.A. 110, 6931–6936. doi: 10.1073/pnas.1220372110
Oliveira, C., Andreata, A. A., Almeida-Toledo, L. F., Toledo Filho, S. A. (1991). Karyotype and nucleolus organizer regions of Pyrrhulina cf. australis (Pisces, Characiformes, Lebiasinidae). Rev. Bras. Genet. 14, 685–690.
Oliveira, E. A., Sember, A., Bertollo, L. A. C., Yano, C. F., Ezaz, T., Moreira-Filho, O., et al, (2018). Tracking the evolutionary pathway of sex chromosomes among fishes: characterizing the unique XX/XY1Y2 system in Hoplias malabaricus (Teleostei, Characiformes). Chromosoma 127, 115–128. doi: 10.1007/s00412-017-0648-3
Oliveira, R. R., Feldberg, E., dos Anjos, M. B., Zuanon, J. (2008). Occurrence of multiple sexual chromosomes (XX/XY1Y2 and Z1Z1Z2Z2/Z1Z2W1W2) in catfishes of the genus Ancistrus(Siluriformes: Loricariidae) from the Amazon basin. Genetica 134, 243–249. doi:10.1007/s10709-007-9231-9
Pansonato-Alves, J. C., Serrano, É. A., Utsunomia, R., Camacho, J. P. M., da Costa Silva, G. J., Vicari, M. R., et al. (2014). Single origin of sex chromosomes and multiple origins of B chromosomes in fish genus Characidium. PLoS One 9, e107169. doi: 10.1371/journal.pone.0107169
Parise-Maltempi, P. P., da Silva, E. L., Rens, W., Dearden, F., O’Brien, P. C., Trifonov, V., et al. (2013). Comparative analysis of sex chromosomes in Leporinus species (Teleostei, Characiformes) using chromosome painting. BMC Genet. 14, 60. doi: 10.1186/1471-2156-14-60
Pendás, A. M., Móran, P., Freije, J. P., Garcia-Vásquez, E. (1994). Chromosomal mapping and nucleotide sequence of two tandem repeats of the Atlantic salmon 5S rDNA. Cytogenet. Cell Genet. 67, 31–36. doi: 10.1159/000133792
Pennell, M. W., Kirkpatrick, M., Otto, S. P., Vamosi, J. C., Peichel, C. L., Valenzuela, N., et al. (2015). Y fuse? Sex chromosome fusions in fishes and reptiles. PLoS Genet. 11, e1005237. doi: 10.1371/journal.pgen.1005237
Phillips, R. B., Konkol, N. R., Reed, K. M., Stein, J. D. (2001). Chromosome painting supports lack of homology among sex chromosomes in Oncorhynchus, Salmo, and Salvelinus (Salmonidae). Genetica 111, 119–123. doi: 10.1023/A:1013743431738
Pokorná, M., Kratochvíl, L., Kejnovský, E. (2011). Microsatellite distribution on sex chromosomes at different stages of heteromorphism and heterochromatinization in two lizard species (Squamata: Eublepharidae: Coleonyx elegans and Lacertidae: Eremias velox). BMC Genet. 12, 90. doi: 10.1186/1471-2156-12-90
Poltronieri, J., Marquioni, V., Bertollo, L. A. C., Kejnovský, E., Molina, W. F., Liehr, T., et al. (2014). Comparative chromosomal mapping of microsatellites in Leporinus species (Characiformes, Anostomidae): unequal accumulation on the W chromosomes. Cytogenet. Genome Res. 142, 40–45. doi: 10.1159/000355908
Reed, K. M., Bohlander, S. K., Phillips, R. B. (1995). Microdissection of the Y chromosome and fluorescence in situ hybridization analysis of the sex chromosomes of lake trout, Salvelinus namaycush. Chromosome Res. 3, 221–226. doi: 10.1007/BF00713046
Sambrook, J., Russell, D. W. (2001). Molecular cloning: a laboratory manual. 3rd Edn. New York, NY: Cold Spring Harbor Laboratory Press.
