Chironomidae Morphological Types and Functional Feeding Groups as a Habitat Complexity Vestige
- 1Department of Invertebrate Zoology and Hydrobiology, University of Lodz, Łódź, Poland
- 2Laboratory of Paleoecology and Archaeobotany, Department of Plant Ecology, Faculty of Biology, University of Gdańsk, Gdańsk, Poland
- 3Institute of Marine and Environmental Sciences, University of Szczecin, Szczecin, Poland
- 4W. Szafer Institute of Botany, Polish Academy of Sciences, Kraków, Poland
- 5Laboratory of Biogeochemistry, Adam Mickiewicz University, Poznań, Poland
- 6Past Landscape Dynamics Laboratory, Institute of Geography and Spatial Organization, Polish Academy of Sciences, Warsaw, Poland
- 7Department of Geology and Geomorphology, Faculty of Geographical Sciences, University of Lodz, Łódź, Poland
- 8Faculty of Geology, Geophysics and Environmental Protection, AGH University of Science and Technology, Kraków, Poland
Chironomid (Diptera: Chironomidae) larvae play an important role in a wide range of aquatic ecosystems. The study focuses on Chironomidae trophic guilds and morphological types as indicator traits in reconstructions of habitat changes in shallow water bodies. Mentum and ventromental plates are important mouthparts whose shape depends on food type and feeding behavior. Chironomidae larvae strongly vary in the mode of life and feeding habits, representing almost every feeding group. Here we classified the mentum types into 16 groups and tested if they indicated similar past habitat changes as the Chironomidae functional feeding groups (FFGs), and tribes/subfamilies. Paleoecological data of biotic and abiotic proxies were derived from short sequences from a Late Glacial oxbow and a nearby medieval moat located in Central Poland. The study revealed that the habitat substratum structure, vegetation and physicochemical conditions are associated both with the feeding types and morphological traits. This provides a valuable tool for future reconstructions of habitat changes.
Introduction
Chironomidae are one of the most abundant and ubiquitous groups of aquatic insects. Their sensitivity to changing limnological conditions and species-specific environmental preferences make them good ecological indicators (Armitage et al., 1995; Porinchu and MacDonald, 2003). A variety of environmental variables affects Chironomidae larvae both in a direct and indirect way. Among local-scale abiotic factors, some of the most important for chironomids are pH, lake depth and water nutrient status (Walker, 2001; Brooks et al., 2007; Płóciennik et al., 2020). As there are some truly rheophile taxa, chironomid subfossils can indicate a connection of peripheral river valley water bodies to the main stream during overbank episodes (Gandouin et al., 2006; Howard et al., 2010; Kittel et al., 2016). They also indicate a stagnant water level in a quantitative way (Luoto, 2009a). While a relation of littoral versus profundal taxa reflects lake-level changes, semi-terrestrial taxa indicate paludification and terrestrialisation processes (Frouz, 1999; Brooks et al., 2007; Lamentowicz et al., 2009). When pH drops below 6.0, chironomid assemblages are mostly replaced by Chaoboridae or Ceratopogonidae (Henrikson et al., 1982; Walker et al., 1985). Even though most chironomid larvae prefer circum-neutral pH, certain taxa are characteristic to acidic or slightly alkaline conditions (Walker, 2001; Brooks et al., 2007). Generally, water pH exceeding 7.5 eliminates many taxa, reducing species richness. It is associated with increased eutrophication and oxygen deficiencies (Brooks et al., 2007). The extraordinarily wide spectrum of preferred water trophic states makes chironomids good indicators of lake nutrient status (Saether, 1979; Walker, 2001; Porinchu and MacDonald, 2003).
Chironomidae are applied in inference models developed as a tool for many quantitative climatic and environmental reconstructions (e.g., Brooks et al., 2001; Luoto, 2009b; Heiri et al., 2011; Kotrys et al., 2020). However, the use of chironomid autecology for reconstruction of aquatic habitats is a time-consuming method and requires high competition in subfossil identification. The acquisition of necessary experience for chironomid identification takes years of work and there are still too few specialists to meet the needs of European paleoecological projects. But, while standard analysis is impossible, the functional trait approach may be applied. This method allows for tracking past shifts in the functional groups of organisms, and thus studying the long-term ecosystem response to past environmental changes (Gregory-Eaves and Beisner, 2011). So far, functional traits of several groups of organisms have been studied according to their application in paleoecology (e.g., Vogt et al., 2010; Fournier et al., 2015; Nevalainen and Luoto, 2017). The functional traits carried by chironomid larvae have so far been studied mainly by Serra et al. (2016, 2017). Here we want to verify the applicability of this method in Chironomidae subfossil analysis.
Functional traits are the features of organisms indicating environmental changes (response traits) or driving such changes in the ecosystems (effect traits) (Nock et al., 2016). They are widely used in community ecology, studying the ecological niche (Kearney et al., 2010), improving ecological process analyses and quantifying the influence of assemblage shifts (Díaz and Cabido, 2001; Nock et al., 2016). Community-trait approach, which takes into considering community weighted means of traits (CWMs), is a noteworthy method (Garnier et al., 2007; Kleyer et al., 2012). Several indices were constructed for functional diversity calculation (reviewed in Pla et al., 2012), providing a broader view for ecosystem complexity than species richness (e.g., Stuart-Smith et al., 2013). The Chironomidae taxonomic and functional diversity across various environmental gradients have been already studied in several regions of the world (e.g., Milošević et al., 2018; Jiang et al., 2019; Motta and Massaferro, 2019; Ni et al., 2020), while Nevalainen et al. (2015) focused on the results provided by the subfossil chironomid assemblages. Kivilä et al. (2019, 2020) used chironomid functional feeding groups (FFGs) to track not only recent habitat changes, but also climate warming in northern Finland. However, food preferences of some taxa are still not well-specified. To avoid loss of data, morphological traits such as the mouthpart structure may serve as a good surrogate. Several studies have proven that morphological features of some organisms, such as testate amoebae (e.g., Fournier et al., 2015; Lamentowicz et al., 2015), cladocerans (Nevalainen and Luoto, 2017) and plants (e.g., Woodward, 1987; Reitalu et al., 2015; Carvalho et al., 2019) can be used to infer past environmental changes, both on a micro- and macroscale. The study by Barboni et al. (2004) indicates that plant response to climate changes (reflected in pollen analysis) is more diagnostic in combinations of traits rather than in individual traits. This assumption is likely applicable to every biological proxy, including chironomids. Consequently, future studies on shifts in habitat structure should apply appropriate methods that take into consideration the complexity of aquatic ecosystems.
Trait-based studies help researchers understand the mechanisms of ecological processes and, therefore, their potential in paleoenvironmental reconstructions cannot be ignored (Marcisz et al., 2020). The studied organisms were affected over time by a range of direct and indirect variables. One of such indirect factors was human activity, which impacted aquatic habitats in many ways. Therefore, the study includes both truly natural and artificial water bodies. The results of the previous study concerning the moat system of the motte-and-bailey stronghold in Rozprza (Kittel et al., 2018a) were utilized. In its case, human impact turned out to be one of the main factors. The inhabitants caused the increase of water trophy, but also changed water dynamics by creating artificial channels (Kittel et al., 2018a). In order to check the applicability of the proposed method in a natural ecosystem, the results of paleoecological studies of the nearby paleo-oxbow were also included.
Morphological characters and well-established ecological preferences are key traits in paleolimnology (Gregory-Eaves and Beisner, 2011). The study focuses on the morphological traits (mouthparts type) which are thought to be (at least partially) dependent on feeding preferences, and thus also associated with the habitat character. The habitat structure is expressed not only by physicochemical features but also by biological variables, such as macrophytes and diatoms, included in our study. Langdon et al. (2010) state that biotic variables are even more important than the abiotic ones in determining zoobenthos communities in temperate shallow lakes, and chironomids are very good indicators of past changes in the primary production, as well as macrophyte abundance and structure. Our primary goal is to recognize if the traits carried by the Chironomidae at different organization levels indicate similar habitat changes in the context of paleoenvironmental reconstructions.
Chironomidae vary not only in their environmental preferences but also in their diet type. Although the larval feeding behavior depends on many factors, such as larval size, food quality, and availability (Berg, 1995), each species seems to have specific preferences, often associated with its habitat type. Representatives of almost every feeding group can be found among chironomids. There are sediment collectors such as Chironomus plumosus-type, phytophagous shredders such as Glyptotendipes caulicola-type, and scrapers (many Orthocladiinae). Such a great variety is associated with their diverse modes of life – some of them live in tubes built out of sediment (e.g., Chironomus and Tanytarsini), while others are free-living and predatory, such as Tanypodinae. Chironomids include detritus feeders, parasites, commensals, and plant miners (Berg, 1995; Walker, 2001; Beiger, 2004; Vallenduuk and Moller Pillot, 2007; Moller Pillot, 2009, 2013; Schiffels, 2014).
Larvae morphology, especially their mouthparts, had to be adapted to their diet and feeding behavior. The relationship between mandibles and chironomid autecology was studied using the geometric morphometrics approach (Đurđević et al., 2017). Mandibles and labrum are important features, useful in diagnostics, but they are often missing from the subfossil material. Other mouthparts, likely related to the diet, are mentum and ventromental plates. This is the reason for choosing them as the key characteristics for morphological types in our study. The shape of the mentum may be similar even between taxonomically distant taxa. For example, the concave mentum of predatory Cryptochironomus resembles the dorsomentum of many Tanypodinae (e.g., Procladius), while striated plates are often characteristic of filtrating Chironominae (Olafsson, 1992; Moller Pillot, 2009).
Considering this, we can assume that some generalization in the morpho-functional traits of Chironomidae can be done. However, we need to be careful with some morphologically close species which vary in their feeding behavior (Monakov, 1972). In paleoecological studies, chironomids are mostly identified to the morphotype level, which usually includes closely related species with similar ecology. On the other hand, it is sometimes impossible to distinguish subfossil head capsules of ecologically different taxa, such as Micropsectra insignilobus and M. atrofasciata, Chironomus tenuistylus and Ch. plumosus, or Stempelinella and Zavrelia (Brooks et al., 2007). Nevertheless, chironomid mouthparts are closely related to their feeding type, which often reflects the habitat structure. It raises the question if the knowledge of certain species’ autecology is always required for reconstructions of environmental conditions? Perhaps some general assessments can be done, even by non-specialists, on the basis of mentum shape. In this case, such preliminary chironomid analyses based on the proposed mentum classification could bring valuable insight into past ecosystems, even when undertaken during a macrofossil analysis.
Mentum and ventromental plates are also the main differing features between subfamilies and tribes. Although there are eleven Chironomidae subfamilies, three of them are the most species-rich: Chironominae, Orthocladiinae, and Tanypodinae (Brooks et al., 2007; Ferrington, 2008). In this study, we focus on these subfamilies, as their representatives were found in examined sediments. Chironominae are usually characterized by distinctive, striated, large ventromental plates and a slightly convex mentum. The mentum of Orthocladiinae is often strongly convex, their head capsules are usually smaller, and ventromental plates are mostly inconspicuous. Tanypodinae have a toothed ligula, a less developed mentum, and no ventromental plates. Some chironomid subfamilies are divided into tribes. In the studied sequences, taxa belonging to Macropelopiini, Natarsiini, Pentaneurini, Procladiini, Tanypodini (Tanypodinae), as well as to Chironomini and Tanytarsini (Chironominae), and to Orthocladiinae were recorded. Among Tanypodinae, only Macropelopiini, Procladiini and Tanypodini have dorsomental teeth. Natarsiini and Pentaneurini are characterized by a bright, elongated head and a membranous toothless mentum that is not well-preserved in subfossil material. Chironomini have relatively large, fan-shaped ventromental plates, while the plates of Tanytarsini are usually bar-shaped and straight (Brooks et al., 2007; Andersen et al., 2013). The Orthocladiinae are rarely divided into tribes (Spies, 2005), so we left this group undivided at the subfamily level.
