Tolerance to Some ACCase Inhibitors in Four Common Roegneria (Roegneria kamoji) Populations From China
- 1State Key Laboratory of Rice Biology, China National Rice Research Institute, Hangzhou, China
- 2School of Forestry and Bio-Technology, Zhejiang A & F University, Hangzhou, China
- 3Jingzhou Academy of Agricultural Sciences, Jingzhou, China
Common roegneria, a perennial weed in Roegneria genera of the tribe Triticeae (family: Poaceae), is widely distributed in China and could not be effectively controlled by acetyl coA carboxylase (ACCase) inhibitors in wheat in some regions. This study aimed to quantify herbicide tolerance level and investigate its mechanisms of ACCase tolerance in common roegneria. Whole-plant dose–response assay indicated that populations ZJJX and HBJZ (collected in wheat fields) exhibited high tolerance [ED50s >16-fold labeled field rate (LFR)] to fenoxaprop-P-ethyl and clodinafop-propargyl [aryloxyphenoxypropionate (APPs)] and pinoxaden (phenylpyrazolin). In addition, two populations collected from uncultivated areas showed similar responses to these herbicides. All common roegneria populations were susceptible to haloxyfop-R-methyl and quizalofop-p-ethyl (APPs), clethodim, and sethoxydim (cyclohexanediones) (ED50s <1/2-fold LFR). The responses to ACCase inhibitors of common roegneria were in complete accord with wheat. ACCase sequencing revealed that the APP tolerance was not conferred by known amino acid substitutions. The sensitivity of common roegneria populations to fenoxaprop-P-ethyl could not be reduced by metabolic inhibitors malathion and 4-chloro-7-nitro-benzoxadiazole. In vitro ACCase enzyme activity assays revealed that the activities of ACCase were increased in ZJJX, ZJHZ populations and wheat after fenoxaprop-P-ethyl treatment, which at 72 h after treatment (HAT) was 1.46-, 1.39-, and 1.34-fold higher than that at 0 HAT, respectively. To our knowledge, this study reported for the first time the natural tolerance to ACCase inhibitors in common roegneria. The enhanced ACCase activity suggested that rapid metabolism of the herbicide might play an important role in the tolerance mechanism of this weed. Rotated with other crops (i.e., oilseed rape) to use different herbicides could serve as important tools for managing common roegneria in wheat.
Introduction
Common roegneria (Roegneria kamoji Ohwi) is a perennial grass belonging to the genus Roegneria of the Poaceae family. It is widespread in China, Korea, and Japan (GBIF; http://www.gbif.org). Common roegneria is commonly found in field borders, roadside, hillside, urban green spaces, and vegetable nurseries, but also commonly occurs as a weed in wheat fields (Li, 1998; Xu et al., 2014). Common roegneria first bloomed in April, with seed mature and dispersal occurring in May to June, and it relies on both seed and rhizome to sustain and renew its populations. It is used for conservation of water and soil or used as forage and possesses potentially valuable traits including wheat improvement (Chang et al., 2011; Zhao et al., 2017b). Since 2016, common roegneria has become a predominant and serious weed in two farms and could not be effectively controlled by fenoxaprop-P-ethyl or clodinafop-propargyl even over the labeled field rates (LFRs) in China (Supplementary Figure 1).
Acetyl coA carboxylase (ACCase, EC 6.4.1.2), catalyzing carboxylation of acetyl-CoA to malonyl-CoA in a multistep reaction, is involved in the first committed step in fatty acid biosynthesis. In plants, two different types of ACCase enzyme were identified, namely, cytoplasmic and plastidic, and the latter contributes up to 80% of the enzyme activity in grasses (Preston and Mallory-Smith, 2001). ACCase-inhibiting herbicides (hereafter referred to as ACCase inhibitors), which inhibit the plastidic ACCase enzyme activity, results in fatty acid depletion, leading to rapid cell death due to membrane dysfunction (Harwood, 1988; Devine, 1997). They have been widely used for grass weeds control in various crops. All ACCase isoforms is composed of three catalytic domains, the biotin carboxyl-carrier, the biotin carboxylase, and the carboxyl transferase (CT) domains. The CT domain is the binding site of widely used ACCase inhibitors (Sasaki et al., 1995; Délye et al., 2005). ACCase inhibitors can be classified into three classes, namely, the aryloxyphenoxypropionates (APPs), cyclohexanediones (CHDs), and phenylpyrazoline (DENs), based on their chemical structures (Wenger et al., 2012). There are many cases of weed populations not effectively controlled by ACCase inhibitors after continuous application due to the evolution of herbicide resistance. To date, biotypes of 48 weed species worldwide and eight throughout China have been documented resistant to ACCase inhibitors (Heap, 2020).
The mechanisms of resistance to ACCase inhibitors may be divided into two major types: target-site resistance (TSR) and non–target-site resistance (NTSR). In many cases, resistance to ACCase inhibitors is due to the presence of an altered target site, typically resulting from a single amino acid substitution in the CT domain that makes the ACCase insensitive to herbicides (Délye et al., 2005; Powles and Yu, 2010). To date, 15 types of amino acid substitutions have been identified at seven different codon positions in the ACCase CT domain: Ile-1781-Val, Leu, Thr, or Gla; Trp1999-Cys, Leu, or Ser; Trp2027-Cys; Ile2041-Asn, Val or Thr; Asn2078-Gly; Cys2088-Arg; and Gly2096-Ala or Ser (Kukorelli et al., 2013; Kaundun, 2014; Guo et al., 2017). Researchers generally describe the amino acid substitutions in the CT domain of ACCase based on the CT domain of blackgrass (Alopecurus myosuroides Huds.) accession AJ310767.
NTSR involves any mechanisms that minimize the amount of active herbicide ingredient reaching the target site (for example, reduced penetration, altered patterns of translocation, sequestration, and enhanced detoxification of the toxophores). To date, increased metabolic rate in response to herbicides has been considered the main NTSR mechanism (Délye et al., 2011). A variety of enzymes are involved in NTSR, of which cytochrome P450 mixed-function oxidases (CytP450) and glutathione S-transferase (GST) and/or glycosyl-transferase have been confirmed to cause enhanced herbicide metabolism in several grass weed species (Cummins et al., 1997; Busi et al., 2011; Ghanizadeh and Harrington, 2017). Several CytP450 inhibitors, such as piperonyl butoxide and malathion, and the GST inhibitor 4-chloro-7-nitro-benzoxadiazole (NBD-Cl), have long been used as indicators of CytP450 or GST involvement in metabolic resistance to ACCase inhibitors (Cummins et al., 2013; Zhao et al., 2017a).
