- Department of Pathobiology, School of Veterinary Medicine, University of Pennsylvania, Philadelphia, PA, United States
DNA transformation of parasitic nematodes enables novel approaches to validating predictions from genomic and transcriptomic studies of these important pathogens. Notably, proof of principle for CRISPR/Cas9 mutagenesis has been achieved in Strongyloides spp., allowing identification of molecules essential to the functions of sensory neurons that mediate behaviors comprising host finding, invasion, and location of predilection sites by parasitic nematodes. Likewise, CRISPR/Cas9 knockout of the developmental regulatory transcription factor Ss-daf-16 has validated its function in regulating morphogenesis of infective third-stage larvae in Strongyloides stercoralis. While encouraging, these studies underscore challenges that remain in achieving straightforward validation of essential intervention targets in parasitic nematodes. Chief among these is the likelihood that knockout of multifunctional regulators like Ss-DAF-16 or its downstream mediator, the nuclear receptor Ss-DAF-12, will produce phenotypes so complex as to defy interpretation and will render affected worms incapable of infecting their hosts, thus preventing establishment of stable mutant lines. Approaches to overcoming these impediments could involve refinements to current CRISPR/Cas9 methods in Strongyloides including regulatable Cas9 expression from integrated transgenes and CRISPR/Cas9 editing to ablate specific functional motifs in regulatory molecules without complete knockout. Another approach would express transgenes encoding regulatory molecules of interest with mutations designed to similarly ablate or degrade specific functional motifs such as the ligand binding domain of Ss-DAF-12 while preserving core functions such as DNA binding. Such mutant transgenes would be expected to exert a dominant interfering effect on their endogenous counterparts. Published reports validate the utility of such dominant-negative approaches in Strongyloides.
Introduction
The advent of transgenesis in parasitic nematodes has opened new means of ascertaining the functions of specific genes in these important pathogens by both gain- and loss-of-function approaches. The first successes in this area involved translating methods for transgenesis in Caenorhabditis elegans to parasites in the genera Strongyloides and Parastrongyloides, which were apt subjects given their capacity to undertake one or more generations of free-living development. Transgenesis in Strongyloides spp. has enabled targeted mutagenesis by CRISPR/Cas9, and this method has already found application in studies that assigned function to genes regulating morphogenesis and host-finding behavior in infective larvae. Transgenes designed to exert dominant interfering effects over specific genes have also revealed essential functions of these genes in growth and developmental of pre-parasitic larvae of Strongyloides stercoralis. Robust new methods for integrative transgenesis in Brugia malayi promise to open these and other avenues of functional genomic study in the filariae and potentially in other obligately parasitic nematodes. None of these functional genomic approaches are new to the basic fields of cellular and molecular biology, but their application to parasitic helminths marks a recent innovation that ranks among the salient advances in parasitology in the past two decades. In view of this, the following review discusses these current findings in detail and proposes new directions in their application to increasingly informative and practical studies of gene function in a group of pathogens that impose the risk of morbidity and mortality on a large segment of the world’s population and exact a heavy toll on the health and welfare of domestic animals.
Transformation with DNA or RNA Constructs is a Prerequisite to Deploying Contemporary Methods for Assessment of Gene Function in Parasitic Nematodes
Approaches to Transgenesis in Clade III and IV Parasitic Nematodes
Parasites in the genus Strongyloides and Parastrongyloides were the first to be successfully transformed with plasmid-based constructs (Lok and Massey, 2002; Grant et al., 2006a; Li et al., 2006; Li et al., 2011). The main factor allowing this advancement was these parasites’ unique ability to execute one or more generations of development as free-living males and females with their progeny in the soil (Viney and Lok, 2015; Grant et al., 2006b). These free-living cycles provide access to the adult germlines of Strongyloides and Parastrongyloides that is unavailable in obligately parasitic nematodes. Free-living generations of Strongyloides and Parastrongyloides can be maintained in agar plate culture using methods adapted from those used for the free-living nematode C. elegans (Grant et al., 2006a; Lok and Unnasch, 2013). Parastrongyloides trichosuri can execute an indefinite number of sequential free-living generations in plate culture, enhancing its utility as a subject for genetic study. Most crucially, the body plans of free-living female Strongyloides and Parastrongyloides and of C. elegans hermaphrodites are so similar that it was straightforward to adapt methods for gene delivery by gonadal microinjection from C. elegans (Fire, 1986; Fire et al., 1990a; Fire et al., 1990b; Mello et al., 1991; Mello and Fire, 1995) to the parasites (Figure 1A) (Lok and Massey, 2002; Grant et al., 2006a; Li et al., 2006; Li et al., 2011). A similar approach was used to achieve transgenesis in Strongyloides stercoralis by microinjection of plasmid constructs into the testes of free-living males (Shao et al., 2017). These approaches, involving transfer of plasmid-based vector constructs, allow promoter-regulated, tissue-specific transgene expression in F1 transformants (Figure 1A) (Grant et al., 2006a; Li et al., 2006; Junio et al., 2008; Li et al., 2011; Shao et al., 2017), and integration of transgene sequences into the chromosomes of Strongyloides ratti permits establishment of stable transgenic lines of this parasite (Figure 1A) (Shao et al., 2012). These methods have enabled studies of gene function in Strongyloides and Parastrongyloides that have revealed anatomical and temporal patterns of gene expression and essential functions of specific genes in development and survival of the pre-infective stages of these parasites (Figure 1A) (Massey et al., 2003; Grant et al., 2006a; Junio et al., 2008; Castelletto et al., 2009; Stoltzfus et al., 2012; Massey et al., 2013; Yuan et al., 2014a; Yuan et al., 2014b; Lei et al., 2017; Yuan et al., 2017).
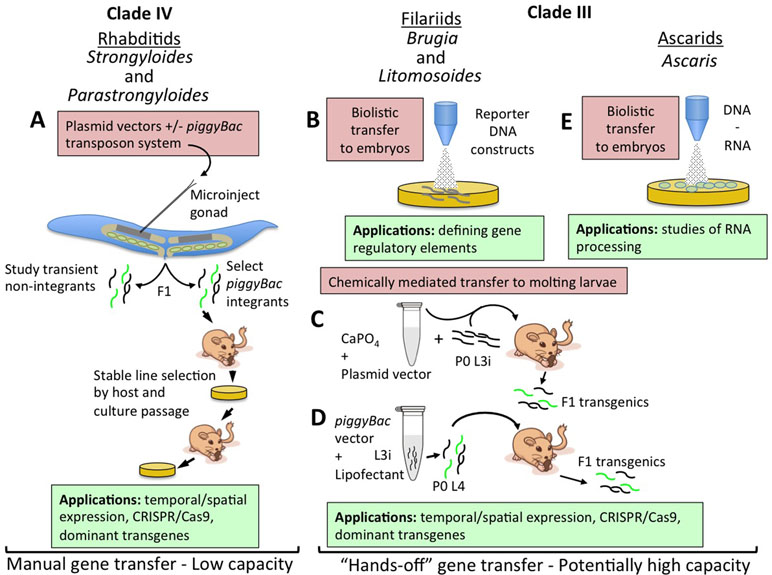
Figure 1 Strategies for transfer of nucleic acids into parasitic nematodes. (A) Microinjection of plasmid vector constructs into the syncytial gonads (shaded area of distal gonad arm in cartoon) of parental free-living female Strongyloides and Parastrongyloides, with or without transposon-mediated integration. Transiently transformed F1 larvae may be investigated for reporter expression patterns or phenotypes induced by dominant transgenes or CRISPR/Cas9-mediated gene disruption or editing. Lines of parasites with transgenes integrated by the co-injected piggyBac transposon system may be selected and maintained by alternating host and culture passage. (B) Biolistic transfer of reporter DNA constructs into Brugia malayi embryos within adult female worms has enabled definition of basic gene regulatory elements and has identified elements for ecdysteroid signaling in this parasite. Embryos transformed in this manner have thus far been incapable of further development. (C) Chemically mediated vector transfer in B. malayi by co-injection of parental infective third-stage larvae (L3i) into susceptible gerbils. Transgenic adult parasites and transgenic F1 microfilariae result from this procedure. (D) Refinement of chemically mediated transfection involving lipofectant transfer of plasmid vectors with the piggyBac transposon system to parental L3i of B. malayi undergoing the molt to L4 in culture and then inoculated intraperitoneally in to susceptible gerbils. F1 transgenic microfilariae include integrants and may prove amenable to selection of stable lines by mosquito and gerbil passage. (E) Biolistic transfer of DNA and RNA into embryos of Ascaris suum. This approach has enabled studies of RNA processing in these parasites.
