Research progress of non-coding RNA in atrial fibrillation
- Department of Cardiology, Aoyang Hospital Affiliated to Jiangsu University, Zhangjiagang, China
Atrial fibrillation (AF) is a common arrhythmia in clinic, and its incidence is increasing year by year. In today's increasingly prevalent society, ageing poses a huge challenge to global healthcare systems. AF not only affects patients' quality of life, but also causes thrombosis, heart failure and other complications in severe cases. Although there are some measures for the diagnosis and treatment of AF, specific serum markers and targeted therapy are still lacking. In recent years, ncRNAs have become a hot topic in cardiovascular disease research. These ncRNAs are not only involved in the occurrence and development of AF, but also in pathophysiological processes such as myocardial infarction and atherosclerosis, and are potential biomarkers of cardiovascular diseases. We believe that the understanding of the pathophysiological mechanism of AF and the study of diagnosis and treatment targets can form a more systematic diagnosis and treatment framework of AF and provide convenience for individuals with AF and the society.
1. Introduction
Atrial fibrillation (AF) is a common arrhythmias in clinic, with a high risk of death, stroke, and peripheral embolism, and its incidence has been increasing year by year. Risk factors for AF are closely related to cardiovascular disease, with organic or functional heart problems being more common. In addition, age, gender and genetic factors are also important factors leading to the occurrence of AF (1, 2). AF not only affects life quality of the patients, but also has complications such as thrombosis and heart failure in severe cases. Atrial remodeling is considered to be the basis of the occurrence and development of AF, including structural remodeling, electrical remodeling, neural remodeling, etc (3–5). The diagnosis of AF mainly depends on electrocardiogram findings, which are often found after complications occur, and there is a certain lag (6). Therefore, biomarkers have potential value in the early diagnosis of AF. Currently, drug therapy for AF patients has poor efficacy and side effects. Radiofrequency ablation is more effective than drug therapy, but the patients are yet able to avoid the operational risks, postoperative recurrence, and high healthcare cost (7–9). Actively searching for new diagnosis and treatment strategies and exploring the molecular mechanism of AF have great clinical significance and translational prospects.
In recent years, non-coding RNA (ncRNA) has become a research hotspot in cardiovascular diseases. ncRNA mainly includes miRNA, LncRNA and CircRNA. These ncRNAs can not only participate in the occurrence and development of AF, but also play a part in the pathophysiological processes such as myocardial infarction and atherosclerosis, which are potential biomarkers for cardiovascular diseases (10). This article reviews the pathophysiological mechanism of AF, introduces the mechanism and potential value of ncRNAs in AF, and provides a theoretical basis for the diagnosis, treatment and prognosis monitoring of AF (Figure 1, Tables 1, 2).
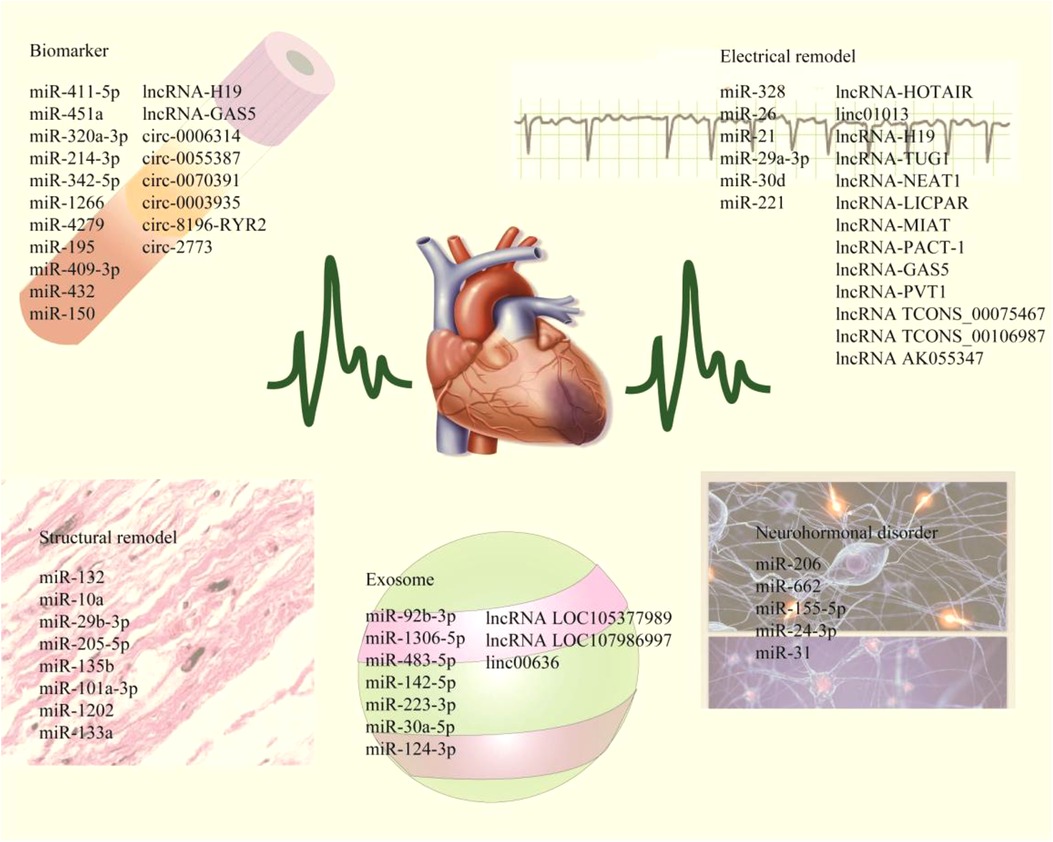
Figure 1. The schematic highlights ncRNAs associated with myocardial electrical remodeling, fibrosis, neurohormone disorders, and exosomes, while including plasma markers associated with AF diagnosis and prognotic monitoring.
2. Pathophysiology of AF
AF can be classified as paroxysmal, persistent and permanent according to the duration of the attack. The pathogenesis of AF involves a variety of factors, mainly including electrical remodeling, structural remodeling, and neurohormonal disorders. These mechanisms lead to the development and maintenance of AF (83, 84). Although the pathogenesis of AF is complex, it is mainly related to electrical remodeling and structural remodeling. Existing studies suggest that ncRNAs play an important role in its occurrence and development (Table 1) (85–87).
3. miRNAs involved in the diagnosis and prognostic monitoring of AF
The early symptoms of AF are not obvious, the main clinical manifestations are palpitation, dyspnea and dizziness, which are easy to be ignored by patients, and routine electrocardiogram is difficult to monitor, so the diagnosis is often missed (88, 89). At present, BNP and troponin are the main clinical biomarkers for the diagnosis of cardiovascular diseases, but they are mainly used for the diagnosis of heart failure and myocardial infarction, and have no significant significance for the diagnosis of AF. In recent years, the research on ncRNA has become increasingly in-depth. The differential expression of ncRNAs in cardiac tissue and blood of patients with AF may become auxiliary diagnostic biomarkers for AF (Table 2) (90, 91).
