- 1Department of Endocrinology and Metabolism, First Hospital of Jilin University, Changchun, China
- 2Department of Health Examination Center, First Hospital of Jilin University, Changchun, China
- 3Department of Clinical Nutrition, First Hospital of Jilin University, Changchun, China
- 4Center for Applied Statistical Research, School of Mathematics, Jilin University, Changchun, China
- 5Department of Clinical Medicine, Yanbian University, Yanji, China
- 6Division of Clinical Research, First Hospital of Jilin University, Changchun, China
Aim: This study aimed to examine the association of liver enzymes, including alanine aminotransferase (ALT), aspartate aminotransferase (AST), and gamma-glutamyl-transferase (GGT), with type 2 diabetes (T2D) risk, particularly their dose-response relationship.
Methods: This cross-sectional study enrolled participants aged >20 years old who underwent physical examination at our local hospital from November 2022 to May 2023. A generalized additive model (GAM) was fit to assess the dose-response relationship between liver enzymes and T2D risk. Furthermore, data from the UK Biobank (n=217,533) and National Health and Nutrition Examination Survey (NHANES 2011-2018; n= 15,528) were analyzed to evaluate whether the dose-response relationship between liver enzymes and T2D differed by population differences.
Results: A total of 14,100 participants were included (1,155 individuals with T2D and 12,945 individuals without diabetes) in the analysis. GAM revealed a non-linear relationship between liver enzymes and T2D risk (P non-linear < 0.001). Specifically, T2D risk increased with increasing ALT and GGT levels (range, <50 IU/L) and then plateaued when ALT and GGT levels were >50 IU/L. Elevated AST within a certain range (range, <35 IU/L) decreased the risk of T2D, whereas mildly elevated AST (>35 IU/L) became a risk factor for T2D. The UK Biobank and NHANES data analysis also showed a similar non-linear pattern between liver enzymes and T2D incidence.
Conclusion: Liver enzymes were non–linearly associated with T2D risk in different populations, including China, the UK, and the US. Elevated ALT and GGT levels, within a certain range, could increase T2D risk. More attention should be given to liver enzyme levels for early lifestyle intervention and early T2D prevention. Further studies are necessary to explore the mechanism of the non-linear association between liver enzymes and T2D risk.
Introduction
Diabetes is a common chronic metabolic disease characterized by sustained hyperglycemia with increasing prevalence worldwide. By 2045, 783 million adults worldwide are estimated to be living with diabetes (1), with type 2 diabetes (T2D) accounting for >90% (2). Long-term hyperglycemic status could increase the risk of developing life-threatening diabetic complications, such as cardiovascular and diabetic kidney diseases (3, 4). Therefore, identifying T2D risk factors is important for early intervention and delay of disease onset.
The liver plays an important role in controlling glucose homeostasis. The most common chronic liver disease is non-alcoholic fatty liver disease (NAFLD), which is characterized by excess fat accumulation in hepatocytes and is associated with T2D risk (5, 6), which might be reflected by increased liver enzymes. Circulating liver enzymes, such as alanine aminotransferase (ALT), gamma-glutamyl-transferase (GGT), and aspartate aminotransferase (AST), are non-invasive biomarkers of liver abnormalities. ALT is located predominantly in the cytoplasm and is the most specific marker of liver damage. Furthermore, ALT is associated with liver fat accumulation (7) and hepatic insulin resistance (8) and has been used as a surrogate marker for NAFLD in epidemiological studies (9, 10). GGT is present on the surface of most cell types and is widely used as an indicator of excessive alcohol intake and liver dysfunction. Moreover, recent studies have shown that GGT is related to components of metabolic syndrome, such as obesity (11), dyslipidemia (12), and hypertension (13). AST is a nonspecific liver enzyme involved in liver injury, and 80% of AST in the liver is present in hepatocyte mitochondria. Severe hepatocyte damage results in a significant increase in the serum AST levels (14).
Studies have investigated the association between liver enzymes and T2D risk; however, the results are controversial. Several studies revealed that ALT, AST, and GGT increase the risk of T2D (15, 16), whereas several studies showed that only ALT and GGT are risk factors for T2D (17–20). A study including 1441 individuals with a 7-year follow-up showed that AST alone is an independent risk factor for T2D after multivariable adjustment (21). Considering that China has the highest number of patients with T2D (1), only a few studies have investigated the association between liver enzymes and T2D risk in the Chinese population, particularly their potential dose-response relationship. Moreover, the distribution of liver enzymes may vary among different populations (22, 23), whether such differences translate to differences in the dose-response relationship between liver enzymes and T2D risk is still unknown.
In this study, we utilized large-scale physical examination data from China to investigate the association between liver enzymes (ALT, AST, and GGT) and T2D risk, particularly their dose-response relationship. Furthermore, the UK Biobank and National Health and Nutrition Examination Survey (NHANES) datasets were analyzed to determine whether the dose-response relationship differed by population differences and to validate the generalizability of the findings.
