Urbanization Effects on Biodiversity Revealed by a Two-Scale Analysis of Species Functional Uniqueness vs. Redundancy
- 1Centre d'Ecologie et des Sciences de la Conservation (CESCO), Muséum National d'Histoire Naturelle, Département Homme et Environnement, Centre National de la Recherche Scientifique, Sorbonne Université, Paris, France
- 2Institut Systématique Evolution Biodiversité (ISYEB), Muséum National d'Histoire Naturelle, Département Origines et Evolutions, CNRS, Sorbonne Université, EPHE, Paris, France
- 3Department Community Ecology, Helmholtz Centre for Environmental Research—UFZ, Halle (Saale), Germany
- 4Department of Ecology, Technische Universität Berlin, Berlin, Germany
- 5German Centre for Integrative Biodiversity Research (iDiv), Halle-Jena-Leipzig, Leipzig, Germany
- 6Martin Luther University Halle-Wittenberg, Geobotany and Botanical Garden, Halle (Saale), Germany
- 7Conservatoire Botanique National du Bassin Parisien (CBNBP), Muséum National d'Histoire Naturelle, Sorbonne Université, Paris, France
- 8Institut Méditerranéen de Biodiversité et d'Ecologie marine et continentale (IMBE), Univ Avignon, Aix Marseille Univ, CNRS, IRD, UT Site Agroparc, Avignon, France
- 9Institut d'Ecologie et des Sciences de l'Environnement de Paris (IEES), Sorbonne Université, Paris, France
Urbanization is one of the most intensive and rapid human-driven factors that threat biodiversity. Finding an indicator of species community responses to urbanization is crucial for predicting the consequences of anthropogenic land cover changes. Here, we develop a framework that relies on functional originality. A species is original or equivalently distinct, regarding its traits, if it possesses rare trait values in a community of species. The most original species have the greatest contributions to the trait diversity of that community. We studied plant species originality, in light of observed changes in the level of species richness, along an urbanization gradient in the region of Paris, France. To evaluate potential impacts of urbanization on species assemblages, we simultaneously considered the local community and regional pool as reference scales where to calculate the originality of each species. Then, for each community, we calculated the mean and skewness of local and regional originalities and the ratio of local to regional originality, providing indication on how functionally diverse a community is, how original it is compared to other communities of the region, how evenly distributed species were in the local and regional functional space, and whether regionally-redundant species become original locally due to limiting similarity. The mean functional originality increased with urbanization at both local and regional scales, although this increase vanished in communities with high species richness. The skewness of originalities increased from zero to positive values with species richness in built-up areas and the ratio of local-to-regional originality increasing along the urbanization gradient, except in species-rich communities. Here our results suggest that urban plant communities are composed of both locally and regionally unique urbanophile species, suggesting processes that limit niche overlap to allow species coexistence. In richer communities, these unique species coexist with regionally-redundant species the occurrence of which could be stochastic. Our conceptual framework shows that species originality can inform on environmental processes that influence biodiversity during community assembly. It is flexible enough to be extended to other regions and other contexts complementing diversity metrics in the research of the mechanisms by which human activities impact species assemblages.
Introduction
The United Nations (2018) predicted that by the year 2050, 68% of the global human population would live in urban areas, constantly growing in surface. As a key aspect of the anthropization of natural areas, urbanization comes with a complex mix of changes, including land-use modifications and environmental disturbances, which increase the pressure on local remnant species diversity (Gaston, 2010) and induce the assembly of novel ecological communities (Swan et al., 2011). Studies of urban biodiversity mainly focused on describing species composition and species richness (e.g., Balmford et al., 2001; Araújo, 2003), species distribution and abundance (e.g., Aronson et al., 2016; Deguines et al., 2016; Guetté et al., 2017), but also species trait distribution and/or phylogenetic positions (e.g., Knapp et al., 2008a; Williams et al., 2015; Kalusová et al., 2017). To date, it has been largely acknowledged that cities, representing a high spatial heterogeneity of green spaces, can harbor an important amount of plant and animal species (Kühn et al., 2004; Godefroid and Koedam, 2007; Pautasso et al., 2011) and that cities play an important role in biodiversity conservation (Kowarik, 2011; Ives et al., 2016; Soanes and Lentini, 2019). However, a likely negative effect of urbanization was observed at large, global scales, expressed by functional homogenization (McKinney, 2006), decreasing phylogenetic diversity (e.g., Ricotta et al., 2009; Ibáñez-Álamo et al., 2017), narrowing the spectrum of present functional traits (e.g., Williams et al., 2015) but with still often a positive effect on an increasing plant and animal species richness (Kühn et al., 2004; Godefroid and Koedam, 2007; Pautasso et al., 2011). The analysis of the diversity of functional traits (i.e., functional diversity) in cities is particularly important as functional traits are those traits that influence the way species respond to environmental conditions or the way they contribute to ecosystem properties (Lavorel and Garnier, 2002). At smaller spatial scales, contradictory effects of urbanization on species and functional diversity have sometimes been observed depending on the location and on the taxa under study (e.g., Kühn and Klotz, 2006; McKinney, 2006; Vimal and Devictor, 2014; Ibáñez-Álamo et al., 2017). A comprehensive analysis of species responses to urbanization and their contribution to community diversity across spatial scales is still lacking.
Characterized by strong environmental changes, urbanized areas incorporate a set of human-induced processes, such as habitat transformation and fragmentation, increasing environmental stressors, human preferences and historical and socioeconomic factors (Williams et al., 2009; Aronson et al., 2016). Species assemblages are driven by multiple interacting processes (e.g., stochasticity, facilitation, competition, adaptation). Among them, many ecological studies in the last two decades have opposed environmental filtering (abiotic factors), which restricts trait-based dissimilarity between species (Zobel, 1997), to the local distribution of resources and inter-specific interactions (biotic factors) that may also restrict trait-based dissimilarity between species if species with similar traits that provide high fitness exclude others, or inversely, limit coexistence of species sharing similar traits to avoid competition (e.g., McArthur and Levins, 1967; Stubbs and Wilson, 2004). These processes altogether shape levels of species and trait-based diversity of communities (e.g., Williams et al., 2009; Swan et al., 2011; Aronson et al., 2016). The mechanisms by which communities are assembled in disturbed environments may however be fundamentally different from those acting in the least disturbed environments (Mason et al., 2011; de Bello et al., 2013). Changes in community assembly mechanisms leave a signature in community diversity patterns. The trait composition of local communities would thus be a product of abiotic and biotic filtering of traits from the regional pool (Zobel, 2016; Spasojevic et al., 2018). At the local scale, the selection of specific traits in urban habitats could lead to reduced functional diversity (Lososová et al., 2016; La Sorte et al., 2018; Fournier et al., 2019). For example, plant species favored in urban areas are often drought/heat-tolerant, photophile species with increased height and competitive ability compared to species from other areas (see e.g., Williams et al., 2015). The share of the same non-native human-introduced species within urban habitats could also decrease the functional diversity of urban communities at the landscape scale (e.g., Sodhi et al., 2019). However, some studies observed trait divergence in urban areas compared to surrounding areas, which they explained by habitat heterogeneity at the large landscape scale and by niche differentiation through interspecific competition at the local community scale (e.g., Concepción et al., 2015).
The hierarchical filtering of species from a regional pool to local communities is still poorly understood in urban areas, mostly because of a multitude of factors acting at the same time (Williams et al., 2015). Thus, a comprehensive analysis of species responses to urbanization and their contribution to community functional diversity across spatial scales is lacking. To evaluate species-specific responses to urbanization, there is a need for a metric estimating the contribution of each species to biodiversity, accounting for ecological differences between coexisting species at multiple spatial scales. Such a species-specific value can be obtained by originality metrics. In contrast to the widely-used diversity metrics measured at the level of a species set, originality metrics give a value to each species in a set. Pavoine et al. (2005) defined species originality as a general term unifying a multitude of concepts related to the contribution of a species to biodiversity: distinctiveness, distinctness, isolation or else uniqueness (see e.g., Redding et al., 2014; Pavoine et al., 2017; Kondratyeva et al., 2019). The idea that certain species contribute disproportionately to functional diversity of a community is based on the assumption that they possess a set of unusual biological characters unshared by the rest of species community (Redding et al., 2015). Indeed, a species is original if it has rare biological characteristics within a reference set of species (Pavoine et al., 2017). Such rare characteristics can be trait values that only few species share (Mouillot et al., 2013a; Brandl et al., 2016; Pavoine et al., 2017). Therefore, originality is a relative measure and can be described as a gradient (Violle et al., 2017; Kondratyeva et al., 2019), with two extreme, yet unlikely cases: unique species on one side, not sharing any biological characteristic with others (100% of unique contribution to the diversity of a set) and redundant species on another side, sharing all of its characters with other species (0% of unique contribution to the diversity of a set).
When studying community assembly, species have to be considered at least at two key spatial scales: the local community and the regional species pool. Indeed, the regional species pool constitutes the set of species that could potentially colonize and establish within a community (Zobel, 1997; Lessard et al., 2012). Measured at different spatial scales, species originality, i.e., species relative contribution to biodiversity, could reveal signatures of scale-dependent ecological processes of community assembly. Indeed, a measure of species originality allows the comparison of a species' trait values to the values of all other species in a regional pool or all other species in a local community, adapting the measurement of species originality to the spatial scale of interest. For example, species that are functionally redundant at the regional scale may co-occur locally because they are adapted to the same environmental conditions, which would lead to local and regional redundancy for these species. However, they could inversely never co-occur locally, being distributed in different areas/communities to avoid competition, which would lead to regional redundancy but local originality for these species. Thus, functional originality (trait-based dissimilarity) of species in local communities is influenced by abiotic filtering and determines species local biotic interactions and coexistence (Cornwell and Ackerley, 2009; de Bello et al., 2012).
To date, few studies have compared processes of urban community assembly based on functional trait values at local and regional levels (see e.g., Fournier et al., 2019). Therefore, our aim here was to explore the impact of urban environmental conditions on plant species functional originality at two spatial scales: the local plant communities and the regional species pool. We also analyzed how species' originality values varied between local urban built-up areas and other land cover types (forest, semi-natural area, agricultural area, green urban spaces). We develop our expectations of how the functional originality of species may vary along an urbanization gradient in Table 1. These expectations are structured into four main scenarios, explained by patterns of mean local originalities and mean regional originalities in communities. Species richness, skewness of originalities and the ratio of local to regional originalities then help to refine these scenarios, and identify key potential processes that could drive species assemblages. To address urban community assembly, we thus analyzed mean and skewness of local and regional species originality in plots distributed among land cover types along an urbanization gradient. This enabled us to investigate three main questions:
1. How does urbanization and species richness influence species' regional and local originality and to what extent do these factors interact?
2. Which biological traits characterize the most original species at regional scale and at local scale across different land cover types?