Risk stratification of subsequent cardiovascular events in patients with AF helps guide prevention strategies. Nossent AY et al. analyzed differentially expressed miRNAs in 26 patients using sequencing technology, and screened out one miR-411-5p in combination with clinical prognosis as a potential valuable prognostic biomarker for patients with AF (69). Recurrent AF after catheter ablation seriously affected the prognosis of patients. Therefore, Garcia-Seara J et al. recruited 42 patients with AF for catheter ablation. The analysis measured the expression of 84 miRNAs in both non-relapsed and relapsed groups, the results showed that miRNA-451a was down-regulated in relapsed patients, and the recurrence of AF was positively correlated with an increased percentage of scars. It is suggested that low expression of miR-451a may play an important role in the recurrence of AF by controlling fibrosis and progression (70). Akselrod AS et al. found that plasma miR-320a-3p level in patients with AF was higher than that in healthy controls, and the expression level was positively correlated with CHADS-VASc score (28). Sasano T et al. identified 11 candidate miRNAs using high-throughput sequencing and clinical sample validation, and found that miR-214-3p and miR-342-5p had high accuracy in the diagnosis of patients with AF combined with clinicpathological parameter analysis (71). Yang et al. observed genome-wide differential expression profiles of miRNAs in 180 peripheral blood samples and found 14 miRNAs with significant differential expression, among which miR-1266, miR-4279 and miR-4666a-3p were significantly increased in expression, which are potential targets for future diagnosis and treatment of AF (72). About one-third of patients undergoing coronary artery bypass grafting will develop postoperative AF, which seriously affects the prognosis of patients. In order to monitor the occurrence of postoperative AF, Athanasiou et al. prospectively recruited 34 patients after surgery, and compared the myocardial tissue with normal sinus rhythm after surgery, and found 16 differentially expressed miRNAs. The expression of miR-208a was significantly decreased, and the expression of miR-483-5p was significantly increased. It is suggested that these differentially expressed miRNAs can be used to predict the recurrence of AF after coronary artery bypass grafting (73). Kilic et al. recruited 63 patients after coronary artery bypass grafting and monitored their heart rate until discharge. Among them, 20 patients developed postoperative AF, and PCR detected the expression of miR-199a and miR-195. The results showed that the expression of miR-199a significantly decreased in the postoperative AF group, demonstrate its effectiveness as a biomarker for cardiac surgery management (74). By Solexa sequencing 100 patients with AF who underwent catheter ablation and 100 healthy individuals, Wu et al. found that miR-409-3p and miR-432 were significantly reduced in the plasma of patients with AF and are potential markers of AF (75). Xia et al. showed for the first time that plasma miRNA-150 levels in patients with atrial fibrillation are significantly lower than those in healthy individuals, which is a potential biomarker to aid in the diagnosis of atrial fibrillation (76). These studies indicate that miRNAs differentially expressed in plasma of patients with AF and postoperative patients can play an important indicator role in the diagnosis and prognosis monitoring of AF.
4. miRNAs involved in the regulation of electrical remodeling
Electrical remodeling of atrial muscle is closely related to the occurrence of AF. Electrical remodeling refers to recurrent episodes of AF or continuous atrial stimulation, which leads to progressive shortening of the effective refractory period of the atrium, and the decrease, reversal or disappearance of the physiological frequency adaptation of the atrial refractory period, making AF more likely to be induced and sustained (87, 92). AF is caused by abnormal electrical activity of atrial myocardium. During the occurrence of AF, many ion channels also have significant changes, mainly including: L-type Ca2+ channel, transient outward K+ channel, strong inward rectification K+ channel (IK1), acetylcholine-activated K+ channel (IK, ACh), and ultra-fast delayed rectification K+ channel (IKur) (93, 94).
Yang et al. found that the expression of miR-328 was increased in the atrial tissue of AF mouse models, and the high expression of miR-328 could reduce the L-type Ca2+ current and shorten the duration of atrial action potential. Mechanism studies have confirmed that CACNA1C and CACNB1 are the target genes of miR-328, and miR-328 can interact with L-type Ca2+ channel protein subunits to participate in atrial electroremodeling in AF (51). Nattel et al. found that the expression of miR-26 was down-regulated in the atrial tissues of AF patients, and low-expressed miR-26 was a potential regulatory gene for the electrophysiological effects of Ca2+ dependent nuclear factor of activated T cells (NFAT) signaling pathway, and an important participant in the persistence of AF (49). Ricardo et al. found that the high expression of miR-21 in cardiomyocytes of patients with AF was negatively correlated with the expression of CACNA1C and the density of I (Ca, L), suggesting that miR-21 may be involved in the downregulation of L-type Ca2+ I (Ca, L) induced by chronic AF, and is the key to the persistence of AF (95). Similarly, Qiu et al. found that CACNA1C is a direct target gene of miR-29a-3p, and miR-29a-3p negatively regulates CACNA1C. miR-29a-3p may be a potential target for AF treatment (41). Lee et al. found that miR-499 was significantly upregulated in AF, resulting in downregulation of small conductance calcium-activated potassium channel 3 (SK3), which may contribute to electrical remodeling of AF and is a novel site associated with the onset of AF (52). Katsushige et al. used high-throughput sequencing analysis to find that miR-30d was significantly up-regulated in myocardial cells of AF patients, and functional enrichment analysis found that miR-30d was a candidate gene for ion channel remodeling. Interference with miR-30d down-regulated the expression of kcnj3/Kir3.1, accompanied by a decrease in the acetylcholine-sensitive internal rectification K+ current (IK.ACh) (43). Barbara et al. found that miR-221 reduced the abundance and function of L-type Ca2+ channels and Kcnj5 channels. MiR-221 can regulate L-type Ca2+ channels and Kcnj5 channels, thus potentially contributing to the generation and propagation of cardiac excitation (50).
5. miRNAs involved in the regulation of structural remodeling
Electrical remodeling is the pathological change in the initial stage of AF, while structural remodeling is the material basis for the long-term maintenance of AF, and it is also the most obvious change of atrium (96, 97). Atrial dilatation and fibrosis are the main features of structural remodeling in AF. Atrial fibrosis may lead to slowing of conduction velocity, conduction block to promote reentry and increase susceptibility to AF (98).
Studies have shown that connective tissue growth factor (CTGF) plays an important role in the process of fibrosis. Zhang et al. found that the expression of miR-132 decreased in AF cardiomyocytes. Luciferase assay confirmed that miR-132 could bind to the 3 ‘-untranslated region of CTGF, thereby inhibiting the expression of CTGF and regulating the fibrosis of cardiac fibroblasts (46). Yang et al. found that overexpression of miR-10a significantly prolonged the duration of AF and decreased Smad7 protein expression. TGF-β1 reversed the inhibitory effect of miR-10a on Smad7, alleviated atrial remodeling, and ultimately inhibited cardiac fibrosis (35). Similarly, Xu et al. found that miR-29b-3p could reduce the degree of atrial fibrosis, and high expression of miR-29b-3p could reduce the expression of fibrosis markers collagen- I and a-SMA, and increase the protein expression of Cx43, thus reversing atrial remodeling (29). Studies have shown that the expression of miR-205-5p is decreased in atrial tissues of patients with AF, and overexpression of miR-205-5p can reduce the expression of TGF-β1, α-SMA, Col III and other fibrosis-related proteins. Mechanism studies have shown that miR-205-5p regulates H3 histone methylation by targeting EHMT2, promotes IGFBP3 expression, and further affects atrial myocyte fibrosis (11). The study found that the expression of miR-29b was low in the atrial tissue of AF rats, overexpression miR-29b can reduce atrial fibrosis, reduce the expression of COL1A1, COL3A1 and TGFβ1, and shorten the duration of AF in rats (14). In addition, the expression of miR-135b was down-regulated in AF tissues, while the expression of miR-135b target genes TGFBR1 and TGFBR2 was up-regulated in myocardial fibroblasts. Quercetin can promote miR-135b expression, inhibit TGF-β/Smads pathway, reduce atrial tissue fibrosis and collagen deposition, and thus relieve AF (19). Xu et al. found that miR-101a-3p may prevent AF in rats by targeting EZH2 to inhibit collagen synthesis and atrial fibrosis, which provides a potential target for the prevention of AF (22). miR-1202 was found to negatively regulate atrial fibrosis by targeting nNOS by reducing cell differentiation, collagen deposition, and TGF-β1/Smad2/3 pathway activity (23). Overexpression of miR-133a can inhibit the proliferation and migration of atrial cells, reduce the expression of fibrosis markers and CTGF protein, and improve myocardial fibrosis (24).