Significant strides have also been made towards transgenesis in the filaria Brugia malayi. This parasite, and all filariae, are far more challenging subjects for molecular genetic study, as they have no free-living generation comparable to Strongyloides and related genera, and furthermore, unlike soil transmitted parasitic nematodes, their pre-infective stages require an arthropod vector for development. These barriers notwithstanding, transient DNA transformation of B. malayi (Higazi et al., 2002; Higazi and Unnasch, 2013) and Litomosoides carinii (Jackstadt et al., 1999) has been achieved by particle bombardment or biolistics (Figure 1B). In the case of B. malayi, the primary object of this approach has been to investigate the structure and function of gene regulatory sequences by transforming embryos within the uteri of female worms with plasmid constructs encoding fluorescent reporters linked to wild-type or mutant regulatory sequences, promoters mainly, and evaluating function by quantifying reporter activation. These bombarded embryos are not developmentally competent, but this approach has, nevertheless, yielded a wealth of basic information on the structural motifs required for promoter activity (Shu et al., 2003; Higazi et al., 2005; de Oliveira et al., 2008; Bailey et al., 2011), trans-splicing (Higazi and Unnasch, 2004; Liu et al., 2007; Liu et al., 2010a), and operon function (Liu et al., 2010b) in B. malayi. This approach to transient transgenesis has also revealed the function of microRNAs in regulation of B. malayi genes (Liu et al., 2015), the presence of tetracycline regulatable promoters (Liu et al., 2011), and the transcriptional targets of ecdysone signaling in this parasite (Tzertzinis et al., 2010; Liu et al., 2012). Notably, the approach of transiently transfecting embryos has also been applied to Ascaris suum (Figure 1E) (Davis et al., 1999).
Stable, heritable transformation has been achieved in B. malayi by chemically mediated transfer of plasmid vector constructs into developing infective third-stage larvae (L3i; Figures 1C, D). Initial applications of this approach involved forming calcium phosphate co-precipitates with plasmid DNA constructs encoding a luciferase (GLuc) reporter (Xu et al., 2011). Mosquito-derived infective third-stage larvae (iL3) were then co-injected along with the calcium phosphate plasmid DNA co-precipitate into the peritoneal cavities of susceptible gerbils. Substantial proportions of B. malayi adults and their microfilarial progeny recovered from gerbils inoculated with transduced iL3 expressed GLuc, proving inheritance of the reporter transgene in the F1 generation (Figure 1C). This method was subsequently refined such that DNA transfer was achieved by lipofection of mosquito-derived L3i of B. malayi that were undergoing the L3i-L4 molt in co-culture with bovine embryo skeletal muscle cells (Liu et al., 2018). Such cultured larvae co-transformed with GLuc and reporters encoding fluorescent proteins and flanked by inverted tandem repeats specific for the piggyBac transposon system as well as a plasmid encoding the piggyBac transpose were capable of establishing patent infection in susceptible gerbils (Figure 1D). Analysis adult B. malayi and F1 microfilariae revealed transgene expression and also integration of transgene sequences into the parasite genome. Altogether, this represents a remarkable advancement in functional genomic methodology for filariae and for all parasitic nematodes. For the former, it marks the first integrative transformation of B. malayi, the most widely used model in experimental filariasis, and the first deployment of fluorescent reporters that will allow rapid visual selection of transgenic parasites and assessment of temporal and anatomical patterns of transgene expression. For all parasitic nematodes, the work on B. malayi identifies chemically mediated DNA transfer as an alternative to gonadal microinjection as a means of transgenesis. This method has the advantage of DNA transfer into the larval germline, making it applicable to obligate parasitic nematodes in which the adult germline is not as easily accessible as it is in the free-living adults of Strongyloides and related parasites. Chemically mediated gene transfer has the additional and paramount advantage of being a “hands-off” method not requiring the meticulous and painstaking process of microinjection and having the potential for high capacity transformation of hundreds or thousands of parasites in a single replication of the procedure (Figure 1B–E). The common factor in success of the two chemically mediated approaches used to date in B. malayi (Xu et al., 2011; Liu et al., 2018) appears to have been that DNA transfer was undertaken during an intermolt period, in this case the L3–L4 molt, in which the nascent cuticle was transiently competent to take up either the calcium phosphate–DNA co-precipitate or the lipofectamine–DNA micelles. Parasitic nematodes such as hookworms or trichostrongyles and related rodent parasites such as Nippostrongylus brasiliensis and Heligmosomoides polygyrus, whose pre-parasitic larvae develop in the soil or on herbage, would seem to be particularly amenable to this approach, as they can be cultured through two molt cycles (L1–L3) in simple laboratory media. The same is true of Strongyloides and related genera, where the combination of life cycles containing both free-living and parasitic generations and a hands-off, high-capacity method of DNA transfer would make a particularly powerful model for molecular genetics in parasitic nematodes.
Future Directions in Transgenesis for Parasitic Nematodes
Gene Editing via Transgenesis in Pre-Parasitic Larvae of Soil-Transmitted Parasitic Nematodes
The microinjection-based methods of gene transfer into free-living females of Strongyloides spp. and of Parastrongyloides trichosuri have not been adapted to obligately parasitic nematodes whose pre-parasitic larvae develop in and are transmitted from the soil or vegetation and whose adult stages reside solely in vertebrate animal host. These include the hookworms and trichostrongyles of paramount importance to human medicine and animal agriculture. While the adult germlines of these parasites are not readily accessible for gene transfer, the rudimentary gonads of their pre-parasitic larvae, which are easily cultured in the laboratory, are accessible. Certainly, the chemically mediated and lipofectant-facilitated systems of gene transfer that have been so successful for ex vivo iL3 of Brugia malayi (Xu et al., 2011; Liu et al., 2018) should be investigated as possible approaches to gene transfer into hookworms and trichostrongyles of humans and domestic animals and their rodent counterparts that are important subjects for immunological research (Bouchery et al., 2017).
In addition to these “hands-off” approaches to gene transfer, it is also possible to transfer CRISPR/Cas9 elements into pre-parasitic larvae of soil-transmitted parasites by microinjection (Adams et al., 2019). Mutations were targeted to the rol-6 ortholog in S. stercoralis by microinjecting a nucleoprotein comprising Cas9 and a target specific gRNA along with lipofectamine into the body cavities of iL3. This approach produced P0 mutant larvae exhibiting a classic “roller” phenotype identical to that seen in rol-6 mutants of C. elegans. This approach might be adapted to the pre-infective larvae of other soil-transmitted parasitic nematodes as well.
