Isolipoic acid-linked gold nanoparticles bearing the thomsen friedenreich tumor-associated carbohydrate antigen: Stability and in vitro studies
- Chemical Biology Laboratory, Center for Cancer Research, National Cancer Institute at Frederick, Frederick, MD, United States
We have previously prepared gold nanoparticles (AuNPs) bearing the Thomsen-Friedenreich antigen disaccharide (TFag), a pan-carcinoma, Tumor-Associated Carbohydrate Antigen (TACA), as tools for various assays and biological applications. Conjugation to AuNPs typically involves the use of thiols due to the affinity of sulfur for the gold surface of the nanoparticle. While a use of a single thiol-containing ligand bound to the gold surface is standard practice, several studies have shown that ligands bearing multiple thiols can enhance the strength of the conjugation in a nearly linear fashion. (R)-(+)-α-Lipoic acid (LA), a naturally occurring disulfide-containing organic acid that is used as a cofactor in many enzymatic reactions, has been used as a linker to conjugate various molecules to AuNPs through its branched di-thiol system to enhance nanoparticle stability. We sought to use a similar system to increase nanoparticle stability that was devoid of the chiral center in (R)-(+)-α-lipoic acid. Isolipoic acid, an isomer of LA, where the exocyclic pentanoic acid chain is shifted by one carbon on the dithiolane ring to produce an achiral acid, was thought to act similarly as LA without the risk of any contaminating (L)-(−) isomer. We synthesized AuNPs with ligands of both serine and threonine glycoamino acids bearing the TFag linked to isolipoic acid and examined their stability under various conditions. In addition, these particles were shown to bind to Galectin-3 and inhibit the interaction of Galectin-3 with a protein displaying copies of the TFag. These agents should prove useful in the design of potential antimetastatic therapeutics that would benefit from achiral linkers that are geometrically linear and achiral.
1 Introduction
Tumor-Associated Carbohydrate Antigens (TACAs) are glycan structures covalently attached to proteins or lipids in various forms on the surface of tumor cells (Feizi and Childs, 1985; Hakomori, 1991; Dabelsteen, 1996). They differ from the normal cell glycan repertoire insofar as the tumor biosynthetic machinery is modified via a disparate regulation of glycosyltransferases and hydrolases. This produces aberrant and distinct cell-surface glycan structures that are unique to tumors, and these structures impart modified biophysical and protein binding characteristics to individual tumor types. In addition, some of these tumor-associated glycans can be recognized as “non-self” by the immune system (hence the moniker, “antigen”) eliciting both humoral and (sometimes) cell-mediated responses (Andreana, 2009). As a result, there have been myriad attempts to prepare vaccine constructs to raise effective and durable immune responses to TACAs (Toyokuni and Singhal, 1995; Xu et al., 2005; Franco, 2008; Guo and Wang, 2009; Liu and Ye, 2012; Yin and Huang, 2012; Amon et al., 2014; Feng et al., 2016; Wei et al., 2018; Jin et al., 2019). In addition, some of these TACAs are also ligands for carbohydrate-binding proteins (CBPs) that are involved in cell adhesion as they relate to cancer progression and metastasis. Thus, inhibition of these interactions is a way to interrupt tumor aggressiveness and metastatic spread.
One particular TACA that has been used in both of the aforementioned therapeutic arenas is the Thomsen Friedenreich antigen (TFag), which is the core 1 O-linked disaccharide Galβ1-3GalNAc found primarily attached in an α-O-linkage to the hydroxyl group of serine and threonine residues of various cell-surface proteins, primarily mucins. TFag is a classically truncated O-glycan found on many tumors of the breast, prostate and pancreas. The TFag has been the subject of a wealth of anticancer therapeutic design, either through inhibition of its binding to cancer-relevant proteins (Peletskaya et al., 1997; Khaldoyanidi et al., 2003; Jeschke et al., 2006; Jeschke et al., 2007; Gao et al., 2012; Glinskii et al., 2012; Poiroux et al., 2017; Hoffmann et al., 2020) or as an immunogen in various vaccine design strategies (Slovin et al., 2005; Awad et al., 2012; Brinas et al., 2012; Gaidzik et al., 2013; Ulsemer et al., 2013; Yi et al., 2013; Bourgault et al., 2014; Johannes et al., 2015; Son et al., 2016; Sun et al., 2016; Trabbic et al., 2016; Flechner et al., 2019; Trabbic et al., 2019; Wu et al., 2019; Kleski et al., 2020; Wu et al., 2021; Berois et al., 2022). Mechanistically, the TFag has been unequivocally shown to interact with the CBP Galectin-3, a β-galactoside binding protein that interacts both intra- and extracellularly with many glycoproteins, is overexpressed in a variety of tumors and whose expression is directly correlated with tumor aggressiveness and metastasis (Takenaka et al., 2002). TFag is involved in adhesion of tumor cells to the endothelium and these interactions can mediate signaling that allows extravasation of primary tumor tissue (Glinsky et al., 2001; Khaldoyanidi et al., 2003; Glinsky, 2006; Yu et al., 2007; Compagno et al., 2014; Hauselmann and Borsig, 2014; Xin et al., 2015). Thus, inhibitors of the Gal-3/TFag interaction are potential therapeutics in several types of cancers. TFag analogues (Glinskii et al., 2012; Santarsia et al., 2018), truncated portions of Gal-3 (John et al., 2003), TFag-mimicking peptides (Glinsky et al., 2000; Newton-Northup et al., 2013) and natural glycopeptides containing multiple copies of TFag (Guha et al., 2013) have all been shown to inhibit this interaction in different cell-based systems. In addition, antibodies to the TFag have been used as anticancer agents, especially against breast and prostate tumors (Glinsky et al., 2001; Glinsky et al., 2003; Tantivejkul et al., 2004). JAA-F11, a monoclonal antibody (mAb) to α-TFag (Rittenhouse-Olson, 2007), has shown good in vitro and in vivo activity in breast tumor models, with inhibition of spontaneous metastasis (Heimburg et al., 2006), distinct tumor staining of TFag-positive tissue (Ferguson et al., 2014; Karacosta et al., 2018) and it has been humanized for potential clinical development (Tati et al., 2017). In our own lab, we have developed and commercialized an antibody to a TFag-containing peptide sequence from the mucin MUC4, a biomarker for pancreatic cancer, that is highly selective for tumor tissue and binds metastatic foci in tissue arrays (Trabbic et al., 2019).
