Corn Yield and Phosphorus Use Efficiency Response to Phosphorus Rates Associated With Plant Growth Promoting Bacteria
- 1Departament of Soil Science, University of São Paulo (ESALQ/USP), Piracicaba, Brazil
- 2Department of Rural Engineering, Plant Health and Soils, São Paulo State University (FEIS/UNESP), Ilha Solteira, São Paulo, Brazil
- 3Department of Agronomy, Federal University of Grande Dourados (UFGD), Dourados, Brazil
The use of plant growth promoting bacteria (PGPB) that can solubilize phosphorus (P) has shown potential to improve nutrient availability in grass crops such as corn (Zea mays L.) This study was developed to investigate if inoculation with Azospirillum brasilense, Bacillus subtilis or Pseudomonas fluorescens associated with P2O5 rates can improve phosphorus use efficiency (PUE) reflecting on greater corn development and yield. The field trial was set up in a Rhodic Hapludox under no-till system under Savannah conditions, in a completely randomized block design with four replicates. Treatments were tested in a full factorial design and included: (i) five P2O5 rates (0 to 105 kg ha–1) and (ii) four PGPB seed inoculation (Control–without inoculation, A. brasilense, B. subtilis or P. fluorescens). Inoculation was found to increase grain yield by 39.5, 29.1, and 15.9% when B. subtilis was inoculated in the absence of P2O5 rates and associated with 17.5 and 70 kg P2O5 ha–1 and by 34.7% when A. brasilense was inoculated with application of 105 kg P2O5 ha–1. In addition, inoculation with B. subtilis and A. brasilense were found to increase P uptake, benefiting productive components development, leading to an improved PUE, and greater corn grain yield. The results of this study showed positive improvements in P uptake as a result of B. subtilis and A. brasilense inoculation, with an increase of 100.5 and 54.6% on PUE, respectively; while the P. fluorescens inoculation were less evident. Further research should be conducted under biotic or/and abiotic conditions such as attack of pathogens and insects, drought, salinity, water flooding, low and high temperature to better understand the role of PGPB, inoculated alone or in combination as the co-inoculated method.
Introduction
Phosphorus (P) is one of the most limiting nutrients in agricultural cropping systems (Roberts and Johnston, 2015; Guignard et al., 2017; Khan et al., 2018). It is estimated that P deficiencies can be found in nearly 67% of world land designated for crop production (Dhillon et al., 2017). Also, P use efficiency (PUE) for cereal production in the world is too low, varying between 15 and 30% (Dhillon et al., 2017). Under tropical conditions, P can precipitate as minerals of Fe, and Al (Penn and Camberato, 2019). Both minerals decrease the availability of P for plant growth (Dhillon et al., 2017). Clay fractions such as amorphous hydrated oxides of Fe and Al, in addition to gibbsite, goethite, and kaolinite are responsible for the greatest P fixation (Dhillon et al., 2017). This has created a cascade of environmental problems (e.g., global warming, air pollution, and eutrophication) that threaten ecosystems and human health (Gu et al., 2015). Therefore, the P demand by crops must be considered. Plants of intense and short-cycle development, such as the corn plant (Zea mays L.), require higher amounts of P in solution and faster adsorbed-P replenishment than perennial crops (Lino et al., 2018). However, fertilizer application is one of the highest input costs for cereal crops and yet most of the P-fertilizer used to supplement crops is lost into the environment, due to the low PUE in cereal crops (Metson et al., 2016; Li et al., 2017).
The use of agroecological practices such as inoculation by plant growth-promoting bacteria (PGPB) can represent a sustainable alternative for increase nutrient use efficiency in tropical agriculture (Galindo et al., 2018a,b, 2019a,b; Martins et al., 2018). The use of these PGPB is growing, particularly in Latin America, for different crops (Souza et al., 2015; Martins et al., 2018; Galindo et al., 2019a). Several PGPB genera show association with different species of agricultural importance, such as Azospirillum, Bacillus and Pseudomonas (Zeffa et al., 2018). These bacteria can stimulate plant growth by a series of mechanisms, including but not restricted, the production of phytohormones, such as salicylic acid, gibberellins, cytokinins and indole-3-acetic acid (IAA) (Cassán and Diaz-Zorita, 2016; Fukami et al., 2017), phosphate solubilization (Ludueña et al., 2018; Qi et al., 2018), nutrient availability increase (Galindo et al., 2018b), production of indolic compounds and siderophores (Ambrosini and Passaglia, 2017), increase on 1−aminocyclopropane−1−carboxylate deaminase activity (Ambrosini and Passaglia, 2017), biological nitrogen fixation (BNF) (Pankievicz et al., 2015), biological control of plants, production of natural antibiotics and protective effect against secondary soil phytopathogens (Zhou et al., 2016; Mishra and Arora, 2018; Shameer and Prasad, 2018).
The Azospirillum spp. is considered one of the most studied plant growth promoter genera (Galindo et al., 2016, 2017). An analysis of field trials conducted worldwide for over 20 years, where various non-legume crops were inoculated with Azospirillum spp. under different weather and soil conditions, concluded that crop yield can increase up to 30% with inoculation (Fukami et al., 2016). Also, positive results in corn development and yield has been reported with Azospirillum brasilense inoculation (strains Ab-V5 and Ab-V6) under tropical conditions (Martins et al., 2018; Oliveira et al., 2018; Galindo et al., 2019b). However, greater responses with other PGPB can be achieved (Pankievicz et al., 2019). New research investigating Bacillus spp. and Pseudomonas spp. as beneficial PGPB are being conducted, especially for annual crops (Oliveira et al., 2019; Pankievicz et al., 2019; Tavanti et al., 2020). For example, under tropical conditions, Bacillus subtilis inoculation (strains Pant001 and QST713) associated with Bradyrhizobium japonicum has been reported to increase soybean [Glycine max (L.) Merr.] yield compared to single inoculation with B. japonicum, besides improving seed quality due to the increase in total storage proteins concentration, seedling emergence percentage and seed vigor (Tavanti et al., 2020). Traoré et al. (2016) reported improved corn seed germination, plant growth, plant production (increase yield by 42%) grain and shoot P biomass content of 34 and 64%, respectively with B. subtilis inoculation (strain DSM10). Lima et al. (2019) verified that B. subtilis (strains AP-3 and PRBS-1) promoted common bean (Phaseolus vulgaris L.) and corn growth, increasing the water use efficiency, leaf water content and the regulation of stomata, without damaging photosynthetic rates. Zarei et al. (2019) concluded that Pseudomonas fluorescens (P1, P3, P8, and P14 – prepared from the collection of Vali-e-Asr University of Rafsanjan) can improve plant water deficit stress tolerance, P solubilization and siderophore production, leading to an increased sweet corn (Zea mays L. var saccharata) growth and yield. Differently, Oliveira et al. (2019), studying six PGPB inoculation in soybean (A. brasilense, B. amyloliquefacens, B. licheniformis, B. pumilus, B. subtilis e P. fluorescens) associated with B. japonicum did not verified plant development and increased grain yield compared to single inoculation with B. japonicum.
Understanding the success or failure of inoculation requires understanding the complex interactions between the roots of inoculated plants, the specificity between hosts and PGPB, and the major microbial communities in the rhizosphere (Bashan et al., 2004; Florio et al., 2017, 2019). Therefore, studies with different PGPB inoculation such as Bacillus spp. and Pseudomonas spp. in tropical conditions should be performed, since new reports can be largely applicable to other important producing countries. Regardless of several benefits may be verified with PGPB inoculation, an increase in PUE and corn grain yield is not always the case (Bounaffaa et al., 2018). Further research with different PGPB inoculation associated with P2O5 rates are needed to determine how to maximize its benefits on PUE, corn development and yield. This study was based on the hypothesis of positive effect between the different PGPB inoculation and P utilization, providing greater nutrient use efficiency, reflecting on corn development and yield. The objective of this study was to evaluate the effect of A. brasilense, B. subtilis, and P. fluorescens inoculation and P2O5 application rates, on the nutritional, productive components PUE and corn grain yield under tropical conditions.
Materials and Methods
The study was conducted under field conditions in Selvíria (Savannah region), state of Mato Grosso do Sul, Brazil [20°22′ south (S) and 51°22′ west (W), 335 m above sea level (a.s.l.)], during the crop year of 2016/17. The soil was classified as clayey Oxisol (Rhodic Hapludox) according to the Soil Survey Staff (2014). The experimental area was cultivated with annual crops (cereal and legume crops) for over 30 years, with the last 12 years using a no-tillage system. The last crop sequence prior to corn was wheat. The maximum and minimum temperatures, air relative humidity and the rainfall verified during the study are presented in Figure 1.