Materials and methods
Study participants
This was a cross-sectional study of 14,180 participants who underwent a routine physical examination at the Department of Health Examination Center of the First Hospital of Jilin University from November 2022 to May 2023. Participants aged >20 years were considered for inclusion in the study. Eighty individuals were excluded based on the following exclusion criteria: other types of diabetes, such as type 1 diabetes; excessive alcohol intake (>30 g/day for men and >20 g/day for women); liver injury with elevated liver enzymes 5-fold greater than the upper limit of normal (approximately ALT> 250 IU/L, AST>200 IU/L, GGT>300 IU/L); renal insufficiency with elevated serum creatinine 3-fold greater than the upper limit of normal (approximately >333 µmol/l); missing liver enzymes; missing fasting plasma glucose; self-reported liver cirrhosis and heart failure; pregnancy; cancer; and heart attack or acute infection in the last 3 months. A total of 14,100 participants (1,155 individuals with T2D and 12,945 individuals without diabetes) were included in the study.
Data collection and measurements
Demographic and questionnaire data, including sex, age, smoking, alcoholic consumption, and medical history (hypertension and diabetes), were collected through an interview. Based on the smoking status, the participants were dichotomized as current and non-current smokers. Information on alcohol consumption was self-reported, and alcohol intake (g/day) was estimated based on the frequency and amount. The status of alcohol intake was divided into mild to moderate drinkers (<30 g/day for men and <20 g/day for women) and non-drinkers. Moreover, all subjects underwent anthropometric measurements, including height, weight, and blood pressure. body mass index (BMI) was calculated as weight (kg) divided by height squared (m2). After overnight fasting of 8–12 h, fasting plasma glucose (FPG), glycosylated hemoglobin (HbA1c), liver enzymes (ALT, AST, and GGT), serum lipid profile [total cholesterol (TC), triglyceride (TG), high-density lipoprotein cholesterol (HDL-C), and low-density lipoprotein cholesterol (LDL-C)] were assayed at the department of laboratory medicine. Furthermore, the presence of fatty liver was determined by abdominal ultrasound examination performed by professional experienced sonographers.
Hypertension was defined as a systolic blood pressure (SBP) ≥140 mmHg, diastolic blood pressure (DBP) ≥90mmHg or past medical history of hypertension. T2D was defined as self-reported T2D, FPG ≥7.0 mmol/l, or HbA1c ≥6.5%.
Analysis of the UK Biobank and NHANES dataset
In addition to the Chinese dataset, we also analyzed the UK Biobank (application number 84347) from the UK population and the NHANES from the US population to reassess the dose-response relationship between liver enzymes and T2D risk. The UK Biobank is the world’s largest biomedical database. Between 2006 and 2010, >500,000 individuals aged 37-73 years were recruited into the cohort at baseline. Our study is a cross-sectional analysis of data from the UK Biobank, and the preliminary data extraction included 502,411 participants after application approval. The NHANES is a nationally representative cross-sectional survey of the noninstitutionalized US population to assess the health and nutritional status of children and adults. We conducted a cross-sectional analysis of data from NHANES 2011-2018 including 39,156 participants. Figure 1 shows the flowcharts of the study population selection process for the UK Biobank and NHANES. We finally included 217,533 and 15,528 participants for UK Biobank and NHANES, respectively. Supplementary File 1 provided details of the data collection, measurement, covariable, and T2D determination of the UN Biobank and NHANES.
Statistical analysis
Continuous variables with normal distribution were expressed as mean ± standard deviation. Student’s t-test and analysis of variance were used for comparison between groups. Continuous variables with non-normal distribution were expressed as median (interquartile range). Mann–Whitney U–test or Kruskal–Wallis H-test was used for comparison between groups. Categorial variables were described as frequencies and percentages, and chi-square tests are used for the analysis of differences in distributions. The relationship between liver enzymes and T2D risk was examined using multivariable logistic regression after adjusting for potential confounding factors. Model 1 was adjusted for sex, age, BMI, hypertension, smoking, and drinking; model 2 was additionally adjusted for TC, TG, and the other two liver enzymes; model 3 was the same as model 2 with additional adjustment for NAFLD. Furthermore, generalized additive models (GAMs) were fit to evaluate the dose-response association between continuous liver enzyme levels and T2D risk in the Chinese dataset, UK Biobank, and NHANES dataset. P < 0.05 was considered statistically significant. Statistical analyses were performed using SPSS (version 22.0; IBM Corporation, Armonk, NY, USA), and GAMs were carried out in R software 4.1.0 (https://www.r-project.org/) using the R package “mgcv”.
Results
We included 14,100 subjects (45.22% men) with a mean age of 45.43 ± 12.71 years who underwent conventional physical examination, including 1155 patients with T2D. All the data were complete without missing values except for HbA1c (3017 individuals with measured HbA1c). Table 1 shows the baseline characteristics of the study population stratified by blood glucose. Compared with the non-diabetes control, the T2D group presented higher age, BMI, blood pressure, and worse serum lipid profile (higher TC, TG and LDL-C and lower HDL-C). The ALT, AST, and GGT levels in the T2D group were significantly higher than those in the non-diabetes group (P < 0.001).
Tables 2–4 list the baseline characteristics of the participants stratified by ALT, AST, and GGT quartiles. With the increase in quartiles of liver enzymes ALT, AST, and GGT, metabolic indicators, such as BMI, SBP, DBP, TC, TG, and LDL-C, increased gradually. Notably, FPG also increased progressively with the increase of quartiles of liver enzymes, which was statistically significant after inter-group comparison, indicating a positive association between liver enzymes and glucose metabolism.
