Functional diversity of shredders, not species richness, drives the decomposition rate of leaf litter in ponds
- 1Department of Biology and General Ecology, Faculty of Ecology and Environmental Sciences, Technical University in Zvolen, Zvolen, Slovakia
- 2Institute of Botany, Plant Science and Biodiversity Center, Slovak Academy of Sciences, Bratislava, Slovakia
- 3Department of Forest Ecology, Faculty of Forestry and Wood Sciences, Czech University of Life Sciences Prague, Prague, Czechia
Leaf litter decomposition is a critical ecosystem-level process in many freshwater habitats. Although ponds are likely to derive a large proportion of their energy from riparian vegetation, allochthonous organic matter decomposition in these water bodies has received little attention. We studied the breakdown rates of black alder (Alnus glutinosa (L.) Gaertn.) litter in ponds and provide the first evidence of the role of the taxonomic and functional diversity of pond-dwelling shredders in this ecosystem process. Despite a strong connection to riparian zones, the litter breakdown rates observed in ponds were generally lower than those reported in headwater streams. It seems that ponds provide less favorable conditions for shredder communities than headwaters. The rate of organic matter decomposition in ponds was significantly positively related to functional diversity, represented by the variability of shredder body size, while shredder species richness did not appear to be a reliable proxy for this ecosystem function. This finding is consistent with theoretical predictions that functional complementarity among species has a systematic effect on ecosystem processes. It also emphasizes that body size is a crucial functional trait mediating the effects of shredder diversity on litter decomposition in ponds.
1 Introduction
Energetic fluxes from terrestrial to aquatic environments are key topics in aquatic ecology. The input of allochthonous organic matter, mainly leaf litter from riparian vegetation, can influence the chemical and physical properties of aquatic ecosystems in a myriad of ways (Webster and Benfield, 1986; Prairie, 2008; Tank et al., 2010). Importantly, allochthonous detritus input has been shown to support secondary production and the flux of energy through food webs in many aquatic ecosystems (Marcarelli et al., 2011; Brett et al., 2017).
When leaf litter enters an aquatic environment, the breakdown of this organic matter is a critical ecosystem-level process involving chemical leaching, physical abrasion, microbial activity, fragmentation and consumption by shredding macroinvertebrates (Gessner et al., 1999; Benfield et al., 2017). The rate at which organic matter is processed can have a profound effect on ecosystem function and biodiversity in aquatic systems (Gessner et al., 1999; Westlake et al., 2009; Brett et al., 2017). The leaf litter breakdown rate is affected by both internal factors (i.e., chemical and physical characteristics of the leaves themselves) and external conditions (i.e., habitat quality and biotic communities). Water temperature, pH, nutrients and dissolved oxygen concentration usually play an important role in this process (Webster and Benfield, 1986; Pozo, 1993; Royer and Minshall, 2003). Among biotic variables, macroinvertebrate communities significantly affect the breakdown rate (Anderson and Sedell, 1979; Hieber and Gessner, 2002). The abundance and diversity of benthic invertebrates feeding preferentially on coarse particulate organic detritus (i.e., shredders) have been shown to correlate with leaf litter breakdown rates (Graça et al., 2001; Jonsson et al., 2001). There is a growing recognition, however, that functional diversity, the range of things that organisms do in ecosystems defined through functional traits, rather than taxonomic diversity on its own, strongly determines ecosystem functioning (Hooper et al., 2005; Cornwell et al., 2008). Conceptually, functional diversity provides a tool to directly link biodiversity with ecosystem functioning, and therefore, functional diversity should be more tightly bound with ecosystem-level processes than taxonomic diversity (Tilman, 2001; Petchey and Gaston, 2006; Cadotte et al., 2011). However, relationships between functional diversity and ecological functions are poorly known in freshwaters (Schmera et al., 2017).
The importance of allochthonous litter as an energy source varies among or even within distinct types of aquatic ecosystems, resulting in considerably different intensities of research interest. Allochthonous litter input and its transport from headwater streams are major energetic fluxes along the river continuum (Vannote et al., 1980), and most studies of allochthonous detritus in aquatic ecosystems have been undertaken in lotic systems, such as forested streams (Richardson, 1991; Wallace et al., 1997; Graça et al., 2001). In contrast, lentic ecosystems have traditionally been thought to be driven by autotrophic processes through primary production, especially in pelagic and littoral zones (Doi, 2009). Therefore, considerably fewer studies have been conducted in lakes, wetlands and ponds (Stoler and Relyea, 2020), leading to a limited understanding of the factors influencing leaf litter decomposition in lentic waters. However, recent studies have suggested that terrestrial organic material subsidizes lentic ecosystem metabolism, supports a significant amount of zooplankton, zoobenthos and fish production (Brett et al., 2017), and greatly influences energy transfer and community structure, especially in unproductive lakes (Jansson et al., 2007; Cole et al., 2011).
In contrast to large lakes, whose organic matter budget is made up almost entirely of autochthonous primary production (Carpenter et al., 1998; Gessner, 2000), small lakes and ponds are likely to derive a large proportion of their energy from allochthonous organic matter (Pace et al., 2004; Cole et al., 2011; Stoler and Relyea, 2020) since their forested riparian zones may be disproportionally larger than the waterbody area compared to those in lakes (Figure S1). Moreover, riparian vegetation in small ponds reduces photosynthetic active radiation by heavy shading, which further contributes to lowering the importance of autotrophic primary production. The transition from heavily shaded ponds with food webs predominantly based on detritus (brown food webs) to lakes with food webs based on primary production (green food webs) resembles the river continuum concept predicting such changes from headwater streams towards downstream reaches (Vannote et al., 1980). If the analogy holds further, detritivore aquatic invertebrates (shredders) should play an important role in leaf litter processing in ponds. The importance of gaining a more comprehensive understanding of litter dynamics in ponds is underscored by the fact that ponds play an important role in the global carbon cycle (Holgerson and Raymond, 2016), and their carbon balance can become highly unstable in the face of climate change (Yvon-Durocher et al., 2017).
In this study, we conducted a field experiment to assess the effect of the taxonomic and functional diversity of shredder communities on the rate of black alder (Alnus glutinosa (L.) Gaertn.) leaf litter breakdown in pond ecosystems. Following the theory of biodiversity–ecosystem functioning (Tilman et al., 1997; Tilman, 2001; Hooper et al., 2005), we expected functional diversity to be more tightly linked than taxonomic diversity with the ecosystem process of leaf litter breakdown.