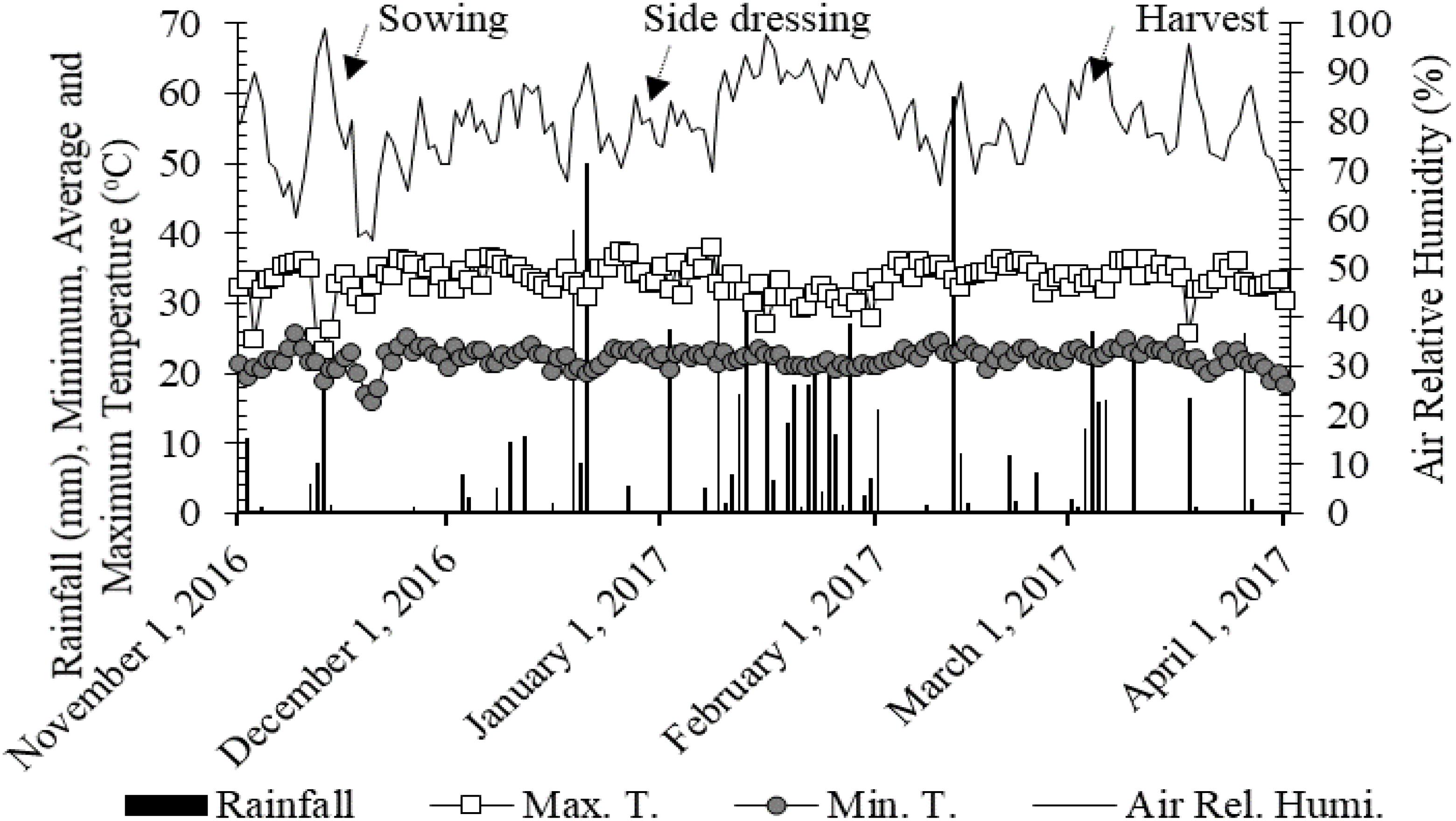
Figure 1. Rainfall, air relative humidity, maximum and minimum temperatures obtained from the weather station located in the Education and Research Farm of FE/UNESP during the corn cultivation in the period November 2016 to April 2017.
The experimental design was a completely randomized block design with four replicates arranged in a 4 × 5 factorial scheme: four PGBP seed inoculations (control–without inoculation, A. brasilense, B. subtilis and P. fluorescens and five P2O5 rates (0, 17.5, 35, 70, and 105 kg ha–1) The experimental plots were composed of seven 3.5 m corn rows spaced at the distance of 0.45 m, with the useful area of the plot being the central three rows, with the exclusion of 0.5 m from each end.
Twenty soil samples were collected, mixed and a random sub-sample was used to determine soil chemical attributes before the beginning of field trial in the 0.0–0.2 m depth. This samples were collected with soil core sample type cup auger (0.4 m × 0.10 m–cup length and diameter, respectively), randomized in the entire experimental site, regardless of experimental blocks and plots. After samples be collected and mixed, the sub-sample was dried in the shade and soil chemical attributes were determined according to the Raij et al. (2001) methodology. The following results were verified in Table 1.
Weed control was performed with herbicides application of glyphosate [1800 g ha–1 of the active ingredient (a.i)] and 2,4-D (670 g ha–1 a.i). The mineral N and K fertilization was performed with 30 kg N ha–1 (urea) and 60 kg K2O ha–1 (potassium chloride) at seedling and for all treatments, based on the soil analysis and corn crop requirements. Also, the application of P2O5 rates was performed at seedling based on soil analysis and crop requirements. The P2O5 rates (0, 17.5, 35, 70 e 105 kg ha–1) corresponds to 0, 25, 50, 100, and 150% of the recommended rate, respectively (Cantarella et al., 1997). The source of P2O5 applied was triple superphosphate (18% of P2O5, 16% of Ca, and 8% of S).
The inoculation with A. brasilense strains Ab-V5 and Ab-V6 was performed at a dose of 100 mL of liquid inoculant per hectare [equivalent to 100 mL of inoculant per 73,400 corn seeds planted – guarantee of 2 × 108 CFU (colony forming unity) mL–1]. The inoculation with B. subtilis strain CCTB04 was performed at a dose of 100 mL of liquid inoculant per hectare (equivalent to 100 mL of inoculant per 73,400 corn seeds planted – guarantee of 1 × 108 CFU mL–1). The inoculation with P. fluorescens strain CCTB03 was performed at a dose of 100 mL of liquid inoculant per hectare (equivalent to 100 mL of inoculant per 73,400 corn seeds planted – guarantee of 1 × 108 CFU mL–1). These are commercial strains used in Brazil [for both A. brasilense (brand name AzoTotal), B. subtilis (brand name Vult) and P. fluorescens (brand name Audax)]. Both inoculations (A. brasilense, B. subtilis, and P. fluorescens) were performed by coating and mixing the inoculants and corn seeds in plastic bags, manually. The seed inoculations were realized one hour before planting the corn crop and after seed treatment with fungicide and insecticide [the fungicides thiophanate-methyl + pyraclostrobin (56 g + 6 g of a.i. per 100 kg of seed) and the insecticide fipronil (62 g of a.i. per 100 kg of seed) were used], when the seeds were completely dry. The control treatment did not receive inoculations, however, the chemical seed treatment with fungicide and insecticide was performed, similarly to inoculated treatments.
The corn simple hybrid used was DOW 2B710 PW and the planting took place on November 11, being planted 73,400 seeds per ha. Seedling emergence occurred 5 days after sowing, on November 16, 2016. When necessary, the corn crop was irrigated with supplementary irrigation, using a center pivot sprinkling system (water depth of 14 mm). Weed and insect control were performed according to crop demand.
Nitrogen fertilizer (side dress application) was spread on the soil surface without incorporation by placing the fertilizer in the middle of the rows when the plants were in the V4 stage (with four leaves completely unfolded) at the dose of 150 kg N ha–1 as urea source, for all plots. After N-fertilization, the area was irrigated (14 mm depth) at night to minimize losses by ammonia volatilization. The plants were harvested manually at 125 days after emergence (DAE) on March 21, 2017.
The following nutritional evaluations were performed: (a) P foliar concentration, in g kg–1 of dry matter, was determined by collecting the middle third of 20 leaves of the main ear insertion in each experimental plot in the female flowering stage, according to the methodology described in Cantarella et al. (1997). Also, the (b) P concentration in biomass and grains were determined, at harvest time, and the P uptake in biomass and grains were calculated, in kg ha–1. P determinations in tissue and grains followed the methodology that was proposed in Malavolta et al. (1997). Five soil samples (depth of 0–0.20 m) per plot were collected at the harvest time to determine (c) P-resin according to Raij et al. (2001).
The following productive components measurements were performed: (d) plant height at maturity, defined as being at a distance (m) from the ground level to the apex of the tassel; (e) stem diameter in the second internode at corn maturation plant using a manual caliper. Ten corn spikes were collected at the harvest time to follow the evaluations: (f) spike diameter; (g) spike length, determined from the base of the spike to the apex; (h) number of rows per spike, obtained by counting the number of all rows in each spike; (i) number of grains per row of spike, determined by counting the number of grains in each row of the spike; (j) number of grains per spike, obtained by counting the number of grains in each spike; (k) mass of 100 grains, determined at 13% moisture (wet basis) by a 0.01 g precision scale; (l) phosphorus use efficiency (PUE) following the Eq. 1; and (m) grain yield, determined by the spike collection in the useful experimental area. After the mechanical track, the grains were quantified and corrected to 13% moisture (wet basis), in kg ha–1.
