Seismostratigraphy of the Ceará Plateau: Clues to Decipher the Cenozoic Evolution of Brazilian Equatorial Margin
- 1Instituto Oceanográfico, Universidade de São Paulo, Praça do Oceanográfico, São Paulo, Brazil
- 2Geology and Petroleum Geology, School of Geosciences, University of Aberdeen, Aberdeen, UK
- 3Department of Earth Sciences, Royal Holloway University of London, Egham, UK
- 4Dipartimento di Scienze della Terra, Università degli Studi di Firenze, Firenze, Italy
- 5Department of Geology, Federal University of Rio Grande do Norte, Natal, Brazil
- 6Departamento de Geologia Regional e Geotectônica - Faculdade de Geologia, Universidade do Estado do Rio de Janeiro, Rio de Janeiro, Brazil
- 7Instituto de Astronomia, Geofísica e Ciências Atmosféricas, Universidade de São Paulo, São Paulo, Brazil
The Ceará Plateau offshore Fortaleza holds some particular characteristics when compared to the other seamounts of the Brazilian Equatorial Margin (BEM). Not only it is the largest and the closest to the continent, it is also located at the boundary between the continental and the oceanic crusts, while all the others seamounts along the BEM are located on oceanic crust. For this reasons, the Ceará Plateau represents a key area to study the stratigraphy of the region throughout proximal to deep ocean facies in relation to sea-level and oceanographic variations. Seismic interpretation is performed providing important stratigraphic features of the sedimentary pattern of the Ceará and Potiguar basins. Moreover, seismic imaging of the Ceará Plateau shows a “disorganized” interior, probably of volcanic origin, overlain by a series of horizontal seismic reflectors that can be interpreted as pelagic/hemipelagic sediments. As large uncertainties exist about the age of the initial formation of this seamount, three scenarios must be considered. If the age of the volcanic edifice is Coniacian (1), then the overlying pelagic/hemipelagic sedimentary succession can include an almost continuous record of the last ~90 Ma at the Equatorial Atlantic Ocean. In the case that the volcanic edifice is Eocene in age (2), the sedimentary sequence would still encompass the upper Paleogene and all the Neogene. There is also the possibility that the volcanic edifice was built during multiple magmatic events (3). In this case, it is likely that the sediments are interfingered with volcanic rocks at the edge of the structure. Although the age estimation (between Coniacian and Eocene) has an uncertainty of more than 40 Myr, the current interpretation is that it developed initially as a volcanic edifice, formed by a series of magmatic events that occurred between the Santonian and the Eocene. Since then, the topography has been leveled by pelagic/hemipelagic sedimentation. Whichever was the initial age, a continuous and constant sequence of sediments deposited onto the Ceará Plateau, at the same latitude, and thus under the same oceanographic conditions, for the last several tens of million years. This would candidate the Ceará Plateau as a suitable opportunity to record a long-term history of the Atlantic Equatorial Margin.
Introduction
Seamounts represent a fascinating feature of the ocean seafloor because they retain important elements to reconstruct the ocean evolution. While most of them have a volcanic origin and are capped by condensed sedimentary sequences, some have a continuous and thick sediment cover (Bader et al., 1970). Because their topography spans over a broad bathymetric range, seamounts have the potential to record a variety of changes in ecological, oceanographic, and sedimentary conditions (Genin et al., 1986).
In this study we present a seismo-stratigraphic reconstruction of the sedimentary sequence of the Ceará Plateau, which is a seamount that raises offshore of the Brazilian Equatorial Margin (BEM). The Ceará Plateau is located between the Ceará and Potiguar basins, some of the deep-water basins offshore the BEM that formed in the Cretaceous. Since the breakup of the northern part of the South Atlantic Ocean, the BEM:
(1) occupies a stable low-latitudinal setting,
(2) is characterized by oceanographic conditions that have not changed significantly (Wagner and Pletsch, 1999; Müller et al., 2008; Torsvik et al., 2009; Heine et al., 2013).
The Ceará Plateau, in particular, crosses different bathymetric regions and can preserve a vertical record of distinctive environments. Here there is the potential to retrieve a high-resolution, multi-record, and continuous sedimentary archive considering that the Plateau:
(1) has been undergoing steady-state subsidence for accommodating an amount of sediments encompassing about 70 Myr,
(2) has maintained a location proximal to the continent, able to guarantee a supply of both pelagic and terrigenous sediments,
(3) was not involved in major tectonic events, which might have affected sediment burial diagenesis and changed its latitude.
Although the BEM has a large number of commercial seismic lines and borehole data, detailed stratigraphic analyses are lacking, moreover paleoceanographic and tectonic/volcanic studies have been limited. This paper will present, for the first time, data supporting the identification of the main horizons and stratigraphic units on the Ceará Plateau, giving insight into the geological evolution of this portion of the BEM through the Cenozoic. This represents a framework necessary for characterizing the tectonic and paleoceanographic history of this region, and which would represent a suitable site for future International Ocean Discovery Program (IODP) drilling expeditions.
Geological Setting
The BEM is characterized by a number of seamounts of different origin. Some of them are linked to the interaction between the margin and tectonic lineaments such as the Romanche fracture zone, while others, observed from the Pará-Maranhão to Potiguar basins, are related to magmatic origin. In Ceará and Potiguar basins magmatic rocks were already sampled revealing different composition and ages, which vary from Coniatian to Pleistocene, with activity peaks during the Santonian and Eocene (Mizusaki et al., 2002; Thomaz Filho et al., 2008; Perlingeiro et al., 2013).
The Ceará Plateau is a flat top seamount that raises in front of the continental shelf of the BEM, offshore the city of Fortaleza (Brazil). This is the largest seamount in the entire BEM, with a surface area of 450 km2 (Fainstein and Milliman, 1979), in water depth of about 400 m. The Ceará Plateau is located between the Potiguar and Ceará basins that are part of the five offshore sedimentary basins bordering the BEM, which include, from NW to SE (Figure 1): Foz do Amazonas, Pará-Maranhão, Barreirinhas, Ceará, and Potiguar, which are roughly limited by the Atlantic Equatorial fracture zones. These basins started their development during the Early Cretaceous, as a series of several continental rift basins through a complex evolution with tectonic regime varying from predominantly normal (distension) to predominantly strike-slip (transtension and transpression) regime. This complexity is due to the overall transform character of Gondwana breakup in this portion of the ancient megacontinent which conditioned the segmentation of the breaked up margin in parts with dominance of normal rifting (distentional tectonic regime) separated by zones with dominance of strike slip rifting (transtension and transpression tectonic regime). These zones nucleated the future oceanic fracture zones of the Equatorial Atlantic Ocean. The BEM is therefore a transform margin, however, the post-breakup tectono-sedimentary evolution has been interpreted as behaving like a normal rifted margin such as the East Brazilian cost, with the main subsidence mechanisms driven by thermal and isostatic subsidence. Nevertheless, it has not ruled out the role of the fracture zones in the tectonic reworking of the drift sedimentary section over the Upper Cretaceous and Cenozoic until the present (Françolin and Szatmari, 1987; Marinho and Mascle, 1987; Szatmari et al., 1987; Zanotto and Szatmari, 1987; Matos, 1999, 2000; Milani and Thomaz Filho, 2000; Mohriak, 2003; Moulin et al., 2010).