2 Materials and methods
2.1 Studied ponds
We performed a field experiment to assess the role of conceptually different measures of shredder diversity in the process of leaf litter breakdown in eight fishless ponds located in Slovakia, Central Europe (Figure 1). The ponds are mesotrophic to eutrophic and cover a relatively broad altitudinal gradient from lowland to mountain areas (117-870 m). The surface area ranges from 18 to 2,315 m2, and the maximum depth ranges from 0.3 to 1.5 m. For further details on the study site characteristics, see Table 1.
2.2 Leaf litter breakdown experiment
Senescent leaves of black alder, one of the most frequently used litter species in breakdown studies and species frequently found in the riparian zones of Central European ponds, were collected just after abscission in November 2016. The leaves were air-dried in the laboratory (~25°C) until they reached a constant dry mass (~7 days). Portions of 3.0 g (± 0.09 g) of dry leaves were enclosed in litter bags (20 × 20 cm, 5.0 mm mesh size), which were used to measure the breakdown rate (Benfield et al., 2017). In total, 60 litter bags were prepared. The litter bags were placed randomly on the bottom of each pond at the end of June 2017. To prevent their loss, the bags were secured with nylon cords attached to riparian vegetation. Originally, we intended to perform the study in ten ponds. Because two sites experienced complete drying shortly before the start of the experiment, we increased the number of litter bags in two ponds (Dvory nad Žitavou, Kunerád1) to utilize all prepared bags. Data loggers (Minilog II-T; Vemco, Bedford, Nova Scotia, Canada) recording water temperature at hourly intervals were placed in each pond near the litter bags. One or two bags (in ponds with doubled numbers) were randomly selected and retrieved over six sampling dates. Since dry leaves are easily broken during transportation and handling (Benfield et al., 2017) and initial leaching often represents a substantial loss of leaf mass (Gessner et al., 1999), we accounted for these losses by harvesting the first bags 24 hours after installation. These bags were used to correct the initial weights of the leaf litter for mass loss unrelated to the biological decomposition processes. Subsequent collection of the bags was conducted after approximately 25, 70, 110, 145 and 170 days of incubation. The litter bags were placed to plastic zip-lock bags and transported in the fridge to the laboratory for processing. The leaves were removed from the bags, gently rinsed under tap water, oven-dried at 45°C for 24 hours and weighed to the nearest 0.01 mg. To account for possible bias caused by mineral deposits on the leaves, we combusted the weighed leaves in a muffle furnace (550°C for 1 hour) and estimated the ash-free dry mass (AFDM). We corrected the initial weights of leaf litter in the bags using the AFDM estimates of the bags retrieved after 24 hours.
The breakdown rates in each pond were estimated using the breakdown coefficient (k) based on the exponential decay model (Petersen and Cummins, 1974). The coefficients were quantified as the mass loss per degree-day (the cumulative sum of daily mean water temperatures) to correct for temperature differences among sites. We also report the breakdown coefficients calculated as mass loss per day to facilitate comparisons among studies. The breakdown rate coefficients were calculated as slopes of the log-linear models regressing the proportion of AFDM remaining (%) on the degree-days and days (Benfield et al., 2017).
2.3 Benthic invertebrate sampling and processing
Each pond was sampled for invertebrates in summer using a standardized semiquantitative PLOCH method (Oertli et al., 2005). As the first step of the PLOCH sampling, mesohabitats were identified, and their coverage was estimated. Five to eight samples were taken at each site depending on the pond area and mesohabitat diversity. The samples were distributed among the mesohabitats in proportion to their relative coverage, with a minimum of one sample per mesohabitat. The samples were collected by sweeping and kicking the mesohabitats using a net with a rectangular frame of size 15 × 11 cm and 0.5 mm mesh size for a fixed time of 30 seconds. The samples were subsequently pooled into one composite sample per site. All collected material was preserved in 4% formaldehyde in the field. Invertebrates were hand-sorted in the laboratory, preserved in 70% alcohol and identified to the lowest possible taxonomic level. The taxa were classified into functional feeding groups following Šporka (2003) and Schmidt-Kloiber and Hering (2015). Only the data on shredding species were used in the subsequent analyses.
The abundance, measured as the average number of shredders per sample, was determined for each pond. The total number of shredder species per pond (species richness) was used as a measure of taxonomic diversity. Functional diversity was calculated as the total branch length of a dendrogram based on functional traits (Petchey and Gaston, 2006). The functional dendrogram was constructed using the Euclidean distance matrix of z score standardized functional traits and the unweighted pair group method with arithmetic means (UPGMA) (Figure S2A). Since the selection of functionally important traits is a critical step in the calculation of functional diversity (Petchey and Gaston, 2006), we used maximum body size and voltinism (Table S1), as these features are essential in the metabolism, energy allocation and food processing of invertebrates (Gillooly et al., 2001; Woodward et al., 2005; Menezes et al., 2010). Information on species body size was obtained from Freude et al. (1971); Freude et al. (1979); Rozkošný (1980); İncekara et al. (2005); Straka and Sychra (2007); Skuhrovec et al. (2011) and Kriska (2013), and data on voltinism were based on Šporka (2003); Grabowski et al. (2007) and Janz (2017).
2.4 Data analysis
We used ordinary least squares models (OLS) to test the influence of pond environmental characteristics (Table 1) and shredder communities (abundance, species richness, functional diversity) on the breakdown rates of black alder leaf litter. The breakdown rates, pond environmental variables (area, depth, conductivity), and shredder characteristics were log-transformed to satisfy the assumption of normality. However, some of the OLS models showed heteroscedastic residuals which may lead to biased standard error estimates and erroneous conclusions of statistical inference (Zar, 2014). Therefore, we used generalized least squares (GLS) models that are designed to provide unbiased estimates when the assumption of constant error variance is violated (Pinheiro and Bates, 2006). We employed GLS incorporating the varying degrees of heteroscedasticity by assigning different weights to individual observations based on an exponential variance structure. GLS considerably improved the overall model performance, as evidenced by diagnostic plots of residuals. We fitted separate models for each predictor and tested their significance using Wald F tests. The relative importance of the predictors was assessed through (pseudo)determination coefficients (R2) (Nagelkerke, 1991) and standardized (β) coefficients (Schielzeth, 2010).