Monovalent carbohydrate-protein interactions (CPIs) are known to be inherently weak and are strengthened by multivalency (the “Velcro effect”) (Nicotra et al., 2014). Cell-surface CPIs utilize multimeric copies of protein and sugar to fine tune the strength of a specific interaction, and this is also the case for TFag-Gal-3 (or other CBP) binding events. Multivalent TFag constructs have been pioneered by the research group of Roy and coworkers (Baek et al., 2001; Baek and Roy, 2002; Roy and Baek, 2003). These studies showed that dendrimers and saccharide polymers of the TFag can potentiate the interaction of the disaccharide with proteins by several orders of magnitude. Our group has made inroads into the use of gold nanoparticles (AuNPs) for the multivalent presentation of TFag and TFag-containing glycopeptides, and showed that these constructs can bind to Galectin-3 (Gal-3) and inhibit Gal-3 interactions with other proteins. (Svarovsky et al., 2005; Sundgren and Barchi, 2008; Barchi, 2011; Brinas et al., 2012; Glinskii et al., 2014; Biswas et al., 2015). In addition, we have performed several antimetastatic in vivo studies in our lab with many of our constructs that early on seemed quite promising, but results have been inconsistent (and hence unpublishable). We reasoned that the stability of the TFag ligand on the AuNP may be an issue when these AuNP are in the systemic circulation in an intact animal. In an attempt to address this, we set out to make a similar construction, with a highly stable and symmetric linker that could be a common reagent for all subsequent nanoparticle syntheses. Comprehensive stability and in vitro studies suggested that this linker strategy is much more desirable than previous designs and will be useful in future therapeutic design strategies.
Development of novel nanomaterials with 3-dimensional self-assembled monolayers of gold (AuNPs) has been an incredibly active area of research in the past two decades. A wide array of these constructions has been designed and synthesized for therapeutic applications against a variety of diseases. In a majority of studies, the synthesis of AuNPs has followed the classic Turkevich (Turkevich et al., 1951; Enustun and Turkevich, 1963) or Brust methods (Brust et al., 1994; Brust et al., 1995). Attachment to the gold surface is usually mediated via a thiol functionality due to the high affinity of Au for sulfur atoms. Several studies have shown that additional means of attachment, through molecules containing 2–3 conjugatable sulfur atoms increases the strength of binding as each S-atom can contribute a defined bond strength in an additive way. (Park et al., 2005; Wojczykowski et al., 2006). This effect is a form of “multivalency” and the added stabilization prevents simple place exchange reactions with various concentrations of added soluble thiols.
One molecule that has garnered substantial attention as a bidentate ligand for AuNP conjugation is α-lipoic acid (LA), sometime referred to as thioctic acid (Figure 1). LA is a naturally occurring cofactor for endogenous enzymatic functions and is sold as a dietary supplement for its antioxidant properties (Goraca et al., 2011). Other than AuNPs, LA has been used in countless applications as a coating for a variety of other nanoparticles, such as nanospheres, cross-linked polymers and quantum dots (Koufaki et al., 2009; Jin et al., 2020), as well as for the preparation of glyco-AuNPs (Yeh et al., 2021) The additional stability, ready availability and ease of use have been the primary features that led to LA’s popularity. In addition to the convenient cyclic disulfide for attachment to metallic nanoparticles, LA has a “built in” conjugatable carboxylate group that is used for simple peptide bond coupling to most any molecular family of ligands, antigens, lipids or nucleic acids. LA has one chiral center and is often used as a racemic mixture. The (R)-(+)-isomer is the naturally occurring enantiomer, but many commercial preparations of “chiral” LA may contain small amounts of the (S)-(−)-isomer. An achiral analogue that retains similar conjugation bond strengths would be desirable in applications where enantiomeric purity is desire. In 2010, Tucker, et al., published an improved synthesis of isolipoic acid (iso-LA, Figure 1B) an achiral analogue of LA where the sidechain was shifted to the central carbon of the disulfide-containing 5 membered ring (Joly et al., 2010). This symmetrical molecule suggested a novel way of attaching ligands or other molecular families to nanoparticles through a “dual-pronged” approach. We present the synthesis and evaluation of TFag-coated AuNPs with a new easily prepared linker based on iso-LA.
2 Results
2.1 Synthesis
Previous syntheses of TFag-containing AuNPs in our lab were built around a TFag glycoamino acid and a specific linker strategy that we developed previously for our AuNP-based vaccine design (Brinas et al., 2012). Modifications to the synthesis were made to accommodate the linkage to a serine or threonine amino acid (Biswas et al., 2015). A very simple adjustment was made to build the linker after the preparation of large amounts the iso-LA precursor (Scheme 1). The yields in all steps of the iso-LA precursor were consistent with literature values. Following a similar protocol to one we published previously, the iso-LA derivatized hexa-PEGylated linker was prepared from commercially available mono-Boc-protected diamine PEG compound 1 and iso-LA via simple EDC/HOBt-mediated peptide coupling followed by Boc deprotection and repeated peptide coupling to attach the TFag-conjugated Fmoc-glycoamino acids of both serine and threonine. Removal of the Fmoc group, N-acetylation and Zemplen deprotection of the O-acetyl groups yielded compounds 8 (Serine derivative) and 9 (Threonine derivative) as precursors to nanoparticle synthesis. An iso-LA-linked hexaPEG conjugate terminated by a hydroxyl group was also prepared as a “control” ligand by simply coupling another commercially available unprotected hydroxyl-terminated PEG amine 10 with iso-LA to give compound 11. All steps were high-yielding and all new compounds were purified by reverse phase HPLC and characterized by high resolution mass spectrometry and multidimensional NMR techniques.
The synthesis of AuNPs proceeded from previously prepared solutions of AuNPs of defined size made via the Turkevich method (Figure 2). Concentrations were such to yield particles in the 16 nm core diameter size range. Solutions of these citrate stabilized AuNPs were analyzed for gold content by Inductively Coupled Plasma Mass Spectrometry (ICP-MS) which allowed calculation of accurate nanoparticle concentrations. Simple place exchange reactions allowed coupling of the TFag-containing and control ligands to be attached to the citrate-stabilized (“naked”) AuNPs after brief treatment with immobilized Tris [2-carboxyethyl] phosphine (TCEP) resin to reduce the cyclic disulfide bond. AuNPs 12–14 were purified by concentrating solutions on 50K cutoff filter membranes followed by resuspension and washing with MilliQ water. Nanoparticles were lyophilized and fully characterized as described in Section 2.2.
2.2 Characterization
While there are now thousands of reports on the synthesis and biomedical applications of AuNPs, characterization data of individual systems is often highly variable and sometimes incomplete. Thus, we set out to perform comprehensive assessments of all of our AuNPs by a variety of methods to ensure quality control and establish a “Lab Standard” for AuNP characterization.
Transmission Electron Microscopy (TEM). All precursor solutions were examined by TEM and showed highly uniform core sizes with narrow size distribution histograms (Figure 3A and Supplementary Figure S1). Liganded nanoparticles met identical criteria for uniformity and size consistency.