Sangpakdee, W., Tanomtong, A., Fan, X., Pinthong, K., Weise, A., Liehr, T. (2016). Application of multicolor banding combined with heterochromatic and locus-specific probes identify evolutionary conserved breakpoints in Hylobates pileatus. Mol. Cytogenet. 9, 17. doi: 10.1186/s13039-016-0228-x
Scacchetti, P. C., Utsunomia, R., Pansonato-Alves, J. C., da Costa Silva, G. J., Vicari, M. R., Artoni, R. F., et al. (2015). Repetitive DNA sequences and evolution of ZZ/ZW sex chromosomes in Characidium (Teleostei: Characiformes). PLoS One 10, e0137231. doi: 10.1371/journal.pone.0137231
Schartl, M., Schmid, M., Nanda, I. (2016). Dynamics of vertebrate sex chromosome evolution: from equal size to giants and dwarfs. Chromosoma 125, 553–571. doi: 10.1007/s00412-015-0569-y
Scheel, J. J. (1973). Fish chromosomes and their evolution. Charlottenlund, Denmark: International Report Danmarks Akvarium, 22 pp.
Sember, A., Bertollo, L. A., Ráb, P., Yano, C. F., Hatanaka, T., de Oliveira, E. A., et al. (2018b). Sex chromosome evolution and genomic divergence in the fish Hoplias malabaricus (Characiformes, Erythrinidae). Front. Genet. 9, 71. doi: 10.3389/fgene.2018.00071
Sember, A., Bohlen, J., Šlechtová, V., Altmanová, M., Pelikánová, Š., Ráb, P. (2018a). Dynamics of tandemly repeated DNA sequences during evolution of diploid and tetraploid botiid loaches (Teleostei: Cobitoidea: Botiidae). PLoS One 13, 1–27. doi: 10.1371/journal.pone.0195054
Sember, A., Bohlen, J., Šlechtová, V., Altmanová, M., Symonová, R., Ráb, P. (2015). Karyotype differentiation in 19 species of river loach fishes (Nemacheilidae, Teleostei): extensive variability associated with rDNA and heterochromatin distribution and its phylogenetic and ecological interpretation. BMC Evol. Biol. 15, 251. doi: 10.1186/s12862-015-0532-9
Smith, D. A., Gordon, I. J., Traut, W., Herren, J., Collins, S., Martins, D. J., et al. (2016). A neo-W chromosome in a tropical butterfly links colour pattern, male-killing, and speciation. Proc. R. Soc. B 283, 20160821. doi: 10.1098/rspb.2016.0821
Soares, R. X., Bertollo, L. A. C., Cioffi, M. B., Costa, G. W. W. F., Molina, W. F. (2014). Chromosomal distribution of two multigene families and the unusual occurrence of an X1X1X2X2/X1X2Y sex chromosome system in the dolphinfish (Coryphaenidae): an evolutionary perspective. Genet. Mol. Res. 13, 2470–2479. doi: 10.4238/2014.April.3.19
Sochorová, J., Garcia, S., Gálvez, F., Symonová, R., Kovařík, A. (2018). Evolutionary trends in animal ribosomal DNA loci: introduction to a new online database. Chromosoma 127, 141–150. doi: 10.1007/s00412-017-0651-8
Sumner, A. T. (1972). A simple technique for demonstrating centromeric heterochromatin. Exp. Cell Res. 75, 304–306. doi: 10.1016/0014-4827(72)90558-7
Symonová, R., Howell, W. (2018). Vertebrate genome evolution in the light of fish cytogenomics and rDNAomics. Genes 9, 96. doi: 10.3390/genes9020096
Symonová, R., Majtánová, Z., Sember, A., Staaks, G. B., Bohlen, J., Freyhof, J., et al. (2013). Genome differentiation in a species pair of coregonine fishes: an extremely rapid speciation driven by stress-activated retrotransposons mediating extensive ribosomal DNA multiplications. BMC Evol. Biol. 13, 42. doi: 10.1186/1471-2148-13-42
Symonová, R., Sember, A., Majtánová, Z., Ráb, P. (2015). “Characterization of fish genomes by GISH and CGH,” in Fish techniques, ray-fin fishes and chondrichthyans. Eds. Ozouf-Costaz, C., Pisano, E., Foresti, F., de Almeida Toledo, L. F. (Boca Ranton, FL: CRC Press), 118–131. doi: 10.1201/b18534-17
Traut, W., Sahara, K., Otto, T. D., Marec, F. (1999). Molecular differentiation of sex chromosomes probed by comparative genomic hybridization. Chromosoma 108, 173–180. doi: 10.1007/s004120050366
van Doorn, G. S., Kirkpatrick, M. (2010). Transitions between male and female heterogamety caused by sex-antagonistic selection. Genetics 186, 629–645. doi: 10.1534/genetics.110.118596
Weise, A., Kosyakova, N., Voigt, M., Aust, N., Mrasek, K., Löhmer, S., et al. (2015). Comprehensive analyses of white-handed gibbon chromosomes enables access to evolutionary conserved breakpoints compared to the human genome. Cytogenet. Genome Res. 145, 42–49. doi: 10.1159/000381764
Weitzman, M., Weitzman, S. H. (2003). “Lebiasinidae (Pencil fishes),” in Checklist of the freshwater fishes of South and Central America. Eds. Reis, R. E., Kullander, S. O., Ferraris, C. J., Jr. (Porto Alegre: EDIPUCRS), 241–251.