Finally, the goal of this study is to check whether (and how) some chironomid groups (mentum types, trophic guilds, or tribes/subfamilies) are correlated with other indicators within studied proxies (macrophytes and other plants, diatoms, sediment type or geochemical components). The applicability of morphological functional traits in paleoecological research was verified. Presumably, they could serve as surrogate indicators where it could be applicable.
Study Area
The study area (51°18′07″ N; 19°40′04″ E; 182–183 m a.s.l.) is situated in Central Poland, approx. 60 km south of Łódź. It is located in the valley floor of the Luciąża River, a 3rd-order river in the Vistula River basin.
The region is a part of the Odranian (Saalian) formerly glaciated area – the last ice sheet was present here in the Wartanian Cold Stage of the Odranian Glaciation (Marks, 2011). Intense transformation of river valleys took place during the Weichselian glacial period under periglacial conditions. While the valley floor is strongly expanding in the Rozprza area, residual terraces occur within the Holocene floodplain (Goździk, 1982; Kittel et al., 2018b).
In the opinion of Wachecka-Kotkowska (2004a, b), the morphology of the Luciąża valley floor was obliterated by the deposition of modern overbank alluvium. However, this landscape reconstruction has not been confirmed by a detailed examination of surficial geology in the Rozprza area (Kittel et al., 2018a, b). In the recent research, numerous subfossil paleomeanders of different sizes have been discovered in the valley floor (Figure 1). A large paleochannel (W4) was recorded as a strong curvilinear magnetic anomaly with a width of ca. 10 m, and a radius of ca. 15 m. It is filled with organic mud and coarse-detritus gyttja reaching 1.3 m thickness and underlain by channel alluvia of sands and gravels with organic admixtures and laminations of organic mud. Radiocarbon data evidences the channel’s cut-off in the Late Allerød. The paleochannel fill is overlain by the overbank alluvia of sandy organic mud (Kittel et al., 2018b).
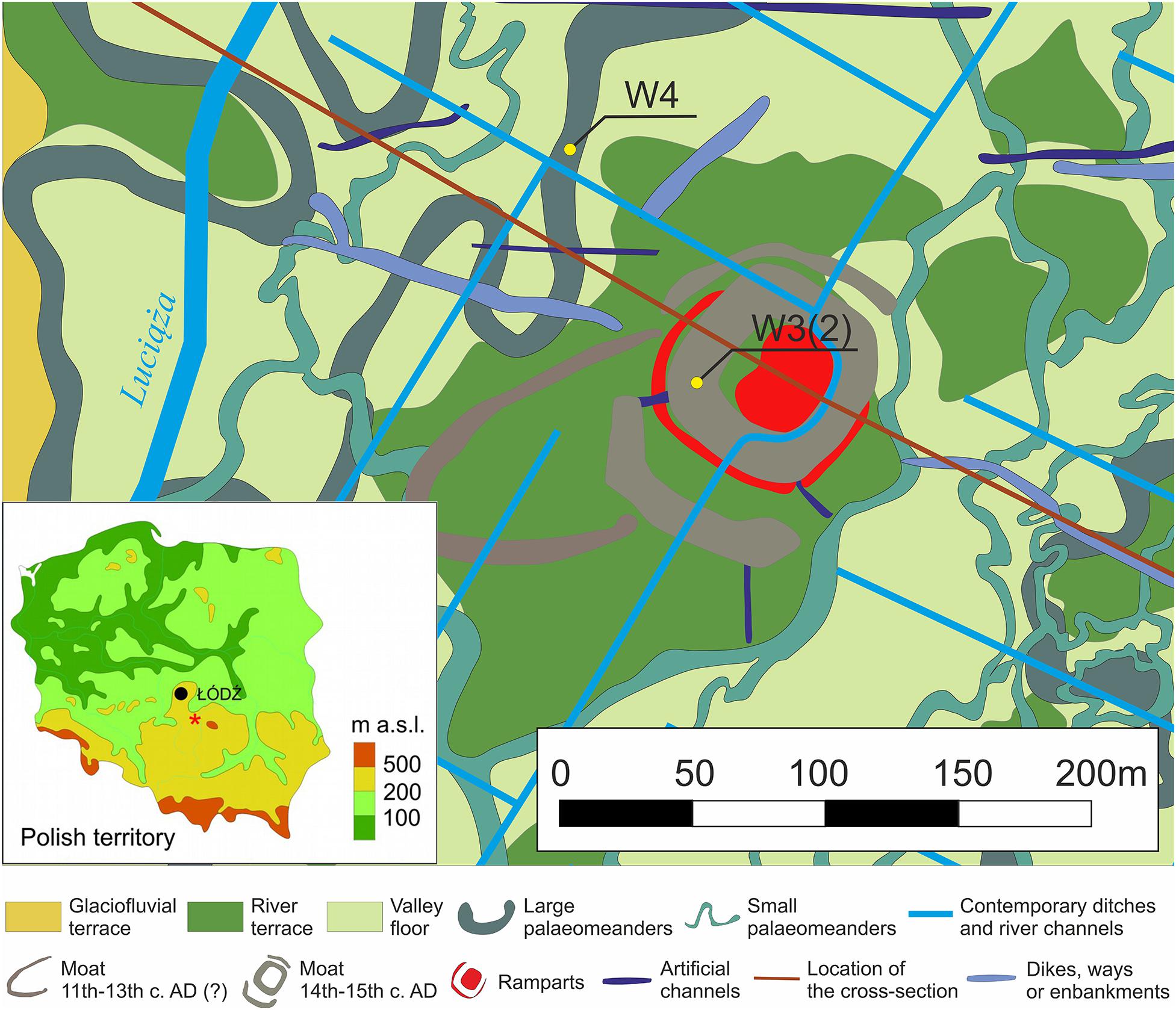
Figure 1. The geomorphological map of the vicinity of Rozprza archaeological site with the location of W4 (paleo-oxbow) and W3(2) (moat) profiles (marked as yellow dots) (after Kittel et al., 2015, 2018b, modified). In the map of Poland, Rozprza is marked with an asterisk.
The Rozprza motte-and-bailey moat system was established in the 1330s AD and is now filled with organic (gyttja and peat) and partially inorganic deposits containing rich remains of wood. The medieval age of the features has been confirmed by dendrochronological and radiocarbon AMS date sets. The fill of the main moat was a subject of a detailed paleoenvironmental study (Kittel et al., 2018a). The accumulation of overbank silty sandy organic mud took place within the moat ditch system as late as in the 18th or 19th c. CE (Kittel et al., 2018a, b).
The potential natural vegetation of the Luciąża River valley would have been mostly lime-oak-hornbeam forests representing the Tilio-Carpinetum association and, to a lesser extent, the Potentillo albae-Quercetum typicum. Immediate surroundings of the water courses would have been overgrown by lowland ash-alder and alder forests of Fraxino-Alnetum and Carici elongatae-Alnetum associations (Matuszkiewicz, 2008).
The sediment cores were taken from the same area, but they are dated to different time periods. The W3(2) sequence originates from the Late Medieval moat, while the W4 core covers the Late Weichselian paleochannel history (Figure 1).
Materials and Methods
Fieldwork and Geochronology
The fieldwork in Rozprza was conducted in 2015–2016, as part of a multidisciplinary archaeological investigation of the medieval ringfort remnants. In order to choose proper sampling sites in the field, not only was topography and geomorphology of studied area thoroughly mapped but also the combination of other non-destructive methods (including aerial photography, geophysical, and geochemical survey) was used (Sikora et al., 2015, 2019; Kittel et al., 2018b).
The sediments for paleoecological analyses were collected from the trench walls as monoliths using metal boxes (50 × 10 × 10 cm each). With this method, the undisturbed structure of the sediments was preserved. Five selected samples of bulk organic deposits collected from the W3(2) profile and two bulk samples from the W4 profile were dated with the radiocarbon (14C) method, using the liquid scintillation technique (LST). Two samples of selected terrestrial plant macrofossils from the W3(2) core and three samples from the W4 core were dated using accelerator mass spectrometry technique (AMS). Moreover, a dendrochronological analysis was performed to determine the age of the moat fill (Kittel et al., 2018a).
Geochemical and Sedimentological Analyses
For geochemical analyses, samples from both cores were dried at 105°C and homogenized. The organic matter content was obtained using the loss on ignition (Heiri et al., 2001). Carbonate content was determined using the volumetric method with Scheibler’s apparatus, and pH by a mean potentiometric method in distilled water. The ash samples were dissolved (with HCl, HNO3, and H2O2) in Teflon bombs using a microwave mineralizer. For the solution analyzed concentrations of such elements as: Na, K, Mg, Ca, Fe, Mn, Cu, Zn, and Pb, the atomic absorption spectrometry (AAS) was used. Grain size composition of the ash samples, remaining after the solution was made in accordance with Clift et al. (2019), was determined using a Mastersizer 3000 laser particle-size analyzer (Malvern). The relationship between the mean grain size and the sorting index (the so-called coordinate system) follows Mycielska-Dowgiałło and Ludwikowska-Kêdzia (2011).
Biotic Proxies
Chironomidae sample processing followed the methods outlined in Brooks et al. (2007). The sediment volume ranged between 1 and 10.5 cm3 per sample. The sediments were passed through a 63 μm mesh sieve. If necessary, kerosene flotation was used according to the method of Rolland and Larocque (2007). Processed sediment was put into a Bogorov counting tray and scanned under a stereo-binocular microscope. Where applicable, at least 50 (preferably 100) chironomid head capsules from each sample were picked and mounted in Euparal® on microscope slides. Identification of chironomid head capsules followed keys by Schmid (1993), Brooks et al. (2007), and Andersen et al. (2013). Chironomidae taxa were classified in three ways: taxonomically (subfamilies and tribes), morphologically and by feeding group. Sixteen morphological types were distinguished based on the larvae mouthpart structure, namely: the mentum ratio (width/height), the median and lateral mentum teeth structure, as well as the size and shape of ventromental plates (Figure 2). Most types were distinguished within Chironomini and Orthocladiinae. Tanypodinae with toothed dorsomentum were grouped together with Cryptochironomus, while for Pentaneurini and Natarsia (with toothless mentum) a separate group was created. Tanytarsini were classified into two types, depending on the shape and length of ventromental plates (Table 1). The recorded taxa were also divided into 12 functional feeding groups (FFGs): collector-gatherers (C), collector-filterers (C/F), collectors/grazers (C/G), shredders/filterers (S/F), shredders/collectors (S/C), grazers/scrapers (G), grazers/collectors (G/C), filterers/collectors (F/C), filterers/grazers (F/G), miners (M), predators (P), and predators/collectors (P/C). The feeding preferences of identified taxa are based mainly on Franquet (1999), Vallenduuk and Moller Pillot (2007), Moller Pillot (2009, 2013), and Serra et al. (2016). The stratigraphic diagrams were created with C2 software (Juggins, 2007).