Where, GYF = Grain Yield with fertilizer and GYW = Grain Yield without fertilizer.
The data was analyzed by ANOVA in a 2-way factorial design with P2O5 application rates and inoculation and their interactions considered fixed effects in the model. Mean separation was done when significant factors or interactions were observed using the test Tukey. Regression analysis was used to discern whether there was a linear or non-linear response to P2O5 rates in R software (R Development Core Team, 2015).
Results
Statistical analysis showed that leaf P concentration, biomass P uptake, grain P uptake, plant height, ear length, ear diameter, number of grains per row, and grains per ear, mass of 100 grains, PUE and grain yield were significantly affected by the interaction between P2O5 rates × PGPB inoculation (Table 2). Phosphorus resin in soil and stem diameter were significantly affected by the main effects of P2O5 rates and PGPB inoculation (Table 2). Number of rows per ear was not affected by P2O5 rates, PGPB inoculation or the interaction between the factors (Table 2).
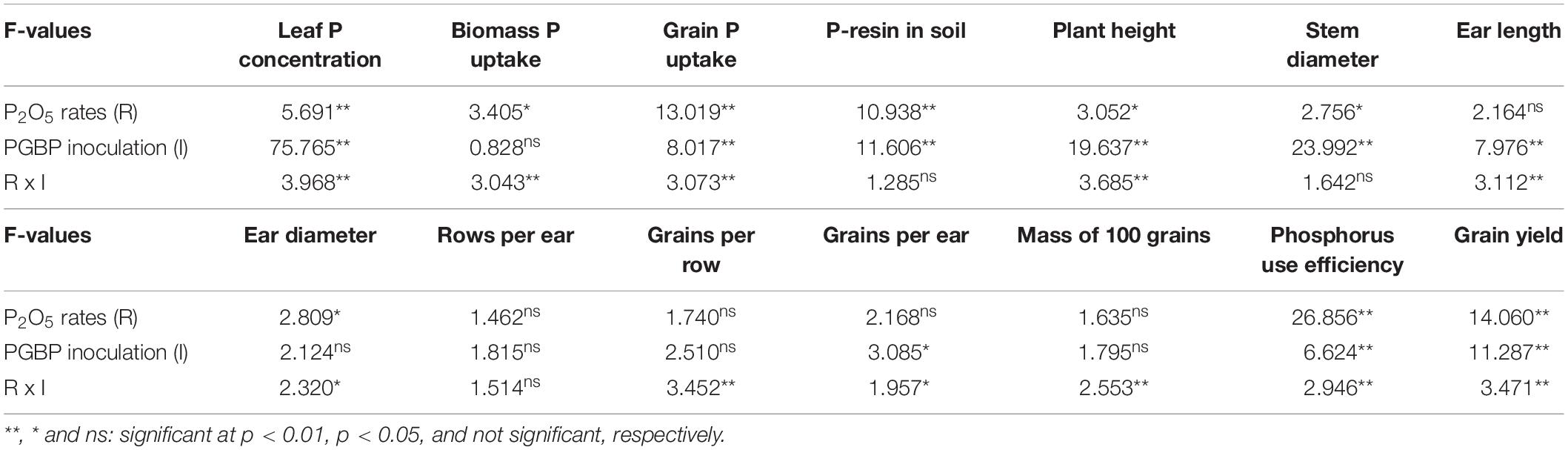
Table 2. F-values for leaf P concentration, biomass P uptake, grain P uptake, P-resin in soil, plant height, stem diameter, ear length, ear diameter, number of rows per ear, grains per row and grains per ear, mass of 100 grains, phosphorus use efficiency and corn grain yield 2016/17.
Control plots associated with low and average P2O5 application rates (0, 17.5 and 35 kg ha–1) resulted in greater leaf P concentration compared to A. brasilense, P. fluorescens and B. subtilis inoculated plots (Figure 2A). Also, control plots associated with high P2O5 rates (105 kg ha–1) resulted in greater leaf P concentration compared to A. brasilense and B. subtilis inoculated plots (Figure 2A). Leaf P concentration responded linearly to P2O5 application rates when P. fluorescens was inoculated (Figure 2A). Differently, leaf P concentration responded non-linearly to P2O5 application rates when B. subtilis was inoculated (Figure 2A).
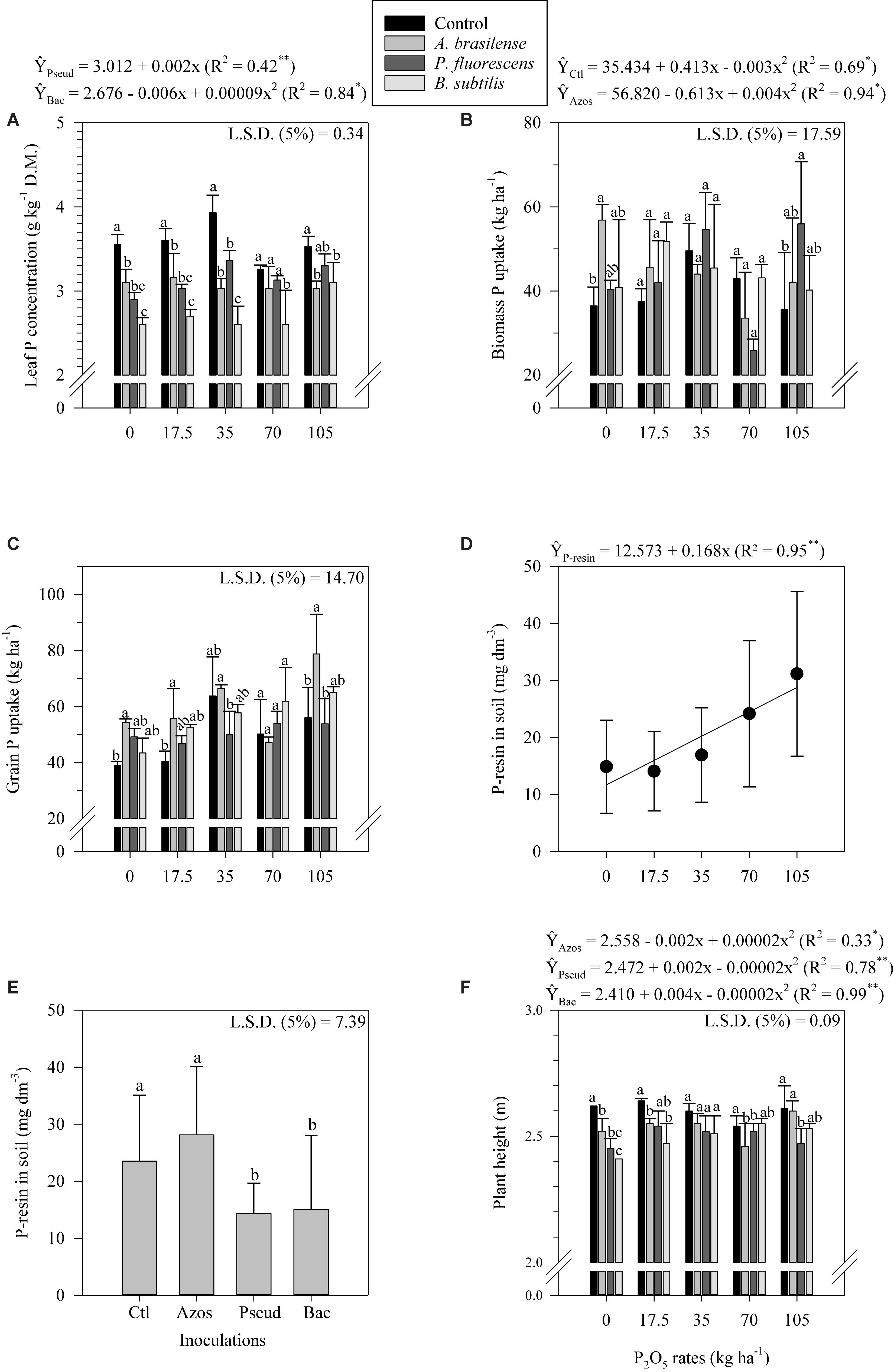
Figure 2. Leaf P concentration (A), Biomass P uptake (B), Grain P uptake (C) as a function of the interaction between P2O5 rates and inoculations, P-resin in soil as a function of P2O5 rates (D) and inoculations (E) and plant height (F) as a function of the interaction between P2O5 rates and inoculations in corn crop. The letters correspond to a significant difference at 5% probability level (p ≤ 0.05). Error bars indicate the standard deviation of the mean (n = 4). Ctl, Azos, Pseud e Bac refers to the control treatment and inoculations with A. brasilense, P. fluorescens and B. subtilis, respectively. L.S.D. (least significant difference), D.M. (dry matter).