Natural tolerance of some cereal crops, such as barley (Hordeum vulgare) and wheat (Triticum aestivum), is due to a less sensitive ACCase enzyme or a higher rate of metabolic degradation (Cataneo et al., 2013). Previous reported grass weeds resistance to ACCase inhibitors has 99% identity to A. myosuroides and 95% identity to T. aestivum in the CT domain. As a wild relative of wheat, it is hypothesized that common roegneria may have similar response to ACCase inhibitors.
In this study, two common roegneria populations known to have survived applications of fenoxaprop-P-ethyl at its LFRs were collected from winter wheat fields, and two populations were collected from wastelands with no herbicide applied history. We aimed to (1) quantify the tolerance level to various ACCase inhibitors in the four common roegneria populations and (2) characterize the potential mechanisms of tolerance to ACCase inhibitors.
Materials and Methods
Plant Materials and Growth Conditions
Seeds of two populations (HBJZ and ZJJX) that were not effectively controlled by fenoxaprop-P-ethyl were collected from wheat fields in Jingzhou City, Hubei Province, and Jiaxing City, Zhejiang Province, in June 2017, respectively (Table 1). These fields were wheat-rice rotations during the last 5 years and had been treated with ACCase inhibitors for grass weeds control in the wheat cropping season. Two populations (HNHY and ZJHZ) were collected from uncultivated wastelands that had never been treated with herbicides in Hengyang City, Hunan Province, and Hangzhou City, Zhejiang Province, in June 2017, respectively. The collected seeds were cleaned, dried at room temperature (20°C ± 5°C) and kept in paper bags until used. A local wheat cultivar (Yangmai 25) was included as a reference material for this study.
The plant seedlings were cultivated as described in Tang et al. (2014) with minor modification. Briefly, seeds of the four common roegneria populations and wheat were germinated in 9-cm Petri dishes containing moistened double layers of filter paper (Double Ring #102, Hangzhou Special Paper Industry Co. Ltd., China). Petri dishes were sealed with Parafilm (American National Can, Greenwich, CT) to prevent evaporation and then cultivated in a germination cabinet (GXZ-300C, Dongnan Instrument Manufacture, Ningbo, Zhejiang, China) at fluctuating day/night temperatures (25°C/15°C) and 80% relative humidity. Dishes were inspected daily, and germinated seeds (with 5-mm coleoptile) were transplanted into 9-cm-diameter plastic pots containing a suitable growing medium (1:1:1:2 vegetable garden soil/compost/peat/dolomite) and grown at ~20°C under natural conditions. There were four uniform seedlings in each pot, and the pots were watered as required.
Tolerance to ACCase Inhibitors Assay
Experiments were conducted in a screenhouse (a 6 × 40-m chamber framed with 2-cm iron mesh and covered overhead with a transparent plastic cover to prevent rain damage) during 2017 and 2018 at the China National Rice Research Institute (CNRRI, 30°04′N, 119°55′E), Hangzhou, Zhejiang Province, China. At the three-leaf growth stage, plants were sprayed with the commercial formulations of ACCase inhibitors in a compressed air laboratory spray tower equipped with a flat fan nozzle (Teejet TP6501E), which delivered 200 L ha−1 solution at 0.21 MPa. After herbicide application, the plants were returned to the screenhouse described above. The aboveground parts of the plant were harvested at 21 days after treatment (DAT) for fresh biomass determination. Fresh weight was expressed as a percentage of the untreated control to standardize comparisons between populations. The experiment was conducted with four pots and was conducted twice. A total of seven ACCase inhibitors were applied in this study and the details are given below (Table 2). The herbicide treatments were determined based on a preliminary study (data not shown) treated at the LFRs of each herbicide.
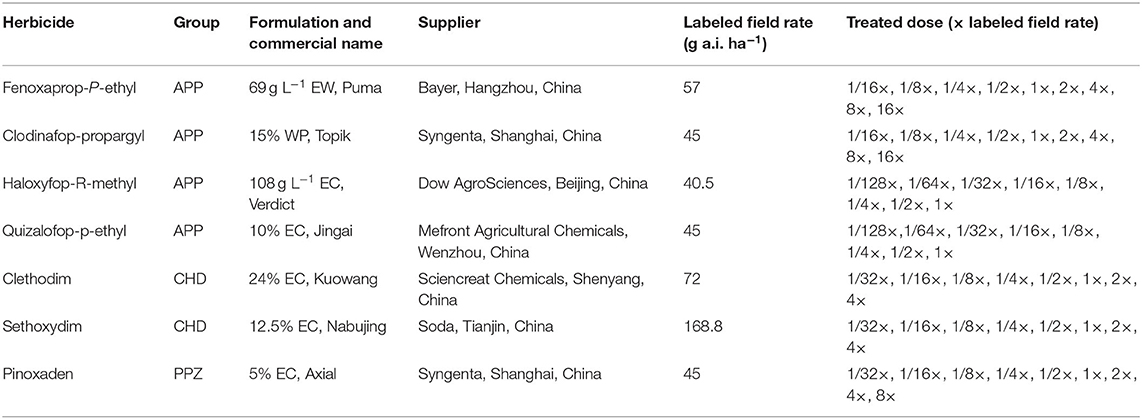
Table 2. Detailed information of herbicides, labeled field rates, and treated doses in the whole-plant dose–response studies.
ACCase Gene Sequencing
The leaf tissues (100 mg) were collected from five individuals of the four common roegneria populations at three- to four-leaf stage, and their genomic DNA was extracted using Plant Genomics DNA secure Plant Kit (DP320, Tiangen Biotech Co. Ltd., Beijing, China) based on the protocol from the manufacturer. The primers (ACCase-F: CTCCTGAATTTCCCAGCGGCAGACAGAT; ACCase-R CCCTTGAGGTTCGAGAACATTACCCTTT) were designed by Primer Premier 5.0, based on the ACCase gene sequence of A. myosuroides (AJ310767). The PCR products were detected with 1% agarose gel. The desired bands were extracted by AxyPrep DNA Gel Extraction Kit (Axygene, US) and cloned into a pMD19-T vector (Takara Biotech, China). The recombinant plasmids were transformed into competent Escherichia coli JM109 (Takara Biotech, China). The positive clones were sequenced by Shanghai Biosune Biological Technology and Services Co., Ltd. (China). At least three clones of each sample were selected for sequencing, and the DNA sequences were translated into protein and compared with ACCase CT domain of A. myosuroides and T. aestivum (ACD46685.1) by BioEdit software.