Samples for a plant macrofossil analysis were wet-sieved in a 200 μm mesh, then boiled with KOH to reduce the amount of sediment and remove humic matter. The material was examined under a microscope. Conservation of plant remains was done with a standard mixture of alcohol, water and glycerine, with addition of thymol. Fragments of plants were then dehydrated in 50% ethyl alcohol. Macrofossils were identified using plant keys, atlases (e.g., Greguss, 1945; Kats et al., 1965; Grosser, 1977; Schweingruber, 1978; Berggren, 1981; Cappers et al., 2006; Velichkevich and Zastawniak, 2006, 2008; Schweingruber et al., 2011), scientific descriptions and publications, a reference collection of modern seeds, fruits, wood and charcoal, and a collection of fossil floras of the W. Szafer Institute of Botany, Polish Academy of Sciences, in Kraków.
A diatom analysis was conducted according to the method by Battarbee (1986). Samples of 1 cm3 each from the cores were processed. Sediments were treated in 10% HCl to remove calcium carbonate and washed several times in distilled water. Afterward, the samples were boiled in 30% H2O2 to digest the organic matter. Finally, the samples were washed several times in distilled water. Microspheres were added to each sample in order to determine the frequency of the diatoms in each sample (Battarbee and Kneen, 1982).
Statistical Analyses
All statistical analyses were made in R software (R Core Team, 2020). Firstly, environmental data were standardized using the robustHD package (Alfons, 2019). The corrplot package (Wei and Simko, 2017) was used to compute Pearson’s correlation matrix in order to check which environmental variables were correlated (correlation >0.70) with each other (Supplementary Figures 1, 2). The most autocorrelated predictors were removed from the analysis. Finally, eight variables: pH, CaCO3, organic matter (OM), K, sand, wood, Carex sp., and U. dioica were included in further analysis (Supplementary Table 1). Next, a dataset for each group of Chironomidae was square-root transformed, in order to reduce the influence of outliers. The “decorana” function from the vegan package (Oksanen et al., 2019) was used to fit the best ordination analysis to the datasets of each group. A Redundancy Analysis (RDA) was performed for each Chironomidae typology using the vegan package (Oksanen et al., 2019). To determine collinearity between environmental factors shaping chironomid composition, variance inflation factors (VIF) analysis was used (Fox and Weisberg, 2011). In addition, ANOVA was conducted to determine statistically significant variables (Oksanen et al., 2019). These variables were used in subsequent RDA analysis. Adjusted R2 indicated how much variability was explained by the RDA analysis. Eigenvalues of RDA axes (RDA1 and RDA2) were computed to compare the proportions of variance explained between each analyzed group of chironomids. Eight environmental factors (pH, CaCO3, organic matter, K, sand, wood, Carex sp., and U. dioica) were used in generalized linear models (GLMs) as fixed effects to assess their influence on the richness of chironomid tribes/subfamilies, mentum types and feeding types. Models were selected using the “dredge” function from the MuMIn package (Bartoń, 2018). Next, the most parsimonious models (with ΔAIC < 2) were averaged into one model to determine statistically significant environmental variables. In addition, the hier.part package (Walsh and Mac Nally, 2013) was used to calculate the independent effects of each variable on the richness as well as its significance. Charts were created using the ggplot2 package (Wickham, 2016).
Results
Chironomidae Stratigraphy
From a short, Late Weichselian paleochannel sequence (W4), 1138 chironomid head capsules were collected and identified to 56 morphospecies. The chironomid fauna in the paleochannel underwent significant fluctuations. After the favorable conditions for chironomid assemblages in the Allerød and Younger Dryas, their number suddenly dropped in the Holocene. Because of a very low chironomid concentration in that period (<50 head capsules), further analyses were based only on the Late Weichselian part of the sequence (ca. 13,000 – 12,200 cal. BP). During this whole period, collectors, represented mainly by Tanytarsini with mentum type 5, clearly dominated. Their relative proportion ranged between 15 and 60%, with the peak in the Late Allerød (194–198 cm b.g.l.). This was the time when the share of subdominant grazers (G, G/C) dropped. Interestingly, in the Younger Dryas (180–190 cm b.g.l.), the share of collectors (here mentum type 5, predominantly Micropsectra) slightly decreased, which coincides with the increase in the proportion of larvae with mentum type 1 (mainly Acricotopus). Besides that, the share of semiterrestrial taxa (grazers/collectors, mostly Limnophyes-Paralimnophyes and Parametriocmenus-Paraphaenocladius) was significant (Figure 3).
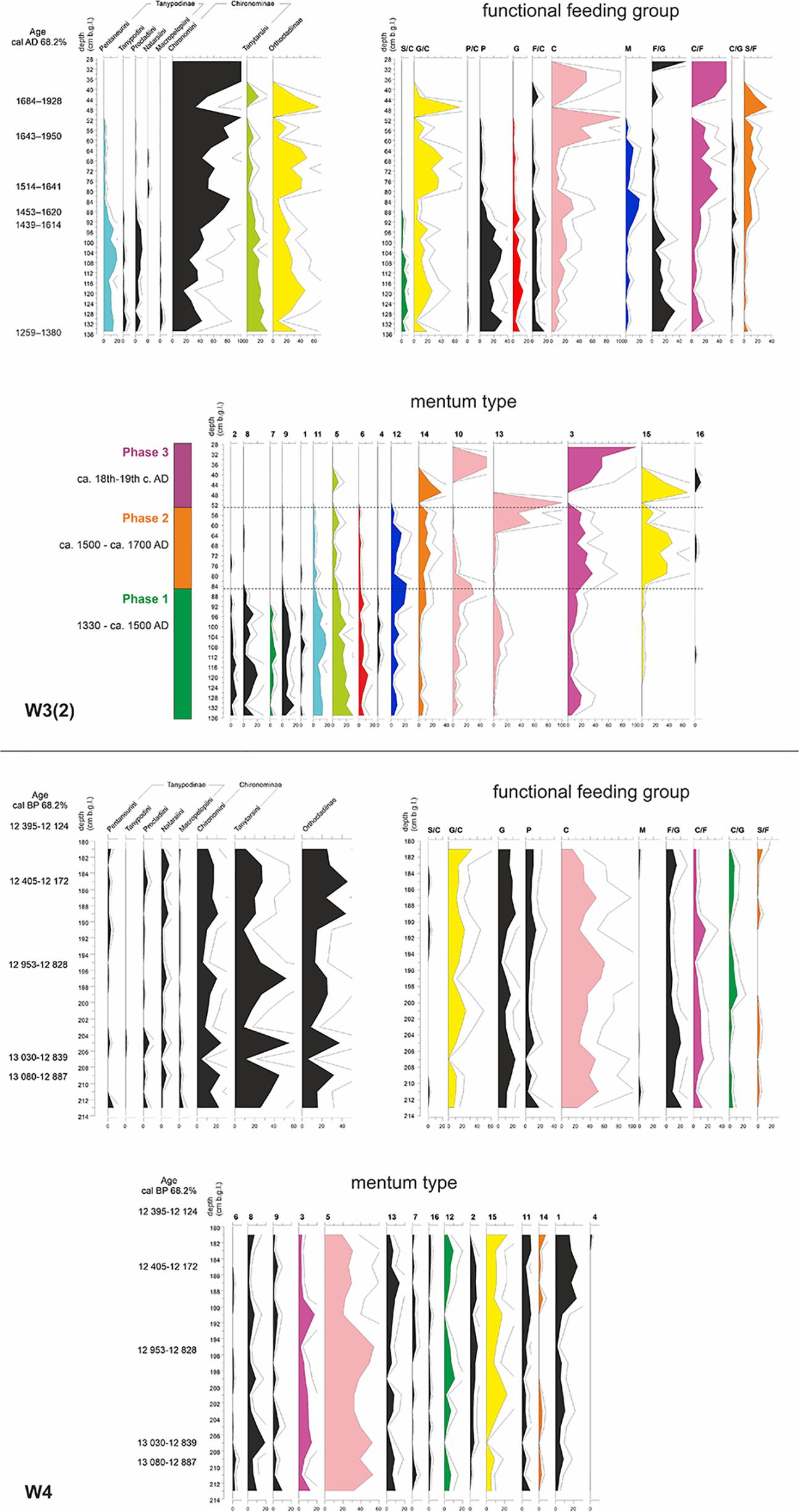
Figure 3. Chironomidae stratigraphic diagrams of the W3(2) (top) and W4 (bottom) cores, according to three typologies: subfamily/tribe, functional feeding groups and mentum types. Similar trends in some groups are outlined by the same graph color. The bar alongside W3(2) diagram represents three phases of the moat development based on Chironomidae assemblages (Kittel et al., 2018a). The abbreviations used for functional feeding groups were as follows: C, collector-gatherers; C/F, collector-filterers; C/G, collectors/grazers; S/F, shredders/filterers; S/C, shredders/collectors; G, grazers/scrapers; G/C, grazers/collectors; F/C, filterers/collectors; F/G, filterers/grazers; M, miners; P, predators; and P/C, predators/collectors. The symbols used for morphological types are explained in Table 1.
In the Late Holocene moat sequence [W3(2)], as many as 2488 head capsules of 83 Chironomidae morphospecies were recorded. The sequence reveals clear shifts in chironomid composition. The first phase is characterized by high complexity of chironomid assemblages. Worth noting is a particularly high share of predatory taxa (mainly Tanypodinae), shredders/collectors (mainly Psectrocladius) and grazers (mainly Corynoneura), but also many other groups, including grazers/collectors represented here by Cricotopus. When the abundance of chironomid larvae decreased in the 2nd half of the 16th century AD, semiterrestrial grazers/collectors started to dominate, accompanied by shredders/filterers (Polypedilum nubifer-type and P. nubeculosum-type). In the last phase, very few Chironomidae were found: firstly grazers/collectors, later mainly collectors (mentum type 10), collector/filterers and filterer/grazers (both representing mentum type 3) (Figure 3). For detailed reconstruction of the moat development, see Kittel et al. (2018a).
Tribes/Subfamilies
The ANOVA analysis for tribes/subfamilies showed that pH, K and organic matter played a significant role in shaping Chironomidae assemblages within analyzed cores (Table 2). The RDA plot (Figure 4A) revealed that W4 samples were associated with relatively low content of organic matter and K. Those two factors were correlated with the RDA2 axis. The samples from W3(2) core representing phases of moat development were clearly separated in the plot. The first phase was influenced by high pH and K values, as well as by abundance of Procladiini and Pentaneurini. Samples from the second phase were characterized by a dominance of Chironomini and high content of organic matter. The third phase, in turn, was characterized by low pH. The RDA explained 38% of variance from the analysis. While the RDA2 axis explained 11% of variance, the RDA1 axis described 31% of total variance. GLMs for Chironomidae tribes/subfamilies were initially calculated for eight predictors. Seven of them (pH, OM, CaCO3, K, sand, wood, and Urtica dioica) were included in the averaged model. Among them, only pH was statistically significant and positively correlated with richness (Figure 5A). On the other hand, hierarchical partitioning (HP) revealed that both pH (independent effects: 70%) and Urtica dioica (independent effects: 14%) were statistically significant and had positive impact on tribes/subfamilies richness (Figure 6A).