3. How can regional and local species originality inform on community assembly processes in an urbanization context?
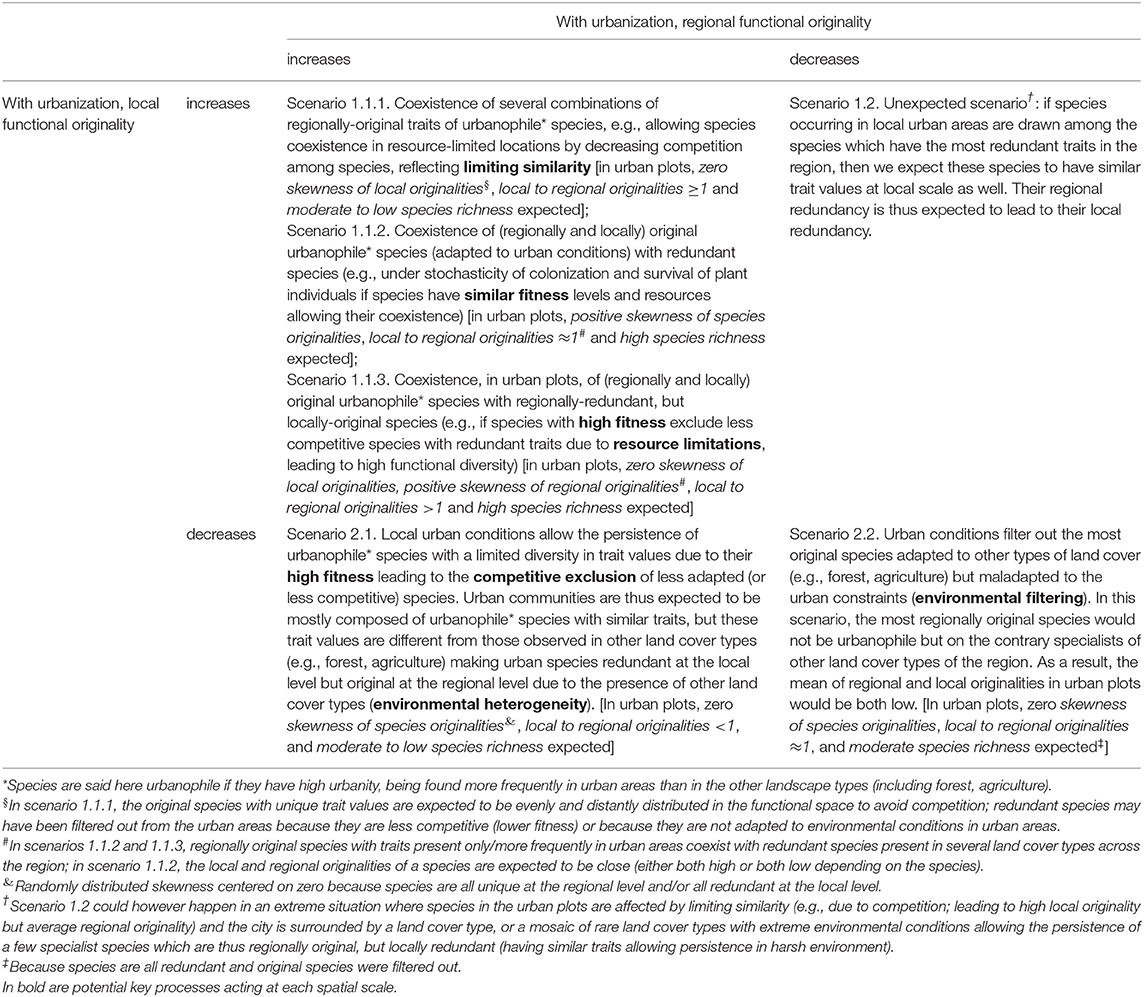
Table 1. Scenarios which could explain each combination of patterns between (mean) local and regional functional originality of the same species along the urbanization gradient.
Materials and Methods
Study Area
We analyzed plant community data sampled across the Île-de-France region by the participants of the national citizen science survey, Vigie-flore (http://www.vigienature.fr/fr/vigie-flore), which constitutes a large national dataset of plant occurrences in diverse habitats. The Île-de-France region occupies a territory of 12,100 km2 and is the most inhabited region of France, with 12.1 million inhabitants. It includes the city of Paris, which is the French capital and largest city with 2.2 million inhabitants in 2016, and ~1,300 other cities and towns with varying human populations (min = 27, max = 119,645, median = 1318, Institut Paris Region, 2018). This region benefits from having the highest sampling effort within the Vigie-flore program as well as a detailed land cover geodatabase.
Land Cover Data
We used vectorized 1/5000 GIS maps of land cover in Île-de-France from Institut Paris Region (2018), available for two time periods (2009 to 2011 and 2012 to 2017). We used land cover categories recovering the totality of the region (Figure 1): (1) forests (24% of Île-de-France area), (2) semi-natural area (2%, including open green spaces and riverbanks), (3) agricultural areas (50%), (4) green urban areas (6%, including urban parks, gardens, lawns, and cemeteries), (5) urban built-up areas (16%, including individual housing, collective housing, industrial and business areas, facilities, quarries, dumps and worksites, and transportation infrastructures), and (6) water (2%, excluded from the following analysis). A detailed description of each category is available online (https://www.institutparisregion.fr). Using the ArcGIS® software for geographical data manipulation (Environmental Systems Research Institute, ESRI, 2018, ArcGIS release 10.5.1), we linked the Vigie-flore plots with land cover data and calculated, as a surrogate for the urbanization gradient, the percentage of built-up area inside each 1 × 1 km2 grid cell where Vigie-flore plots occurred.
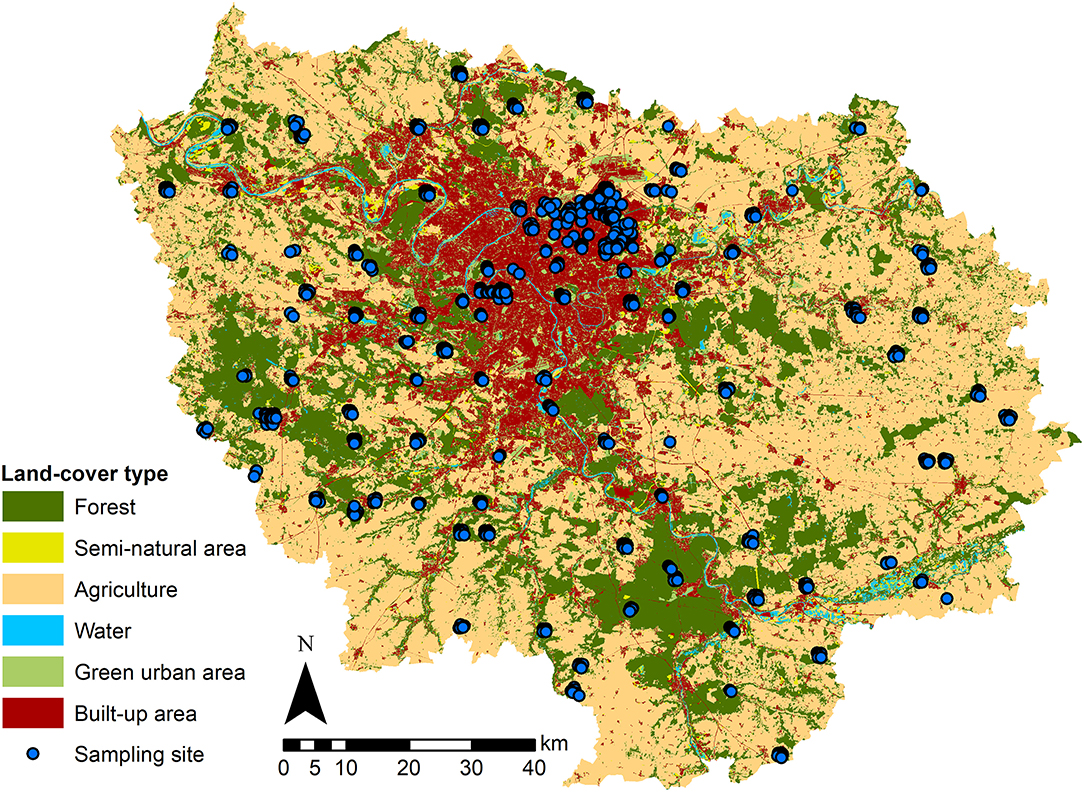
Figure 1. Land cover and spatial distribution of the sampling plots (spatial resolution of 12.5 meters). The map shows the Île-de-France region, including its largest city Paris and ~1,300 other cities and towns, which are represented by built-up areas [red, 16%, including individual housing (8%), collective housing (2%), industrial and business areas (2%), facilities (1%), quarries, dumps and worksites (0.5%) and transportation infrastructure (2.5%)]. The built-up areas and other land cover types that correspond to forests (dark green; 24% of total area), semi-natural areas (green yellow, 2%), agricultural areas (beige, 50%), green urban spaces (bright green, 6%) and rivers and water points (blue, 2%) were extracted from data from the Institut Paris Region (2018). The blue dots represent the 2,362 sampling plots of Vigie-flore from 2009 to 2017 (superimposed for the same plot surveyed in different years).
Biological Data
Following the standardized protocol of the Vigie-flore program, skilled amateur botanists investigated randomly selected 1 × 1 km2 grid cells. Each grid cell contains eight systematically distributed 10 m2 plots across land cover types. Note that this standard 10 m2 area of a plot (adopted by other programs, e.g., in Switzerland, Bühler and Roth, 2011) is reduced, compared to the area needed to fully characterize plant communities in certain habitat types, such as forest, but it allows a representative sampling of flora at the national level. In each plot, the presence of all vascular plants was recorded at most once a year. We considered the set of species in each 10 m2 plot as a local community assemblage. We analyzed the subset of 620 plots surveyed over the years 2009 to 2017 in the Île-de-France region (in average one plot was surveyed 7 times in 10 years) in all types of land cover. For computational reasons, only plots with a minimum of three species were retained. Indeed, it is meaningless to measure originality in a plot with one species where there are no other species, and in a plot with two species, each of the species would have equal originality relative to one another. Out of 812 species recorded by Vigie-flore in Île-de-France region, 586 herbaceous angiosperm species were retained. We removed some species from the initial species dataset: (1) species occurring in plots with 1 or 2 species only that were excluded for computational reasons (see explanation above); (2) species, which are not included in the Île-de-France plant species list established by the Conservatoire Botanique National du Bassin Parisien (CBNBP, 2016) which were probably plant identification mistakes; (3) species that rely on human assistance (strictly cultivated or planted species); (4) Pteridophytes, because they need specific descriptors of their morphology (for example, they have spores and not fruits, fronds and not leaves etc.) and were not always identified by many amateur botanists; (5) woody species, because they take more time to respond to environmental changes in urban areas than herbaceous species and because of their outlying trait values compared to many herbaceous species. Woody and herbaceous species were distinguished following the LEDA trait database (Kleyer et al., 2008).