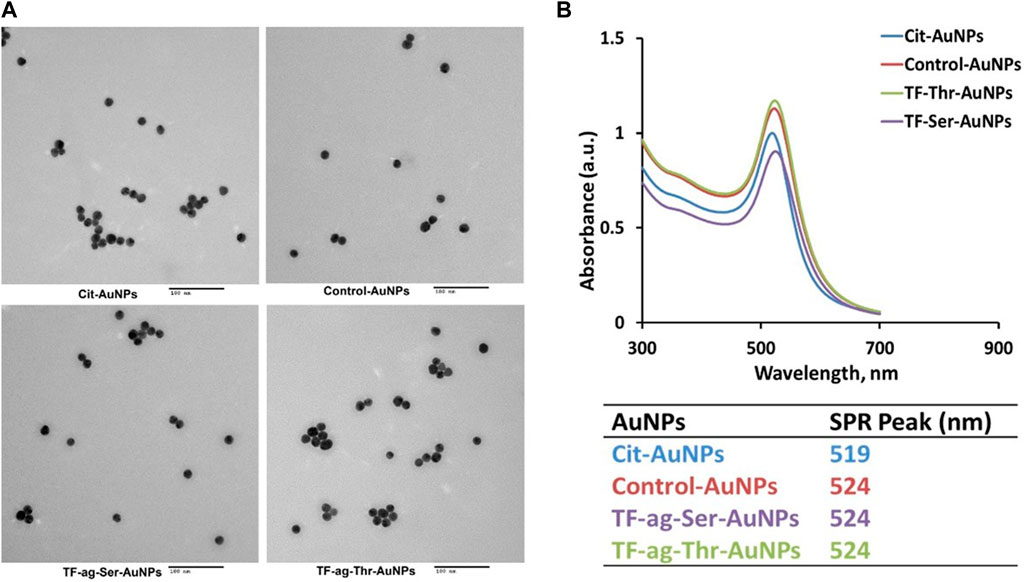
FIGURE 3. (A) Transmission electron micrographs of citrate-stabilized AuNPs and of AuNPs 12–14. (B) Plasmon band UV/Vis spectra of same AuNPs as in A, along with table of SP maximum for each material.
UV-Vis Surface Plasmon Band. Three-dimensional self-assembled spherical AuNPs are known to interact with light at specific wavelengths to cause oscillation of the free electrons at the gold surface; these oscillations are in resonance with the incoming light frequency, resulting in an absorption/emission spectrum where the absorption is in the 520 nm range. Emission is dependent on size and shape and is often in the red spectrum (700 nm). Citrate stabilized AuNPs of 16 nm have a maximum at 520 nm; addition of ligand causes a slight but consistent red shift to 524 nm (Figure 3B). This is also indicative of particle dispersiveness whereas aggregation cause a loss of signal and is characteristic of agglomeration (vide infra).
MALDI Mass Spectrometry. There have been several studies that have used MALDI mass spectrometry to study the morphology and the ligand distribution of AuNPs (Luo et al., 2017). MALDI was used to determine the presence of ligand on the particles. Supplementary Figure S2 shows the MALDI mass spec data for each of the AuNPs X-Y. Each spectrum contains peaks from the sodium adducts of each ligand that was coated on the gold core.
Dynamic Light Scattering (DLS). Hydrodynamic diameters were determined by DLS. All materials prepared gave quite uniform and single peak in the spectrum (Supplementary Figure S3). Intensity and volume measurements were similar where very few larger particles were observed in the intensity data. All correlation functions converged and thus the uniformity of the AuNPs were very high with Polydispersity Indices (PDI) all below 0.1 (Table 1).
Zeta Potential. Citrate-stabilized AuNPs are coated with anionic citrate ions that impart a highly negative zeta potential that is partially neutralized by the addition of neutral organic ligand layers, such as the ones used in this study. This was the case here as the zeta potential adjusts from −35 mV for the naked particles to between (−15)–(−17) mV for the coated particles. This drop in voltage did not have any detrimental effect on the stability or aggregative properties of the prepared AuNPs (vide infra).
Quantitation of TFag Disaccharide on AuNPs. The copy number of the serine and threonine-linked TFag-containing ligands on the AuNPs were quantitated by the well-known Phenol-Sulfuric acid method using a standard curve with varying concentration of β-lactose. Table 2 shows this number to be close to 1,600 for each nanoparticle prepared. This number was consistent among different batches and the copy number was essentially identical for each ligand, suggesting that the choice of amino acid conjugate does not alter the place exchange process. Table 2 also shows the average occupied surface area for each ligand as calculated from the core diameter and known surface properties of the gold 3-dimensional self-assembled monolayers.
AuNP Stability in high salt and human serum. The UV plasmon band of each prepared AuNP was monitored in sodium chloride solutions from concentrations ranging from 0 to 1-M salt. The known aggregation of citrate-stabilized nanoparticles, indicated by the loss of this band at 520 nm, occurs at around 50 mM NaCl, whereas of AuNPs 12–14 show no loss of UV absorption in this region over the entire salt concentration range (Supplementary Figure S4). Similar behavior was observed in human serum. Equal volumes of AuNPs and human serum were incubated at 37°C. After 24 h, the AuNPs were pelleted by centrifugation, the supernatant discarded and the AuNPs were re-dissolved in MilliQ water and the hydrodynamic diameter measured by DLS. Figure 4 shows very little, if any, change in size distribution after incubation with serum, suggesting that the nanoparticles will maintain their physical properties and not aggregate if used in an intact organism.
2.3 In vitro biological activity
Binding to Galectin-3. As mentioned previously, the binding of Gal-3 to the TFag is an important functional interaction in the metastatic spread of various tumor cell types. Both homotypic adhesion of tumor cells to form clusters and the subsequent binding of these to the endothelium prior to extravasation and tumor spread have both been shown to be mediated by the TFag-Gal-3 interaction (Glinsky et al., 2001; Takenaka et al., 2002; Glinsky et al., 2003; Yu et al., 2007; Zhao et al., 2010; Glinskii et al., 2012; Reticker-Flynn and Bhatia, 2015; Rodriguez et al., 2015). We evaluated our particles in three in vitro assays in binding to Gal-3: 1) aggregation assay, 2) direct binding assay and 3) competitive inhibition assay.
First, Aggregation of Gal-3 by the particles was performed by addition of varying concentrations of Gal-3 to a defined concentration of AuNPs and recording both absorption spectra and DLS size measurements vs. time (Supplementary Figures S5, S6). Aggregation is indicated by an obvious increase in hydrodynamic diameter of the particle solution and a dramatic red shift of the plasmon band in the UV spectrum (Boden et al., 2017) (Otten et al., 2016). Both the serine and threonine-coated AuNPs caused rapid and complete aggregation of Gal-3 where the control particles showed no activity at any concentration, indicating that a multivalent display of the TFag structure is critical and that simply a random display of hydroxyl groups is incapable of causing agglomeration. Plots of absorbance at 700 nm as well as size increase vs. time from DLS data were used to calculate the kinetics of aggregation (Hill function in GraphPad/Prism). The apparent calculated Kd values of aggregation were 116 nM and 101 nM for AuNPs 12 and 13, respectively.
