Evaluation of the in vitro antioxidant and antitumor activity of hydroalcoholic extract from Jatropha mollissima leaves in Wistar rats
- 1College of Marine Science and Biological Engineering, Qingdao University of Science and Technology, Qingdao, China
- 2Key Laboratory of Marine Drugs, The Ministry of Education, School of Medicine and Pharmacy, Ocean University of China, Qingdao, China
- 3Fatima Tu Zahara Department of Life Sciences, Muhammad Institute of Medical and Allied Sciences, Multan, Pakistan
- 4Department of Pharmacy, MNS University of Agriculture, Multan, Pakistan
- 5The Affiliated Qingdao Central Hospital of Qingdao University, Qingdao, China
Introduction: Despite modern sciences and advancements in new drugs or chemicals, the new era now rushes natural remedies for various illnesses and diseases that lead to end organ damage. In this study, we investigated Jatropha mollissima ethanolic extract’s effect against doxorubicin-induced cardiotoxicity and renal toxicity.
Methods: To determine phytochemicals, a phytochemical screening was conducted. Various assays were used to measure the antioxidant activity, including the DPPH (2,2-diphenylpicrylhydrazyl), SOD (superoxide dismutase), NO (nitric oxide), and others. The antiproliferative effect of Jm was assessed by MTT assay; morphological analysis was performed using an inverted and phase contrast microscope, ultra morphological analysis of apoptosis with acridine orange (AO)/propidium iodide (PI) staining.
Results: It was seen that doxorubicin caused elevated serum markers and abnormal changes in histological patterns. The significant reduction in cardiac and renal marker levels seen in groups given either 400 or 600 mg/kg of crude extract demonstrates that Jm has a protective effect against doxorubicin-induced cardiotoxicity due to the presence of active phytoconstituents having antioxidant potential. There is a dose-dependent decrease in cell viability when using J. mollissima. Apoptosis was observed in the treated cells.
Conclusion: In conclusion, our research lends credence to the idea that J. mollissima could be used for cancer management and have cardioprotective and nephroprotective effects.
1 Introduction
Doxorubicin, a type of anthracycline, was first isolated in the 1960s from the pigment-producing bacterium Streptomyces peucetius. Doxorubicin works by inhibiting topoisomerase II, which increases the amount of cleavable enzyme-DNA linked complexes during DNA replication and thus blocks the ligation of nucleotide strands after double-strand breaks. Moreover, it inhibits protein synthesis and prevents DNA replication by intercalating between base pairs in the DNA helix. Anthracycline is the most effective anti-cancer drug used for the treatment of breast, lung, gastric, ovarian cancer, pediatric cancers, hematologic malignancies, soft tissue sarcomas, Wilms’ tumor, neuroblastoma, bone sarcomas, transitional cell bladder carcinoma, bronchogenic carcinoma, and thyroid carcinoma (Kirstein, 2009). The major toxicity caused by doxorubicin includes cardiomyopathy, renal toxicity, hepatotoxicity (Ghigo et al., 2016).
Multiple mechanisms of doxorubicin produce cardiotoxicity; however, the exact mechanism is still controversial, which includes the formation of ROS occurs due to oxidative stress by Cytochrome P450 reductase, which causes a reduction of the quinone form of doxorubicin into free radical semiquinone form in mitochondrial cells. These semi-quinine forms are rapidly oxidized into quinine by producing superoxide anions, which convert into oxygen and generate hydrogen peroxide, which turns into superoxide free radicals by ferrous ions. This superoxide-free radical build up in myocytes, causing lipid peroxidation, which destroys the mitochondrial membrane, endoplasmic reticulum, and nucleic acid. Mitochondrial cells are abundantly present in cardiac cells and more susceptible to free radical toxicity (Salazar-Mendiguchía et al., 2014), inhibition of synthesis of macromolecules is caused by DNA intercalation (Outomuro et al., 2007), DNA alkylation, DNA cross-linking, Protein and nucleic acid synthesis are stifled, expression of several vasoactive amines, including prostaglandins, histamine, etc., Transmission of adenosine triphosphate (ATP) production within cardiac cells results from adenylate cyclase activity alterations. Doxorubicin also causes elevated levels of nitric oxide (NO) by stimulation of endothelial NOS (eNOS) expression and superoxide formation by enhancing the activity of endothelial proteins. The eNOS has a key role in cardiotoxicity by initiating ROS apoptosis of endothelium, calcium overload in ventricular myocardium, induction of apoptosis causing DNA fragmentation and cell shrinkage by inhibiting RNA polymerases and oxidative damage, lysosomal changes, alteration in Na+/K+ adenosine triphosphate (ATPase) pump and Ca2+ ATPase pump (Ruggeri et al., 2018) and Myocytes suffer iron-dependent oxidative damage due to the formation of semiquinone metabolites that cause the delocalization of Fe (II) from ferritin, leading to the production of hydrogen peroxide, which in turn sustains the generation of hydroxyl radicals and lipid peroxidation (Ma et al., 2013).
Doxorubicin-producing free radicals have a more damaging effect on the heart due to fewer antioxidants like superoxide dismutase, glutathione, and catalase than other organs like the kidney and liver. However, it causes damaging effects on kidney and liver but to a lesser extent than the heart because the heart contains cardiolipin. Mitochondrial membrane phospholipids with a high affinity for DOX accumulate in cardiac cells (Yancy et al., 2013).
Doxorubicin damages the kidney, causing lipid peroxidation and generation of ROS (reactive oxygen species), which causes activation of NF-KB, thus stimulating nitric oxide and TNF-α which causes nephrotoxicity, increase in hydroxyproline in the kidney and increases protein level in the urine (Chen et al., 2016). Lipid peroxidation and kidney damage are caused by the byproducts of NADPH-Cytochrome P-450’s conversion of doxorubicin to the semiquinone free radical, which in turn generates hydroxyl radical and superoxide anion (Nagai et al., 2018). Renal damage was also reported due to doxorubicin, which causes iron-dependent oxidative damage to renal cells by forming semiquinone metabolites that delocalize Fe (II) from ferritin that produce hydrogen peroxide and form hydroxyl radicals and lipid peroxidation and elevated levels of serum albumin, serum creatinine, and BUN. Previous studies also reported high MDA levels (Ahmed et al., 2019).
Given the rich diversity of plant life in Pakistan, this study set out to identify medicinal plants with cardioprotective and nephroprotective properties. Herbal medications are among the natural chemicals being studied by scientists because of their less negative effects. These plants can potentially provide low-cost, high-yield therapeutic options for the conditions mentioned above (Hosseini and Sahebkar, 2017). Cancer is a leading cause of death worldwide. Traditional therapy based on plant extracts has received renewed interest in the fight against cancer after being neglected for centuries. The wide variety of phytochemical substances found in plants and their relatively few side effects have led to a renewed interest in their application in cancer prevention and treatment in recent years (Kelloff, 1999).
In traditional medicine, the plant species Jatropha mollissima (Euphorbiaceae) is used for a wide variety of purposes, including as an anti-inflammatory remedy (de Queiroz Neto et al., 2019), antibacterial treatment (Bastos-Cavalcante et al., 2020), antioxidant protection (Dias et al., 2019), anthelmintic action (Abogmaza et al., 2020), kidney protection (Iqbal and Yahya, 2021; Omer Iqbal et al., 2021), liver protection (Iqbal et al., 2022), heart protection (Iqbal et al., 2021), and appetite suppression (de Albuquerque et al., 2007). The significance of J. mollissima in counteracting anxiety and depression has not been studied, despite the many research documenting the plant’s beneficial effects on health. The purpose of this research is to determine if J. mollissima has in vitro antioxidant and antitumor activity.
2 Methodology
2.1 Plant collection
Jatropha mollissima was purchased fresh from Multan, Pakistan. Bahauddin Zakariya University’s Botany Department Taxonomists validated the species and assigned a voucher number (R.R. Stewart F.W. Pak.725/16) for future reference.
2.2 Preparation of crude extract
Separated J. mollissima plants were sun-dried for 15 days before ground into a powder in a blender. It was first ground using an electric grinder before being submerged in a mixture of 70% ethanol and 30% distilled water for nine period of 3 days while being periodically shaken and agitated. Muslin fabric was used for preliminary coarse filtering before Whatman filter paper (NO.1) was used for final polishing.
The filtrate was then evaporated in a rotary evaporator (Heidolph Laborota 4000 efficient, Germany) at 30°C–40°C under vacuum. The filtrate was dried in an incubator until it reached a semi-solid consistency, then placed in a refrigerator (−40°C) for later use.
2.3 Chemicals and reagents and cell line
Doxorubicin, formalin, ketamine (Indus Pharma Lahore), xylazine (Prix Pharmaceutical Lahore), and ether were all utilized as analytical grade chemicals in scientific studies. DPPH, ferric chloride, 2-deoxyribose, NADH, nitroblue tetrazolium (NBT), and phenazine methosulfate (PMS) 3-(4,5-dimethythiazol-2-yl)-2,5-diphenyl tetrazolium bromide (MTT), were purchased from Sigma-Aldrich; Merck KGaA, respectively. The hepatocellular carcinoma (HepG2) cell line was used in this study.
2.4 Experimental animals and conditions
Male Wistar Albino rats weighing between 250 and 320 g were purchased from the animal house at the Muhammad Institute of Medical Allied Sciences in Multan. In the Pharmacology Research Laboratory at the Muhammad Institute of Medical and Allied Health Sciences, the animals were kept in polycarbonate cages with raw dust that was changed every 3 days in a standard laboratory setting (27°C ± 2°C). The rats had unlimited access to water and standard diet pallets. According to the National Research Council (Touitou et al., 2004), the research projects were approved by the Muhammad Institute of Medical and Allied Science’s Ethical Committee in Multan, Pakistan. A valid MIMAS/07-21/25261 authorization number was provided.
2.5 Study design
Twenty-four rats of the Wistar strain were split into four groups of six.
The first group (the control group) received saline solution without any additives.
Doxorubicin was administered to a group designated as “Group 2.”
Group 3 was given Doxo + Jm extract (400 mg/kg).
Group 4 was given Doxo + given (600 mg/kg) of Jm extract.
The first group (the control group) received saline solution 4 mL/kg for 21 days via nasal gastric tubing. Group 2 was the Doxorubicin group intoxicated with doxorubicin 10 mg/kg given intraperitoneal single bolus dose and N/S 4 mL/kg for 21 days via gastric tubing. Groups 3 and 4 received Jm extract treatments of 400 and 600 mg/kg, respectively, orally for 21 days, and on first day these groups received intraperitoneal injections of doxorubicin.