Species Biological Characteristics
To assemble as much trait data as possible, we standardized species taxonomic nomenclature among datasets by using several synonymous names and updating them by using The Plant List (http://www.theplantlist.org/), the Tela Botanica website of the French botanist's network (https://www.tela-botanica.org/) and Flora Gallica (Tison and de Foucault, 2014). We finally kept species names as found in Vigie-flore data with a taxonomical reference TAXREF V5.0 (https://inpn.mnhn.fr/programme/referentiel-taxonomique-taxref). We collected information on the biological traits of each species from several databases (Table S1): the BiolFlor database on biological and ecological traits of the German flora (Klotz et al., 2002), LEDA database of life-history traits of the Northwest European flora (Kleyer et al., 2008), TRY global database of plant traits (Kattge et al., 2011), Ecoflora database of British Isles (www.ecoflora.co.uk, Fitter and Peat, 1994), and Catminat database of French flora (Julve, 1998) along with numerous botanical garden resources across Europe (e.g., POWO, 2019). The data included three binary (life span, pollen vector, seed dispersal mode), one ordinal (type of reproduction), one circular (beginning of flowering), and six quantitative traits (seed weight, leaf dry matter content, leaf size, specific leaf area, plant height, and duration of flowering). We selected traits related to dispersal, establishment, reproduction and persistence, as each group of traits can be differently selected by diverse urban filters. We selected traits that are widely used in functional ecology studies for their presumed roles in the context of urbanization (Knapp et al., 2010; Williams et al., 2015; Kalusová et al., 2017) and that were available for the majority of our species. We investigated the correlations among all retained biological traits to avoid potential collinearity. Highly correlated traits were not retained if the absolute values of Pearson correlation coefficient were larger than 0.7 (Dormann et al., 2013). In complement to the biological traits, we calculated species urbanity following Hill et al. (2002), which is an environmental association and not a trait per se (sensu Garnier et al., 2017). We used species urbanity a posteriori to link species urban preferences with their functional originality values. We calculated species urbanity as the mean percentage of urbanized area in all grid cells where the species occurred. In addition, we used the native status of the species that was extracted from the Île-de-France plant species list established by the Conservatoire Botanique National du Bassin Parisien (CBNBP, 2016) to compare the originality of native and non-native species. A species was defined as non-native if it was introduced by humans into the region after the year 1,500 AD.
Data Analyses
All statistical analyses were performed with R software version 3.6.0 (R Core Team, 2019), and for statistical significance, we considered a nominal type-I error α = 0.05.
Species Originality
In order to determine the originality of each species, we used the metric named AV for “average” which represents the average dissimilarity between a focal species and all others in a set (Pavoine et al., 2017). The “AV” metric requires a matrix of dissimilarity between species. Here we calculated dissimilarities in species trait values, hereafter referred to as functional distances. For that, we first log-transformed the most asymmetrically distributed quantitative traits (canopy height, leaf size, and mean seed weight) to ensure a bell-shaped distribution of values. We then used the mixed-variable coefficient of dissimilarity (Pavoine et al., 2009) associated with the most appropriate coefficients to estimate functional distances between pairs of species: the Jaccard metric (Jaccard, 1901) for binary traits, the Podani metric (Podani, 1999) for ordinal traits and the Manhattan metric (Gower, 1971) for quantitative traits.
Using the functional distances between species, we calculated the functional originality scores of each species with the distinctDis function of the R package adiv (Pavoine, 2018). Following Redding et al. (2015), for each species, we calculated a regional originality score considering all species in our dataset (rAVi) and, for each plot P, a local originality score considering only the species observed in the plot (lAViP). Thus, for a given species, local originality varies depending on the composition of plots, whereas regional originality will be constant over all plots (Figure 2). Let R designate the region (here defined as the union of all sampled plots). NP is the number of species in plot P, NR the regional number of species, and dij is the functional distance between species i and j. The regional originality of species i is calculated as follows:
If species i occurs in plot P, its local originality in plot P is calculated as follows:
To determine which species are the most original, we ranked species by their regional and local functional originality values. We also searched for species that were original at the local scale although they were not at the regional scale. To do so, we calculated, for each species i and each plot P where it occurred, the ratio of this species' local originality value to its regional originality value (lAViP / rAVi) (Figure 2).
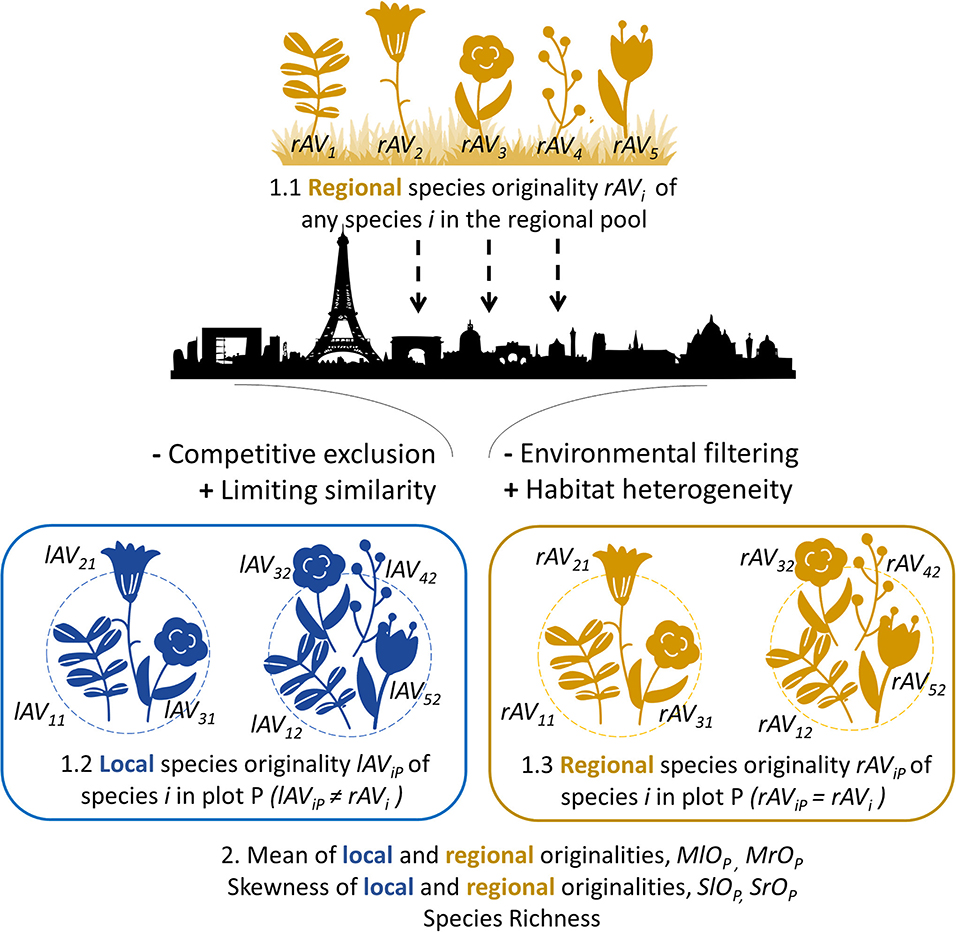
Figure 2. Scheme of our methodological framework for the analysis of species originality at regional and local spatial scales. We illustrate this framework with a theoretical set of five plant species. First, the functional originality of species is calculated at the regional scale considering all species from the regional pool (1.1, rAVi) and these values are reported for each species in a local plot P (1.3, rAViP = rAVi); originality values are also calculated at the local scale considering only species from a local plot P (1.2, lAViP). Finally, community metrics are measured for each plot P (2): the mean of regionally-measured originality values (MrOP = mean of rAViP values over i) and their skewness (SrOP = skewness of rAViP values over i), the mean of locally-measured originality values (MlOP = mean of lAViP values over i) and their skewness (SlOP = skewness of lAViP values over i), and the species richness (number of species). To illustrate potential effects of urbanization (represented by the city silhouette) on plant species communities, we indicated four key possible processes acting on the assembly of urban communities with potential positive or negative effect on community metrics (see Table 1 for a detailed description of possible scenarios of functional originality patterns in urban communities).
Community-Level Metrics
In order to assess how urbanization filters species from the regional pool (Table 1), we first calculated the mean of the regional species originalities, MrOP, taken from regionally calculated values (rAVi) but only for species composing plot P (Figure 2):
Then, for plot P, we also calculated the mean of the local originalities (lAViP) of its species, MlOP:
MlOP turns out to be a measure of the local functional diversity within plot P, equal to the index previously named “average of the pairwise distances” (Heemsbergen et al., 2004; Petchey and Gaston, 2006; APWD). The advantages of our formulations of MlOP over APWD are first, the possibility to partition MlOP into species-specific originality values in order to assess the respective contribution of each species to functional diversity and second, the possibility to extend this formulation to have a local mean of species originalities measured across different spatial scales (notably the local scale with MlOP and the regional scale with MrOP). Thus, species responses to environmental factors can be studied across spatial scales, measured by their originality, as shown in Table 1 and Figure 2.
In addition, to test if the observed variations in local MrOP and MlOP were due to filtering of many or few highly original species, we calculated the skewness (index b1 in Joanes and Gill, 1998, implemented in the R package e1071, Meyer et al., 2019) of the functional originalities in each plot P, noted as SrOP for regional originalities and SlOP for local originalities:
Negative skewness of originality (=left-skewed originality) represented scenarios with many species of high originality (approaching uniqueness) and few species of low originality (approaching redundancy). In contrast, positive skewness of originality (=right-skewed originality) represented many species of low originality and a few highly original species (Figure S1). Close-to-zero skewness represented a balanced distribution between redundant and unique species. Skewness measures of the shape of the originality distribution in a plot can complement mean measures to identify potential processes of community assembly as shown in Table 1.
We then created a dataset without non-native species (85 species) and recalculated all local and regional originality scores and all community-level metrics (species richness and mean and skewness of the originalities) to assess the contributions of non-native species to originality. Finally, the species richness was calculated per plot for both datasets (complete and without non-natives).
Statistical Modeling
In order to estimate the effects of urbanization filters, species richness and survey year on local community assembly and diversity, we modeled the variation in community-level metrics with the complete dataset and the only native species dataset with generalized mixed linear models (GLMM) in the R package glmmTMB (Brooks et al., 2017). The mean of regional MrOP and local MlOP originalities, the skewness of regional SrOP and local SlOP originalities and the ratio of local to regional originality lAViP/rAVi were modeled with a Gaussian distribution, while the species richness was modeled with a negative binomial distribution and a quadratic link between the mean and the variance. We included as fixed explanatory variables: the percentage of built-up areas in 1 km2 cell where a given plot was located (urbanization), the species richness (except for the model where species richness was a response variable) and the survey year, which was transformed by subtracting its minimum value to allow the comparison between units of variables. We also included an interaction effect between urbanization and species richness as both could simultaneously influence species originality. The IDs of the grid cells and the IDs of the plots were modeled as random nested variables to account for the pseudoreplication of the plots sampled in the same locations but in different years. To test how species originality varies between land cover types we fitted the same GLMM by replacing the percentage of built-up areas by a categorical variable indicating the land cover type where each plot was located.