Second, direct binding was measured by simple ELISA where the AuNPs themselves were coated on 96 well plates. A small range of concentrations of Gal-3 were examined and the data is shown in Figure 5. A clear dose response was observed even though the concentrations studied only covered a narrow range (2 μM–4 μM). Both AuNP’s 12 and 13 displayed almost identical binding, while the control AuNPs 14 were completely inactive. The relatively large increase in optical density over this short range of Gal-3 concentrations suggest a multivalent mechanism is operational in this binding event.
Third, we performed a competitive binding assay to assess whether the AuNPs would inhibit the binding of a known ligand for Gal-3 (Bumba et al., 2018). Thus, we examined the behavior of nanomaterials 12–14 as inhibitors of the binding of Gal-3 to asialofetuin (ASF). ASF contains several complex N-linked glycans whose branches are terminated by N-acetyl-lactosamine (LacNAc) units, and hence has been employed previously as a multivalent “carrier” of LacNAc. (Dam et al., 2005). Endogenous LacNAc is considered a natural ligand for many galectin family proteins. Inhibitory studies were carried out by treating ASF-coated 96 well plates with Galectin-3, with or without compounds 12–14; LacNAc was used as a positive control. Figure 6 shows that AuNPs 12 and 13 inhibit the binding of Gal-3 to ASF at low nanomolar concentrations, whereas control AuNPs 14 were again inactive. Inhibition by LacNAc showed a similar trend as our nanoparticles, although at concentrations that were more than 3 orders of magnitude higher, again arguing for the multivalent binding/inhibitory effect of the AuNPs.
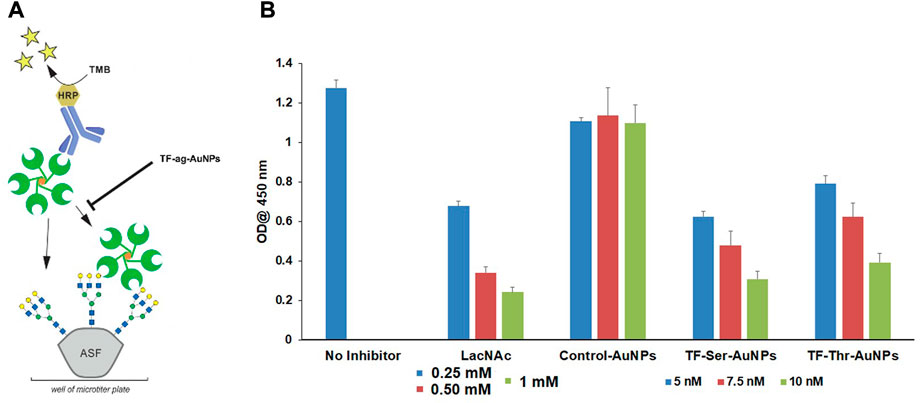
FIGURE 6. Inhibition of Gal-3 binding to ASF by TFag-coated AuNP. (A) Schematic depiction of the assay as shown in reference (Otten et al., 2016). (B) Bar graph depicting inhibition of Gal-3 binding to ASF by compounds materials 12–14.
2.4 Discussion
AuNPs have been prepared in many different guises and used in a variety of biomedical applications for more than two decades. Even with their relatively low toxicity, ability to be made biocompatible and the chemistry available to coat them with any family of molecule, there is yet to be any true AuNPs approved for clinical use in therapeutic arenas such as cancer. Continued research into the development of AuNPs that possess the necessary properties for translation should foster FDA approval of selected metal-based nanomaterials.
In this work we developed a new linker strategy for preparation of AuNP tools for functional studies that hopefully could lead to their use as anticancer therapeutics. The use of lipoic acid has been a mainstay for “dual-pronged” bis-thiol attachment to gold and other metallic surfaces, while “isolipoic” has only been used in one other instance for attachment to a gold surface (Joly et al., 2010). Our reasoning to use of iso-LA in place of LA for the preparation of functional AuNPs was twofold: 1) The achiral nature of iso-LA solves the issue of “contamination” by the unnatural isomer of LA, thus preventing any unwanted immune responses for use in any in vivo setting and 2) Positioning the sidechain in the center of the dithiolane ring produces a linker with a linear directionality from the nanoparticle core, which may relieve any unwanted crowding of ligands like those with LA linkers that protrude from the surface at a distinct angle.
Similar to LA linkers, our TFag ligands were attached more strongly to the AuNP as those with a single thiol end group. Stability studies showed that these particles maintain excellent uniformity under relatively harsh conditions (1.5 M salt, 50% human serum). All physical properties were aligned with well-constructed AuNPs, where solubility and functionality were maintained in all synthetic materials. AuNPs 12–14 were stable for many months at 4°C with minimal signs of flocculation or aggregation. Preliminary bioassay evaluation of these constructs showed binding Gal-3, a lectin that is involved in tumor aggression and metastasis. Inhibition of Gal-3 binding to ASF, a known protein ligand of Gal-3, was also shown to be a function of these AuNPs; the concentrations for this inhibitory effect were much lower than the same level of inhibition by the monomeric disaccharide LacNAc, a known cognate ligand of many galectins. These results suggest that a multivalent effect is operational with these constructs in inhibition of protein-carbohydrate binding. As stated in the introduction, many platforms that display multiple copies of TFag have been prepared and also function through multivalency. We feel that these particles are unique in that 1) Their stability rivals other particles, even those with covalent conjugation of the ligand, 2) Synthesis is relatively simple and high yielding and 3) A host of other ligands from diverse structural families can be attached in to these platforms in a straightforward manner.
These properties prompted us to perform a large in vivo study of AuNPs 12–14 to evaluate their antimetastatic properties in a 4T1 triple negative breast cancer model. Four groups of 14 animals each were treated either with AuNPs 12, 13 or 14 where the fourth group was treated with PBS. While little toxicity was evident in the animals after AuNP treatment, all groups were very similar with respect to survival, lung metastasis and overall health. While the in vitro data suggested some therapeutic effects would translate to antitumor/antimetastatic effects in vivo, this disappointing result suggested that either the AuNPs were simply inactive in this model or the model itself is not suitable for this mode of treatment. We are comprehensively evaluating the biodistribution and in vivo stability of these materials in hopes to achieve therapeutic efficacy in future in vivo studies.
The results herein show that a simple modification of a popular linker can produce AuNPs of excellent stability while maintaining function. Our data show that these reagents are useful for analyzing TFag binding and inhibition, and, when compared to other AuNPs with LA-based or other bifunctional linkers, can offer clues as to the optimum disposition of ligands for usefulness as analytical or therapeutic tools. Comparisons of various in vitro and in vivo bioassays between the present iso-LA-based AuNPs with those based on other linkers is currently in progress.