2.6 Preliminary phytochemical evaluation
Aqueous-ethanolic (30:70) extract of J. mollissima was evaluated for the possible presence of vital phytochemical classes using standard protocol (Ain, 2022).
2.7 Antioxidant activity
Antioxidant activity was performed using 2,2-diphenylpicrylhydrazyl (DPPH) and Nitric oxide (NO) assay, SOD and peroxide.
2.7.1 2,2-diphenylpicrylhydrazyl (DPPH) Assay
As mentioned earlier, the DPPH test has been carried out (Mensor et al., 2001). The diluted sample with ethanol was mixed with an aqueous-ethanolic extract (30:70) from J. mollissima to make a final volume of 5 mL with different concentrations (4 mL). Then, for 40 min, this mixture was stored in the dark. The stated solution’s 517 nm absorbance was measured using a spectrophotometer. Each study was done three times, and the percentage of inhibition in ascorbic acid equivalency was measured. Equation below was used to compute the percentage of DPPH scavenging potential:
2.7.2 Measurement of nitric oxide (NOx) scavenging capacity
J. mollissima was extracted using an aqueous-ethanolic solution at a concentration of 10 mg/mL. Standard (Ascorbic acid) and extract were both serially diluted with distilled water to achieve concentrations of 1,000 and 2,000 μg/mL. Experiment solutions were stored at a constant 4°C. A freshly made Griess reagent was utilised in the process. To determine the optimal concentration of extract (1,000 and 2,000 g/mL), 0.5 mL of 10 mM sodium nitroprusside in phosphate-buffered saline was added to 1 mL of each extract concentration and incubated at 25°C for 3 h. The extract was spiked with a freshly made Griess reagent of the same volume. The extracts were left out of the control samples, but the volume of buffer used was kept constant. There were adequate quantities of extracts in the various hued tubes, but no sodium nitroprusside. A 96-well plate was loaded with 150 L of the reaction mixture. Based on our prior correspondence (Khan et al., 2021a; Khan et al., 2021b; Khan et al., 2022), we used a UV-Vis microplate reader (Alibaba, Hangzhou, China) to measure the absorbance at 546 nm. Using the following formula, we were able to determine the percentage of nitrite radical scavenging activity of extracts and ascorbic acid, as well as the percentage of inhibition by the standard.
2.7.3 Peroxide radical scavenging assay
To conduct our experiments, we adapted a previously published method (Ruch, Cheng and Klaunig, 1989), preparing extract and standard solutions at two different concentrations (1,000 and 2,000 μg/mL), as well as a 43 mM H2O2 solution in a 0.1 M phosphate buffer (pH 7.4). After combining the sample solutions with the 3.4 mL of phosphate buffer, we added the H2O2 solution (43 mM) at a volume of 0.6 mL. A UV spectrophotometer was used to measure the absorbance at 230 nm. A sodium phosphate buffer devoid of hydrogen peroxide served as a blank. The following formula was used to determine the scavenging efficiency (in percentage terms) of H2O2:
2.7.4 Reducing power scavenging assay
The ability to reduce iron (III) is often used as a proxy for phenolic antioxidant activity (Ebrahimzadeh et al., 2010). The extracts’ reducing potential was determined using a method similar to that proposed by (Yen and Chen, 1995). Each extract was diluted in water to a concentration of between 1,000 and 2,000 μg/mL, then combined with potassium ferricyanide [K3Fe(CN)6] (2.5 mL, 1% concentration) and phosphate buffer (2.5 mL, 0.2 M, pH 6.6). The combination was simmered at 50°C for 20 min. Centrifuging at 3,000 rpm for 10 min and then adding 2.5 mL of 10% trichloroacetic acid interrupted the process. Two and a half millilitres of the upper solution layer were mixed with two and a half millilitres of distilled water and 0.5 mL of FeCl3 (0.1%) to determine the absorbance at 700 nm. The reaction mixture’s absorbance went up, showing that the reducing power had increased. Ascorbic acid was used as a good proxy for a positive control.
2.7.5 Superoxide dismutase assay
Using a slightly altered version of the method reported by (Gülçin et al., 2004), the herb’s capacity to scavenge superoxide anion radicals was determined. The PMS, NADH, and NBT system was applied for the generation of superoxide radicals. We put 50 µL of the tested materials at different concentrations, along with 125 µL of NBT (300 M) and 125 µL of NADH (468 M) in a test tube containing 625 µL of Tris-HCl buffer (16 mM, pH 8.0). The reaction began after 125 µL (60 mM) of PMS was added to the mixture. Five minutes of room temperature incubation was followed by a vigorous vortexing of the mixture. And finally, the absorbance was measured with a spectrophotometer (Hitachi, U-1900 UV/VIS, Hitachi High-Technologies Corporation).
2.8 Anticancer activity
2.8.1 Principle of MTT assay
The cell viability of plant extracts can be measured with a colorimetric assay using 3-(4,5-dimethythiazol-2-yl)-2,5-diphenyl tetrazolium bromide (MTT). Only viable cells with active mitochondrial enzymes can be utilized in this method to determine the cytotoxicity of a plant extract. The mitochondrial enzyme mitochondrial reductase contributes to this method. The formation of formazan causes the yellow MTT reagents to change hue to a deep blue during the reaction. Toxicity to cells, disruption of metabolic maturation, and a diminished bioassay presence are all components of this complex. Mitochondrial reductase is the enzyme that converts the yellow MTT reagent into the purple formazan (Mosmann, 1983). Afterward, the formazan was mixed with the DMSO and the ethanol. The MTT assay was chosen as the cell viability assay due to its low cost and rapid turnaround time.
2.8.1.1 Diluting herbal extracts
The plant extract was dissolved in DMSO or water depending on the user’s preference. Every one of the 100 mM stock solutions was frozen at −20°C until use. After being combined with a DMEM medium, the pH levels of these solutions were found to be between 7 and 8.
2.8.1.2 Protocol for the MTT assay
The cells were seeded at a density of 1 × 105 cells/mL in 100 ul complete growth medium in a 96-well plate and incubated for 24 h at 37°C and 5% CO2. 100 ul of 1:20 diluted extracts were used to treat the cells at concentrations of 0 (Vehicle control), 100, 200, 300, and 400 ug/mL after incubation at 37°C with 5% carbon dioxide for 4, 24, and 48 h. After 24 h, the 96-well plates were foil-wrapped to maintain the MTT’s sensitivity to light, and 10 ul of yellow MTT reagent from a 5 mg/mL stock solution was added to each well. After that, the plates were incubated for another two to 4 hours at 37°C and 5% carbon dioxide. The precipitated crystals were entirely dissolved in 100ul of DMSO. To determine the absorbance of the samples in the microplates, a microplate reader (Tecan Infinite® M200) was used. There were three independent trials of the experiments done.
2.9 Cell morphology analysis
Under an inverted and phase contrast microscope, we observed morphological changes in HepG2 cells treated with 25 ug/mL of Jm after 24, 48, and 72 h of incubation (Leica, Wetzlar, Germany).
2.10 Ultra morphological analysis of apoptosis with acridine orange (AO)/propidium iodide (PI) staining
Fluorescence microscopy was used for analysis after standard protocols for viewing cells with AO and PI had been performed. (Leica coupled with Q-Floro Software; Leica Microsystems, Wetzlar, Germany). About 1 × 106 cells/mL were seeded into a 25 mL culture flask, and the cells were subsequently treated to 6 g/mL of Jm for 48 h. The cells were centrifuged at 200 g for 5 min to remove the medium. Before being stained with AO/PI, the cells were given two cold washes in phosphate-buffered saline (PBS). The cells on the glass slide were then treated with freshly produced AO/PI at a 10 g/mL concentration. The slides were analyzed around 30 min before the fluorescence faded by using fluorescence microscopy.
2.11 Annexin V and propidium iodide staining
Six-well microtiter plates were used to cultivate the cells (at a concentration of 105–106 cells/mL) in serum-free conditions for 24 h. The following 24, 48, and 72 h, they were administered escalating doses of Jm. Annexin V/propidium iodide (PI) staining was used to distinguish between apoptotic, late apoptotic, and non-apoptotic cells at the specified timeframes. To stain for PI and annexin V-FITC, cells were washed twice in ice-cold PBS and then suspended in 100 L of Annexin V binding buffer (0.1 MHepes/NaOH (pH 7.4), 1.4M NaCl, 25 mM CaCl2) for 15 min. FACS analysis was performed on a FACS Calibur flow cytometer using Cell Quest software (BD Biosciences, San Jose, CA, United States) to ascertain cell viability.
2.12 Collection of metabolic data and blood sample
Urine samples were taken from rats on days 0 and 10, and 21 while they were housed in metabolic cages with free access to running water. Urine volume and water consumption were recorded. The sodium and potassium concentrations in the urine and the urine production rate were measured.
Blood samples were also taken on the 0, 10th and 21st days to measure CK-MB, LDH, Troponin I, serum sodium, and potassium level by collecting blood by the retro-orbital method.
On day 21, after fasting for 12 h, we weighed all the animals and put them to sleep with a mixture of xylazine and ketamine (1:10). Heart puncture was used to draw blood and clot it for testing. Centrifugation at 3,000 rpm for 15 min was used to separate the serum.
We washed the heart in normal saline to get rid of the blood, then put it in formalin for later histopathological examination.
2.13 Biochemical parameters in serum for cardiotoxicity
Serum concentrations of creatinine kinase-MB (CK-MB), Troponin-I, and lactate dehydrogenase (LDH) were estimated using commercially available standard enzymatic kits. Serum electrolytes like serum sodium and serum potassium were also measured.
2.13.1 Calculation of CK-MB
CK-MB (Creatine kinase myocardial band) is a very sensitive cardiac biomarker used to detect any cardiac damage due to any toxic drug or myocardial infarction.
CK-MB assay kits are used for determining its values in the blood. SBio assay kit measured CK-MB levels in the animals intoxicated by doxorubicin.
A blood sample was collected at 0, 10th, and 21st day and allowed to stand for 20 min. Then, it was centrifuged to obtain serum and analyzed using a UV spectrophotometer at 340 nm using the following procedure.