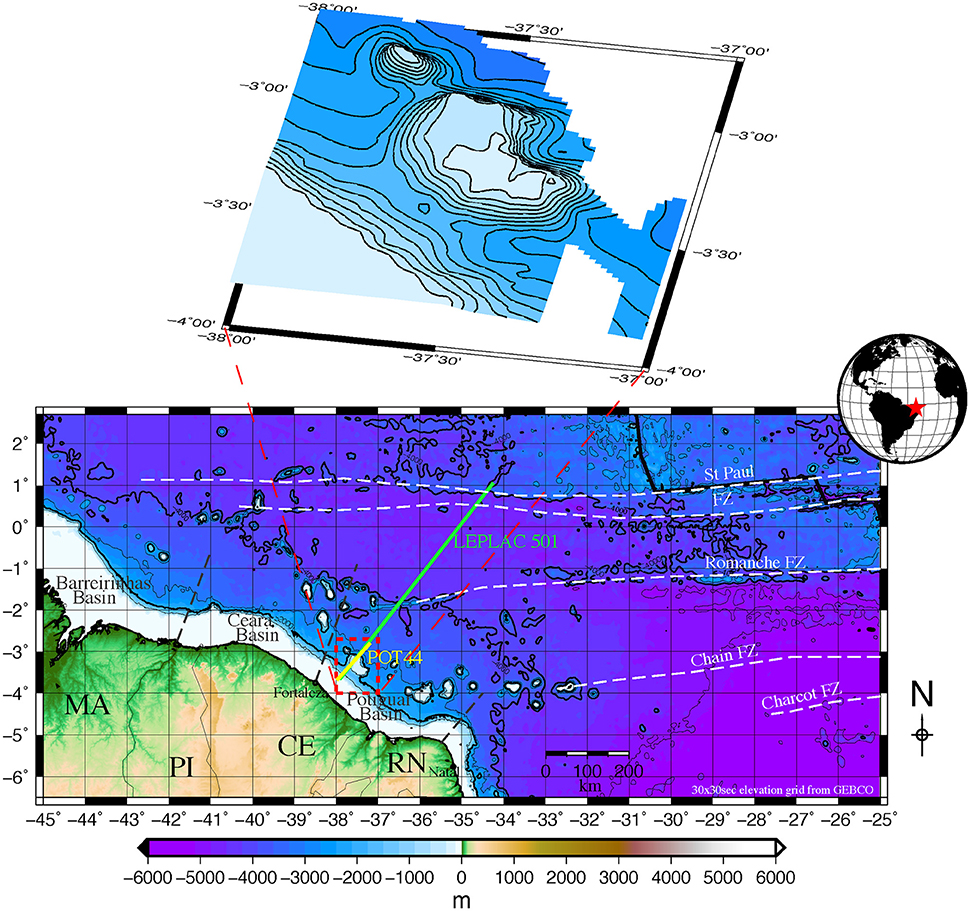
Figure 1. Map of the Brazilian Equatorial Margin and bathymetric map of the Ceará Plateau (Bathymetric grid: GEBCO_2014. Available at: https://www.bodc.ac.uk/data/online_delivery/gebco/). Tectonic fracture Zones based on Müller and Roest (1992) and Basile et al. (2005).
In Ceará and Potiguar Basins, the syn-rift sedimentary deposits of assumed pre-Aptian and early Aptian ages are fresh water continental and lacustrine (Pletsch et al., 2001; Condé et al., 2007; Pessoa Neto et al., 2007). The first marine incursion on continental crust in those basins was registered during pre-breakup time, in the late Aptian. This is confirmed by the presence of more than 100 m of salt, late Aptian in age, related to shallow marine facies drilled in the present day shallow water of Ceará basin (Condé et al., 2007).
The BEM breakup is diachronous among the basins. Those located to the south of the Romanche fracture zone (Ceará and Potiguar) breaked up at the end of the Aptian and those located to the north of the referred zone breaked up at the end of the Albian. The Romanche fracture zone acted for some million years as an accommodation zone for the strike slip tectonic stress at the Equatorial Margin breakup (Matos, 1999, 2000; Condé et al., 2007; Figueiredo et al., 2007; Pessoa Neto et al., 2007; Soares et al., 2007; Trosdtorf et al., 2007; Moulin et al., 2010).
The final opening of the Equatorial Atlantic Ocean marked a dramatic change in water mass distribution and circulation, with profound effects on climate and ecosystems (Burke, 1975; Azevedo, 2004). The conditions transitioned toward more persistent bottom oxygenation from the Late Cretaceous through the Paleogene, with deep-water connection between the North and the South Atlantic fully established in the Campanian-Maastrichtian (Friedrich and Erbacher, 2006; Sexton and Wilson, 2009).
Stratigraphy
The BEM is a stable latitude passive margin (Leyden, 1976; Milani and Thomaz Filho, 2000; Zalán, 2001; Basile et al., 2005; McHone, 2006). The post-rift sedimentary succession in shallow water settings is characterized by a series of depositional sequences separated by regional unconformities of the order of 2–3 million years (Rabinowitz and LaBrecque, 1979; Dias, 1991; Souza-Lima and Hamsi, 2003; Condé et al., 2007). In deep water settings the deposition is more continuous and erosion is localized (Dias, 1991; Condé et al., 2007; Pessoa Neto et al., 2007). Some of the erosional events might be interpreted as related to sequence boundaries and, consequently, to erosion phases due to intensified bottom currents (Condé et al., 2007; Pessoa Neto et al., 2007).