Table 5 shows the odd ratios (OR) and corresponding 95% confidence intervals (CI) of T2D by quartiles of liver enzymes. In the crude model, elevated ALT, AST, and GGT levels increased T2D risk (top versus bottom quartile ALT: OR 3.89 [95% CI 3.17-4.78], AST: 1.76 [1.49-2.08], GGT 6.98 [5.52-8.82]). In model 1, with adjustment of age, sex, BMI, hypertension, smoking, and drinking, ALT and GGT were still risk factors of T2D, although the effect was attenuated. However, elevated AST levels were a protective factor of T2D. The association was evident after additionally adjusting for serum lipid (TC and TG), and the other two liver enzymes in model 2. With further additional adjustment for NAFLD in model 3, the results indicated that ALT and GGT levels in quartile 4 had an approximately 1.58-fold and 2.27-fold higher risk of developing T2D compared with ALT and GGT in the lowest quartile, respectively. The AST levels in quartile 4 could significantly decrease the risk of T2D (OR: 0.39; 95% CI:0.31-0.49).
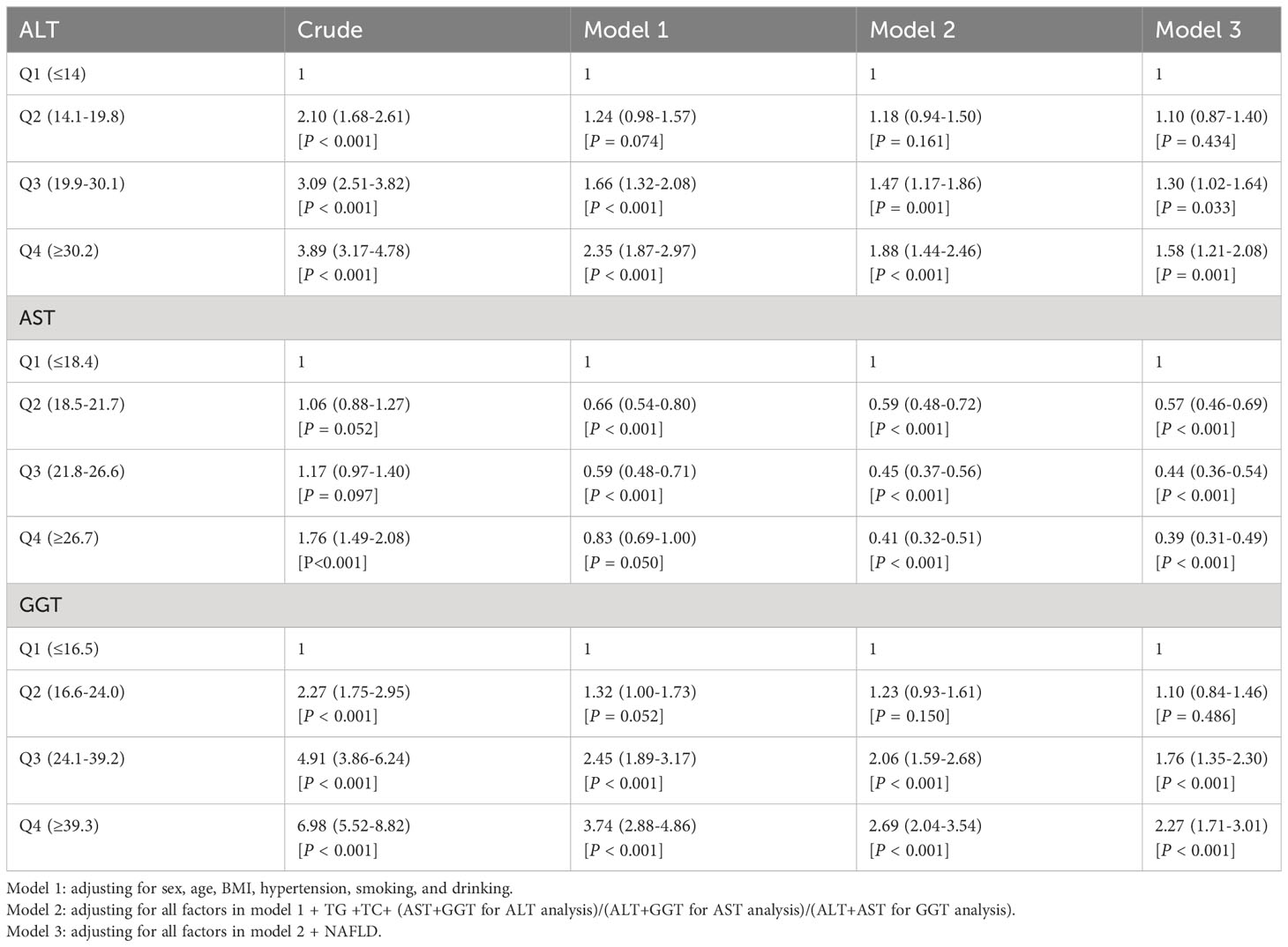
Table 5 Multivariable logistic regression models assessing the relationship between liver enzymes and T2D.