Backward selection of variables was based on Akaike information criterion (AIC) values, where the lowest AIC value denoted the model we selected. We calculated the marginal and conditional R2 values as proposed in Nakagawa and Schielzeth (2013). The marginal R2 value describes the proportion of variance explained by fixed covariables only. The conditional R2 value describes the proportion of variance explained by fixed and random covariables. We tested for spatial independence in the residuals of the GLMM. We used the local indicator of spatial association (Anselin, 1995), and visualized it with a correlogram of 500 m increments for neighborhood definition. The P-value was assessed based on 999 randomizations with the R package ncf (Bjørnstad and Falck, 2001). No spatial autocorrelation was detected in the residuals of the models, and thus, no correction was required (Figure S2).
In order to assess which traits characterize the most functionally original species at regional scale and at local scale we modeled, in each land cover type, species regional originality (rAVi) and the local originalities (lAViP) averaged over all plots, with generalized least squares (GLS). GLS allowed us to include a phylogenetic correlation structure in the residuals of the model of species functional originality explained by species traits, assuming a Brownian motion model of trait evolution across the phylogenetic tree (functions corBrownian and gls from packages ape, Paradis and Schliep, 2018, and nlme, Pinheiro et al., 2019, respectively). We used DaPhnE (Durka and Michalski, 2012), a dated phylogeny that is resolved to the species level and covers the vascular flora of the British Isles, Germany, the Netherlands and Switzerland and thus all taxa from the LEDA and BiolFlor trait databases. We pruned the final tree to only include our regional species pool (Table S2). Species with missing trait values were removed from each model. Species traits were centered and scaled so that the coefficients of the GLS models were comparable. In order to visualize the GLS model results, we applied a principal component analysis (PCA) to the coefficients of GLS models. We also modeled species originality explained by species urbanity, native status and species frequency (number of times that species occurred locally across the years) with GLS models.
Results
Community-Level Metrics
The results of GLMM applied to the complete dataset are presented in Tables 2, 3. We observed a significant positive effect of urbanization percentage on mean regional and local originality values (MlO and MrO). Species richness had a significant positive effect only on MlO: the higher species richness was, the more the species differed in their traits within plots and thus the more they were functionally original at the local scale. There was, however, a significant interaction effect between urbanization and species richness, such that the positive effect of urbanization on MlO and MrO tended to decrease with increasing species richness and vice versa to the point of becoming negative for the highest levels of species richness (Figure 3). Local functional diversity MlO significantly decreased with the year of survey; so the proportion of redundant species increased locally (Table 2). Using land cover types, we similarly observed that both MlO and MrO were greater in built-up areas than in any other land cover types. We also observed the significant interaction effect between species richness and land cover types so that MlO and MrO in built-up areas decreased with increasing species richness, whereas it increased with increasing species richness in other land cover types (Table 3). The models relying on land cover type also confirmed that local functional diversity MlO significantly decreased with the year of survey (Table 3).
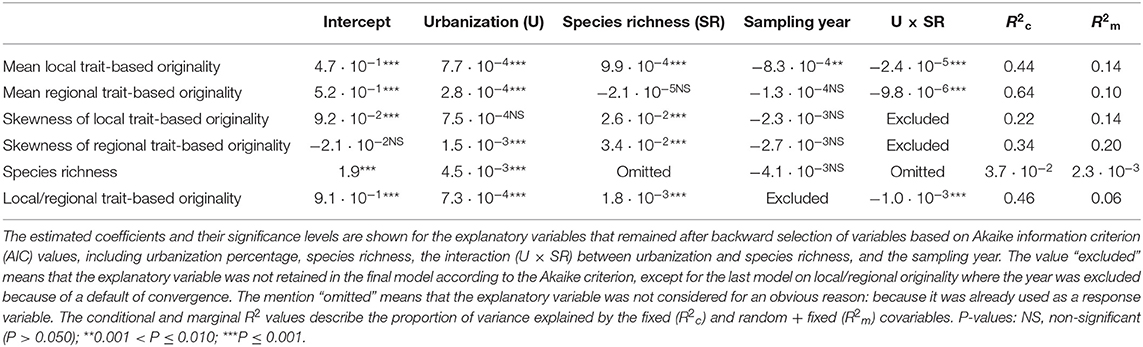
Table 2. Results for the generalized linear mixed models (GLMM) that were run on the complete dataset (native + non-native species), including community level metrics (mean and skewness of local and regional functional originalities and species richness) and the species-specific ratio of functional local originality to regional originality.
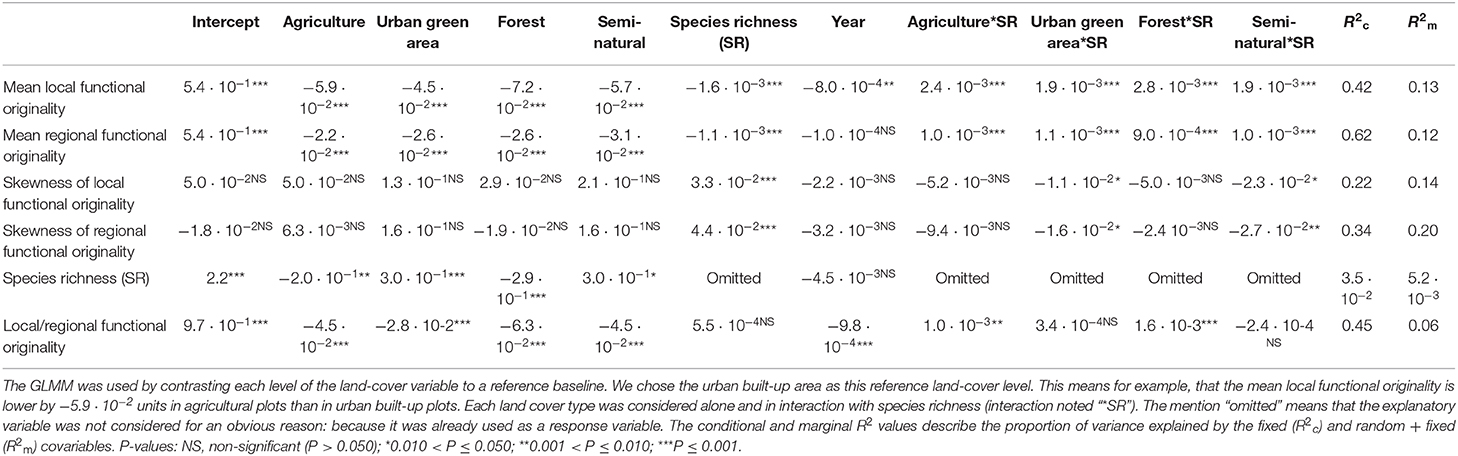
Table 3. Results for the generalized linear mixed models (GLMM) that were run on the complete dataset (native + non-native species) to explain five community level metrics (mean and skewness of local and regional functional and species richness), and the species-specific ratio of functional local originality to regional originality by different land cover types.
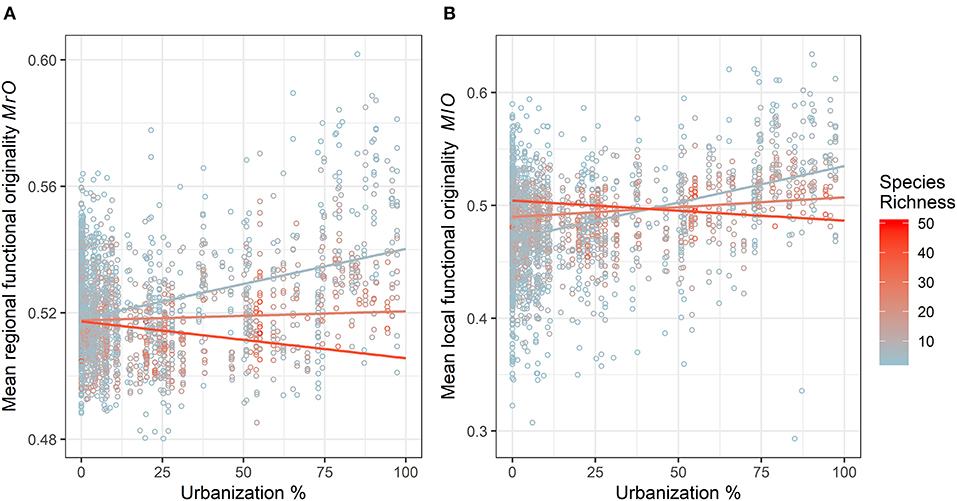
Figure 3. Variation in (A) mean regional functional originality and (B) mean local functional originality (local functional diversity) according to the percentage of urbanization within a 1 km2 grid cell (grid cell corresponds to the Vigie-flore protocol) and species richness with the complete species dataset (natives and non-natives). One data point represents one species plot. The colors represent a scale of species richness (SR) going from blue for low SR (minimum of 3 species) to red for high SR (maximum of 50 species). Colored curves represent the estimated trends of an interaction between urbanization and SR for 5 (blue), 25 (salmon), and 40 (red) species retrieved from the mean originality GLMM models.
Skewness in functional originalities (both local SlO and regional SrO) increased from close-to-zero to positive values with species richness (Figure 4) and skewness in regional originalities increased with urbanization percentage (Table 2). The models relying on land-cover rather than urbanization percentage underline that skewness increase with species richness is higher in forested, agricultural and built-up areas than in urban green areas and semi-natural areas (Table 3). The sampling year was not linked with the skewness of functional originality in any model (Table 3).
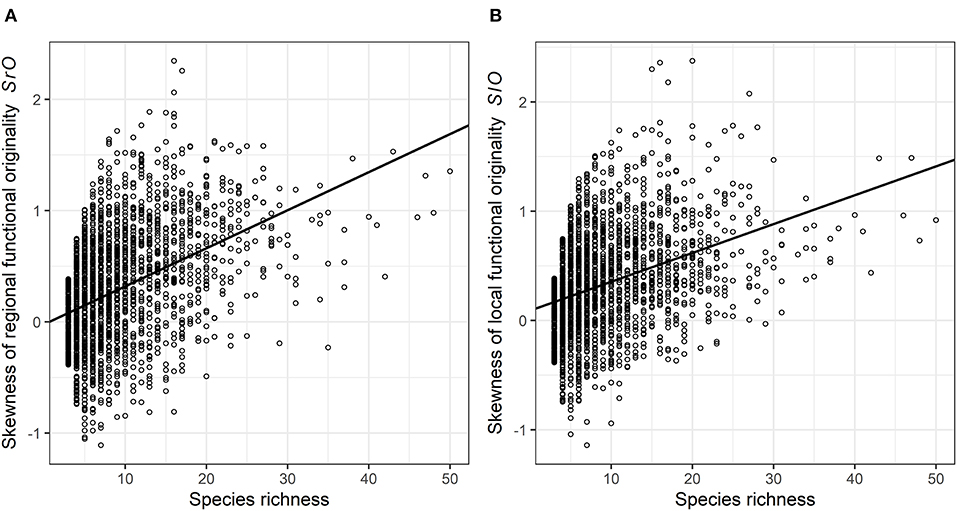
Figure 4. The skewness of the (A) regional and (B) local functional originality according to the species richness (SR) in each species plot of the complete dataset. One data point represents one plot. The black curve represents the estimated trend retrieved from the skewness of generalized mixed linear models.