6. miRNAs involved in the regulation of neurohormonal disorders
Autonomic dysfunction is a type of dysfunction that occurs when the balance between sympathetic and parasympathetic nerves is disrupted. cardiac autonomic nerve remodeling (ANR) refers to the changes in the distribution density and spatial arrangement of the autonomic nerve caused by some diseases of the heart (99–102).
Studies have shown that the contents of tetrahydrobioterin (BH4) and NO are related to nerve regeneration. GCH1 is the rate-limiting enzyme of BH4 synthesis. Hou et al. found that the expression of miR-206 was increased in atrial fibrillation myocarde. High expression of miR-206 could inhibit GCH1, thus affecting the content of BH4 and NO in myocarde (103). In a similar study, miR-206 expression was increased in the the left superior ganglionated plexus (SLGPs). High expression of miR-206 inhibited the expression of superoxide dismutase 1 (SOD1) and increased the levels of reactive oxygen species (ROS) in vitro and in vivo, further exacerbating ANR (45). miR-662 can also regulate the expression of neuropeptides and participate in the occurrence and development of AF after myocardial infarction (17). It was found that the levels of miR-155-5p and miR-24-3p were significantly decreased and the levels of eNOS and NO were increased in patients with AF after ablation compared with those who did not receive ablation therapy (36). Casadei B et al. found that atrial specific upregulation of miR-31 in AF resulted in inhibition of muscular dystrophin (DYS) translation and accelerated degradation of nNOS mRNA, leading to significant reductions in atrial DYS and nNOS protein content and nitric oxide availability. Inhibition of miR-31 restores DYS and nNOS in human AF and normalizes APD and rate dependence of APD (42).
7. ncRNAs and AF-beyond miRNAs
With the increase of studies on ncRNAs in AF, lncRNAs and circRNAs play an increasingly significant role in AF. Therefore, in addition to miRNAs, this manuscript also discussed the current research content of other ncRNAs in AF.
CHA 2 ds2-VASc score was originally used to stratify stroke risk in patients with AF, in order to study whether lncRNAs could improve the predictive ability of CHA 2 ds2-VASc score for stroke. Li et al. added the ability of lncRNA expression level to predict stroke in CHA 2 ds2-VASc scoring model. The results showed that lncRNA H19 plasma expression level was correlated with the risk of stroke in patients with AF, which could significantly improve the ability to predict the risk of stroke in patients with AF, and was a potential prognostic monitoring marker (77). LncRNA GAS5 is significantly down-regulated in the plasma of patients with AF, which is a potential biomarker for the diagnosis and prognosis monitoring of AF (78). Similar studies have found that has_circ_0006314 and hsa_circ_0055387 also have potential predictive value for postoperative AF (79). Fan et al. used GEO database to screen out two different circRNAs. The expression of hsa_circ_0070391 in plasma was up-regulated and hsa_circ_0003935 down-regulated. The area under ROC curve indicated that both of them had high diagnostic efficiency (80). Wang et al. examined plasma circ 8196-RYR2 levels in 136 patients following ablation of AF, suggesting that circ 8196-RYR2 could be used as a new predictor of late recurrence after surgical ablation (81). Another study also show that low expression of circRNA_2773 is a potential diagnostic marker for AF (82).
AF is often accompanied by excessive proliferation of cardiac fibroblasts (CFs). It was found that the expression of HOTAIR was increased in the myocardium of patients with AF, and Ang II significantly increased the activity of atrial fibroblasts. HOTAIR knockdown can significantly inhibit AF cardiac tissue fibrosis by regulating Wnt signaling pathway (9). Knocking down LINC01013 reduced baseline expression of fibrosis markers and their response to TGF-β1. TGF-β1 stimulated atrial fibroblasts to induce the expression of LINC01013, and its knockdown reduced the activation of fibroblasts (56). Plasma H19 levels were significantly higher in patients with AF compared with healthy volunteers. Upregulation of H19 expression contributes to the proliferation and synthesis of extracellular matrix (ECM) related proteins, thereby promoting myocardial fibrosis (53). It was found that the serum TUG1 level was elevated and the expression of miR-29b-3p was low in patients with AF. Pearson correlation analysis showed that TUG1 was negatively correlated with miR-29b-3p expression in AF patients. TUG1 knockdown inhibits vascular endothelium-induced cardiomyocyte proliferation (57). NEAT1 expression was up-regulated in atrial tissues of patients with AF, and was positively correlated with the expression of type I collagen (coll I) and type III collagen (coll III). In addition, the loss of NEAT1 attenuates angiotensin II (Ang II), leading to atrial fibroblast proliferation, migration, and collagen production. These findings suggest that NEAT1 plays an important role in atrial fibrosis and is a new potential molecular target for the treatment of AF (54). In AF patients, LICPAR and TGF-β1 expression were up-regulated and positively correlated. Further analysis showed that Ang II increased LIPCAR, Smad2/3 phosphorylation, and α-smooth muscle actin (α-SMA) levels. Up-regulation of LIPCAR could further promote the promoting effects of Ang II on the phosphorylation levels of LIPCAR, Collagen I, Collagen II, α-SMA and Smad2/3, cell viability and proliferation of atrial fibroblasts. These studies suggest that lncRNA LICPAR regulates atrial fibrosis primarily by regulating the TGF-β/Smad pathway (55). Studies found that down-regulation of lncRNA MIAT could significantly relieve AF, increase atrial effective refractory period (AERP), inhibit the expression of fibrosis-related genes coll I, coll III, CTGF, TGF-β1, and effectively reduce AF induced atrial fibrosis (61). PCAT-1 expression was increased in AF patients. PCAT-1 knockdown inhibited the proliferation of AC16 cells. Mechanism studies showed that TGF-β1 was the target of PCAT-1, and its expression in AF tissues was positively correlated with that of PCAT-1. PCAT-1 can promote the proliferation of AF cells by promoting TGF-β1 (58). The expression of GAS5 in myocardium of AF patients was significantly decreased. Overexpression of GAS5 can inhibit the growth of AC16 cells. In addition, further experiments showed that ALK5 was the target of GAS5, and its expression in AF tissue was negatively correlated with that of GAS5. lncRNA GAS5 may inhibit AF cell fibrosis by inhibiting ALK5 (60). The expression of PVT1 in AF patients was increased and positive for coll I and coll III. Overexpression of PVT1 promoted Ang-II-induced atrial fibroblast proliferation, collagen generation, and TGF-β1/Smad signaling activation, while PVT1 knockdown did the opposite. Mechanically, PVT1 acts as a sponge for miR-128-3p and promotes Sp1 expression, thereby activating the TGF-β1/Smad signaling pathway (62).
Hou et al. found that lncRNA TCONS_00075467 may also participate in atrial myocardial electrical remodeling. Interference with TCONS_00075467 can shorten the effective refractory period of the atria in vivo and reduce the duration of L-type calcium current and action potential in vitro (104). Similarly, lncRNA TCONS-00106987 is up-regulated in atrial tissue of patients with AF. Mechanism studies have shown that TCONS_00106987 induces the transcription of its target gene KCNJ2 through miR-26, and increases the inward rectification K+ current (IK1). Thus facilitating electrical reconfiguration (59). Studies have shown that interference with lncRNA AK055347 can inhibit the activity of cardiomyocytes, accompanied by the downregulation of Cyp450 and ATP synthase. Mechanism studies have confirmed that AK055347 may regulate the mitochondrial energy production by regulating Cyp450, ATP synthase and MSS51, thus participating in the pathogenesis of AF (64).