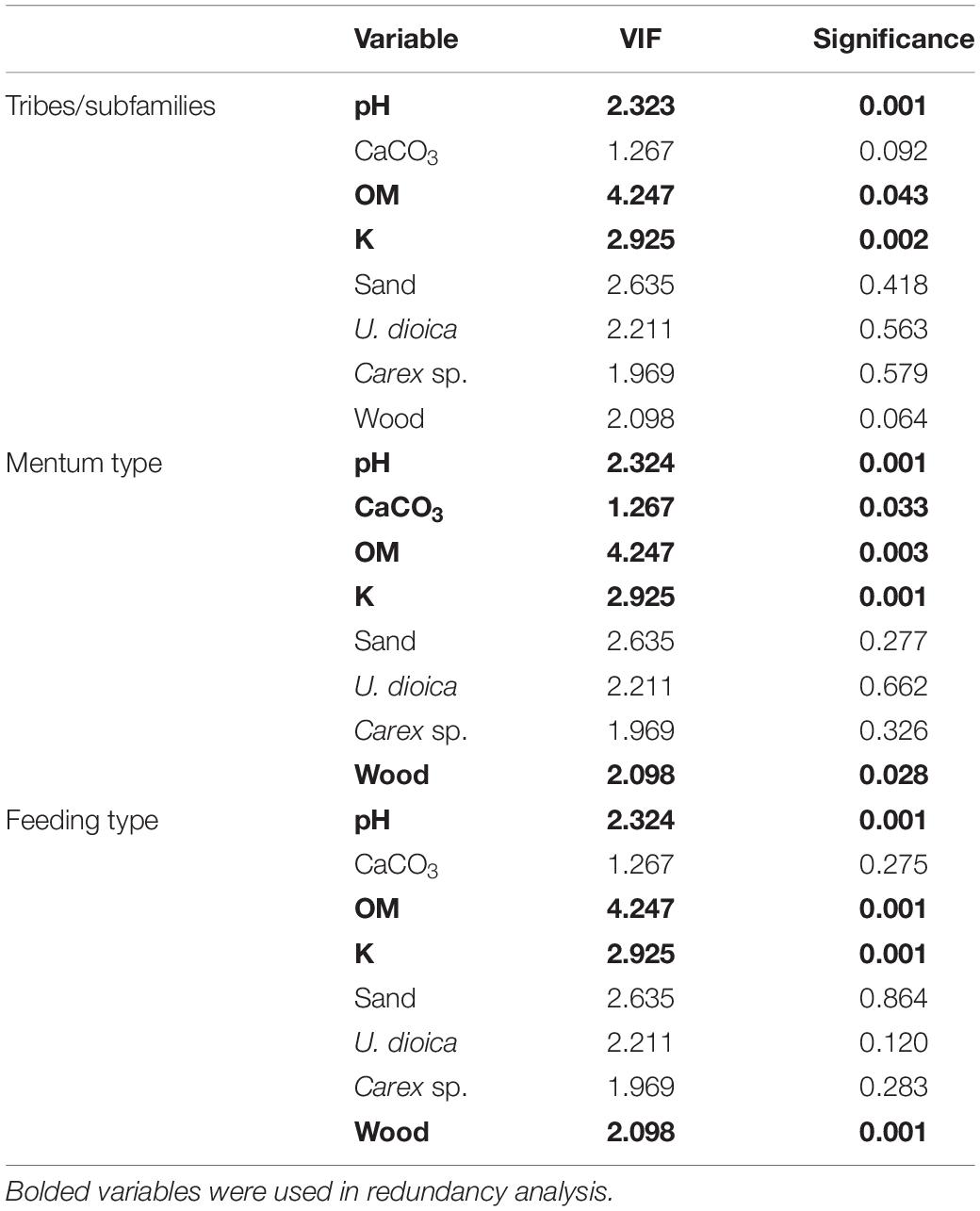
Table 2. Variance influence factors (VIFs) and significance of eight environmental factors selected from correlation matrices.
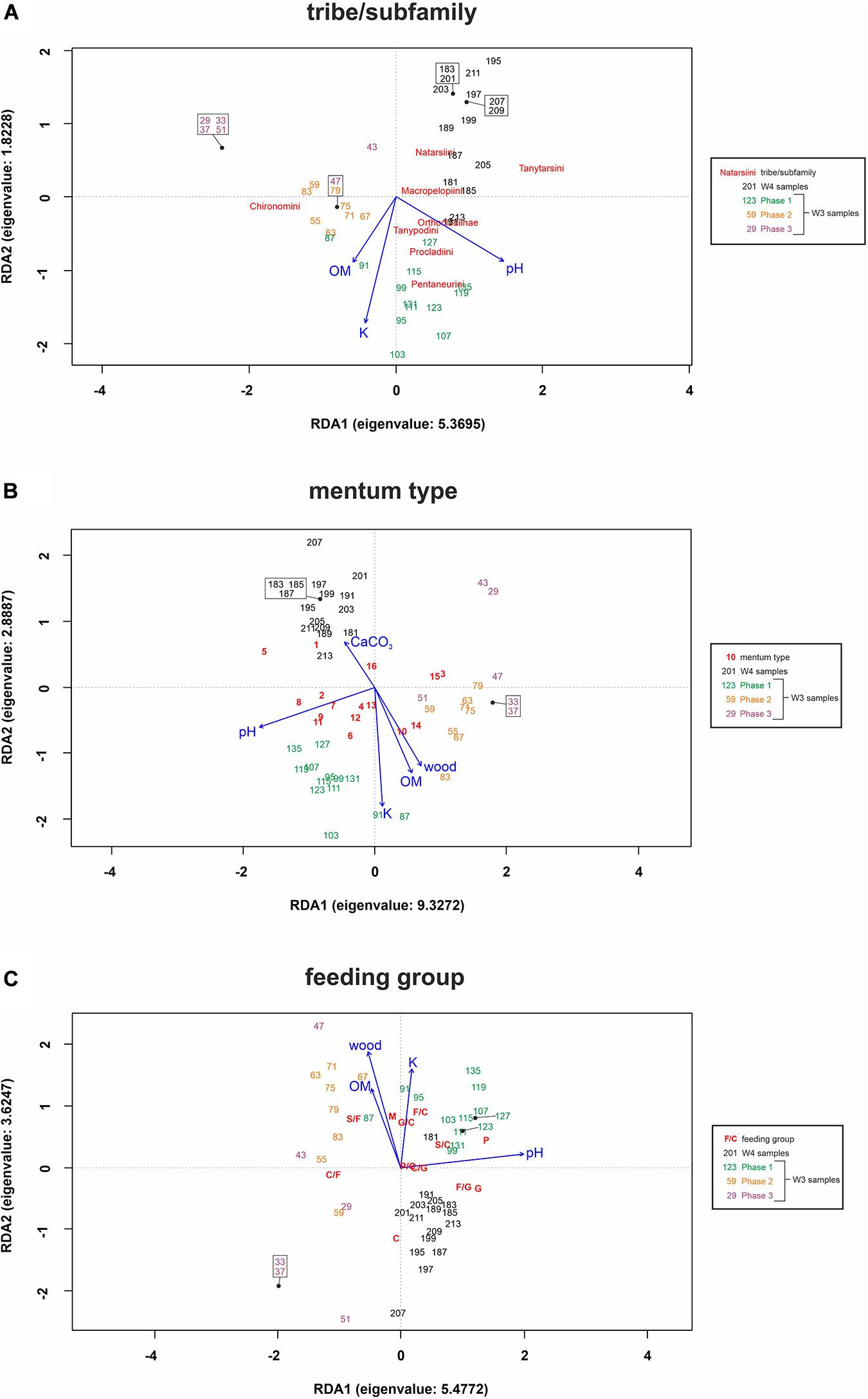
Figure 4. Redundancy Analysis for tribes/subfamilies (A), mentum types (B) and feeding groups (C) showing composition differences according to selected environmental factors (blue) in samples within two cores [W3(2) and W4]. The abbreviations used for functional feeding groups were as follows: C – collector-gatherers; C/F – collector-filterers; C/G – collectors/grazers; S/F – shredders/filterers; S/C – shredders/collectors; G – grazers/scrapers; G/C – grazers/collectors; F/C – filterers/collectors; F/G – filterers/grazers; M – miners; P – predators; and P/C – predators/collectors. The symbols used for morphological types are explained in Table 1. For full names of variables, see Supplementary Table 1.
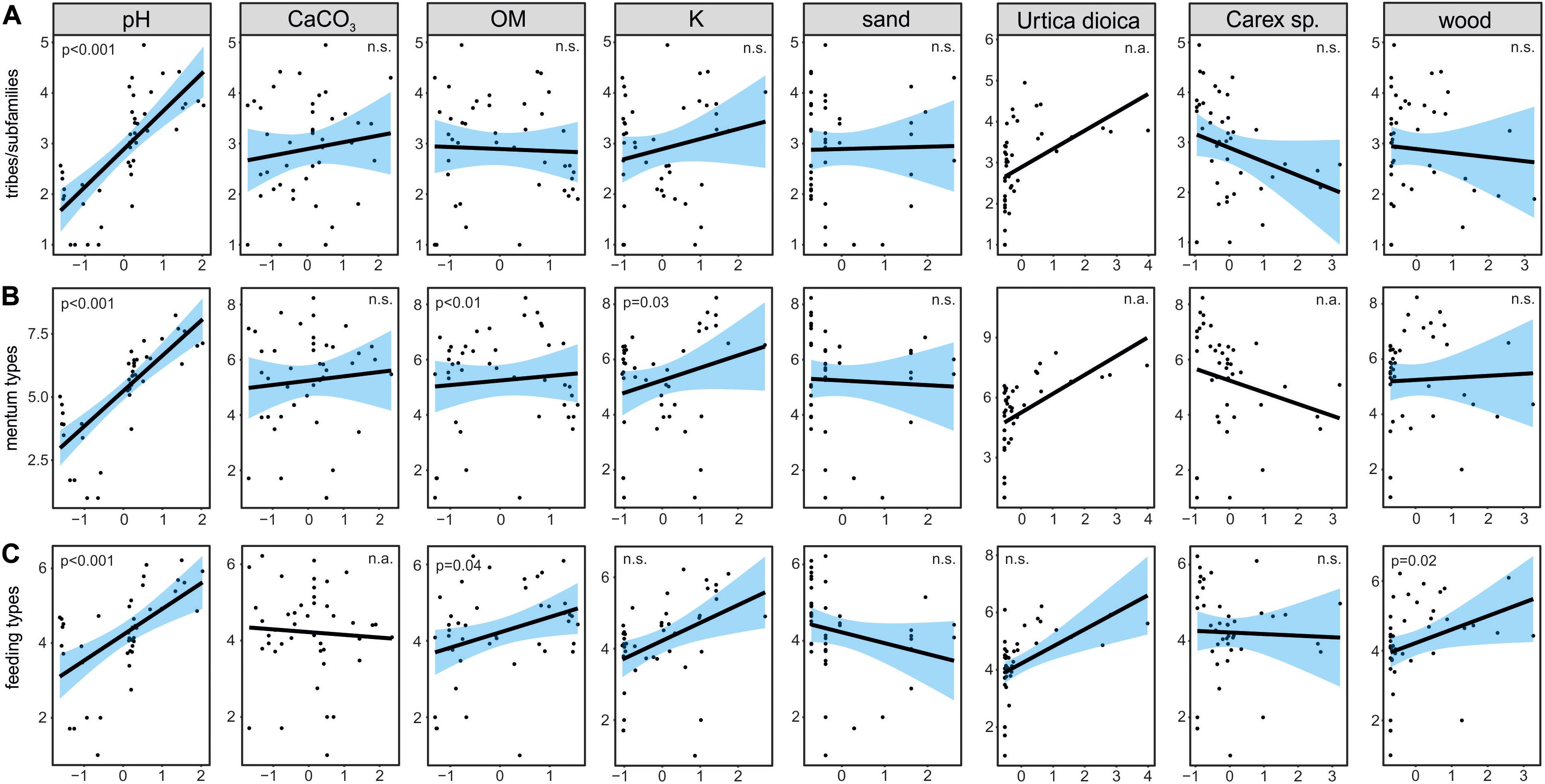
Figure 5. Visualization of generalized linear models testing for effects of selected environmental factors on richness of chironomid tribes/subfamilies (A), mentum types (B) and feeding type (C), where “n.a.” means that the factor was not included in the models, and “n.s.” that its explanatory power was not significant.