Finally, species richness of the plots slightly increased along the urbanization gradient and did not change significantly across the years (Table 2). More specifically, species richness in built-up areas was significantly lower than in urban green areas and semi-natural areas and higher than in agricultural and forested areas (Table 3). However, the conditional and marginal R2 values of the models of species richness were all low, indicating that the position of a plot along the urbanization gradient was a poor predictor of species richness.
Removing 85 non-native species (representing 14.6% of the regional pool), which occurred in 709 out of 2,362 sampling plots, had a minimal effect on the coefficients of the previous models (Tables S3, S4). We found two differences. First, the year of sampling had a significant negative effect on the mean of regional functional originalities (the effect was not significant with the complete dataset). Second, the increase in skewness of local functional originality with species richness was not significantly different in urban green spaces compared to the built-up areas (lower increase in urban green spaces obtained with the complete dataset), while the increase in skewness of regional functional originality with species richness was lower in agricultural areas than in the built-up areas (equivalent increase with the complete dataset).
Species Originality
The ratio of local-to-regional functional originality (lAViP/rAVi) increased significantly with urbanization percentage and species richness and it was higher in built-up areas than in other land cover types (Tables 2, 3). Due to the interaction with species richness, the positive effect of urbanization on the ratio of local-to-regional originality decreased in richer plots and vice versa (Table 2 and Figure S3): the increase of the ratio with species richness was significant only in forested and agricultural plots (Table 3). We also found that Spearman's correlation between the mean of the local functional originalities of a species and its regional functional originality was highly significant (r = 0.78, p < 0.001).
According to GLS models with species traits as covariables, we found that regional functional originality increased in species with earlier flowering date, longer flowering duration, higher SLA and LDMC values, as well as with biannual and pluriannual life span (Figure 5). To the contrary, regional functional originality decreased in species with seed dispersal by wind, animals, humans or water; with insect and self-pollination and with perennial life span. Relatively similar patterns were found for the local functional originality particularly in built-up areas, although some correlations were not significant (Figure 5, see Table S5 and Figure S4 for details). In particular, the first axis of PCA applied to the coefficients of GLS models explained 79% of total variation indicating strong similarities in the traits that explain species functional originality across the land cover types (Figure 5). The second and third axes show more subtle differences between land cover types: notably, compared to the average trends across all land covers, species dispersed by animals were more functionally original in forested areas; perennial species were more original in agricultural and forested areas; and species pollinated by insects were less original in agricultural areas (Figure S4 and Table S5).
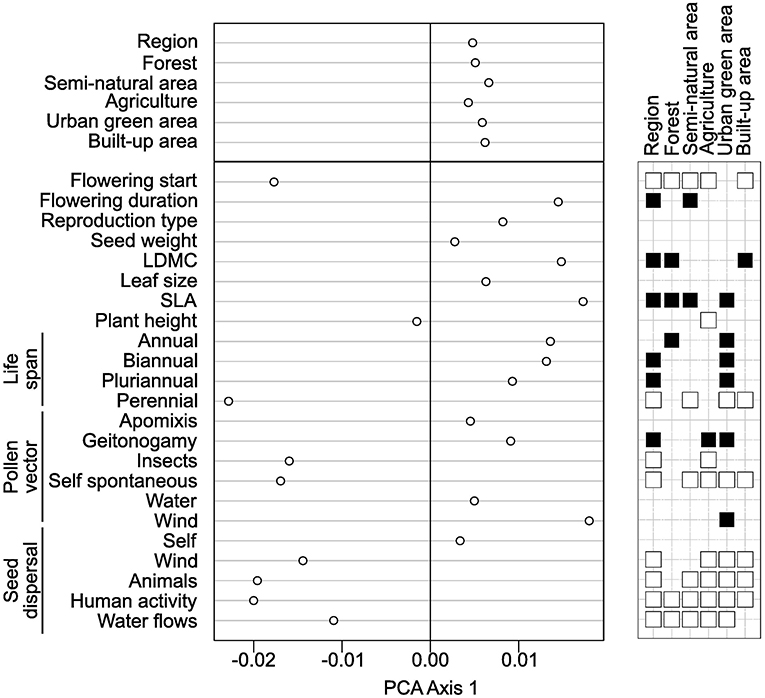
Figure 5. Analysis of the coefficients of the generalized least squares (GLS) models applied to species functional originalities with traits as explanatory variables. As indicated in the main text, we performed one model for the regional originality and one for each of the land cover types. Then, we applied a principal component analysis (PCA) on the table with land cover types as columns, traits as rows and the coefficients of the GLS models as entries (Table S5). The left part of the figure displays a dot plot with the coordinates of land covers and of traits on the first axis of the PCA. Next to the dot plot, a table indicates which traits significantly increased (black squares) or decreased (white squares) with species functional originality. The absence of a square indicates that species functional originality was found independent of the trait.
The functional originality of the 85 non-native species were on average significantly higher than those of natives at regional scale and at local scale in all land cover types (Table S6 and Figure S5). The regional originality (and local originality in urban green space) decreased with species frequency (number of plots where a species occurred). Local functional originality increased with species urbanity in green urban areas and built-up areas (Table S6). Finally, we ranked species by their local and regional functional originalities and provide the top 5% most original in Table S7.
Discussion
Urbanization affects natural ecosystem filters at multiple scales (Aronson et al., 2016) consequently changing community functional structure and trait distributions (Williams et al., 2015). However, it is still unclear how species are filtered by urbanization across spatial scales and how each species contributes to community diversity. We suggest a promising framework that relies on the originality values calculated for plant species relative to the co-occurring species within a local community or within a regional pool (Table 1). Our approach proposes a way to reveal signatures of potential processes that act on local community assembly: simultaneously giving a value to each species at two spatial scales, local and regional. Indeed, the ecological meaning of species originality is scale-dependent. While a species can be functionally redundant at the regional scale, the very same species can have a high functional originality at the local scale, if it is the sole representative species of its trait values in a local community. For example, competition may increase local originality if species with similar traits exclude each other (see e.g., Table 1, scenario 1.1.3). Inversely, while a species can be functionally original at the regional scale, the very same species can have a low functional originality in a local community, if for example rare, extreme environmental conditions in the community allow the existence of a few, atypical species that share similar trait values, which are rare at the regional scale (see Table 1, scenario 2.1). These originality patterns can be translated into community-level metrics, by calculating the mean and the skewness of species regional or local originalities (Ricotta et al., 2016; Kondratyeva et al., 2019) and the ratio of local to regional originalities (Table 1). Compared to more traditional studies centered on diversity indices, our framework allows the identification of the traits which drive species originality at the regional and at the local scales, considering different land cover types. Our study allowed both the development and a first application of such a framework of species originality to a real dataset extracted from a citizen science program.
As expected, highly original species at the regional scale were the least frequent (found in a low number of plots). Species functionally-original at local and regional scales were urbanophile, i.e., found more frequently in the urban environmental conditions than in the surrounding non-urban environment, which corresponds to scenarios 1.1.1, 1.1.2, and 1.1.3 in Table 1. Urbanophile species are often restricted to some specific urban habitats or urban ecological conditions, thus, even in urban areas they are often scarce (Hill et al., 2002; Vallet et al., 2016). We identified trait values associated with species originality and part of these trait values were previously found to be favored in urban environment: early and long flowering, promoted by higher urban temperatures (Mimet et al., 2009); annual and biannual life span more adapted to rapidly changing urban environments (Duncan et al., 2011); wind-pollination in absence of animal pollinators (Lososová et al., 2006); higher specific leaf area values favoring plant competitive abilities in high soil nutrient conditions present in urbanized areas (Song et al., 2019). High leaf dry matter content (LDMC) indicates a slower growth rate and longer life span as an adaptation to rarely disturbed environments (Cornelissen et al., 2003) contrary to low LDMC values more likely to be observed in areas disturbed by urbanization (Cornelissen et al., 2003; Knapp et al., 2008b). Higher LDMC values observed in more original species in built-up areas could thus be related to a few urbanophobe or urbanoneutral species that remain in the urbanized areas. The fact that both local and regional originalities increased with urbanization suggests that original urbanophile species may have different combinations of those original trait values, leading to increased functional diversity within urban communities.
Non-native species are often promoted in areas disturbed by urbanization (Kowarik, 2008). We thus searched whether they could be responsible for the observed increase in functional originality and diversity in built-up areas. Nevertheless, in our dataset non-natives represented 14% of the total species pool, which could not be sufficient to contribute substantially to the community diversity, and their removal in our data hardly influenced community structure and diversity. Moreover, our 586 species set was a sample of the whole Île-de-France region flora, where more than 2,064 vascular plant species have been recorded (CBNBP, 2016). Thus, probably many non-native species were missing at the regional scale in our study, as the Vigie-flore program does not aim to provide an exhaustive sampling of the flora of Île-de-France region. Nevertheless, we found that non-native species were more functionally original than native species at both the local and regional scales. Non-native species which are frequent in urban areas, have some specific environmental preferences, making them highly original specialists within urban habitat patches (McKinney, 2006; Godefroid and Ricotta, 2018). They may thus contribute partly to the observed increased originality in urban areas. As removing non-native species from our data set had a minimal effect on the results of our models, the increased originality in built-up areas also emerges from the non-random distribution of native species among the different land cover types of the region.
We indeed observed an increase with urbanization of both mean local and mean regional originality with and without non-native species. This increase corresponds to the scenarios 1.1.1, 1.1.2, and 1.1.3 in Table 1 where high regional originality in urban areas may be due to urban conditions allowing the persistence of species with unique traits not observed, or observed less frequently in other land cover types (e.g., forest, agriculture) (e.g., Williams et al., 2009). Regionally-original species in that case tend to be urbanophile and their originality results from the environmental heterogeneity of the region. Our analyses of the skewness of species originalities, of the ratio of local to regional originalities and of species richness complemented those of the mean originality to distinguish between the three alternative scenarios (1.1.1, 1.1.2, and 1.1.3 in Table 1). Locally, interspecific interactions, such as competition between species for limited resources, limit the functional similarity between species (Stubbs and Wilson, 2004). This limitation may restrict the number of species and increase functional originality in the most urbanized areas, promoting several combinations of urbanophile traits (Scenario 1.1.1, Table 1). The same originality pattern would also arise from facilitation interactions between species, making plant communities to function interdependently (Lortie et al., 2004). However, mean originality decreased with species richness in built-up areas whereas it hardly varied at the regional scale in other land cover types and even increased at the local scale in other land cover types. The increase in mean species originality was thus limited to a certain level of species richness in urbanized communities. Beyond that level, the number of redundant species started to increase, as reflected by the positive skewness of the originality values. In built-up areas, the ratio of local-to-regional originalities was close to unity, indicating similar values of originality at both scales. These patterns suggest that scenario 1.1.2 in Table 1 could explain the composition of species-rich communities in built-up areas. Therefore, in richer urbanized communities, interspecific competition may be relaxed, allowing the coexistence of original urbanophile species and more frequent redundant species (e.g., under stochasticity of colonization and survival of plant individuals if species have similar fitness levels and resources allowing their coexistence in urban species-rich plots, Scenario 1.1.2, Table 1). These observed interactions between urbanization and species richness in patterns of mean and skewness of functional originalities show that urban conditions involve different constraints than the other non-urban land cover types on the number of unique vs. redundant species that can coexist locally.