8. Exosome-associated ncRNAs involved in the regulation of AF
In recent years, it has been found that exosome-derived ncRNAs have different expression profiles in various diseases and are a potential non-invasive diagnostic biomarker, which has been widely studied in the medical field. Similarly, exosomes can also be detected in body fluids of patients with atrial fibrillation, and the non-coding RNA carried by them is of great significance for auxiliary diagnosis and prognostic monitoring of AF (105, 106).
Wei et al. demonstrated differences in the expression of miRNAs in plasma exosomes in patients with AF. Among them, miR-92b-3p, miR-1306-5p and miR-let-7b-3p had significant differences, and gene enrichment analysis showed that these miRNAs and target genes were mainly involved in the occurrence of AF through affecting biological processes such as energy metabolism, lipid metabolism, inflammation and enzyme activity (107). Similar studies have found that miR-483-5p, miR-142-5p and miR-223-3p are also involved in the occurrence and development of AF (108). Joung et al. found that exosomes in the peripheral blood of patients with atrial fibrillation can reduce cardiomyocyte viability, lead to abnormal Ca2+ channel and induce reactive oxygen species (ROS) production. High-throughput sequencing found that miR-30a-5p expression was decreased in peripheral blood exosomes of patients with AF, and exosomes with high expression of miR-30a-5p could attenuate pacemaker induced Ca2+ channel abnormalities (109). Hou et al. screened the differential miRNAs of peripheral blood and exosomes in 40 patients with AF, and found that miR-124-3p was significantly up-regulated, and the high expression of miR-124-3p could improve the viability and proliferation ability of myocardial fibroblasts. Mechanism studies have shown that miR-124-3p can promote the activation and proliferation of fibroblasts through AXIN1 by regulating the WNT/β-catenin signaling pathway (110). Similarly, Exosomal lncRNAs are also potential biomarkers for AF. Joung et al. identified 26 differentially expressed lncrnas in serum exosomes from patients with persistent AF. lncRNAs LOC105377989 and LOC107986997 continued to increase, has significant diagnostic effectiveness for AF, and is a potential biomarker for the diagnosis of AF (106). Lei et al. using GEO database, LINC00636 was found to be an antifibrotic molecule with decreased expression in peripheral blood exosomes of patients with AF. Mechanism studies have shown that LINC00636 can promote the expression of miR-450a-2-3p, thereby inhibiting the expression of MAPK1, and thereby improve cardiac fibrosis in patients with AF (111).
9. Conclusions
In recent years, with the deepening of research, ncRNAs play an important role in the occurrence and development of AF. Differential expression of ncRNAs in peripheral blood of patients with AF provides a new theoretical basis for auxiliary diagnosis of AF. At the same time, ncRNAs are involved in myocardial cell remodeling and ion channel remodeling, providing a new scheme for the treatment of AF.
This manuscript reviews the research progress of ncRNAs in the occurrence, treatment and potential biomarkers of AF. According to the existing studies, we can find that ncRNAs are closely related to AF and involved in the occurrence and progression of AF, which is worthy of further study and has great clinical significance.
Author contributions
ZX and JZ drafted the manuscript; JL and LW reviewed and edited the manuscript. JD provided ideas. All authors contributed to the article and approved the submitted version.
Funding
This work was supported by the Science and Technology Project of Zhangjiagang City (ZKS2043), Zhangjiagang City Health Youth Science and Technology Project (ZJGQNKJ202113) and Suzhou Science and Technology Development Plan (SKJYD2021006).
Conflict of interest
The authors declare that the research was conducted in the absence of any commercial or financial relationships that could be construed as a potential conflict of interest.
Publisher's note
All claims expressed in this article are solely those of the authors and do not necessarily represent those of their affiliated organizations, or those of the publisher, the editors and the reviewers. Any product that may be evaluated in this article, or claim that may be made by its manufacturer, is not guaranteed or endorsed by the publisher.
References
1. Lau DH, Nattel S, Kalman JM, Sanders P. Modifiable risk factors and atrial fibrillation. Circulation. (2017) 136:583–96. doi: 10.1161/CIRCULATIONAHA.116.023163
2. Staerk L, Sherer JA, Ko D, Benjamin EJ, Helm RH. Atrial fibrillation: epidemiology, pathophysiology, and clinical outcomes. Circ Res. (2017) 120:1501–17. doi: 10.1161/CIRCRESAHA.117.309732
3. Abed HS, Wittert GA, Leong DP, Shirazi MG, Bahrami B, Middeldorp ME, et al. Effect of weight reduction and cardiometabolic risk factor management on symptom burden and severity in patients with atrial fibrillation: a randomized clinical trial. Jama. (2013) 310:2050–60. doi: 10.1001/jama.2013.280521
4. Lau DH, Linz D, Sanders P. New findings in atrial fibrillation mechanisms. Card Electrophysiol Clin. (2019) 11:563–71. doi: 10.1016/j.ccep.2019.08.007
5. Voskoboinik A, Kalman JM, De Silva A, Nicholls T, Costello B, Nanayakkara S, et al. Alcohol abstinence in drinkers with atrial fibrillation. N Engl J Med. (2020) 382:20–8. doi: 10.1001/jama.2013.280521
6. Antman EM, Leopold JA, Sauer WH, Zei PC. Atrial fibrillation—what is it and how is it treated? N Engl J Med. (2022) 387:e38. doi: 10.1056/NEJMp2212939
7. Dawson K, Wakili R, Ordög B, Clauss S, Chen Y, Iwasaki Y, et al. MicroRNA29: a mechanistic contributor and potential biomarker in atrial fibrillation. Circulation. (2013) 127:1466–75. 1475e1461–1428. doi: 10.1161/CIRCULATIONAHA.112.001207
8. Hijazi Z, Oldgren J, Lindbäck J, Alexander JH, Connolly SJ, Eikelboom JW, et al. The novel biomarker-based ABC (age, biomarkers, clinical history)-bleeding risk score for patients with atrial fibrillation: a derivation and validation study. Lancet. (2016) 387:2302–11. doi: 10.1016/S0140-6736(16)00741-8
9. Tan W, Wang K, Yang X, Wang K, Wang N, Jiang TB. LncRNA HOTAIR promotes myocardial fibrosis in atrial fibrillation through binding with PTBP1 to increase the stability of Wnt5a. Int J Cardiol. (2022) 369:21–8. doi: 10.1016/j.ijcard.2022.06.073
10. Halushka PV, Goodwin AJ, Halushka MK. Opportunities for microRNAs in the crowded field of cardiovascular biomarkers. Annu Rev Pathol. (2019) 14:211–38. doi: 10.1146/annurev-pathmechdis-012418-012827
11. Xiao Z, Xie Y, Huang F, Yang J, Liu X, Lin X, et al. MicroRNA-205-5p plays a suppressive role in the high-fat diet-induced atrial fibrosis through regulation of the EHMT2/IGFBP3 axis. Genes Nutr. (2022) 17:11. doi: 10.1186/s12263-022-00712-z
12. Lai YJ, Tsai FC, Chang GJ, Chang SH, Huang CC, Chen WJ, et al. miR-181b targets semaphorin 3A to mediate TGF-β-induced endothelial-mesenchymal transition related to atrial fibrillation. J Clin Invest. (2021) 23(1):6. doi: 10.3390/ijms23010006
13. Park H, Park H, Park J. Circulating microRNA-423 attenuates the phosphorylation of calcium handling proteins in atrial fibrillation. Mol Med Rep. (2015) 66(1):1–11.