Synergistic Effect of Malathion and NBD-Cl
In 2017 and 2018, seeds of two common roegneria populations (ZJJX from wheat field and ZJHZ from uncultivated area) and wheat were planted as described above in plastic pots. Half of the seedlings at three-leaf growth stage were treated with malathion at 1,000 g ha−1, 30 min before herbicide application. Fenoxaprop-P-ethyl was sprayed and followed the same doses as described in Table 2. For GST inhibitor experiments, three-leaf growth stage seedlings were treated with 80 g ha−1 NBD-Cl plus 0.125% (vol/vol) non-ionic surfactant 48 h before fenoxaprop-P-ethyl applied at doses same as in Table 2. The aboveground plant material was harvested at 21 DAT, and the fresh weights were measured and expressed as a percent of the control group.
In vitro Assay of ACCase and GST Activity to Fenoxaprop-P-ethyl
Seedlings of two common roegneria populations (ZJJX and ZJHZ) and wheat were cultivated as described above. At three-leaf growth stage, the seedlings were sprayed with fenoxaprop-P-ethyl at 57 g a.i. ha−1 and fresh leaf tissues were collected at 24, 48, and 72 h after treatment (HAT). The untreated samples were also collected as control. To test the ACCase activity variation between the four common roegneria populations and wheat after herbicide treatment, 2 g leaf tissues were homogenized with liquid nitrogen and suspended with extract buffer [100 mmol/L Tris-HCl, (pH 8.3), 300 mmol/L glycerinum, 5 mmol/L DTT, 2 mmol/L EDTA, 0.5 mmol/L PMSF, and 0.01% (vol/vol) Triton X-100] and centrifuged at 1,500 g for 10 min at 4°C. The protein concentration in the enzyme extracts were measured by Bradford method (Kruger, 2002). The ACCase activity assay was performed with the Plant ACCase ELISA Kit (Meimian, Jiangsu, China) using the manufacturer's protocol. The activity unit (U/min/mg leaf protein) of ACCase was defined as the amount of the enzyme that catalyzes the conversion of μmol of substrate per minute per mg protein. To determine whether the GST is involved in the tolerance of common roegneria, 0.2 g leaf tissues were homogenized with liquid nitrogen and suspended with 0.8 mL extraction buffer (1 × phosphate-buffered saline, pH 7.4). The homogenates were stirred for 10 min on ice and centrifuged at 10,000 g for 5 min at 4°C. The supernatant was immediately used for GST activity and protein concentration assay. The protein concentration in the enzyme extracts were measured by Bradford method (Kruger, 2002). The GST activity assay was performed with the GSH–(ST) Detection Kit (Jiancheng, China). The activity unit (U/min/mg leaf protein) of GST was defined as the amount of the enzyme that catalyzes the conversion of μmol of substrate per minute per mg protein. Each treatment had five replications, and the whole experiment was conducted twice.
Statistical Analysis
All experiments were conducted using a completely randomized design with four replications. The data from the repeated runs were subjected to analysis of variance using general linear model procedure in SPSS software, version 13.0 (SPSS, Chicago, IL). No statistically significant (P > 0.05) trial by treatment interaction was revealed, so the data of the repeated experiments were pooled for analysis. Data of the plant fresh biomass were analyzed by fitting with a log-logistic function (Equation 1) (Seefeldt et al., 1995) using Origin 8.0 (OriginLab Corp. Northampton, MA). The function fitted was:
where C is the lower response limit, D is the upper response limit, x is the herbicide application dose, b is the slope of the curve through ED50, and y is the response at the herbicide dose x. In this study, ED50 is the effective dose of herbicide resulting in 50% growth inhibition. As no susceptible populations were detected in a preliminary experiment, the tolerance index (TI) was calculated or estimated by the ratio of ED50 between the common roegneria populations and the LFR of the herbicide.
Data obtained from GST activity assay were subjected to analysis of variance by using SPSS software, version 13.0 (SPSS, Chicago, IL). Mean comparison was performed using Fisher protected least significant difference (LSD) test, where the overall differences were significant (P ≤ 0.05).
Results and Discussion
Whole-Plant Dose–Response to ACCase Inhibitors
As expected, all the plants of ZJJX and HBJZ collected from wheat fields survived the fenoxaprop-P-ethyl treatments at 21 DAT during both experimental runs (2017 and 2018). Spot yellowing was observed on the spear leaves of these two populations of the 4- to 16-fold LFR treatments at 10 DAT; however, the symptoms disappeared gradually, and no visual phytotoxicity symptoms were observed after 15 DAT. However, populations of ZJHZ and HNHY, which were collected from areas without herbicide application history, showed the same response pattern to fenoxaprop-P-ethyl. Results of the whole-plant dose–response assay showed a high level of tolerance in all the four populations, with ED50s >16-fold LFR to fenoxaprop-P-ethyl (Table 3). A similar response trend was observed in wheat, which survival or fresh weight biomass was not affected at 4-fold LFR of fenoxaprop-P-ethyl. The TIs of the common roegneria populations and wheat were >16. The ED50 of a susceptible Alopecurus japonicas population collected from the same field of HBJZ tested at the same period was 5.2 g a.i. ha−1, which was 1/10-fold LFR (data not shown). From this point of view, the common roegneria populations were highly tolerant to fenoxaprop-P-ethyl. Similarly, all the common roegneria populations were highly tolerant to clodinafop-propargyl (TI > 16) and pinoxaden (TI > 8) in both experimental runs (Table 3).