The Ceará Plateau is located at the boundary between the Ceará and the Potiguar basins (Figure 1). Its sedimentary succession includes the Ubarana Formation (Fm), which comprises all the post-rift deep-water successions, from the Lower Cretaceous (Albian) to the Present (Figure 2; Dias, 1991; Beltrami et al., 1994; Condé et al., 2007; Pessoa Neto et al., 2007). The Ubarana Fm consists of two members: the Uruburetama Member (Albian-Campanian), and the Itapagé Member (Campanian-Present). The Uruburetama Member represents a long-term transgressive cycle resulting from the high subsidence rate of the continental margin over the first tens of million years after the breakup. This long-term transgressive cycle is punctuated by higher order regressive events, which individualize at least 6 (six) third or second order depositional sequences (Condé et al., 2007; Pessoa Neto et al., 2007). The Itapagé Member represents a long-term regressive cycle following the overall shelf progradation into the basin, due to the decrease in subsidence rate since the Campanian (Condé et al., 2007). This long-term regressive cycle is punctuated by minor transgressive events, which includes at least 5 (five) third or second order depositional sequences (Condé et al., 2007; Pessoa Neto et al., 2007). The most important discontinuities of the Cenozoic occurred at: (1) the middle-upper Miocene boundary (the Tortonian unconformity); (2) the Rupelian-Chattian boundary (the Chattian unconformity); and (3) the Ypresian-Lutetian boundary (Figure 2). During the Upper Cretaceous the most important discontinuities occurred during: (1) the Maastrichtian (Intra-Maastrichtian unconformity); (2) the Maastrichtian-Campanian boundary (base Maastrichtian unconformity); (3) the Campanian (Mid-Campanian unconformity—MCU); (4) the Turonian (Intra-Turonian unconformity); (5) the Cenomanian (Intra-Cenomanian unconformity); (5) the Cenomanian-Albian boundary (Albo-Cenomanian unconformity; Figure 2). These unconformities are associated to the main sequence boundaries identified in the basin from the previous studies. The geological record in deep water is composed of hemipelagic/pelagic fine-grained sediments, with localized gravity flows carrying coarse-grained sediments (Zalán, 2001; Condé et al., 2007; Pessoa Neto et al., 2007).
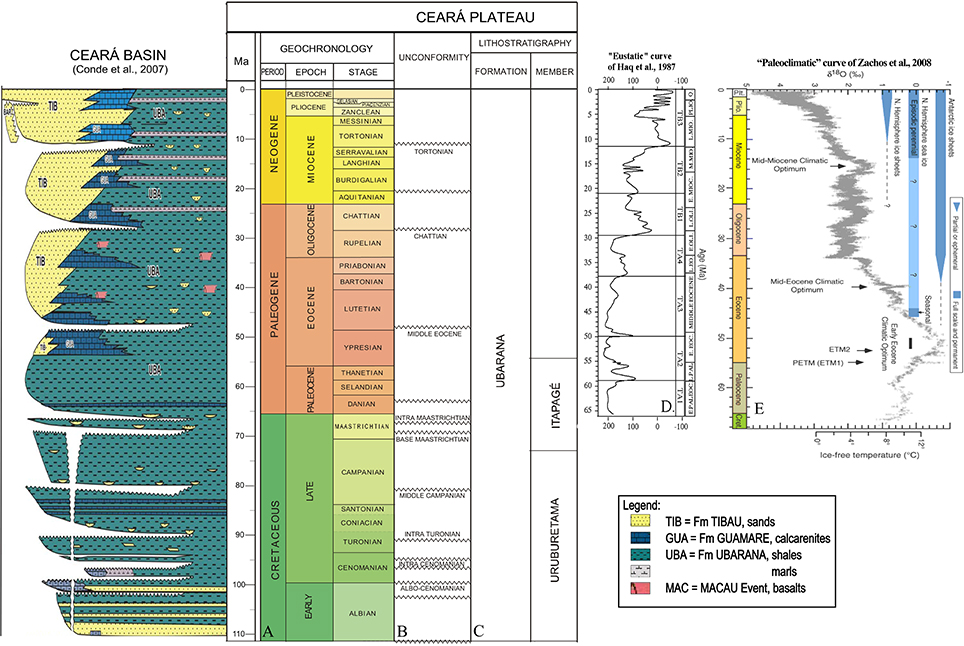
Figure 2. Stratigraphic scheme of Ceará basin (Condé et al., 2007) together with (A) Geochronology, (B) discordances in the Ceará Plateau and (C) lithostratigraphy (formation and members) following Condé et al. (2007) and Pessoa Neto et al. (2007), (D) Eustatic curve of Haq et al. (1987), and (E) Paleoclimatic curve of Zachos et al. (2008). The Macau Event seems to occur in mainly 5 phases: 48.9 ± 2.9, 31.4 ± 1.5, and 14.7 ± 0.9 (Pessoa Neto et al., 2007).
Magmatic Intrusive Events
Dozens of seamounts are present offshore the BEM. Most of them are clearly located on oceanic crust, therefore they are clearly dated as younger than the continental breakup. Potential field data suggest that the seamounts are volcanic cored (Leyden, 1976; Sá et al., 1993; Ussami et al., 1993). Only a few volcanic rocks are dated from shallow water (continental crust) offshore the Ceará and Potiguar basins, and they reveal ages spanning from 83 to 28 Ma (Santonian to Lower Oligocene; Mizusaki et al., 2002; McHone, 2006). Onshore the Potiguar basin, magmatism spans from about 89 to 14 Ma. In the Fernando de Noronha Archipelago the ages of magmatic rocks range from about 12.5 to 1.3 Ma (Mizusaki et al., 2002; Perlingeiro et al., 2013). This post-breakup—Coniacian to Pleistocene—volcanism is known in the literature as “Macau volcanism” (Mizusaki et al., 2002; Thomaz Filho et al., 2008; Perlingeiro et al., 2013). So far, however, there is no direct sampling and dating of any offshore seamount from the BEM, except for Fernando de Noronha Archipelago. Therefore, there is no clear assessment of the relationship between sediments and volcanic rocks in the seamounts, and the current age estimate for the latest volcanic emplacement ranges between the Coniacian and the Eocene, thus has an uncertainty of more than 40 Myr.
Dataset and Methodology
The dataset used for this interpretation consists of post-stack 2D seismic lines of the Plano de Levantamento da Plataforma Continental Brasileira (LEPLAC) III. They have been acquired originally in 1989 by the Brazilian Navy Diretoria de Hidrografia e Navegação (DHN) and by the drilling log named 1-CES-112, and were previously used by Condé et al. (2007) to reconstruct the stratigraphy of the area. The data have been acquired with an air gun source (7 m depth), using a group interval offset of 25 m and a streamer of 2.5 km length, which retained a range of frequencies between 8 and 90 Hz. The seismic lines have been reprocessed using a classic processing stream including: editing and de-multiplexing, geometric spreading correction, spike deconvolution (removing source signature), CDP grouping and determination of stacking velocity, pre-stacking migration and stacking, post-stack deconvolution, migration and band filtering and equalization. The processing was mostly oriented on the recognition of the regional features, although it did not successfully eliminate some of the deep large scale multiple. The drilling logs 1-CES-112, used by Condé et al. (2007) for the stratigraphy of the region (Figure 2), and other lines have been provided by the Agencia Nacional do Petróleo, Gás Natural e Biocombustíveis (ANP) after being collected by Petrobras.