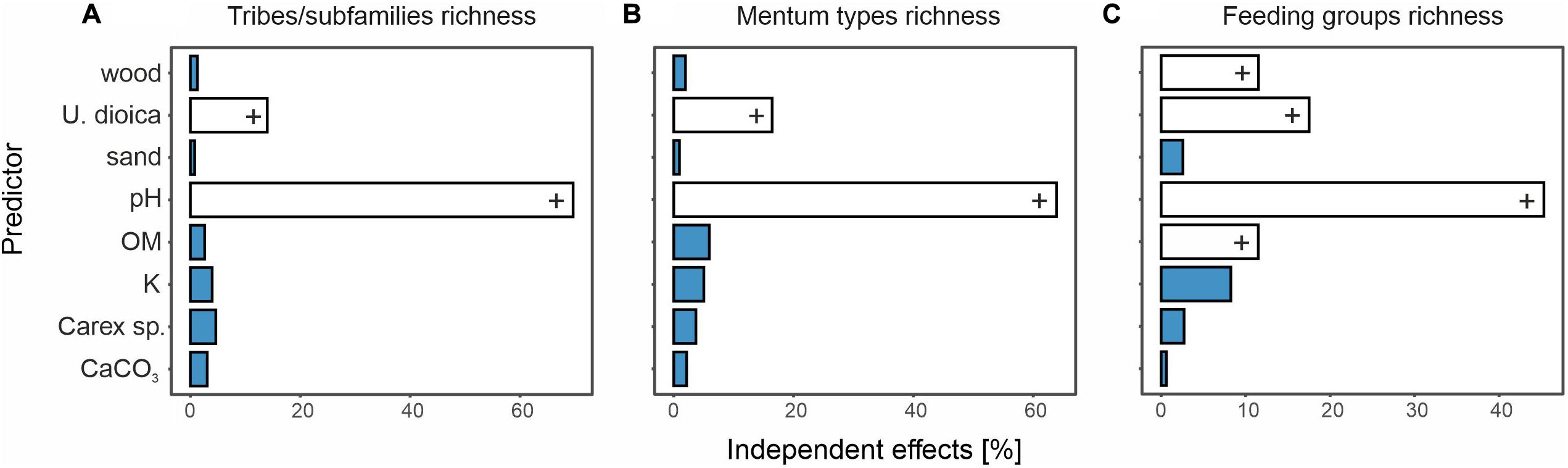
Figure 6. Relative contribution of each predictor to shared variability of full models testing for effects of environmental factors and richness of tribes/subfamilies (A), mentum types (B), and feeding types (C). A positive impact of environmental variables was marked with a plus (+) and negative impact with a minus (–). Predictors with statistical significance of response variables are given in white. For full predictor names, see Supplementary Table 1.
Mentum Types
Five environmental factors: pH, organic matter, CaCO3, K, and wood macrofossils were statistically significant in the ANOVA analysis for mentum types (Table 2). The RDA analysis showed that samples from different cores and phases were clearly distinguished. Chironomids with mentum types 1 and 5 were the most abundant in the W4 core, which may be associated with low content of wood, organic matter and potassium (factors correlated with the RDA2 axis). On the other hand, high pH (correlated with the RDA1 axis) and K values impacted the higher abundance of chironomids with 7, 8, 9, and 11 mentum types in the first phase of W3(2) core. The second phase was shaped by high content of wood, organic matter and low content of CaCO3. Low pH values characterized phase 3 of the W3(2) core. The first two RDA axes explained 21% (RDA1) and 14% (RDA2) of variance, but with Adjusted R2, the whole RDA analysis described 34% of variance. Six out of eight predictors: pH, CaCO3, organic matter, K, sand, and wood macrofossils were included in the GLMs for richness of Chironomidae mentum types. However, only pH, organic matter and potassium were statistically significant and positively correlated with richness (Figure 5B). Hierarchical partitioning results for mentum types were similar to those for tribes/subfamilies. They revealed that both pH and Urtica dioica were significant and had positive correlation with independent effects reaching 64% and 16%, respectively (Figure 6B).
Feeding Groups
The ANOVA analysis revealed that four factors: pH, OM, K, and wood were significant for the composition of chironomid feeding groups (Table 2). RDA analysis supported the results for other chironomid typologies, where samples representing each core and phase created clear aggregations (Figure 4C). W4 samples were characterized by a low content of organic matter, wood and K (factors correlated with the RDA2 axis), as well as high abundance of collectors (C). Samples from the W3(2) core were distributed along the RDA1 axis. The first-phase samples were under the influence of pH (correlated with the RDA1 axis), with high abundance of S/C, P, and F/C feeding types. High content of organic matter and wood affected the aquatic organisms in the second phase, which might be reflected in the abundance of shredders/filterers (S/F). Phase 3 assemblages were shaped by low pH. The coefficient of determination (Adj R2) for this dataset is 0.36. The RDA axis 1 described 26% of variance, while the second axis explained only 8%. Generalized Linear Models for feeding type richness included seven predictors: pH, organic matter, K, sand, Urtica dioica, Carex sp., and wood. However, only pH, organic matter and wood were statistically significant and showed positive correlation with richness (Figure 5C). Moreover, hierarchical partitioning indicated pH, OM, Urtica dioica, and wood (independent effect: 59, 11, 18, and 12%, respectively) as significant for shaping chironomid feeding types. All these factors were positively correlated with richness of feeding groups (Figure 6C).
The VIF values of all environmental variables in each RDA analysis were <10.0 (Table 2). Generally, pH seems to be the main factor shaping Chironomidae composition in each typology. Besides that, redundancy analyses revealed the importance of K and organic matter (Figure 4). In W3(2) samples, pH is strongly correlated (0.88) with diatom concentration (Supplementary Figure 1), while they are absent from the W4 sequence. This is the reason why, although they are an important food source for some chironomid larvae, they were not included in the analysis. Another important factor is Urtica dioica, which reveals higher significance treated individually in the hierarchical partitioning than in the averaged GLMs.
All combinations of the most parsimonious models used to compute average models for each analysis are listed in Supplementary Table 2. For final values of each environmental factor in averaged models, see Supplementary Table 3.
Discussion
Chironomidae Habitat Preferences in the Paleo-Oxbow and the Moat
Both generalized linear models (Figure 5) and hierarchical partitioning (Figure 6) clearly show that the crucial factors shaping Chironomidae functional composition are pH and OM. However, each site is different, depending on the levels of these factors. In the paleochannel (W4), pH was stable and circum-neutral, as indicated by the geochemical analysis. That provided favorable conditions for a complex, functionally diverse ecosystem. In the moat system (W3), pH conditions underwent significant change, from slightly alkaline in the first phase of its development to slightly acidic in the following phases. Those changes are associated with primary human-induced eutrophication and water acidification after ringfort abandonment (Kittel et al., 2018a; Figure 4). The K, OM, and wood positively shaped Chironomidae assemblages in the first phase of moat development, during human settlement in the ringfort (Kittel et al., 2018a). Taxa typical of permanent stagnant, partly overgrown water bodies (e.g., Paratanytarsus penicillatus-type, Glyptotendipes pallens-type and Cricotupus intersectus-type) were dominant. Mining chironomids were represented mainly by Endochironomus tendens-type and Glyptotendipes pallens-type. In the group of eutrophic species that are mining several macrophyte species (as Endochironomus impar-type in Berg, 1995; Beiger, 2004; Moller Pillot, 2009), many just live in coarse organic detritus, without direct association with living plant tissue (Bijlmakers, 1983). Tóth et al. (2012) state that the above-mentioned chironomids are in fact mostly opportunistic in selecting macrophyte species, while the structure of vegetation is more important. They could also settle on decaying wood particles, bark and small branches. Among terrestrial plants, Urtica dioica is strongly correlated with chironomid community richness according to its independent effect (Figure 6). This is an indicator of wet, nitrogen-rich soil (Hill et al., 1999). Urtica is often found in swampy habitats, but also in great abundance in habitats where high trophic state is the result of human impact (Šrùtek and Teckelmann, 1998). Both Urtica abundance and Chironomidae species richness result from high trophic conditions in the moat (high OM and pH) (Figure 6). Potassium (K) that shaped communities with high significance, indirectly marks denudation processes associated with human influence on the moat, in its first stage of development (W3). The ringfort surroundings were strongly exposed to trampling, lithogenic elements were also moved from local gardens and fields (Kittel et al., 2018a). Denudation processes influenced moat bank habitats affecting chironomid communities even on a high taxonomic level. These processes are associated with the inwash of organic and inorganic suspension, including fine particulate organic matter. Thus, the filtrators and collector-gatherers were abundant. The moat history ends with a sudden decrease of Chironomidae abundance and its transformation into the semiterrestrial peaty habitat (low pH but high OM, K, and wood accumulation) (Figure 4). It is associated with the complete change of the conditions in the moat, which were no longer favorable for most chironomid taxa. The scarcity of water, acidification, and macrophyte composition transformation meant that only taxa adapted to such conditions could survive. Within the chironomid fauna of that time, there were single individuals of Limnophyes-Paralimnophyes, Pseudosmittia, and Pseudorthocladius (mentum type 15), along with some Chironominae (mostly collectors) typical of muddy bottoms of the retain ditch of the moat (Kittel et al., 2018a).
Whereas the samples from W3(2) are more scattered and arranged according to depth, the W4 samples are aggregated closely together in the ordination analysis (Figure 4). This results from the habitat conditions in the paleochannel (W4) during Late Weichselian, which were more uniform and stable for a long time than in the moat habitat. They reveal significantly lower importance of lithogenic elements (like K) than in W3(2), indicating that Medieval human settlement in the valley caused higher denudation than during Allerød-Younger Dryas transition. Lower trophic state in this case does not follow acidification like in W3(2) but comes from generally low OM content in the Lateglacial landscape (Birks and Birks, 2004). Initial plant communities (Feurdean et al., 2014) did not generate much wood substrate for the mining chironomid communities. The morphological traits varied across the CaCO3 gradient from W3(2) to W4 mostly due to its high content in W4, as opposed to low content in W3(2). The influence of this variable on chironomids is associated with the periods of increased leaching from the shallow groundwater. During the Late Glacial oxbows in the regional river valleys were supplied by carbonate groundwater (Płóciennik et al., 2015; Pawłowski et al., 2016a). The water supply (precipitation, floods, and groundwater) is important for the biota composition in river valley wetlands. When groundwater seeps to the oxbows there appear taxa typical to cold, alkaline springs and brooks (Płóciennik et al., 2015; Pawłowski et al., 2016b).
Despite covering a similar timespan (ca. 600 years), out of both studied cores, the W3(2) sequence was far more ecologically diverse. Without doubt, human impact was crucial here. It changed both physical and chemical water composition, and thus also flora and fauna within the moat system and in its immediate vicinity (Kittel et al., 2018a). Ringfort inhabitants supplied the moat system with a significant amount of wood (mostly Quercus sp.), which served as a hard substrate for periphyton, including diatoms. This way, even if it was not a direct food source for the xylophiles, it created a suitable microhabitat for a range of trophic guilds, mostly scrapers. Such coarse organic matter is important not only for phytophilous grazers and filter feeders (such as Glyptotendipes pallens-type and Dicrotendipes nervosus-type) but also for collector-gatherers and shredders, represented here by larvae with mentum types 12 and 14 (Polypedilum, Phaenopsectra, and Endochironomus). Lower habitat variability and higher environmental stability (manifested here, e.g., by the narrow pH gradient) in the paleo-oxbow implied lower diversity of chironomid groups. In the W4 sequence, only 15 (out of 16) mentum types and 10 (out of 12) functional feeding groups were recorded (Figure 3).