Data availability statement
The original contributions presented in the study are included in the article/Supplementary Material; further inquiries can be directed to the corresponding author.
Author contributions
UM designed and performed most all experiments, collected characterization data and analyzed experimental data; JB conceived the project, designed and oversaw all experiments and wrote the paper.
Funding
This project has been funded in whole or in part with Federal funds from the National Cancer Institute, National Institutes of Health, under Contract No. HHSN261200800001E.
Acknowledgments
The authors thank James Kelley of the CBL for help obtaining high resolution Mass Spectrometry data and Kunio Nagashima and the Electron Microscopy Laboratory, Frederick National Laboratories for TEM images.
Conflict of interest
The authors declare that the research was conducted in the absence of any commercial or financial relationships that could be construed as a potential conflict of interest.
Publisher’s note
All claims expressed in this article are solely those of the authors and do not necessarily represent those of their affiliated organizations, or those of the publisher, the editors and the reviewers. Any product that may be evaluated in this article, or claim that may be made by its manufacturer, is not guaranteed or endorsed by the publisher.
Supplementary material
The Supplementary Material for this article can be found online at: https://www.frontiersin.org/articles/10.3389/fchem.2022.1002146/full#supplementary-material
References
Amon, R., Reuven, E. M., Ben-Arye, S. L., and Padler-Karavani, V. (2014). Glycans in immune recognition and response. Carbohydr. Res. 389, 115–122. doi:10.1016/j.carres.2014.02.004
Andreana, P. R. (2009). Zwitterionic polysaccharide (ps a1) as an immune elicitor for vaccine development. Abstr. Pap. Am. Chem. S 237, 748.
Awad, L., Madani, R., Gillig, A., Kolympadi, M., Philgren, M., Muhs, A., et al. (2012). A c-linked disaccharide analogue of thomsen-friedenreich epitope induces a strong immune response in mice. Chem. Eur. J. 18, 8578–8582. doi:10.1002/chem.201200364
Baek, M. G., Rittenhouse-Olson, K., and Roy, R. (2001). Synthesis and antibody binding properties of glycodendrimers bearing the tumor related t-antigen. Chem. Commun., 257–258. doi:10.1039/b008664i
Baek, M. G., and Roy, R. (2002). Synthesis and protein binding properties of t-antigen containing glycopamam dendrimers. Bioorg. Med. Chem. 10, 11–17. doi:10.1016/s0968-0896(01)00248-6
Barchi, J. J. (2011). Glyco-nanoparticles as platforms for antitumor therapeutic strategies. Petite Sweet Glyco-Nanotechnology as a Bridge New Med. 1091, 161. doi:10.1021/bk-2011-1091.ch010
Berois, N., Pittini, A., and Osinaga, E. (2022). Targeting tumor glycans for cancer therapy: Successes, limitations, and perspectives. Cancers 14 (3), 645. doi:10.3390/cancers14030645
Biswas, S., Medina, S. H., and Barchi, J. J. (2015). Synthesis and cell-selective antitumor properties of amino acid conjugated tumor-associated carbohydrate antigen-coated gold nanoparticles. Carbohydr. Res. 405, 93–101. doi:10.1016/j.carres.2014.11.002
Boden, S., Wagner, K. G., Karg, M., and Hartmann, L. (2017). Presenting precision glycomacromolecules on gold nanoparticles for increased lectin binding. Polym. (Basel). 9, 716. doi:10.3390/polym9120716
Bourgault, J. P., Trabbic, K. R., Shi, M. C., and Andreana, P. R. (2014). Synthesis of the tumor associative alpha-aminooxy disaccharide of the tf antigen and its conjugation to a polysaccharide immune stimulant. Org. Biomol. Chem. 12, 1699–1702. doi:10.1039/c4ob00128a
Brinas, R. P., Sundgren, A., Sahoo, P., Morey, S., Rittenhouse-Olson, K., Wilding, G. E., et al. (2012). Design and synthesis of multifunctional gold nanoparticles bearing tumor-associated glycopeptide antigens as potential cancer vaccines. Bioconjug. Chem. 23, 1513–1523. doi:10.1021/bc200606s
Brust, M., Fink, J., Bethell, D., Schiffrin, D. J., and Kiely, C. (1995). Synthesis and reactions of functionalised gold nanoparticles. J. Chem. Soc. Chem. Commun., 1655–1656. doi:10.1039/c39950001655
Brust, M., Walker, M., Bethell, D., Schiffrin, D. J., and Whyman, R. (1994). Synthesis of thiol-derivatised gold nanoparticles in a two-phase Liquid–Liquid system. J. Chem. Soc. Chem. Commun. 0, 801–802. doi:10.1039/c39940000801
Bumba, L., Laaf, D., Spiwok, V., Elling, L., Kren, V., and Bojarova, P. (2018). Poly-n-acetyllactosamine neo-glycoproteins as nanomolar ligands of human galectin-3: Binding kinetics and modeling. Int. J. Mol. Sci. 19, 372. doi:10.3390/ijms19020372
Compagno, D., Gentilini, L. D., Jaworski, F. M., Perez, I. G., Contrufo, G., and Laderach, D. J. (2014). Glycans and galectins in prostate cancer biology, angiogenesis and metastasis. Glycobiology 24, 899–906. doi:10.1093/glycob/cwu055
Dabelsteen, E. (1996). Cell surface carbohydrates as prognostic markers in human carcinomas. J. Pathol. 179, 358–369. doi:10.1002/(sici)1096-9896(199608)179:4<358::aid-path564>3.0.co;2-t
Dam, T. K., Gabius, H. J., Andre, S., Kaltner, H., Lensch, M., and Brewer, C. F. (2005). Galectins bind to the multivalent glycoprotein asialofetuin with enhanced affinities and a gradient of decreasing binding constants. Biochemistry 44, 12564–12571. doi:10.1021/bi051144z
Enustun, B. V., and Turkevich, J. (1963). Coagulation of colloidal gold. J. Am. Chem. Soc. 85, 3317–3328. doi:10.1021/ja00904a001
Feizi, T., and Childs, R. A. (1985). Carbohydrate structures of glycoproteins and glycolipids as differentiation antigens, tumour-associated antigens and components of receptor systems. Trends biochem. Sci. 10, 24–29. doi:10.1016/0968-0004(85)90012-x
