First Detection of the Crayfish Plague Pathogen Aphanomyces astaci in Costa Rica: European Mistakes Should Not Be Repeated
- 1Department of Mycology, Real Jardín Botánico-CSIC, Madrid, Spain
- 2Unidad de Investigación Pesquera y Acuicultura (UNIP), Centro de Investigación en Ciencias del Mar y Limnología (CIMAR), Universidad de Costa Rica, San José, Costa Rica
The crayfish plague pathogen Aphanomyces astaci is one of the main factors responsible for the decline in European and Asian native crayfish species. This pathogen was transported to these regions through its natural carriers, North American crayfish species, which were introduced during the last century. Since then, the carrier species and the pathogen have spread worldwide due to globalization and the highly invasive nature of these species. In Europe, five carrier species have been categorized as high-risk as they are responsible for the loss of provisioning services, which endangers freshwater ecosystems. The red swamp crayfish Procambarus clarkii, in particular, is currently one of the most concerning species as its spread threatens crayfish biodiversity and freshwater ecosystems worldwide. In this study, we describe the first detection of A. astaci in an introduced population of P. clarkii in Central America, specifically in Costa Rica. Using molecular approaches, we analyzed 48 crayfish samples collected from Reservoir Cachí and detected the presence of A. astaci in four of these samples. The introduction of P. clarkii and the incorrect management of the species (related to its fishery and the commercialization of live specimens) over the past decades in Europe are mistakes that should not be repeated elsewhere. The detection of the pathogen is a warning sign about the dangerous impact that the introduction of this invasive crayfish may have, not only as a carrier of an emerging disease but also as a direct risk to the invaded ecosystems. Our results may serve to (1) assess current and future consequences, and (2) direct future research activities, such as determining the potential impacts of A. astaci on native decapod species, or on other introduced crayfish species that are used for aquaculture purposes, such as Cherax quadricarinatus.
Introduction
Freshwater crayfishes are a highly diverse group of aquatic organisms that comprises more than 650 described species (Crandall and Buhay, 2008; Crandall and De Grave, 2017). These crustacean decapods are threatened by habitat destruction, water diversion, pollution, and invasive alien species, particularly other crayfish species (Kawai and Crandall, 2016). For example, native Eurasian crayfishes (i.e., species of the genera Astacus, Austropotambius, Pontastacus, and Cambaroides) are keystone species that have alarmingly declined in the last few decades (Kouba et al., 2014; Martín-Torrijos et al., 2018). The introduction and spread of North American crayfish species is one of the main factors responsible for this decline (reviewed in Rezinciuc et al., 2015). The rapid life cycle, dispersal capacities, burrowing activities, high population densities, and aggressive behavior of crayfishes destabilize trophic chains (Souty-Grosset et al., 2016). In addition, North American crayfish species act as vectors for the crayfish plague agent Aphanomyces astaci (see Huang et al., 1994). This pathogen, one of the 100 world’s worst invasive alien species (Lowe et al., 2000), is responsible for the European crayfish decline during the past century (reviewed in Holdich et al., 2009; Rezinciuc et al., 2015).
Aphanomyces astaci belongs to the class Oomycetes, which includes important pathogenic species of both plants and animals (Beakes et al., 2012). This pathogen chronically infects its natural hosts, North American crayfish species, by establishing a balanced host-pathogen interaction (Unestam, 1969, 1972; Cerenius et al., 2003). The first documented introductions of North American crayfish species to Europe, and subsequent crayfish plague outbreaks, occurred during the 19th century (Cornalia, 1860; Bott, 1950). The ensuing large-scale and worldwide importation of these species, and their spread by illegal translocations, has caused outbreaks throughout Europe (Taugbøl et al., 1993; Huang et al., 1994; Diéguez-Uribeondo et al., 1997; Lilley et al., 1997; Machino and Diéguez-Uribeondo, 1998; Vennerström et al., 1998; Diéguez-Uribeondo and Söderhäll, 1999; Oidtmann et al., 1999; Diéguez-Uribeondo, 2006; Kozubíková et al., 2009; Rezinciuc et al., 2014; Jussila et al., 2015; Martín-Torrijos et al., 2019) and other biogeographical regions of the world (Kawai and Crandall, 2016; Martín-Torrijos et al., 2018). The introduced North American crayfish species pose a real threat to European crayfish biodiversity, public health, and the economy. Indeed, five introduced crayfish species (Faxonius limosus, Faxonius virilis, Pacifastacus leniusculus, Procambarus clarkii, and Procambarus virginalis) have already been included in the “List of Invasive Alien Species of Union concern” (the Union list). This legislation, which aims to prevent, control and/or eradicate invasive alien species, has been adopted by all member states (EUR-lex, 2014, 2016; Kopf et al., 2017).
Over the past few decades, several studies have assessed the devastation caused by introduced North American crayfishes on native European crayfish populations (Huang et al., 1994; Diéguez-Uribeondo et al., 2006; Kouba et al., 2014; Martín-Torrijos et al., 2019). In particular, the red swamp crayfish P. clarkii is a well-known threat, not only as a vector of A. astaci but also for its countless impact on freshwater ecosystems (Gherardi et al., 2011; Arce and Dieguez-Uribeondo, 2015; Souty-Grosset et al., 2016). The natural distribution of P. clarkii includes northern Mexico, and the southern and southeastern United States (Nagy et al., 2020). This species, however, has been introduced to all continents, except Australia and Antarctica (Kawai and Crandall, 2016); furthermore, it has also been seized from Australian pet trade (Queensland Government, 2019). To date, all A. astaci haplotypes analyzed from P. clarkii belong to the same lineage, also named D-haplogroup (Makkonen et al., 2018; Martín-Torrijos et al., 2018, 2019, 2021a,b). Some strains of the D-haplogroup can grow, sporulate, and disperse their zoospores at higher temperatures (by more than 5°C) than those belonging to other genetic groups, suggesting that they are better adapted to warmer freshwater environments compared with other strains (Diéguez-Uribeondo et al., 1995). The presence of both P. clarkii and A. astaci has been confirmed in several tropical regions, including Brazil (Peiró et al., 2016) and Indonesia (Putra et al., 2018). Although several Central American countries, including Costa Rica, Nicaragua, Guatemala, and Belize, have reported the presence of the invasive species P. clarkii (Wehrtmann et al., 2016), the presence of A. astaci in its Central American populations has not yet been tested.
Several cases of the introduction of alien animal species have been reported for Costa Rica (e.g., Barrientos-Llosa and Monge-Nájera, 2010; Barquero and Araya, 2016), a known biodiversity hotspot (Myers et al., 2000). However, to date, only two invasive crayfish species, P. clarkii and Cherax quadricarinatus, have been reported for the country (Figure 1; Torres and Álvarez, 2012; Wehrtmann et al., 2016; Azofeifa-Solano et al., 2017). Procambarus clarkii was introduced to Costa Rica from Louisiana in 1966 (Huner, 1977). The population present in the Reservoir Cachí, Province of Cartago, likely represents the oldest and largest population in the country (Figure 1). The crayfish resource in this reservoir is harvested and commercialized by locals for human consumption; however, it is suspected that individuals from this population are also captured for the pet trade or for their translocation to other water bodies (FV-R, CIMAR, personal communication).