14. Han X, Wang S, Yong Z, Zhang X, Wang X. miR-29b ameliorates atrial fibrosis in rats with atrial fibrillation by targeting TGFβRΙ and inhibiting the activation of smad-2/3 pathway. J Bioenerg Biomembr. (2022) 54:81–91. doi: 10.1007/s10863-022-09934-7
15. Wiedmann F, Kraft M, Kallenberger S, Büscher A, Paasche A. MicroRNAs regulate TASK-1 and are linked to myocardial dilatation in atrial fibrillation. J Am Heart Assoc. (2022) 11:e023472. doi: 10.1161/JAHA.121.023472
16. Pradhan K, Niehues P, Neupane B, Maleck C, Sharif-Yakan A, Emrani M, et al. MicroRNA-21 mediated cross-talk between cardiomyocytes and fibroblasts in patients with atrial fibrillation. Front Cardiovasc Med. (2023) 10:1056134. doi: 10.3389/fcvm.2023.1056134
17. Zhang P, Liu B. Integrative bioinformatics analysis reveals that infarct-mediated overexpression of potential miR-662/CREB1 pathway-induced neuropeptide VIP is associated with the risk of atrial fibrillation: a correlation analysis between myocardial electrophysiology and neuroendocrine. Dis Markers. (2022) 132(13):e142548. doi: 10.1155/2021/8116633
18. Wei F, Ren W, Zhang X, Wu P, Fan J. miR-425-5p is negatively associated with atrial fibrosis and promotes atrial remodeling by targeting CREB1 in atrial fibrillation. J Cardiol. (2022a) 79:202–10. doi: 10.1016/j.jjcc.2021.09.012
19. Wang H, Jiang W, Hu Y, Wan Z, Bai H, Yang Q, et al. Quercetin improves atrial fibrillation through inhibiting TGF-β/smads pathway via promoting MiR-135b expression. Phytomedicine. (2021a) 93:153774. doi: 10.1016/j.phymed.2021.153774
20. Ye Q, Liu Q, Ma X, Bai S, Chen P, Zhao Y, et al. MicroRNA-146b-5p promotes atrial fibrosis in atrial fibrillation by repressing TIMP4. J Cell Mol Med. (2021) 25:10543–53. doi: 10.1111/jcmm.16985
21. Garcia-Elias A, Tajes M, Yañez-Bisbe L, Enjuanes C, Comín-Colet J, Serra SA, et al. Atrial fibrillation in heart failure is associated with high levels of circulating microRNA-199a-5p and 22-5p and a defective regulation of intracellular calcium and cell-to-cell communication. Int J Mol Sci. (2023) 10:1056134. doi: 10.3390/ijms221910377
22. Zhu J, Zhu N, Xu J. Mir-101a-3p overexpression prevents acetylcholine-CaCl(2)-induced atrial fibrillation in rats via reduction of atrial tissue fibrosis, involving inhibition of EZH2. Mol Med Rep. (2021) 2021:8116633.
23. Xiao J, Zhang Y, Tang Y, Dai H, Ouyang Y, Li C, et al. MiRNA-1202 promotes the TGF-β1-induced proliferation, differentiation and collagen production of cardiac fibroblasts by targeting nNOS. PLoS One. (2021a) 16:e0256066.34428251
24. Su H, Su H, Liu CH, Hu HJ, Zhao JB, Zou T, et al. H(2)S inhibits atrial fibrillation-induced atrial fibrosis through miR-133a/CTGF axis. Cytokine. (2021) 146:155557. doi: 10.1016/j.cyto.2021.155557
25. Xiao Z, Reddy DPK, Xue C, Liu X, Chen X, Li J, et al. Profiling of miR-205/P4HA3 following angiotensin II-induced atrial fibrosis: implications for atrial fibrillation. Front Cardiovasc Med. (2021b) 8:609300. doi: 10.3389/fcvm.2021.609300
26. Xiao J, Zhang Y. hsa-miR-4443 inhibits myocardial fibroblast proliferation by targeting THBS1 to regulate TGF-β1/α-SMA/collagen signaling in atrial fibrillation. Braz J Med Biol Res. (2021) 54:e10692. doi: 10.1590/1414-431X202010692
27. Wang J, Ye Q, Bai S, Chen P, Zhao Y, Ma X, et al. Inhibiting microRNA-155 attenuates atrial fibrillation by targeting CACNA1C. J Mol Cell Cardiol. (2021b) 155:58–65. doi: 10.1016/j.yjmcc.2021.02.008
28. Zhelankin AV, Vasiliev SV, Stonogina DA, Babalyan KA, Sharova EI, Doludin YV, et al. Elevated plasma levels of circulating extracellular miR-320a-3p in patients with paroxysmal atrial fibrillation. Int J Mol Sci. (2021) 16(8):e0256066. doi: 10.3390/ijms21103485
29. Lv X, Lu P, Hu Y, Xu T. Overexpression of MiR-29b-3p inhibits atrial remodeling in rats by targeting PDGF-B signaling pathway. Oxid Med Cell Longev. (2021) 146:155557. doi: 10.1155/2021/3763529
30. Xu J, Lei S, Sun S, Zhang W, Zhu F, Yang H, et al. MiR-324-3p regulates fibroblast proliferation via targeting TGF-β1 in atrial fibrillation. Int Heart J. (2020) 61:1270–8. doi: 10.1536/ihj.20-423
31. Chen Y, Chang G. IL-6-miR-210 suppresses regulatory T cell function and promotes atrial fibrosis by targeting Foxp3. Mol Cells. (2020) 43:438–47. doi: 10.14348/molcells.2019.2275
32. Lv X, Li J, Hu Y, Wang S, Yang C, Li C, et al. Overexpression of miR-27b-3p targeting Wnt3a regulates the signaling pathway of wnt/β-catenin and attenuates atrial fibrosis in rats with atrial fibrillation. Oxid Med Cell Longev. (2021) 155:58–65. doi: 10.1155/2019/5703764
33. Yu RB, Li K, Wang G, Gao GM, Du JX. MiR-23 enhances cardiac fibroblast proliferation and suppresses fibroblast apoptosis via targeting TGF-β1 in atrial fibrillation. Eur Rev Med Pharmacol Sci. (2019) 23:4419–24.31173317
34. Cheng WL, Kao YH. MicroRNA-133 suppresses ZFHX3-dependent atrial remodelling and arrhythmia. Acta Physiol (Oxf). (2019) 227:e13322. doi: 10.1111/apha.13322
35. Li PF, He RH, Shi SB, Li R, Wang QT, Rao GT, et al. Modulation of miR-10a-mediated TGF-β1/smads signaling affects atrial fibrillation-induced cardiac fibrosis and cardiac fibroblast proliferation. Biosci Rep. (2020) 61(6):1270–8. doi: 10.1042/BSR20181931
36. Wang M, Sun L. Ablation alleviates atrial fibrillation by regulating the signaling pathways of endothelial nitric oxide synthase/nitric oxide via miR-155-5p and miR-24-3p. J Cell Biochem. (2019) 120:4451–62. doi: 10.1002/jcb.27733