Feng, D. Y., Shaikh, A. S., and Wang, F. S. (2016). Recent advance in tumor-associated carbohydrate antigens (tacas)-based antitumor vaccines. ACS Chem. Biol. 11, 850–863. doi:10.1021/acschembio.6b00084
Ferguson, K., Yadav, A., Morey, S., Abdullah, J., Hrysenko, G., Eng, J. Y., et al. (2014). Preclinical studies with jaa-f11 anti-thomsen-friedenreich monoclonal antibody for human breast cancer. Future Oncol. 10, 385–399. doi:10.2217/fon.13.209
Flechner, A., Butschak, G., Loffler, A., Ruhmann, J., Nishimura, S. I., Dolling, R., et al. (2019). Cluster binding studies with two anti-thomsen-friedenreich (anti-core-1, cd176, tf) antibodies: Evidence for a multiple tf epitope. Int. Immunopharmacol. 72, 186–194. doi:10.1016/j.intimp.2019.03.058
Franco, A. (2008). Glycoconjugates as vaccines for cancer immunotherapy: Clinical trials and future directions. Anticancer. Agents Med. Chem. 8, 86–91. doi:10.2174/187152008783330888
Gaidzik, N., Westerlind, U., and Kunz, H. (2013). The development of synthetic antitumour vaccines from mucin glycopeptide antigens. Chem. Soc. Rev. 42, 4421–4442. doi:10.1039/c3cs35470a
Gao, X. G., Zhi, Y., Zhang, T., Xue, H. T., Wang, X., Foday, A. D., et al. (2012). Analysis of the neutral polysaccharide fraction of mcp and its inhibitory activity on galectin-3. Glycoconj. J. 29, 159–165. doi:10.1007/s10719-012-9382-5
Glinskii, O. V., Li, F., Wilson, L. S., Barnes, S., Rittenhouse-Olson, K., Barchi, J. J., et al. (2014). Endothelial integrin alpha 3 beta 1 stabilizes carbohydrate-mediated tumor/endothelial cell adhesion and induces macromolecular signaling complex formation at the endothelial cell membrane. Oncotarget 5, 1382–1389. doi:10.18632/oncotarget.1837
Glinskii, O. V., Sud, S., Mossine, V. V., Mawhinney, T. P., Anthony, D. C., Glinsky, G. V., et al. (2012). Inhibition of prostate cancer bone metastasis by synthetic tf antigen mimic/galectin-3 inhibitor lactulose-l-leucine. Neoplasia 14, 65–73. doi:10.1593/neo.111544
Glinsky, V. V., Glinsky, G. V., Glinskii, O. V., Huxley, V. H., Turk, J. R., Mossine, V. V., et al. (2003). Intravascular metastatic cancer cell homotypic aggregation at the sites of primary attachment to the endothelium. Urologic Oncol. Seminars Orig. Investigations 63, 80–3811. doi:10.1016/j.urolonc.2003.12.009
Glinsky, V. V., Glinsky, G. V., Rittenhouse-Olson, K., Huflejt, M. E., Glinskii, O. V., Deutscher, S. L., et al. (2001). The role of thomsen-friedenreich antigen in adhesion of human breast and prostate cancer cells to the endothelium. Cancer Res. 61, 4851
Glinsky, V. V., Huflejt, M. E., Glinsky, G. V., Deutscher, S. L., and Quinn, T. P. (2000). Effects of thomsen-friedenreich antigen-specific peptide p-30 on beta-galactoside-mediated homotypic aggregation and adhesion to the endothelium of mda-mb-435 human breast carcinoma cells. Cancer Res. 60, 2584
Glinsky, V. V. (2006). Intravascular cell-to-cell adhesive interactions and bone metastasis. Cancer Metastasis Rev. 25, 531–540. doi:10.1007/s10555-006-9029-8
Goraca, A., Huk-Kolega, H., Piechota, A., Kleniewska, P., Ciejka, E., and Skibska, B. (2011). Lipoic acid - biological activity and therapeutic potential. Pharmacol. Rep. 63, 849–858. doi:10.1016/s1734-1140(11)70600-4
Guha, P., Kaptan, E., Bandyopadhyaya, G., Kaczanowska, S., Davila, E., Thompson, K., et al. (2013). Cod glycopeptide with picomolar affinity to galectin-3 suppresses t-cell apoptosis and prostate cancer metastasis. Proc. Natl. Acad. Sci. U. S. A. 110, 5052–5057. doi:10.1073/pnas.1202653110
Guo, Z. W., and Wang, Q. L. (2009). Recent development in carbohydrate-based cancer vaccines. Curr. Opin. Chem. Biol. 13, 608–617. doi:10.1016/j.cbpa.2009.08.010
Hakomori, S. I. (1991). New directions in cancer-therapy based on aberrant expression of glycosphingolipids - antiadhesion and ortho-signaling therapy. Cancer cells. 3, 461
Hauselmann, I., and Borsig, L. (2014). Altered tumor-cell glycosylation promotes metastasis. Front. Oncol. 4, 28. doi:10.3389/fonc.2014.00028
Heimburg, J., Yan, J., Morey, S., Glinskii, O. V., Huxley, V. H., Wild, L., et al. (2006). Inhibition of spontaneous breast cancer metastasis by anti-thomsen-friedenreich antigen monoclonal antibody jaa-f11. Neoplasia 8, 939–948. doi:10.1593/neo.06493
Hoffmann, M., Hayes, M. R., Pietruszka, J., and Elling, L. (2020). Synthesis of the thomsen-friedenreich-antigen (tf-antigen) and binding of galectin-3 to tf-antigen presenting neo-glycoproteins. Glycoconj. J. 37, 457–470. doi:10.1007/s10719-020-09926-y
Jeschke, U., Karsten, U., Wiest, I., Schulze, S., Kuhn, C., Friese, K., et al. (2006). Binding of galectin-1 (gal-1) to the thomsen-friedenreich (tf) antigen on trophoblast cells and inhibition of proliferation of trophoblast tumor cells in vitro by gal-1 or an anti-tf antibody. Histochem. Cell Biol. 126, 437–444. doi:10.1007/s00418-006-0178-1
Jeschke, U., Mayr, D., Schiessl, B., Mylonas, I., Schulze, S., Kuhn, C., et al. (2007). Expression of galectin-1, -3 (gal-1, gal-3) and the thomsen-friedenreich (tf) antigen in normal, iugr, preeclamptic and hellp placentas. Placenta 28, 1165–1173. doi:10.1016/j.placenta.2007.06.006
Jin, K. T., Lan, H. R., Chen, X. Y., Wang, S. B., Ying, X. J., Lin, Y., et al. (2019). Recent advances in carbohydrate-based cancer vaccines. Biotechnol. Lett. 41, 641–650. doi:10.1007/s10529-019-02675-5
Jin, Z. C., Kapur, A., Wang, W. T., and Mattoussi, H. (2020). Lipoic acid as anchoring groups and reactive sites on nanoparticles coated with multi-coordinating polymers. Proc. Spie 11255, 112550S. doi:10.1117/12.2543992