Deployment of “Hands-Off”, High-Capacity Gene Transfer in Strongyloides and Other Soil-Transmitted Parasitic Nematodes
A major shortcoming of gene transfer by gonadal microinjection in Strongyloides and related parasites has been the limited numbers of F1 transgenic larvae that can be produced by this approach (Figure 1A). This creates difficulties in amassing samples of transgenic larvae of sufficient size to achieve statistical power and is a particular hurdle to the establishment of transgenic lines of S. stercoralis, where the most susceptible rodent host, the gerbil, requires an approximate minimum of 100 transgenic larvae to mount a patent infection yielding transgenic F2 progeny. This situation calls for a concerted effort to achieve chemically or lipofectant-mediated gene transfer into pre-infective larvae of Strongyloides spp. in a manner similar to the successful methods now available for ex vivo iL3 of Brugia spp. (Xu et al., 2011; Liu et al., 2018). It seems likely that these “hands-off” methods (Figure 1B–E) could be similarly deployed for gene transfer into pre-parasitic larvae of hookworms, trichostrongyles, and their rodent-infecting counterparts.
CRISPR/Cas9 Mutagenesis is Feasible in Parasitic Nematodes
DNA transformation of parasitic nematodes opens the formal possibility of deploying CRISPR/Cas9 for targeted mutagenesis as a means of directly assessing gene function in these pathogens. Proof of principle for CRISPR/Cas9 mutagenesis has been achieved in Strongyloides stercoralis and Strongyloides ratti. This includes preliminary evidence of precisely targeted double-stranded DNA breaks (DSB) and homology-directed repair with a co-transfected DNA template (Lok et al., 2017) and comprehensive demonstration and phenotypic evaluation of targeted disruption of Ss-unc-22 and Sr-unc-22 in S. stercoralis and S. ratti, respectively (Gang et al., 2017). Mutations have been created in Strongyloides by transducing free-living females with basic CRISPR elements, including gRNA, Cas9 codon-optimized for expression in Strongyloides, and selectable markers encoded in plasmid vectors (Gang et al., 2017; Lok et al., 2017) or by microinjecting gonads of free-living females with pre-formed nucleoproteins comprising recombinant Cas9 and in vitro transcribed gRNAs (Gang et al., 2017; Adams et al., 2019). To date, transduction of parental worms with plasmid-encoded CRISPR/Cas9 elements has resulted in more efficient mutagenesis than is seen with delivery of pre-formed nucleoproteins (Gang et al., 2017).
Informative phenotypes have been associated with both large deletions at those sites mediated by non-homologous end joining (NHEJ) and with insertional mutagenesis via homology-directed repair (HDR) of CRISPR/Cas9-induced DSB. Most notably, a prominent “twitcher” phenotype, which is exacerbated by nicotine exposure, results from CRISPR/Cas9 disruption of Ss-unc-22 and Sr-unc-22. These genes, like their C. elegans ortholog, encode the intracellular muscle protein twitchin (Moerman and Baillie, 1979; Moerman et al., 1988; Gang et al., 2017). The unc-22 phenotypes in Strongyloides spp. are highly reminiscent of the twitcher phenotype resulting from mutations in C. elegans unc-22. Notably, the C. elegans twitcher phenotype is dominant, occurring in both homozygous and heterozygous unc-22 mutants. Homology-directed repair has been exploited to integrate short disrupting oligonucleotides containing multiple stop codons and novel priming sites for genotyping at the site of CRISPR/Cas9-induced DSBs (Lok et al., 2017) as well as a full reporter cassette encoding a fluorescent reporter that serves to both disrupt the target gene and to provide a convenient marker for mutant selection (Gang et al., 2017). In the latter instance, F1 larvae expressing the red fluorescent protein mRFPmars under the body wall-specific promoter for Ss-act-2 from the repair template in an episomal array or from the template integrated into a CRISPR/Cas9 cut site in Ss-unc-22 could be distinguished by PCR using primers specific for the repair template and the genomic region flanking the cut site and by the consistent pattern of body wall expression in integrants (Gang et al., 2017). Integrants in this case exhibited a uniform twitcher phenotype, providing proof of principle for the use of reporter-encoding repair templates to disrupt target genes by CRISPR/Cas9- and HDR-mediated integration.
Most significantly, CRISPR/Cas9-induced mutations in S. stercoralis are heritable and may be propagated by passage in gerbils, which constitute the most authentic rodent model of the entire spectrum of S. stercoralis infection and disease in humans (Nolan et al., 1993; Gang et al., 2017).
In addition to the studies of Ss-unc-22, which demonstrated that its encoded muscle component is required for normal motility of iL3 (Gang et al., 2017), CRISPR/Cas9 and the Strongyloides model have found application in studies of genes involved in the sensory biology of these infective larvae. Soil transmitted parasitic nematodes such as hookworms and Strongyloides spp. invade the host by skin penetration and develop as free-living pre-parasitic larvae in contaminated soil. iL3 of these parasites orient toward potential hosts using a complex of chemical and physical cues (Bryant and Hallem, 2018b; Castelletto et al., 2014). Orienting towards a thermal source is a behavior that many species of environmentally motile parasitic nematode iL3 use to acquire a host (Castelletto et al., 2014; Bryant and Hallem, 2018a; Bryant and Hallem, 2018b; Bryant et al., 2018). In C. elegans, thermal signals are received by receptor guanylate cyclases in sensory neurons of the amphidial complex, which are in contact with the external environment. These receptors signal through a heteromeric cGMP-activated cation channel, comprising TAX-2 and TAX-4 subunit proteins, that regulates polarization of the sensory neuron and potentiation of thermotactic responses (Komatsu et al., 1996; Bargmann and Mori, 1997; Komatsu et al., 1999). The tax-4 ortholog in S. stercoralis has been disrupted by CRISPR/Cas9, and knockout iL3 exhibit diminished themotaxis towards 34°C compared to controls derived from parental worms receiving all CRISPR/Cas9 elements except the Cas9 endonuclease. This provides direct evidence that tax-4 is required for normal thermotaxis by iL3 of this parasite (Bryant and Hallem, 2018a; Bryant and Hallem, 2018b; Bryant et al., 2018). Given the involvement of the TAX-2/TAX-4 channel in other sensory signaling pathways in free-living nematodes (Komatsu et al., 1996; Komatsu et al., 1999), it is likely that S. stercoralis tax-4 knockouts will exhibit decrements in chemotactic as well as thermotactic behaviors when these mutants are subjected to the appropriate assays (Bryant and Hallem, 2018b; Bryant et al., 2018).
Future Directions for CRISPR/Cas9 in Parasitic Nematodes
Deployment of CRISPR/Cas9 in Filariae
Robust systems for both integrative (Liu et al., 2018) and non-integrative (Xu et al., 2011) transgenesis are now available for Brugia malayi. With these systems in place, targeted mutagenesis and gene editing in this important model of human filariasis are imminently possible. The toolkit for integrative transgenesis assembled by Liu et al. (Liu et al., 2018) comprises a set of multifunctional vectors encoding multiple elements of the piggyBac transposon system that could be readily adapted to encode CRISPR/Cas9 elements such as the Cas9 endonuclease, gene-specific gRNAs, and templates for homology-directed repair in a compact and efficient gene delivery system. The time is right for this advancement.
Single-Copy Transgene Integrations
Among the very significant findings that arose from proof of principle for CRISPR/Cas9 in Strongyloides (Gang et al., 2017) was that full gene coding sequences, in this case an Ss-act-2 transcriptional reporter encoding mRFPmars, can be integrated into the parasite genome at CRISPR/Cas9 cut sites by homology-directed repair. This approach provides a distinct advantage over current transposon-based methods for transgene integration, which integrate multiple transgene copies at random sites in the genome. Integrations by piggyBac typically favor coding sequences, creating the possibility of confounding insertional mutagenesis (Shao et al., 2012; Lok, 2013).