In the absence of P2O5 application, control plots resulted in lower biomass P uptake compared to A. brasilense inoculated plots (Figure 2B). Also, control plots associated with high P2O5 application rates (105 kg ha–1) resulted in lower biomass P uptake compared to P. fluorescens inoculated plots (Figure 2B). Biomass P uptake responded non-linearly to P2O5 application rates without inoculation and when A. brasilense was inoculated (Figure 2B). The A. brasilense inoculated plots associated with low and high P2O5 application rates (0, 17.5 and 105 kg ha–1) resulted in greater grain P uptake compared to control plots (Figure 2C). In addition, A. brasilense inoculated plots associated with 35 kg P2O5 ha–1 resulted in greater grain P uptake compared to P. fluorescens inoculated plots (Figure 2C). Grain P uptake responded linearly to P2O5 application rates when B. subtilis was inoculated (Figure 2C). Differently, grain P uptake responded non-linearly to P2O5 application rates when control and A. brasilense was inoculated (Figure 2C). Phosphorus resin in soil responded linearly to P2O5 application rates (Figure 2D). In addition, control and A. brasilense inoculated plots resulted in greater P-resin in soil compared to P. fluorescens and B. subtilis inoculated plots (Figure 2E).
Control plots associated with low P2O5 application rates (0 and 17.5 kg ha–1) resulted in greater plant height compared to A. brasilense, P. fluorescens and B. subtilis inoculated plots (Figure 2F). In addition, control plots associated with 105 kg P2O5 ha–1 resulted in greater plant height compared to P. fluorescens inoculated plots (Figure 2F). However, B. subtilis inoculated plots associated with 70 kg P2O5 ha–1 resulted in greater plant height compared to A. brasilense inoculated plots (Figure 2F). Plant height responded non-linearly to P2O5 application rates when A. brasilense, P. fluorescens and B. subtilis were inoculated (Figure 2F). Stem diameter responded linearly to P2O5 application rates (Figure 3A). In addition, A. brasilense inoculated plots resulted in greater stem diameter compared to control, P. fluorescens and B. subtilis inoculated plots (Figure 3B).
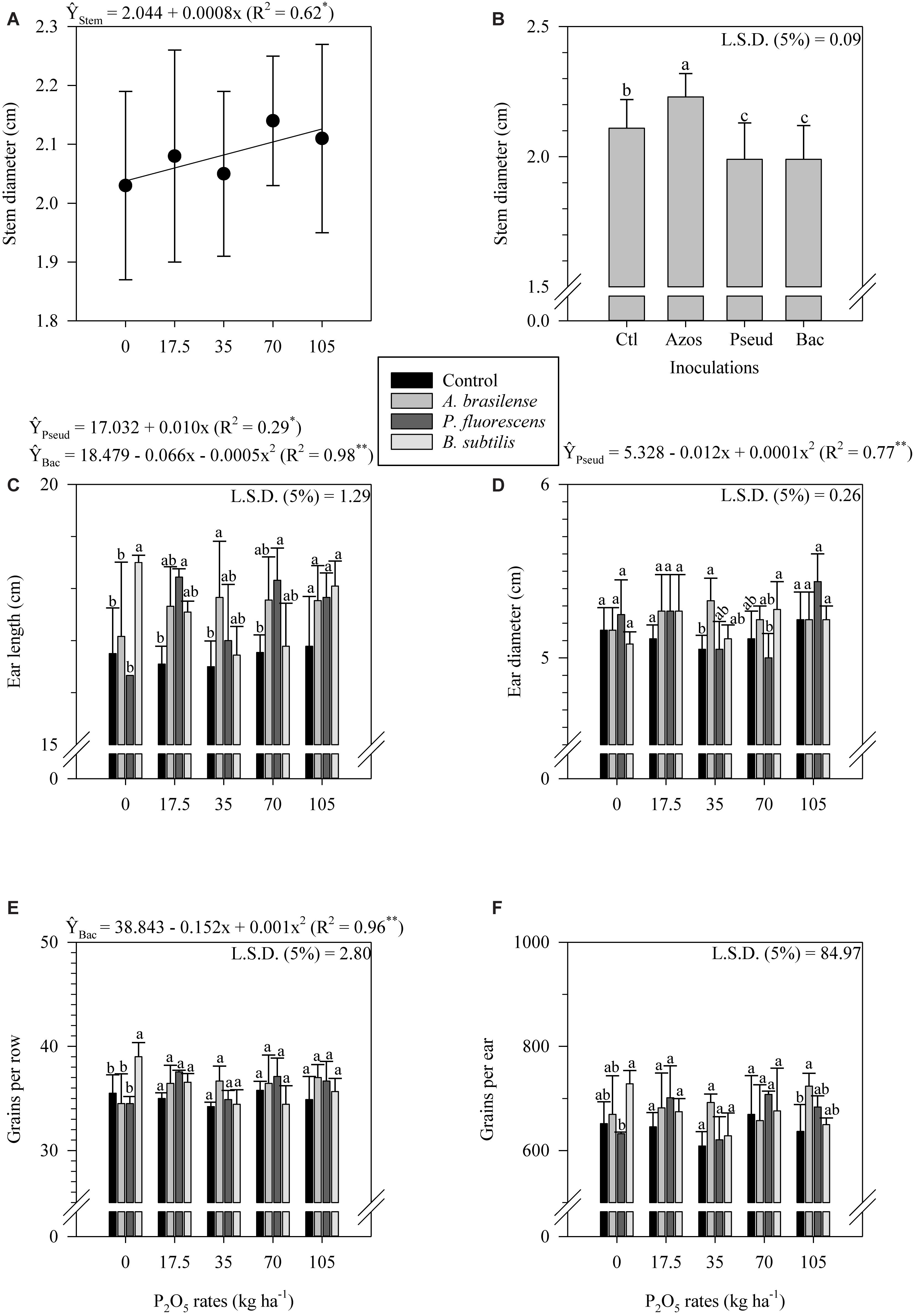
Figure 3. Stem diameter as a function of P2O5 rates (A) and inoculations (B), ear length (C), ear diameter (D), grains per row (E), and grains per ear (F) as a function of the interaction between P2O5 rates and inoculations in corn crop. The letters correspond to a significant difference at 5% probability level (p ≤ 0.05). Error bars indicate the standard deviation of the mean (n = 4). Ctl, Azos, Pseud e Bac refers to the control treatment and inoculations with A. brasilense, P. fluorescens and B. subtilis, respectively. L.S.D. (least significant difference).
Ear length fluctuated throughout the P2O5 application rates; however, in general, control plots showed reduced ear length compared to A. brasilense, P. fluorescens and B. subtilis inoculated plots (Figure 3C). Ear length responded linearly to P2O5 application rates when P. fluorescens was inoculated (Figure 3C). Differently, ear length responded non-linearly to P2O5 application rates when B. subtilis was inoculated (Figure 3C). Control plots and P. fluorescens inoculated plots associated with average P2O5 rates (35 and 70 kg ha–1) tended to result in lower ear diameter compared to A. brasilense and B. subtilis inoculated plots (Figure 3D). Ear diameter responded non-linearly to P2O5 application rates when control and P. fluorescens was inoculated (Figure 3D).
In the absence of of P2O5 application, B. subtilis inoculated plots resulted in greater number of grains per row compared to control, A. brasilense and P. fluorescens inoculated plots (Figure 3E). Grains per row responded non-linearly to P2O5 application rates when control and B. subtilis was inoculated (Figure 3E). Similarly, in the absence of of P2O5 application, B. subtilis inoculated plots resulted in greater number of grains per ear compared to control, A. brasilense and P. fluorescens inoculated plots (Figure 3F). Also, A. brasilense inoculated plots associated with 105 kg P2O5 ha–1 resulted in greater number of grains per ear compared to control plots (Figure 3F). Control plots and P. fluorescens inoculated plots associated with average and high P2O5 application rates (70 and 105 kg ha–1) tended to result in lower mass of 100 grains compared to A. brasilense and B. subtilis inoculated plots (Figure 4A). Mass of 100 grains responded linearly to P2O5 application rates when B. subtilis was inoculated (Figure 4A). Differently, mass of 100 grains responded non-linearly to P2O5 application rates when control and A. brasilense and P. fluorescens were inoculated (Figure 4A).