The seismic picking interpretation was performed by several users, which used different software (Petrel®, IHS Kingdom®, GeoSuite® and OpenDetect®), and included the use of some basic image processing. The data set was interpreted in two way time (twt) and the stratigraphy calibrated with data of two wells: 1-CES-112 and MAS-35 (Figure 3; see next paragraph for an extensive description). The interpretation of the seismic data resulted in 6 horizons or reflectors mapped throughout both line LEPLAC 501 (Figure 3) and line POT44 (Figure 4), using the stratigraphic picks of the well 1-CES-112. The interpretation of the data was conducted with basic principles of seismic reflectors identification based on amplitude, phase, and continuity. Once the mapped seismic horizons were tied to the well, they were identified according to the depth along the seismic data crossing with the well position. The image processing applied to the stacked seismic lines included the use of Root Mean Square (RMS) amplitude attribute (equivalent to the square root of the average of the squared values of the waveform) (Chopra and Marfurt, 2007). A gray scale and an opacity color scale (Figure 4) have been applied to enhance eventual impedance contrasts related to the basement units and the post Cretaceous cover (Figures 3, 4). During the interpretation, in areas with absence of well data and interruption of reflectors continuity, a comparison of the seismic attributes has been applied to recognize repeated equivalent lateral units or potential seismic facies (Figures 3–6).
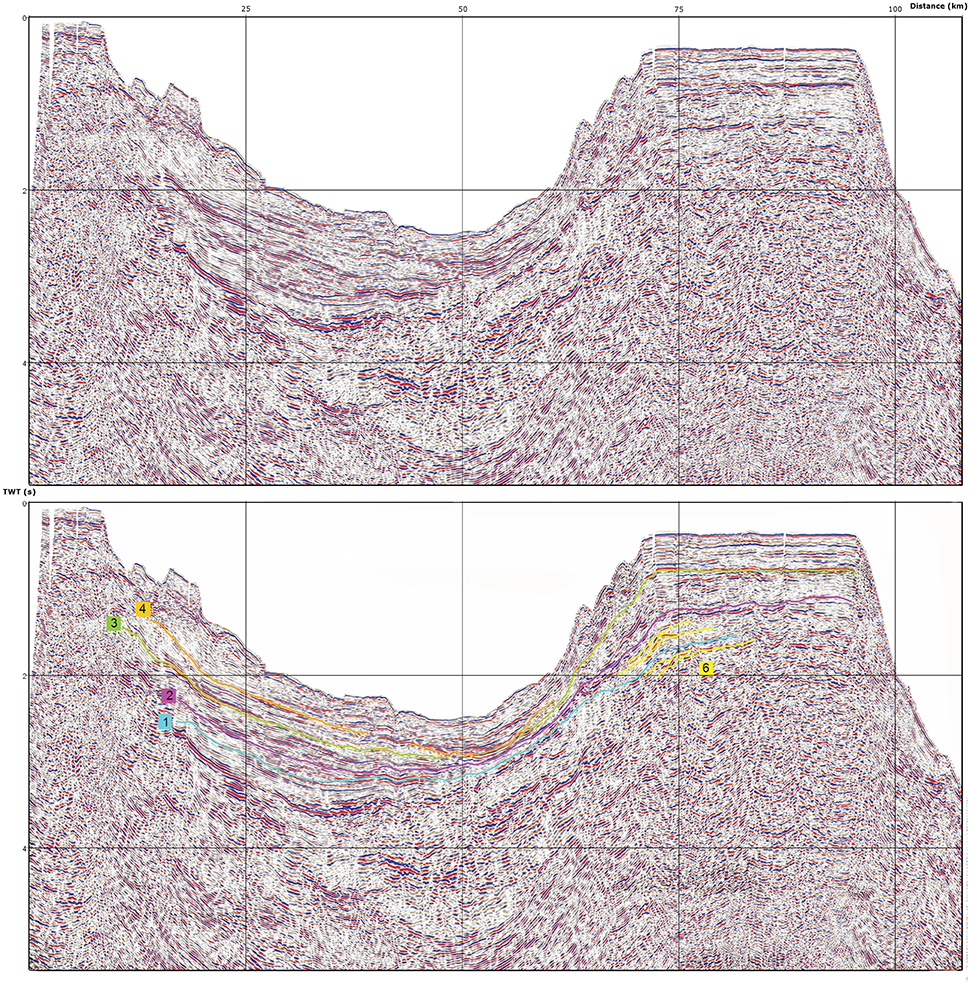
Figure 3. Seismic profile of the seismic line LEPLAC 501 across the Ceará Plateau showing seismic sequences horizons H1 (Cretaceous-Paleogene boundary in light-blue), H2 (Middle Eocene in pink), H3 (Oligocene or lower Miocene in green), and H4 (Tortonian unconformity in orange) and the reflector R6 (Continentalward prograding wedges in yellow).
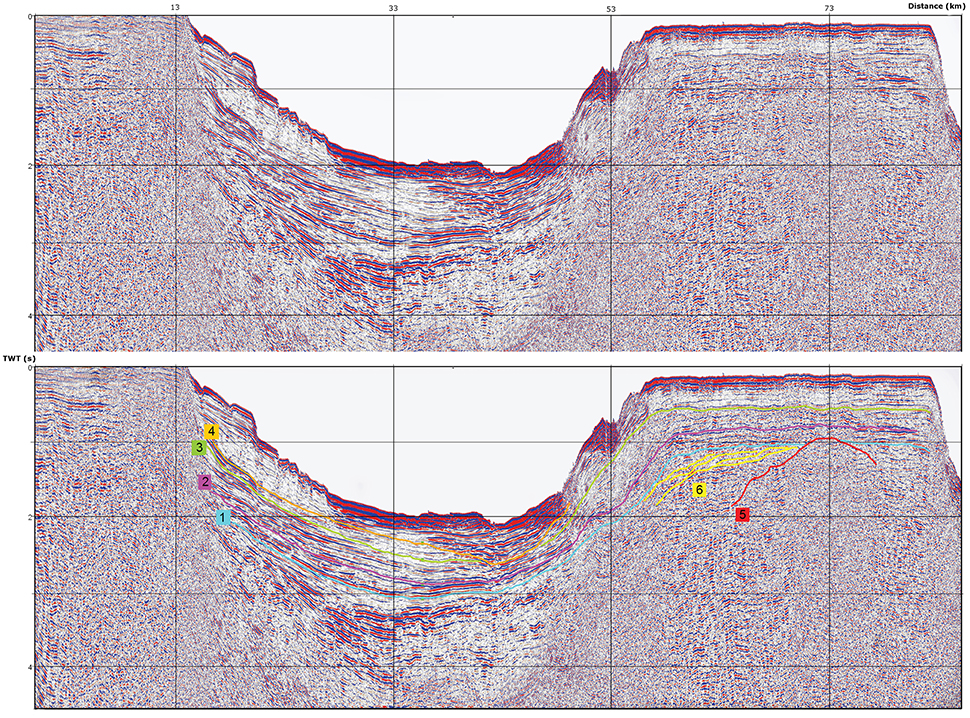
Figure 4. Seismic profile with AGC applied of the seismic line POT44 across the Ceará Plateau showing seismic sequences horizons H1 (Cretaceous-Paleogene boundary in light-blue), H2 (Middle Eocene in pink), H3 (Oligocene or lower Miocene in green), and H4 (Tortonian unconformity in orange); and the reflectors R5 (acoustic basement interpreted as volcanic emplacement in red) and R6 (Continentalward prograding wedges in yellow).