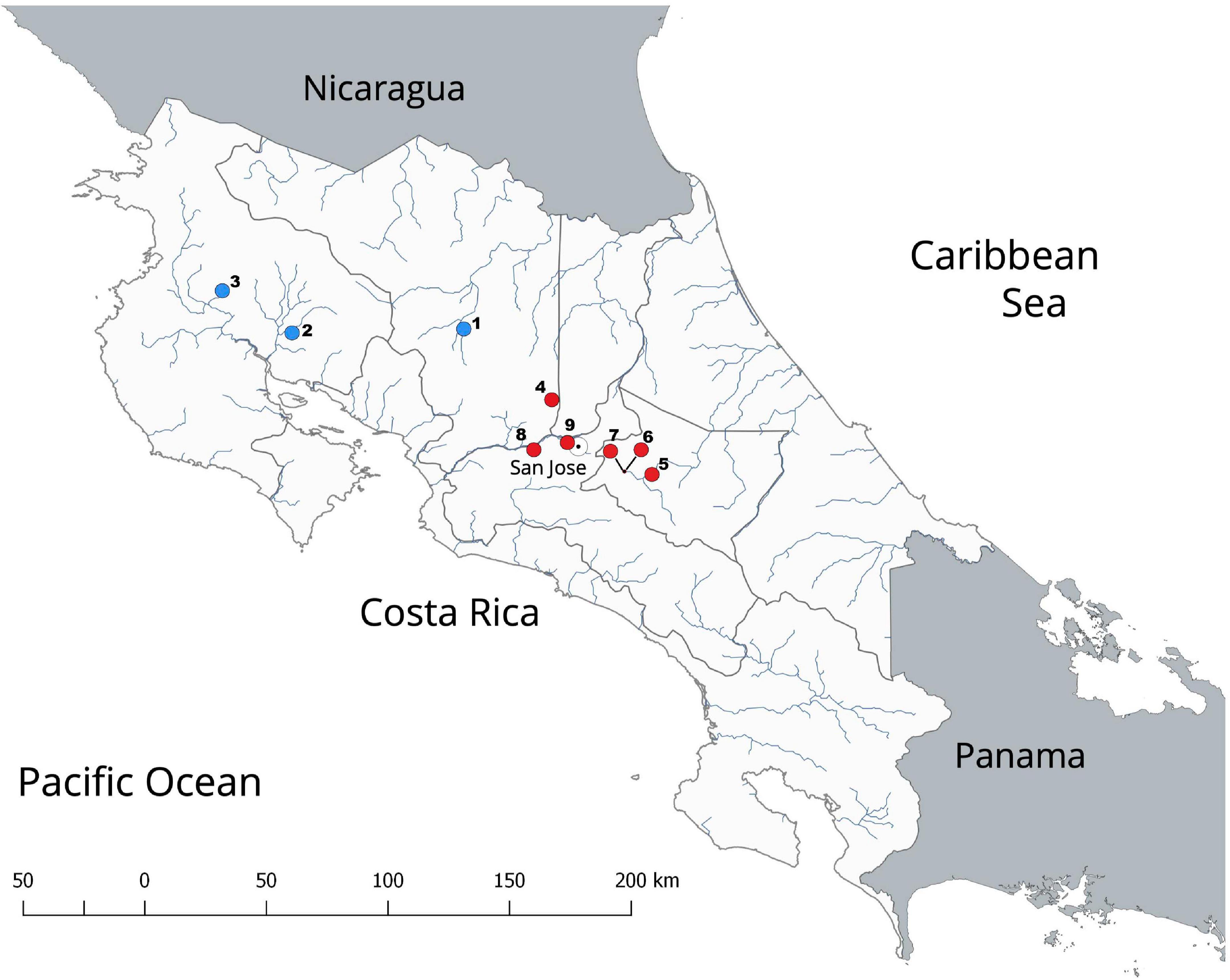
Figure 1. Map showing the locations of the reported invasive alien crayfishes in Costa Rica. Red circles indicate Procambarus clarkii populations; blue circles indicate Cherax quadricarinatus populations. Numbers (1–9) indicate the following locations: (1) Río Balsa, Ciudad Quesada, Alajuela Province; (2) Universidad Técnica Nacional, Bagaces, Guanacaste Province; (3) Irrigation Channels in Bagatzi, Guanacaste Province; (4) Fraijanes Lagoon, Alajuela Province; (5) Hydroelectric Dam Cachí, Cartago Province; (6) Jardín Botánico Lankester Lagoons, Cartago Province; (7) Instituto Tecnológico Costarricense Lagoons, Cartago Province; (8) Peace Park, El Rodeo, San José Province; (9) La Sabana Lake, San José Province.
The high ecological plasticity of both P. clarkii and A. astaci in tropical environments makes the presence of P. clarkii in Costa Rica a matter of great concern (Villalobos-Rojas, 2019), as does their continued introduction to other parts of the world, despite the well-documented negative impacts that both species have had, and continue to have, on European biodiversity. In this study, we aimed to determine whether the crayfish plague pathogen A. astaci is already present in the P. clarkii population at Reservoir Cachí. By using a specific mitochondrial marker [the ribosomal small subunit (rnnS) (Makkonen et al., 2018)], we confirm the presence of the pathogen A. astaci in Costa Rica, and provide information about its genetic diversity. Finally, we discuss our results in light of the European experience as a lesson learned to emphasize the potential danger of the introduction of both invasive alien species (P. clarkii and A. astaci) to tropical freshwater ecosystems.
Materials and Methods
Crayfish Sampling
In May 2019, we collected a total of 48 live crayfishes from a population of P. clarkii introduced into Reservoir Cachí (Province of Cartago, Costa Rica) (Table 1, Figure 1, and Supplementary Table 1). The specimens were transferred into aquaria at the Centro de Investigación en Ciencias del Mar y Limnología (CIMAR) of the Universidad de Costa Rica, located in San José (Costa Rica). The aquaria were maintained at 25°C, and crayfishes were checked daily for the presence of molts. Molts were maintained in sterile distilled water for 3 days prior to their examination under a microscope to detect for the presence of A. astaci, as described in Martín-Torrijos et al. (2021b). Finally, molts were individually preserved in 96% ethanol for further examination and the molecular analyses.
Macroscopic and Microscopic Examination
Each collected individual was examined macroscopically for the presence of melanized areas or spots in the soft cuticle and appendages (common indications of potential A. astaci infection). Because crayfish can regenerate pereiopods (Shull, 1909), we collected one pereiopod per individual (suspected to be infected) and individually preserved them in 96% ethanol for the molecular analyses. Prior to these analyses, each pereiopod was examined microscopically for the presence of melanized hyphae using an Olympus CKX41SF inverted microscope (Olympus Optical, Tokyo, Japan). Light micrographs of colonizing hyphae were captured using a QImaging Micropublisher 5.0 digital camera (QImaging, Burnaby, BC, Canada).