Regulatable CRISPR/Cas9
The capability to target transgene integrations to precise locations within the Strongyloides genome by CRISPR/Cas9 creates the potential to establish lines of these parasites that stably express the Cas9 endonuclease from single transgene copies. Parasites from such lines could subsequently be transformed to express gene-specific gRNAs to disrupt target genes and assess resulting phenotypes. Constitutive expression of Cas9 in C. elegans exerts some deleterious effects on fitness of the transgenic worms (Waaijers et al., 2013). Likewise, disrupting genes essential for invasion or establishment of the worms in mammalian hosts would prevent propagation of mutants and assessment of phenotypes affecting host infectivity, or crucial host–parasite interactions. A solution to these problems could be conditional or inducible Cas9 expression from integrated transgene sequences in stable parasite lines. We envision two possible approaches to regulating Cas9 expression in this context. The first would be to express Cas9 under promoters that are activated in the presence of a small molecule regulator such as tetracycline (Tet). Tet-responsive genes have been identified in Brugia malayi (Liu et al., 2011; Wurmthaler et al., 2019), and a tet-regulated promoter has been deployed for inducible transgene expression in C. elegans (Liu et al., 2011; Wurmthaler et al., 2019). These findings argue for identifying Tet-responsive genes in Strongyloides that could be similarly incorporated into regulatable transgene expression systems generally and particularly for the regulated expression of Cas9 in stable parasite lines.
Another approach to regulatable expression of Cas9 and other transgene-encoded factors would be to fuse these to degradation domains that are stabilized in the presence of small molecules. Under these conditions, the transgene product would be stabilized in the presence of the small molecule but targeted to the proteasome for degradation in its absence. Such a system, incorporating the degradation domain of dihydrofolate reductase, which is stabilized in the presence of the folate-targeting drug trimethoprim, has been used to regulate transgene expression in Plasmodium falciparum (Muralidharan et al., 2011), and it has shown promise in preliminary experiments with transgenic Strongyloides (Lok et al., 2017).
Transgenes With Dominant Mutations are Tools for Functional Genomic Study in Parasitic Nematodes.
Functions of Genes Encoding a Variety of Catalytic or Regulatory Proteins may be Interrogated With Dominant Interfering Transgenes
Prior to the advent of targeted mutagenesis, transgenesis in Strongyloides spp. enabled a functional genomic approach based on expression of transgenes encoding mutant proteins designed to exert a dominant interfering effect on the target of interest. This approach may be used to suppress the functions of catalytic, signaling, and regulatory proteins that comprise separate functional domains and domains that bind to substrates, ligands, or DNA (Figure 2). The strategy holds that when overexpressed from multi-copy arrays relative to the single-copy endogenous target in the subject organism, transgene-encoded mutant proteins that have disrupted functional domains and intact binding domains will out-compete their endogenous wild-type counterparts for binding partners but fail to undertake their putative functions, thus resulting in dominant loss of function in the target gene of interest. Basic mechanisms for this approach to simulate loss of function in target genes are illustrated for cytoplasmic signaling kinases and metabolic enzymes (Figure 2A), membrane receptor kinases (Figure 2B), and transcription factors (Figure 2C).
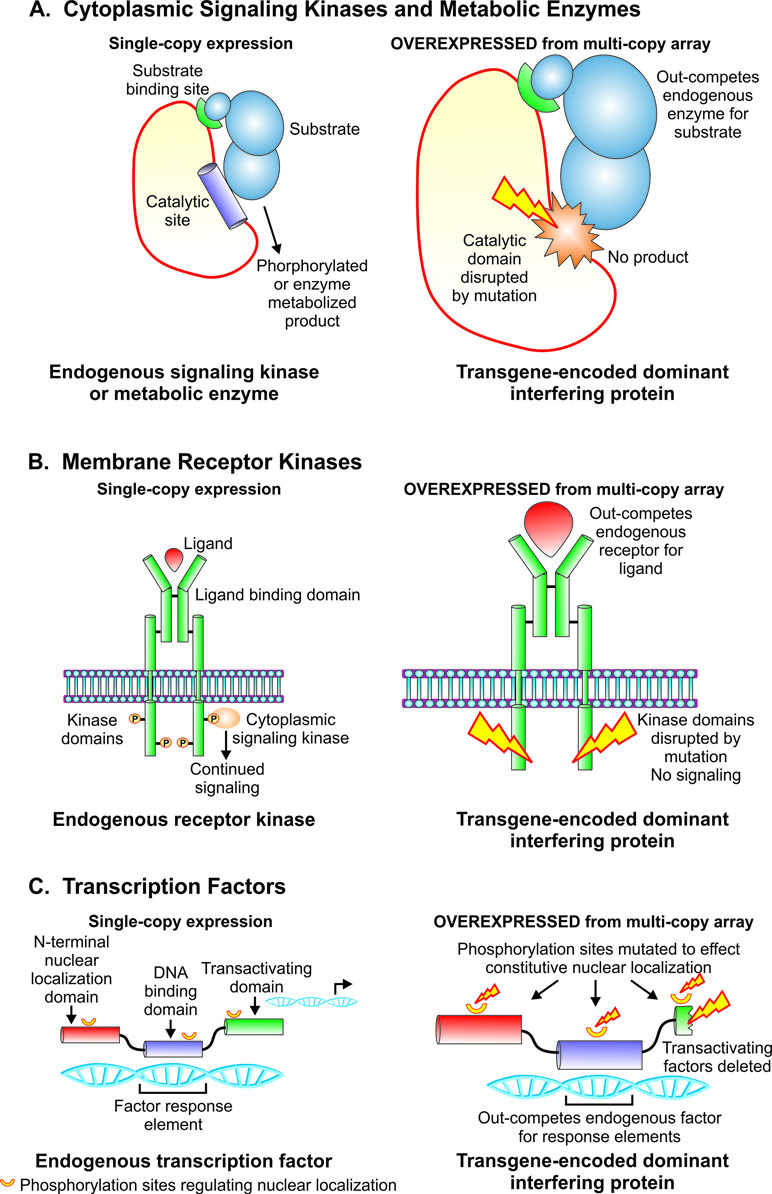
Figure 2 Strategies for creating dominant interfering transgene constructs to study functions of catalytic, signaling, and gene regulatory proteins. (A). Strategy for dominant interfering transgenes targeting functions of metabolic enzymes and cytoplasmic signaling kinases. Overexpression of mutant construct with intact substrate binding domain and catalytic site disrupted by mutation serves to outcompete endogenous gene product for substrate while yielding no phosphorylated or enzyme metabolized product. (B). Design of dominant interfering transgenes targeting functions of membrane receptor kinases. Ablation of the kinase domain by mutation, an intact ligand binding domain and overexpression combine to out-compete the endogenous receptor kinase for ligand while failing to phosphorylate downstream cytoplasmic signaling elements. (C). Design of dominant interfering transgenes targeting the action of transcription factors. Overexpression of a mutant transgene encoding an intact DNA binding domain, mutations in phosphorylation sites to effect constitutive nuclear localization, and disruption of the transactivating domain serve to outcompete the endogenous transcription factor for genomic response elements while failing to execute gene regulatory function.