The main horizons have been tied and recognized through the (a) use of synthetic data analysis obtained from the well gamma ray and P-wave velocity log information of the 1-CES-112, (b) the cross cutting relationships and investigation of seismic facies of the main horizons. Chronology information from the well tops data helped to assign ages to the horizons and reflectors that were traced and mapped (Figures 3–6).
Results
The study and interpretation of the seismic data revealed 6 horizons and/or reflectors mapped throughout both LEPLAC 501 and POT44 lines (Figures 3–6). Based on the sequences recognized in the 1-CES-112 well (Figure 2), we can assign the following ages to the horizons:
• Horizon 1: Cretaceous-Paleogene boundary
• Horizon 2: Middle Eocene
• Horizon 3: Oligocene or Lower Miocene
• Horizon 4: Tortonian unconformity
While, based on seismostratigraphic observations, we recognized also some reflectors, of which the age is attributed speculatively:
• Reflector 5: Coniacian Volcanic intrusive emplacement
• Reflector 6: Upper Cretaceous Continental prograding wedges
The four horizons mapped on the seismic data have moderate to strong reflectivity, namely difference between acoustic impedance (Figures 3–6). Horizon 1 (H1, light-blue) is related to the Cretaceous-Paleogene boundary. It has higher amplitudes in the southwest, but it is still well traceable to the north, toward the Ceará Plateau. Horizon 2 (H2, pink) was dated to the middle Eocene and, as H1, can be traced from the southwest to the entire extension of the Plateau. Despite its slightly lower amplitudes, when compared to H1, H2 it shows a better continuity, which allowed continuous mapping. Horizon 3 (H3, green) was mapped above H2 and it was related to the Oligocene or Lower Miocene. As H2, H3 also could be traced from the southwest part of the line up to the Ceará Plateau. A major discontinuity affecting H3 was observed at around 50 km from the continental shelf (SW) (Figures 3, 5). H3 seems to terminate in onlap against a sequence boundary, which is Horizon 4 in this region (H4, orange). Here, the erosive event responsible for the Tortonian unconformity of Horizon 4 (H4, orange) has possibly eroded H3.
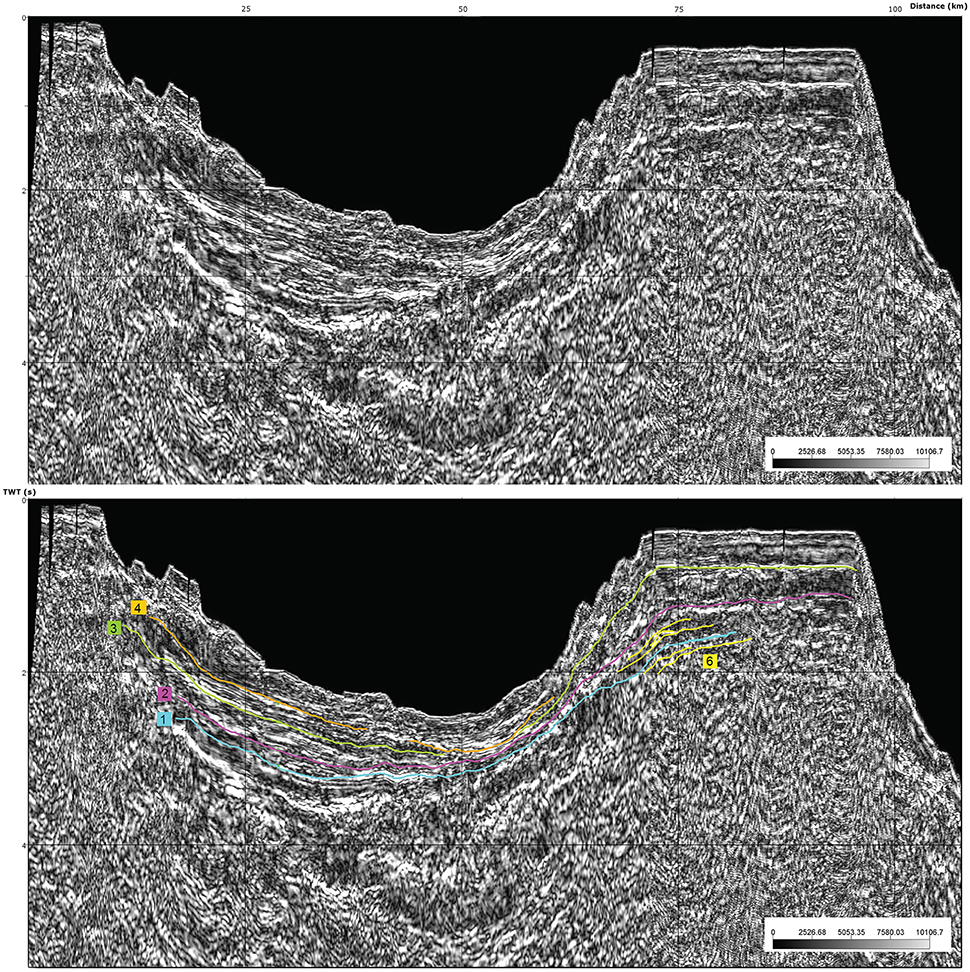
Figure 5. RMS profile of the seismic line LEPLAC 501 across the Ceará Plateau showing seismic sequences horizons H1 (Cretaceous-Paleogene boundary in light-blue), H2 (Middle Eocene in pink), H3 (Oligocene or lower Miocene in green), and H4 (Tortonian unconformity in orange) and the reflector R6 (Continentalward prograding wedges in yellow).
The mean sediment accumulation rates calculated between the sea bottom surface and the horizons H4, between H4 and H3, between H3 and H2, and between H2 and H1 are of ~40, ~15, ~12, and ~4 m/Myr. The Cenozoic should be represented in a maximum thickness of ~1400 (~1 s twt) for the horizon H1 (K/Pg boundary). Mean sediment accumulation rate increases sensibly upward in relation to (1) increase of sediment input from the continent through the geological time, in relation to paleoceanographic and paleoclimatic changes, and/or (2) burial compaction of the fine hemipelagic/pelagic deposits.