Weitzman, S. H., Vari, R. P. (1988). Miniaturization in South American freshwater fishes: an overview and discussion. Proc. Biol. Soc. Wash. 101, 444–465.
Xiaobo, F., Pinthong, K., Mkrtchyan, H., Siripiyasing, P., Kosyakova, N., Supiwong, W., et al. (2013). First detailed reconstruction of the karyotype of Trachypithecus cristatus (Mammalia: Cercopithecidae). Mol. Cytogenet. 6, 58. doi: 10.1186/1755-8166-6-58
Yang, F., Graphodatsky, A. S. (2009). “Animal probes and ZOO-fish,” in Fluorescence In Situ Hybridization (FISH). Ed. Liehr, T. (Berlin: Springer). doi: 10.1007/978-3-540-70581-9_29
Yang, F., Trifonov, V., Ng, B. L., Kosyakova, N., Carter, N. P. (2009). “Generation of paint probes by flow-sorted and microdissected chromosomes,” in Fluorescence In Situ Hybridization (FISH). Ed. Liehr, T. Application Guide (Berlin, Heidelberg: Springer). doi: 10.1007/978-3-540-70581-9_3
Yano, C. F., Bertollo, L. A., Cioffi, M. B. (2017a). “Fish-FISH: molecular cytogenetics in fish species,” in Fluorescence in situ hybridization (FISH)– Application guide, 2nd Edn. Ed. Liehr, T. (Berlin: Springer), 429–444.
Yano, C. F., Bertollo, L. A., Ezaz, T., Trifonov, V., Sember, A., Liehr, T., et al. (2017b). Highly conserved Z and molecularly diverged W chromosomes in the fish genus Triportheus (Characiformes, Triportheidae). Heredity 118, 276–283. doi: 10.1038/hdy.2016.83
Yasukochi, Y., Miura, N., Nakano, R., Sahara, K., Ishikawa, Y. (2011). Sex-linked pheromone receptor genes of the European corn borer, Ostrinia nubilalis, are in tandem arrays. PLoS One 6, e18843. doi: 10.1371/journal.pone.0018843
Zrzavá, M., Hladová, I., Dalíková, M., Šíchová, J., Õunap, E., Kubíčková, S., et al. (2018). Sex chromosomes of the iconic moth Abraxas grossulariata (Lepidoptera, Geometridae) and its congener A. sylvata. Genes 9, 279. doi: 10.3390/genes9060279
Keywords: fishes, molecular cytogenetics, sex chromosome, chromosomal painting, comparative genomic hybridization (CGH), karyotype evolution
Citation: de Moraes RLR, Sember A, Bertollo LAC, de Oliveira EA, Ráb P, Hatanaka T, Marinho MMF, Liehr T, Al-Rikabi ABH, Feldberg E, Viana PF and Cioffi MB (2019) Comparative Cytogenetics and Neo-Y Formation in Small-Sized Fish Species of the Genus Pyrrhulina (Characiformes, Lebiasinidae). Front. Genet. 10:678. doi: 10.3389/fgene.2019.00678
Received: 04 April 2019; Accepted: 27 June 2019;
Published: 02 August 2019.
Edited by:
Philipp G. Maass, Hospital for Sick Children, CanadaReviewed by:
Ricardo Utsunomia, São Paulo State University, BrazilMara Cristina De Almeida, Universidade Estadual de Ponta Grossa, Brazil
Copyright © 2019 de Moraes, Sember, Bertollo, de Oliveira, Ráb, Hatanaka, Marinho, Liehr, Al-Rikabi, Feldberg, Viana and Cioffi. This is an open-access article distributed under the terms of the Creative Commons Attribution License (CC BY). The use, distribution or reproduction in other forums is permitted, provided the original author(s) and the copyright owner(s) are credited and that the original publication in this journal is cited, in accordance with accepted academic practice. No use, distribution or reproduction is permitted which does not comply with these terms.
*Correspondence: Thomas Liehr, Thomas.Liehr@med.uni-jena.de
†These authors share first authorship.