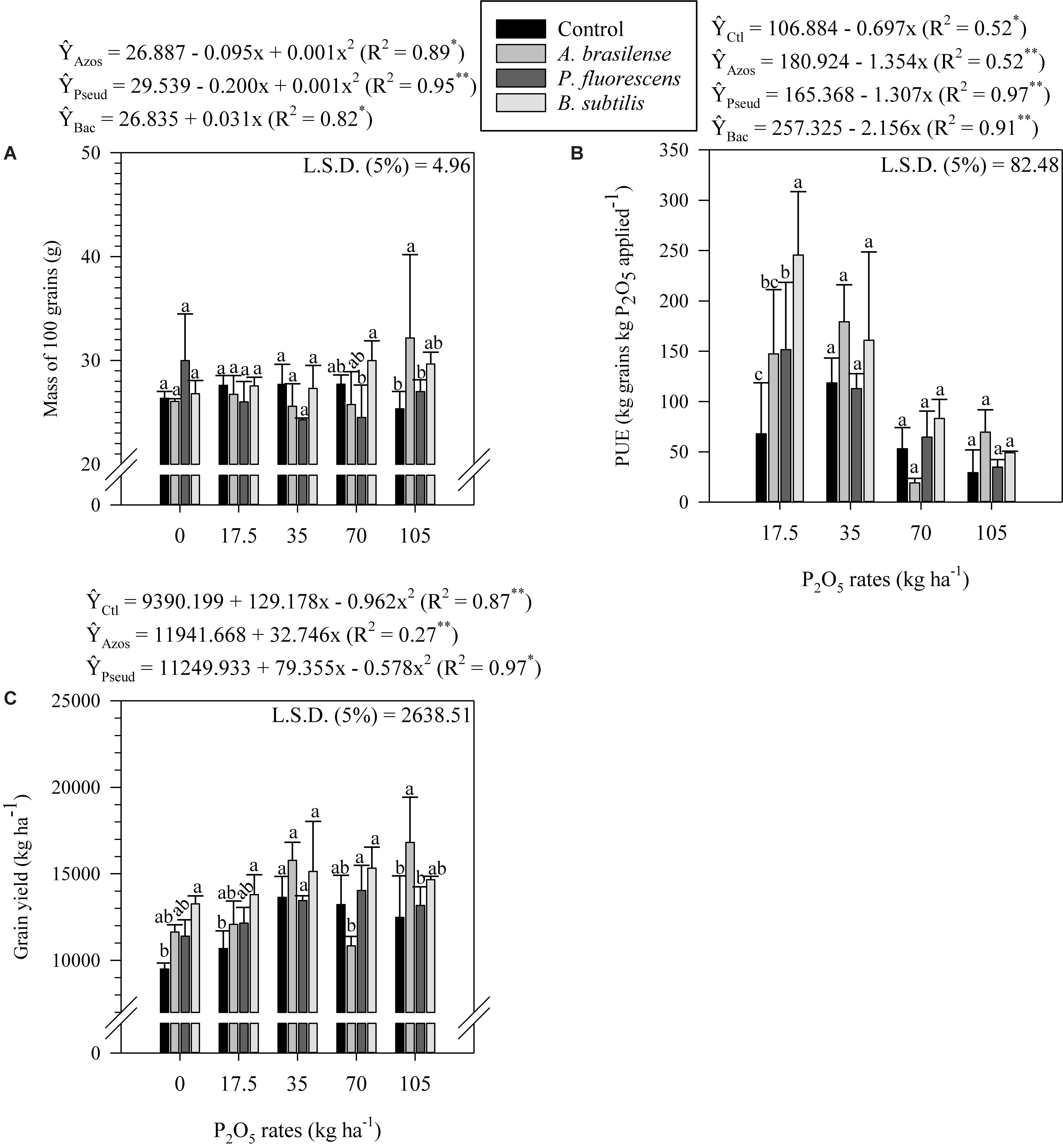
Figure 4. Mass of 100 grains (A), PUE (B), and grain yield (C) as a function of the interaction between P2O5 rates and inoculations in corn crop. The letters correspond to a significant difference at 5% probability level (p ≤ 0.05). Error bars indicate the standard deviation of the mean (n = 4). Ctl, Azos, Pseud e Bac refers to the control treatment and inoculations with A. brasilense, P. fluorescens and B. subtilis, respectively. L.S.D. (least significant difference), PUE (P use efficiency).
The B. subtilis inoculated plots associated with 17.5 kg P2O5 ha–1 resulted in greater PUE compared to control, A. brasilense and P. fluorescens inoculated plots (Figure 4B). Phosphorus use efficiency responded linearly to P2O5 application rates regardless of inoculations (Figure 4B). The B. subtilis inoculation also benefited grain yield when associated with low P2O5 rates (0 and 17.5 kg ha–1) as verified by greater grain yield compared to control (Figure 4C). The A. brasilense inoculated plots associated with 70 kg P2O5 ha–1 resulted in lower grain yield compared to P. fluorescens and B. subtilis inoculated plots (Figure 4C). However, A. brasilense inoculated plots associated with 105 kg P2O5 ha–1 resulted in greater grain yield compared to control and P. fluorescens inoculated plots (Figure 4C). Grain yield responded linearly to P2O5 application rates when A. brasilense was inoculated (Figure 4C). Differently, grain yield responded non-linearly to P2O5 application rates when control and P. fluorescens were inoculated (Figure 4C).
Discussion
Phosphorus is the second nutrient that is most demanded by corn plants and directly affects crop development and yield (Dhillon et al., 2017). Thus, the higher P availability as a function of the P2O5 application probably favored initial root system development, reflecting on corn grain yield. The better growth of P2O5-fertilized plants can be attributed to the P readily available for absorption after being added to the soil as verified by the linear increasing response in P content in soil to P2O5 application rates. Phosphorus plays important roles in plant nutrition and development (Lollato et al., 2019), for example composition of adenosine triphosphate (ATP). In addition, P is responsible for the storage and transport of energy for endergonic processes, such as the synthesis of organic compounds and the active uptake of nutrients (Marschner, 2012). Also, P is related to root system development and plant growth (Sulieman and Tran, 2015; Fink et al., 2016; Zhang et al., 2016). There are several studies reporting the P fertilization benefits in corn crop (Wen et al., 2016; Li et al., 2017; Schlegel and Havlin, 2017; Ortas and Islam, 2018; Preston et al., 2019). However, plant nutritional demand is limited as verified by the linear decreasing response in PUE to P2O5 application rates, regardless of PGPB inoculation. In addition, almost 80% of P content in soil can be fixed in forms unavailable to plants (White and Hammond, 2008; Zhang et al., 2016). Therefore, P application must be rational and optimized, since the increased P2O5 application rates results in increased losses and less utilization by cropping systems.
Although the response to PGPB inoculation under P2O5 application rates were different, the response to B. subtilis and A. brasilense inoculation associated with P2O5 rates were greater than control and P. fluorescens inoculation mainly when P. fluorescens inoculation was associated with P2O5 application rates. We verified this behavior on lower grain P uptake (associated with 35 and 105 kg P2O5 ha–1), ear diameter (with 35 and 70 kg P2O5 ha–1), mass of 100 grains (with 70 and 105 kg P2O5 ha–1) and grain yield (with 105 kg P2O5 ha–1) when P. fluorescens inoculation was performed. Positive responses to B. subtilis inoculation were verified mainly in the absence and application of 70 kg P2O5 ha–1. In the absence of P2O5 application rates B. subtilis inoculation showed greater ear length, number of grains per row and grains per ear. With application of 70 kg P2O5 ha–1, B. subtilis inoculation showed greater ear diameter and mass of 100 grains. In addition, when 17.5 kg P2O5 ha–1 was applied, B. subtilis inoculation showed greater PUE. Grain yield was also positive affected by B. subtilis inoculation in the absence of P2O5 application rates (increase of 39.5, 13.9, and 16.3%), 17.5 kg P2O5 ha–1 (increase of 29.1, 14.2, and 13.5%) and 70 kg P2O5 ha–1 (increase of 15.9, 41.3, and 9.2 compared to control, A. brasilense and P. fluorescens, respectively). Similarly, positive responses to A. brasilense inoculation were verified mainly with application of 35 and 105 kg P2O5 ha–1. With application of 35 kg P2O5 ha–1, A. brasilense inoculation showed greater ear length and ear diameter. When 105 kg P2O5 ha–1 was applied, A. brasilense showed greater mass of 100 grains and grain yield. Grain yield increased by 34.7, 27.7, and 14.8% compared to control, P. fluorescens and B. subtilis inoculated treatments, respectively, when A. brasilense was inoculated and 105 kg P2O5 ha–1was applied. In addition, regardless of P2O5 application rates, A. brasilense inoculation showed greater P-resin in soil (increase of 19.7, 96.7, and 87.2%) and stem diameter (increase of 5.7, 12.1, and 12.1% compared to control, P. fluorescens and B. subtilis, respectively).
There was a dilution effect on P concentration as a function of B. subtilis and A. brasilense inoculation. It was observed that B. subtilis and A. brasilense inoculation promoted greater P uptake, optimizing the use of the absorbed P by corn plant. Our results showed that the inoculation with B. subtilis provided an increase of 9.7% for biomass P uptake (40.37 to 44.28 kg ha–1, respectively) and 12.6% for grain P uptake (49.87 to 56.13 kg ha–1, respectively) compared to control, regardless of P2O5 application rates. Also, the inoculation with A. brasilense provided an increase of increase of 10.% for biomass P uptake (40.37 to 44.42 kg ha–1) and 21.3% for grain P uptake (49.87 to 60.50 kg ha–1, respectively) compared to control, regardless of P2O5 application rates. The exact mechanisms underlying the B. subtilis and A. brasilense effect on corn growth was not evaluated in the present study, however, it is very likely that the improvement in P availability and uptake reflecting on improved PUE and grain yield by corn verified in this study is associated with the well-known ability of A. brasilense and B. subtilis to promote plant growth (Fukami et al., 2017; Jang et al., 2018; Martins et al., 2018; Posada et al., 2018; Salvo et al., 2018). The increase on PUE and corn yield as a function B. subtilis and A. brasilense inoculation compared to control was, on average, equivalent to 100.5 and 54.6% for PUE and 17.4 and 12.8% for grain yield, respectively, regardless of P2O5 application rates. Similar results with B. subtilis and A. brasilense inoculation were reported with greater corn yield between 3.8 to 34% in inoculated plants compared with non-inoculated plants (Kuan et al., 2016; Müller et al., 2016; Traoré et al., 2016; Ahmad et al., 2019; Galindo et al., 2019b).