The analyses were performed in R (R Core Team, 2019) using the packages ggplot2 (Wickham, 2016), nlme (Pinheiro et al., 2019), rcompanion (Mangiafico, 2019) and vegan (Oksanen et al., 2022).
3 Results
The breakdown rates of black alder leaves observed in the studied ponds ranged from 0.0008 to 0.0012 degree-days−1 [uncorrected for temperature: 0.009–0.021 days−1], meaning that 50% of the initial litter AFDM is expected to be processed within 32–75 days (Table S2).
Considering the influence of pond environmental characteristics, we did not find any significant relationship between the measured variables and the rate of black alder leaf litter decomposition (Table 2).
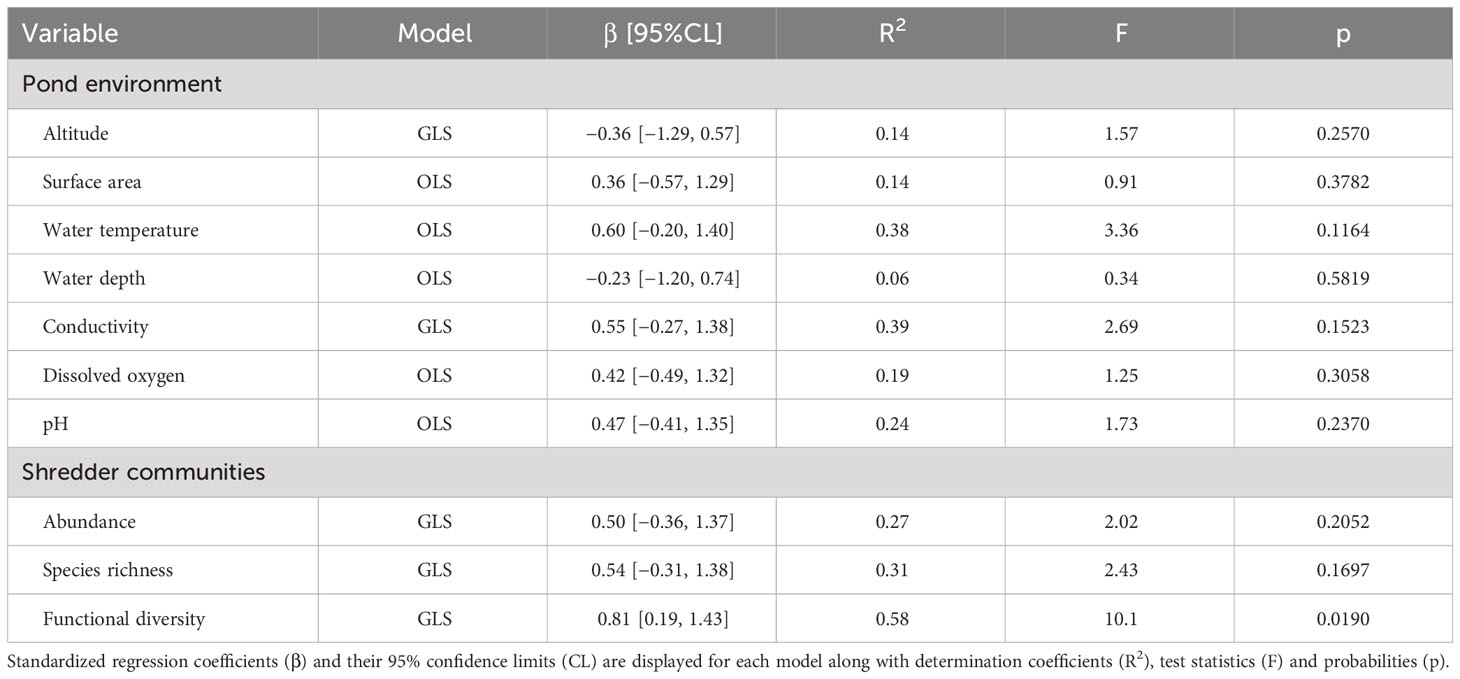
Table 2 Summary of ordinary least squares (OLS) and generalized least squares (GLS) models testing for the effect of environmental characteristics and shredder communities on the breakdown rate of black alder leaf litter in ponds.
Altogether, 30 shredder species from four taxonomic groups (Crustacea, Coleoptera, Trichoptera and Diptera) were identified (Table S1). Shredder abundance ranged from 0.7 to 4.2 individuals, on average, per sample. Species richness varied from 2 to 9 species, and functional diversity varied from 0.96 to 12.8 (Table S3). All characteristics of the shredder communities were positively linked with the black alder breakdown rate (Figure 2). However, GLS models revealed functional diversity as the only significant predictor of the breakdown rate (Table 2). Subsequently, we recalculated functional diversity using single traits only to identify the relative importance of individual traits. While heterogeneity in voltinism emerged as non-significant (GLS: F = 0.50, p = 0.505, R2 = 0.08), variability in body length contributed significantly (GLS: F = 18.31, p = 0.005, R2 = 0.82) to the positive relationship between diversity and ecosystem functioning (Figure S3).