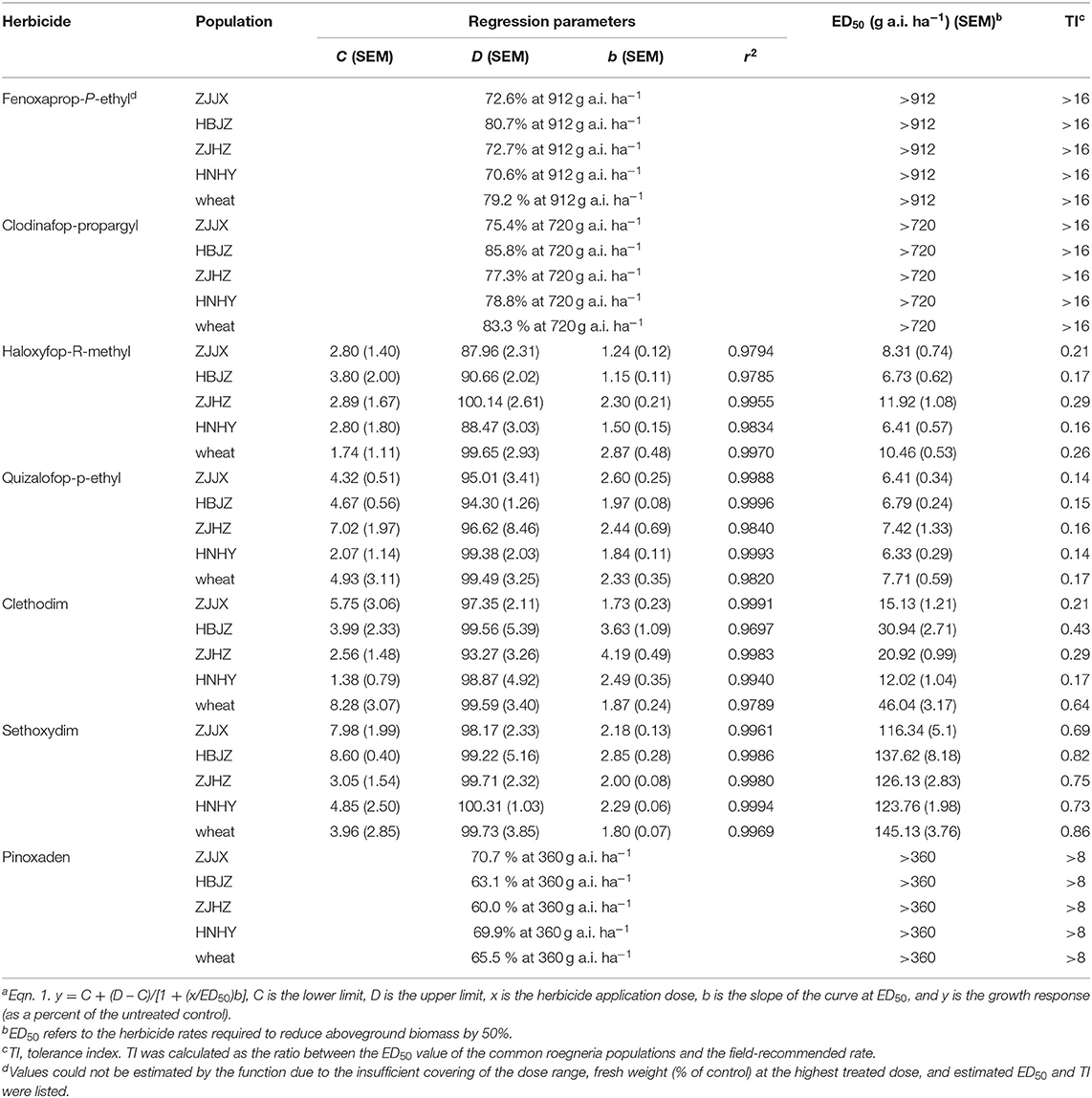
Table 3. Dose–response parameters of log-logistic function (Eqn. 1a used to fit the plant fresh weight data (as a percent of the control) for common roegneria populations treated with different ACCase inhibitors.
The ED50 values of the four common roegneria populations and wheat to the other two APP herbicides haloxyfop-R-methyl and quizalofop-p-ethyl, were <12 and <8 g a.i.−1, respectively (Table 3). Based on the TI values (<0.3 and <0.2), common roegneria populations were deemed susceptible to haloxyfop-R-methyl and quizalofop-p-ethyl. The herbicide doses required for 95% fresh weight reduction were 2- and 4-fold LFR for CHD herbicides clethodim and sethoxydim, respectively. The ED50 values of the four common roegneria populations and wheat to CHD herbicides clethodim and sethoxydim were <50 and <150 g a.i.−1, respectively. Based on the corresponding TI values (<0.7 and <0.9), common roegneria populations were susceptible to CHD herbicides. This contrasts with TSR mechanisms in ACCase resistance that confer broader resistance to ACCase herbicides, such as APP specific (mutations at codon positions 2,027, 2,041, and 2,096) and APP/CHD specific (mutations at codon positions 1,781 and 2,078) previously reported in A. myosuroides (Kaundun, 2014).
ACCase Gene Sequencing
The fragments of ACCase gene CT domain were amplified from four common roegneria populations. The predicted open reading frame (ORF) of ACCase CT domain in common roegneria was found by ORF finder, and these fragments covered all the reported resistance-related substitutions. After BLAST analysis of the ACCase CT domain of common roegneria (GenBank accession MT877224) in NCBI database, we found that ACCase protein of common roegneria has 99% identity to wheat and 95% identity to A. myosuroides (Figure 1). Using BioEdit to compare the amino acid sequence of four common roegneria populations, A. myosuroides, and wheat, the results showed that some amino acids of common roegneria are different from wheat, but none of them were related to the reported resistant mutations. These results indicated that the tolerance to ACCase inhibitors in common roegneria populations may not be caused by TSR, but likely by NTSR.
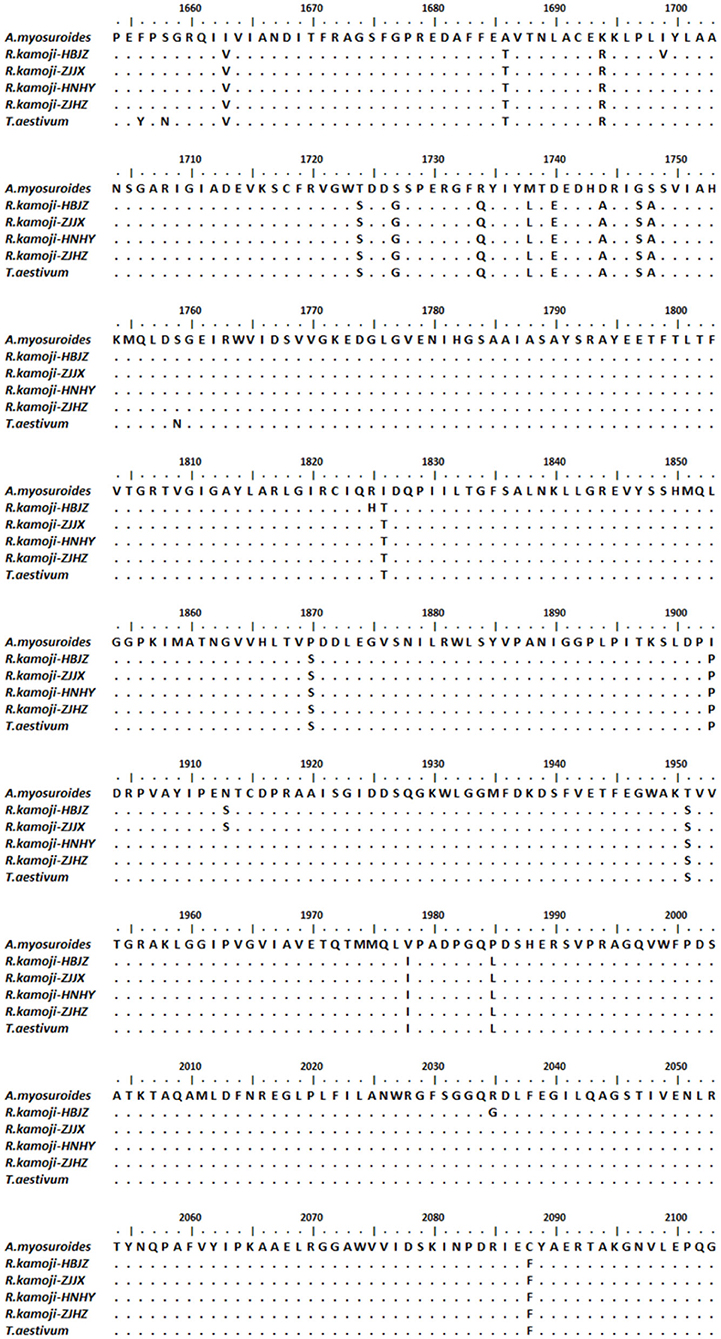
Figure 1. Sequence alignment of a highly conserved region of ACCase gene from four common roegneria populations and blackgrass (Alopecurus myosuroides Huds., accession number: AJ310767).