Johannes, M., Reindl, M., Gerlitzki, B., Schmitt, E., and Hoffmann-Roder, A. (2015). Synthesis and biological evaluation of a novel muc1 glycopeptide conjugate vaccine candidate comprising a 4'-deoxy-4'-fluoro-thomsen-friedenreich epitope. Beilstein J. Org. Chem. 11, 155–161. doi:10.3762/bjoc.11.15
John, C. M., Leffler, H., Kahl-Knutsson, B., Svensson, I., and Jarvis, G. A. (2003). Truncated galectin-3 inhibits tumor growth and metastasis in orthotopic nude mouse model of human breast cancer. Clin. Cancer Res. 9, 2374
Joly, K. M., Mirri, G., Willener, Y., Horswell, S. L., Moody, C. J., and Tucker, J. H. R. (2010). Synthesis of an achiral isomer of lipoic acid as an anchor group for sam formation on gold surfaces. J. Org. Chem. 75, 2395–2398. doi:10.1021/jo9024545
Karacosta, L. G., Fisk, J. C., Jessee, J., Tati, S., Turner, B., Ghazal, D., et al. (2018). Preclinical analysis of jaa-f11, a specific anti-thomsen-friedenreich antibody via immunohistochemistry and in vivo imaging. Transl. Oncol. 11, 450–466. doi:10.1016/j.tranon.2018.01.008
Khaldoyanidi, S. K., Glinsky, V. V., Sikora, L., Glinskii, A. B., Mossine, V. V., Quinn, T. P., et al. (2003). Mda-mb-435 human breast carcinoma cell homo- and heterotypic adhesion under flow conditions is mediated in part by thomsen-friedenreich antigen-galectin-3 interactions. J. Biol. Chem. 278, 4127–4134. doi:10.1074/jbc.m209590200
Kleski, K. A., Trabbic, K. R., Shi, M. C., Bourgault, J. P., and Andreana, P. R. (2020). Enhanced immune response against the thomsen-friedenreich tumor antigen using a bivalent entirely carbohydrate conjugate. Molecules, 1319. doi:10.3390/molecules25061319
Koufaki, M., Detsi, A., and Kiziridi, C. (2009). Multifunctional lipoic acid conjugates. Curr. Med. Chem. 16, 4728–4742. doi:10.2174/092986709789878274
Liu, C. C., and Ye, X. S. (2012). Carbohydrate-based cancer vaccines: Target cancer with sugar bullets. Glycoconj. J. 29, 259–271. doi:10.1007/s10719-012-9399-9
Luo, Z., Hou, J., Menin, L., Ong, Q. K., and Stellacci, F. (2017). Evolution of the ligand shell morphology during ligand exchange reactions on gold nanoparticles. Angew. Chem. Int. Ed. Engl. 56, 13706–13710. doi:10.1002/ange.201708190
Newton-Northup, J. R., Dickerson, M. T., Ma, L. X., Besch-Williford, C. L., and Deutscher, S. L. (2013). Inhibition of metastatic tumor formation in vivo by a bacteriophage display-derived galectin-3 targeting peptide. Clin. Exp. Metastasis 30, 119–132. doi:10.1007/s10585-012-9516-y
Nicotra, F., Gabrielli, L., Bini, D., Russo, L., Sgambato, A., and Cipolla, L. (2014). Multivalent glycidic constructs toward anti-cancer therapeutics. Spr. Carb Ch. 40, 491. doi:10.1039/9781849739986-00491
Otten, L., Vlachou, D., Richards, S.-J., and Gibson, M. I. (2016). Glycan heterogeneity on gold nanoparticles increases lectin discrimination capacity in label-free multiplexed bioassays. Analyst 141, 4305–4312. doi:10.1039/c6an00549g
Park, J.-S., Vo, A. N., Barriet, D., Shon, Y.-S., and Lee, T. R. (2005). Systematic control of the packing density of self-assembled monolayers using bidentate and tridentate chelating alkanethiols. Langmuir 21, 2902–2911. doi:10.1021/la0475573
Peletskaya, E. N., Glinsky, V. V., Glinsky, G. V., Deutscher, S. L., and Quinn, T. P. (1997). Characterization of peptides that bind the tumor-associated thomsen-friedenreich antigen selected from bacteriophage display libraries. J. Mol. Biol. 270, 374–384. doi:10.1006/jmbi.1997.1107
Poiroux, G., Barre, A., van Damme, E. J. M., Benoist, H., and Rouge, P. (2017). Plant lectins targeting o-glycans at the cell surface as tools for cancer diagnosis, prognosis and therapy. Int. J. Mol. Sci. 18, 1232. doi:10.3390/ijms18061232
Reticker-Flynn, N. E., and Bhatia, S. N. (2015). Aberrant glycosylation promotes lung cancer metastasis through adhesion to galectins in the metastatic niche. Cancer Discov. 5, 168–181. doi:10.1158/2159-8290.cd-13-0760
Rittenhouse-Olson, K. (2007). Jaa-f11-Extending the life of mice with breast cancer. Expert Opin. Biol. Th 7, 923–928. doi:10.1517/14712598.7.7.923
Rodriguez, M. C., Yegorova, S., Pitteloud, J. P., Chavaroche, A. E., Andre, S., Arda, A., et al. (2015). Thermodynamic switch in binding of adhesion/growth regulatory human galectin-3 to tumor-associated tf antigen (cd176) and muc1 glycopeptides. Biochemistry 54, 4462–4474. doi:10.1021/acs.biochem.5b00555
Roy, R., and Baek, M. G. (2003). Multivalent breast cancer t-antigen markers scaffolded onto pamam dendrimers. Methods Enzymol. 362, 240–249. doi:10.1016/S0076-6879(03)01017-6
Santarsia, S., Grosso, A. S., Trovao, F., Jimenez-Barbero, J., Carvalho, A. L., Nativi, C., et al. (2018). Molecular recognition of a thomsen-friedenreich antigen mimetic targeting human galectin-3. Chemmedchem 13, 2030–2036. doi:10.1002/cmdc.201800525
Slovin, S. F., Ragupathi, G., Musselli, C., Fernandez, C., Diani, M., Verbel, D., et al. (2005). Thomsen-friedenreich (tf) antigen as a target for prostate cancer vaccine: Clinical trial results with tf cluster (c)-klh plus qs21 conjugate vaccine in patients with biochemically relapsed prostate cancer. Cancer Immunol. Immunother. 54, 694–702. doi:10.1007/s00262-004-0598-5
Son, H. Y., Apostolopoulos, V., and Kim, C. W. (2016). T/tn immunotherapy avoiding immune deviation. Int. J. Immunopathol. Pharmacol. 29, 812–817. doi:10.1177/0394632016674018
Sun, S., Zheng, X. J., Huo, C. X., Song, C. C., Li, Q., and Ye, X. S. (2016). Synthesis and evaluation of glycoconjugates comprising n-acyl-modified thomsen-friedenreich antigens as anticancer vaccines. Chemmedchem 11, 1090–1096. doi:10.1002/cmdc.201600094