The assay required 1 mL of working reagent, which was heated to 37 °C for 1 min, followed by adding 0.05 mL of sample. After 10 min of blending, check the initial absorbance (A0) and take additional readings at 1-, 2-, and 3-min intervals. Please use the following formula to determine the average absorbance rate of change (ΔA/min)
2.13.2 Measurement of LDH in serum
LDH (Lactate dehydrogenase) was measured using a Human kit in which a blood sample was collected at 0, 10th, and 21st day and allowed to clot for 20 min. Then, this clotted blood was centrifuged in the centrifugation machine for 15 min at 3,000 rpm. The serum layer was separated from the blood and was collected. The serum was analyzed in a UV spectrophotometer at 340 nm using the following procedure.
The average absorbance variation per minute (ΔA/min) is used to derive absorbance readings. Multiplying the sample’s ΔA/min by 8,095 yields a measure of LDH activity.
where X is the LDH value and is measured in triplicate.
2.13.3 Estimation of serum potassium and sodium levels
Electrolyte profiles screen electrolytes and acid-base imbalances and monitor treatment efficacy. When diagnosing a medical issue, electrolytes like sodium, potassium, chloride, and bicarbonate may be indicators (Iqbal and Yahya, 2021; Omer Iqbal et al., 2021).
Serum samples were analyzed using a flame photometer to determine sodium and potassium concentrations (Sherwood Model 410, United Kingdom).
Samples were diluted 1:200 for the measurement of sodium and potassium in serum; The entire process was carried out twice to ensure its integrity.
2.14 Statistical analysis of data
Data analysis and an indication of statistical significance between groups in an experiment were conducted using analysis of variance (ANOVA) using one-way analytical variance and Bonferroni’s all-mean post hoc test. To analyze the information, Graph Pad Prism was used. Results were deemed to be statistically significant when the p-value was less than 0.05.
3 Results
This research was carried out to ascertain whether or not J. mollissima may mitigate the cardiotoxicity and nephrotoxicity caused by doxorubicin. 70% aqueous ethanolic extract of J. mollissima (Jm) was prepared and used for experimental purposes.
3.1 Preliminary phytochemical evaluation and antioxidant assays
A phytochemical analysis of J. mollissima hydroalcoholic extract revealed a rich profile of bioactive components (Table 1). In a number of different antioxidant studies, the 1,000 g/mL concentration of Jm hydroalcoholic extract showed the greatest DPPH level (87.80%) and the highest reducing power (73.24%); NO (Nitric oxide), H2O2 (hydrogen peroxide), and SOD (superoxide dismutase) showed 92.12, 89.86, and 80.40% inhibition, respectively. Table 2 lists some of the many tests that have been used to assess antioxidant activity.

TABLE 2. Results from multiple assays examining Jatropha mollissima plant extract for antioxidant activity.
3.2 Protective effect of Jatropha mollissima (Jm) against doxorubicin-induced cardiotoxicity and nephrotoxicity
Doxorubicin is an anthracycline antibiotic used for various types of cancers like breast, lung, gastric, ovarian cancer, pediatric cancers, hematologic malignancies, and soft tissue sarcomas, Wilms’ tumor, neuroblastoma, bone sarcomas, transitional cell bladder carcinoma, bronchogenic carcinoma, and thyroid carcinoma. The use of anthracyclines is limited due to their toxic effects. Several mechanisms are proposed through which it causes toxicity. It may generate free radical formation by NADPH-dependent reductases, which form anthracycline semiquinone free radicals by reducing anthracyclines, causing apoptosis in cardiac cells (Kirstein, 2009; Ghigo et al., 2016).
Doxorubicin (Doxo) interacts with mitochondrial enzymes to generate reactive oxygen species (ROS), which in turn cause lipid peroxidation and DNA damage; DNA binding; DNA alkylation; inhibition of DNA unwinding, strand separation, and helicase activity; inhibition of enzyme topoisomerase II, which results in DNA damage and apoptosis; and membrane damage; in the presence of iron, doxorubicin forms a complex with it to bind with DNA to generate reduced They are not only toxic to the heart but also to the kidneys and liver (Outomuro et al., 2007; Salazar-Mendiguchía et al., 2014).
3.2.1 Effect of Jm on body weights of animals
The weight of the animals was recorded on days 0 and 10th, and 21st. During the 21-day experiment, the animals received intraperitoneal injections of 10 mg/kg of doxorubicin on day 1. The groups had no statistically significant difference in day 0 body weight (all p > 0.05). Doxorubicin-treated rats lost weight throughout the study compared to their weight on day zero (all p < 0.05). Weight increases were observed across all Jm-treated groups, particularly those given 400 and 600 mg/kg (all p < 0.05) as shown in Figure 1.
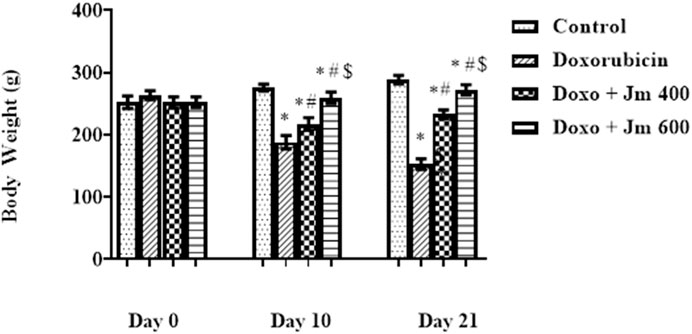
FIGURE 1. Body weight of normal control, doxorubicin, Dox + Jm 400, and Dox + Jm 600. Results are deemed to be statistically significant (*) if p is less than 0.005. For each day, a * denotes statistical significance when compared to the control group, # to Doxorubicin, and $ to Dox + Jm 400.
In conclusion, both the control and Jm groups of rats had a rise in body weight. Contrary to this, Dox-treated groups showed a decreasing body weight pattern due to decreased appetite and less food intake. In contrast, body weight was significantly increased in animals treated with Jm intoxicated with Doxo.
3.2.2 Heart weight
Heart weight was found to be considerably lower in doxorubicin-treated rats relative to the control group. Co-administered different doses of J. mollissima extracts, i.e., 400 and 600 mg/kg with doxorubicin, caused a slight increase in heart weight, respectively as shown in Table 3.
3.3 Effect of Jm on cardiac marker enzymes
3.3.1 Effect of Jm on serum creatine kinase (CK-MB)
Creatine kinase is categorized into three types: CK-BB, CK-MB, and CK- form, which is specific for the brain, heart, and muscle form, respectively. The heart is sensitive to the reactive oxygen species due to fewer anti-oxidant enzymes like superoxide dismutase, catalase, and glutathione, which are protective enzymes in the heart. Cardiolipin is a phospholipid found in the mitochondrial membrane of heart cells, and doxorubicin binds to it quite strongly (El-Sayed et al., 2011).
Serum levels of cardiac marker enzyme CK-MB increased dramatically in Doxorubicin-treated rats compared to control (all p < 0.05) 21 days after administration of a single bolus dose of doxorubicin (10 mg/kg) (all p > 0.05). Serum levels decreased (all p < 0.05) in response to Jm administration in the 400 mg/kg and 600 mg/kg treatment groups, as depicted in the Figure 2 below.
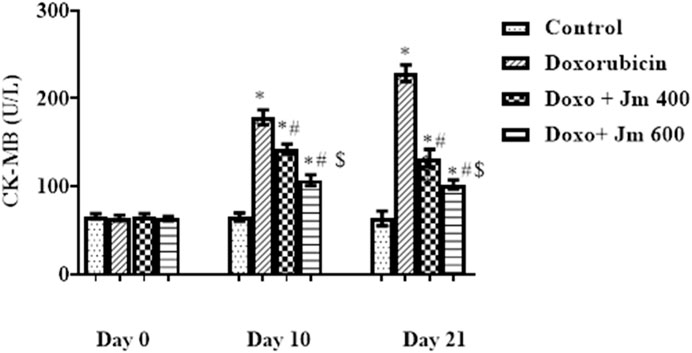
FIGURE 2. Serum CK-MB levels of normal control, doxorubicin, Dox + Jm 400, and Dox + Jm 600. Results are deemed to be statistically significant (*) if p is less than 0.005. For each day, a * denotes statistical significance when compared to the control group, # to Doxorubicin, and $ to Dox + Jm 400.
3.3.2 Effect of Jm on serum lactate dehydrogenase (LDH)
Lactate dehydrogenase is a biomarker for heart muscle damage present in two isoforms, i.e., H form is specified for the heart while M is specific for muscles. Increased level of LDH indicates heart damage. It is a quantitative index of cell integrity (Giri et al., 2004).
Serum levels of LDH were increased dramatically in Doxorubicin-treated rats compared to control (all p < 0.05) 21 days after administration of a single bolus dose of doxorubicin (10 mg/kg) (all p > 0.05). Serum levels of LDH are decreased (all p < 0.05) in response to Jm administration in both the 400 mg/kg and 600 mg/kg treatment groups, as depicted in the Figure 3 below.
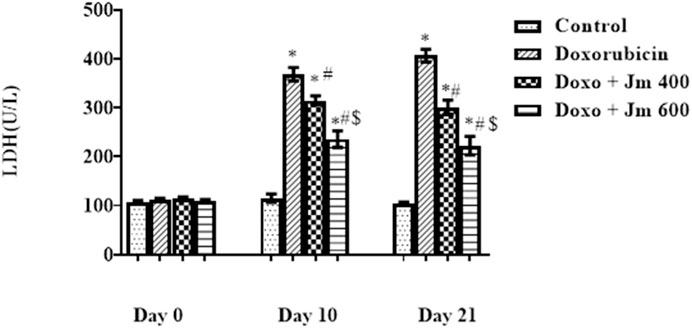
FIGURE 3. Serum LDH levels of normal control, doxorubicin, Dox + Jm 400 and Dox + Jm 600. Results are deemed to be statistically significant (*) if p is less than 0.005. For each day, * denotes statistical significance when compared to the control group, # to Doxorubicin, and $ to Dox + Jm 400.
3.3.3 Effect of Jm on troponin I (cTnI)
Troponin is a very sensitive parameter to detect any myocardial damage. It comprises three proteins—troponin I, C, and T—along the muscle’s thin filament. Troponin I provides a longer duration for detecting myocardial injury, i.e., 6–10 days, compared to troponin C and T, which exits only for 4–6 h (Rashid et al., 2013).
Levels of Troponin I were increased dramatically in Doxorubicin-treated rats compared to control (all p < 0.05) 21 days after administration of a single bolus dose of doxorubicin (10 mg/kg) (all p > 0.05). Troponin I (cTnI) levels are decreased (all p < 0.05) in response to Jm administration in both the 400 mg/kg and 600 mg/kg treatment groups, as depicted in the Figure 4 below.