The resistance pattern to ACCase inhibitors, caused both by TSR or NTSR mechanism, has proven to show special resistance to a class of ACCase inhibitors or resistant to all ACCase inhibitors. Polypogon fugax and Lolium rigidum, for instance, segregating the Ile-2041-Asn mutation, are only cross-resistant to APPs (Liu et al., 2007; Tang et al., 2014). Several L. rigidum populations were resistant to ACCase inhibitors by the enhancement of herbicide metabolism (Holtum et al., 1991; Neve and Powles, 2005; Manalil et al., 2012; Yu and Powles, 2014). In this study, the common roegneria populations were determined only highly tolerant to APP herbicides applied in wheat and were susceptible to APP herbicides applied in other crops. In addition, no susceptible populations of common roegneria were identified; the tolerance pattern of this species is in complete accord with wheat and different to the reported resistant weed species.
Synergistic Effect of Malathion and NBD-Cl
Common roegneria plant responses to fenoxaprop-P-ethyl with or without malathion pretreatment were determined. Pretreatment with malathion showed no enhancement of the phytotoxicity of fenoxaprop-P-ethyl when sprayed on ZJJX and ZJHZ plants, respectively (Figure 2A). The ED50 values for fenoxaprop-P-ethyl treatments of ZJJX and ZJHZ plants were similar and all >16 × LFR. Similarly, NBD-Cl did not enhance the herbicidal activity of fenoxaprop-P-ethyl when applied to ZJJX and ZJHZ populations (Figure 2B).
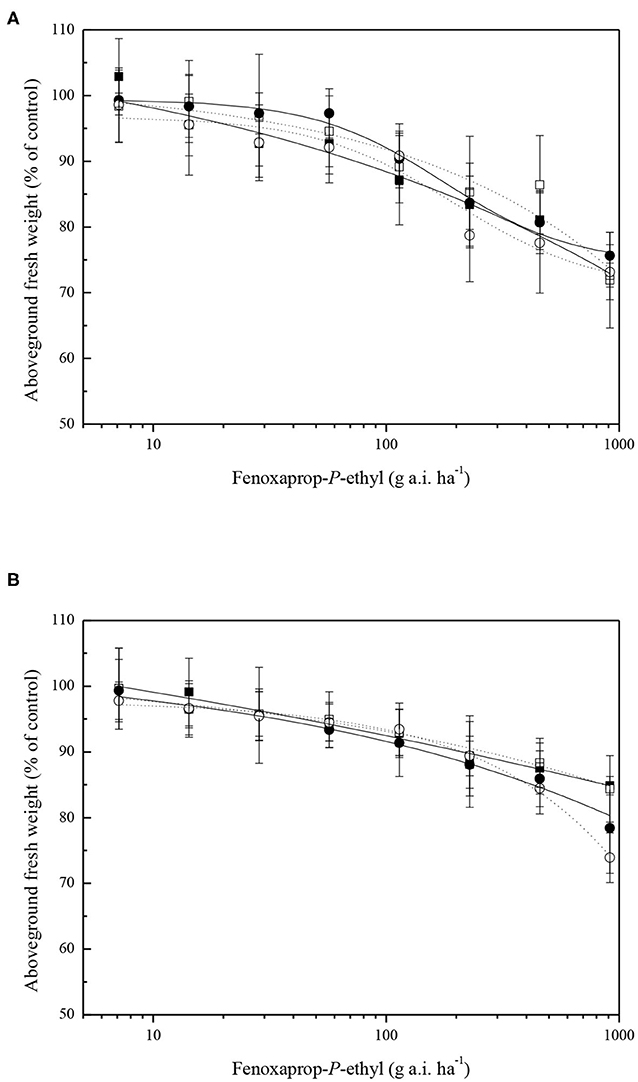
Figure 2. Dose–response curves for fresh weight reduction of two common roegneria populations (ZJJX, ■ or □) and (ZJHZ, • or °) treated with a range of fenoxaprop-P-ethyl doses plus (filled symbols) or minus (open symbols) 1,000 g ha−1 of malathion (A) and 80 g ha−1 NBD-Cl (B). Vertical bars represent mean ± SE.
Pretreatment with malathion before herbicide application has long been used to detect whether CytP450 was involved in NTSR mechanism. Malathion can reverse the resistance to herbicides in some resistant weed species (Christopher et al., 1994; Feng et al., 2016; Zhao et al., 2017a). NBD-Cl, derivatives of which target GST in tumor cells, has proven enhanced the herbicidal activity of fenoxaprop-P-ethyl, clodinafop-propargyl and chlorotoluron (Ricci et al., 2005; Turella et al., 2006; Cummins et al., 2013). Instead, we propose that CytP450 or GST may be involved in the metabolic detoxification of fenoxaprop-P-ethyl in common roegneria. However, both malathion and NBD-Cl did not reverse the tolerance of common roegneria to fenoxaprop-P-ethyl. The results of the current study suggest that CytP450 or GST might play a different role in the ACCase inhibitors tolerance in common roegneria plants.