Molecular Analyses and Phylogenetic Approximations
All samples were washed with TE buffer (TRIS 10 mM/EDTA 1 mM, pH 8) prior to DNA extraction. Samples were transferred into individual 2-ml Eppendorf tubes, frozen at −80°C and then lyophilized in a VirTis BenchTop K freeze dryer for 24 h (≤−50°C; ≤20 mTorr). Subsequently, samples were mechanical ruptured using a TissueLyser (QIAGEN, Venlo, Netherlands). Genomic DNA was isolated using the E.Z.N.A.® Insect DNA Kit (Omega Bio-Tek, Norcross, GA, United States).
In order to detect the presence and diversity of A. astaci, we attempted to amplify fragments of the mitochondrial ribosomal small (rnnS) and large (rnnL) subunits using two primer pairs described in Makkonen et al. (2018). The positive control for the rnnS and rnnL primers was the A. astaci strain SAP-Malaga 5 (which originated from the Iberian Peninsula) (Martín-Torrijos et al., 2019); milliQ water was used as the negative control. All PCR products were checked on 1% agarose gels containing 0.5 μM of SYBR Safe. Both strands were sequenced using an automated sequencer (Applied Biosystems 3730xl DNA) by Macrogen (Netherlands).
Sequences were assembled and edited using the program Geneious® 10.2.3 (Kearse et al., 2012). Two phylogenetic approximations, Bayesian Inference (BI) and Maximum Likelihood (ML), were used to reconstruct phylogenetic relationships, following Makkonen et al. (2018). Reference sequences were obtained from Makkonen et al. (2018) and Martín-Torrijos et al. (2019). Aphanomyces frigidophilus was used as the outgroup.
Results
Macroscopic and Microscopic Examination
We obtained a total of two molts (1CR and 23CR) from the collected P. clarkii specimens (Supplementary Table 1). Macroscopic observations of these molts revealed that both exhibited melanized areas in the soft abdominal cuticle and pereiopods characteristic of infection by A. astaci. Moreover, under microscopic observation, we found melanized hyphae growing within the pereiopods of specimen 1CR (Figure 2).
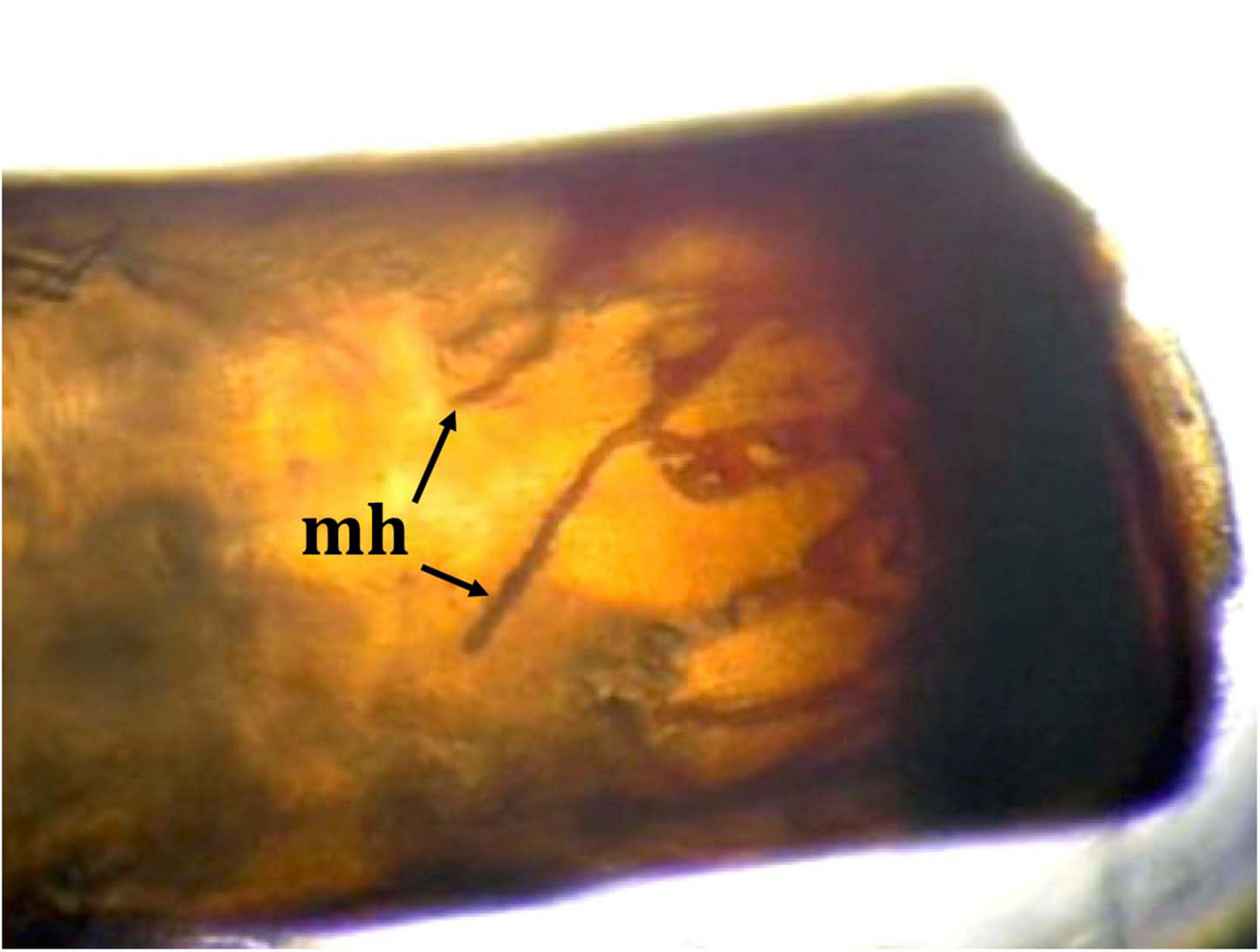
Figure 2. A pereiopod of a Procambarus clarkii specimen from Reservoir Cachí, Costa Rica, showing the presence of melanized hyphae (mh), signs of a strong immune reaction against the crayfish plague pathogen Aphanomyces astaci.
Molecular Analyses and Phylogenetic Approximations
The rnnS subunit was amplified from four of the 48 crayfish specimens (3CR, 5CR, 8CR, and 10CR; Supplementary Table 1). The obtained sequences showed 100% identity to the d1/d2-haplotypes of the D-haplogroup (GenBank accession numbers MW181669–MW181672) (Supplementary Figure 1 and Supplementary Table 1). However, we could not amplify the rnnL subunit from these specimens. Also, neither of the subunits could be amplified from either of the two collected molts.
The two phylogenetic approximations (BI and ML) based on the rnnS data (Supplementary Figure 1) recovered congruent topologies, and showed the correspondence of the analyzed A. astaci sequences from P. clarkii in Costa Rica with the D-haplogroup.