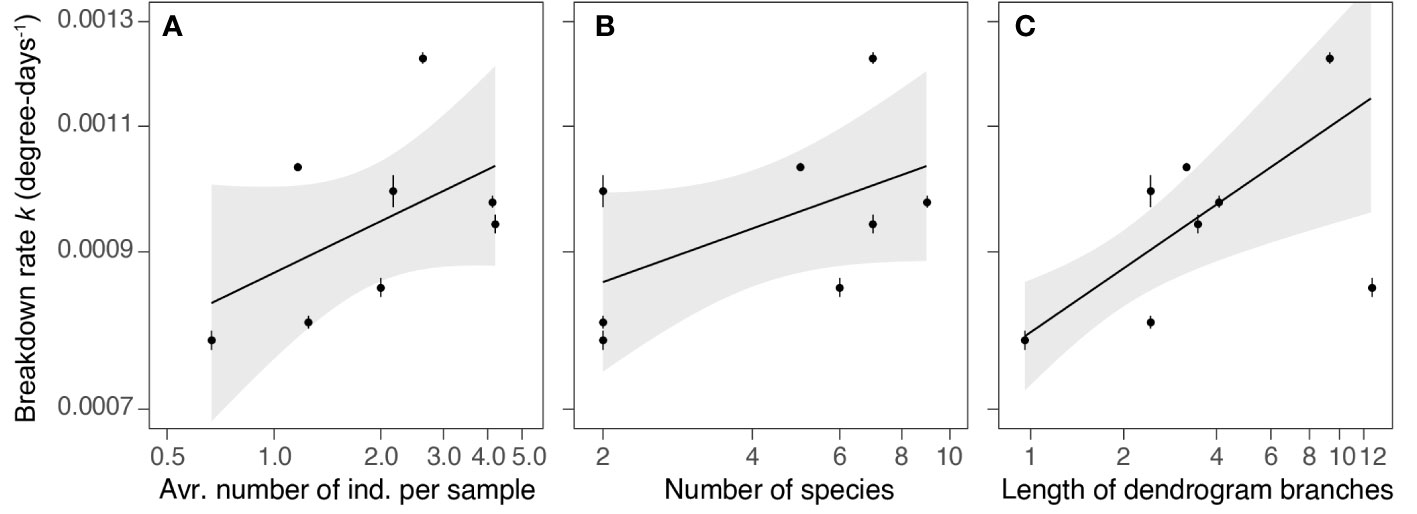
Figure 2 Relationships between black alder leaf litter breakdown rates and shredder community abundance (A), species richness (B) and functional diversity (C) in ponds. Expected breakdown rates based on the GLS models (lines ± 95% conf. intervals in grey) are displayed along with observed values (circles ± 95% CI error bars). For numeric details, see Table 2.
4 Discussion
Our study provides the first evidence of the role of aquatic detritivore functional diversity in pond litter decomposition, demonstrating that greater functional diversity is linked to faster decomposition. Such a result is theoretically expected since functional diversity represents resource use complementarity (Petchey et al., 2004). However, empirical evidence on the value of functional diversity for aquatic ecosystem processes is generally limited (Schmera et al., 2017), especially in standing waters. In lotic ecosystems, similarly to our findings, higher litter processing rates were observed in habitats occupied by shredder communities with distinct functional traits, as demonstrated by Frainer et al. (2014) and Frainer and McKie (2015). Nevertheless, it is important to note that the effect of functional diversity has been shown to vary among habitats and seasons. This underscores the significance of fluctuations in the relative abundances of traits for ecosystem process rates in real ecosystems.
4.1 Body size is a crucial functional trait mediating the effects of shredder diversity on litter decomposition
We specifically showed that the alder leaf litter decomposed faster in communities with broader spectra of shredder body size than in communities consisting of similar-sized species. The plausible mechanisms behind the observed positive functional diversity effect on decomposition include resource partitioning and facilitation (Loreau, 1998; Cardinale et al., 2002). Animals of different sizes may exhibit distinct feeding strategies, and such resource partitioning within the same trophic guild can reduce competition while enhancing the overall ecosystem process rate (Kleynhans et al., 2011). In freshwater detritivores, small chironomids can mine inside the leaves, mid-sized isopods and amphipods – such as Asellus and Echinogammarus – preferentially consume the more palatable parts of the leaves, and they may also scrub the leaf surfaces. On the other hand, large shredders such as Sericostoma and Gammarus are capable of biting through the leaf material, including the tougher parts such as veins (Graça et al., 1993; Friberg and Jacobsen, 1994; Wantzen and Wagner, 2006; Tonin et al., 2018). Apart from field observations, Tonin et al. (2018) conducted a controlled experiment to show how this feeding niche complementarity could enhance litter decomposition rates in aquatic ecosystems. These findings are consistent with theoretical predictions that complementarity among species has a systematic effect on ecosystem processes (Loreau, 1998).
In addition, facilitation between species is also thought to be an important mechanism by which biodiversity affects the rates of resource use that govern ecosystem processes (Cardinale et al., 2002). For example, the feeding activity of larger shredders that break down leaves into smaller fragments may facilitate litter consumption by smaller species, leading to elevated rates of leaf litter decomposition (Tonin et al., 2018).
Body size integrates numerous species traits that are relevant to ecosystem functioning (Woodward et al., 2005), and a loss of heterogeneity in body size within communities may have serious consequences for ecosystem processes (Handa et al., 2014). Our study corroborates these findings and highlights the importance of considering the distribution of detritivore body size as a critical community characteristic when examining the relationships between biodiversity and leaf litter decomposition rates in ponds.
4.2 Functional diversity of shredder communities is a better predictor of litter decomposition than species richness
The rate of organic matter decomposition observed in our study was more tightly linked with functional diversity than with species richness. Likewise, Reiss et al. (2011) found that variation in detritivore body size may have a stronger effect on detrital processing rates than species diversity. These results align with the expectation of Gessner et al. (2010) who assumed that direct functional characterization of communities, such as functional diversity, should be a more compelling predictor of aquatic biodiversity effects on litter decomposition than measures of taxonomic diversity. From an ecological niche perspective, when species exhibit trade-offs in resource utilization, a higher diversity of coexisting species can lead to more efficient resource utilization and enhanced overall ecosystem functioning (Chase and Leibold, 2003). Although functional diversity was positively correlated with species richness in the studied ponds (r = 0.57) and such positive relationships were also observed in other aquatic ecosystems (Schmera et al., 2017), species richness is apparently not a reliable proxy for ecosystem functioning. Díaz and Cabido (2001) argue that species richness might be an adequate surrogate for functional diversity under the assumption of improbable scenarios involving random or uniform occupation of niche space. However, aquatic species usually show non-random assembly (Urban, 2004; Brown and Swan, 2010) and clumped occupation of niche space, resulting in the aggregation of species into strong functional types (Petchey and Gaston, 2002). For example, species recorded in our study were clustered in five homogeneous groups with convergent traits (Figure S2A). Consequently, a unit increase in species richness may affect functional diversity disproportionally if a new species belongs to a complementary group. On the other hand, the addition of a functionally redundant species with similar traits may cause only negligible changes in functional diversity. The strong clumping of pond-dwelling shredders in the trait space and high functional redundancy are evident in horizontal stratification and the saturating relationship between functional diversity and species richness (Figure S2B). Due to the high functional redundancy within our dataset, functional diversity can efficiently explain variation in the rate of litter decomposition even when species richness does not.