A Dominant Transgene Approach has been Used to Investigate the Function of Ss-DAF-16 in S. Stercoralis
The gene encoding this transcription factor is the ortholog of daf-16 in C. elegans, which encodes a forkhead transcription factor that is regulated by insulin-like signaling and controls lifespan and the switch between dauer and continuous larval development (Lin et al., 1997; Ogg et al., 1997; Lee et al., 2001; Massey et al., 2003; Massey et al., 2006). A loss-of-function approach to interrogating the function of Ss-daf-16 employed a dominant interfering construct. In this construct, AKT phosphorylation sites necessary for cytoplasmic localization of the protein were disrupted and the C-terminal domain of the molecule was truncated sufficiently to delete predicted transactivator binding sites (Figure 2C). Sequence encoding the DNA-binding domain of the mutant Ss-DAF-16 was left intact. The putative dominant interfering transgene also fused the coding sequence of the mutant Ss-daf-16 with that of gfp to confirm constitutive nuclear localization of the encoded protein (Castelletto et al., 2009). This construct, therefore, was designed to express a mutant form of Ss-DAF-16 that is constitutively localized to the nucleus, but lacks the transactivating functions of the wild-type transcription factor. We also assume that the mutant transgene, like other plasmid-encoded transgenes introduced to nematodes by gonadal microinjection, will be incorporated into a multi-copy episomal array (Mello et al., 1991) and therefore overexpressed relative to the endogenous single-copy target gene (Figure 2C). Indeed, a proportion of F1 progeny of free-living female S. stercoralis microinjected with the mutant Ss-daf-16 construct exhibited pronounced nuclear localization of GFP and a range of phenotypes including severe defects in intestinal morphology, loss of secretory granules from intestinal cells, retention of rhabditiform pharyngeal morphology in third-stage larvae (L3), and initiation of an aberrant L3–L4 molt (Castelletto et al., 2009). These last two phenotypes are particularly noteworthy given that 100% of wild-type progeny of free-living S. stercoralis females arrest their development as infective third-stage larvae, which have filariform, as opposed to rhabditiform morphology (Schad, 1989; Viney and Lok, 2015). In contrast to parasites expressing the putative dominant interfering construct, F1 progeny transformed with a control construct fusing the wild-type Ss-daf-16 coding sequence to gfp exhibited normal intestinal architecture, with intestinal cells replete with secretory granules, filariform pharyngeal morphology in L3, and no evidence of L3–L4 molting (Castelletto et al., 2009).
A Dominant Interfering Transgene Construct was Used to Assess the Function of Ss-riok-1 in S. Stercoralis
Ss-riok-1 encodes a RIO protein kinase homologous to RIOK-1 in C. elegans (Yuan et al., 2014b). RIO kinases are conserved in eukaryotic organisms and are essential for ribosomal biogenesis and cell cycle progression (Angermayr et al., 2002; Widmann et al., 2012; Yuan et al., 2014b). The putative dominant interfering construct encoded Ss-riok-1 with a D282A mutation in its catalytic site that served to disrupt kinase activity. Sequence encoding the substrate binding site of Ss-riok-1 was left intact in the putative dominant interfering construct (Figure 2A). As with the Ss-daf-16 constructs described above, both the wild-type and mutant constructs encoding Ss-RIOK-1 fused its coding sequence to that of gfp to confirm an authentic pattern of expression under the Ss-riok-1 promoter. As expected, both mutant and wild-type constructs were expressed in head and tail neurons and hypodermal cells of transgenic S. stercoralis larvae (Yuan et al., 2014b; Yuan et al., 2017). It was also expected that, by virtue of its situation in a multi-copy episomal array, the mutant protein expressed from the dominant transgene construct would outcompete endogenous single-copy Ss-RIOK-1 for substrate binding but fail to exert its kinase function (Figure 2A). Larvae transformed with the construct encoding the kinase dead Ss-RIOK-1 exhibited profound decrements in motility and a significant blockade in development to the infective third-stage. By contrast, S. stercoralis larvae expressing a control construct encoding a fusion of Ss-RIOK-1 and GFP exhibited typical progressive motility and developed normally to the iL3 (Yuan et al., 2017). The study of Ss-riok-1 function included an additional and crucial control experiment in which co-expression of wild-type Ss-RIOK-1 with the kinase-dead mutant served to rescue the motility and developmental phenotypes to a degree roughly proportional to concentration of wild-type vector plasmid microinjected into parental free-living female worms (Yuan et al., 2017). This important control experiment served to confirm the specificity of the dominant interfering transgene’s effects on Ss-RIOK-1 function.
Future Directions in the Use of Dominant Transgene Constructs to Study Gene Function in Parasitic Nematodes
Controlling Experiments Involving Dominant Interfering Constructs
Both studies employing dominant interfering constructs to evaluate specific gene function in S. stercoralis (Castelletto et al., 2009; Yuan et al., 2017) controlled for confounding effects of transgene overexpression by comparing phenotypes in cohorts of parasites overexpressing fusions of wild-type target proteins fused to GFP to those exhibited by parasites expressing mutant ones. This is crucial given the possibility that overexpression alone can, in some instances, produce dominant effects in transgenic organisms (Chandler and Werr, 2003). Another crucial control introduced in the study of Ss-RIOK-1 function was rescue of dominant interfering phenotypes by co-overexpression of the wild-type protein. In this case, the dose dependency of rescue was established by assessing frequencies of mutant phenotypes in F1 larvae derived from parental females microinjected with the mutant construct along with increasing concentrations of vector plasmid encoding the wild-type protein fused to GFP. There was a decreasing trend in the frequency of phenotypes with increasing concentration of wild-type vector plasmid, confirming the specificity of the mutant constructs dominant effect (Castelletto et al., 2009; Yuan et al., 2017). Inclusion of both of these essential controls will be crucial to the correct interpretation of data in future experiments with dominant interfering transgene constructs.
Deployment of Dominant Transgenes Incorporating Heterologous Activator or Suppressor Domains
To date, dominant interfering effects have been achieved with transgenes encoding the target gene with synthetic loss-of-function mutations in functional domains and wild-type sequence in DNA, protein, or substrate binding sites to facilitate competition for binding partners with the product of the endogenous target gene (Figure 2). Another approach to creating dominant interfering constructs targeting transcription factors in parasitic nematodes is to design the constructs to encode a chimeric protein comprising a heterologous transcriptional repressor domain fused to the DNA binding domain of the target factor. This serves to direct a strong repressor to promoters of genes regulated by the target transcription factor. An example of this approach is the use of the Drosophila ENGRAILED homeodomain to impart repressor function to the transcription factors, thereby creating loss-of-function phenotypes in both invertebrate and mammalian models (Badiani et al., 1994; John et al., 1995; Tolkunova et al., 1998; Chandler and Werr, 2003). Conversely, transgene constructs comprising viral activator domains such as VP16 from herpes simplex virus can activate target genes when fused to GAL-4 (Sevin-Pujol et al., 2017). The specific gene targeting capabilities of nucleoproteins comprising an inactive form of the Cas9 endonuclease, dCas9, and gRNAs can also be harnessed to tether repressor domains such as KRAB or activators such as VP64 to precise genome loci to achieve transcriptional repression (CRISPRi) or activation (CRISPRa) of target genes (Dominguez et al., 2016).
Conclusions
Transgenesis in Strongyloides and related parasitic nematodes in phylogenetic Clade IV and in the filaria Brugia malayi within Clade III opens possibilities for unprecedented studies of gene function in these pathogens that degrade the health of hundreds of millions of people. These approaches have enabled experiments employing dominant interfering transgenes to reveal functions of genes essential to ribosomal biogenesis and to morphogenesis and developmental arrest of infective larvae in Strongyloides stercoralis. Moreover, transgenesis has recently supported proof of principle for targeted mutagenesis via CRISPR/Cas9 in Strongyloides spp. Recent successes with integrative transgenesis in Brugia malayi create the possibility that similar studies of gene function will be possible in the filariae. Recent work with Brugia (Liu et al., 2018) and Strongyloides (Adams et al., 2019) illustrate the potential of lipofectant-mediated gene transfer, alone or in combination with microinjection, to facilitate applications of transgenesis and CRISPR/Cas9 gene disruption and editing to functional genomic study of a wide range of obligately parasitic nematodes with vector borne and free-living pre-parasitic larvae.
Key Concepts
Gonadal microinjection refers to a method of gene transfer whereby solutions of plasmid or linear transgene constructs are injected into the germlines of parental male or female worms. Nematode gonads frequently have syncytial regions that comprise germ cell nuclei within a common cytoplasm. Infusion of transgene construct solutions into these gonadal syncytia results in significant numbers of transgenic oocytes or sperm.
Chemically mediated gene transfer, in the present context, involves gene transfer across the cuticles or exposed cellular surfaces of nematodes facilitated by incorporating transgene DNA into calcium phosphate co-precipitates or lipofectant micelles. Presenting these DNA preparations during intermolt periods of developing larvae, presumably when nascent cuticles are competent to take them up, may be crucial to this process.