Volcanic events are instead responsible for the other two reflectors traced on the Ceará Plateau. On Figures 4, 6 the Reflector 5 (R5, red) was traced from 2 s twt up to around 0.9 s twt, cutting through H4. To the southwest of that emplacement, a series of package units identified by the reflectors 6 (R6, yellow) were mapped and interpreted as related to the volcanic edifice. The shape of R6 reflectors can be explained as a set of wedges prograding toward the continental margin. If this is the case then the progradation was probably induced by a rapid growth of the edifice controlled either by the volcanic eruptions or by coral reef development. The presence of the prograding wedges would also suggest that the volcanic edifice is not entirely controlled by an intrusive process.
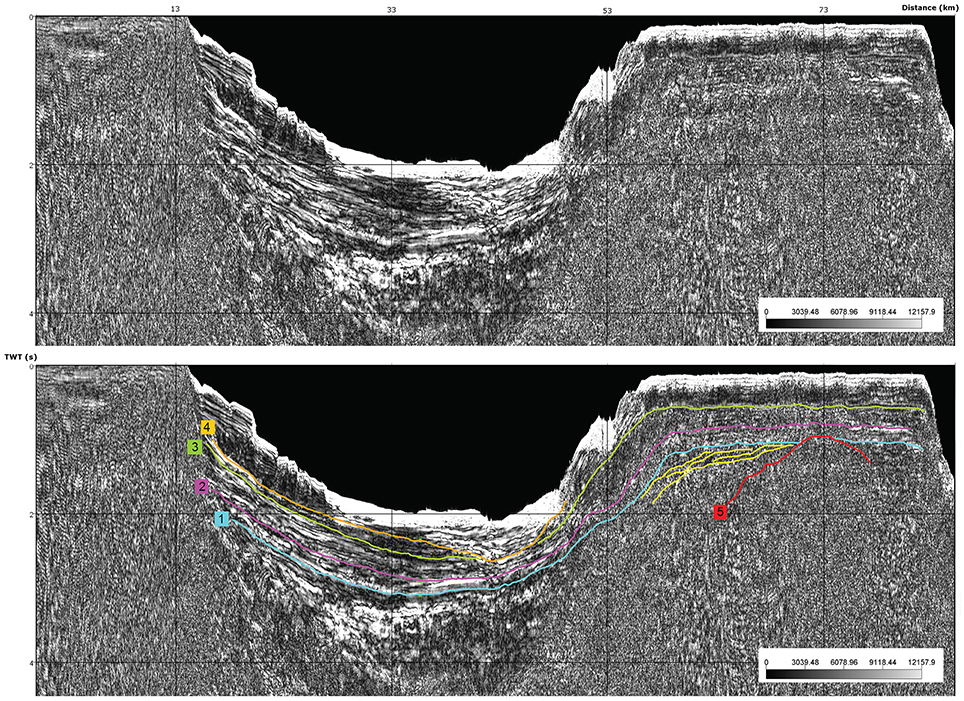
Figure 6. RMS profile with AGC applied of the seismic line POT44 across the Ceará Plateau showing seismic sequences horizons H1 (Cretaceous-Paleogene boundary in light-blue), H2 (Middle Eocene in pink), H3 (Oligocene or lower Miocene in green), and H4 (Tortonian unconformity in orange); and the reflectors R5 (acoustic basement interpreted as volcanic emplacement in red) and R6 (Continentalward prograding wedges in yellow).
Despite being characterized by a low Signal/Noise (S/N) response, the seismic imaging of the Ceará Plateau shows a seismically disorganized internal architecture, overlain by a series of horizontal seismic reflectors that can be interpreted as pelagic/hemipelagic sediments. If the age of the volcanic edifice is Coniacian (scenario 1) (Condé et al., 2007; Pessoa Neto et al., 2007), then the overlying pelagic/hemipelagic sedimentary succession can be formed by an almost continuous record of the last 80 Ma deposited at the Equatorial Atlantic Ocean. If the volcanic edifice is younger (Eocene) (scenario 2), then the sedimentary sequence encompasses the upper Paleogene and all the Neogene. Moreover, we cannot rule out the possibility that the volcanic edifice is the result of multiple magmatic intrusive events (scenario 3).
Due to thermal subsidence and sedimentary load, after the initial breakup, the entire continental margin has been subjected to a continuous subsidence (Dias, 1991; Milani and Thomaz Filho, 2000). The drowning margin is characterized by different subsidence rates between basins (Dias, 1991; Zalán, 2001; Mohriak, 2003). However, it is possible that the volcanic edifice was subaerial during its active phase, but then, as suggested by the presence of a sedimentary succession leveling the original volcanic topography, it became subaqueous at certain point due to the prograding features. The present day morphology of the Plateau suggests that the magmatic activity ceased for several million years. During the inactive magmatic phase, sedimentary processes have been dominating as demonstrated by a thick sequence of plano-parallel reflectors. This includes aggradation of pelagic/hemipelagic beds and the possible development of coral reef platforms, which resulted in horizontal propagation. After that, the surface of the Plateau has been subjected to constant deepening as showed by the constant and well-developed sequence of reflectors and the fact that nowadays the whole structure is 400 m below sea level.
From the seismic dataset there is no evidence of drifted terrigenous materials from the continent. Terrigenous sediments are, instead, observed and recognized on the continental shelf and in the proximal part of slope, shifted further down to the lower slope by gravity mass transport (Figures 3–6).
Discussion
The seismic interpretation of the LEPLAC 501 and POT44 (Figures 3–6) lines performed and proposed in this paper highlights the presence of a sedimentary succession in a relatively deep (hundreds of meters) marine environment covering the volcanic edifice. This structure is surrounded by prograding wedges, which represent the possible development of coral reefs or volcanoclastic platforms at the edge of the rise in some stages, as suggested by the structures observed in the seismic lines (Figures 4, 6). The current age estimation for latest volcanic emplacement ranges between the Coniacian and Eocene, with an uncertainty of more than 40 Myr (Dias, 1991; Condé et al., 2007; Pessoa Neto et al., 2007). The units onlapping and draping above the intrusive volcanic units indicates that even if the volcanic edifice was subaerial during its active phase the magmatism in this region has been inactive for a long time, from the Paleogene to present (Figure 3). During the inactive magmatic phase, sedimentary processes and their related deposits have controlled the construction of the rise. These include aggradation of pelagic/hemipelagic beds, which resulted in a horizontal elevation increase (Figures 3–6). Using the reprocessed seismo-stratigraphic dataset of LEPLAC 501 (Figures 3, 5), we recognize a stratigraphic sequence characterized by several reflectors. The Tortonian and Chattian unconformities are the most remarkable Cenozoic unconformities in the entire Brazilian continental margin (Condé et al., 2007). The whole continental shelf, in fact, was exposed above sea-level during those times (Condé et al., 2007; Figueiredo et al., 2007; Pessoa Neto et al., 2007; Soares et al., 2007, 2008; Trosdtorf et al., 2007). Nevertheless, in line POT44 (Figures 4, 6), we identified some features that resemble a quite entrenched channel complex, proximal to the Ceará Plateau. This would be compatible with a long-term sea-level drop during the Tortonian. If the shelf break was exposed during that time and the rise was quite shallow, the marine currents should have been much stronger than today because of funneling in the narrow corridor between the continental shelf and the rise (Soares and Castro Filho, 1996; Silva et al., 2005). These stronger currents would have been able to carve an entrenched erosional channel parallel to the coast during the Tortonian.