4.3 Breakdown rates across aquatic ecosystems
Black alder litter decomposition observed in the studied ponds proceeded at an average rate of k = 0.013 days−1, which can be classified as fast breakdown (Petersen and Cummins, 1974). Due to a relatively strong connection between riparian vegetation and aquatic systems in ponds and low-order streams, we expected that leaf breakdown rates in ponds would resemble those observed in upper stream reaches. However, the observed breakdown rates seem to be lower than usually reported for headwater streams of 1st to 3rd order (Figure S4). The significance of shredding detritivores for organic matter breakdown was convincingly demonstrated in streams (Cuffney et al., 1990; Wallace et al., 1995; Jonsson et al., 2001) and in pond-like mesocosms (Auber et al., 2011) and natural ponds (Oertli, 1993). Nevertheless, the role and importance of shredders differ among aquatic systems.
Low-order streams are typically characterized by cool, well-oxygenated water, and these habitats often host a high abundance and diversity of shredders (Vannote et al., 1980; Minshall et al., 1983). In contrast, ponds, owing to their morphometric characteristics such as shallow depths and limited volume, might offer less favorable conditions characterized by fluctuating temperatures and often low oxygen contents. In our study, for instance, all ponds experienced maximum recorded temperatures exceeding 14°C, and oxygen concentrations dropped below 2 mg/L at several sites. These conditions have the potential to eliminate important shredder groups, such as gammarids and stoneflies (Toman and Dall, 1998; Pardo and García, 2016). Indeed, we observed low species numbers and abundances of shredders in all studied ponds. Depauperated communities of pond-dwelling shredders have already been reported elsewhere (Oertli, 1993; Bottollier-Curtet et al., 2011). Indeed, studies of litter decomposition in ponds are too scarce to allow for wider generalization, but it seems that slower decomposition of alder leaves in ponds may reflect low diversity and abundance of shredders in these systems.
5 Conclusions
We have shown that shredder functional diversity, represented by heterogeneity in body size, can efficiently explain variation in the rate of litter decomposition in ponds, while species richness does not seem to be a reliable proxy for this ecosystem process. This empirical evidence agrees with theoretical expectations and emphasizes functional diversity as a powerful tool in pond ecosystem research. Our study corroborates these findings and highlights the importance of considering the distribution of detritivore body sizes as a critical community characteristic when examining the relationships between biodiversity and leaf litter decomposition rates in ponds. Ecosystem functions are of top conservation priority due to human dependence on the services they provide. Due to its important role, variation in shredder body size should be specifically considered as a prominent community characteristic in pond conservation efforts. Leaf litter decomposition appears to be slower in ponds than in headwater streams, despite the comparably strong connections to riparian zones. Ponds presumably provide less favorable conditions for shredder communities than headwater streams, which may diminish the rate of litter decomposition in a pond environment. Nevertheless, the role of shredders has been studied extensively in headwater streams, while the information from ponds is rudimentary. Moreover, further studies should explicitly address the role of microbial communities in litter decomposition in ponds. While we did not observe a significant correlation between breakdown rate and conductivity, which is used as a proxy for nutrient levels assumed to support microbial decomposition, this indirect result implies the dominant influence of shredders in the process. Indeed, microbial activity can still play a significant role in decomposition and warrants further investigation. To enhance our understanding of cross-ecosystem variability in leaf litter decomposition and the role of shredding invertebrates in this mechanism, it is essential to gather additional field data from a broader spectrum of aquatic ecosystems, including ponds. This is especially important in light of recent studies suggesting the disproportionately large importance of ponds in the global carbon cycle and the high instability of their carbon balance in the face of climate change.
Data availability statement
The raw data supporting the conclusions of this article will be made available by the authors, without undue reservation.
Ethics statement
The manuscript presents research on animals that do not require ethical approval for their study.
Author contributions
VD: Writing – original draft, Writing – review & editing, Conceptualization, Data curation, Investigation. MN: Investigation, Project administration, Writing – review & editing. IS: Conceptualization, Writing – review & editing. MS: Conceptualization, Data curation, Formal Analysis, Funding acquisition, Investigation, Methodology, Software, Supervision, Visualization, Writing – original draft, Writing – review & editing.
Funding
The author(s) declare financial support was received for the research, authorship, and/or publication of this article. The study was supported by the Slovak Research and Development Agency under contract No. APVV-16-0236. This publication is the result of the project “Comprehensive research of determinants for ensuring environmental health” (ENVIHEALTH), ITMS 313011T721, supported by the Operational Programme Integrated Infrastructure (OPII) funded by the European Regional Development Fund (ERDF).
Conflict of interest
The authors declare that the research was conducted in the absence of any commercial or financial relationships that could be construed as a potential conflict of interest.
Publisher’s note
All claims expressed in this article are solely those of the authors and do not necessarily represent those of their affiliated organizations, or those of the publisher, the editors and the reviewers. Any product that may be evaluated in this article, or claim that may be made by its manufacturer, is not guaranteed or endorsed by the publisher.
Supplementary material
The Supplementary Material for this article can be found online at: https://www.frontiersin.org/articles/10.3389/fevo.2023.1286672/full#supplementary-material
References
Anderson N. H., Sedell J. R. (1979). Detritus processing by macroinvertebrates in stream ecosystems. Annu. Rev. Entomol. 24, 351–377. doi: 10.1146/annurev.en.24.010179.002031
Auber A., Roucaute M., Togola A., Caquet T. (2011). Structural and functional effects of conventional and low pesticide input crop-protection programs on benthic macroinvertebrate communities in outdoor pond mesocosms. Ecotoxicology 20, 2042. doi: 10.1007/s10646-011-0747-5
Benfield E. F., Fritz K. M., Tiegs S. D. (2017). Leaf-Litter Breakdown (London, United Kingdom: Elsevier Inc).