Throughout the sampling years, removing non-native species from our data set, we observed a decrease in mean regional functional originalities (the decrease was not significant with the complete dataset). This may indicate that the decrease through time of the proportion of native, regionally-original species in communities is compensated, as regards regional functional diversity, by the presence of non-native regionally-original species. Independently of the consideration of non-native species, functional originality decreased at the local scale, which may indicate a decreased proportion of original urbanophile species in the most urbanized areas (as reflected by a decrease in mean local originality) and a decreased importance of processes limiting similarity between species (such as competition for resources; as reflected by the decrease in the ratio of local to regional originality). Urban land modification may intensify with time and indicate a growing homogenization of plant communities across the region (McKinney, 2006) and an impoverishment of the diversity of species traits. More research would be needed to determine whether the most original species will persist with growing urbanization and whether they have an important role in ecosystem functioning. Indeed, the most important ecosystem processes are usually ensured by common and redundant species (Mouillot et al., 2013b; Brandl et al., 2016). For example, Taraxacum sect. Ruderalia and Poa annua are urbanoneutral species (Hill et al., 2002). They are frequent in disturbed habitats of both urban and rural landscapes (such as lawns, road verges, streets, cultivated areas) and are less affected than other species by urbanization disturbances (Vallet et al., 2016). As original species could maintain unique, potentially vulnerable, ecosystem functions (Mouillot et al., 2013b), irreplaceable at community level, the presence of such original species in highly urbanized areas questions the stability of plant biodiversity in Île-de-France region, if these species disappeared.
In our study, the urbanization gradient was characterized by a single measure, i.e., the proportion of built-up areas (including individual and collective housing, industrial and business areas, facilities, quarries, dumps and worksites and transportation infrastructure). Although this urbanization measure combines diverse types of urban infrastructures and impervious surfaces, it does not distinguish among the range of urban microhabitats and does not account for many urbanization-related changes, such as soil compaction, eutrophication and air, soil and water pollution (McDonnell and Hahs, 2008). However, we found significant differences in species functional originality and diversity between built-up areas and green urban areas. In particular, mean local and regional functional originalities were higher in built-up areas than in green urban areas and they decreased with species richness in built-up areas but did not in green urban areas. Therefore, future urban ecology studies could use multidimensional composite measures of urbanization to provide a more holistic understanding of the effects of urbanization on functional originality and diversity (Moll et al., 2019).
In addition, these conclusions rely on the presence/absence of species in plots as our dataset did not allow us to account for species abundance. Abundance data could change part of our conclusions about ecosystem functioning, if a high number of individuals supporting the same trait can ensure greater stability (Walker et al., 1999). The framework we proposed can easily be extended to abundance data for future studies as the AV originality index for a given species has already been extended to a weighted mean of the functional distances to all other species by Ricotta et al. (2016). The weighting of originality measure with species relative abundances could regulate the relative importance given to rare vs. abundant species (Kondratyeva et al., 2019).
With this study, we demonstrated that applied to the urban context, originality measures can help to depict patterns of biodiversity and community assembly by integrating multiscale biological species information and to identify potential processes that could explain these patterns. Cities are complex urban ecosystems, and their functioning tends to be different than that of natural ecosystems because of the human element (Rebele, 1994; Grimm et al., 2000). Studying species-specific response to urbanization necessitates data for local communities across multiple locations. Today, increasingly burgeoning citizen science programs, as the one we used here, allow the assembly of large amounts of such monitoring data (Kobori et al., 2016; Silvertown, 2016). Therefore, as citizen science data accumulate across space and time, their usefulness in ecology and conservation studies is increasingly acknowledged (Dickinson et al., 2010; McKinley et al., 2017). Citizen science programs, which are deployed in urban areas, are also a good starting base for exchange in sustainable city development, which includes scientists, policymakers and citizens. Finally, future research could now aim to develop cross-city comparative research on species originality using urban system networks, such as the Urban Biodiversity Research Coordination Network (UrBioNet, http://urbionet.weebly.com/). We also demonstrated that our framework is flexible so that future studies could include more spatial scales and abundance data. Finally, while our case study focused on an urbanization context, the originality framework can be easily applied to other contexts and other regions.
Data Availability Statement
This study used plant trait values from several datasets that can be found online:
BiolFlor: data available after registration at www.biolflor.de
LEDA: available at http://www.leda-traitbase.org
Ecoflora: http://www.ecoflora.co.uk
Catminat: http://philippe.julve.pagesperso-orange.fr/catminat.htm
TRY initiative and database: http://www.try-db.org
Seed Information Database: http://data.kew.org/sid/ (September 2019)
DaPhnE phylogeny: http://esapubs.org/archive/ecol/E093/214/
CBNBP: the database of CBNBP used in this article is the catalogue that is freely accessible online http://cbnbp.mnhn.fr/cbnbp/ressources/catalogues.jsp
The datasets for land cover data and plant occurrence data are not publicly available. Requests to access these datasets should be directed to assad.ali-cherif@institutparisregion.fr for land cover data of the Île-de-France region and to vigie-flore@mnhn.fr for Vigie-flore plant occurrence data.
Author Contributions
AK and SP conceived the ideas of the research presented herein. AK, SP, and IK designed the analytical methods. WD and AK assembled the project phylogeny. AK, WD, and IK assembled the trait data. NM, GM, EM, and JV supervised the Vigie-flore data collection and database management. AK analyzed the data and wrote the first version of the manuscript. All authors contributed to the final manuscript edition and approved the submitted version.
Funding
This work was funded by a grant from the Agence Nationale de la Recherche under the LabEx BCDiv project reference 10-LABX-0003 within the framework of the program Investing for the future (11-IDEX-0004).
Conflict of Interest
The authors declare that the research was conducted in the absence of any commercial or financial relationships that could be construed as a potential conflict of interest.
Acknowledgments
We thank Emmanuelle Porcher for the valuable comments on previous versions of the manuscript and all Vigie-flore volunteers for data collection. The study has been supported by the TRY initiative on Plant Traits (http://www.try-db.org). The TRY initiative and database is hosted, developed, and maintained by J. Kattge and G. Boenisch (Max Planck Institute for Biogeochemistry, Jena, Germany). TRY is currently supported by Future Earth/bioDISCOVERY and the German Center for Integrative Biodiversity Research (iDiv) Halle-Jena-Leipzig.
Supplementary Material
The Supplementary Material for this article can be found online at: https://www.frontiersin.org/articles/10.3389/fevo.2020.00073/full#supplementary-material
References
Anselin, L. (1995). Local indicators of spatial association-LISA. Geogr. Anal. 27, 93–116. doi: 10.1111/j.1538-4632.1995.tb00338.x
Araújo, M. B. (2003). The coincidence of people and biodiversity in Europe. Glob. Ecol. Biogeogr. 12, 5–12. doi: 10.1046/j.1466-822X.2003.00314.x
Aronson, M. F. J., Nilon, C. H., Lepczyk, C. A., Parker, T. S., Warren, P. S., Cilliers, S. S., et al. (2016). Hierarchical filters determine community assembly of urban species pools. Ecology 97, 2952–2963. doi: 10.1002/ecy.1535
Balmford, A., Moore, J. L., Brooks, T., Burgess, N., Hansen, L. A., Williams, P. H., et al. (2001). Conservation conflicts across Africa. Science 291, 2616–2619. doi: 10.1126/science.291.5513.2616
Bjørnstad, O. N., and Falck, W. (2001). Nonparametric spatial covariance functions: estimation and testing. Environ. Ecol. Stat. 8, 53–70. doi: 10.1023/A:1009601932481
Brandl, S. J., Emslie, M. J., and Ceccarelli, D. M. (2016). Habitat degradation increases functional originality in highly diverse coral reef fish assemblages. Ecosphere 7:e01557. doi: 10.1002/ecs2.1557
Brooks, M. E., Kristensen, K., van Benthem, K. J., Magnusson, A., Berg, C. W., Nielsen, A., et al. (2017). glmmTMB balances speed and flexibility among packages for zero-inflated generalized linear mixed modeling. R. J. 9, 378–400. doi: 10.32614/RJ-2017-066
Bühler, C., and Roth, T. (2011). Spread of common species results in local-scale floristic homogenization in grassland of Switzerland: floristic homogenization in Swiss grassland. Divers. Distrib. 17, 1089–1098. doi: 10.1111/j.1472-4642.2011.00799.x
CBNBP (2016). Catalogue de la Flore de l'Île-de-France, Version mai 2016. Available online at: http://cbnbp.mnhn.fr/cbnbp/ressources/catalogues.jsp (accessed February 1, 2019).