Despite the apparent continuity of the succession we cannot exclude that abrupt sea-level changes might have exposed temporally the rise and create some hiatuses. After the last Tortonian transgression the drowning of the coral reef/volcanoclastic platforms, constantly covered by hemipelagic sediments, which could have leveled the topography maintaining the upper surface horizontal. Therefore, the surface of the rise has been subjected to constant deepening, with no direct influence of drifted terrigenous materials from the continent. Thus, the terrigenous sediments deposited on the continental shelf and in the proximal part of slope have been transported to the lower slope by gravity. Based on the seismic response of the studied lines, we infer that the materials above the rise are mostly hemipelagic sediments, mainly composed of carbonate produced in the upper water column and by fine detrital material delivered from the continent.
Even taking into account episodic erosion and abrupt sea level changes, the Ceará Plateau at the BEM encompasses a rather continuous Cenozoic and possibly uppermost Cretaceous depositional sequence, overlying the Cretaceous (Coniacian-Santonian) volcanic body. Therefore, this sequence represents a unique archive of global ocean and climate history up to the last 110 Myr, with a very high importance to unravel the evolution of the major paleoclimatic events. This margin would then represents a high-quality multi-record archive for the Cenozoic, due to the almost continuous stratigraphy, which allows the integration of high-resolution records of fossils assemblages distribution, stable isotopes, paleomagnetism, biomarkers, and additional lithologic and seismic attributes. However, detailed and integrated stratigraphic information on both carbonate and terrigenous deposits are lacking. The Plateau has, therefore, the potential to disclose an archive showing the “equatorial perspective” of the major cooling events of the Pliocene, middle Miocene, and Oligocene, which formed of permanent ice sheets at high latitudes at about 3, 14, and 33 Ma (Zachos et al., 2008). Moreover, this location is key to analyze the climate warming events of the Early/Middle Miocene, middle Eocene, and early Eocene/Paleocene hypertermals (Zachos et al., 2008; Coccioni et al., 2012), which can lead to a better understanding of the climatic consequences of today global warming. Ongoing studies characterizing the 3D extension of seismostratigraphical packages, defined by the reflectors and horizons recognized, aims to recognize regional paleoceanographic signatures and global paleoclimatic changes. Several implications between the dataset and the climate evolution can be raised following the scenario 1 (Figures 2, 7). The Horizon 1 is of fundamental importance because it may represent the K/Pg boundary, which marks the end of the Cretaceous with the mass extinction of the dinosaurs and the beginning of the Cenozoic (Alvarez et al., 1980; Renne et al., 2013). Global climate proxies indicate warm conditions from the K/Pg boundary until the early Eocene (Zachos et al., 2001, 2008), followed by a long-term global cooling trend characterizing the Eocene. However, short- (5–100 kyr) and mid-term episodes (>100 kyr) of warming climate such as the hyperthermals (Lourens et al., 2005; Zachos et al., 2010) and the middle Eocene Climatic Optimum (MECO; Bohaty and Zachos, 2003; Jovane et al., 2007; Savian et al., 2014, 2016) occurred throughout the Eocene. Horizon 2 can be correlated to the strong and important middle Eocene reflector along the BEM, which has probably a direct relation to the MECO event. These rapid events stand out in the carbon and oxygen isotope records as excursions of about 1–3% toward lower values, indicating that the rising temperatures were accompanied by 12C enrichment of the oceanic dissolved inorganic carbon reservoir. Moreover, they are coeval with decreasing CaCO3 content in sedimentary records, suggesting enhanced carbonate dissolution in the deep ocean. These hyperthermal events resemble the well-known Paleocene-Eocene Thermal Maximum related to oceanic methane release (PETM—Dickens et al., 1995; Svensen et al., 2004; Zachos et al., 2005; Storey et al., 2007), although it is still unclear whether they had similar triggers. The strongest evidence against a common origin is that hyperthermals seem to occur with an orbitally forced pacing, whereas the PETM is not in phase with changes in the orbital parameters. After the middle Eocene, a progressive climate cooling brought to the Antarctica complete glaciation at the Eocene-Oligocene transition (33.5 Ma), which coincided with major current redistribution and intensification of thermohaline circulation (Jovane et al., 2004, 2009; Savian et al., 2016). Between the Eocene and the late Oligocene atmospheric pCO2 decreased reaching values similar to today (Pagani et al., 2005; Zachos et al., 2008). The end of Oligocene and beginning of Miocene may be represented by the Horizon 3, which is a significant transition during in which deep-waters warmed ~3°C (~20–16 Ma; Wright et al., 1992) and stable conditions existed in Antarctica (Miller et al., 1991; Zachos et al., 2001; Pekar and Christie-Blick, 2008). In this period several hiatuses are also observed in the Southern Ocean deep-sea records and they are interpreted as probably related to Antarctic Circumpolar Current (ACC) intensification (Pekar et al., 2006; Barker et al., 2007; Lyle et al., 2007) and to global ocean circulation changes (Wright and Miller, 1996; Poore et al., 2006). At 14 Ma, after the middle Miocene Climatic Optimum (MMCO, Lear et al., 2000; Zachos et al., 2001; Warny et al., 2009), another glacial stage is recognized in Antarctica (Sugden et al., 1993; Marchant and Denton, 1996). Between the MMCO and the first intensification of the northern Hemisphere glaciation (iNHG = MIS100—Mudelsee and Raymo, 2005; DeConto et al., 2008; Bailey et al., 2012) a long cooling trend is observed, interrupted by the early Pliocene warmth (5.5–3 Ma; Ravelo et al., 2004; Dowsett et al., 2005; Robinson et al., 2008). This cooling trend is punctuated by a major cold event related to the Tortonian sea-level fall (Miller et al., 1998; Westerhold et al., 2005), which is shown by Horizon 4.