Figure 2 shows the results of the dose-response relationship between continuous liver enzyme levels and T2D risk. Overall, a non-linear association was identified between ALT, AST, and GGT and T2D risk (P non-linear < 0.001) after adjustment for sex, age, BMI, hypertension, smoking, alcohol consumption, TC, TG, the other two liver enzymes, and NAFLD. Specifically, ALT and GGT <50 IU/L could increase the T2D risk, which plateaued when ALT and GGT levels were >50 IU/L. Elevated AST levels <35 IU/L played a protective role in T2D development, whereas mildly elevated AST at >35 IU/L could also be a risk factor of T2D.
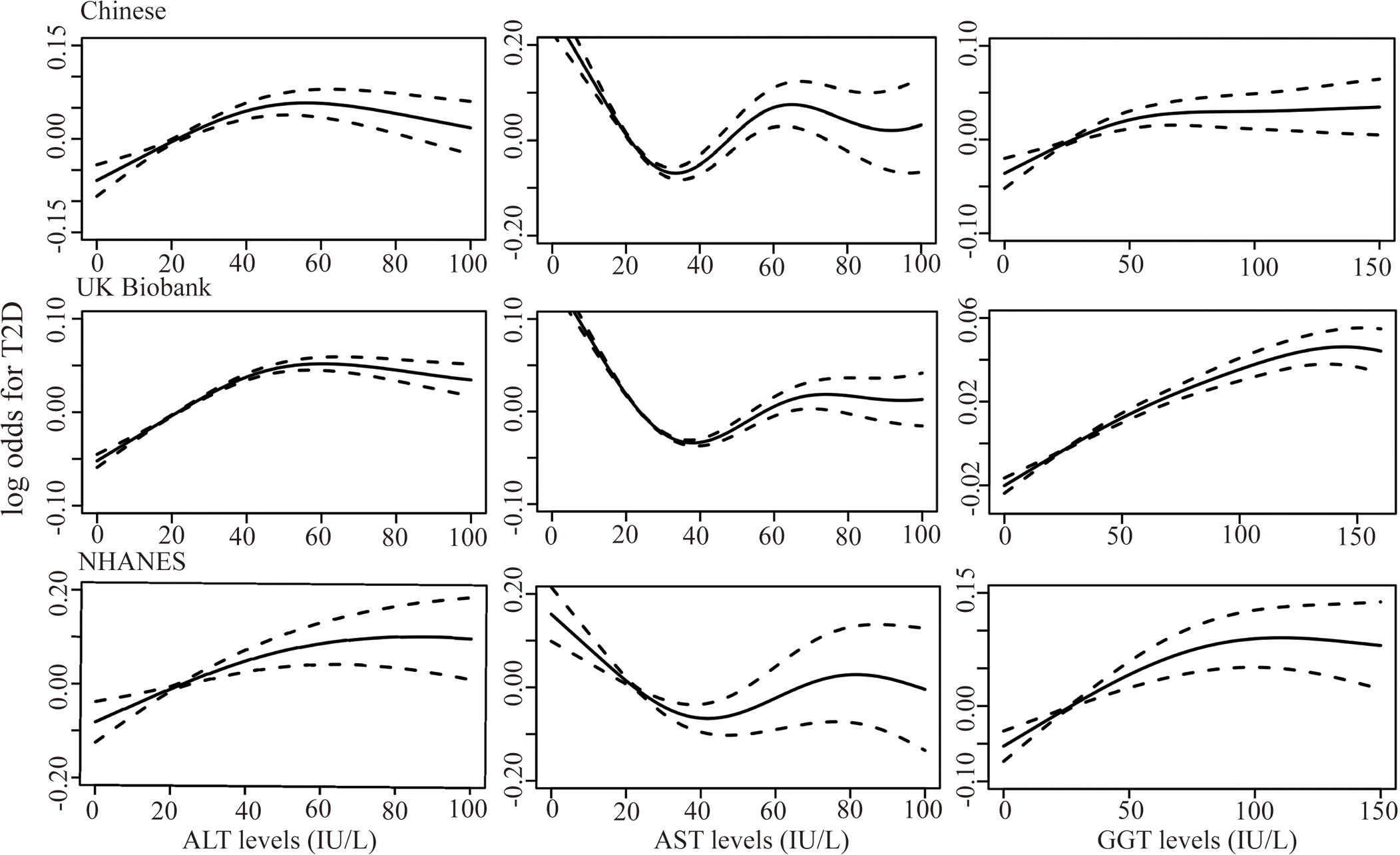
Figure 2 Generalized additive models were fit to assess the non-linear relationship between liver enzymes, ALT, AST, and GGT, and risk of T2D in the Chinese dataset (n=14100), UK Biobank (n=217533), and the NHANES dataset (n=15528) (all P < 0.001). X-axis represented the levels of liver enzymes and the y-axes represented the log odds for T2D.
The dose-response relationship between liver enzymes and T2D risk in the UK and US populations was also analyzed. We included 217,533 and 15,528 participants from the UK Biobank and NHANES in the analysis, including 11,157 (5.13%) and 2426 (15.62%) patients with T2D, respectively. We identified a similar non-linear pattern between liver enzymes and T2D risk in UK and US populations (P non-linear < 0.001, Figure 2). The ALT and AST levels, corresponding to the peak and bottom risk of T2D, were 50 and 35 IU/L, respectively, which was consistent with the results from the Chinese dataset. However, in the UK Biobank, elevated GGT levels within 125 IU/L could increase the T2D risk, then plateaus thereafter, which was higher than that in the Chinese and NHANES datasets. In the GAMs, potential confounding factors, including sex, age, BMI, hypertension, physical activity (International Physical Activity Questionnaire for UK Biobank and categorical physical activity, including vigorous, moderate physical activity and inactivity for NHANES), family history of diabetes, smoking, drinking, TG, TC, other two liver enzymes, and NAFLD were adjusted.