Discussion
This is the first reported case of the crayfish plague pathogen A. astaci in Central America. The presence of this pathogen was found in Reservoir Cachí in Costa Rica as a consequence of translocations of the invasive alien crayfish species P. clarkii. We also determined that the A. astaci found in this P. clarkii population belongs to the D-haplogroup. Four haplotypes have been identified for A. astaci in P. clarkii: d1, d2, d3, and usa6 (Makkonen et al., 2018; Martín-Torrijos et al., 2018, 2021b). The four mitochondrial rnnS sequences obtained in this study are all identical to those corresponding to either the d1 or d2 rnnS haplotypes reported by Makkonen et al. (2018). However, to identify a specific A. astaci haplotype with confidence, both the rnnS and rnnL regions must be analyzed (Casabella-Herrero et al., 2021). Therefore, the lack of rnnL sequences for these samples makes it impossible to confirm their specific haplotype. Nevertheless, rnnS data are reliable enough to identify both the pathogen and its genetic group (rnnS is a new reliable marker, and can be used to identify genetic variability in order to differentiate A. astaci from close-related taxa) (Casabella-Herrero et al., 2021). The fact that the analyzed Costa Rican sequences belong to the D-haplogroup and that some strains of this genetic group appear to be better adapted to warm environments (Diéguez-Uribeondo et al., 1995) suggest an increased likelihood of A. astaci transmission to other suitable hosts (freshwater decapods) in this tropical environment.
In Costa Rica, although there are no native crayfish species, there are approximately 26 native species of freshwater decapods (21 shrimp species belonging to the families Atyidae and Palaemonidae, and 15 crab species belonging to Pseudothelphusidae) (Lara and Wehrtmann, 2011; Lara et al., 2013; Magalhães et al., 2015). Despite the seemingly narrow host range of A. astaci (Unestam, 1972; Diéguez-Uribeondo et al., 2009), several studies have shown transmission of the pathogen to other freshwater decapods including Atya gabonensis, Atyopsis moluccensis, Eriocheir sinensis, Macrobrachium dayanum, Macrobrachium lanchesteri, Neocaridina davidi, Palaemon kadiakensis, and Potamon potamios (Schrimpf et al., 2014; Svoboda et al., 2014; Putra et al., 2018; Mrugała et al., 2019; Martín-Torrijos et al., 2021b). Although the coexistence of native freshwater decapods with P. clarkii has not been reported yet in Costa Rica, the potential impact of the invasive species presence on freshwater fauna in the country should be evaluated. Also, the occurrence of P. clarkii in other Central American countries (Wehrtmann et al., 2016) indicates a need for genetic studies of these other populations to determine the extent of A. astaci distribution in the region and assess any possible threats to the local native fauna. Moreover, the accessibility to live specimens by people makes the translocation and further spread of P. clarkii and the pathogen highly probable. It is already clear that once North American crayfish species have been introduced, their expansion becomes difficult to control. The main mechanisms of spread of this invasive crayfish and the disease in Europe have been identified as fishery-related activities, fishing as a means to control increasing populations, and the commercialization of live crayfishes (Alonso et al., 2000; Diéguez-Uribeondo, 2006). Therefore, control and biosecurity measures aimed at these particular activities, among others, should be implemented in other countries or regions to prevent the dispersion of the crayfish plague pathogen (e.g., prevent the movement of potentially infected live or dead crayfish and potentially contaminated water and equipment from sites with a potential or known presence of the pathogen to uninfected sites) [OIE (World Organization for Animal Health), 2019].
Furthermore, the effects of P. clarkii as an invasive species has been extensively studied in Europe, where the species has been recorded in at least 16 territories (Souty-Grosset et al., 2016). In these areas, freshwater biodiversity has been severely affected due to constant predation of the invasive species on fishes (Ilhéu et al., 2007; Reynolds, 2011), shrimps (Banha and Anastácio, 2011), amphibians (Ficetola et al., 2012; Nunes et al., 2014), mollusks (Correia et al., 2005; Chucholl, 2013), and other macroinvertebrates (Correia et al., 2005; Correia and Anastácio, 2008). Therefore, at management level, P. clarkii is considered an agricultural pest, and a threat to water drainage systems and the restoration of several European water bodies (Souty-Grosset et al., 2016). Thus, P. clarkii is considered a high-risk species, responsible for the loss of provisioning services (associated with wide changes in ecological communities and increased costs to agriculture and water management) (Souty-Grosset et al., 2016).
Additionally, in 1985, another invasive crayfish, the Australian species C. quadricarinatus, was introduced to Costa Rica for aquaculture purposes (Luis Rolier Lara personal communication in Wehrtmann et al., 2016). Since its introduction, accidental releases have occurred (INCOPESCA, personal communication), and in 2017, the presence of this species in both Pacific and Caribbean freshwater drainages was reported (Azofeifa-Solano et al., 2017) (see Figure 1), making the situation in Costa Rica even more complex. These unintentional releases from holding facilities (Azofeifa-Solano et al., 2017) highlight the problematic use of invasive alien species for aquaculture purposes. Cherax quadricarinatus, as with other Australian crayfish species, has been reported as highly susceptible to the crayfish plague pathogen (Unestam, 1975; Marino et al., 2014; Hsieh et al., 2016; Mazza et al., 2018). In fact, some of these studies have even shown that invasive alien species such P. clarkii can eradicate other invasive alien species (e.g., C. quadricarinatus or Cherax destructor) due to the transmission of pathogens, and not direct competition or predation (Marino et al., 2014; Mazza et al., 2018).
Considering Costa Rica is a biodiversity hotspot, the presence of high-risk species such as P. clarkii and C. quadricarinatus should be a cause of concern for national authorities. The native freshwater fauna may become threatened if more translocations of these invasive alien crayfish species occur. This may be especially true for amphibians: Brannelly et al. (2015) found evidence of Batrachochytrium dendrobatidis (Bd) infections in farmed and natural populations of Procambarus spp. These authors describe how even a low prevalence of Bd infection could have implications for global amphibian conservation. Zumbado-Ulate et al. (2019) summarized the data on prevalence of Bd in Costa Rica and how it has irreversibly affected amphibian populations since the 1980s. Low-intensity Bd infections in amphibian, below the threshold associated with mortalities, seem common in the country. However, populations of P. clarkii present in Costa Rica (see Table 1 and Figure 1) could act as additional reservoirs for Bd and contribute to its further spread.