Sundgren, A., and Barchi, J. J. (2008). Varied presentation of the thomsen-friedenreich disaccharide tumor-associated carbohydrate antigen on gold nanoparticles. Carbohydr. Res. 343, 1594–1604. doi:10.1016/j.carres.2008.05.003
Svarovsky, S. A., Szekely, Z., and Barchi, J. J. (2005). Synthesis of gold nanoparticles bearing the thomsen-friedenreich disaccharide: A new multivalent presentation of an important tumor antigen. Tetrahedron Asymmetry 16, 587–598. doi:10.1016/j.tetasy.2004.12.003
Takenaka, Y., Fukumori, T., and Raz, A. (2002). Galectin-3 and metastasis. Glycoconj. J. 19, 543–549. doi:10.1023/b:glyc.0000014084.01324.15
Tantivejkul, K., Kalikin, L. M., and Pienta, K. J. (2004). Dynamic process of prostate cancer metastasis to bone. J. Cell. Biochem. 91, 706–717. doi:10.1002/jcb.10664
Tati, S., Fisk, J. C., Abdullah, J., Karacosta, L., Chrisikos, T., Philbin, P., et al. (2017). Humanization of jaa-f11, a highly specific anti-thomsen-friedenreich pancarcinoma antibody and in vitro efficacy analysis. Neoplasia 19, 716–733. doi:10.1016/j.neo.2017.07.001
Toyokuni, T., and Singhal, A. K. (1995). Synthetic carbohydrate vaccines based on tumour-associated antigens. Chem. Soc. Rev. 24, 231. doi:10.1039/cs9952400231
Trabbic, K. R., Bourgault, J. P., Shi, M. C., Clark, M., and Andreana, P. R. (2016). Immunological evaluation of the entirely carbohydrate-based thomsen-friedenreich - ps b conjugate. Org. Biomol. Chem. 14, 3350–3355. doi:10.1039/c6ob00176a
Trabbic, K. R., Whalen, K., Abarca-Heideman, K., Xia, L., Temme, J. S., Edmondson, E. F., et al. (2019). A tumor-selective monoclonal antibody from immunization with a tumor-associated mucin glycopeptide. Sci. Rep. 9, 5662. doi:10.1038/s41598-019-42076-2
Turkevich, J., Stevenson, P. C., and Hillier, J. (1951). A study of the nucleation and growth processes in the synthesis of colloidal gold. Discuss. Faraday Soc. 55.
Ulsemer, P., Henderson, G., Toutounian, K., Loffler, A., Schmidt, J., Karsten, U., et al. (2013). Specific humoral immune response to the thomsen-friedenreich tumor antigen (cd176) in mice after vaccination with the commensal bacterium bacteroides ovatus d-6. Cancer Immunol. Immunother. 62, 875–887. doi:10.1007/s00262-013-1394-x
Wei, M. M., Wang, Y. S., and Ye, X. S. (2018). Carbohydrate-based vaccines for oncotherapy. Med. Res. Rev. 38, 1003–1026. doi:10.1002/med.21493
Wojczykowski, K., Meißner, D., Jutzi, P., Ennen, I., Hütten, A., Fricke, M., et al. (2006). Reliable stabilization and functionalization of nanoparticles through tridentate thiolate ligands. Chem. Commun., 3693–3695. doi:10.1039/b606360h
Wu, X. J., McFall-Boegeman, H., Rashidijahanabad, Z., Liu, K., Pett, C., Yu, J., et al. (2021). Synthesis and immunological evaluation of the unnatural beta-linked mucin-1 thomsen-friedenreich conjugate. Org. Biomol. Chem. 19, 2448–2455. doi:10.1039/d1ob00007a
Wu, X. J., McKay, C., Pett, C., Yu, J., Schorlemer, M., Ramadan, S., et al. (2019). Synthesis and immunological evaluation of disaccharide bearing muc-1 glycopeptide conjugates with virus-like particles. ACS Chem. Biol. 14, 2176–2184. doi:10.1021/acschembio.9b00381
Xin, M., Dong, X. W., and Guo, X. L. (2015). Role of the interaction between galectin-3 and cell adhesion molecules in cancer metastasis. Biomed. Pharmacother. 69, 179–185. doi:10.1016/j.biopha.2014.11.024
Xu, Y., Sette, A., Sidney, J., Gendler, S. J., and Franco, A. (2005). Tumor-associated carbohydrate antigens: A possible avenue for cancer prevention. Immunol. Cell Biol. 83, 440–448. doi:10.1111/j.1440-1711.2005.01347.x
Yeh, C.-M., Chen, M.-C., Wu, T.-C., Chen, J.-W., and Lai, C.-H. (2021). Lectin-triggered aggregation of glyco-gold nanoprobes for activity-based sensing of hydrogen peroxide by the naked eye. Chem. Asian J. 16, 3462–3468. doi:10.1002/asia.202100865
Yi, B., Zhang, Z., Zhang, M., Schwartz-Albiez, R., and Cao, Y. (2013). Cd176 antiserum treatment leads to a therapeutic response in a murine model of leukemia. Oncol. Rep. 30, 1841–1847. doi:10.3892/or.2013.2639
Yin, Z. J., and Huang, X. F. (2012). Recent development in carbohydrate based anticancer vaccines. J. Carbohydr. Chem. 31, 143–186. doi:10.1080/07328303.2012.659364
Yu, L. G., Andrews, N., Zhao, Q., McKean, D., Williams, J. F., Connor, L. J., et al. (2007). Galectin-3 interaction with thomsen-friedenreich disaccharide on cancer-associated muc1 causes increased cancer cell endothelial adhesion. J. Biol. Chem. 282, 773–781. doi:10.1074/jbc.m606862200
Keywords: Thomsen-Friedenreich antigen, tumor-associated carbohydrates, gold nanoparticles, galectin-3, multivalency
Citation: Mondal UK and Barchi JJ (2022) Isolipoic acid-linked gold nanoparticles bearing the thomsen friedenreich tumor-associated carbohydrate antigen: Stability and in vitro studies. Front. Chem. 10:1002146. doi: 10.3389/fchem.2022.1002146
Received: 24 July 2022; Accepted: 20 September 2022;
Published: 10 October 2022.
Edited by:
Keith Stine, University of Missouri–St. Louis, United StatesReviewed by:
Boris Vauzeilles, Institut de Chimie des Substances Naturelles CNRS-ICSN, FranceChian-Hui Lai, National Chung Hsing University, Taiwan
Copyright © 2022 Mondal and Barchi. This is an open-access article distributed under the terms of the Creative Commons Attribution License (CC BY). The use, distribution or reproduction in other forums is permitted, provided the original author(s) and the copyright owner(s) are credited and that the original publication in this journal is cited, in accordance with accepted academic practice. No use, distribution or reproduction is permitted which does not comply with these terms.
*Correspondence: Joseph J. Barchi, barchij@mail.nih.gov