Discussion
We investigated the association of liver enzymes with T2D risk. We identified a non-linear relationship of ALT, AST, and GGT with T2D after controlling for potential confounding factors. T2D risk was continuously increasing with increasing ALT and GGT levels within a certain range. However, T2D risk plateaued when ALT and GGT increased to a certain degree. Elevated AST levels within a certain range initially exhibited a protective role, and mildly elevated AST levels were a risk factor of T2D. Additionally, the UK Biobank and NHANES dataset verified the non-linear association between liver enzymes and T2D risk, indicating a credible result for the Chinese dataset. Moreover, the non-linear association was scarcely affected by population differences.
Our findings were generally consistent with previous studies in South Korea (18, 19), Japan (24), Iran (25), and Germany (26), and we expanded previous reports by assessing the dose-response association of ALT and GGT with T2D risk. Elevated ALT and GGT levels within 50 IU/L could increase T2D risk, and T2D risk plateaued (“saturation effect”) when ALT and GGT levels were > 50 IU/L for Chinese populations. Although the genetic background and distribution of liver enzymes were different in different populations, a similar non-linear pattern was identified in the UK and US populations. However, the cutoff value of GGT in the UK Biobank was higher than that in the Chinese and NHANES dataset. Our findings were consistent with one meta-analysis conducted only on GGT and T2D risk, which also showed a non-linear association (27).
The findings have clinical significance as follows. First, the cutoff values of ALT and GGT at 50 IU/L could be considered the upper limit of the normal reference range in the general population, suggesting that increased ALT and GGT levels within their normal ranges are risk factors of T2D. One individual had elevated ALT and GGT levels (<50 IU/L) as compared to previous ones, indicating increased risk of T2D even though the glucose metabolism indicators such as FPG were within normal range. Therefore, early lifestyle intervention is needed for early T2D prevention. Second, measurements of liver enzymes are sensitive, standardized, and economical in clinical practice. ALT and GGT may clinically serve as useful markers in identifying individuals with higher T2D risk.
The association between AST and T2D risk is uncertain (17, 21, 25, 28, 29). Our study used continuous AST values and identified a non-linear association between AST and T2D risk, which was also verified in two other large-scale datasets. One study, including 9895 subjects, showed that elevated AST levels were a risk factor of diabetes (elevated versus normal AST; OR:1.75 [1.32-2.32]), whereas AST within the normal ranges of quartiles 2, 3, and 4 was negatively associated with T2D risk compared with quartile 1 (Q2, Q3, Q4 versus Q1, OR<1, 95% CI <1) (25), indicating that the association between AST and diabetes risk may be a non-linear trend of initially decreasing followed by an increasing trend. The non-linear association between continuous AST levels and T2D risk was revealed for the first time, and more studies are necessary to help provide the mechanisms for the non-linear association.
Although the mechanism underlying the positive association of ALT and GGT with T2D risk is unclear, it could be explained by ALT and GGT both reflecting fat accumulation in the liver (7, 30), resulting in hepatic insulin resistance, causing increased hepatic glucose production. These pathophysiological changes are involved in the pathogenic mechanism of T2D (31). In our study, we adjusted fatty liver in the GAMs, and the results still showed a positive association of ALT and GGT with T2D. Additionally, a study including 3556 individuals without ultrasound-diagnosed fatty liver also indicated that increased ALT and GGT levels are risk factors for T2D (32). This suggests that ALT and GGT are independent risk factors of T2D independent of fatty liver, and extra mechanisms might exist accounting for the positive association. GGT plays an essential role in maintaining the production of intracellular antioxidant glutathione, also considered a biomarker of oxidative stress (33). Furthermore, elevated ALT and GGT levels are associated with chronic inflammation (34, 35). Oxidative stress, together with inflammation, could damage the insulin signal pathway, which contributes to the development of T2D (36–38).
Furthermore, we hypothesize the following conjecture to account for the non-linear association between ALT and GGT and T2D risk. The regulation of blood glucose homeostasis is involved in multiple organs, especially the liver (39). It may cause minor liver damage when the liver tries to maintain glucose homeostasis, which clinically manifests as elevated ALT and GGT levels, even within their roughly normal ranges. Thus, elevated ALT and GGT levels within a certain range may be the consequences of self-regulation by the liver to maintain glucose homeostasis. However, when abnormal glucose homeostasis develops to a certain extent wherein the liver is unable to maintain glucose homeostasis at the cost of damaging itself (elevated ALT and GGT within a certain range), the association of ALT and GGT with T2D risk disappears. Further investigation, however, is required to test this research hypothesis.
The strengths of the present study are twofold. First, extensive large-scale data from up to 14,100 individuals were collected, and the dose-response relationship between liver enzymes and T2D in the Chinese population was systematically explored, which was rarely studied and poorly understood. Second, the analysis and comparison of three large-scale datasets comprehensively offered a significant advantage. Thus, it increased the credibility and generalizability of the findings, and the strategy could detect the possible population differences of the findings.