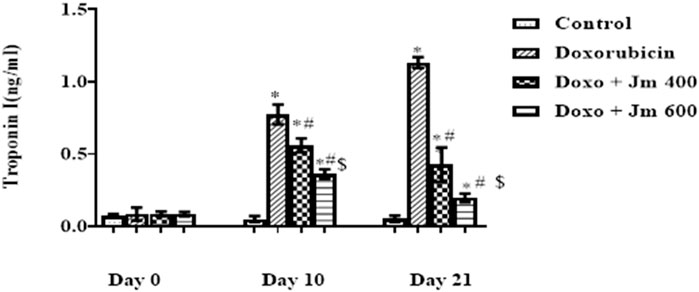
FIGURE 4. Serum Troponin I (cTnI) levels of normal control, doxorubicin, Dox + Jm 400 and Dox + Jm 600. Results are deemed to be statistically significant (*) if p is less than 0.005. For each day, a * denotes statistical significance when compared to the control group, # to Doxorubicin, and $ to Dox + Jm 400.
3.3.4 The implication of Jm in sodium concentration in blood
The sodium concentration was determined by centrifuging blood at 3,000 rpm for 15 min to separate serum. The sample was diluted 1:200 and examined by a flame photometer. Increases in serum sodium were seen in the doxorubicin-treated group compared to the control group after a 10 mg/kg dosage on day 1. A small but statistically significant sodium decrease between groups gave 400 and 600 mg kg of Jm (all p < 0.05) as shown in Figure 5.
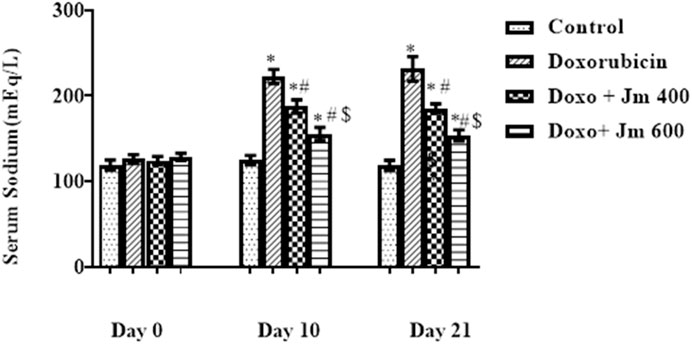
FIGURE 5. Serum sodium levels of normal control, doxorubicin, Dox + Jm 400, and Dox + Jm 600. Results are deemed to be statistically significant (*) if p is less than 0.005. For each day, a * denotes statistical significance when compared to the control group, # to Doxorubicin, and $ to Dox + Jm 400.
3.3.5 The implication of Jm in potassium concentration in blood
The potassium concentration was determined by centrifuging blood at 3,000 rpm for 15 min to separate serum. It was then analyzed by using a flame photometer. Serum potassium levels were lower in the group that received a single dose of doxorubicin (10 mg/kg) on day one compared to the normal control group (all p > 0.05). In contrast, there is a modest rise in sodium levels in groups given Jm at doses of, i.e., 400 and 600 mg kg (all p < 0.05), as depicted in Figure 6.
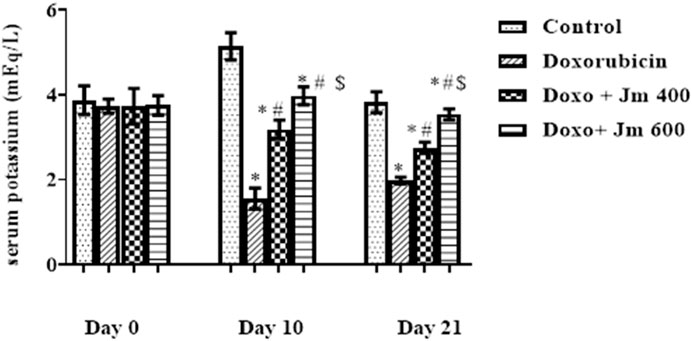
FIGURE 6. Serum potassium levels of normal control, doxorubicin, Dox + Jm 400, and Dox + Jm 600. Results are deemed to be statistically significant (*) if p is less than 0.005. For each day, a * denotes statistical significance when compared to the control group, # to Doxorubicin, and $ to Dox + Jm 400.
3.4 Histopathology
Hearts from treated and untreated rats were histologically examined to detect lesions and damage caused by doxorubicin. And to see if J. mollissima extract can prevent the heart damage caused by doxorubicin as shown in Figure 7.
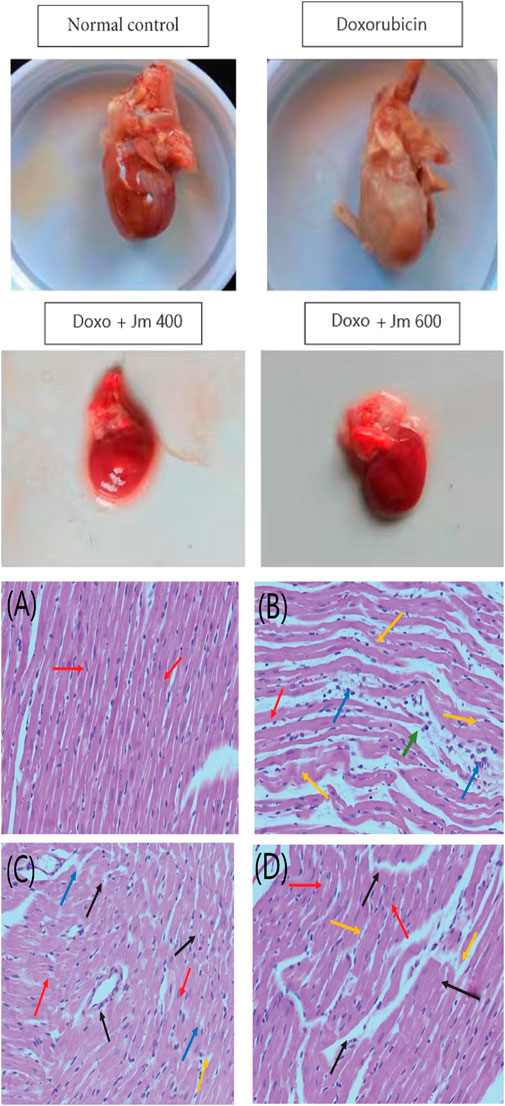
FIGURE 7. Histopathological section of the heart; (A) Control group (B) Doxorubicin (10 mg/kg) (C) Treatment group Doxo + Jm 400 mg/kg (D) Treatment group (Doxo + Jm 600 mg/kg) in doxorubicin-induced cardiotoxicity. The red arrow represents a healthy cardiomyofibril; the green arrow demonstrates perivascular cuffing of the vasa vasorum, which is associated with intimal fibrosis; the orange arrow demonstrates myocytolysis; and the blue arrow demonstrates myonecrosis.
3.5 Jatropha mollissima protective action against the kidney damage caused by doxorubicin
3.5.1 Kidney weight
In animals given doxorubicin, kidney weight increased dramatically compared to controls. Combining extract and doxorubicin led to a moderate decrease in kidney mass as shown in Table 4.
3.5.2 Effect of Jm hydroalcoholic extract on urine output
Rats were placed in metabolic cages for 24 h with access to running water but no food to determine this. After 24 h, the urine volume was measured, and it was shown that the Doxorubicin intoxicated group had much lower urine volume and a darker color than the normal control group. Figure 8 shows that as the study progresses, the Jm-treated and the Doxo-intoxicated groups produced more urine when given Jm at 400 and 600 mg kg (all p < 0.005).
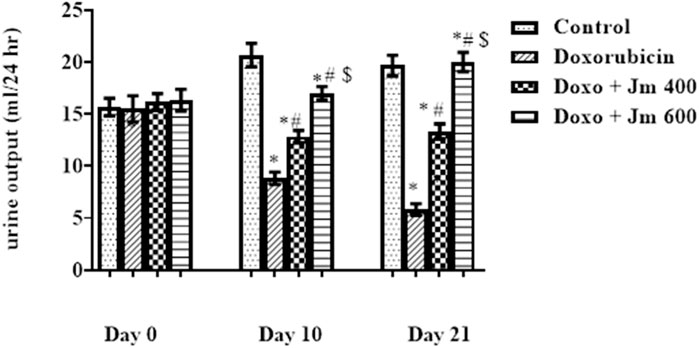
FIGURE 8. Urine output of normal control, doxorubicin, Dox + Jm 400 and Dox + Jm 600. The results are considered significant (*) if p < 0.005. * indicates p < 0.05 vs. normal control, # indicates p < 0.05 vs. Doxorubicin, $ indicates p < 0.05 vs. Dox + Jm 400 on respective days.
3.5.3 Effect of Jm hydroalcoholic extract on urinary sodium levels
For 24 h, rats were given free access to running water and kept in metabolic cages but no food to determine this. After 24 h, urinary sodium was measured, and it was shown that the Doxorubicin intoxicated group had much lower levels of sodium in the urine and a darker color than the normal control group. The Figure 9 shows that as the study progresses, both the Jm-treated and the Doxo-intoxicated groups increase the sodium level in urine when given Jm at doses of 400 and 600 mg kg (all p < 0.005).
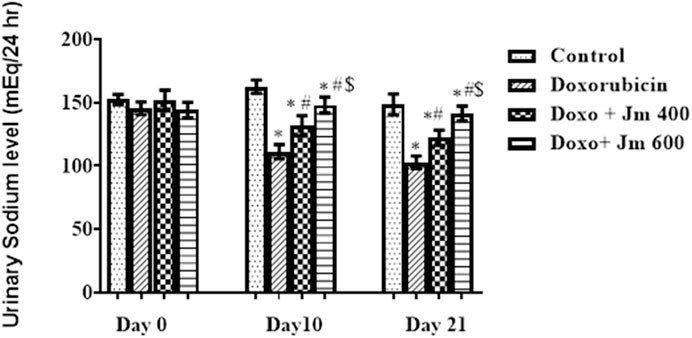
FIGURE 9. Urinary sodium levels of normal control, doxorubicin, Dox + Jm 400 and Dox + Jm 600. The results are considered significant (*) if p < 0.005. * indicates p < 0.05 vs. normal control, # indicates p < 0.05 vs. Doxorubicin, $ indicates p < 0.05 vs. Dox + Jm 400 on respective days.