Bottollier-Curtet M., Charcosset J.-Y., Planty-Tabacchi A.-M., Tabacchi E. (2011). Degradation of native and exotic riparian plant leaf litter in a floodplain pond. Freshw. Biol. 56, 1798–1810. doi: 10.1111/j.1365-2427.2011.02620.x
Brett M. T., Bunn S. E., Chandra S., Galloway A. W. E., Guo F., Kainz M. J., et al. (2017). How important are terrestrial organic carbon inputs for secondary production in freshwater ecosystems? Freshw. Biol. 62, 833–853. doi: 10.1111/fwb.12909
Brown B. L., Swan C. M. (2010). Dendritic network structure constrains metacommunity properties in riverine ecosystems. J. Anim. Ecol. 79, 571–580. doi: 10.1111/j.1365-2656.2010.01668.x
Cadotte M. W., Carscadden K., Mirotchnick N. (2011). Beyond species: functional diversity and the maintenance of ecological processes and services. J. Appl. Ecol. 48, 1079–1087. doi: 10.1111/j.1365-2664.2011.02048.x
Cardinale B. J., Palmer M. A., Collins S. L. (2002). Species diversity enhances ecosystem functioning through interspecific facilitation. Nature 415, 426–429. doi: 10.1038/415426a
Carpenter S. R., Cole J. J., Kitchell J. F., Pace M. L. (1998). Impact of dissolved organic carbon, phosphorus, and grazing on phytoplankton biomass and production in experimental lakes. Limnol. Oceanogr. 43, 73–80. doi: 10.4319/lo.1998.43.1.0073
Chase J. M., Leibold M. A. (2003). Ecological niches: linking classical and contemporary approaches (Chicago, USA: University of Chicago Press).
Cole J. J., Carpenter S. R., Kitchell J., Pace M. L., Solomon C. T., Weidel B. (2011). Strong evidence for terrestrial support of zooplankton in small lakes based on stable isotopes of carbon, nitrogen, and hydrogen. P. Natl. A. Sci. 108, 1975–1980. doi: 10.1073/pnas.1012807108
Cornwell W. K., Cornelissen J. H. C., Amatangelo K., Dorrepaal E., Eviner V. T., Godoy O., et al. (2008). Plant species traits are the predominant control on litter decomposition rates within biomes worldwide. Ecol. Lett. 11, 1065–1071. doi: 10.1111/j.1461-0248.2008.01219.x
Cuffney T. F., Wallace J. B., Lugthart G. J. (1990). Experimental evidence quantifying the role of benthic invertebrates in organic matter dynamics of headwater streams. Freshw. Biol. 23, 281–299. doi: 10.1111/j.1365-2427.1990.tb00272.x
Díaz S., Cabido M. (2001). Vive la différence: plant functional diversity matters to ecosystem processes. Trends. Ecol. Evol. 16, 646–655. doi: 10.1016/S0169-5347(01)02283-2
Doi H. (2009). Spatial patterns of autochthonous and allochthonous resources in aquatic food webs. Popul. Ecol. 51, 57–64. doi: 10.1007/s10144-008-0127-z
Frainer A., McKie B. G. (2015). Shifts in the diversity and composition of consumer traits constrain the effects of land use on stream ecosystem functioning. Adv. Ecol. Res. 52, 169–200. doi: 10.1016/bs.aecr.2015.03.002
Frainer A., McKie B. G., Malmqvist B. (2014). When does diversity matter? Species functional diversity and ecosystem functioning across habitats and seasons in a field experiment. J. Anim. Ecol. 83, 460–469. doi: 10.1111/1365-2656.12142
Freude H., Harde K. W., Lohse G. A. (1971). Die Käfer Mitteleuropas. Bd 3, Adephaga 2, Palpicornia, Histeroidea, Staphylinoidea 1. (Krefeld, Germany: Goecke and Evers).
Freude H., Harde K. W., Lohse G. A. (1979). “The beetles of central Europe,” in Diversicornia, vol. 6. (Krefeld, Germany: Goecke and Evers).
Friberg N., Jacobsen D. (1994). Feeding plasticity of two detritivore-shredders. Freshw. Biol. 32, 133–142. doi: 10.1111/j.1365-2427.1994.tb00873.x
Gessner M. O. (2000). Breakdown and nutrient dynamics of submerged phragmites shoots in the littoral zone of a temperate hardwater lake. Aquat. Bot. 66, 9–20. doi: 10.1016/S0304-3770(99)00022-4
Gessner M. O., Chauvet E., Dobson M. (1999). A perspective on leaf litter breakdown in streams. Oikos 85, 377–384. doi: 10.2307/3546505
Gessner M. O., Swan C. M., Dang C. K., McKie B. G., Bardgett R. D., Wall D. H., et al. (2010). Diversity meets decomposition. Trends. Ecol. Evol. 25, 372–380. doi: 10.1016/j.tree.2010.01.010
Gillooly J. F., Brown J. H., West G. B., Savage V. M., Charnov E. L. (2001). Effects of size and temperature on metabolic rate. Science 293, 2248–2251. doi: 10.1126/science.1061967
Grabowski M., Bacela K., Konopacka A. (2007). How to be an invasive gammarid (Amphipoda: Gammaroidea)–comparison of life history traits. Hydrobiologia 590, 75–84. doi: 10.1007/s10750-007-0759-6
Graça M. A. S., Ferreira R. C. F., Coimbra C. N. (2001). Litter processing along a stream gradient: the role of invertebrates and decomposers. J. North. Am. Benthol. Soc 20, 408–420. doi: 10.2307/1468038
Graça M. A. S., Maltby L., Calow P. (1993). Importance of fungi in the diet of Gammarus pulex and Asellus aquaticus I: feeding strategies. Oecologia 93, 139–144. doi: 10.1007/BF00321203
Handa I. T., Aerts R., Berendse F., Berg M. P., Bruder A., Butenschoen O., et al. (2014). Consequences of biodiversity loss for litter decomposition across biomes. Nature 509, 218–221. doi: 10.1038/nature13247
Hieber M., Gessner M. O. (2002). Contribution of stream detrivores, fungi, and bacteria to leaf breakdown based on biomass estimates. Ecology 83, 1026–1038. doi: 10.1890/0012-9658(2002)083[1026:COSDFA]2.0.CO;2
Holgerson M. A., Raymond P. A. (2016). Large contribution to inland water CO2 and CH4 emissions from very small ponds. Nat. Geosci. 9, 222–226. doi: 10.1038/ngeo2654
Hooper D. U., Chapin F. S. III, Ewel J. J. (2005). Effects of biodiversity on ecosystem functioning: a consensus of current knowledge. Ecol. Monogr. 75, 3–35. doi: 10.1890/04-0922
İncekara Ü., Mart A., Erman O. (2005). Studies on turkish hydrophilidae (Coleoptera) I. Genus enochrus thomso. Turk. J. Zool. 29, 155–158.