The bacteria B. subtilis is well known to possess properties of plant growth promotion, phosphate solubilization, phytopathogen inhibition and heavy metal absorption (Traoré et al., 2016; Rekha et al., 2017; Muñoz-Moreno et al., 2018; Prakash and Arora, 2019). In addition, the strains Ab-V5 and Ab-V6 of A. brasilense share the genes related to the synthesis of auxins (Hungria et al., 2018). Also, Azospirillum spp. inoculation is related to nutrient availability increase (Galindo et al., 2018b) and BNF (Pankievicz et al., 2015). This growth promotion mechanisms might have improved the ability of the plants to more efficiently explore the soil and uptake P, as indicated in previous studies using B. subtilis and A. brasilense (Traoré et al., 2016; Martins et al., 2018; Prakash and Arora, 2019; Zeffa et al., 2019). According to Cormier et al. (2013), two strategies may be devised for nutrient use efficiency improvement: increasing the yield at a constant nutrient supply and/or maintaining high yield when reducing nutrient supply. Therefore, the results of our research show that inoculation with B. subtilis and A. brasilense may be a potential strategy to help improving PUE. It is not unusual that PGPB displays several different plant-beneficial properties (Bruto et al., 2014; Vacheron et al., 2016), which is thought to provide higher positive effects on the plant (Bashan and de-Bashan, 2010). This is expected to take place because (i) the effects of different modes of action may add-up quantitatively, or (ii) it could ensure that at least one mode of action is expressed in particular environmental conditions (Vacheron et al., 2016). Indeed, the most effective PGPB are typically multi-function strains (Almario et al., 2014).
The inoculation with P. fluorescens had little quantifiable effect on P uptake, corn development and grain yield. However, P. fluorescens has been reported to favor biological control of plants, production of natural antibiotics and protective effect against secondary soil phytopathogens (Garrido-Sanz et al., 2016), phosphate solubilization (Oteino et al., 2015) and N2 fixation (Vacheron et al., 2016). Also, P. fluorescens resulted in 35.3 and 7.8% increase in PUE and corn grain yield compared to control treatment, respectively, regardless of P2O5 application rates. Evidently, there is still great divergence in the use of PGPB in corn and other grasses due to the variable results with inoculation. However, it is important to highlight the importance of research on the subject and the potential of using this technology, mainly because it is easy to apply, is low cost, and has a great potential to promote plant growth.
Conclusion
Inoculation with B. subtilis and A. brasilense associated with P2O5 application rates were found to increase P uptake, benefiting productive components development, leading to an improved PUE, and greater corn grain yield. Yield increased by 39.5, 29.1, and 15.9% when plants were inoculated with B. subtilis in the absence of P2O5 application rates, associated with 17.5 and 70 kg P2O5 ha–1, respectively. Inoculation with A. brasilense increased grain yield by 34.7% when 105 kg P2O5 ha–1 was applied, showing the potential for improve PUE by the B. subtilis and A. brasilense inoculation, positively reflecting on corn grain yield. The inoculation with P. fluorescens had small effects on P uptake, plant development and grain yield, however, resulted in 35.3 and 7.8% increase on PUE and corn grain yield compared to control. Therefore, studies conducted under biotic or/and abiotic conditions are necessary to better understand the role of PGPB, inoculated alone or in combination as the co-inoculated method.
Data Availability Statement
The datasets generated for this study are available on request to the corresponding author.
Author Contributions
FG and MT wrote the manuscript, with contributions from NP. MT, RG, and ED corrected and improved the manuscript. NP, FG, PR, and EM conducted the samplings and data collection. NP did the analysis, with the support of MT and FG.
Funding
This research was funded by FAPESP (Fundação de Amparo à Pesquisa do Estado de São Paulo), grant number 17/01881-1, and CNPq (Conselho Nacional de desenvolvimento Científico e Tecnológico), award number 312359/2017-9.
Conflict of Interest
The authors declare that the research was conducted in the absence of any commercial or financial relationships that could be construed as a potential conflict of interest.
References
Ahmad, M., Adil, Z., Hussain, A., Mumtaz, M. Z., Nafees, M., Ahmad, I., et al. (2019). Potential of phosphate solubilizing Bacillus strains for improving growth and nutrient uptake in mungbean and maize crops. Pak. J. Agri. Sci. 56, 283–289. doi: 10.21162/PAKJAS/19.7285
Almario, J., Müller, D., Défago, G., and Moënne-Loccoz, Y. (2014). Rhizosphere ecology and phytoprotection in soils naturally suppressive to Thielaviopsis black root rot of tobacco. Environ. Microbiol. 16, 1949–1960. doi: 10.1111/1462-2920.12459
Ambrosini, A., and Passaglia, L. M. P. (2017). Plant growth–promoting bacteria (PGPB): isolation and screening of PGP activities. Curr. Prot. Plant Biol. 2, 190–209. doi: 10.1002/pb.20054
Bashan, Y., and de-Bashan, L. E. (2010). Chapter two – How the plant growth-promoting bacterium Azospirillum promotes plant growth – a critical assessment. Adv. Agron. 108, 77–136. doi: 10.1016/S0065-2113(10)08002-8
Bashan, Y., Holguin, G., and de-Bashan, L. E. (2004). Azospirillum-plant relationships: physiological, molecular, agricultural, and environmental advances (1997-2003). Can. J. Microbiol. 50, 521–577. doi: 10.1139/w04-035
Bounaffaa, M., Florio, A., Le Roux, X., and Jayet, P.-A. (2018). Economic and environmental analysis of maize inoculation by plant growth promoting rhizobacteria in the French Rhône-Alpes region. Ecol. Econ. 146, 334–346. doi: 10.1016/j.ecolecon.2017.11.009
Bruto, M., Prigent-Combaret, C., Müller, D., and Moënne-Loccoz, Y. (2014). Analysis of genes contributing to plant-beneficial functions in plant growth-promoting rhizobacteria and related Proteobacteria. Sci. Rep. 4:6261. doi: 10.1038/srep06261
Cantarella, H., Raij, B., and Camargo, C. E. O. (1997). “Cereals,” in Liming and fertilization Recommendations for the State of São Paulo, eds B. Raij, H. van Cantarella, J. A. Quaggio, and A. M. C. Furlani (Campinas: Instituto Agronômico de Campinas), 285.
Cassán, B., and Diaz-Zorita, M. (2016). Azospirillum sp. in current agriculture: from the laboratory to the field. Soil Biol. Biochem. 103, 117–130. doi: 10.1016/j.soilbio.2016.08.020
Cormier, F., Faure, S., Dubreuil, P., Heumez, E., Beauchêne, K., Lafarge, S., et al. (2013). A multi-environmental study of recent breeding progress on nitrogen use efficiency in wheat (Triticum aestivum L.). Theor. Appl. Genet. 126, 3035–3048. doi: 10.1007/s00122-013-2191-9
Dhillon, J., Torres, G., Driver, E., Figueiredo, B., and Raun, W. R. (2017). World phosphorus use efficiency in cereal crops. Agron. J. 109, 1670–1677. doi: 10.2134/agronj2016.08.0483
Fink, J. R., Inda, A. V., Bavaresco, J., Sánchez-Rodríguez, A. R., Barrón, V., Torrent, J., et al. (2016). Diffusion and uptake of phosphorus, and root development of corn seedlings, in three contrasting subtropical soils under conventional tillage or no-tillage. Biol. Fert. Soils 52, 203–210. doi: 10.1007/s00374-015-1067-3
Florio, A., Bréfort, C., Gervaix, J., Bérard, A., and Le Roux, X. (2019). The responses of NO2- – and N2O-reducing bacteria to maize inoculation by the PGPR Azospirillum lipoferum CRT1 depend on carbon availability and determine soil gross and net N2O production. Soil Biol. Biochem. 136:107524. doi: 10.1016/j.soilbio.2019.107524
Florio, A., Pommier, T., Gervaix, J., Bérard, A., and Le Roux, X. (2017). Soil C and N statuses determine the effect of maize inoculation by plant growth-promoting rhizobacteria on nitrifying and denitrifying communities. Sci. Rep. 7:8411. doi: 10.1038/s41598-017-08589-4
Fukami, J., Nogueira, M. A., Araujo, R. S., and Hungria, M. (2016). Accessing inoculation methods of maize and wheat with Azospirillum brasilense. AMB Express 6, 3–16. doi: 10.1186/s13568-015-0171-y
Fukami, J., Ollero, F. J., Megías, M., and Hungria, M. (2017). Phytohormones and induction of plant-stress tolerance and defense genes by seed and foliar inoculation with Azospirillum brasilense cells and metabolites promote maize growth. AMB Express 7, 153–163. doi: 10.1186/s13568-017-0453-7
Galindo, F. S., Rodrigues, W. L., Biagini, A. L. C., Fernandes, G. C., Baratella, E. B., da Silva Junior, C. A., et al. (2019a). Assessing forms of application of Azospirillum brasilense associated with silicon use on wheat. Agron 9:678.