3.6 Histopathology of kidney
Doxorubicin causes nephrotoxicity, which was confirmed by histopathology as lesions were formed in contrast to the control group. Improvement was observed in slides of groups treated with Jm at 400 and 600 mg/kg doses as shown in Figure 10.
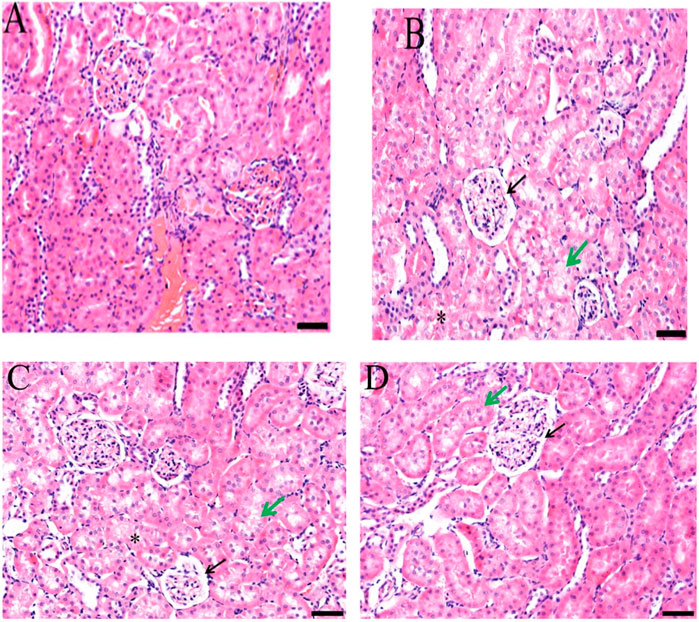
FIGURE 10. Histopathological section of the kidney; (A) Control group (B) Doxorubicin (C) Treatment group (Dox + Jm 400 mg/kg) (D) Treatment group (Dox + Jm 400 mg/kg) in doxorubicin-induced nephrotoxicity. Necrosis atropy detected in epithelial cells of proximal and distal tubule (*); Green arrow indicates widespread oedema, vacuolation, and hydrophic degeneration; Black arrow denotes atrophic glomerulus and extensive capsular space.
3.7 Determination of cell viability by MTT assay
The hepatocellular carcinoma (HepG2) cell line also showed a concentration-dependent response to the plant extracts. The highest concentration of plant extracts (400 g/mL) was shown to inhibit cells to a greater extent than any of the lower doses tested. As shown in the Figure 11, the percentage of cells inhibited rose with the concentration of the extracts. However, the effect on cell proliferation was minimal after 24 h of treatment with doses up to 90 g/mL of crude ethanolic extract in HepG2 cells. Cell viability declined by over 50% at doses higher than 100 μg/mL (Figure 11). Cell viability decreased proportionally after treatment with a different concentration of Jm extract. However, as Figure demonstrates, safe levels for healthy cells with a 90% or above viability are below 90 or 100 μg/mL. This study showed that Jm’s crude ethanolic extract was safe at modest dosages in animal models, while it cautioned against using high doses for an extended period.
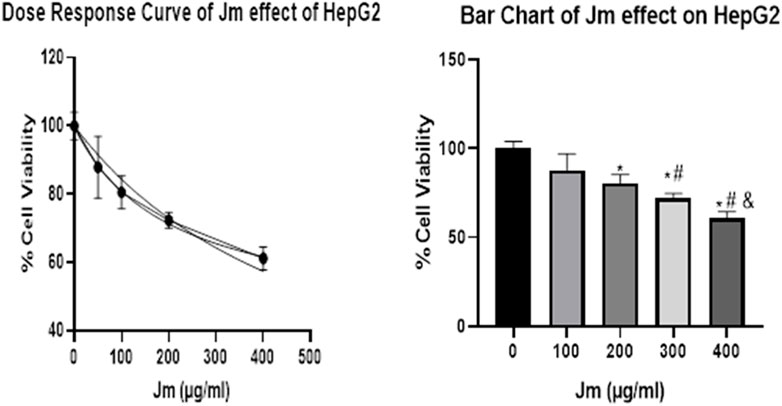
FIGURE 11. Cell Viability Assay. Jatropha mollissima extracts on the HepG2 cell line as a percentage of cell viability by MTT assay (A). Jm’s effect on HepG2 as a function of dose (B) Bar chart of Jm effect on HepG2 results 24 h after treatment at various doses on HepG2. One-way ANOVA was used to make the comparisons between the samples. The results were reported as the mean standard error of the mean (n = 3), with triplicates of each experiment included. **p < 0.01, ***p < 0.001, and ****p < 0.0001 versus the control group.
3.8 Morphological alterations in the HepG2 cell line caused by Jm
Using inverted and phase contrast microscopy, this study compared the morphology of untreated HepG2 hepatocellular carcinoma cell lines to that of HepG2 cells treated with active extracts of J. mollissima after 24, 48, and 72 h. Apoptosis was observed in the treated cells, as evidenced by the production of apoptotic bodies, membrane blebbing, nuclear condensation, and cell shrinkage (as depicted in the Figure 12). The results were stunning when comparing the total number of treated cells to that of untreated cells.
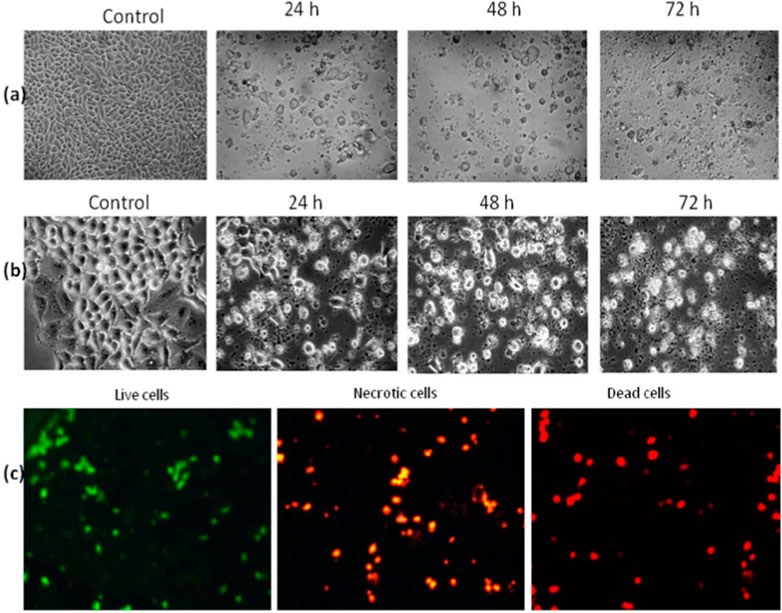
FIGURE 12. Morphological observation: (A) Inverted microscopic observation of HepG2 (B) Phase contrast imaging of HepG2, © AO/PI staining-based fluorescent analysis.
3.9 Morphological alteration in HepG2 cells by Jm by acridine orange (AO)/propidium iodide (PI) staining
The cells were examined in a customary way using AO and PI, and the results were analyzed using fluorescence microscopy. (Leica coupled with Q-Floro Software; Leica Microsystems, Wetzlar, Germany). According to our findings, viable cells were identified as having intact nuclei and being stained with green fluorescence. Apoptotic cells may be distinguished by the orange coloration caused by AO binding to denatured DNA, which revealed nuclear condensation, fragmentation, apoptotic bodies, and cytoplasmic blebbing. Additionally, the binding of AO to denatured DNA results in a crimson color, indicating dead or necrotic cells.
3.10 Staining using annexin V-FITC and propidium iodide
When HepG2 cells are exposed to J. mollissima, a greater proportion enter the early apoptotic phase (as indicated in Figure 13 below) extract compared to untreated cells (p < 0.05). From these results, it can be concluded that Jm can significantly and dose-dependently trigger apoptosis in Hep G2 cells. One of the six diagnostic features of cancer is the avoidance of apoptosis. Additional research on the possible mechanism is required in the future.
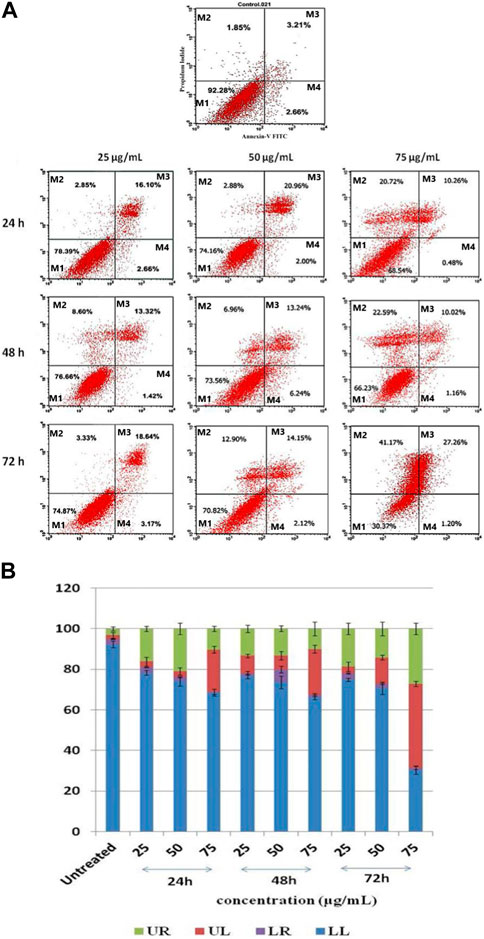
FIGURE 13. (A) Jatropha mollissima extract-induced apoptosis in HepG2 cancer cells as examined by Annexin V-FITC/PI. 25, 50, and 75 g/mL are the corresponding concentrations of Jm. The durations of Jm incubation of the cells were 24, 48, and 72 h. M1 represents viable cells, M2 represents primary necrotic cells, M3 presents late apoptotic cells, and M4 presents apoptosis. The results were reported as the mean standard error of the mean (n = 3), with triplicates of each experiment included. **p < 0.01, ***p < 0.001, and ****p < 0.0001 versus the control group. (B) A histogram showing the percentages of HepG2 cells that have undergone late apoptosis (UR), primary necrosis (UL), apoptosis (LR), and viable cells (LL).