Comparison of Typologies
It is not surprising that the results of ordination analyses based on three Chironomidae typologies are to some extent convergent. They reveal similar trends as they are based on the same data, though classified differently. However, mentum types and FFGs allow for a more detailed ecological interpretation than tribe classification. As feeding ecology strongly influenced mouthparts evolution, FFGs may aggregate taxa phylogenetically distant but having similar mouthparts (Armitage et al., 1995). Among the examples are Procladiini and Cryptochironomus with a similar, concave mentum, belonging to two different subfamilies, semiterrestrial Orthocladiinae representing different genera, and phytophile taxa from mentum types 10 and 12. The mentum shape fits feeding ecology across the taxonomic classification, as can be seen in the stratigraphic diagrams presented in Figure 3. The FFGs and mentum type stratigraphy are consistent, because habitat transformations cause changes in the available food – from suitable for P and G throughout G/C, C/F, and M typical of eutrophic, overgrown shallow water, to C and C/F living in wet moss and soil, and thus having a different food base. In fact, chironomid communities often consist of taxa with a similar type of mentum according to habitat conditions (pers. obs.).
Functional Groups as Habitat Indicators
Chironomidae functional traits have so far been studied mainly by Serra et al. (2016, 2017), who developed a trait database for European genera, analyzed it for subfamilies and compared it with the North American database (USEPA, 2012). However, such a taxonomic level seems to be too general for several traits. Creating such a database for larvae morphotypes, commonly used in paleolimnology, is worth considering.
Birks (2020) indicates that the functional trait approach in plant paleoecology has several limitations. While pollen-based ecological information is generalized, as it often can be identified only to the family or genus level, plant macrofossil analyses are problematic because of mixed data types and the possibility of ‘false absences’ (Birks, 2014, 2020). Here, chironomid subfossils could play a significant complementing role. The functional groups approach seems to work well in microhabitat reconstructions. Chironomidae FFGs and mentum types correspond to several environmental factors, such as the substrate and vegetation type, as well as physicochemical conditions. Although dividing taxa into groups is itself a generalization and may lead to loss of some data, it is also an easier and more systematized method to obtain comparable results. As functional guilds work well in climate reconstructions (see Kivilä et al., 2019), why not use them in tracking changes in the habitat structure? The chironomids serve well at the morphotype level as indicators of habitat complexity in terms of plant richness and density (Langdon et al., 2010). In fact, their association with vegetation is generally established within FFGs and, most likely, also morphological groups.
In this study, we classified each recorded Chironomidae morphospecies to a morphological group. The groups were distinguished on the basis of mentum and ventromental plates, as these elements are usually preserved well in the sediment. Whereas this classification may be enhanced and complemented in the future, it may serve as a good surrogate for functional feeding groups in habitat reconstructions. However, we need to be aware that, while the mouthpart type is strongly associated with feeding behavior, it is not the only factor shaping it. Mouthpart morphology is associated with many aspects of species biology and ecology, so it is not as strong an indicator of habitat changes as feeding groups. Moreover, as food preferences may change throughout life of chironomid larvae, only the 3rd and 4th instars should be included in such analyses. In fact, head capsules of the 1st and 2nd instars of chironomid larvae hardly ever preserve well in the sediment. In the future, the feeding groups and morphological types should also be tested within the contemporary assemblages ranging through measured ecological gradients. It would give a picture of more direct linkages between chironomid larvae and their habitat.
Conclusion
The results indicate that Chironomidae FFGs and morphological types reveal similar reactions to the biotic and abiotic environmental factors. Thus, they could serve as surrogate indicators where applicable. A well-designed mouthpart typology would be an easy and systematized method to obtain basic results comparable with functional feeding groups. Chironomidae morphological types can be easily recognized, e.g., during plant or beetle macrofossil analysis, and pre-analyzed without knowing the exact ecology of each taxon. If such preliminary examination give promising results, further detailed analysis should be undertaken by a specialist. Such an approach would enable the implementation of Chironomidae analyses in a wider range of paleolimnological and archeological research. The trait-based approach in subfossil Chironomidae studies is worth developing and can prove useful in the future application in paleolimnology. The potential use of morphological types should be checked during further studies based on modern Chironomidae assemblages ranging through known ecological gradients.
To conclude, such simplified para-taxonomic analyses based on generalized morphological types never fully replace detailed species-specific autecological approach. However, in cases where a comprehensive chironomid study is not possible, the proposed method can serve as a useful tool to obtain some ecological information.
†ORCID:
Olga Antczak-Orlewska
orcid.org/0000-0001-9392-623X
Mateusz Płóciennik
orcid.org/0000-0003-1487-6698
Robert Sobczyk
orcid.org/0000-0001-7425-6058
Daniel Okupny
orcid.org/0000-0002-8836-6044
Renata Stachowicz-Rybka
orcid.org/0000-0002-0802-0570
Monika Rzodkiewicz
orcid.org/0000-0002-6672-2183
Jacek Siciński
orcid.org/0000-0002-5235-3188
Agnieszka Mroczkowska
orcid.org/0000-0002-3534-7843
Marek Krąpiec
orcid.org/0000-0003-4270-1668
Michał Słowiński
orcid.org/0000-0002-3011-2682
Piotr Kittel
orcid.org/0000-0001-6987-7968
Data Availability Statement
The raw data supporting the conclusions of this article will be made available by the authors, without undue reservation.
Author Contributions
OA-O and MP conceived the study. RS-R, MR, and DO contributed paleobotanical and geochemical data. PK analyzed the geomorphology and paleogeography of the area and conducted the fieldwork at the study site. MK provided radiocarbon and dendrological dates. RS provided statistical analyses. OA-O, RS, and MP interpreted the data and wrote the manuscript with contributions from all authors. RS, OA-O, AM, and PK created the figures. JS, AM, and MS edited the final manuscript. All authors contributed to the article and approved the submitted version.
Funding
This study is a contribution to the grants from National Centre of Science based on decisions Nos. DEC-2013/11/B/HS3/0385 and 2018/31/B/ST10/02498. The research was co-financed by the Faculty of Biology and Environmental Protection, University of Lodz and by the Institute of Marine and Environmental Sciences, University of Szczecin.
Conflict of Interest
The authors declare that the research was conducted in the absence of any commercial or financial relationships that could be construed as a potential conflict of interest.
Acknowledgments
We are indebted to Julita Tomkowiak, MSc and Jerzy Sikora, Ph.D. for help with geochemical sample processing and field investigation. We would like to thank two reviewers whose suggestions helped to improve and clarify this manuscript.
Supplementary Material
The Supplementary Material for this article can be found online at: https://www.frontiersin.org/articles/10.3389/fevo.2020.583831/full#supplementary-material
Supplementary Figure 1 | Correlation matrices with Pearson’s r correlation coefficients calculated for the W3(2) core: (A) all predictors and (B) predictors left for further analyses. For full predictor names, see Supplementary Table 1.
Supplementary Figure 2 | Correlation matrices with Pearson’s r correlation coefficients calculated for the W4 core: (A) all predictors and (B) predictors left for further analyses. For full predictor names, see Supplementary Table 1.
Supplementary Table 1 | Variables for cores analyzed in the study. The data was standardized during analyses. Bolded variables were included in further analysis.
Supplementary Table 2 | Most supported (ΔAIC < 2) models testing for impact of environmental factors on richness of chironomid groups in each typology.
Supplementary Table 3 | Impact of environmental factors used in averaged models on richness of analyzed chironomid groups (generalized linear regression).
References
Alfons, A. (2019). robustHD: Robust Methods for High-Dimensional Data. R package version 0.6.1. https://CRAN.R-project.org/package=robustHD.
Andersen, T., Cranston, P. S., and Epler, J. H. (2013). Chironomidae of the Holarctic region: keys and diagnoses. Part 1. Larvae. Insect Syst. Evol. Suppl. 66, 1–571. doi: 10.11646/zootaxa.60.1.1
Armitage, P. D., Cranston, P. S., and Pinder, L. C. V. (1995). The Chironomidae. Biology and Ecology of Non-biting Chironomids. London: Chapman & Hall.
Barboni, D., Harrison, S. P., Bartlein, P. J., Jalut, G., New, M., and Prentice, I. C. (2004). Relationships between plant traits and climate in the Mediterranean region: a pollen data analysis. J. Veg. Sci. 15, 635–646. doi: 10.1111/j.1654-1103.2004.tb02305.x
Bartoń, K. (2018). MuMIn: Multi-model Inference. R package version 1.42.1. https://CRAN.R-project.org/package=MuMIn.
Battarbee, R. W. (1986). “Diatom analysis,” in Handbook of Holocene Paleoecology and Paleohydrology, ed. B. E. Berglund (Chichester: John Wiley and Sons ltd), 527–570.
Battarbee, R. W., and Kneen, M. J. (1982). The use of electronically counted microspheres in absolute diatom analysis. Limnol. Oceanogr. 27, 184–188. doi: 10.4319/lo.1982.27.1.0184
Beiger, M. (2004). Owady minujące Polski. Klucz do oznaczania na podstawie min. Poznań: Bogucki Wydawnictwo Naukowe.
Berg, M. B. (1995). “Larval food and feeding behaviour,” in The Chironomidae: Biology and Ecology of Non-biting Chironomids, eds P. D. Armitage, P. S. Cranston, and L. V. Pinder (London: Chapman and Hall), 136–198. doi: 10.1007/978-94-011-0715-0_7
Berggren, G. (1981). Atlas of seeds and small fruits of Northwest European plant species (Sweden, Norway, Denmark, East Fennoscandia and Iceland) with morphological descriptions, part 3. Berlings: Salicaceae-Cruciferae.
Bijlmakers, L. (1983). De verspreiding en oecologie van chironomide larven (Chironomidae: Diptera) in twee vennen in de omgeving van Oisterwijk (N.Br.). Versl: K.U. Nijmegen.
Birks, H. J. B. (2014). Challenges in the presentation and analysis of plant macrofossil stratigraphical data. Veget. History Archaeobot. 23, 309–330. doi: 10.1007/s00334-013-0430-2
Birks, H. J. B. (2020). Reflections on the Use of Ecological Attributes and Traits in Quaternary Botany. Front. Ecol. Evolut. 8, 1–13. doi: 10.3389/fevo.2020.00166
Brooks, S. J., Bennion, H., and Birks, H. J. B. (2001). Tracing lake trophic history with a chironomid-total phosphorus inference model. Freshw. Biol. 46, 513–533. doi: 10.1046/j.1365-2427.2001.00684.x
Brooks, S. J., Langdon, P. G., and Heiri, O. (2007). The identification and use of Palaearctic Chironomidae larvae in palaeoecology. Quat. Res. Assoc. 53, 2129–2130. doi: 10.1111/j.1365-2427.2007.01926.x
Cappers, R. T. J., Bekker, R. M., and Jans, J. E. A. (2006). Digital seed atlas of the Netherlands. Groningen: Groningen University Library.