In vitro Assay of GST and ACCase Activities to Fenoxaprop-P-Ethyl
The results showed that in wheat plants without fenoxaprop-P-ethyl treatment, the GST activity 17.08 was U/min/mg leaf protein−1, which was 4.50 and 3.53 times higher than that in ZJHZ and ZJJX common roegneria plants, respectively (Figure 3). After fenoxaprop-P-ethyl treatment, GST activity was significantly decreased in wheat at 24 HAT, but subsequently increased and reached its peak at 72 HAT. However, the activity of GST was not significantly affected in both ZJJX and ZJHZ populations with fenoxaprop-P-ethyl treatment, suggesting GST activity was more sensitive to fenoxaprop-P-ethyl in wheat than common roegneria. It is reported that relatively higher glutathione transferase activities in wheat and barley are responsible for resistance to fenoxaprop-P-ethyl (Romano et al., 1993; Cummins et al., 1997). In this study, GST activity in common roegneria populations is lower than that in wheat, but the GST activity was not affected with herbicide treatment, suggesting that GST may play a different role in the detoxification mechanism of fenoxaprop-P-ethyl in common roegneria as compared to wheat.
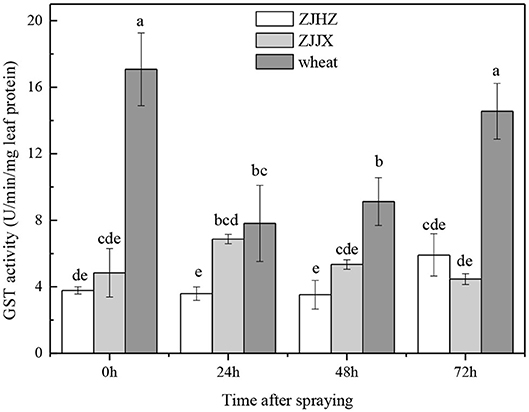
Figure 3. GST activity in wheat and common roegneria populations ZJJX and ZJHZ at 0, 24, 48, and 72 h after fenoxaprop-P-ethyl treated. Bars are mean ± SE (n = 10). Bars designated by the same lowercase letter are not different according to Fisher protected LSD at P ≤ 0.05.
The ACCase activities of common roegneria populations were similar to wheat before fenoxaprop-P-ethyl spraying (Figure 4). The ACCase activities of the ZJJX and ZJHZ populations after fenoxaprop-P-ethyl treatment were 1.46- and 1.39-fold higher at 72 HAT than that in 0 HAT, respectively. The ACCase activity of wheat showed similar trend with the common roegneria populations after fenoxaprop-P-ethyl treatment. It indicated that ACCase activities of common roegneria and wheat were not inhibited after the application of fenoxaprop-P-ethyl; instead, the activity of the target enzymes was increased, which may have been caused by rapid metabolism of the herbicide.
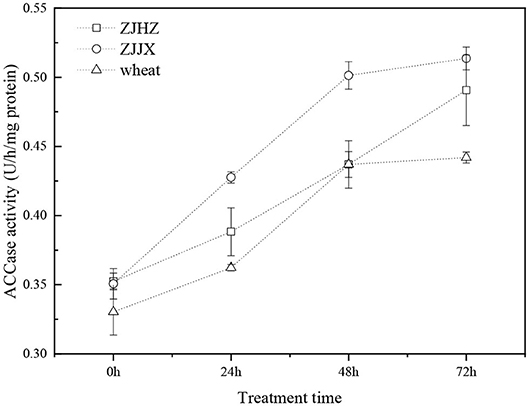
Figure 4. ACCase activity in wheat and common roegneria populations ZJJX and ZJHZ at 0, 24, 48, and 72 h after fenoxaprop-P-ethyl treated. Data are the means ± SE from five replicates of two experiments (n = 10).
General Tolerance to ACCase Inhibitors
It has been reported in many weed species that resistance to APP herbicides is caused by the reduction in ACCase sensitivity. For example, insensitive ACCases are responsible for resistance to ACCase inhibitors in L. rigidum (Cocker et al., 1999), Avena fatua (Seefeldt et al., 1996), Avena sterilis (Shukla et al., 1997), and Echinochloa crus-galli (Huan et al., 2013). However, in these cases, the presence of insensitive ACCases was related to mutations in the ACCase gene. Moreover, certain ACCase inhibitors can be used selectively in cereals because of the addition of safeners (e.g., mefenpyr-diethyl and clonquintocet-mexyl), which result in enhanced metabolization and detoxification of the herbicidal ingredients (Cataneo et al., 2013; Taylor et al., 2013). In this study, we determined that there were no reported mutations in the ACCase gene, and the ACCase activity was increased by the application of fenoxaprop-P-ethyl; therefore, it suggests that the increase in ACCase activity might have caused the tolerance.
Chang et al. (2011) investigated the leaf anatomical characteristics of six Roegneria species and indicated that anatomical structure was similar to Triticeae species. The phylogenic relationships between Roegneria and Triticeae species was analyzed, and results indicated that common roegneria was closely related to Triticeae (Zhang et al., 2014). This might explain the similar responses to ACCase inhibitors between common roegneria and wheat. In our recent study, the effects of flooding on seed survival were compared under field conditions, and results showed that seed germination and perennial rhizome regenerative ability decreased significantly after 60 days under flooded paddy soil than in dryland soil (data not shown). It is likely that the water-saving cropping practices (i.e., direct-seeding rice or no-tilling after wheat cropping in wheat-rice rotation system) adopted recently in the middle and lower regions of the Yangtze River might have accelerated the survival and spread of common roegneria.
In summary, common roegneria was tolerant to ACCase inhibitors applied in wheat, which have the potential to spread and develop into a serious weed in wheat or cereal crops based on chemical control of grass weeds. In addition, common roegneria is a perennial weed, which could reproduce both by seeds and rhizome and makes it difficult to eradicate. Rotating to other winter crops (i.e., oilseed rape) with rice, to allow the use of different herbicides, or adopting transplant rice after winter crops to ensure a relatively longer flooding period may help manage this weed.
Data Availability Statement
The original contributions presented in the study are included in the article/Supplementary Material, further inquiries can be directed to the corresponding author/s.
Author Contributions
WT and XY designed the research study and wrote the manuscript. WT, XY, JC, and LX performed the experimental work. WT carried out the data analysis. YL provided helpful suggestions during the experiments and preparation of the manuscript. All authors discussed the results of the study and approved it for publication.
Conflict of Interest
The authors declare that the research was conducted in the absence of any commercial or financial relationships that could be construed as a potential conflict of interest.