Concepción, E. D., Moretti, M., Altermatt, F., Nobis, M. P., and Obrist, M. K. (2015). Impacts of urbanisation on biodiversity: the role of species mobility, degree of specialisation and spatial scale. Oikos 124, 1571–1582. doi: 10.1111/oik.02166
Cornelissen, J. H. C., Lavorel, S., Garnier, E., Díaz, S., Buchmann, N., Gurvich, D. E., et al. (2003). Handbook of protocols for standardised and easy measurement of plant functional traits worldwide. Aust. J. Bot. 51, 335–380. doi: 10.1071/BT02124
Cornwell, W. K., and Ackerley, D. D. (2009). Community assembly and shifts in plant trait distributions across an environmental gradient in coastal California. Ecol. Monogr. 79, 109–126. doi: 10.1890/07-1134.1
de Bello, F., Price, J. N., Munkermuller, T., Liira, J., Zobel, M., Thuiller, W., et al. (2012). Functional species pool framework to test for biotic effects on community assembly. Ecology 93, 2263–2273. doi: 10.1890/11-1394.1
de Bello, F., Vandewall, M., Reitalu, T., Lepš, J., Prentice, H. C., Lavorel, S., et al. (2013). Evidence for scale- and disturbance-dependent trait assembly patterns in dry semi-natural grasslands. J. Ecol. 101, 1237–1244. doi: 10.1111/1365-2745.12139
Deguines, N., Julliard, R., de Flores, M., and Fontaine, C. (2016). Functional homogenization of flower visitor communities with urbanization. Ecol. Evol. 6, 1967–1976. doi: 10.1002/ece3.2009
Dickinson, J. L., Zuckerberg, B., and Bonter, D. N. (2010). Citizen science as an ecological research tool: challenges and benefits. Annu. Rev. Ecol. Evol. Syst. 41, 149–172. doi: 10.1146/annurev-ecolsys-102209-144636
Dormann, C. F., Elith, J., Bacher, S., Buchmann, C., Carl, G., Carré, G., et al. (2013). Collinearity: a review of methods to deal with it and a simulation study evaluating their performance. Ecography 36, 27–46. doi: 10.1111/j.1600-0587.2012.07348.x
Duncan, R. P., Clemants, S. E., Corlett, R. T., Hahs, A. K., McCarthy, M. A., McDonnell, M. J., et al. (2011). Plant traits and extinction in urban areas: a meta-analysis of 11 cities. Glob. Ecol. Biogeogr. 20, 509–519. doi: 10.1111/j.1466-8238.2010.00633.x
Durka, W., and Michalski, S. G. (2012). Daphne: a dated phylogeny of a large European flora for phylogenetically informed ecological analyses. Ecology 93, 2297–2297. doi: 10.1890/12-0743.1
Fitter, A. H., and Peat, H. J. (1994). The ecological flora database. J. Ecol. 82, 415–425. doi: 10.2307/2261309
Fournier, B., Frey, D., and Moretti, M. (2019). The origin of urban communities: from the regional species pool to community assemblages in city. J. Biogeorg. 47, 615–629. doi: 10.1111/jbi.13772
Garnier, E., Stahl, U., Laporte, M. A., Kattge, J., Mougenot, I., Kühn, I., et al. (2017). Towards a thesaurus of plant characteristics: an ecological contribution. J. Ecol. 105, 298–309. doi: 10.1111/1365-2745.12698
Gaston, K. (ed.). (2010). Urban Ecology. Cambridge: Cambridge University Press. doi: 10.1017/CBO9780511778483
Godefroid, S., and Koedam, N. (2007). Urban plant species patterns are highly driven by density and function of built-up areas. Landsc. Ecol. 22, 1227–1239. doi: 10.1007/s10980-007-9102-x
Godefroid, S., and Ricotta, C. (2018). Alien plant species do have a clear preference for different land uses within urban environments. Urban Ecosyst. 21, 1189–1198. doi: 10.1007/s11252-018-0792-4
Gower, J. C. (1971). A general coefficient of similarity and some of its properties. Biometrics 27, 857–871. doi: 10.2307/2528823
Grimm, N. B., Grove, J. M., Pickett, S. T. A., and Redman, C. A. (2000). Integrated approaches to long-term studies of urban ecological systems. BioScience 50, 571–584. doi: 10.1641/0006-3568(2000)050[0571:IATLTO]2.0.CO;2
Guetté, A., Gaüzère, P., Devictor, V., Jiguet, F., and Godet, L. (2017). Measuring the synanthropy of species and communities to monitor the effects of urbanization on biodiversity. Ecol. Indic. 79, 139–154. doi: 10.1016/j.ecolind.2017.04.018
Heemsbergen, D. A., Berg, M. P., Loreau, M., van Hal, J. R., Faber, J. H., and Verhoef, H. A. (2004). Biodiversity effects on soil processes explained by intraspecific functional dissimilarity. Science 306, 1019–1020. doi: 10.1126/science.1101865
Hill, M. O., Roy, D. B., and Thompson, K. (2002). Hemeroby, urbanity and ruderality: bioindicators of disturbance and human impact. J. Appl. Ecol. 39, 708–720. doi: 10.1046/j.1365-2664.2002.00746.x
Ibáñez-Álamo, J. D., Rubio, E., Benedetti, Y., and Morelli, F. (2017). Global loss of avian evolutionary uniqueness in urban areas. Glob. Chang. Biol. 23, 2990–2998. doi: 10.1111/gcb.13567
Institut Paris Region (2018). Population des communes et mode d'occupation de sol (MOS) en Île-de-France. Paris. Available online at: https://www.institutparisregion.fr/ (accessed July 2, 2018).
Ives, C. D., Lentini, P. E., Threlfall, C. G., Ikin, K., Shanahan, D. F., Garrard, G. E., et al. (2016). Cities are hotspots for threatened species. Glob. Ecol. Biogeogr. 25, 117–126. doi: 10.1111/geb.12404
Jaccard, P. (1901). Etude comparative de la distribution florale dans une portion des Alpes et des Jura. Bull. Soc. Vaud. Sci. Nat. 37, 547–579.
Joanes, D. N., and Gill, C. A. (1998). Comparing measures of sample skewness and kurtosis. Statistician 47, 183–189. doi: 10.1111/1467-9884.00122
Julve, P. (1998). Baseflor. Index Botanique, Écologique et Chorologique de la Flore de France. Version 2019. Available online at: http://philippe.julve.pagesperso-orange.fr/catminat.htm (accessed February 1, 2019).
Kalusová, V., Ceplová, N., and Lososová, Z. (2017). Which traits influence the frequency of plant species occurrence in urban habitat types? Urban Ecosyst. 20, 65–75. doi: 10.1007/s11252-016-0588-3
Kattge, J., Díaz, S., Lavorel, S., Prentice, I. C., Leadley, P., and Bönisch, G. (2011). TRY – a global database of plant traits. Glob. Change Biol. 17, 2905–2935. doi: 10.1111/j.1365-2486.2011.02451.x
Kleyer, M., Bekker, R. M., Knevel, I. C., Bakker, J. P., Thompson, K., Sonnenschein, M., et al. (2008). The LEDA traitbase: a database of life-history traits of the Northwest European flora. J. Ecol. 96, 1266–1274. doi: 10.1111/j.1365-2745.2008.01430.x
Klotz, S., Kühn, I., and Durka, W. (eds.). (2002). BIOLFLOR - Eine Datenbank zu Biologisch-Ökologischen Merkmalen der Gefäßpflanzen in Deutschland. Bonn: Bundesamt für Naturschutz.
Knapp, S., Kühn, I., Schweiger, O., and Klotz, S. (2008a). Challenging urban species diversity: contrasting phylogenetic patterns across plant functional groups in Germany. Ecol. Lett. 11, 1054–1064. doi: 10.1111/j.1461-0248.2008.01217.x
Knapp, S., Kühn, I., Stolle, J., and Klotz, S. (2010). Changes in the functional composition of a Central European urban flora over three centuries. Perspect. Plant Ecol. Evol. Syst. 12, 235–244. doi: 10.1016/j.ppees.2009.11.001
Knapp, S., Kühn, I., Wittig, R., Ozinga, W. A., Poschlod, P., and Klotz, S. (2008b). Urbanization causes shifts in species' trait state frequencies. Preslia 80, 375–388.
Kobori, H., Dickinson, J. L., Washitani, I., Sakurai, R., Amano, T., Komatsu, N., et al. (2016). Citizen science: a new approach to advance ecology, education, and conservation. Ecol. Res. 31, 1–19. doi: 10.1007/s11284-015-1314-y
Kondratyeva, A., Grandcolas, P., and Pavoine, S. (2019). Reconciling the concepts and measures of diversity, rarity and originality in ecology and evolution. Biol. Rev. 94, 1317–1337. doi: 10.1111/brv.12504
Kowarik, I. (2008). “On the role of alien species in urban flora and vegetation,” in: Urban Ecology. An International Perspective on the Interaction Between Humans and Nature, eds J. M. Marzluff, E. Shulenberger, W. Endlicher, M. Alberti, G. Bradley, C. Ryan, U. Simon, and C. ZumBrunnen (New York, NY: Springer), 321–338.
Kowarik, I. (2011). Novel urban ecosystems, biodiversity, and conservation. Environ. Pollut. 159, 1974–1983. doi: 10.1016/j.envpol.2011.02.022
Kühn, I., Brandl, R., and Klotz, S. (2004). The flora of German cities is naturally species rich. Evol. Ecol. Res. 6, 749–764.
Kühn, I., and Klotz, S. (2006). Urbanization and homogenization - comparing the floras of urban and rural areas in Germany. Biol. Conserv. 127, 292–300. doi: 10.1016/j.biocon.2005.06.033
La Sorte, F. A., Lepczyk, C. A., Aronson, M. F. J., Goddard, M. A., Hedblom, M., Katti, M., et al. (2018). The phylogenetic and functional diversity of regional breeding bird assemblages is reduced and constricted through urbanization. Divers. Distrib. 24, 928–938. doi: 10.1111/ddi.12738
Lavorel, S., and Garnier, E. (2002). Predicting changes in community composition and ecosystem functioning from plant traits: revisiting the Holy Grail. Funct. Ecol. 16, 545–556. doi: 10.1046/j.1365-2435.2002.00664.x
Lessard, J. P., Belmaker, J., Myers, J. A., Chase, J. M., and Rahbek, C. (2012). Inferring local ecological processes amid species pool influences. Trends Ecol. Evol. 27, 600–607. doi: 10.1016/j.tree.2012.07.006
Lortie, C. J., Brooker, R. W., Choler, P., Kikvidze, Z., Michalet, R., Pugnaire, F. I., et al. (2004). Rethinking plant community theory. Oikos 107, 433–438. doi: 10.1111/j.0030-1299.2004.13250.x
Lososová, Z., Ceplová, N., Chytrý, M., Tichý, L., Danihelka, J., Fajmon, K., et al. (2016). Is phylogenetic diversity a good proxy for functional diversity of plant communities? A case study from urban habitats. J. Veg. Sci. 27, 1036–1046. doi: 10.1111/jvs.12414
Lososová, Z., Chytrý, M., Kühn, I., Hájek, O., Horáková, V., Pyšek, P., et al. (2006). Patterns of plant traits in annual vegetation of man-made habitats in central Europe. Perspect. Plant Ecol. Evol. Syst. 8, 69–81. doi: 10.1016/j.ppees.2006.07.001
Mason, N. W. H., de Bello, F., Doležal, J., and Lepš, J. (2011). Niche overlap reveals the effects of competition, disturbance and contrasting assembly processes in experimental grassland communities. J. Ecol. 99, 788–796. doi: 10.1111/j.1365-2745.2011.01801.x
McArthur, R. H., and Levins, R. (1967). The limiting similarity, convergence, and divergence of coexisting species. Am. Nat. 101, 377–385. doi: 10.1086/282505
McDonnell, M. J., and Hahs, A. K. (2008). The use of gradient analysis studies in advancing our understanding of the ecology of urbanizing landscapes: current status and future directions. Landsc. Ecol. 23, 1143–1155. doi: 10.1007/s10980-008-9253-4
McKinley, D. C., Miller-Rushing, A. J., Ballard, H. L., Bonney, R., Brown, H., Cook-Patton, S. C., et al. (2017). Citizen science can improve conservation science, natural resource management, and environmental protection. Biol. Conserv. 208, 15–28. doi: 10.1016/j.biocon.2016.05.015
McKinney, M. L. (2006). Urbanization as a major cause of biotic homogenization. Biol. Conserv. 127, 247–260. doi: 10.1016/j.biocon.2005.09.005
Meyer, D., Dimitriadou, E., Hornik, K., Weingessel, A., and Leisch, F. (2019). e1071: Misc Functions of the Department of Statistics, Probability Theory Group (Formerly: E1071), TU Wien. R package version 1.7-3. Available online at: https://CRAN.R-project.org/package=e1071 (accessed January 30, 2020).