In 2014, the European Union implemented legislation to prevent and regulate the introduction and spread of invasive alien species that focused on control and eradication (EUR-lex, 2014, 2016; Kopf et al., 2017). In this context, the Council of Europe has drafted a series of voluntary codes of conduct based on the Convention on the Conservation of European Wildlife and Natural Habitats (Bern Convention). These codes of conduct and other guidelines aim to make industries and institutions that handle or encounter non-native species aware of the risks that these species can have on native biodiversity [European Alien Species Information Network (EASIN), 2021]. Similarly, in 2017, Costa Rica implemented the Regulations to the Wildlife Conservation Law (Decreto No. 26435 MINAE), which deals with invasive alien species (República de Costa Rica, 2005). However, the importation of live, captive bred invasive alien species (fauna and flora) and products of inland fisheries is still permitted under this legislation (República de Costa Rica, 2005; Young, 2006). If ever released, these species could negatively impact Costa Rican freshwater biodiversity. Currently, there are no official lists of introduced invasive alien species in Costa Rica; however, as of 2017, at least 461 vertebrate species (6 amphibians, 68 birds, 23 mammals, 12 reptiles, and 352 teleost fishes) are considered to have been introduced (Eduardo Chacón-Madrigal, Universidad de Costa Rica, personal communication).
The bad experience and mistakes made by European countries in their treatment of invasive crayfish species offer a lesson for other countries starting to experience the impact of introduced crayfish species, such as Costa Rica. The sooner that these countries are aware and concerned about the detrimental impact crayfish invasions can have on native ecosystems and their biodiversity, the greater the chance they can act to minimize the impact. Moreover, wildlife managers, conservationists, aquaculture companies, and authorities should also be made aware of the threats that each invasive alien species (crayfish and pathogen) may have on already threatened species. To better understand the scope of these threats, additional studies are necessary to assess their potential impact on the native freshwater fauna in Costa Rica and other tropical regions worldwide.
Data Availability Statement
The datasets presented in this study can be found in online repositories. The names of the repository/repositories and accession number(s) can be found in the article/Supplementary Material.
Author Contributions
LM-T, IW, and JD-U contributed to the design, supervision of the project, and writing of the manuscript. AC-V conducted the field sampling, performed the molecular analyses (DNA extraction and haplotyping), and helped with the writing of the manuscript. JA-S conducted the field sampling and helped with the writing of the manuscript. FV-R contributed to the supervision of the project and writing of the manuscript. All authors contributed to the article and approved the submitted version.
Funding
We acknowledge support of the publication fee by the CSIC Open Access Publication Support Initiative through its Unit of Information Resources for Research (URICI). JD-U was supported by Project CGL2016-80526-R of the Ministerio de Economía y Competitividad, Spain, and AC-V was supported by the Máster en Biodiversidad en Áreas Tropicales y su Conservación, Universidad Internacional Menéndez Pelayo, and Consejo Superior de Investigaciones Científicas, UIMP–CSIC. Additional support for this study was made available through the project 808-B7-285 financed by the Universidad de Costa Rica.
Conflict of Interest
The authors declare that the research was conducted in the absence of any commercial or financial relationships that could be construed as a potential conflict of interest.
Acknowledgments
We thank the assistance of the Unidad de Investigación Pesquera y Acuicultura (UNIP) from CIMAR for providing us with the facilities to maintain the Procambarus clarkii specimens. We also thank María Martinez-Ríos and Gloria Casabella-Herrero from the Real Jardín Botánico–Consejo Superior de Investigaciones Científicas (RJB-CSIC) for their technical assistance.
Supplementary Material
The Supplementary Material for this article can be found online at: https://www.frontiersin.org/articles/10.3389/fevo.2021.623814/full#supplementary-material
References
Alonso, F., Temiño, C., and Diéguez-Uribeondo, J. (2000). Status of the white clawed crayfish, Austropotamobius pallipes (Lereboullet, 1858) in Spain: distribution and legislation. B. Fr. Pêche Piscic. 356, 31–54. doi: 10.1051/kmae:2000003
Arce, J., and Dieguez-Uribeondo, J. (2015). Structural damage caused by the invasive crayfish Procambarus clarkii (Girard, 1852) in rice fields of the Iberian Peninsula: a study case. J. Fundam. Appl. Limnol. 186, 259–269. doi: 10.1127/fal/2015/0715
Azofeifa-Solano, J. C., Naranjo-Elizondo, B., Rojas-Carranza, A. H., and Cedeño-Fonseca, M. (2017). Presence of the Australian redclaw crayfish Cherax quadricarinatus (Von Martens, 1868) (Parastacidae, Astacoidea) in a freshwater system in the Caribbean drainage of Costa Rica. Bioinvasions Rec. 6, 351–355. doi: 10.3391/bir.2017.6.4.08
Banha, F., and Anastácio, P. M. (2011). Interactions between invasive crayfish and native river shrimp. Knowl. Manag. Aquat. Ecosyst. 401:17. doi: 10.1051/kmae/2011033
Barquero, M. D., and Araya, M. F. (2016). First record of the Greenhouse frog, Eleutherodactylus planirostris (Anura: Eleutherodactylidae), in Costa Rica. Herpetol. Notes 9, 145–147.
Barrientos-Llosa, Z., and Monge-Nájera, J. (2010). Especies introducidas en la Meseta Central de Costa Rica. Biocenosis 23, 32–37.
Beakes, G. W., Glockling, S. L., and Sekimoto, S. (2012). The evolutionary phylogeny of the oomycete “fungi”. Protoplasma 249, 3–19. doi: 10.1007/s00709-011-0269-2
Bott, R. (1950). Die Flußkrebse Europas (Decapoda, Astacidae). Abh. Senckenberg. Naturforsch. Gesell. 483, 1–36.
Brannelly, L. A., McMahon, T. A., Hinton, M., Lenger, D., and Richards-Zawacki, C. L. (2015). Batrachochytrium dendrobatidis in natural and farmed Louisiana crayfish populations: prevalence and implications. Dis. Aquat. Org. 112, 229–235. doi: 10.3354/dao02817
Casabella-Herrero, G., Martínez-Ríos, M., Viljamaa-Dirks, S., Martín-Torrijos, L., and Diéguez-Uribeondo, J. (2021). Aphanomyces astaci mtDNA: insights into the pathogen’s differentiation and its genetic diversity from other closely related oomycetes. Fungal Biol. 125, 316–325. doi: 10.1016/j.funbio.2020.11.010
Cerenius, L., Bangyeekhun, E., Keyser, P., Söderhäll, I., and Söderhäll, K. (2003). Host prophenoloxidase expression in freshwater crayfish is linked to increased resistance to the crayfish plague fungus, Aphanomyces astaci. Cell. Microbiol. 5, 353–357. doi: 10.1046/j.1462-5822.2003.00282.x
Chucholl, C. (2013). Invaders for sale: trade and determinants of introduction of ornamental freshwater crayfish. Biol. Invasions 15, 125–141. doi: 10.1007/s10530-012-0273-2
Correia, A. M., and Anastácio, P. M. (2008). Shifts in aquatic macroinvertebrate biodiversity associated with the presence and size of an alien crayfish. Ecol. Res. 23, 729–734. doi: 10.1007/s11284-007-0433-5
Correia, A. M., Bandeira, N., and Anastácio, P. M. (2005). Predator-prey interactions of Procambarus clarkii with aquatic macroinvertebrates in single and multiple prey systems. Acta Oecol. 28, 337–343. doi: 10.1016/j.actao.2005.06.002
Crandall, K. A., and Buhay, J. E. (2008). Global diversity of crayfish (Astacidae, Cambaridae, and Parastacidae-Decapoda) in freshwater. Hydrobiologia 595, 295–301. doi: 10.1007/s10750-007-9120-3
Crandall, K. A., and De Grave, S. (2017). An updated classification of the freshwater crayfishes (Decapoda: Astacidea) of the world, with a complete species list. J. Crustac. Biol. 37, 615–653. doi: 10.1093/jcbiol/rux070
Diéguez-Uribeondo, J. (2006). The dispersion of the Aphanomyces astaci-carrier Pacifastacus leniusculus by humans represents the main cause of disappearance of the indigenous crayfish Austropotamobius pallipes in Navarra. B. Fr. Pêche Piscic. 380-381, 1303–1312. doi: 10.1051/kmae:2006036
Diéguez-Uribeondo, J., and Söderhäll, K. (1999). RAPD evidences for the origin of an outbreak of aphanomycosis in Spain. Freshw. Crayfish 12, 313–318.