4 Discussion
Doxorubicin, an anthracycline antibiotic, is useful in treating a wide variety of cancers, such as breast, lung, gastric, ovarian cancer, pediatric cancers, hematologic malignancies, and soft tissue sarcomas, Wilms’ tumor, neuroblastoma, bone sarcomas. However, it has limited use due to severe toxic side effects, including cardiotoxicity, which may lead to cardiomyopathy and heart failure depending on the dose of doxorubicin from acute or chronic drug harmful effects (Outomuro et al., 2007; Salazar-Mendiguchía et al., 2014).
There is a worldwide trend towards natural remedies to cure different diseases as they play an important role in many cardiac diseases with minimal or no side effects. Jatropha mollissima has an anti-oxidant potential as it possesses free radical scavenging potential. The ability of J. mollissima extract to suppress superoxide and hydroxyl free radicals is dose-dependent. Additionally, it dose-dependently inhibits lipid peroxidation and protein oxidation (M. O. Iqbal and Yahya, 2021). They allow the body to recover its normal levels of antioxidant enzymes like catalase, superoxide dismutase, and peroxidases that shield the body from toxic substances. My research aimed to ascertain whether or not J. mollissima extract offered protection against the cardiotoxicity and nephrotoxicity caused by doxorubicin.
Excessive fluid collection in the pleural, pericardial, and peritoneal cavities indicated cardiomyopathy in my experimental models after intraperitoneal doxorubicin treatment (Herman et al., 1988). Due to aberrant cardiac functioning and the development of ascites, animals given doxorubicin had a 40% higher mortality rate than the control group by the end of the trial (Renu et al., 2018).
Before the termination of the experiment, a 40% mortality rate was observed in Doxo intoxicated group due to severe cardiac toxicity. As previously mentioned, ascites occurred due to tubular dysfunction and salt retention, which caused extracellular volume growth and fluid leaking towards the interstitium (Deschênes and Doucet, 2000). Multiple renal indicators pointed to nephrotoxicity, likely contributing to the high fatality rate. Due to the medications’ toxic effect on the intestinal mucosa, many people found they were eating less and losing weight. That was achieved by the drugs’ indirect effect on the digestive system, lowering release of endogenous hormones and reducing hunger (Zima et al., 2001). Previous reports indicated that using Jm led to increased food intake and improved intestinal mucosa, contributing to the rise in body weight compared to the intoxicated group (El-Far et al., 2016).
Cardiac cell apoptosis was due to reactive oxygen species (ROS) produced by DOX, which caused a damaging effect on lipids, DNA, and proteins and caused impaired cells (Abogmaza et al., 2020).
Creatine isoenzymes CK-MB, lactate dehydrogenase plus troponin I are the most reliable indicators of cardiac cell damage. Their level was highly elevated in the Doxo group, indicating severe cardiac cell damage. These markers were released due to oxidative damage of cardiac myocytes by Doxo, which caused the release of these cardiac biomarkers in the circulation (Abd-Allah et al., 2002). Increased formation of free radicals, especially superoxide anion, causes an inflammatory cascade. The heart is vulnerable to free radicals due to less free radical detoxifying substances like glutathione, catalase, and superoxidase dismutase (Olson and Mushlin, 1990).
The cardioprotective action of Jm was confirmed by the decrease in mortality rate and the reduction in CK-MB, troponin I, and LDH levels after administration of J. mollissima extract at doses of 400 & 600 mg/kg. It was suggested that Jm possesses anti-oxidant potential due to phenols, carotenoids, and anthocyanins (Pongprot et al., 2012). Jm possesses free radical scavenging activity causing a significant reduction in the lipid peroxide concentration, which gives protection from lipid peroxidation (Arafa et al., 2014). Also, doxorubicin has a high affinity to cardiolipin and phospholipids in cardiac tissues.
Increased plasma sodium levels were seen in doxorubicin-treated rats compared to controls, but these levels returned to baseline in Jm-treated groups. Increased sodium levels have also been reported in rats with cardiac damage (Oz and Ilhan, 2006).
As it had been previously investigated that cardiomyopathy caused hypokalemia, doxorubicin treatment decreased plasma potassium levels compared to the normal control group. In contrast, treatment with Jm increased potassium levels to almost normal values in the intoxicated group, as shown in earlier research (Stevens and Levey, 2005).
Doxorubicin was found to cause nephrotoxicity through oxidative stress, with the resulting free radicals leading to tubular atrophy and heightened glomerular capillary permeability. Damage to biological macromolecules and lipid peroxidation due to iron-dependent oxidative damage contributed to nephrotoxicity (Ma et al., 2013). Because doxo metabolites are partially excreted by the kidney, the progression of kidney disease is dose- and time-dependent. Renal injury can also be caused by the generation of hydroxyl radicals and superoxide anions due to the conversion of doxorubicin to semiquinone free radical by NADPH-Cytochrome P-450 (Yancy et al., 2013). Kidney and heart lesions and damage have been related to a number of drugs and environmental toxins (Abogmaza et al., 2020). Some drugs have been associated to shifts in cell and tissue lipid peroxides and metabolites. Pro-oxidants such as reactive oxygen (ROS) species can induce tissue damage (M. O. Iqbal and Yahya, 2021). Herbs with nephroprotective and cardioprotective properties abound, and these often include antioxidant-rich plants. Doxorubicin reduces acetyl-CoA concentrations, inhibits lipogenesis, inhibits glucose production, reduces urea production, and inhibits lipid peroxidation in rats. We found that Jm at both dosages (400 and 600 mg/kg) significantly (p > 0.005) mitigated the doxorubicin-induced oxidative stress and slowed the degradation of renal and heart function. Their ability to serve as radical scavengers and hydrogen donors is a result of the antioxidant strength provided by Jm phenols. Antioxidants found naturally in plants are secondary plant metabolites (Mosmann, 1983; Gülçin et al., 2004).
Due to impaired sodium reabsorption caused by tubular abnormalities, doxorubicin-treated groups excreted less sodium in their urine than the normal control group. Previous research revealed that activation of Na+/K+ ATPase in the cortical collecting duct was responsible for this sodium retention (Ahmed et al., 2019).
Sodium excretion was higher in Jm-treated groups, suggesting that the extract may have a positive effect on renal function thanks to Jm’s nephroprotective action, which consists of repairing proximal tubular damage with its antioxidative properties (Rodriguez de Sotillo and Hadley, 2002).
According to the most recent data (Mattiuzzi and Lippi, 2019) cancer is the second largest cause of death worldwide. It was responsible for an estimated 9.6 million deaths in 2018, up nearly 10% from 2015’s total of 8.8 million, according to (Demain and Vaishnav, 2011). This has a major impact on the global economy and patients’ quality of life.
Due to the global economic effect and the rising number of cancer fatalities, finding a cancer therapy has been the primary research focus. For over 40 years, oncology has benefited from using natural products (Qamar, Rehman and Chauhan, 2019). The researchers set out to answer the question, “Could a component of this plant be used as a treatment option for cancer patients?” and to build the groundwork for future research into the use of medicinal plants in cancer treatment.
In 2018, cancer was the second top killer worldwide. Several harmful effects have been linked to the rising use of chemotherapy and radiotherapy to increase cancer patient survival (Nayim et al., 2021). As a result, researchers have been looking for novel anticancer drugs that are more potent with fewer negative side effects. Recent research has shown that plant-based anticancer medications have minimal cytotoxic values in a dose-dependent MTT experiment, inhibiting tumor development without causing unacceptable side effects (Elmore, 2007). Thus, there has been a trend toward investigating the phytochemical content of medicinal plants in the quest for safe and effective cancer treatments.
The hepatocellular carcinoma (HepG2) cell line also showed a concentration-dependent response to the plant extracts. The highest concentration of plant extracts (400 g/mL) was shown to inhibit cells to a greater extent than any of the lower doses tested. As shown in the Figure 11, the percentage of cells inhibited rose with the concentration of the extracts. However, the effect on cell proliferation was minimal after 24 h of treatment with doses up to 90 g/mL of crude ethanolic extract in HepG2 cells. Cell viability declined by over 50% at doses higher than 100 μg/mL (Figure Figure11). Cell viability decreased proportionally after treatment with a different concentration of Jm extract. However, as Figure demonstrates, safe levels for healthy cells with a 90% or above viability are below 90 or 100 μg/mL. These results jived with a recently conducted study that evaluated the in vivo toxicological effect of the IC crude extract (Ho et al., 2009).
Therefore, determining which type of cell death is induced by the plant extracts, apoptosis or necrosis, is important. Apoptosis is characterized by the following events: cell detachment and shrinkage; extensive blebbing of the plasma membrane; and the formation of apoptotic bodies, which are composed of cytoplasm with tightly packed cellular organelles and may or may not contain nuclear fragments (Fesik, 2005). These entities are protected from lysis in vivo because macrophages and neighboring cells, including parenchymal and neoplastic cells, phagocytose them. In contrast, in an in vitro setting, the absence of phagocytes causes the apoptotic bodies to expand and lyse, leading to subsequent necrosis (Nicholson, 2000).
The ability of cells to avoid programmed cell death, often called apoptosis, is one of the six important modifications in cell physiology that control the growth of tumor cells (Van Engeland et al., 1998). This is because necrotic cells are less likely to undergo apoptosis than healthy cells, which can lead to uncontrolled tumor cell growth, failure to adapt to cellular stress, damaging mutations, and DNA damage (Moss et al., 1991).
The Annexin V-FITC Apoptosis detection kit is utilized in this study to identify the precise form of cell death caused by the bioactive plant extracts. The kit includes a mix of Annexin V, Propidium Iodide, and fluorescein isothiocyanate (FITC) conjugate to detect and differentiate apoptotic, necrotic, and dead cells. The negatively charged phospholipid phosphatidylserine (PS) is normally located in the cytosolic leaflet of the plasma membrane lipid bilayer. It was discovered by that annexin V binds to PS very strongly in the presence of physiological amounts of Calcium ions (Ca2+) (Moss et al., 1991; Kaufmann and Vaux, 2003), annexin V binds to PS during the transition from the inner to the outer leaflet of the plasma membrane during the early stages of apoptosis. When propidium iodide enters a cell and bonds to the DNA there, it shows that it is dead. The inability to induce programmed cell death (apoptosis) is a major obstacle to the effective use of anticancer drugs (Cummings et al., 2004).
It was determined that the phenols, flavonoids, and flavone in J. mollissima have anti-oxidant potential and can prevent doxorubicin-induced damage to the heart and kidneys.
5 Conclusion and recommendations
Based on the findings of this investigation, it appears that antioxidant enzymes could be normalized, cardiac enzymes could be improved, and pathways triggering cardiac apoptosis could be altered by co-administering J. mollissima extract with doxorubicin.