Mimet, A., Pellissier, V., Quénol, H., Aguejdad, R., Dubreuil, V., and Rozé, F. (2009). Urbanisation induces early flowering: evidence from Platanus acerifolia and Prunus cerasus. Int. J. Biometeorol. 53, 287–298. doi: 10.1007/s00484-009-0214-7
Moll, R. J., Cepek, J. D., Lorch, P. D., Dennis, P. M., Tans, E., Robison, T., et al. (2019). What does urbanization actually mean? A framework for urban metrics in wildlife research. J. Appl. Ecol. 56, 1289–1300. doi: 10.1111/1365-2664.13358
Mouillot, D., Bellwood, D. R., Baraloto, C., Chave, J., Galzin, R., Harmelin-Vivien, M., et al. (2013a). Rare species support vulnerable functions in high-diversity ecosystems. PLoS Biol. 11:e1001569. doi: 10.1371/journal.pbio.1001569
Mouillot, D., Graham, N. A. J., Villéger, S., Mason, N. W. H., and Bellwood, D. R. (2013b). A functional approach reveals community responses to disturbances. Trends Ecol. Evol. 28, 167–177. doi: 10.1016/j.tree.2012.10.004
Nakagawa, S., and Schielzeth, H. (2013). A general and simple method for obtaining R2 from generalized linear mixed-effects models. Methods Ecol. Evol. 4, 133–142. doi: 10.1111/j.2041-210x.2012.00261.x
Paradis, E., and Schliep, K. (2018). Ape 5.0: an environment for modern phylogenetics and evolutionary analyses in R. Bioinformatics 35, 526–528. doi: 10.1093/bioinformatics/bty633
Pautasso, M., Böhning-Gaese, K., Clergeau, P., Cueto, V. R., Dinetti, M., Fernández-Juricic, E., et al. (2011). Global macroecology of bird assemblages in urbanized and semi-natural ecosystems. Glob. Ecol. Biogeogr. 20, 426–436. doi: 10.1111/j.1466-8238.2010.00616.x
Pavoine, S. (2018). Adiv: Analysis of Diversity. R package version 1.2. Available online at: https://CRAN.R-project.org/package=adiv (accessed January 30, 2020).
Pavoine, S., Bonsall, M. B., Dupaix, A., Jacob, U., and Ricotta, C. (2017). From phylogenetic to functional originality: guide through indices and new developments. Ecol. Indic. 82, 196–205. doi: 10.1016/j.ecolind.2017.06.056
Pavoine, S., Ollier, S., and Dufour, A. B. (2005). Is the originality of a species measurable? Ecol. Lett. 8, 579–586. doi: 10.1111/j.1461-0248.2005.00752.x
Pavoine, S., Vallet, J., Dufour, A.-B., Gachet, S., and Daniel, H. (2009). On the challenge of treating various types of variables: application for improving the measurement of functional diversity. Oikos 118, 391–402. doi: 10.1111/j.1600-0706.2008.16668.x
Petchey, O. L., and Gaston, K. J. (2006). Functional diversity: back to basics and looking forward. Ecol. Lett. 9, 741–758. doi: 10.1111/j.1461-0248.2006.00924.x
Pinheiro, J., Bates, D., DebRoy, S., Sarkar, D., and R Core Team. (2019). nlme: Linear and Nonlinear Mixed Effects Models. R package version 3.1–142. Available online at: https://CRAN.R-project.org/package=nlme (accessed January 30, 2020).
Podani, J. (1999). Extending gower's general coefficient of similarity to ordinal characters. Taxon 48, 331–340. doi: 10.2307/1224438
POWO (2019). Plants of the World Online. Facilitated by the Royal Botanic Gardens, Kew. Available online at: http://www.plantsoftheworldonline.org/ (accessed January 1, 2019).
R Core Team (2019). R: A Language and Environment for Statistical Computing. Vienna: R Foundation for Statistical Computing. Available online at: http://www.r-project.org/
Rebele, F. (1994). Urban ecology and special features of urban ecosystems. Glob. Ecol. Biogeogr. 4, 173–187. doi: 10.2307/2997649
Redding, D. W., Mazel, F., and Mooers, A. Ø. (2014). Measuring evolutionary isolation for conservation. PLOS ONE 9:e113490. doi: 10.1371/journal.pone.0113490
Redding, D. W., Mooers, A. O., Sekercioglu, Ç. H., and Collen, B. (2015). Global evolutionary isolation measures can capture key local conservation species in Nearctic and Neotropical bird communities. Phil. Trans. R Soc. Lond B Biol. Sci. 370:20140013. doi: 10.1098/rstb.2014.0013
Ricotta, C., de Bello, F., Moretti, M., Caccianiga, M., Ceranolini, B., and Pavoine, S. (2016). Measuring the functional redundancy of biological communities: a quantitative guide. Methods Ecol. Evol. 7, 1386–1395. doi: 10.1111/2041-210X.12604
Ricotta, C., La Sorte, F. A., Pyšek, P., Rapson, G. L., Celesti-Grapow, L., and Thompson, K. (2009). Phyloecology of urban alien floras. J. Ecol. 97, 1243–1251. doi: 10.1111/j.1365-2745.2009.01548.x
Silvertown, J. (2016). A new dawn for citizen science. Trends Ecol. Evol. 24, 467–471. doi: 10.1016/j.tree.2009.03.017
Soanes, K., and Lentini, P. E. (2019). When cities are the last chance for saving species. Front. Ecol. Environ. 17, 225–231. doi: 10.1002/fee.2032
Sodhi, D. S., Livingstone, S. W., Carboni, M., and Cadotte, M. W. (2019). Plant invasion alters trait composition and diversity across habitats. Ecol. Evol. 9, 6199–6210. doi: 10.1002/ece3.5130
Song, G., Wang, J., Han, T., Wang, Q., Ren, H., Zhu, H., et al. (2019). Changes in plant functional traits and their relationships with environmental factors along an urban-rural gradient in Guangzhou, China. Ecol. Indic. 106:105558. doi: 10.1016/j.ecolind.2019.105558
Spasojevic, M. J., Catano, C. P., LaManna, J. A., and Myers, J. A. (2018). Integrating species into species pools. Ecology 99, 1265–1276. doi: 10.1002/ecy.2220
Stubbs, W. J., and Wilson, J. B. (2004). Evidence for limiting similarity in a sand dune community. J. Ecol. 92, 557–567. doi: 10.1111/j.0022-0477.2004.00898.x
Swan, C. M., Pickett, S. T. A., Szlavecz, K., Waren, P., and Willey, T. (2011). “Chapter 3.5: Urban ecology: patterns, processes, and applications,” in Biodiversity and Community Composition in Urban Ecosystems: Coupled Human, Spatial, and Metacommunity Processes, ed J. Niemela (Oxford: Oxford University Press), 179–186. doi: 10.1093/acprof:oso/9780199563562.003.0021
United Nations (2018). World Urbanization Prospects. The 2018 Revision. Highlights. Available online at: https://population.un.org/wup/Publications/ (accessed June 30, 2019).
Vallet, J., Rambaud, M., Filoche, S., and Hendoux, F. (2016) Fiche Indicateur CBNBP-Indic003-IdF: Portrait de la Flore en Milieu Urbain, Paris, Conservatoire Botanique National du Bassin Parisien. Available online at: https://hal.archives-ouvertes.fr/hal-01922837v2/document (accessed February 1, 2019).
Vimal, R., and Devictor, V. (2014). Building relevant ecological indicators with basic data: Species and community specialization indices derived from atlas data. Ecol. Indic. 50, 1–7. doi: 10.1016/j.ecolind.2014.10.024
Violle, C., Thuiller, W., Mouquet, N., Munoz, F., Kraft, N. J. B., Cadotte, M. W., et al. (2017). Functional rarity: the ecology of outliers. Trends Ecol. Evol. 32, 356–367. doi: 10.1016/j.tree.2017.02.002
Walker, B., Kinzig, A., and Langridge, J. (1999). Plant attribute diversity, resilience, and ecosystem function: the nature and significance of dominant and minor species. Ecosystems 2, 95–113. doi: 10.1007/s100219900062
Williams, N. S. G., Hahs, A. K., and Vesk, P. A. (2015). Urbanisation, plant traits and the composition of urban floras. Perspect. Plant Ecol. Evol. Syst. 17, 78–86. doi: 10.1016/j.ppees.2014.10.002
Williams, N. S. G., Schwartz, M. W., Vesk, P. A., McCarthy, M. A., Hahs, A. K., Clemants, S. E., et al. (2009). A conceptual framework for predicting the effects of urban environments on floras. J. Ecol. 97, 4–9. doi: 10.1111/j.1365-2745.2008.01460.x
Zobel, M. (1997). The relative role of species pools in determining plant species richness. An alternative explanation of species coexistence? Trends Ecol. Evol. 12, 266–269. doi: 10.1016/S0169-5347(97)01096-3
Keywords: biodiversity measure, community assembly, disturbance, environmental filtering, functional trait, originality measure, spatial scale
Citation: Kondratyeva A, Knapp S, Durka W, Kühn I, Vallet J, Machon N, Martin G, Motard E, Grandcolas P and Pavoine S (2020) Urbanization Effects on Biodiversity Revealed by a Two-Scale Analysis of Species Functional Uniqueness vs. Redundancy. Front. Ecol. Evol. 8:73. doi: 10.3389/fevo.2020.00073
Received: 23 September 2019; Accepted: 04 March 2020;
Published: 24 March 2020.
Edited by:
Heikki Martti Setälä, University of Helsinki, FinlandReviewed by:
John Kress, Smithsonian National Museum of Natural History (SI), United StatesAndreas Prinzing, University of Rennes 1, France
Copyright © 2020 Kondratyeva, Knapp, Durka, Kühn, Vallet, Machon, Martin, Motard, Grandcolas and Pavoine. This is an open-access article distributed under the terms of the Creative Commons Attribution License (CC BY). The use, distribution or reproduction in other forums is permitted, provided the original author(s) and the copyright owner(s) are credited and that the original publication in this journal is cited, in accordance with accepted academic practice. No use, distribution or reproduction is permitted which does not comply with these terms.
*Correspondence: Anna Kondratyeva, anna.kondratyeva@edu.mnhn.fr; Sandrine Pavoine, sandrine.pavoine@mnhn.fr