This study has several disadvantages. First, the participants in the Chinese dataset did not undergo virologic testing; therefore, we cannot exclude individuals with positive viral hepatitis, which may be a confounder of this association. Second, the history of alcohol consumption was self-reported, and information bias may be present. Third, we could not fully adjust the potential confounding factors or exclude residual confounding factors. For example, although ultrasound has been suggested as a first-line screening tool for defining liver steatosis in the general population (40), it is less sensitive when liver fat is <30% (41); therefore, a small part of an individual with mild fatty liver may be neglected. Fourth, we could not comprehensively explore the association of liver enzymes with the onset and development of T2D, as the course of self-reported T2D was not recorded. Finally, the participants were from the northeast of China, which could not represent the characteristics of the overall Chinese population.
In conclusion, liver enzymes ALT, AST, and GGT presented a non-linear pattern with T2D risk after adjustment for comprehensive diabetes risk factors, which was generalizable to different populations from China, the UK, and the US. Higher ALT and GGT levels, within a certain range, were indicative of the development of T2D. More attention should be given to liver enzymes levels for early lifestyle intervention and early T2D prevention. Meanwhile, more studies are warranted to explore the underlying mechanisms for the non-linear association between liver enzymes and T2D risk.
Data availability statement
The original contributions presented in the study are included in the article/Supplementary Material. Further inquiries can be directed to the corresponding authors.
Ethics statement
The studies involving humans were approved by Ethics Committee of the First Hospital of Jilin University. The studies were conducted in accordance with the local legislation and institutional requirements. The participants provided their written informed consent to participate in this study.
Author contributions
YB: Data curation, Investigation, Methodology, Writing – original draft. YY: Data curation, Formal analysis, Writing – original draft. XY: Data curation, Methodology, Resources, Writing – original draft. JW: Data curation, Methodology, Software, Writing – original draft. TW: Data curation, Methodology, Software, Writing – original draft. ZL: Methodology, Writing – original draft. ST: Conceptualization, Visualization, Writing – review & editing. CS: Conceptualization, Visualization, Writing – review & editing.
Funding
The author(s) declare financial support was received for the research, authorship, and/or publication of this article. This work was supported by the Science Technology Department of Jilin Province (20230505002ZP, YDZJ202201ZYTS121) and Health Commission of Jilin Province (2023LC008).
Acknowledgments
The authors thank the participants in the study.
Conflict of interest
The authors declare that the research was conducted in the absence of any commercial or financial relationships that could be construed as a potential conflict of interest.
Publisher’s note
All claims expressed in this article are solely those of the authors and do not necessarily represent those of their affiliated organizations, or those of the publisher, the editors and the reviewers. Any product that may be evaluated in this article, or claim that may be made by its manufacturer, is not guaranteed or endorsed by the publisher.
Supplementary material
The Supplementary Material for this article can be found online at: https://www.frontiersin.org/articles/10.3389/fendo.2024.1340604/full#supplementary-material
References
1. Sun H, Saeedi P, Karuranga S, Pinkepank M, Ogurtsova K, Duncan BB, et al. Idf diabetes atlas: global, regional and country-level diabetes prevalence estimates for 2021 and projections for 2045. Diabetes Res Clin Pract. (2022) 183:109119. doi: 10.1016/j.diabres.2021.109119
2. Zimmet P, Alberti KG, Shaw J. Global and societal implications of the diabetes epidemic. Nature. (2001) 414(6865):782–7. doi: 10.1038/414782a
3. Laakso M. Hyperglycemia and cardiovascular disease in type 2 diabetes. Diabetes. (1999) 48(5):937–42. doi: 10.2337/diabetes.48.5.937
4. Alicic RZ, Rooney MT, Tuttle KR. Diabetic kidney disease: challenges, progress, and possibilities. Clin J Am Soc Nephrol: CJASN. (2017) 12(12):2032–45. doi: 10.2215/cjn.11491116
5. Brunt EM, Wong VW, Nobili V, Day CP, Sookoian S, Maher JJ, et al. Nonalcoholic fatty liver disease. Nat Rev Dis Primers. (2015) 1:15080. doi: 10.1038/nrdp.2015.80