Regulatable transgene expression is a strategy that allows transgene expression to be activated by the experimenter. This may be accomplished by using promoters that respond by activation to small molecules such as tetracycline or by coupling transgene encoded proteins of interest to degradation domains that are stabilized in the presence of some small molecule and degraded in the proteasome in its absence.
CRISPR/Cas9. Based on a prokaryotic defense response to foreign DNA, CRISPR/Cas9 harnesses sequence-specific targeting of the Cas9 endonuclease to precise gene loci by short “guide RNAs” to induce double-stranded DNA breaks. These may be repaired by non-homologous end joining, leaving random insertions or deletions, or by homology-directed repair, which can serve to incorporate a synthetic DNA repair template.
Dominant interfering transgenes encode mutations that ablate functional domains of a recombinant protein of interest, but leave crucial protein- or DNA-binding domains intact. When overexpressed, products of these dominant interfering transgenes outcompete the endogenous wild-type gene product for binding partners but fail to execute their designated functions, creating a loss-of-function phenotype in the subject.
Author Contributions
JL formulated the concepts in the review, searched and analyzed the articles referenced, wrote all drafts of the text and designed and drafted the figure, which was then rendered by a graphic artist in the author’s department and who is acknowledged in the paper.
Funding
The author has received support from grants AI50688, AI105856, AI144572, and OD P40-10939 from the US National Institutes of Health and from a grant from the University of Pennsylvania Research Foundation.
Conflict of Interest Statement
The authors declare that the research was conducted in the absence of any commercial or financial relationships that could be construed as a potential conflict of interest.
Acknowledgments
The author is grateful to Drs. Hongguang Shao, Xinshe Li, and Tegegn Jaleta for helpful discussions and to Ms. Deborah Argento for assistance in preparing this paper.
References
Adams, S., Pathak, P., Shao, H., Lok, J. B., Pires-daSilva, A. (2019). Liposome-based transfection enhances RNAi and CRISPR-mediated mutagenesis in non-model nematode systems. Sci. Rep. 9, 483. doi: 10.1038/s41598-018-37036-1
Angermayr, M., Roidl, A., Bandlow, W. (2002). Yeast Rio1p is the founding member of a novel subfamily of protein serine kinases involved in the control of cell cycle progression. Mol. Microbiol. 44, 309–324. doi: 10.1046/j.1365-2958.2002.02881.x
Badiani, P., Corbella, P., Kioussis, D., Marvel, J., Weston, K. (1994). Dominant interfering alleles define a role for c-Myb in T-cell development. Genes Dev. 8, 770–782. doi: 10.1101/gad.8.7.770
Bailey, M., Chauhan, C., Liu, C., Unnasch, T. R. (2011). The role of polymorphisms in the spliced leader addition domain in determining promoter activity in Brugia malayi. Mol Biochem. Parasitol. 176, 37–41. doi: 10.1016/j.molbiopara.2010.11.012
Bargmann, C. I., Mori, I. (1997). “Chemotaxis and Thermotaxis,” in C. elegans II. Eds. Riddle, D. L., Blumenthal, T., Meyer, B. J., Priess, J. R. (Plainview, NY: Cold Spring Harbor Laboratory Press), 717–737.
Bouchery, T., Volpe, B., Shah, K., Lebon, L., Filbey, K., LeGros, G., et al. (2017). The study of host immune responses elicited by the model murine hookworms Nippostrongylus brasiliensis and Heligmosomoides polygyrus. Curr. Protoc. Mouse Biol. 7, 236–286. doi: 10.1002/cpmo.34
Bryant, A. S., Hallem, E. A. (2018a). Temperature-dependent behaviors of parasitic helminths. Neurosci. Lett. 687, 290–303. doi: 10.1016/j.neulet.2018.10.023
Bryant, A. S., Hallem, E. A. (2018b). Terror in the dirt: sensory determinants of host seeking in soil-transmitted mammalian-parasitic nematodes. Int. J. Parasitol. Drugs Drug. Resist. 8, 496–510. doi: 10.1016/j.ijpddr.2018.10.008
Bryant, A. S., Ruiz, F., Gang, S. S., Castelletto, M. L., Lopez, J. B., Hallem, E. A. (2018). A critical role for thermosensation in host seeking by skin-penetrating nematodes. Curr. Biol. 28 (14), 2338–2347.e6. doi: 10.1016/j.cub.2018.05.063
Castelletto, M. L., Gang, S. S., Okubo, R. P., Tselikova, A. A., Nolan, T. J., Platzer, E. G., et al. (2014). Diverse host-seeking behaviors of skin-penetrating nematodes. PLoS Pathog. 10, e1004305. doi: 10.1371/journal.ppat.1004305
Castelletto, M. L., Massey, H. C., Jr., Lok, J. B. (2009). Morphogenesis of Strongyloides stercoralis infective larvae requires the DAF-16 ortholog FKTF-1. PLoS Pathog. 5, e1000370. doi: 10.1371/journal.ppat.1000370
Chandler, J. W., Werr, W. (2003). When negative is positive in functional genomics. Trends Plant Sci. 8, 279–285. doi: 10.1016/S1360-1385(03)00101-8
Davis, R. E., Parra, A., LoVerde, P. T., Ribeiro, E., Glorioso, G., Hodgson, S. (1999). Transient expression of DNA and RNA in parasitic helminths by using particle bombardment. Proc. Natl. Acad. Sci. U.S.A. 96, 8687–8692. doi: 10.1073/pnas.96.15.8687
de Oliveira, A., Katholi, C. R., Unnasch, T. R. (2008). Characterization of the promoter of the Brugia malayi 12kDa small subunit ribosomal protein (RPS12) gene. Int. J. Parasitol. 38, 1111–1119. doi: 10.1016/j.ijpara.2008.02.002
Dominguez, A. A., Lim, W. A., Qi, L. S. (2016). Beyond editing: repurposing CRISPR-Cas9 for precision genome regulation and interrogation. Nat. Rev. Mol. Cell Biol. 17, 5–15. doi: 10.1038/nrm.2015.2
Fire, A. (1986). Integrative transformation of Caenorhabditis elegans. Embo J. 5, 2673–2680. doi: 10.1002/j.1460-2075.1986.tb04550.x
Fire, A., Harrison, S. W., Dixon, D. (1990a). A modular set of lacZ fusion vectors for studying gene expression in Caenorhabditis elegans. Gene 93, 189–198. doi: 10.1016/0378-1119(90)90224-F
Fire, A., Kondo, K., Waterston, R. (1990b). Vectors for low copy transformation of C. elegans. Nucleic. Acids Res. 18, 4269–4270. doi: 10.1093/nar/18.14.4269
Gang, S. S., Castelletto, M. L., Bryant, A. S., Yang, E., Mancuso, N., Lopez, J. B., et al. (2017). Targeted mutagenesis in a human-parasitic nematode. PLoS Pathog. 13, e1006675. doi: 10.1371/journal.ppat.1006675
Grant, W. N., Skinner, S. J., Newton-Howes, J., Grant, K., Shuttleworth, G., Heath, D. D., et al. (2006a). Heritable transgenesis of Parastrongyloides trichosuri: a nematode parasite of mammals. Int. J. Parasitol. 36, 475–483. doi: 10.1016/j.ijpara.2005.12.002
Grant, W. N., Stasiuk, S., Newton-Howes, J., Ralston, M., Bisset, S. A., Heath, D. D., et al. (2006b). Parastrongyloides trichosuri, a nematode parasite of mammals that is uniquely suited to genetic analysis. Int. J. Parasitol. 36, 453–466. doi: 10.1016/j.ijpara.2005.11.009
Higazi, T. B., Unnasch, T. R. (2004). Intron encoded sequences necessary for trans splicing in transiently transfected Brugia malayi. Mol. Biochem. Parasitol. 137, 181–184. doi: 10.1016/j.molbiopara.2004.04.014
Higazi, T. B., Unnasch, T. R. (2013). Biolistic transformation of Brugia malayi. Methods Mol. Biol. 940, 103–115. doi: 10.1007/978-1-62703-110-3_9
Higazi, T. B., Merriweather, A., Shu, L., Davis, R., Unnasch, T. R. (2002). Brugia malayi: transient transfection by microinjection and particle bombardment. Exp. Parasitol. 100, 95–102. doi: 10.1016/S0014-4894(02)00004-8
Higazi, T. B., Deoliveira, A., Katholi, C. R., Shu, L., Barchue, J., Lisanby, M., et al. (2005). Identification of elements essential for transcription in Brugia malayi promoters. J. Mol. Biol. 353, 1–13. doi: 10.1016/j.jmb.2005.08.014
Jackstadt, P., Wilm, T. P., Zahner, H., Hobom, G. (1999). Transformation of nematodes via ballistic DNA transfer. Mol. Biochem. Parasitol. 103, 261–266. doi: 10.1016/S0166-6851(99)00089-4
John, A., Smith, S. T., Jaynes, J. B. (1995). Inserting the Ftz homeodomain into engrailed creates a dominant transcriptional repressor that specifically turns off Ftz target genes in vivo. Development 121, 1801–1813.