Galindo, F. S., Teixeira Filho, M. C. M., Buzetti, S., Pagliari, P. H., Santini, J. M. K., Alves, C. J., et al. (2019b). Maize yield response to nitrogen rates and sources associated with Azospirillum brasilense. Agron. J. 111, 1985–1997. doi: 10.2134/agronj2018.07.0481
Galindo, F. S., Teixeira Filho, M. C. M., Buzetti, S., Rodrigues, W. L., Boleta, E. H. M., Rosa, P. A. L., et al. (2018a). Technical and economic viability of corn with Azospirillum brasilense associated with acidity correctives and nitrogen. J. Agri. Sci. 10, 213–227. doi: 10.5539/jas.v10n3p213
Galindo, F. S., Teixeira Filho, M. C. M., Buzetti, S., Rodrigues, W. L., Fernandes, G. C., Boleta, E. H. M., et al. (2018b). Nitrogen rates associated with the inoculation of Azospirillum brasilense and application of Si: effects on micronutrients and silicone concentration in irrigated corn. Open Agric. 3, 510–523. doi: 10.1515/opag-2018-0056
Galindo, F. S., Teixeira Filho, M. C. M., Buzetti, S., Santini, J. M. K., Alves, C. J., and Ludkiewicz, M. G. Z. (2017). Wheat yield in the Cerrado as affected by nitrogen fertilization and inoculation with Azospirillum brasilense. Pesq. Agropec. Bras. 52, 794–805. doi: 10.1590/S0100-204X2017000900012
Galindo, F. S., Teixeira Filho, M. C. M., Buzetti, S., Santini, J. M. K., Alves, C. J., Nogueira, L. M., et al. (2016). Corn yield and foliar diagnosis affected by nitrogen fertilization and inoculation with Azospirillum brasilense. Rev. Brasil. Ciênc. Solo 40:e015036. doi: 10.1590/18069657rbcs20150364
Garrido-Sanz, D., Meier-Kolthoff, J. P., Göker, M., Martín, M., Rivilla, R., and Redondo-Nieto, M. (2016). Genomic and genetic diversity within the Pseudomonas fluorescens complex. PLoS One 11:e0153733. doi: 10.1371/journal.pone.0153733
Gu, B., Ju, X., Chang, J., Ge, Y., and Vitousek, P. M. (2015). Integrated reactive nitrogen budgets and future trends in China. Proc. Nat. Acad. Sci. U.S.A. 112, 8792–8797. doi: 10.1073/pnas.1510211112
Guignard, M. S., Leitch, A. R., Acquisti, C., Eizaguirre, C., Elser, J. J., Hessen, D. O., et al. (2017). Impacts of nitrogen and phosphorus: from genomes to natural ecosystems and agriculture. Front. Ecol. Evol. 5:70. doi: 10.3389/fevo.2017.00070
Hungria, M., Ribeiro, R. A., and Nogueira, M. A. (2018). Draft genome sequences of Azospirillum brasilense strains Ab-V5 and Ab-V6, commercially used in inoculants for grasses and legumes in Brazil. Genome Announc. 6:e0393-18. doi: 10.1128/genomeA.00393-18
Jang, J. H., Kim, S.-H., Khaine, I., Kwak, M. J., Lee, H. K., Lee, T. Y., et al. (2018). Physiological changes and growth promotion induced in poplar seedlings by the plant growth-promoting rhizobacteria Bacillus subtilis JS. Photosynthetica 56, 1188–1203. doi: 10.1007/s11099-018-0801-0
Khan, A., Lu, G., Ayaz, M., Zhang, H., Wang, R., Lv, F., et al. (2018). Phosphorus efficiency, soil phosphorus dynamics and critical phosphorus level under long-term fertilization for single and double cropping systems. Agric. Ecosyst. Environ. 256, 1–11. doi: 10.1016/j.agee.2018.01.006
Kuan, K. B., Othman, R., Rahim, K. A., and Shamsuddin, Z. H. (2016). Plant growth-promoting rhizobacteria inoculation to enhance vegetative growth, nitrogen fixation and nitrogen remobilisation of maize under greenhouse conditions. PLoS One 11:e0152478. doi: 10.1371/journal.pone.0152478
Li, H., Mollier, A., Ziadi, N., Shi, Y., Parent, L. -É, and Morel, C. (2017). The long-term effects of tillage practice and phosphorus fertilization on the distribution and morphology of corn root. Plant Soil 412, 97–114. doi: 10.1007/s11104-016-2925-y
Lima, B. C., Moro, A. L., Santos, A. C. P., Bonifacio, A., Araujo, A. S. F., and de Araujo, F. F. (2019). Bacillus subtilis ameliorates water stress tolerance in maize and common bean. J. Plant Interac. 14, 432–439. doi: 10.1080/17429145.2019.1645896
Lino, A. C. M., Buzetti, S., Teixeira Filho, M. C. M., Galindo, F. S., Maestrelo, P. R., and Rodrigues, M. A. C. (2018). Effect of phosphorus applied as monoammonium phosphate-coated polymers in corn culture under no-tillage system. Semina 39, 99–112. doi: 10.5433/1679-0359.2018v39n1p99
Lollato, R. P., Figueiredo, B. M., Dhillon, J. S., Arnall, D. B., and Raun, W. R. (2019). Wheat grain yield and grain-nitrogen relationships as affected by N, P, and K fertilization: a synthesis of long-term experiments. Field Crops Res. 236, 42–57. doi: 10.1016/j.fcr.2019.03.005
Ludueña, L. M., Anzuay, M. S., Angelini, J. G., McIntosh, M., Becker, A., Rupp, O., et al. (2018). Strain Serratia sp. S119: a potential biofertilizer for peanut and maize and a model bacterium to study phosphate solubilization mechanisms. Appl. Soil Ecol. 126, 107–112. doi: 10.1016/j.apsoil.2017.12.024
Malavolta, E., Vitti, G. C., and Oliveira, S. A. (1997). Evaluation of the Nutritional Status of Plants: Principles and Applications, 2nd Edn, Piracicaba: Universidade de São Paulo.
Marschner, P. (2012). Marschner’s Mineral Nutrition Of Higher Plants, 3rd Edn, New York, NY: Academic Press.
Martins, M. R., Jantalia, C. P., Reis, V. M., Döwich, I., Polidoro, J. C., Alves, B. J. R., et al. (2018). Impact of plant growth-promoting bacteria on grain yield, protein content, and urea-15 N recovery by maize in a cerrado oxisol. Plant Soil 422, 239–250. doi: 10.1007/s11104-017-3193-1
Metson, G. S., MacDonald, G. K., Haberman, D., Nesme, T., and Bennett, E. M. (2016). Feeding the corn belt: opportunities for phosphorus recycling in U.S. agriculture. Sci. Total Environ. 542, 1117–1126. doi: 10.1016/j.scitotenv.2015.08.047
Mishra, J., and Arora, N. K. (2018). Secondary metabolites of fluorescent pseudomonads in biocontrol of phytopathogens for sustainable agriculture. Appl. Soil Ecol. 125, 35–45. doi: 10.1016/j.apsoil.2017.12.004
Moll, R. H., Kamprath, E. J., and Jackson, W. A. (1982). Analysis and interpretation of factors which contribute to efficiency of nitrogen utilization. Agron. J. 74, 562–564.