37. Xie H, Fu JL, Xie C. MiR-138-5p is downregulated in patients with atrial fibrillation and reverses cardiac fibrotic remodeling via repressing CYP11B2. Eur Rev Med Pharmacol Sci. (2018) 22:4642–7.30058705
38. Wang Y, Cai H, Li H, Gao Z, Song K. Atrial overexpression of microRNA-27b attenuates angiotensin II-induced atrial fibrosis and fibrillation by targeting ALK5. Hum Cell. (2018) 31:251–60. doi: 10.1007/s13577-018-0208-z
39. Xu J, Wu H, Chen S, Qi B, Zhou G, Cai L, et al. MicroRNA-3°c suppresses the pro-fibrogenic effects of cardiac fibroblasts induced by TGF-β1 and prevents atrial fibrosis by targeting TGFβRII. J Cell Mol Med. (2018) 22:3045–57. doi: 10.1111/jcmm.13548
40. Cañón S, Caballero R, Herraiz-Martínez A, Pérez-Hernández M, López B, Atienza F, et al. miR-208b upregulation interferes with calcium handling in HL-1 atrial myocytes: implications in human chronic atrial fibrillation. J Mol Cell Cardiol. (2016) 99:162–73. doi: 10.1016/j.yjmcc.2016.08.012
41. Zhao Y, Yuan Y, Qiu C. Underexpression of CACNA1C caused by overexpression of microRNA-29a underlies the pathogenesis of atrial fibrillation. Med Sci Monit. (2016) 22:2175–81. doi: 10.12659/MSM.896191
42. Reilly S, Liu X, Carnicer R, Rajakumar T, Sayeed R, Krasopoulos G, et al. Evaluation of the role of miR-31-dependent reduction in dystrophin and nNOS on atrial-fibrillation-induced electrical remodelling in man. Lancet. (2015) 385(Suppl 1):S82. doi: 10.1016/S0140-6736(15)60397-X
43. Morishima M, Iwata E, Nakada C, Tsukamoto Y, Takanari H, Miyamoto S, et al. Atrial fibrillation-mediated upregulation of miR-30d regulates myocardial electrical remodeling of the G-protein-gated K(+) channel. IK ACh Circ J. (2016) 80:1346–55. doi: 10.1253/circj.CJ-15-1276
44. Yuan CT, Li XX, Cheng QJ, Wang YH, Wang JH, Liu CL. MiR-30a regulates the atrial fibrillation-induced myocardial fibrosis by targeting snail 1. Int J Clin Exp Pathol. (2015) 8:15527–36.26884822
45. Zhang Y, Zheng S, Geng Y, Xue J, Wang Z, Xie X, et al. MicroRNA profiling of atrial fibrillation in canines: miR-206 modulates intrinsic cardiac autonomic nerve remodeling by regulating SOD1. PLoS One. (2015) 10:e0122674.25816284
46. Qiao G, Xia D, Cheng Z, Zhang G. Mir-132 in atrial fibrillation directly targets connective tissue growth factor. Mol Med Rep. (2017) 16:4143–50. doi: 10.3892/mmr.2017.7045
47. Zhu H, Xue H, Jin QH, Guo J, Chen YD. Increased expression of ryanodine receptor type-2 during atrial fibrillation by miR-106-25 cluster independent mechanism. Exp Cell Res. (2019) 375:113–7. doi: 10.1016/j.yexcr.2018.11.025
48. Adam O, Löhfelm B, Thum T, Gupta SK, Puhl SL, Schäfers HJ, et al. Role of miR-21 in the pathogenesis of atrial fibrosis. Basic Res Cardiol. (2012) 107:278. doi: 10.1007/s00395-012-0278-0
49. Luo X, Pan Z, Shan H, Xiao J, Sun X, Wang N, et al. MicroRNA-26 governs profibrillatory inward-rectifier potassium current changes in atrial fibrillation. J Clin Invest. (2013) 123:1939–51. doi: 10.1172/JCI62185
50. Binas S, Knyrim M, Hupfeld J, Kloeckner U, Rabe S, Mildenberger S, et al. miR-221 and −222 target CACNA1C and KCNJ5 leading to altered cardiac ion channel expression and current density. Cell Mol Life Sci. (2020) 77:903–18. doi: 10.1007/s00018-019-03217-y
51. Lu Y, Zhang Y, Wang N, Pan Z, Gao X, Zhang F, et al. MicroRNA-328 contributes to adverse electrical remodeling in atrial fibrillation. Circulation. (2010) 122:2378–87. doi: 10.1161/CIRCULATIONAHA.110.958967
52. Ling TY, Wang XL, Chai Q, Lau TW, Koestler CM, Park SJ, et al. Regulation of the SK3 channel by microRNA-499–potential role in atrial fibrillation. Heart Rhythm. (2013) 10:1001–9. doi: 10.1016/j.hrthm.2013.03.005
53. Guo F, Tang C. LncRNA H19 drives proliferation of cardiac fibroblasts and collagen production via suppression of the miR-29a-3p/miR-29b-3p-VEGFA/TGF-β axis. Mol Cells. (2022) 45:122–33. doi: 10.14348/molcells
54. Dai H, Zhao N, Liu H, Zheng Y, Zhao L. LncRNA nuclear-enriched abundant transcript 1 regulates atrial fibrosis via the miR-320/NPAS2 axis in atrial fibrillation. Front Pharmacol. (2021) 12:647124. doi: 10.3389/fphar.2021.647124
55. Wang H, Song T, Zhao Y, Zhao J, Wang X, Fu X. Long non-coding RNA LICPAR regulates atrial fibrosis via TGF-β/smad pathway in atrial fibrillation. Tissue Cell. (2020) 67:101440. doi: 10.1016/j.tice.2020.101440
56. Quaife NM, Chothani S, Schulz JF, Lindberg EL, Vanezis K, Adami E, et al. LINC01013 Is a determinant of fibroblast activation and encodes a novel fibroblast-activating micropeptide. J Cardiovasc Transl Res. (2023) 16:77–85. doi: 10.1007/s12265-022-10288-z
57. Guo Y, Sun Z, Chen M, Lun J. LncRNA TUG1 regulates proliferation of cardiac fibroblast via the miR-29b-3p/TGF-β1 axis. Front Cardiovasc Med. (2021) 8:646806. doi: 10.3389/fcvm.2021.646806
58. Chen Q, Feng C, Liu Y, Li QF, Qiu FY, Wang MH, et al. Long non-coding RNA PCAT-1 promotes cardiac fibroblast proliferation via upregulating TGF-β1. Eur Rev Med Pharmacol Sci. (2019) 23:10517–22.31841207
59. Du J, Li Z, Wang X, Li J, Liu D, Wang X, et al. Long noncoding RNA TCONS-00106987 promotes atrial electrical remodelling during atrial fibrillation by sponging miR-26 to regulate KCNJ2. J Cell Mol Med. (2020) 24:12777–88.doi: 10.1111/jcmm.15869