Junio, A. B., Li, X., Massey, H. C., Jr., Nolan, T. J., Todd Lamitina, S., Sundaram, M. V., et al. (2008). Strongyloides stercoralis: cell- and tissue-specific transgene expression and co-transformation with vector constructs incorporating a common multifunctional 3’ UTR. Exp. Parasitol. 118, 253–265. doi: 10.1016/j.exppara.2007.08.018
Komatsu, H., Jin, Y. H., L’Etoile, N., Mori, I., Bargmann, C. I., Akaike, N., et al. (1999). Functional reconstitution of a heteromeric cyclic nucleotide-gated channel of Caenorhabditis elegans in cultured cells. Brain. Res. 821, 160–168. doi: 10.1016/S0006-8993(99)01111-7
Komatsu, H., Mori, I., Rhee, J. S., Akaike, N., Ohshima, Y. (1996). Mutations in a cyclic nucleotide-gated channel lead to abnormal thermosensation and chemosensation in C. elegans. Neuron 17, 707–718. doi: 10.1016/S0896-6273(00)80202-0
Lee, R. Y., Hench, J., Ruvkun, G. (2001). Regulation of C. elegans DAF-16 and its human ortholog FKHRL1 by the daf-2 insulin-like signaling pathway. Curr. Biol. 11, 1950–1957. doi: 10.1016/S0960-9822(01)00595-4
Lei, W. Q., Lok, J. B., Yuan, W., Zhang, Y. Z., Stoltzfus, J. D., Gasser, R. B., et al. (2017). Structural and developmental expression of Ss-riok-2, an RIO protein kinase encoding gene of Strongyloides stercoralis. Sci. Rep. 7, 8693. doi: 10.1038/s41598-017-07991-2
Li, X., Massey, H. C., Jr., Nolan, T. J., Schad, G. A., Kraus, K., Sundaram, M., et al. (2006). Successful transgenesis of the parasitic nematode Strongyloides stercoralis requires endogenous non-coding control elements. Int. J. Parasitol. 36, 671–679. doi: 10.1016/j.ijpara.2005.12.007
Li, X., Shao, H., Junio, A., Nolan, T. J., Massey, H. C., Jr., Pearce, E. J., et al. (2011). Transgenesis in the parasitic nematode Strongyloides ratti. Mol. Biochem. Parasitol. 179, 114–119. doi: 10.1016/j.molbiopara.2011.06.002
Lin, K., Dorman, J. B., Rodan, A., Kenyon, C. (1997). daf-16: an HNF-3/forkhead family member that can function to double the life-span of Caenorhabditis elegans.. Science 278, 1319–1322. doi: 10.1126/science.278.5341.1319
Liu, C., Chauhan, C., Unnasch, T. R. (2010a). The role of local secondary structure in the function of the trans-splicing motif of Brugia malayi. Mol. Biochem. Parasitol. 169, 115–119. doi: 10.1016/j.molbiopara.2009.10.003
Liu, C., Enright, T., Tzertzinis, G., Unnasch, T. R. (2012). Identification of genes containing ecdysone response elements in the genome of Brugia malayi. Mol. Biochem. Parasitol. 186, 38–43. doi: 10.1016/j.molbiopara.2012.09.005
Liu, C., Kelen, P. V., Ghedin, E., Lustigman, S., Unnasch, T. R. (2011). Analysis of transcriptional regulation of tetracycline responsive genes in Brugia malayi. Mol. Biochem. Parasitol. 180, 106–111. doi: 10.1016/j.molbiopara.2011.09.004
Liu, C., de Oliveira, A., Higazi, T. B., Ghedin, E., DePasse, J., Unnasch, T. R. (2007). Sequences necessary for trans-splicing in transiently transfected Brugia malayi. Mol. Biochem. Parasitol. 156, 62–73. doi: 10.1016/j.molbiopara.2007.07.012
Liu, C., Oliveira, A., Chauhan, C., Ghedin, E., Unnasch, T. R. (2010b). Functional analysis of putative operons in Brugia malayi. Int. J. Parasitol. 40, 63–71. doi: 10.1016/j.ijpara.2009.07.001
Liu, C., Mhashilkar, A. S., Chabanon, J., Xu, S., Lustigman, S., Adams, J. H., et al. (2018). Development of a toolkit for piggyBac-mediated integrative transfection of the human filarial parasite Brugia malayi. PLoS Negl. Trop. Dis. 12, e0006509. doi: 10.1371/journal.pntd.0006509
Liu, C., Voronin, D., Poole, C. B., Bachu, S., Rogers, M. B., Jin, J., et al. (2015). Functional analysis of microRNA activity in Brugia malayi. Int. J. Parasitol. 45, 579–583. doi: 10.1016/j.ijpara.2015.04.004
Lok, J. B. (2013). piggyBac: a vehicle for integrative DNA transformation of parasitic nematodes. Mobile Genet. Elem. 3, e24417. doi: 10.4161/mge.24417
Lok, J. B., Massey, H. C., Jr. (2002). Transgene expression in Strongyloides stercoralis following gonadal microinjection of DNA constructs. Mol Biochem. Parasitol. 119, 279–284. doi: 10.1016/S0166-6851(01)00414-5
Lok, J. B., Unnasch, T. R. (2013). “Transgenesis in animal parasitic nematodes: Strongyloides spp. and Brugia spp,” in WormBook, ed. The C. elegans Research Community (WormBook), 1–18. doi: 10.1895/wormbook.1.163.1
Lok, J. B., Shao, H., Massey, H. C., Li, X. (2017). Transgenesis in Strongyloides and related parasitic nematodes: historical perspectives, current functional genomic applications and progress towards gene disruption and editing. Parasitology 144, 327–342. doi: 10.1017/S0031182016000391
Massey, H. C., Jr., Ranjit, N., Stoltzfus, J. D., Lok, J. B. (2013). Strongyloides stercoralis daf-2 encodes a divergent ortholog of Caenorhabditis elegans DAF-2. Int. J. Parasitol. 43, 515–520. doi: 10.1016/j.ijpara.2013.01.008
Massey, H. C., Bhopale, M. K., Li, X., Castelletto, M., Lok, J. B. (2006). The fork head transcription factor FKTF-1b from Strongyloides stercoralis restores DAF-16 developmental function to mutant Caenorhabditis elegans. Int. J. Parasitol. 36, 347–352. doi: 10.1016/j.ijpara.2005.11.007
Massey, H. C., Jr., Nishi, M., Chaudhary, K., Pakpour, N., Lok, J. B. (2003). Structure and developmental expression of Strongyloides stercoralis fktf-1, a proposed ortholog of daf-16 in Caenorhabditis elegans. Int. J. Parasitol. 33, 1537–1544. doi: 10.1016/S0020-7519(03)00205-4
Mello, C., Fire, A. (1995). DNA transformation. Methods Cell Biol. 48, 451–482. doi: 10.1016/S0091-679X(08)61399-0
Mello, C. C., Kramer, J. M., Stinchcomb, D., Ambros, V. (1991). Efficient gene transfer in C. elegans: extrachromosomal maintenance and integration of transforming sequences. Embo J. 10, 3959–3970. doi: 10.1002/j.1460-2075.1991.tb04966.x
Moerman, D. G., Baillie, D. L. (1979). Genetic organization in Caenorhabditis elegans: fine-structure analysis of the unc-22 gene. Genetics 91, 95–103.