Acknowledgments
We thank Ms. Yufang Chen for her technical assistance for part of the experiment. This research was funded by the Rice Pest Management Research Group of the Agricultural Science and Technology Innovation Program of Chinese Academy of Agricultural Sciences, the National Natural Science Foundation of China (No. 32071508), and the China Agriculture Research System (CARS-01-02A). The authors declare that the research was conducted in the absence of any commercial or financial relationships that could be construed as a potential conflict of interest.
Supplementary Material
The Supplementary Material for this article can be found online at: https://www.frontiersin.org/articles/10.3389/fagro.2020.587651/full#supplementary-material
References
Busi, R., Vila-Aiub, M. M., and Powles, S. B. (2011). Genetic control of a cytochrome P450 metabolism-based herbicide resistance mechanism in Lolium rigidum. Heredity 106, 817–824. doi: 10.1038/hdy.2010.124
Cataneo, A. C., Ferreira, L. C., Mischan, M. M., Velini, E. D., Corniani, N., and Cerdeira, A. L. (2013). Mefenpyr-diethyl action on fenoxaprop-P-ethyl detoxification in wheat varieties. Planta Daninha 31, 387–393. doi: 10.1590/S0100-83582013000200016
Chang, X. G., Xu, Z., Yi, J., and Yan, W. H. (2011). Comparison of leaf anatomical characteristics of six species of Roegneria. Acta Agrestica Sin. 19, 443–450. doi: 10.1016/j.bjp.2018.04.006
Christopher, J., Preston, C., and Powles, S. (1994). Malathion antagonizes metabolism-based chlorsulfuron resistance. Pestic. Biochem. Physiol. 49, 172–182. doi: 10.1006/pest.1994.1045
Cocker, K. M., Moss, S. R., and Coleman, J. O. D. (1999). Multiple mechanisms of resistance to fenoxaprop-P-ethyl in United Kingdom and other European populations of herbicide-resistant Alopecurus myosuroides (black-grass). Pestic. Biochem. Physiol. 65, 169–180. doi: 10.1006/pest.1999.2439
Cummins, I., Moss, S., Cole, D. J., and Edwards, R. (1997). Glutathione transferases in herbicide-resistant and herbicide-susceptible black-grass (Alopecurus myosuroides). Pestic. Sci. 51, 244–250. doi: 10.1002/(SICI)1096-9063(199711)51:3<244::AID-PS643>3.0.CO;2-2
Cummins, I., Wortley, D., Sabbadin, F., He, Z. S., Coxon, C. R., Straker, H. E., et al. (2013). Key role for a glutathione transferase in multiple-herbicide resistance in grass weeds. Proc. Natl. Acad. Sci. U.S.A. 110, 5812–5817. doi: 10.1073/pnas.1221179110
Délye, C., Gardin, J. A. C., Boucansaud, K., Chauvel, B., and Petit, C. (2011). Non-target-site-based resistance should be the centre of attention for herbicide resistance research: Alopecurus myosuroides as an illustration. Weed Res. 51, 433–437. doi: 10.1111/j.1365-3180.2011.00864.x
Délye, C., Zhang, X. Q., Michel, S., Matejicek, A., and Powles, S. B. (2005). Molecular bases for sensitivity to acetyl-coenzyme a carboxylase inhibitors in black-grass. Plant Physiol. 137, 794–806. doi: 10.1104/pp.104.046144
Devine, M. D. (1997). Mechanisms of resistance to acetyl-coenzyme A carboxylase inhibitors: a review. Pestic. Sci. 51, 259–264. doi: 10.1002/(SICI)1096-9063(199711)51:3<259::AID-PS644>3.0.CO;2-S
Feng, Y., Gao, Y., Zhang, Y., and Dong, L. (2016). Mechanisms of resistance to pyroxsulam and ACCase inhibitors in Japanese foxtail (Alopecurus japoicus). Weed Sci. 64, 695–704. doi: 10.1614/WS-D-16-00042.1
Ghanizadeh, H., and Harrington, K. C. (2017). Non-target site mechanisms of resistance to herbicides. Crit. Rev. Plant Sci. 36, 24–34. doi: 10.1080/07352689.2017.1316134
Guo, W. L., Zhang, L. L., Wang, H. Z., Li, Q., Liu, W. T., and Wang, J. X. (2017). A rare Ile-2041-Thr mutation in the ACCase gene confers resistance to ACCase-inhibiting herbicides in shortawn foxtail (Alopecurus aequalis). Weed Sci. 65, 239–246. doi: 10.1017/wsc.2016.32
Harwood, J. L. (1988). Fatty acid metabolism. Annu. Rev. Plant Physiol. 39, 101–138. doi: 10.1146/annurev.pp.39.060188.000533
Heap, I. M. (2020). The International Survey of Herbicide Resistant Weeds. Available online at: http://weedscience.org/Summary/MOA.Aspx (accessed June 8, 2020).
Holtum, J. A. M., Matthews, J. M., Häusler, R. E., Liljegren, D. R., and Powles, S. B. (1991). Cross-resistance to herbicides in annual ryegrass (Lolium rigidum). III. on the mechanism of resistance to diclofop-methyl. Plant Physiol. 97, 1026–1034. doi: 10.1104/pp.97.3.1026
Huan, Z. B., Xu, Z., Lü, D. Z., and Wang, J. X. (2013). Determination of ACCase sensitivity and gene expression in quizalofop–ethyl-resistant and -susceptible barnyardgrass (Echinochloa crus-galli) biotypes. Weed Sci. 61, 537–542. doi: 10.1614/WS-D-13-00010.1
Kaundun, S. S. (2014). Resistance to acetyl-CoA carboxylase-inhibiting herbicides. Pest Manag. Sci. 70, 1405–1417. doi: 10.1002/ps.3790
Kruger, N. J. (2002). “The Bradford method for protein quantitation,” in The Protein Protocols Handbook, 2nd Edn, ed J. M. Walker (New Jersey, Totowa: Humana Press), 15–21.