Diéguez-Uribeondo, J., Cerenius, L., Dyková, I., Gelder, S. R., Henttonen, P., Jiravanichpaisal, P., et al. (2006). “Pathogens, parasites and ectocomensals,” in Atlas of Crayfish in Europe, eds C. Souty-Grosset, D. M. Holdich, P. Y. Noël, J. D. Reynolds, and P. Haffner (Paris: Muséum national d’ Histoire naturelle, Patrimoines naturelle), 133–149.
Diéguez-Uribeondo, J., García, M. A., Cerenius, L., Kozubíková, E., Ballesteros, I., Windels, C., et al. (2009). Phylogenetic relationships among plant and animal parasites, and saprotrophs in Aphanomyces (Oomycetes). Fungal Genet. Biol. 46, 365–376.
Diéguez-Uribeondo, J., Huang, T.-S., Cerenius, L., and Söderhäll, K. (1995). Physiological adaptation of an Aphanomyces astaci strain isolated from the freshwater crayfish Procambarus clarkii. Mycol. Res. 99, 574–578. doi: 10.1016/S0953-7562(09)80716-8
Diéguez-Uribeondo, J., Temiño, C., and Múzquiz, J. L. (1997). The crayfish plague fungus (Aphanomyces astaci) in Spain. B. Fr. Pêche Piscic. 70, 753–763. doi: 10.1051/kmae/1997051
European Alien Species Information Network (EASIN) (2021). Codes of conduct and guidelines. Available online at: https://easin.jrc.ec.europa.eu/easin/Documentation/Codesofconduct (accessed March 27, 2021).
EUR-lex (2014). Regulation (EU) No 1143/2014 of the European Parliament and of the Council of 22 October 2014 on the Prevention and Management of the Introduction and Spread of Invasive Alien Species. Edited by European Parliament and of the Council of the European Union. No 1143/2014. Luxembourg: Official Journal of the European Union.
EUR-lex (2016). Commission Implementing Regulation (EU)2016/1141 of 13 July 2016 COMMISSION IMPLEMENTING REGULATION (EU) 2016/1141 of 13 July 2016 Adopting a List of Invasive Alien Species of Union Concern Pursuant to Regulation (EU) No 1143/2014 of the European Parliame. Edited by European Parliament and of the Council of the European Union. No 1141/2016. Luxembourg: Official Journal of the European Union.
Ficetola, G. F., Siesa, M. E., Padoa-Schioppa, E., and De Bernard, F. (2012). Wetland features, amphibian communities and distribution of the alien crayfish, Procambarus clarkii. Alytes 29, 75–87.
Gherardi, F., Aquiloni, L., Diéguez-Uribeondo, J., and Tricarico, E. (2011). Managing invasive crayfish: Is there a hope? Aquat. Sci. 73, 185–200. doi: 10.1007/s00027-011-0181-z
Holdich, D. M., Reynolds, J. D., Souty-Grosset, C., and Sibley, P. J. (2009). A review of the ever increasing threat to European crayfish from non-indigenous crayfish species. Knowl. Manag. Aquat. Ecosyst. 11, 394–395. doi: 10.1051/kmae/2009025
Hsieh, C. Y., Huang, C. W., and Pan, Y. C. (2016). Crayfish plague Aphanomyces astaci detected in redclaw crayfish, Cherax quadricarinatus in Taiwan. Invertebr. Pathol. 136, 117–123. doi: 10.1016/j.jip.2016.03.015
Huang, T.-S., Cerenius, L., and Söderhäll, K. (1994). Analysis of genetic diversity in the crayfish plague fungus, Aphanomyces astaci, by random amplification of polymorphic DNA. Aquaculture 126, 1–9. doi: 10.1016/0044-8486(94)90243-7
Huner, J. V. (1977). Introductions of the Louisiana red swamp crayfish, Procambarus clarkii (Girard): an update. Freshw. Crayfish 3, 193–202. doi: 10.5869/fc.1977.v3.193
Ilhéu, M., Bernardo, J. M., and Fernandes, S. (2007). “Predation of invasive crayfish on aquatic vertebrates: the effect of Procambarus clarkii on fish assemblages in Mediterranean temporary streams,” in Biological Invaders in Inland Waters: Profiles, Distribution, and Threats, ed. F. Gherardi (Dordrecht: Springer), 543–558. doi: 10.1007/978-1-4020-6029-8_29
Jussila, J., Vrezec, A., Makkonen, J., Kortet, R., and Kokko, H. (2015). “8. “Invasive crayfish and their invasive diseases in Europe with the focus on the virulence evolution of the crayfish plague,” in Biological Invasions in Changing Ecosystems, ed. J. Canning-Clode (Berlin: Sciendo Migration), 183–211.
Kawai, T., and Crandall, K. A. (2016). “Global diversity and conservation of freshwater crayfish (Crustacea: Decapoda: Astacoidea),” in A Global Overview of the Conservation of Freshwater Decapod Crustaceans, eds T. Kawai and N. Cumberlidge (Cham: Springer International Publishing), 65–114. doi: 10.1007/978-3-319-42527-6
Kearse, M., Moir, R., Wilson, A., Stones-Havas, S., Cheung, M., Sturrock, S., et al. (2012). Geneious Basic: an integrated and extendable desktop software platform for the organization and analysis of sequence data. Bioinformatics 28, 1647–1649. doi: 10.1093/bioinformatics/bts199
Kopf, R. K., Nimmo, D. G., Humphries, P., Baumgartner, L. J., Bode, M., Bond, N. R., et al. (2017). Confronting the risks of large-scale invasive species control. Nat. Ecol. Evol. 1:172. doi: 10.1038/s41559-017-0172
Kouba, A., Petrusek, A., and Kozák, P. (2014). Continental-wide distribution of crayfish species in Europe: update and maps. Knowl. Manag. Aquat. Ecosyst. 413:05. doi: 10.1051/kmae/2014007
Kozubíková, E., Filipová, L., Kozák, P., Ďuriš, Z., Martín, M. P., Diéguez-Uribeondo, J., et al. (2009). Prevalence of the crayfish plague pathogen Aphanomyces astaci in invasive American crayfishes in the Czech Republic. Conserv. Biol. 23, 1204–1213. doi: 10.1111/j.1523-1739.2009.01240.x
Kozubikova, E., Viljamaa-Dirks, S., Heinikainen, S., and Petrusek, A. (2011). Spiny-cheek crayfish Orconectes limosus carry a novel genotype of the crayfish plague pathogen Aphanomyces astaci. J. Invertebr. Pathol. 108, 214–216. doi: 10.1016/j.jip.2011.08.002
Lara, L. R., and Wehrtmann, I. S. (2011). “Diversity, abundance and distribution of river shrimps (Decapoda: Caridea) in the largest river basin of Costa Rica, Central America,” in New Frontiers in Crustacean Biology, eds A. Asakura and C. Fransen (Leiden: Koninklijke Brill NV), 197–211.