6. Tanase DM, Gosav EM, Costea CF, Ciocoiu M, Lacatusu CM, Maranduca MA, et al. The intricate relationship between type 2 diabetes mellitus (T2dm), insulin resistance (Ir), and nonalcoholic fatty liver disease (Nafld). J Diabetes Res. (2020) 2020:3920196. doi: 10.1155/2020/3920196
7. Westerbacka J, Cornér A, Tiikkainen M, Tamminen M, Vehkavaara S, Häkkinen AM, et al. Women and men have similar amounts of liver and intra-abdominal fat, despite more subcutaneous fat in women: implications for sex differences in markers of cardiovascular risk. Diabetologia. (2004) 47(8):1360–9. doi: 10.1007/s00125-004-1460-1
8. Vozarova B, Stefan N, Lindsay RS, Saremi A, Pratley RE, Bogardus C, et al. High alanine aminotransferase is associated with decreased hepatic insulin sensitivity and predicts the development of type 2 diabetes. Diabetes. (2002) 51(6):1889–95. doi: 10.2337/diabetes.51.6.1889
9. Yoo J, Lee S, Kim K, Yoo S, Sung E, Yim J. Relationship between insulin resistance and serum alanine aminotransferase as a surrogate of nafld (Nonalcoholic fatty liver disease) in obese Korean children. Diabetes Res Clin Pract. (2008) 81(3):321–6. doi: 10.1016/j.diabres.2008.05.006
10. Yun KE, Shin CY, Yoon YS, Park HS. Elevated alanine aminotransferase levels predict mortality from cardiovascular disease and diabetes in Koreans. Atherosclerosis. (2009) 205(2):533–7. doi: 10.1016/j.atherosclerosis.2008.12.012
11. Ali N, Sumon AH, Fariha KA, Asaduzzaman M, Kathak RR, Molla NH, et al. Assessment of the relationship of serum liver enzymes activity with general and abdominal obesity in an urban Bangladeshi population. Sci Rep. (2021) 11(1):6640. doi: 10.1038/s41598-021-86216-z
12. Kathak RR, Sumon AH, Molla NH, Hasan M, Miah R, Tuba HR, et al. The association between elevated lipid profile and liver enzymes: A study on Bangladeshi adults. Sci Rep. (2022) 12(1):1711. doi: 10.1038/s41598-022-05766-y
13. Liu CF, Gu YT, Wang HY, Fang NY. Gamma-glutamyltransferase level and risk of hypertension: A systematic review and meta-analysis. PloS One. (2012) 7(11):e48878. doi: 10.1371/journal.pone.0048878
14. Woreta TA, Alqahtani SA. Evaluation of abnormal liver tests. Med Clinics North America. (2014) 98(1):1–16. doi: 10.1016/j.mcna.2013.09.005
15. Chen SC, Tsai SP, Jhao JY, Jiang WK, Tsao CK, Chang LY. Liver fat, hepatic enzymes, alkaline phosphatase and the risk of incident type 2 diabetes: A prospective study of 132,377 adults. Sci Rep. (2017) 7(1):4649. doi: 10.1038/s41598-017-04631-7
16. Zhang J, Cheng N, Ma Y, Li H, Cheng Z, Yang Y, et al. Liver enzymes, fatty liver and type 2 diabetes mellitus in a Jinchang cohort: A prospective study in adults. Can J Diabetes. (2018) 42(6):652–8. doi: 10.1016/j.jcjd.2018.02.002
17. Wannamethee SG, Shaper AG, Lennon L, Whincup PH. Hepatic enzymes, the metabolic syndrome, and the risk of type 2 diabetes in older men. Diabetes Care. (2005) 28(12):2913–8. doi: 10.2337/diacare.28.12.2913
18. Ko SH, Baeg MK, Han KD, Ko SH, Ahn YB. Increased liver markers are associated with higher risk of type 2 diabetes. World J Gastroenterol. (2015) 21(24):7478–87. doi: 10.3748/wjg.v21.i24.7478
19. Ahn HR, Shin MH, Nam HS, Park KS, Lee YH, Jeong SK, et al. The association between liver enzymes and risk of type 2 diabetes: the Namwon study. Diabetol Metab Syndrome. (2014) 6(1):14. doi: 10.1186/1758-5996-6-14
20. Sato KK, Hayashi T, Nakamura Y, Harita N, Yoneda T, Endo G, et al. Liver enzymes compared with alcohol consumption in predicting the risk of type 2 diabetes: the Kansai healthcare study. Diabetes Care. (2008) 31(6):1230–6. doi: 10.2337/dc07-2184
21. Nannipieri M, Gonzales C, Baldi S, Posadas R, Williams K, Haffner SM, et al. Liver enzymes, the metabolic syndrome, and incident diabetes: the Mexico City diabetes study. Diabetes Care. (2005) 28(7):1757–62. doi: 10.2337/diacare.28.7.1757
22. Browning JD, Szczepaniak LS, Dobbins R, Nuremberg P, Horton JD, Cohen JC, et al. Prevalence of hepatic steatosis in an urban population in the United States: impact of ethnicity. Hepatol (Baltimore Md). (2004) 40(6):1387–95. doi: 10.1002/hep.20466
23. Whitfield JB. Gamma glutamyl transferase. Crit Rev Clin Lab Sci. (2001) 38(4):263–355. doi: 10.1080/20014091084227
24. Doi Y, Kubo M, Yonemoto K, Ninomiya T, Iwase M, Tanizaki Y, et al. Liver enzymes as a predictor for incident diabetes in a Japanese population: the Hisayama study. Obes (Silver Spring Md). (2007) 15(7):1841–50. doi: 10.1038/oby.2007.218
25. Noroozi Karimabad M, Khalili P, Ayoobi F, Esmaeili-Nadimi A, La Vecchia C, Jamali Z. Serum liver enzymes and diabetes from the Rafsanjan cohort study. BMC Endocr Disord. (2022) 22(1):127. doi: 10.1186/s12902-022-01042-2