Jansson M., Persson L., De Roos A. M., Jones R. I., Tranvik L. J. (2007). Terrestrial carbon and intraspecific size-variation shape lake ecosystems. Trends. Ecol. Evol. 22, 316–322. doi: 10.1016/j.tree.2007.02.015
Janz P. (2017). Risikobewertung von Chemikalien mit Fließgewässermodellökosystemen: die Entwicklung eines Mesokosmos-Testsystems und eine Pilotstudie mit dem Tierarzneimittel Ivermectin. (Gießen, Germany: Justus-Liebig-Universität Gießen).
Jonsson M., Malmqvist B., Hoffsten P. (2001). Leaf litter breakdown rates in boreal streams: does shredder species richness matter? Freshw. Biol. 46, 161–171. doi: 10.1046/j.1365-2427.2001.00655.x
Kleynhans E. J., Jolles A. E., Bos M. R. E., Olff H. (2011). Resource partitioning along multiple niche dimensions in differently sized African savanna grazers. Oikos 120, 591–600. doi: 10.1111/j.1600-0706.2010.18712.x
Kriska G. (2013). Freshwater invertebrates in Central Europe: a field guide (Cham, Switzerland: Springer Science & Business Media).
Loreau M. (1998). Biodiversity and ecosystem functioning: a mechanistic model. P. Natl. A. Sci. 95, 5632–5636. doi: 10.1073/pnas.95.10.5632
Mangiafico S. (2019). “rcompanion: Functions to support extension education program evaluation,” in R package version 2.0. 10.
Marcarelli A. M., Baxter C. V., Mineau M. M., Hall J. R.O. (2011). Quantity and quality: unifying food web and ecosystem perspectives on the role of resource subsidies in freshwaters. Ecology 92, 1215–1225. doi: 10.1890/10-2240.1
Menezes S., Baird D. J., Soares A. M. V. M. (2010). Beyond taxonomy: A review of macroinvertebrate trait-based community descriptors as tools for freshwater biomonitoring. J. Appl. Ecol. 47, 711–719. doi: 10.1111/j.1365-2664.2010.01819.x
Minshall G. W., Petersen R. C., Cummins K. W., Bott T. L., Sedell J. R., Cushing C. E., et al. (1983). Interbiome comparison of stream ecosystem dynamics. Ecol. Monogr. 53, 1–25. doi: 10.2307/1942585
Nagelkerke N. J. D. (1991). A note on a general definition of the coefficient of determination. Biometrika 78, 691–692. doi: 10.1093/biomet/78.3.691
Oertli B. (1993). Leaf litter processing and energy flow through macroinvertebrates in a woodland pond (Switzerland). Oecologia 96, 466–477. doi: 10.1007/BF00320503
Oertli B., Joye D. A., Castella E., Juge R., Lehmann A., Lachavanne J. B. (2005). PLOCH: A standardized method for sampling and assessing the biodiversity in ponds. Aquat. Conserv. 15, 665–679. doi: 10.1002/aqc.744
Oksanen J., Simpson G. L., Blanchet F. G., Kindt R., Minchin P. L., P. R., et al. (2022) vegan: Community Ecology Package. Available at: https://github.com/vegandevs/vegan.
Pace M. L., Cole J. J., Carpenter S. R., Kitchell J. F., Hodgson J. R., Van de Bogert M. C., et al. (2004). Whole-lake carbon-13 additions reveal terrestrial support of aquatic food webs. Nature 427, 240–243. doi: 10.1038/nature02227
Pardo I., García L. (2016). Water abstraction in small lowland streams: unforeseen hypoxia and anoxia effects. Sci. Total. Environ. 568, 226–235. doi: 10.1016/j.scitotenv.2016.05.218
Petchey O. L., Gaston K. J. (2002). Functional diversity (FD), species richness and community composition. Ecol. Lett. 5, 402–411. doi: 10.1046/j.1461-0248.2002.00339.x
Petchey O. L., Gaston K. J. (2006). Functional diversity: Back to basics and looking forward. Ecol. Lett. 9, 741–758. doi: 10.1111/j.1461-0248.2006.00924.x
Petchey O. L., Hector A., Gaston K. J. (2004). How do different measures of functional diversity perform? Ecology 85, 847–857. doi: 10.1890/03-0226
Petersen R. C., Cummins K. W. (1974). Leaf processing in a woodland stream. Freshw. Biol. 4, 343–368. doi: 10.1111/j.1365-2427.1974.tb00103.x
Pinheiro J., Bates D. (2006). Mixed-effects models in S and S-PLUS (New York, USA: Springer Science & Business Media).
Pinheiro J., Bates D., DebRoy S., Sarkar D. (2019). “R core team, (2019).‘nlme: linear and nonlinear mixed effects models,” in R Package Version 3, 1–140.
Pozo J. (1993). Leaf litter processing of alder and eucalyptus in the Agüera stream system (North Spain). I. Chem. changes. Arch. Hydrobiol. 127, 299. doi: 10.1127/archiv-hydrobiol/127/1993/299
Prairie Y. T. (2008). Carbocentric limnology: looking back, looking forward. Can. J. Fish. Aquat. Sci. 65, 543–548. doi: 10.1139/f08-011
R Core Team (2019). R: A language and environment for statistical computing (Vienna, Austria: R Foundation for Statistical Computing).
Reiss J., Bailey R. A., Perkins D. M., Pluchinotta A., Woodward G. (2011). Testing effects of consumer richness, evenness and body size on ecosystem functioning. J. Anim. Ecol. 80, 1145–1154. doi: 10.1111/j.1365-2656.2011.01857.x
Richardson J. S. (1991). Seasonal food limitation of detritivores in a montane stream: an experimental test. Ecology 72, 873–887. doi: 10.2307/1940589
Royer T. V., Minshall G. W. (2003). Controls on leaf processing in streams from spatial-scaling and hierarchical perspectives. J. North. Am. Benthol. Soc 22, 352–358. doi: 10.2307/1468266
Rozkošný R. (1980). Klíč vodních larev hmyzu. (Praha, ČSSR: Academia-nakladatelství Československé akademie věd).