Jatropha mollissima hydroalcoholic extract also showed nephroprotective action by increasing the amount of free radical scavenging enzymes due to its anti-oxidant potential, captured free radicals, and reduced lipid peroxidation as it contains phenols and melatonin, which are free radical scavengers. Our results further demonstrated that J. mollissima ethanolic extracts are cytotoxic to the hepatocellular carcinoma (HepG2) cell line.
Therefore, it could be recommended that J. mollissima extract is effective against Doxo - induced cardiotoxicity and nephrotoxicity without attenuating the clinical efficacy of doxorubicin. To control Hepatocellular carcinoma, Jm is therefore considered a promising medicinal substance. The results indicated that the plant could inhibit tumor growth through various mechanisms (such as inducing apoptosis). The prevention of hepatocellular cancer has been greatly advanced due to this study. However, there is still a lot of research to be done to evaluate the relevant signaling pathway.
Jatropha mollissima extract could be used before treatment with doxorubicin or given during therapy to prevent cardiotoxicity and nephrotoxicity, evade the need for other medications, and thus improve the quality of the patient’s life.
Data availability statement
The original contributions presented in the study are included in the article/Supplementary Material, further inquiries can be directed to the corresponding authors.
Ethics statement
The animal study was approved by the research projects were approved by the Muhammad Institute of Medical and Allied Science’s Ethical Committee in Multan, Pakistan. A valid MIMAS/07-21/25261 authorization number was provided. The study was conducted in accordance with the local legislation and institutional requirements.
Author contributions
MI: Conceptualization, Data curation, Formal Analysis, Investigation, Methodology, Project administration, Resources, Software, Validation, Visualization, Writing–original draft, Writing–review and editing. YG: Supervision, Validation, Visualization, Writing–review and editing. IK: Resources, Writing–review and editing. RW: Funding acquisition, Software, Writing–review and editing. JC: Funding acquisition, Validation, Writing–review and editing.
Funding
The author(s) declare financial support was received for the research, authorship, and/or publication of this article. This work was supported by the program of Qingdao Marine Science and Technology Centre (No. 2022QNLM030003-4, No. 8-01) and the project of Qingdao University of Science and Technology talent introduction research start-up fund.
Conflict of interest
The authors declare that the research was conducted in the absence of any commercial or financial relationships that could be construed as a potential conflict of interest.
Publisher’s note
All claims expressed in this article are solely those of the authors and do not necessarily represent those of their affiliated organizations, or those of the publisher, the editors and the reviewers. Any product that may be evaluated in this article, or claim that may be made by its manufacturer, is not guaranteed or endorsed by the publisher.
References
Abd-Allah, A. R., Al-Majed, A. A., Mostafa, A. M., Al-Shabanah, O. A., Din, A. G., and Nagi, M. N. (2002). Protective effect of Arabic gum against cardiotoxicity induced by doxorubicin in mice: a possible mechanism of protection. J. Biochem. Mol. Toxicol. 16 (5), 254–259. doi:10.1002/jbt.10046
Abogmaza, A. F., Keer, K. F., Takrizzah, A., and Yahya, E. B. (2020). A review on the medicinal and aromatic plants growing in Libya and their therapeutic properties. Int. Res. J. Sci. Technol. 2 (1), 327–334. doi:10.46378/irjst.2020.020105
Ahmed, O. M., Abdul-Hamid, M. M., El-Bakry, A. M., Mohamed, H., and Abdel Rahman, F. (2019). Effects of green tea infusion and epicatechin on doxorubicin-induced renocardiotoxicity in male albino rats. IJPSR 10 (5), 1000–1014. doi:10.13040/IJPSR.0975-8232.10(5).2210-23
Ain, Q. U. (2022). Anticoagulant and thrombolytic activities of leaf extract of mangifera indica in smokers. Tob. Regul. Sci. (TRS), 1189–1201. doi:10.18001/TRS.8.1.96
Arafa, M. H., Mohammad, N. S., Atteia, H. H., and Abd-Elaziz, H. R. (2014). Protective effect of resveratrol against doxorubicin-induced cardiac toxicity and fibrosis in male experimental rats. J. Physiol. Biochem. 70 (3), 701–711. doi:10.1007/s13105-014-0339-y
Bastos-Cavalcante, N., dos Santos-Barbosa, C. R., Silva-Pereira, R. L., Feitosa-Muniz, D., de Melo-Coutinho, H. D., Araújo-Rolim, L., et al. (2020). “Phytochemical analysis, antibacterial activity and antibiotic modifying action of Jatropha mollissima (Pohl.) Baill.(Euphorbiaceae),” in Anales de Biología USA, (Servicio de Publicaciones de la Universidad de Murcia), 85–94.
Chen, X., Zhang, Y., Zhu, Z., Liu, H., Guo, H., Xiong, C., et al. (2016). Protective effect of berberine on doxorubicin-induced acute hepatorenal toxicity in rats. Mol. Med. Rep. 13 (5), 3953–3960. doi:10.3892/mmr.2016.5017
Cummings, J., Ward, T. H., Ranson, M., and Dive, C. (2004). Apoptosis pathway-targeted drugs—from the bench to the clinic. Biochimica Biophysica Acta (BBA)-Reviews Cancer 1705 (1), 53–66. doi:10.1016/j.bbcan.2004.09.005
de Albuquerque, U. P., Muniz de Medeiros, P., de Almeida, A. L., Monteiro, J. M., Machado de Freitas Lins Neto, E., Gomes de Melo, J., et al. (2007). Medicinal plants of the caatinga (semi-arid) vegetation of NE Brazil: a quantitative approach. J. Ethnopharmacol. 114 (3), 325–354. doi:10.1016/j.jep.2007.08.017
Demain, A. L., and Vaishnav, P. (2011). Natural products for cancer chemotherapy. Microb. Biotechnol. 4 (6), 687–699. doi:10.1111/j.1751-7915.2010.00221.x
de Queiroz Neto, R. F., de Araújo Júnior, H. N., Freitas, C. I. A., Costa, K. M. d. F. M., Abrantes, M. R., de Almeida, J. G. L., et al. (2019). The Jatropha mollissima (Pohl) Baill: chemical and pharmacological activities of the latex and its extracts. Semina Ciências Agrárias, Londrina 40 (6), 2613–2624. doi:10.5433/1679-0359.2019v40n6p2613
Deschênes, G., and Doucet, A. (2000). Collecting duct (Na+/K+)-ATPase activity is correlated with urinary sodium excretion in rat nephrotic syndromes. J. Am. Soc. Nephrol. 11 (4), 604–615. doi:10.1681/asn.V114604
Dias, W., do Vale Junior, E., de Oliveira, M. d. D. A., Barbosa, Y., do Nascimento Silva, J., da Costa Júnior, J., et al. (2019). Cytogenotoxic effect, phytochemical screening and antioxidant potential of Jatropha mollissima (Pohl) Baill leaves. South Afr. J. Bot. 123, 30–35. doi:10.1016/j.sajb.2019.02.007
Ebrahimzadeh, M. A., Nabavi, S. F., Nabavi, S. M., and Eslami, B. (2010). Antihemolytic and antioxidant activities of Allium paradoxum. Central Eur. J. Biol. 5, 338–345. doi:10.2478/s11535-010-0013-5
El-Far, A. H., Ahmed, H. A., and Shaheen, H. M. (2016). Dietary supplementation of Phoenix dactylifera seeds enhances performance, immune response, and antioxidant status in broilers. Oxid. Med. Cell. Longev. 2016, 1–9. doi:10.1155/2016/5454963
Elmore, S. (2007). Apoptosis: a review of programmed cell death. Toxicol. Pathol. 35 (4), 495–516. doi:10.1080/01926230701320337
El-Sayed, E. M., El-azeem, A. S., Afify, A. A., Shabana, M. H., and Ahmed, H. H. (2011). Cardioprotective effects of Curcuma longa L. extracts against doxorubicin-induced cardiotoxicity in rats. J. Med. Plants Res. 5 (17), 4049–4058. doi:10.5897/JMPR.9000318
Fesik, S. W. (2005). Promoting apoptosis as a strategy for cancer drug discovery. Nat. Rev. Cancer 5 (11), 876–885. doi:10.1038/nrc1736
Ghigo, A., Li, M., and Hirsch, E. (2016). New signal transduction paradigms in anthracycline-induced cardiotoxicity. Biochim. Biophys. Acta 1863 (7 Pt B), 1916–1925. doi:10.1016/j.bbamcr.2016.01.021
Giri, S. N., Al-Bayati, M. A., Du, X., Schelegle, E., Mohr, F. C., and Margolin, S. B. (2004). Amelioration of doxorubicin-induced cardiac and renal toxicity by pirfenidone in rats. Cancer Chemother. Pharmacol. 53 (2), 141–150. doi:10.1007/s00280-003-0703-z
Gülçin, I., Küfrevioǧlu, Ö. İ., Oktay, M., and Büyükokuroǧlu, M. E. (2004). Antioxidant, antimicrobial, antiulcer and analgesic activities of nettle (Urtica dioica L.). J. Ethnopharmacol. 90 (2-3), 205–215. doi:10.1016/j.jep.2003.09.028
Herman, E. H., Ferrans, V. J., Young, R. S., and Hamlin, R. L. (1988). Effect of pretreatment with ICRF-187 on the total cumulative dose of doxorubicin tolerated by beagle dogs. Cancer Res. 48 (23), 6918–6925. Available at: http://aacrjournals.org/cancerres/article-pdf/48/23/6918/2434104/cr0480236918.pdf
Ho, K., Yazan, L. S., Ismail, N., and Ismail, M. (2009). Apoptosis and cell cycle arrest of human colorectal cancer cell line HT-29 induced by vanillin. Cancer Epidemiol. 33 (2), 155–160. doi:10.1016/j.canep.2009.06.003
Hosseini, A., and Sahebkar, A. (2017). Reversal of doxorubicin-induced cardiotoxicity by using phytotherapy: a review. J. Pharmacopuncture 20 (4), 243–256. doi:10.3831/kpi.2017.20.030
Iqbal, M. O., Khan, I. A., Manzoor, A., Arshad, S., Sial, A. S., Dar, E., et al. (2021). Cardioprotective effect of hydroalcoholic leaf extract of Jatropha mollissima on isoproterenol-induced myocardial infarction in rats. Pharmacogn. Mag. 17 (Suppl. 2), S251–S256. doi:10.4103/pm.pm_16_21
Iqbal, M. O., Naeem, M., Mumtaz, A., Ahmed, M. M., Ahmad, A., Riaz, R., et al. (2022). Biochemical evaluation and medicinal ability of Jatropha mollissima in hepatic disorders. Am. J. Transl. Res. 14 (10), 7178–7188.