Kukorelli, G., Reisinger, P., and Pinke, G. (2013). ACCase inhibitor herbicides – selectivity, weed resistance and fitness cost: a review. Int. J. Pest Manag. 59, 165–173. doi: 10.1080/09670874.2013.821212
Liu, W., Harrison, D. K., Chalupska, D., Gornicki, P., O'Donnell, C. C., Adkins, S. W., et al. (2007). Single-site mutations in the carboxyltransferase domain of plastid acetyl-CoA carboxylase confer resistance to grass-specific herbicides. Proc. Natl. Acad. Sci. U.S.A. 104, 3627–3636. doi: 10.1073/pnas.0611572104
Manalil, S., Busi, R., Renton, M., and Powles, S. B. (2012). An herbicide-susceptible rigid ryegrass (Lolium rigidum) population made even more susceptible. Weed Sci. 60, 101–105. doi: 10.1614/WS-D-11-00076.1
Neve, P., and Powles, S. (2005). Recurrent selection with reduced herbicide rates results in the rapid evolution of herbicide resistance in Lolium rigidum. Theor. Appl. Genet. 110, 1154–1166. doi: 10.1007/s00122-005-1947-2
Powles, S. B, and Yu, Q. (2010). Evolution in action: plants resistant to herbicides. Annu. Rev. Plant Biol. 61, 317–347. doi: 10.1146/annurev-arplant-042809-112119
Preston, C., and Mallory-Smith, C. A. (2001). “Biochemical mechanisms, inheritance, and molecular genetics,” in Herbicide Resistance and World Grains, eds S. B. Powles, and D. L. Shaner (Boca Raton, FL: CRC Press), 23–60. doi: 10.1201/9781420039085-2
Ricci, G., De Maria, F., Antonini, G., Turella, P., Bullo, A., Stella, L., et al. (2005). 7-Nitro-2,1,3-benzoxadiazole derivatives, a new class of suicide inhibitors for glutathione S-transferases: Mechanism of action of potential anticancer drugs. J. Biol. Chem. 280, 26397–26405. doi: 10.1074/jbc.M503295200
Romano, M. L., Stephenson, G. R., Tal, A., and Hall, J. C. (1993). The effect of monooxygenase and glutathione S-transferase inhibitors on the metabolism of diclofop-methyl and fenoxaprop-ethyl in barley and wheat. Pestic. Biochem. Physiol. 46, 181–189. doi: 10.1006/pest.1993.1049
Sasaki, Y., Konishi, T., and Nagano, Y. (1995). The compartmentation of acetyl-coenzyme A carboxylase in plants. Plant Physiol. 108, 445–449. doi: 10.1104/pp.108.2.445
Seefeldt, S. S., Fuerst, E. P., Gealy, D. R., Shukla, A., Irzyk, G. P., and Devine, M. D. (1996). Mechanisms of resistance to diclofop of two wild oat (Avena fatua) biotypes from the Willamette Valley of Oregon. Weed Sci. 44, 776–781. doi: 10.1017/S0043174500094704
Seefeldt, S. S., Jensen, J. E., and Fuerst, E. P. (1995). Log-logistic analysis of herbicide dose-response relationships. Weed Technol. 9, 218–227. doi: 10.1017/S0890037X00023253
Shukla, A., Dupont, S., and Devine, M. D. (1997). Resistance to ACCase-inhibitor herbicides in wild oat: evidence for target site-based resistance in two biotypes from Canada. Pestic. Biochem. Physiol. 57, 147–155. doi: 10.1006/pest.1997.2267
Tang, W., Zhou, F. Y., Chen, J., and Zhou, X. G. (2014). Resistance to ACCase-inhibiting herbicides in an Asia minor bluegrass (Polypogon fugax) population in China. Pestic. Biochem. Physiol. 108, 16–20. doi: 10.1016/j.pestbp.2013.11.001
Taylor, V. L., Cummins, I., Brazier-Hicks, M., and Edwards, R. (2013). Protective responses induced by herbicide safeners in wheat. Environ. Exp. Bot. 88, 93–99. doi: 10.1016/j.envexpbot.2011.12.030
Turella, P., Filomeni, G., Dupuis, M. L., Ciriolo, M. R., Molinari, A., De Maria, F., et al. (2006). A strong glutathione S-transferase inhibitor overcomes the P-glycoprotein-mediated resistance in tumor cells: 6-(7-Nitro-2,1,3-benzoxadiazol-4-ylthio) hexanol (NBDHEX) triggers a caspase-dependent apoptosis in MDR1-expressing leukemia cells. J. Biol. Chem. 281, 23725–23732. doi: 10.1074/jbc.M604372200
Wenger, J., Niderman, T., and Mathews, C. (2012). “Acetyl-CoA carboxylase inhibitors,” in Modern Crop Protection Compounds, eds W. Kramer, U. Schirmer, P. Jeschke, and M. Witschel (Weinheim, Germany: Wiley-VCH), 447–477.
Xu, Z. H., Qi, H. Y., Lu, Y. L., Yang, W. D., and Xie, G. X. (2014). Weed Identification and Management. (Hangzhou: Zhejiang University Press).
Yu, Q., and Powles, S. B. (2014). Metabolism-based herbicide resistance and cross-resistance in crop weeds: a threat to herbicide sustainability and global crop production. Plant Physiol.166, 1106–1118. doi: 10.1104/pp.114.242750
Zhang, H. X., Ji, Y. L., Lu, T., Wang, Y., Liu, B. H., and Wang, Z. W. (2014). Identification of grass without inflorescence collected from Korqin, Inner Mongolia. J. Nanjing Agri. Univ. 26, 41–45.
Zhao, B. C., Fu, D. N., Yu, Y., Huang, C. T., Yan, K. C., Li, P. S., et al. (2017a). Non-target-site resistance to ALS-inhibiting herbicides in a Sagittaria trifolia L. population. Pestic. Biochem. Physiol. 140, 79–84. doi: 10.1016/j.pestbp.2017.06.008
Keywords: acetyl coA carboxylase (ACCase), fenoxaprop-P-ethyl, ACCase activity, non-target-site mechanism, tolerance
Citation: Tang W, Yu X, Chen J, Xie L and Lu Y (2020) Tolerance to Some ACCase Inhibitors in Four Common Roegneria (Roegneria kamoji) Populations From China. Front. Agron. 2:587651. doi: 10.3389/fagro.2020.587651
Received: 27 July 2020; Accepted: 21 September 2020;
Published: 23 October 2020.
Edited by:
Hanwen Wu, New South Wales Department of Primary Industries, AustraliaReviewed by:
Md Asaduzzaman, New South Wales Department of Primary Industries, AustraliaSimerjeet Kaur, Punjab Agricultural University, India
Copyright © 2020 Tang, Yu, Chen, Xie and Lu. This is an open-access article distributed under the terms of the Creative Commons Attribution License (CC BY). The use, distribution or reproduction in other forums is permitted, provided the original author(s) and the copyright owner(s) are credited and that the original publication in this journal is cited, in accordance with accepted academic practice. No use, distribution or reproduction is permitted which does not comply with these terms.
*Correspondence: Yongliang Lu, luyongliang@caas.cn
†These authors have contributed equally to this work