Schielzeth H. (2010). Simple means to improve the interpretability of regression coefficients. Methods Ecol. Evol. 1, 103–113. doi: 10.1111/j.2041-210X.2010.00012.x
Schmera D., Heino J., Podani J., Erős T., Dolédec S. (2017). Functional diversity: a review of methodology and current knowledge in freshwater macroinvertebrate research. Hydrobiologia 787, 27–44. doi: 10.1007/s10750-016-2974-5
Schmidt-Kloiber A., Hering D. (2015). www. freshwaterecology. info–An online tool that unifies, standardises and codifies more than 20,000 European freshwater organisms and their ecological preferences. Ecol. Indic. 53, 271–282. doi: 10.1016/j.ecolind.2015.02.007
Skuhrovec J., Gosik R., Stejskal R., Sprick P., Caldara R., Team, E. C (2011). Digital-weevil-determination for curculionoidea of west palaearctic. Transalpina: bagous (Bagoinae). Snudebiller 12, 39–56.
Šporka F. (2003). Vodné bezstavovce (makroevertebráta) Slovenska, súpis druhov a autekologické charakteristiky (Bratislava: Slovenský hydrometeorologický ústav).
Stoler A. B., Relyea R. A. (2020). Reviewing the role of plant litter inputs to forested wetland ecosystems: leafing through the literature. Ecol. Monogr. 90, e01400. doi: 10.1002/ecm.1400
Straka M., Sychra J. (2007). Determinační kurz makrozoobentosu: Coleoptera (Brno: Ústav botaniky a zoologie PřF Masarykovy univerzity a VÚV TG Masaryka).
Tank J., Rosi-Marshall E., Griffiths N. A., Entrekin S. A., Stephen M. L. (2010). A review of allochthonous organic matter dynamics and metabolism in streams. J. North. Am. Benthol. Soc. 29, 118–146. doi: 10.1899/08-170.1
Tilman D. (2001). Functional diversity. Encyclopedia biodiversity 3, 109–120. doi: 10.1016/B0-12-226865-2/00132-2
Tilman D., Knops J., Wedin D., Reich P., Ritchie M., Siemann E. (1997). The influence of functional diversity and composition on ecosystem processes. Science 277, 1300–1302. doi: 10.1126/science.277.5330.1300
Toman M. J., Dall P. C. (1998). Respiratory levels and adaptations in four freshwater species of Gammarus (Crustacea: Amphipoda). Int. Rev. Hydrobiol. 83, 251–263. doi: 10.1002/iroh.19980830308
Tonin A. M., Pozo J., Monroy S., Basaguren A., Pérez J., Goncalves J. F. Jr., et al. (2018). Interactions between large and small detritivores influence how biodiversity impacts litter decomposition. J. Anim. Ecol. 87, 1465–1474. doi: 10.1111/1365-2656.12876
Urban M. C. (2004). Disturbance heterogeneity determines freshwater metacommunity structure. Ecology 85, 2971–2978. doi: 10.1890/03-0631
Vannote R. L., Minshall G. W., Cummins K. W., Sedell J. R., Cushing C. E. (1980). The river continuum concept. Can. J. Fish. Aquat. Sci. 37, 130–137. doi: 10.1139/f80-017
Wallace J. B., Eggert S., Meyer J. L., Webster J. R. (1997). Multiple trophic levels of a forest stream linked to terrestrial litter inputs. Science 277, 102–104. doi: 10.1126/science.277.5322.102
Wallace J. B., Webster J. R., Meyer J. L. (1995). Influence of log additions on physical and biotic characteristics of a mountain stream. Can. J. Fish. Aquat. Sci. 52, 2120–2137. doi: 10.1139/f95-805
Wantzen K. M., Wagner R. (2006). Detritus processing by invertebrate shredders: a neotropical–temperate comparison. J. North. Am. Benthol. Soc. 25, 216–232. doi: 10.1899/0887-3593(2006)25[216:DPBISA]2.0.CO;2
Webster J. R., Benfield E. F. (1986). Vascular plant breakdown in freshwater ecosystems. Annu. Rev. Ecol. Syst. 17, 567–594. doi: 10.1146/annurev.es.17.110186.003031
Westlake D. F., Kvet J., Szczepanski A. (2009). The production ecology of wetlands: the IBP synthesis (Cambridge, United Kingdom: Cambridge University Press).
Woodward G., Ebenman B., Emmerson M., Montoya J. M., Olesen J. M., Valido A., et al. (2005). Body size in ecological networks. Trends. Ecol. Evol. 20, 402–409. doi: 10.1016/j.tree.2005.04.005
Yvon-Durocher G., Hulatt C. J., Woodward G., Trimmer M. (2017). Long-term warming amplifies shifts in the carbon cycle of experimental ponds. Nat. Clim. Change 7, 209–213. doi: 10.1038/nclimate3229
Keywords: breakdown rate, functional ecology, body size, detritivore diversity, Alnus glutinosa
Citation: Dekanová V, Novikmec M, Svitková I and Svitok M (2023) Functional diversity of shredders, not species richness, drives the decomposition rate of leaf litter in ponds. Front. Ecol. Evol. 11:1286672. doi: 10.3389/fevo.2023.1286672
Received: 31 August 2023; Accepted: 23 October 2023;
Published: 06 November 2023.
Edited by:
Sahadevan Seena, University of Coimbra, PortugalReviewed by:
Sudeep Ghate, Nitte University, IndiaKandikere Ramaiah Sridhar, Mangalore University, India
Copyright © 2023 Dekanová, Novikmec, Svitková and Svitok. This is an open-access article distributed under the terms of the Creative Commons Attribution License (CC BY). The use, distribution or reproduction in other forums is permitted, provided the original author(s) and the copyright owner(s) are credited and that the original publication in this journal is cited, in accordance with accepted academic practice. No use, distribution or reproduction is permitted which does not comply with these terms.
*Correspondence: Marek Svitok, svitok@tuzvo.sk