Carvalho, F. A., Brown, K. A., Waller, M. P., Bunting, M. J., Boom, A., and Leng, M. J. (2019). A method for reconstructing temporal changes in vegetation functional trait composition using Holocene pollen assemblages. PLoS One 14:e0216698. doi: 10.1371/journal.pone.0216698
Clift, P. D., Olson, E. D., Lechnowskyj, A., Moran, M. G., Barbato, A., and Lorenzo, J. M. (2019). Grain-size variability within a mega-scale point-bar system. False Riv. Louis. Sedimentol. 66, 408–434. doi: 10.1111/sed.12528
Díaz, S., and Cabido, M. (2001). Vive la différence: plant functional diversity matters to ecosystem processes. Trends Ecol. Evol. 16, 646–655. doi: 10.1016/S0169-5347(01)02283-2
Đurđević, A., Savić, D., Lazarević, M., Žikić, V., and Milošević, D. (2017). “Can we guess the autecology following the variability of mandible shape in the Chironomidae family?,” in 20th International Symposium on Chironomidae. Abstract Book of the 20th International Symposium on Chironomidae, ed. V. Lencioni (Groningen: Groningen University Library), 64.
Ferrington, L. C. (2008). Global diversity of non-biting chironomids (Chironomidae. Insecta-Diptera) in freshwater. Hydrobiologia 595, 447–455. doi: 10.1007/978-1-4020-8259-7_45
Feurdean, A., Perşoiu, A., Tançău, I., Stevens, I., and Magyari, E. K. (2014). Climate variability and associated vegetation response throughout Central and Eastern Europe (CEE) between 60 and 8 ka. Quat. Sci. Rev. 106, 206–224. doi: 10.1016/j.quascirev.2014.06.003
Fournier, B., Lara, E., Jassey, V. E. J., and Mitchell, E. A. D. (2015). Functional traits as a new approach for interpreting testate amoeba palaeo-records in peatlands and assessing the causes and consequences of past changes in species composition. Holocene 25, 1375–1383. doi: 10.1177/0959683615585842
Fox, J., and Weisberg, S. (2011). An {R} Companion to Applied Regression, Second Edition. Thousand Oaks CA: Sage.
Franquet, E. (1999). Chironomid assemblage of a Lower-Rhone dike field: relationships between substratum and biodiversity. Hydrobiologia 397, 121–131.
Frouz, J. (1999). Use of soil dwelling Diptera (Insecta, Diptera) as bioindicators: a review of ecological requirements and response to disturbance. Agricult. Ecosyst. Environ. 74, 167–186. doi: 10.1016/b978-0-444-50019-9.50013-3
Gandouin, E., Maasri, A., Van Vliet-Lano, B., and Franquet, E. (2006). Chironomid (Insecta: Diptera) assemblages from a gradient of lotic and lentic waterbodies in river floodplains of France: a methodological tool for paleoecological applications. J. Paleolimnol. 35, 149–166. doi: 10.1007/s10933-005-8149-4
Garnier, E., Lavorel, S., Ansquer, P., Castro, H., Cruz, P., and Dolezal, J. (2007). Assessing the effects of land-use change on plant traits, communities and ecosystem functioning in grasslands: a standardized methodology and lessons from an application to 11 European sites. Anna. Bot. 99, 967–985. doi: 10.1093/aob/mcl215
Goździk, J. (1982). Środowisko przyrodnicze osadnictwa średniowiecznego okolic Rozprzy. Prace i Materiały Muzeum Archeologicznego i Etnograficznego w śodzi. Ser. Archeol. 29, 138–151.
Gregory-Eaves, I., and Beisner, B. E. (2011). Palaeolimnological insights for biodiversity science: An emerging field. Freshwater Biol. 56, 2653–2661. doi: 10.1111/j.1365-2427.2011.02677.x
Greguss, P. (1945). The identification of Central-European dicotyledonous trees and shrubs based on xylotomy. Budapest: Hungarian Museum of Natural History.
Grosser, D. (1977). Die Hölzer Mitteleuropas. Ein mikrophotographischer Lehratlas. Berlin: Springer-Verlag.
Heiri, O., Brooks, S. J., Birks, H. J. B., and Lotter, A. F. (2011). A 274-lake calibration data-set and inference model for chironomid-based summer air temperature reconstruction in Europe. Quat. Sci. Rev. 30, 3445–3456. doi: 10.1016/j.quascirev.2011.09.006
Heiri, O., Lotter, A. F., and Lemcke, G. (2001). Loss on ignition as a method for estimating organic and carbonate content in sediments: reproducibility and comparability of results. J. Paleolimnol. 25, 101–110.
Henrikson, L., Olafsson, J. B., and Oscarson, H. G. (1982). The impact of acidification on Chironomidae (Diptera) as indicated by subfossil stratification. Hydrobiologia 86, 223–229. doi: 10.1007/bf00006140
Hill, M. O., Mountford, J. O., Roy, D. B., and Bunce, R. G. H. (1999). Ellenberg’s indicator values for British plants. ECOFACT Volume 2 Technical Annex. Huntingdon: Institute of Terrestrial Ecology.
Howard, L. C., Wood, P. J., Greenwood, M. T., Rendell, H. M., Brooks, S. J., Armitage, P. D., et al. (2010). Subfossil Chironomidae as indicators of palaeoflow regimes: integration into the PalaeoLIFE flow index. J. Quat. Sci. 25, 1270–1283. doi: 10.1002/jqs.1409
Jiang, X., Pan, B., Song, Z., and Xie, Z. (2019). Do functional traits of chironomid assemblages respond more readily to eutrophication than taxonomic composition in Chinese floodplain lakes? Ecol. Indicat. 103, 355–362. doi: 10.1016/j.ecolind.2019.04.029
Juggins, S. (2007). C2 version 1.5 user guide. Software for Ecological and Palaeoecological Data Analysis and Visualisation. Newcastle upon Tyne: Newcastle University.
Kats, N. Y., Kats, S. V., and Kipiani, M. G. (1965). Atlas i opredelitel’ plodov i semyan vstrechayushchikhsya v chetviertichnykh otlozheniyach SSSR. Izdat: Nauka.
Kearney, M., Simpson, S. J., Raubenheimer, D., and Helmuth, B. (2010). Modelling the ecological niche from functional traits. Phil. Trans. R. Soc. B 365, 3469–3483. doi: 10.1098/rstb.2010.0034
Kittel, P., Płóciennik, M., Borówka, R. K., Okupny, D., Pawłowski, D., Peyron, O., et al. (2016). Early Holocene hydrology and environments of the Ner River (Poland). Quat. Res. 85, 187–203. doi: 10.1016/j.yqres.2015.12.006
Kittel, P., Sikora, J., and Wroniecki, P. (2015). The morphology of the Luciąża River valley floor in the vicinity of the Rozprza medieval ring-fort in light of geophysical survey. Bull. Geogr. Phys. Geogr. Ser. 8, 95–106. doi: 10.1515/bgeo-2015-0008
Kittel, P., Sikora, J., and Wroniecki, P. (2018b). A Late Medieval motte-and-bailey settlement in a lowland river valley landscape of central Poland. Geoarchaeology 33, 558–578. doi: 10.1002/gea.21676
Kittel, P., Sikora, J., Antczak, O., Brooks, S. J., Elias, S. A., Krąpiec, M., et al. (2018a). The palaeoecological development and functioning of the Late Medieval moat – multiproxy research at Rozprza. Centr. Poland. Quart. Int. 482, 131–156. doi: 10.1016/j.quaint.2018.03.026
Kivilä, E. H., Luoto, T. P., Rantala, M. V., and Nevalainen, L. (2020). Late-Holocene variability in chironomid functional assemblages and carbon utilization in a tundra lake food web. Hydrobiologia 847, 895–911. doi: 10.1007/s10750-019-04151-7
Kivilä, E. H., Luoto, T. P., Rantala, M. V., Kiljunen, M., Rautio, M., and Nevalainen, L. (2019). Environmental controls on benthic food web functions and carbon resource use in subarctic lakes. Freshwater Biol. 64, 643–658. doi: 10.1111/fwb.13250
Kleyer, M., Dray, S., Bello, F., Lepš, J., Pakeman, R. J., Strauss, B., et al. (2012). Assessing species and community functional responses to environmental gradients: which multivariate methods? J. Veget. Sci. 23, 805–821. doi: 10.1111/j.1654-1103.2012.01402.x
Kotrys, B., Płóciennik, M., Sydor, P., and Brooks, S. J. (2020). Expanding the Swiss-Norwegian chironomid training set by incorporating Polish data and assessing its transfer function capability using temperature reconstructions from Lake Żabieniec. Boreas 49, 89–107.
Lamentowicz, M., Forysiak, J., Balwierz, Z., Płóciennik, M., Kittel, P., Kloss, M., et al. (2009). Multiproxy study of anthropogenic and climatic changes in the last two millennia from a small mire in central Poland. Hydrobiologia 631, 213–230. doi: 10.1007/978-90-481-3387-1_13
Lamentowicz, M., Gałka, M., Lamentowicz, Ĺ, Obremska, M., Kühl, N., Lücke, A., et al. (2015). Reconstructing climate change and ombrotrophic bog development during the last 4000 years in northern Poland using biotic proxies, stable isotopes and trait-based approach. Palaeog. Palaeocl. Palaeoecol. 418, 261–277. doi: 10.1016/j.palaeo.2014.11.015
Langdon, P. G., Ruiz, Z., Wynne, S., Sayer, C. D., and Davidson, T. A. (2010). Ecological influences on larval chironomid communities in shallow lakes: implications for palaeolimnological interpretations. Freshwat. Biol. 55, 531–545. doi: 10.1111/j.1365-2427.2009.02345.x
Luoto, T. P. (2009a). A Finnish chironomid- and chaoborid-based inference model for reconstructing past lake levels. Quat. Sci. Rev. 28, 1481–1489. doi: 10.1016/j.quascirev.2009.01.015
Luoto, T. P. (2009b). Subfossil Chironomidae (Insecta: Diptera) along a latitudinal gradient in Finland: development of a new temperature inference model. J. Quat. Sci. 24, 150–158. doi: 10.1002/jqs.1191
Marcisz, K., Jassey, V. E. J., Kosakyan, A., Krashevska, V., Lahr, D. J. G., and Lara, E. (2020). Testate Amoeba Functional Traits and Their Use in Paleoecology. Front. Ecol. Evol 8, 1–28. doi: 10.3389/fevo.2020.575966
Marks, L. (2011). “Quaternary Glaciations in Poland,” in Developments in Quaternary Sciences 15, eds J. Ehlers, P. L. Gibbard, and P. D. Hughes (Izdat: Nauka), 299–303. doi: 10.1016/b978-0-444-53447-7.00023-4
Matuszkiewicz, J. M. (2008). Potencjalna roślinność naturalna Polski (Potential natural vegetation of Poland), IGiPZ PAN, Warszawa. Available online at: http://www.igipz.pan.pl/Roslinnosc-potencjalna-zgik.html (accessed on March 10, 2017)
Milošević, D., Stojanović, K., Djurdjević, A., Marković, Z., Stojković Piperac, M., Živić, M., et al. (2018). The response of chironomid taxonomy- and functional trait-based metrics to fish farm effluent pollution in lotic systems. Environ. Pollut. 242, 1058–1066. doi: 10.1016/j.envpol.2018.07.100
Moller Pillot, H. K. M. (2009). Chironomidae larvae. Biology and Ecology of the Chironomini. Zeist: KNNV Publishing.
Moller Pillot, H. K. M. (2013). Chironomidae larvae of the Netherlands and adjacent lowlands, Biology and Ecology of the aquatic Orthocladiinae, Prodiamesinae, Diamesinae. Buchonomyiinae: KNNV Publishing.