26. Ford ES, Schulze MB, Bergmann MM, Thamer C, Joost HG, Boeing H. Liver enzymes and incident diabetes: findings from the European prospective investigation into cancer and nutrition (Epic)-potsdam study. Diabetes Care. (2008) 31(6):1138–43. doi: 10.2337/dc07-2159
27. Kunutsor SK, Abbasi A, Adler AI. Gamma-glutamyl transferase and risk of type ii diabetes: an updated systematic review and dose-response meta-analysis. Ann Epidemiol. (2014) 24(11):809–16. doi: 10.1016/j.annepidem.2014.09.001
28. Cho NH, Jang HC, Choi SH, Kim HR, Lee HK, Chan JC, et al. Abnormal liver function test predicts type 2 diabetes: A community-based prospective study. Diabetes Care. (2007) 30(10):2566–8. doi: 10.2337/dc07-0106
29. Kunutsor SK, Abbasi A, Apekey TA. Aspartate aminotransferase - risk marker for type-2 diabetes mellitus or red herring? Front Endocrinol. (2014) 5:189. doi: 10.3389/fendo.2014.00189
30. Perry IJ, Wannamethee SG, Shaper AG. Prospective study of serum gamma-glutamyltransferase and risk of niddm. Diabetes Care. (1998) 21(5):732–7. doi: 10.2337/diacare.21.5.732
31. Birkenfeld AL, Shulman GI. Nonalcoholic fatty liver disease, hepatic insulin resistance, and type 2 diabetes. Hepatol (Baltimore Md). (2014) 59(2):713–23. doi: 10.1002/hep.26672
32. Kim CH, Park JY, Lee KU, Kim JH, Kim HK. Association of serum gamma-glutamyltransferase and alanine aminotransferase activities with risk of type 2 diabetes mellitus independent of fatty liver. Diabetes/Metabolism Res Rev. (2009) 25(1):64–9. doi: 10.1002/dmrr.890
33. Lee DH, Blomhoff R, Jacobs DR Jr. Is serum gamma glutamyltransferase a marker of oxidative stress? Free Radical Res. (2004) 38(6):535–9. doi: 10.1080/10715760410001694026
34. Ali SS, Oni ET, Blaha MJ, Veledar E, Feiz HR, Feldman T, et al. Elevated gamma-glutamyl transferase is associated with subclinical inflammation independent of cardiometabolic risk factors in an asymptomatic population: A cross-sectional study. Nutr Metab. (2016) 13:37. doi: 10.1186/s12986-016-0097-7
35. Yamada J, Tomiyama H, Yambe M, Koji Y, Motobe K, Shiina K, et al. Elevated serum levels of alanine aminotransferase and gamma glutamyltransferase are markers of inflammation and oxidative stress independent of the metabolic syndrome. Atherosclerosis. (2006) 189(1):198–205. doi: 10.1016/j.atherosclerosis.2005.11.036
36. Lima J, Moreira NCS, Sakamoto-Hojo ET. Mechanisms underlying the pathophysiology of type 2 diabetes: from risk factors to oxidative stress, metabolic dysfunction, and hyperglycemia. Mutat Res Genet Toxicol Environ Mutagenesis. (2022) 874-875:503437. doi: 10.1016/j.mrgentox.2021.503437
37. Lontchi-Yimagou E, Sobngwi E, Matsha TE, Kengne AP. Diabetes mellitus and inflammation. Curr Diabetes Rep. (2013) 13(3):435–44. doi: 10.1007/s11892-013-0375-y
38. Yaribeygi H, Sathyapalan T, Atkin SL, Sahebkar A. Molecular mechanisms linking oxidative stress and diabetes mellitus. Oxid Med Cell Longevity. (2020) 2020:8609213. doi: 10.1155/2020/8609213
39. Han HS, Kang G, Kim JS, Choi BH, Koo SH. Regulation of glucose metabolism from a liver-centric perspective. Exp Mol Med. (2016) 48(3):e218. doi: 10.1038/emm.2015.122
40. Papatheodoridi M, Cholongitas E. Diagnosis of non-alcoholic fatty liver disease (Nafld): current concepts. Curr Pharm Design. (2018) 24(38):4574–86. doi: 10.2174/1381612825666190117102111
Keywords: alanine aminotransferase, aspartate aminotransferase, gamma-glutamyl transferase, type 2 diabetes, National Health and Nutrition Examination Survey, UK Biobank
Citation: Bi Y, Yang Y, Yuan X, Wang J, Wang T, Liu Z, Tian S and Sun C (2024) Association between liver enzymes and type 2 diabetes: a real-world study. Front. Endocrinol. 15:1340604. doi: 10.3389/fendo.2024.1340604
Received: 18 November 2023; Accepted: 29 January 2024;
Published: 20 February 2024.
Edited by:
Mark M. Smits, University of Copenhagen, DenmarkReviewed by:
Ershad Mondal, Mymensingh Medical College, BangladeshMarcin Kosmalski, Medical University of Lodz, Poland
Copyright © 2024 Bi, Yang, Yuan, Wang, Wang, Liu, Tian and Sun. This is an open-access article distributed under the terms of the Creative Commons Attribution License (CC BY). The use, distribution or reproduction in other forums is permitted, provided the original author(s) and the copyright owner(s) are credited and that the original publication in this journal is cited, in accordance with accepted academic practice. No use, distribution or reproduction is permitted which does not comply with these terms.
*Correspondence: Suyan Tian, wmxt@jlu.edu.cn; Chenglin Sun, chenglins@jlu.edu.cn
†These authors have contributed equally to this work and share first authorship