Lara, L. R., Wehrtmann, I. S., Magalhães, C., and Mantelatto, F. L. (2013). Species diversity and distribution of freshwater crabs (Decapoda: Pseudothelphusidae) inhabiting the basin of the río Grande de Térraba, Pacific. Lat. Am. J. Aquat. Res. 41, 685–695.
Lilley, J. H., Cerenius, L., and Söderhäll, K. (1997). RAPD evidence for the origin of crayfish plague outbreaks in Britain. Aquaculture 157, 181–185. doi: 10.1016/S0044-8486(97)00153-1
Lowe, S., Browne, M., Boudjelas, S., and De Poorter, M. (2000). 100 of the World’s Worst Invasive Alien Species a Selection from the Global Invasive Species Database. The Invasive Species Specialist Group (ISSG) a specialist group of the Species Survival Commission (SSC) of the World Conservation Union (IUCN). Auckland: ISSG.
Machino, Y., and Diéguez-Uribeondo, J. (1998). Un cas de peste des écrevisses en France dans le bassin de la Seine. L’Astaciculteur France 54, 2–11.
Magalhães, C., Wehrtmann, I. S., Lara, L. R., and Mantelatto, F. (2015). Taxonomy of the freshwater crabs of Costa Rica, with a revision of the genus Ptychophallus Smalley, 1964 (Crustacea: Decapoda: Pseudothelphusidae). Zootaxa 3905, 301–344. doi: 10.11646/zootaxa.3905.3.1
Makkonen, J., Jussila, J., Panteleit, J., Keller, N. S., Schrimpf, A., Theissinger, K., et al. (2018). MtDNA allows the sensitive detection and haplotyping of the crayfish plague disease agent Aphanomyces astaci showing clues about its origin and migration. Parasitology 145, 1210–1218. doi: 10.1017/S0031182018000227
Marino, F., Pretto, T., Tosi, F., Monaco, S., De Stefano, C., Manfrin, A., et al. (2014). Mass mortality of Cherax quadricarinatus (von Martens, 1868) reared in Sicily (Italy): Crayfish plague introduced in an intensive farming. Freshw. Crayfish 20, 93–96. doi: 10.5869/fc.2014.v20-1.93
Martín-Torrijos, L., Correa-Villalona, A. J., Pradillo, A., and Diéguez-Uribeondo, J. (2021a). Coexistence of two invasive species, Procambarus clarkii and Aphanomyces astaci, in Brackish waters of a mediterranean coastal lagoon. Front. Ecol. Evol. 8:622434. doi: 10.3389/fevo.2020.622434
Martín-Torrijos, L., Kawai, T., Makkonen, J., Jussila, J., Kokko, H., and Dieguez-Uribeondo, J. (2018). Crayfish plague in Japan: a real threat to the endemic Cambaroides japonicus. PLoS One 13:e0195353. doi: 10.1371/journal.pone.0195353
Martín-Torrijos, L., Kokko, H., Makkonen, J., Jussila, J., and Diéguez-Uribeondo, J. (2019). Mapping 15 years of crayfish plague in the Iberian Peninsula: the impact of two invasive species on the endangered native crayfish. PLoS One 14:e0219223. doi: 10.1371/journal.pone.0219223
Martín-Torrijos, L., Martinez-Ríos, M., Casabella-Herrero, G., Adams, S. B., Jackson, C. R., and Diéguez-Uribeondo, J. (2021b). Chasing the crayfish plague: Southeastern United States as the center for Aphanomyces astaci diversity. Sci. Rep. 11:9332. doi: 10.1038/s41598-021-88704-8
Mazza, G., Scalici, M., Inghilesi, A. F., Aquiloni, L., Pretto, T., Monaco, A., et al. (2018). The red alien vs. the blue destructor: the eradication of Cherax destructor by Procambarus clarkii in Latium (Central Italy). Diversity 10:126. doi: 10.3390/d10040126
Mrugała, A., Buřič, M., Petrusek, A., and Kouba, A. (2019). May atyid shrimps act as potential vectors of crayfish plague? NeoBiota 51, 65–80. doi: 10.3897/neobiota.51.37718
Myers, N., Mittermeier, R. A., Mittermeier, C. G., da Fonseca, G. A. B., and Kent, J. (2000). Biodiversity hotspots for conservation priorities. Nature 403, 853–858.
Nagy, R., Fusaro, A., Conard, W., and Morningstar, C. (2020). Procambarus Clarkii (Girard, 1852). U.S. Geological Survey, Nonindigenous Aquatic Species Database. Available online at: https://nas.er.usgs.gov/queries/factsheet.aspx?SpeciesID=217 (accessed October 20, 2020).
Nunes, A. L., Orizaola, G., Laurila, A., and Rebelo, R. (2014). Morphological and life-history responses of anurans to predation by an invasive crayfish: an integrative approach. Ecol. Evol. 4, 1491–1503. doi: 10.1002/ece3.979
Oidtmann, B., Cerenius, L., Schmid, I., Hoffmann, R., and Söderhäll, K. (1999). Crayfish plague epizootics in Germany–classification of two German isolates of the crayfish plague fungus Aphanomyces astaci by random amplification of polymorphic DNA. Dis. Aquat. Organ. 35, 235–238. doi: 10.3354/dao035235
OIE (World Organization for Animal Health) (2019). Manual of Diagnostic tests for Aquatic Animals: Infection with Aphanomyces astaci (crayfish plague). Available online at: https://www.oie.int/en/standard-setting/aquatic-manual/access-online/?htmfile=chapitre_aphanomyces_astaci.htm (accessed March 27, 2021).