Müller, T. M., Sandini, I. E., Rodrigues, J. D., Novakowiski, J. H., Basi, S., and Kaminski, T. H. (2016). Combination of inoculation methods of Azospirilum brasilense with broadcasting of nitrogen fertilizer increases maize yield. Circ. Rural 46, 210–215. doi: 10.1590/0103-8478cr20131283
Muñoz-Moreno, C. Y., Cruz-Rodríguez, Y., Vega-Arreguín, J., Alvarado-Rodríguez, M., Gómez-Soto, J. M., Alvarado-Gutiérrez, A., et al. (2018). Draft genome sequence of Bacillus subtilis 2C-9B, a strain with biocontrol potential against chili pepper root pathogens and tolerance to Pb and Zn. Genome Announc. 6, e1502–e1517. doi: 10.1128/genomeA.01502-17
Oliveira, I. J., Fontes, J. R. A., Pereira, B. F. F., and Muniz, A. W. (2018). Inoculation with Azospirillum brasiliense increases maize yield. Chem. Biol. Technol. Agric. 5:6. doi: 10.1186/s40538-018-0118-z
Oliveira, L. B. G., Teixeira Filho, M. C. M., Galindo, F. S., Nogueira, T. A. R., Barco Neto, M., and Buzetti, S. (2019). Forms and types of coinoculation in the soybean crop in Cerrado region (In Portuguese, abstract in english). Rev. Cienc. Agrar. 42, 924–932. doi: 10.19084/rca.15828
Ortas, I., and Islam, K. R. (2018). Phosphorus fertilization impacts on corn yield and soil fertility. Commun. Soil Sci. Plant Anal. 49, 1684–1694. doi: 10.1080/00103624.2018.1474906
Oteino, N., Lally, R. D., Kiwanuka, S., Lloyd, A., Ryan, D., Germaine, K. J., et al. (2015). Plant growth promotion induced by phosphate solubilizing endophytic Pseudomonas isolates. Front. Microbiol. 6:745. doi: 10.3389/fmicb.2015.00745
Pankievicz, V. C. S., Amaral, F. P., Santos, K. F. D. N., Agtuca, B., Xu, Y., Schueller, M. J., et al. (2015). Robust biological nitrogen fixation in a model grass-bacterial association. Plant J. 81, 907–919. doi: 10.1111/tpj.12777
Pankievicz, V. C. S., Irving, T. B., Maia, L. G. S., and Ané, J. M. (2019). Are we there yet? The long walk towards the development of efficient symbiotic associations between nitrogen-fixing bacteria and non-leguminous crops. BMC Biol. 17:99. doi: 10.1186/s12915-019-0710-0
Penn, C. J., and Camberato, J. (2019). A critical review on soil chemical processes that control how soil pH affects phosphorus availability to plants. Agriculture 9:120. doi: 10.3390/agriculture9060120
Posada, L. E., Álvarez, J. C., Romero-Tabarez, M., de-Bashan, L., and Villegas-Escobar, V. (2018). Enhanced molecular visualization of root colonization and growth promotion by Bacillus subtilis EA-CB0575 in different growth systems. Microbiol. Res. 217, 69–80. doi: 10.1016/j.micres.2018.08.017
Prakash, J., and Arora, N. K. (2019). Phosphate-solubilizing Bacillus sp. enhances growth, phosphorus uptake and oil yield of Mentha arvensis. L. 3 Biotech. 9:126. doi: 10.1007/s13205-019-1660-5
Preston, C. L., Diaz, D. A. R., and Mengel, D. B. (2019). Corn response to long-term phosphorus fertilizer application rate and placement with strip-tillage. Agron. J. 111, 841–850. doi: 10.2134/agronj2017.07.0422
Qi, G., Pan, Z., Sugawa, Y., Andriamanohiarisoamanana, F. J., Yamashiro, T., Iwasaki, M., et al. (2018). Comparative fertilizer properties of digestates from mesophilic and thermophilic anaerobic digestion of dairy manure: focusing on plant growth promoting bacteria (PGPB) and environmental risk. J. Mat. Cycles Waste Manag. 20, 1–10. doi: 10.1007/s10163-018-0708-7
R Core Team (2015). R: A Language and Environment for Statistical Computing. Vienna: R Foundation for Statistical Computing.
Raij, B., van Andrade, J. C., Cantarella, H., and Quaggio, J. A. (2001). Chemical Analysis for Fertility Evaluation Of Tropical Soils. Campinas: IAC.
Rekha, K., Baskar, B., Srinath, S., and Usha, B. (2017). Plant-growth-promoting rhizobacteria Bacillus subtilis RR4 isolated from rice rhizosphere induces malic acid biosynthesis in rice roots. Can J. Microbiol. 18, 1–8. doi: 10.1139/cjm-2017-0409
Roberts, T. L., and Johnston, A. E. (2015). Phosphorus use efficiency and management in agriculture. Res. Cons. Rec. Part B 105, 275–281. doi: 10.1016/j.resconrec.2015.09.013
Salvo, L. P., Ferrando, L., Fernandéz-Scavino, A., and Salamone, I. E. G. (2018). Microorganisms reveal what plants do not: wheat growth and rhizosphere microbial communities after Azospirillum brasilense inoculation and nitrogen fertilization under field conditions. Plant Soil 424, 405–417. doi: 10.1007/s11104-017-3548-7
Schlegel, A. J., and Havlin, J. L. (2017). Corn yield, grain nutrient uptake from 50 years of nitrogen and phosphorus fertilization. Agron. J. 109, 335–342. doi: 10.2134/agronj2016.05.0294
Shameer, S., and Prasad, T. N. V. K. V. (2018). Plant growth promoting rhizobacteria for sustainable agricultural practices with special reference to biotic and abiotic stresses. Plant Growth Reg. 84, 603–615. doi: 10.1007/s10725-017-0365-1
Souza, R., Ambrosini, A., and Passaglia, L. M. P. (2015). Plant growth-promoting bacteria as inoculants in agricultural soils. Gen. Mol. Biol. 38, 401–419. doi: 10.1590/S1415-475738420150053
Sulieman, S., and Tran, L.-S. P. (2015). Phosphorus homeostasis in legume nodules as an adaptive strategy to phosphorus deficiency. Plant Sci. 239, 36–43. doi: 10.1016/j.plantsci.2015.06.018
Tavanti, T. R., Tavanti, R. F. R., Galindo, F. S., Simões, I., Dameto, L. S., and de Sá, M. E. (2020). Yield and quality of soybean seeds inoculated with Bacillus subtilis strains. Rev. Brasil. Eng. Agríc. Amb. 24, 65–71. doi: 10.1590/1807-1929/agriambi.v24n1p65-71
Traoré, L., Babana, H., Antoun, H., Lahbib, M., Sacko, O., Nakatsu, C., et al. (2016). Isolation of six phosphate dissolving rhizosphere bacteria (Bacillus subtilis) and their effects on the growth, phosphorus nutrition and yield of maize (Zea mays L.) in Mali. J. Agric. Sci. Technol. B. 6, 93–107. doi: 10.17265/2161-6264/2016.02.005
Vacheron, J., Moënne-Loccoz, Y., Dubost, A., Gonçalves-Martins, M., Müller, D., and Prigent-Combaret, C. (2016). Fluorescent Pseudomonas strains with only few plant-beneficial properties are favored in the maize rhizosphere. Front. Plant Sci. 7:1212. doi: 10.3389/fpls.2016.01212
Wen, Z. H., Shen, J. B., Blackwell, M., Li, H. G., Zhao, B. Q., and Yuan, H. M. (2016). Combined applications of nitrogen and phosphorus fertilizers with manure increase maize yield and nutrient uptake via stimulating root growth in a long-term experiment. Pedosphere 26, 62–73. doi: 10.1016/S1002-0160(15)60023-6
White, P. J., and Hammond, J. P. (2008). “Phosphorus nutrition of terrestrial plants,” in The Ecophysiology Of Plant-Phosphorus Interactions, eds P. J. White and J. P. Hammond (Berlin: Springer), 51–81.
Zarei, T., Moradi, A., Kazemeini, S. A., Farajee, H., and Yadavi, A. (2019). Improving sweet corn (Zea mays L. var saccharata) growth and yield using Pseudomonas fluorescens inoculation under varied watering regimes. Agric. Water Manag. 226:105757. doi: 10.1016/j.agwat.2019.105757
Zeffa, D. M., Fantin, L. H., Santos, O. J. A. P., Oliveira, A. L. M., Canteri, M. G., Scapim, C. A., et al. (2018). The influence of topdressing nitrogen on Azospirillum spp. inoculation in maize crops through meta-analysis. Bragantia 77, 493–500. doi: 10.1590/1678-4499.2017273
Zeffa, D. M., Perini, L. J., Silva, M. B., de Sousa, N. V., Scapim, C. A., Oliveira, A. L. M., et al. (2019). Azospirillum brasilense promotes increases in growth and nitrogen use efficiency of maize genotypes. PLoS One 14:e0215332. doi: 10.1371/journal.pone.0215332
Zhang, Y., Thomas, C. L., Xiang, J., Long, Y., Wang, X., Zou, J., et al. (2016). QTL meta-analysis of root traits in Brassica napus under contrasting phosphorus supply in two growth systems. Sci. Rep. 6:33113. doi: 10.1038/srep33113
Keywords: Azospirillum brasilense, Bacillus subtilis, phosphorus fertilization management, Pseudomonas fluorescens, Zea mays L.
Citation: Pereira NCM, Galindo FS, Gazola RPD, Dupas E, Rosa PAL, Mortinho ES and Teixeira Filho MCM (2020) Corn Yield and Phosphorus Use Efficiency Response to Phosphorus Rates Associated With Plant Growth Promoting Bacteria. Front. Environ. Sci. 8:40. doi: 10.3389/fenvs.2020.00040
Received: 19 November 2019; Accepted: 19 March 2020;
Published: 07 April 2020.
Edited by:
Tim George, The James Hutton Institute, United KingdomReviewed by:
Alessandro Florio, Institut National de la Recherche Agronomique, FranceRoberta Fulthorpe, University of Toronto Scarborough, Canada
Copyright © 2020 Pereira, Galindo, Gazola, Dupas, Rosa, Mortinho and Teixeira Filho. This is an open-access article distributed under the terms of the Creative Commons Attribution License (CC BY). The use, distribution or reproduction in other forums is permitted, provided the original author(s) and the copyright owner(s) are credited and that the original publication in this journal is cited, in accordance with accepted academic practice. No use, distribution or reproduction is permitted which does not comply with these terms.
*Correspondence: Marcelo Carvalho Minhoto Teixeira Filho, mcm.teixeira-filho@unesp.br