Moerman, D. G., Benian, G. M., Barstead, R. J., Schriefer, L. A., Waterston, R. H. (1988). Identification and intracellular localization of the unc-22 gene product of Caenorhabditis elegans. Genes Dev. 2, 93–105. doi: 10.1101/gad.2.1.93
Muralidharan, V., Oksman, A., Iwamoto, M., Wandless, T. J., Goldberg, D. E. (2011). Asparagine repeat function in a Plasmodium falciparum protein assessed via a regulatable fluorescent affinity tag. Proc. Natl. Acad. Sci. U.S.A. 108, 4411–4416. doi: 10.1073/pnas.1018449108
Nolan, T. J., Megyeri, Z., Bhopale, V. M., Schad, G. A. (1993). Strongyloides stercoralis: the first rodent model for uncomplicated and hyperinfective strongyloidiasis, the Mongolian gerbil (Meriones unguiculatus). J. Infect. Dis. 168, 1479–1484. doi: 10.1093/infdis/168.6.1479
Ogg, S., Paradis, S., Gottlieb, S., Patterson, G. I., Lee, L., Tissenbaum, H. A., et al. (1997). The fork head transcription factor DAF-16 transduces insulin-like metabolic and longevity signals in C. elegans. Nature 389, 994–999. doi: 10.1038/40194
Schad, G. A. (1989). “Morphology and life history of Strongyloides stercoralis,” in Strongyloidiasis: a major roundworm infection of man. Ed. Grove, D. I. (Philadelphia: Taylor & Francis Inc.), 85–104.
Sevin-Pujol, A., Sicard, M., Rosenberg, C., Auriac, M. C., Lepage, A., Niebel, A., et al. (2017). Development of a GAL4-VP16/UAS trans-activation system for tissue specific expression in Medicago truncatula. PLoS One 12, e0188923. doi: 10.1371/journal.pone.0188923
Shao, H., Li, X., Lok, J. B. (2017). Heritable genetic transformation of Strongyloides stercoralis by microinjection of plasmid DNA constructs into the male germline. Int. J. Parasitol. 9, 511–515. doi: 10.1016/j.ijpara.2017.04.003
Shao, H., Li, X., Nolan, T. J., Massey, H. C., Jr., Pearce, E. J., Lok, J. B. (2012). Transposon-mediated chromosomal integration of transgenes in the parasitic nematode Strongyloides ratti and establishment of stable transgenic lines. PLoS Pathog. 8, e1002871. doi: 10.1371/journal.ppat.1002871
Shu, L., Katholi, C. R., Higazi, T., Unnasch, T. R. (2003). Analysis of the Brugia malayi HSP70 promoter using a homologous transient transfection system. Mol Biochem. Parasitol. 128, 67–75. doi: 10.1016/S0166-6851(03)00052-5
Stoltzfus, J. D., Massey, H. C., Jr., Nolan, T. J., Griffith, S. D., Lok, J. B. (2012). Strongyloides stercoralis age-1: a potential regulator of infective larval development in a parasitic nematode. PLoS One 7, e38587. doi: 10.1371/journal.pone.0038587
Tolkunova, E. N., Fujioka, M., Kobayashi, M., Deka, D., Jaynes, J. B. (1998). Two distinct types of repression domain in engrailed: one interacts with the groucho corepressor and is preferentially active on integrated target genes. Mol. Cell Biol. 18, 2804–2814. doi: 10.1128/MCB.18.5.2804
Tzertzinis, G., Egana, A. L., Palli, S. R., Robinson-Rechavi, M., Gissendanner, C. R., Liu, C., et al. (2010). Molecular evidence for a functional ecdysone signaling system in Brugia malayi. PLoS Negl. Trop. Dis. 4, e625. doi: 10.1371/journal.pntd.0000625
Viney, M. E., Lok, J. B. (2015). “The biology of Strongyloides spp.,” in WormBook, ed. The C. elegans Research Community (WormBook), 1–17. doi: 10.1895/wormbook.1.141.2
Waaijers, S., Portegijs, V., Kerver, J., Lemmens, B. B., Tijsterman, M., van den Heuvel, S., et al. (2013). CRISPR/Cas9-targeted mutagenesis in Caenorhabditis elegans. Genetics 195, 1187–1191. doi: 10.1534/genetics.113.156299
Widmann, B., Wandrey, F., Badertscher, L., Wyler, E., Pfannstiel, J., Zemp, I., et al. (2012). The kinase activity of human Rio1 is required for final steps of cytoplasmic maturation of 40S subunits. Mol. Biol. Cell 23, 22–35. doi: 10.1091/mbc.e11-07-0639
Wurmthaler, L. A., Sack, M., Gense, K., Hartig, J. S., Gamerdinger, M. (2019). A tetracycline-dependent ribozyme switch allows conditional induction of gene expression in Caenorhabditis elegans. Nat. Commun. 10, 491. doi: 10.1038/s41467-019-08412-w
Xu, S., Liu, C., Tzertzinis, G., Ghedin, E., Evans, C. C., Kaplan, R., et al. (2011). In vivo transfection of developmentally competent Brugia malayi infective larvae. Int. J. Parasitol. 41, 355–362. doi: 10.1016/j.ijpara.2010.10.005
Yuan, W., Liu, Y., Lok, J. B., Stoltzfus, J. D., Gasser, R. B., Lei, W., et al. (2014a). Exploring features and function of Ss-riok-3, an enigmatic kinase gene from Strongyloides stercoralis. Parasit. Vectors 7, 561. doi: 10.1186/PREACCEPT-1041797881140939
Yuan, W., Lok, J. B., Stoltzfus, J. D., Gasser, R. B., Fang, F., Lei, W. Q., et al. (2014b). Toward understanding the functional role of Ss-RIOK-1, a RIO protein kinase-encoding gene of Strongyloides stercoralis. PLoS Negl. Trop. Dis. 8, e3062. doi: 10.1371/journal.pntd.0003062
Keywords: transgenesis, parasitic nematode, CRISPR/Cas9, dominant transgene, mutagenesis
Citation: Lok JB (2019) CRISPR/Cas9 Mutagenesis and Expression of Dominant Mutant Transgenes as Functional Genomic Approaches in Parasitic Nematodes. Front. Genet. 10:656. doi: 10.3389/fgene.2019.00656
Received: 28 March 2019; Accepted: 21 June 2019;
Published: 16 July 2019.
Edited by:
Makedonka Mitreva, Washington University Medical Center, United StatesReviewed by:
Anil Kumar Challa, University of Alabama at Birmingham, United StatesRui Chen, Baylor College of Medicine, United States
Copyright © 2019 Lok. This is an open-access article distributed under the terms of the Creative Commons Attribution License (CC BY). The use, distribution or reproduction in other forums is permitted, provided the original author(s) and the copyright owner(s) are credited and that the original publication in this journal is cited, in accordance with accepted academic practice. No use, distribution or reproduction is permitted which does not comply with these terms.
*Correspondence: James B. Lok, jlok@vet.upenn.edu