Iqbal, M. O., and Yahya, E. B. (2021). In vivo assessment of reversing aminoglycoside antibiotics nephrotoxicity using Jatropha mollissima crude extract. Tissue Cell. 72, 101525. doi:10.1016/j.tice.2021.101525
Kaufmann, S. H., and Vaux, D. L. (2003). Alterations in the apoptotic machinery and their potential role in anticancer drug resistance. Oncogene 22 (47), 7414–7430. doi:10.1038/sj.onc.1206945
Kelloff, G. J. (1999). Perspectives on cancer chemoprevention research and drug development. Adv. Cancer Res. 78, 199–334. doi:10.1016/s0065-230x(08)61026-x
Khan, I. A., Hussain, M., Hussain, N., Alqahtani, A. M., and Alqahtani, T. (2022). Cardioprotective effect of rumex vesicarius linn. Leaf extract against catecholamine-induced cardiotoxicity. Molecules 27 (11), 3383. doi:10.3390/molecules27113383
Khan, I. A., Hussain, M., Munawar, S. H., Iqbal, M. O., Arshad, S., Manzoor, A., et al. (2021a). Jasminum sambac: a Potential candidate for drug development to cure cardiovascular ailments. Molecules 26 (18), 5664. doi:10.3390/molecules26185664
Khan, I. A., Hussain, M., Syed, S. K., Saadullah, M., Alqahtani, A. M., Alqahtani, T., et al. (2021b). Pharmacological justification for the medicinal use of Plumeria rubra Linn. in cardiovascular disorders. Molecules 27 (1), 251. doi:10.3390/molecules27010251
Kirstein, M. (2009). Neoplastic disorders and their treatment: general principles. Applied therapeutics-the clinical Use of drugs. 19th edition. Philadelphia: Lippincott Williams & Wilkins, 1–32.
Ma, J., Wang, Y., Zheng, D., Wei, M., Xu, H., and Peng, T. (2013). Rac1 signalling mediates doxorubicin-induced cardiotoxicity through both reactive oxygen species-dependent and -independent pathways. Cardiovasc Res. 97 (1), 77–87. doi:10.1093/cvr/cvs309
Mattiuzzi, C., and Lippi, G. (2019). Current cancer epidemiology. J. Epidemiol. Glob. health 9 (4), 217. doi:10.2991/jegh.k.191008.001
Mensor, L. L., Menezes, F. S., Leitão, G. G., Reis, A. S., Santos, T. C. d., Coube, C. S., et al. (2001). Screening of Brazilian plant extracts for antioxidant activity by the use of DPPH free radical method. Phytotherapy Res. 15 (2), 127–130. doi:10.1002/ptr.687
Mosmann, T. (1983). Rapid colorimetric assay for cellular growth and survival: application to proliferation and cytotoxicity assays. J. Immunol. methods 65 (1-2), 55–63. doi:10.1016/0022-1759(83)90303-4
Moss, S. E., Edwards, H. C., and Crumpton, M. J. (1991). “Diversity in the annexin family,” in Novel calcium-binding proteins: fundamentals and clinical implications (Germany: Springer), 535–566.
Nagai, K., Fukuno, S., Otani, K., Nagamine, Y., Omotani, S., Hatsuda, Y., et al. (2018). Prevention of doxorubicin-induced renal toxicity by theanine in rats. Pharmacology 101 (3-4), 219–224. doi:10.1159/000486625
Nayim, P., Sudhir, K., Mbaveng, A. T., Kuete, V., and Sanjukta, M. (2021). In vitro anticancer activity of Imperata cylindrica root’s extract toward human cervical cancer and identification of potential bioactive compounds. BioMed Res. Int. 2021, 1–12. doi:10.1155/2021/4259777
Nicholson, D. W. (2000). From bench to clinic with apoptosis-based therapeutic agents. Nature 407 (6805), 810–816. doi:10.1038/35037747
Olson, R. D., and Mushlin, P. S. (1990). Doxorubicin cardiotoxicity: analysis of prevailing hypotheses. FASEB J. 4 (13), 3076–3086. doi:10.1096/fasebj.4.13.2210154
Omer Iqbal, M., Bashir Yahya, E., Andleeb, S., Masood Ahmed, M., Umar Javaid, M., Shakeel, W., et al. (2021). In vivo assessment of reversing Cisplatin-Induced nephrotoxicity using Jatropha mollissima crude extract and its potential cytotoxicity. Saudi J. Biol. Sci. 28 (12), 7373–7378. doi:10.1016/j.sjbs.2021.08.057
Outomuro, D., Grana, D. R., Azzato, F., and Milei, J. (2007). Adriamycin-induced myocardial toxicity: new solutions for an old problem? Int. J. Cardiol. 117 (1), 6–15. doi:10.1016/j.ijcard.2006.05.005
Oz, E., and Ilhan, M. N. (2006). Effects of melatonin in reducing the toxic effects of doxorubicin. Mol. Cell. Biochem. 286 (1-2), 11–15. doi:10.1007/s11010-005-9003-8
Pongprot, Y., Sittiwangkul, R., Charoenkwan, P., and Silvilairat, S. (2012). Use of cardiac markers for monitoring of doxorubixin-induced cardiotoxicity in children with cancer. J. Pediatr. Hematol. Oncol. 34 (8), 589–595. doi:10.1097/MPH.0b013e31826faf44
Qamar, H., Rehman, S., and Chauhan, D. (2019). Current status and future perspective for research on medicinal plants with anticancerous activity and minimum cytotoxic value. Curr. Drug Targets 20 (12), 1227–1243. doi:10.2174/1389450120666190429120314
Rashid, S., Ali, N., Nafees, S., Ahmad, S. T., Arjumand, W., Hasan, S. K., et al. (2013). Alleviation of doxorubicin-induced nephrotoxicity and hepatotoxicity by chrysin in Wistar rats. Toxicol. Mech. Methods 23 (5), 337–345. doi:10.3109/15376516.2012.759306
Renu, K., Arunachalam, S., and P.b., T. P. (2018). Molecular mechanism of doxorubicin-induced cardiomyopathy - an update. Eur. J. Pharmacol. 818, 241–253. doi:10.1016/j.ejphar.2017.10.043
Rodriguez de Sotillo, D. V., and Hadley, M. (2002). Chlorogenic acid modifies plasma and liver concentrations of: cholesterol, triacylglycerol, and minerals in (fa/fa) Zucker rats. J. Nutr. Biochem. 13 (12), 717–726. doi:10.1016/s0955-2863(02)00231-0
Ruch, R. J., Cheng, S.-j., and Klaunig, J. E. (1989). Prevention of cytotoxicity and inhibition of intercellular communication by antioxidant catechins isolated from Chinese green tea. Carcinogenesis 10 (6), 1003–1008. doi:10.1093/carcin/10.6.1003
Ruggeri, C., Gioffré, S., Achilli, F., Colombo, G. I., and D'Alessandra, Y. (2018). Role of microRNAs in doxorubicin-induced cardiotoxicity: an overview of preclinical models and cancer patients. Heart Fail Rev. 23 (1), 109–122. doi:10.1007/s10741-017-9653-0
Salazar-Mendiguchía, J., González-Costello, J., Roca, J., Ariza-Solé, A., Manito, N., and Cequier, A. (2014). Anthracycline-mediated cardiomyopathy: basic molecular knowledge for the cardiologist. Arch. Cardiol. Mex. 84 (3), 218–223. doi:10.1016/j.acmx.2013.08.006
Stevens, L. A., and Levey, A. S. (2005). Measurement of kidney function. Med. Clin. 89 (3), 457–473. doi:10.1016/j.mcna.2004.11.009
Touitou, Y., Portaluppi, F., Smolensky, M. H., and Rensing, L. (2004). Ethical principles and standards for the conduct of human and animal biological rhythm research. Chronobiol Int. 21 (1), 161–170. doi:10.1081/cbi-120030045
Van Engeland, M., Nieland, L. J., Ramaekers, F. C., Schutte, B., and Reutelingsperger, C. P. (1998). Annexin V-affinity assay: a review on an apoptosis detection system based on phosphatidylserine exposure. Cytom. J. Int. Soc. Anal. Cytol. 31 (1), 1–9. doi:10.1002/(sici)1097-0320(19980101)31:1<1::aid-cyto1>3.0.co;2-r
Yancy, C. W., Jessup, M., Bozkurt, B., Butler, J., Casey, D. E., Drazner, M. H., et al. (2013). 2013 ACCF/AHA guideline for the management of heart failure: executive summary: a report of the American College of Cardiology Foundation/American Heart Association Task Force on practice guidelines. Circulation 128 (16), 1810–1852. doi:10.1161/CIR.0b013e31829e8807
Yen, G.-C., and Chen, H.-Y. (1995). Antioxidant activity of various tea extracts in relation to their antimutagenicity. J. Agric. food Chem. 43 (1), 27–32. doi:10.1021/jf00049a007
Keywords: antioxidant, cardioprotective, nephroprotective, doxorubicin, anticancer, Jatropha mollissima
Citation: Iqbal MO, Gu Y, Khan IA, Wang R and Chen J (2023) Evaluation of the in vitro antioxidant and antitumor activity of hydroalcoholic extract from Jatropha mollissima leaves in Wistar rats. Front. Chem. 11:1283618. doi: 10.3389/fchem.2023.1283618
Received: 26 August 2023; Accepted: 04 December 2023;
Published: 18 December 2023.
Edited by:
Mohd Imran, Northern Border University, Saudi ArabiaReviewed by:
Mohammad Sheibani, Iran University of Medical Sciences, IranHadia Almahli, University of Cambridge, United Kingdom
Copyright © 2023 Iqbal, Gu, Khan, Wang and Chen. This is an open-access article distributed under the terms of the Creative Commons Attribution License (CC BY). The use, distribution or reproduction in other forums is permitted, provided the original author(s) and the copyright owner(s) are credited and that the original publication in this journal is cited, in accordance with accepted academic practice. No use, distribution or reproduction is permitted which does not comply with these terms.
*Correspondence: Jin Chen, chenjin110@zju.edu.cn; Ruihong Wang, qdhong2009@163.com