Peiró, D. F., Almerão, M. P., Delaunay, C., Jussila, J., Makkonen, J., Bouchon, D., et al. (2016). First detection of the crayfish plague pathogen Aphanomyces astaci in South America: a high potential risk to native crayfish. Hydrobiologia 781, 181–190. doi: 10.1007/s10750-016-2841-4
Putra, M. D., Bláha, M., Wardiatno, Y., Krisanti, M., Yonvitner, Jerikho, R., et al. (2018). Procambarus clarkii (Girard, 1852) and crayfish plague as new threats for biodiversity in Indonesia. Aquat. Conserv. Mar. Freshw. Ecosyst. 28, 1434–1440. doi: 10.1002/aqc.2970
Queensland Government (2019). Business Queensland. Red swamp crayfish. Available online at: https://www.business.qld.gov.au/industries/farms-fishing-forestry/agriculture/land-management/health-pests-weeds-diseases/pests/invasive-animals/prohibited/red-swamp-crayfish (accessed March 27, 2021).
República de Costa Rica (2005). Reglamento a La Ley de Conservación de La Vida Silvestre. Available online at: http://www.pgrweb.go.cr/scij/Busqueda/Normativa/Normas/nrm_texto_completo.aspx?param1=NRTC&nValor1=1&nValor2=84592&nValor3=109223&strTipM=TC (accessed October 20, 2020).
Reynolds, J. D. (2011). A review of ecological interactions between crayfish and fish, indigenous and introduced. Knowl. Manag. Aquat. Ecosyst. 401, 1–21. doi: 10.1051/kmae/2011024
Rezinciuc, S., Galindo, J., Montserrat, J., and Diéguez-Uribeondo, J. (2014). AFLP-PCR and RAPD-PCR evidences of the transmission of the pathogen Aphanomyces astaci (Oomycetes) to wild populations of European crayfish from the invasive crayfish species, Procambarus clarkii. Fungal Biol. 118, 612–620. doi: 10.1016/j.funbio.2013.10.007
Rezinciuc, S., Sandoval-Sierra, J. V., Oidtmann, B., and Diéguez-Uribeondo, J. (2015). “The biology of crayfish plague pathogen Aphanomyces astaci: current answers to most frequent questions,” in Freshwater Crayfish: A Global Overview, eds T. Kawai, Z. Faulkes, and G. Scholtz (Boca Raton, FL: CRC Press), 182–204.
Schrimpf, A., Schmidt, T., and Schulz, R. (2014). Invasive chinese mitten crab (Eriocheir Sinensis) transmits crayfish plague pathogen (Aphanomyces astaci). Aquat. Invasions 9, 203–209. doi: 10.3391/ai.2014.9.2.09
Shull, C. (1909). Some abnormalities and regeneration of pleiopods in cambarus and other decapoda. Biol. Bull. 16, 297–312. doi: 10.2307/1536190
Souty-Grosset, C., Anastácio, P. M., Aquiloni, L., Banha, F., Choquer, J., Chucholl, C., et al. (2016). The red swamp crayfish Procambarus clarkii in Europe: impacts on aquatic ecosystems and human well-being. Limnologica 58, 78–93. doi: 10.1016/j.limno.2016.03.003
Svoboda, J., Mrugała, A., Kozubíková-Balcarová, E., Kouba, A., Diéguez-Uribeondo, J., and Petrusek, A. (2014). Resistance to the crayfish plague pathogen, Aphanomyces astaci, in two freshwater shrimps. J. Invertebr. Pathol. 121, 97–104. doi: 10.1016/j.jip.2014.07.004
Taugbøl, T., Skurdal, J., and Håstein, T. (1993). Crayfish plague and management strategies in Norway. Biol. Conserv. 63, 75–82. doi: 10.1016/0006-3207(93)90076-D
Torres, E., and Álvarez, F. (2012). Genetic variation in native and introduced populations of the red swamp crayfish Procambarus clarkii (Girard, 1852) (Crustacea, Decapoda, Cambaridae) in Mexico and Costa Rica. Aquat. Invasions 7, 235–241. doi: 10.3391/ai.2012.7.2.009
Unestam, T. (1969). Resistance to the crayfish plague in some American, Japanese and European crayfishes. Rep. Inst. Freshw. Res. 49, 202–209.
Unestam, T. (1972). On the host range and origin of the crayfish plague fungus. Rep. Inst. Freshw. Res. 52, 192–198.
Unestam, T. (1975). Defence reactions in and susceptibility of Australian and New Guinean freshwater crayfish to European-crayfish-plague fungus. Aust. J. Exp. Biol. Med. Sci. 53, 349–359. doi: 10.1038/icb.1975.40
Vennerström, P., Söderhäll, K., and Cerenius, L. (1998). The origin of two crayfish plague (Aphanomyces astaci) epizootics in Finland on noble crayfish, Astacus astacus. Ann. Zool. Fenn. 35, 43–46.
Villalobos-Rojas, F. (2019). Propuesta de Plan de Manejo Para las Langostas Exóticas de Agua Dulce Cherax Quadricarinatus (von Martens, 1868) y Procambarus clarkii (Girard, 1852) Para el Sistema Nacional de Áreas De Conservación (SINAC), Costa Rica. Master’s thesis. San José: Universidad para la Cooperación Internacional.
Wehrtmann, I. S., Ramírez, A., and Pérez-Reyes, O. (2016). “Freshwater decapod diversity and conservation in Central America and the Caribbean,” in A Global Overview of the Conservation of Freshwater Decapod Crustaceans, eds T. Kawai and N. Cumberlidge (Cham: Springer Nature), 267–301. doi: 10.1007/978-3-319-42527-6
Young, T. R. (2006). National and Regional Legislation for Promotion and Support to the Prevention, Control, and Eradication of Invasive Species. Biodiversity Series: The International Bank for Reconstruction and Development/THE WORLD BANK, n∘108. Washington, DC: The world bank environment department.
Keywords: biodiversity, invasive alien species, crayfish plague, Procambarus clarkii, Cherax quadricarinatus, mtDNA, conservation
Citation: Martín-Torrijos L, Correa-Villalona AJ, Azofeifa-Solano JC, Villalobos-Rojas F, Wehrtmann IS and Diéguez-Uribeondo J (2021) First Detection of the Crayfish Plague Pathogen Aphanomyces astaci in Costa Rica: European Mistakes Should Not Be Repeated. Front. Ecol. Evol. 9:623814. doi: 10.3389/fevo.2021.623814
Received: 30 October 2020; Accepted: 17 May 2021;
Published: 17 June 2021.
Edited by:
Leopold Füreder, University of Innsbruck, AustriaReviewed by:
Elena Tricarico, University of Florence, ItalyAdam Petrusek, Charles University, Czechia
Copyright © 2021 Martín-Torrijos, Correa-Villalona, Azofeifa-Solano, Villalobos-Rojas, Wehrtmann and Diéguez-Uribeondo. This is an open-access article distributed under the terms of the Creative Commons Attribution License (CC BY). The use, distribution or reproduction in other forums is permitted, provided the original author(s) and the copyright owner(s) are credited and that the original publication in this journal is cited, in accordance with accepted academic practice. No use, distribution or reproduction is permitted which does not comply with these terms.
*Correspondence: Javier Diéguez-Uribeondo, dieguez@rjb.csic.es