60. Lu J, Xu FQ, Guo JJ, Lin PL, Meng Z, Hu LG, et al. Long noncoding RNA GAS5 attenuates cardiac fibroblast proliferation in atrial fibrillation via repressing ALK5. Eur Rev Med Pharmacol Sci. (2019) 23:7605–10.31539152
61. Yao L, Zhou B, You L, Hu H, Xie R. LncRNA MIAT/miR-133a-3p axis regulates atrial fibrillation and atrial fibrillation-induced myocardial fibrosis. Mol Biol Rep. (2020) 47:2605–17. doi: 10.1007/s11033-020-05347-0
62. Cao F, Li Z, Ding WM, Yan L, Zhao QY. LncRNA PVT1 regulates atrial fibrosis via miR-128-3p-SP1-TGF-β1-smad axis in atrial fibrillation. Mol Med. (2019) 25:7. doi: 10.1186/s10020-019-0074-5
63. Shen C, Kong B, Liu Y, Xiong L, Shuai W, Wang G, et al. YY1-induced Upregulation of lncRNA KCNQ1OT1 regulates angiotensin II-induced atrial fibrillation by modulating miR-384b/CACNA1C axis. Biochem Biophys Res Commun. (2018) 505:134–40. doi: 10.1016/j.bbrc.2018.09.064
64. Chen G, Guo H, Song Y, Chang H, Wang S, Zhang M, et al. Long non-coding RNA AK055347 is upregulated in patients with atrial fibrillation and regulates mitochondrial energy production in myocardiocytes. Mol Med Rep. (2016) 14:5311–7. doi: 10.3892/mmr.2016.5893
65. Zhang L, Lou Q, Zhang W, Yang W, Li L, Zhao H, et al. CircCAMTA1 facilitates atrial fibrosis by regulating the miR-214-3p/TGFBR1 axis in atrial fibrillation. J Mol Histol. (2023) 54:55–65. doi: 10.1007/s10735-022-10110-9
66. Gao Y, Liu Y, Fu Y, Wang Q, Liu Z, Hu R, et al. The potential regulatory role of hsa_circ_0004104 in the persistency of atrial fibrillation by promoting cardiac fibrosis via TGF-β pathway. BMC Cardiovasc Disord. (2021) 21:25. doi: 10.1186/s12872-021-01847-4
67. Liu X, Wu M, He Y, Gui C, Wen W, Jiang Z, et al. Construction and integrated analysis of the ceRNA network hsa_circ_0000672/miR-516a-5p/TRAF6 and its potential function in atrial fibrillation. Sci Rep. (2023) 13:7701. doi: 10.1038/s41598-023-34851-z
68. Wu N, Li C, Xu B, Xiang Y, Jia X, Yuan Z, et al. Circular RNA mmu_circ_0005019 inhibits fibrosis of cardiac fibroblasts and reverses electrical remodeling of cardiomyocytes. BMC Cardiovasc Disord. (2021) 21:308. doi: 10.1186/s12872-021-02128-w
69. Nopp S, Van Der Bent ML, Kraemmer D. Circulatory miR-411-5p as a novel prognostic biomarker for Major adverse cardiovascular events in patients with atrial fibrillation. Int J Mol Sci. (2016) 14(6):5311–7. doi: 10.3390/ijms24043861
70. Lage R, Cebro-Márquez M. Circulating miR-451a expression may predict recurrence in atrial fibrillation patients after catheter pulmonary vein ablation. Cells. (2023) 54(1):55–65. doi: 10.3390/cells12040638
71. Natsume Y, Oaku K, Takahashi K, Nakamura W, Oono A, Hamada S, et al. Combined analysis of human and experimental murine samples identified novel circulating MicroRNAs as biomarkers for atrial fibrillation. Circ J. (2018) 82:965–73. doi: 10.1253/circj.CJ-17-1194
72. Xu G, Cui Y, Jia Z, Yue Y, Yang S. The values of coronary circulating miRNAs in patients with atrial fibrillation. PLoS One. (2016) 11:e0166235.27855199
73. Harling L, Lambert J, Ashrafian H, Darzi A, Gooderham NJ, Athanasiou T. Elevated serum microRNA 483-5p levels may predict patients at risk of post-operative atrial fibrillation. Eur J Cardiothorac Surg. (2017) 51:73–8. doi: 10.1093/ejcts/ezw245
74. Yamac AH, Kucukbuzcu S, Ozansoy M, Gok O, Oz K, Erturk M, et al. Altered expression of micro-RNA 199a and increased levels of cardiac SIRT1 protein are associated with the occurrence of atrial fibrillation after coronary artery bypass graft surgery. Cardiovasc Pathol. (2016) 25:232–6. doi: 10.1016/j.carpath.2016.02.002
75. Liu T, Zhong S, Rao F, Xue Y, Qi Z, Wu S. Catheter ablation restores decreased plasma miR-409-3p and miR-432 in atrial fibrillation patients. Europace. (2016) 18:92–9. doi: 10.1093/europace/euu366
76. Liu Z, Zhou C, Liu Y, Wang S, Ye P, Miao X, et al. The expression levels of plasma micoRNAs in atrial fibrillation patients. PLoS One. (2012) 7:e44906. doi: 10.1371/journal.pone.0044906
77. Zhang Y, Wang D, Wu N, Chen X, Yuan Z, Jia X, et al. Role of circulating long non-coding RNA for the improvement of the predictive ability of the CHA 2 DS 2 -VASc score in patients with atrial fibrillation. Chin Med J (Engl). (2022) 135:1451–8. doi: 10.1097/CM9.0000000000002213
78. Shi J, Chen L, Chen S, Wu B, Yang K, Hu X. Circulating long noncoding RNA, GAS5, as a novel biomarker for patients with atrial fibrillation. J Clin Lab Anal. (2021) 35:e23572. doi: 10.1002/jcla.23572
79. Chen Y, Ouyang T, Yin Y, Fang C, Tang CE, Luo J, et al. Analysis of infiltrated immune cells in left atriums from patients with atrial fibrillation and identification of circRNA biomarkers for postoperative atrial fibrillation. Front Genet. (2022) 13:1003366. doi: 10.3389/fgene.2022.1003366
80. Wei F, Zhang X, Kuang X, Gao X, Wang J, Fan J. Integrated analysis of circRNA-miRNA-mRNA-mediated network and its potential function in atrial fibrillation. Front Cardiovasc Med. (2022b) 9:883205. doi: 10.3389/fcvm.2022.883205
81. Zhu X, Wang Y, Mo R, Chong H, Cao C, Fan F, et al. Left atrial appendage circular RNAs are new predictors of atrial fibrillation recurrence after surgical ablation in valvular atrial fibrillation patients. Heart Surg Forum. (2021b) 24:E968–e976. doi: 10.1532/hsf.4125
82. Wen JL, Ruan ZB, Wang F, Chen GC, Zhu JG, Ren Y, et al. Construction of atrial fibrillation-related circRNA/lncRNA-miRNA-mRNA regulatory network and analysis of potential biomarkers. J Clin Lab Anal. (2023) 37:e24833.36604807