Monakov, A. V. (1972). Review of studies on feeding of aquatic invertebrates conducted at the Institute of Biology of Inland Waters, Academy of Science, USSR. J. Fisher. Res. Board Can. 29, 363–383. doi: 10.1139/f72-064
Motta, L., and Massaferro, J. (2019). Climate and site-specific factors shape chironomid taxonomic and functional diversity patterns in northern Patagonia. Hydrobiologia 839, 131–143. doi: 10.1007/s10750-019-04001-6
Mycielska-Dowgiałło, E., and Ludwikowska-Kêdzia, M. (2011). Alternative interpretations of grain-size data from Quaternary deposits. Geologos 17, 189–203.
Nevalainen, L., and Luoto, T. P. (2017). Relationship between cladoceran (Crustacea) functional diversity and lake trophic gradients. Funct. Ecol. 31, 488–498. doi: 10.1111/1365-2435.12737
Nevalainen, L., Luoto, T. P., Manca, M., and Weisse, T. (2015). A paleolimnological perspective on aquatic biodiversity in Austrian mountain lakes. Aquat. Sci. 77, 59–69. doi: 10.1007/s00027-014-0363-6
Ni, Z., Zhang, E., Herzschuh, U., Mischke, S., Chang, J., Sun, W., et al. (2020). Taxonomic and functional diversity differentiation of chironomid communities in northern Mongolian Plateau under complex environmental impacts. Hydrobiologia 847, 2155–2167. doi: 10.1007/s10750-020-04241-x
Nock, C. A., Vogt, R. J., and Beisner, B. E. (2016). Functional Traits. eLS. Chichester: John Wiley & Sons, Ltd, doi: 10.1002/9780470015902.a0026282
Oksanen, J., Blanchet, F. G., Friendly, M., Kindt, R., Legendre, P., McGlinn, D., et al. (2019). Vegan: Community Ecology Package. R package version 2.5-4. https://CRAN.R-project.org/package=vegan.
Olafsson, J. S. (1992). A comparative study on mouthpart morphology of certain larvae of Chironomini (Diptera: Chironomidae) with a reference to the larval feeding habits. J. Zool. Lond. 228, 183–204. doi: 10.1111/j.1469-7998.1992.tb04602.x
Pawłowski, D., Borówka, R. K., Kowalewski, G., Luoto, T. P., Milecka, K., Nevalainen, L., et al. (2016a). Late Weichselian and Holocene record of the paleoenvironmental changes in a small river valley in Central Poland. Quat. Sci. Rev. 135, 24–40. doi: 10.1016/j.quascirev.2016.01.005
Pawłowski, D., Borówka, R. K., Kowalewski, G., Luoto, T. P., Milecka, K., Nevalainen, L., et al. (2016b). The response of flood-plain ecosystems to the Late Glacial and Early Holocene hydrological changes: a case study from a small Central European river valley. Catena 147, 411–428. doi: 10.1016/j.catena.2016.07.034
Pla, L., Casanoves, F., and Di Rienzo, J. (2012). “Functional Diversity Indices,” in L. Pla, F. Casanoves and J. Di Rienzo. SpringerBriefs in Environmental Science, Quantifying Functional and Biodiversity (Dordrecht: Springer), 27–51. doi: 10.1007/978-94-007-2648-2_3
Płóciennik, M., Kruk, A., Forysiak, J., Pawłowski, D., Mianowicz, K., Elias, S., et al. (2015). Fen ecosystem response to water-level fluctuations during the early and middle Holocene in Central Europe: a case study from Wilczków. Poland. Boreas 44, 721–740. doi: 10.1111/bor.12129
Płóciennik, M., Pawłowski, D., Vilizzi, L., and Antczak-Orlewska, O. (2020). From oxbow to mire: Chironomidae and Cladocera as habitat palaeoindicators. Hydrobiologia 847, 3257–3275. doi: 10.1007/s10750-020-04327-6
Porinchu, D. F., and MacDonald, G. M. (2003). The use and application of freshwater chironomids (Chironomidae: Insecta: Diptera) in geographical research. Progr. Geogr. 27, 378–422. doi: 10.1191/030913303767888491
R Core Team (2020). R: A language and environment for statistical computing. Austria: R Foundation for Statistical Computing.
Reitalu, T., Gerhold, P., Poska, A., Pärtel, M., Väli, V., and Veski, S. (2015). Novel insights into post-glacial vegetation change: functional and phylogenetic diversity in pollen records. J. Veg. Sci. 26, 911–922. doi: 10.1111/jvs.12300
Rolland, N., and Larocque, I. (2007). The efficiency of kerosene flotation for extraction of chironomid head capsules from lake sediments samples. J. Paleolimnol. 37, 565–572. doi: 10.1007/s10933-006-9037-2
Saether, O. A. (1979). Chironomid communities as water quality indicators. Holarctic Ecol. 2, 65–74. doi: 10.1111/j.1600-0587.1979.tb00683.x
Schmid, P. E. (1993). A key to the Chironomidae and their instars from Austrian Danube region streams and rivers. Part I, Diamesinae, Prodiamesinae and Orthocladiinae. Wien: Federal Institute for Water Quality of the Ministry of Agriculture and Forestry.
Schweingruber, F. H. (1978). Mikroskopische Holzanatomie. Swiss Federal Institute of Forestry Research, Birmensdorf. Wien: Federal Institute for Water Quality of the Ministry of Agriculture and Forestry.
Schweingruber, F. H., Börner, A., and Schulze, E.-D. (2011). Atlas of Stem Anatomy in Herbs, Shrubs and Trees, Vol. 1. Heidelberg: Springer.
Serra, S. R. Q., Cobo, F., Graça, M. A. S., Dolédec, S., and Feio, M. J. (2016). Synthesising the trait information of European Chironomidae (Insecta: Diptera): Toward a new database. Ecol. Indicat. 61, 282–292. doi: 10.1016/j.ecolind.2015.09.028
Serra, S. R. Q., Graça, M. A. S., Dolédec, S., and Feio, M. J. (2017). Chironomidae of the Holarctic region: a comparison of ecological and functional traits between North America and Europe. Hydrobiologia. 794, 273–285. doi: 10.1007/s10750-017-3102-x
Sikora, J., Kittel, P., and Wroniecki, P. (2015). From a point on the map to a shape in the landscape. Non-invasive verification of medieval ring-forts in Central Poland: Rozprza case study. Archaeol. Pol. 53, 510–514.
Sikora, J., Kittel, P., Frączek, M., Głąb, Z., Golyeva, A., Mueller-Bieniek, A., et al. (2019). A palaeoenvironmental reconstruction of the rampart construction of the medieval ring-fort in Rozprza. Centr. Pol. Archaeol. Anthropol. Sci. 11, 4187–4219. doi: 10.1007/s12520-018-0753-0
Spies, M. (2005). On selected family group names in Chironomidae (Insecta, Diptera), and related nomenclature. Zootaxa 894, 1–12. doi: 10.11646/zootaxa.894.1.1
Šrùtek, M., and Teckelmann, M. (1998). Review of biology and ecology of Urtica dioica. Presha 70, 1–19. doi: 10.2307/2255757
Stuart-Smith, R., Bates, A., Lefcheck, J., Duffy, E., Baker, S. C., Thomson, R. J., et al. (2013). Integrating abundance and functional traits reveals new global hotspots of fish diversity. Nature 501, 539–542. doi: 10.1038/nature12529
Tóth, M., Móra, A., Kiss, B., Dévai, G., and Specziár, A. (2012). Are macrophyte-dwelling Chironomidae (Diptera) largely opportunistic in selecting plant species? Eur. J. Entomol. 109, 247–260. doi: 10.14411/eje.2012.033
USEPA (2012). Freshwater Biological Traits Database (EPA/600/R-11/038F). Environmental Protection Agency. Washington, DC: Washington univercity.
Vallenduuk, H. J., and Moller Pillot, H. K. M. (2007). Chironomidae Larvae of the Netherlands and Adjacent Lowlands. General ecology and Tanypodinae. Zeist: KNNV Publishing.
Velichkevich, F. Y., and Zastawniak, E. (2006). Atlas of Pleistocene vascular plant macroremains of Central and Eastern Europe, Part I – Pteridophytes and monocotyledons. W. Cracow: Polish Academy of Sciences.
Velichkevich, F. Y., and Zastawniak, E. (2008). Atlas of vascular plant macroremains from the Pleistocene of central and eastern Europe, Part II – Herbaceous dicotyledons. W. Poland: Szafer Institute of Botany.
Vogt, R. J., Beisner, B. E., and Prairie, Y. T. (2010). Functional diversity is positively associated with biomass for lake diatoms. Freshwater Biol. 55, 1636–1646.
Wachecka-Kotkowska, L. (2004a). Ewolucja doliny Luciąży – uwarunkowania klimatyczne a lokalne. Acta Geogr. Lodziensia 86, 1–161.
Wachecka-Kotkowska, L. (2004b). Zmiany środowiska doliny dolnej Luciąży w holocenie. Acta Universitatis Lodziensis. Folia Geogr. Phys. 6, 47–70.
Walker, I. R. (2001). “Chironomids: Chironomidae and related Diptera,” in Tracking environmental change using lake sediments, Volume 4: Zoological Indicators, eds J. P. Smol, H. J. B. Birks, and W. M. Last (Do: Kluwer Academic Press), 43–66.
Walker, I. R., Fernando, C. H., and Paterson, C. G. (1985). Association of Chironomidae (Diptera) of shallow, acid, humic lakes and bog pools in Atlantic Canada, and a comparison with an earlier paleoecological investigation. Hydrobiologia 120, 11–22. doi: 10.1007/bf00034586
Walsh, C., and Mac Nally, R. (2013). hier.part: Hierarchical Partitioning. R package version 1.0-4. https://CRAN.R-project.org/package=hier.part.
Wei, T., and Simko, V. (2017). R package “corrplot”: Visualization of a Correlation Matrix (Version 0.84). https://guthub.com/taiyun/corrplot.
Keywords: Diptera, functional feeding groups, trophic guilds, paleoecology, paleolimnology, habitat reconstruction, environmental changes, mouthpart morphology
Citation: Antczak-Orlewska O, Płóciennik M, Sobczyk R, Okupny D, Stachowicz-Rybka R, Rzodkiewicz M, Siciński J, Mroczkowska A, Krąpiec M, Słowiński M and Kittel P (2021) Chironomidae Morphological Types and Functional Feeding Groups as a Habitat Complexity Vestige. Front. Ecol. Evol. 8:583831. doi: 10.3389/fevo.2020.583831
Received: 15 July 2020; Accepted: 04 December 2020;
Published: 11 January 2021.
Edited by:
Vincent Jassey, UMR 5245 Laboratoire Ecologie Fonctionnelle et Environnement (ECOLAB), FranceReviewed by:
Karen Taylor, University College Cork, IrelandTriin Reitalu, Tallinn University of Technology, Estonia
Copyright © 2021 Antczak-Orlewska, Płóciennik, Sobczyk, Okupny, Stachowicz-Rybka, Rzodkiewicz, Siciński, Mroczkowska, Krąpiec, Słowiński and Kittel. This is an open-access article distributed under the terms of the Creative Commons Attribution License (CC BY). The use, distribution or reproduction in other forums is permitted, provided the original author(s) and the copyright owner(s) are credited and that the original publication in this journal is cited, in accordance with accepted academic practice. No use, distribution or reproduction is permitted which does not comply with these terms.
*Correspondence: Olga Antczak-Orlewska, olga.antczak-orlewska@ug.edu.pl; olga.antczak@biol.uni.lodz.pl