83. Sagris M, Vardas EP. Atrial fibrillation: pathogenesis. Predisposing Factors, and Genetics. (2016) 25(3):232–6.
84. Brundel B, Ai X. Atrial fibrillation. Nat Rev Dis Primers. (2022) 8:21. doi: 10.1038/s41572-022-00347-9
85. Nattel S, Harada M. Atrial remodeling and atrial fibrillation: recent advances and translational perspectives. J Am Coll Cardiol. (2014) 63:2335–45. doi: 10.1016/j.jacc.2014.02.555
86. Mahajan R, Lau DH, Brooks AG, Shipp NJ, Manavis J, Wood JP, et al. Electrophysiological, electroanatomical, and structural remodeling of the atria as consequences of sustained obesity. J Am Coll Cardiol. (2015) 66:1–11. doi: 10.1016/j.jacc.2015.04.058
87. Takemoto Y, Ramirez RJ, Kaur K, Salvador-Montañés O, Ponce-Balbuena D, Ramos-Mondragón R, et al. Eplerenone reduces atrial fibrillation burden without preventing atrial electrical remodeling. J Am Coll Cardiol. (2017) 70:2893–905. doi: 10.1016/j.jacc.2017.10.014
88. Davidson KW, Barry MJ, Mangione CM, Cabana M, Caughey AB, Davis EM, et al. Screening for atrial fibrillation: uS preventive services task force recommendation statement. Jama. (2022) 327:360–7. doi: 10.1001/jama.2022.5207
89. Lee C. Screening for atrial fibrillation in asymptomatic older adults. N Engl J Med. (2022) 387:565–7. doi: 10.1056/NEJMclde2203726
90. Roberts E, Ludman AJ, Dworzynski K, Al-Mohammad A, Cowie MR, Mcmurray JJ, et al. The diagnostic accuracy of the natriuretic peptides in heart failure: systematic review and diagnostic meta-analysis in the acute care setting. Br Med J. (2015) 350:h910. doi: 10.1136/bmj.h910
91. Honda Y, Mok Y, Ishigami J, Ashley KE, Hoogeveen RC, Ballantyne CM, et al. The fifth-generation cardiac troponin T and cardiovascular disease in the community. J Am Coll Cardiol. (2021) 78:2019–21. doi: 10.1016/j.jacc.2021.08.066
92. Yoo S, Pfenniger A, Hoffman J, Zhang W, Ng J, Burrell A, et al. Attenuation of oxidative injury with targeted expression of NADPH oxidase 2 short hairpin RNA prevents onset and maintenance of electrical remodeling in the canine atrium: a novel gene therapy approach to atrial fibrillation. Circulation. (2020) 142:1261–78. doi: 10.1161/CIRCULATIONAHA.119.044127
93. Harada M, Tadevosyan A, Qi X, Xiao J, Liu T, Voigt N, et al. Atrial fibrillation activates AMP-dependent protein kinase and its regulation of cellular calcium handling: potential role in metabolic adaptation and prevention of progression. J Am Coll Cardiol. (2015) 66:47–58. doi: 10.1016/j.jacc.2015.04.056
94. Schmidt C, Wiedmann F, Zhou XB, Heijman J, Voigt N, Ratte A, et al. Inverse remodelling of K2P3.1K+ channel expression and action potential duration in left ventricular dysfunction and atrial fibrillation: implications for patient-specific antiarrhythmic drug therapy. Eur Heart J. (2017) 38:1764–74.28057773
95. Barana A, Matamoros M, Dolz-Gaitón P, Pérez-Hernández M, Amorós I, Núñez M, et al. Chronic atrial fibrillation increases microRNA-21 in human atrial myocytes decreasing L-type calcium current. Circ Arrhythm Electrophysiol. (2014) 7:861–8. doi: 10.1161/CIRCEP.114.001709
96. Beyer C, Tokarska L, Stühlinger M, Feuchtner G, Hintringer F, Honold S, et al. Structural cardiac remodeling in atrial fibrillation. JACC Cardiovasc Imaging. (2021) 14:2199–208. doi: 10.1016/j.jcmg.2021.04.027
97. Yamaguchi N, Xiao J, Narke D, Shaheen D, Lin X, Offerman E, et al. Cardiac pressure overload decreases ETV1 expression in the left atrium, contributing to atrial electrical and structural remodeling. Circulation. (2021) 143:805–20. doi: 10.1161/CIRCULATIONAHA.120.048121
98. Narducci ML, Volpe M. Atrial fibrosis detected by cardiac magnetic resonance in persistent atrial fibrillation: a useful risk stratifier but not ideal electrophysiological endpoint for catheter ablation. Eur Heart J. (2022) 43:3196–7. doi: 10.1093/eurheartj/ehac424
99. Wang W, Wang X, Zhang Y, Li Z, Xie X, Wang J, et al. Transcriptome analysis of canine cardiac fat pads: involvement of two novel long non-coding RNAs in atrial fibrillation neural remodeling. J Cell Biochem. (2015) 116:809–21. doi: 10.1002/jcb.25037
100. Oliveira M, Silva Júnior ELD, Martins YO, Rocha HaL. Cardiac autonomic nervous system remodeling may play a role in atrial fibrillation: a study of the autonomic nervous system and myocardial receptors. Arq Bras Cardiol. (2021) 117:999–1007. doi: 10.36660/abc.20200725
101. Jiang W, Xu M, Qin M, Zhang D, Wu S, Liu X, et al. Role and mechanism of lncRNA under magnetic nanoparticles in atrial autonomic nerve remodeling during radiofrequency ablation of recurrent atrial fibrillation. Bioengineered. (2022) 13:4173–84. doi: 10.1080/21655979.2021.2024324
102. Zhao J, Zhang Y, Yin Z, Zhu Y, Xin F, Zhang H, et al. Impact of proinflammatory epicardial adipose tissue and differentially enhanced autonomic remodeling on human atrial fibrillation. J Thorac Cardiovasc Surg. (2023) 165:e158–74. doi: 10.1016/j.jtcvs.2022.03.013
103. Wei J, Zhang Y, Li Z, Wang X, Chen L, Du J, et al. GCH1 Attenuates cardiac autonomic nervous remodeling in canines with atrial-tachypacing via tetrahydrobiopterin pathway regulated by microRNA-206. Pacing Clin Electrophysiol. (2018) 41:459–71. doi: 10.1111/pace.13289
104. Li Z, Wang X, Wang W, Du J, Wei J, Zhang Y, et al. Altered long non-coding RNA expression profile in rabbit atria with atrial fibrillation: tCONS_00075467 modulates atrial electrical remodeling by sponging miR-328 to regulate CACNA1C. J Mol Cell Cardiol. (2017) 108:73–85. doi: 10.1016/j.yjmcc.2017.05.009
105. Hao H, Dai C, Han X, Li Y. A novel therapeutic strategy for alleviating atrial remodeling by targeting exosomal miRNAs in atrial fibrillation. Biochim Biophys Acta Mol Cell Res. (2022) 1869:119365. doi: 10.1016/j.bbamcr.2022.119365
106. Kang JY, Mun D, Kim H, Yun N, Joung B. Serum exosomal long noncoding RNAs as a diagnostic biomarker for atrial fibrillation. Heart Rhythm. (2022) 19:1450–8. doi: 10.1016/j.hrthm.2022.05.033
107. Wei Z, Bing Z, Shaohuan Q, Yanran W, Shuo S, Bi T, et al. Expression of miRNAs in plasma exosomes derived from patients with atrial fibrillation. Clin Cardiol. (2020) 43:1450–9. doi: 10.1002/clc.23461
108. Wang S, Min J, Yu Y, Yin L, Wang Q, Shen H, et al. Differentially expressed miRNAs in circulating exosomes between atrial fibrillation and sinus rhythm. J Thorac Dis. (2019) 11:4337–48. doi: 10.21037/jtd.2019.09.50
109. Mun D, Kim H, Kang JY, Yun N, Youn YN, Joung B. Small extracellular vesicles derived from patients with persistent atrial fibrillation exacerbate arrhythmogenesis via miR-30a-5p. Clin Sci (Lond). (2022) 136:621–37. doi: 10.1042/CS20211141
110. Zhu P, Li H, Zhang A, Li Z, Zhang Y, Ren M, et al. MicroRNAs sequencing of plasma exosomes derived from patients with atrial fibrillation: miR-124-3p promotes cardiac fibroblast activation and proliferation by regulating AXIN1. J Physiol Biochem. (2022) 78:85–98. doi: 10.1007/s13105-021-00842-9
Keywords: atrial fibrillation, ncRNAs, biomarker, diagnosis, exosome
Citation: Xue Z, Zhu J, Liu J, Wang L and Ding J (2023) Research progress of non-coding RNA in atrial fibrillation. Front. Cardiovasc. Med. 10:1210762. doi: 10.3389/fcvm.2023.1210762
Received: 28 April 2023; Accepted: 27 June 2023;
Published: 14 July 2023.
Edited by:
Daniel M. Johnson, The Open University, United KingdomReviewed by:
Miron Sopic, University of Belgrade, Serbia© 2023 Xue, Zhu, Liu, Wang and Ding. This is an open-access article distributed under the terms of the Creative Commons Attribution License (CC BY). The use, distribution or reproduction in other forums is permitted, provided the original author(s) and the copyright owner(s) are credited and that the original publication in this journal is cited, in accordance with accepted academic practice. No use, distribution or reproduction is permitted which does not comply with these terms.
*Correspondence: Jijun Ding 732388316@qq.com