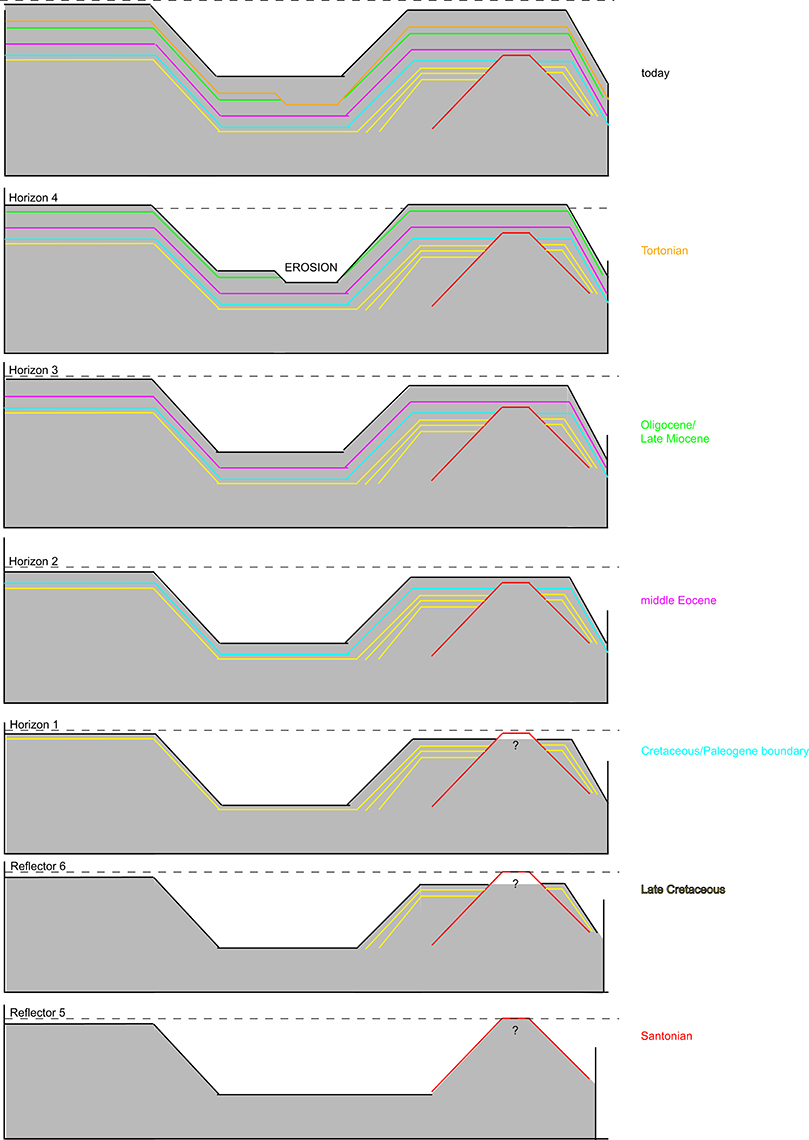
Figure 7. Evolutional scheme of the Ceará Plateau in relation to the Brazilian Equatorial Margin trough time showing seafloor surface as expressed by H1 (Cretaceous-Paleogene boundary), H2 (middle Eocene), H3 (Oligocene or lower Miocene), and H4 (Tortonian unconformity); and the reflectors R5 (acoustic basement interpreted as volcanic emplacement) and R6 (Continentalward prograding wedges).
Conclusions
The Ceará Plateau is a flat top relief at a water depth of about 400 m, which is located next to the continental shelf, at the boundary between the Ceará and Potiguar basins, in front of the city of Fortaleza (CE, Brazil). Deciphering the evolution of this Plateau would allow the understanding of the geological and paleoceanographic history of the BEM. Seismic data is presented here showing that the Ceará Plateau is likely to preserve a volcanic and sedimentary history that started with the breakup between Africa and South America at ~120 Ma. The horizontal and continuous hemipelagic succession (~1s-thick = ~1400 meters) overlying the volcanic body of possible Cretaceous age is, therefore, a primary target to investigate the global paleoceanographic changes occurred at equatorial latitudes from the Cenozoic to present.
Author Contributions
LJ coordinated the research. LJ, JF, DA, DI analyzed and interpreted the data. LJ, JF, DA, DI, MG, and PV wrote the text. DD, FB, HV, IR, and EM provide important insights to develop and finalize the research.
Conflict of Interest Statement
The authors declare that the research was conducted in the absence of any commercial or financial relationships that could be construed as a potential conflict of interest.
Acknowledgments
This research is part of the projects of LJ: Ciencia do Mar II—CAPES, Paleoceanografia da Margem Equatorial Brasileira and of HV: IODP-CAPES—Geohazards e Tectônica—A influência de zonas de fratura na reativação de margens passivas: Margem Equatorial Brasileira. MG and LJ acknowledges the support of the Fundação de Amparo à Pesquisa do Estado de São Paulo (FAPESP process n. 2012/15995-5 and 2011/22018-3, respectively).
References
Alvarez, L. W., Alvarez, W., Asaro, F., and Michel, H. V. (1980). Extraterrestrial cause for the Cretaceous–Tertiary extinction. Science 208, 1095–1108. doi: 10.1126/science.208.4448.1095
Azevedo, R. L. (2004). Paleoceanografia e evolução do Atlântico Sul no Albiano. Bol. Geoc. Petrobras 12, 231–249.
Bader, R. G., Gerard, R. D., Benson, W. E., Bolli, H. M., Hay, W. W., Rothwell, W. T. Jr., et al. (1970). Deep Sea Drilling Project, Initial Reports, Introduction, Volume IV. doi: 10.2973/dsdp.proc.4.101.1970
Bailey, I., Foster, G. L., Wilson, P. A., Jovane, L., Storey, C. D., Becker, J., et al. (2012). Last Glacial-magnitude ice-rafted debris deposition and its provenance in the earliest Pleistocene sub-polar North Atlantic Ocean. Earth Planet. Sci. Lett. 341–344, 222–233. doi: 10.1016/j.epsl.2012.05.034
Barker, P. F., Filippelli, G. M., Florindo, F., Martin, E. E., and Scher, H. D. (2007). Onset and role of the Antarctic Circumpolar Current. Deep Sea Res. II 54, 2388–2398. doi: 10.1016/j.dsr2.2007.07.028
Basile, C., Mascle, J., and Guiraud, R. (2005). Phanerozoic geological evolution of the Equatorial domain. J. Afric. Earth Sci. 43, 275–282. doi: 10.1016/j.jafrearsci.2005.07.011
Beltrami, C. V., Alves, L. E. M., and Feijó, F. J. (1994). Bacia do Ceará. Bol. Geoc. Petrobras 8, 117–125.
Bohaty, S. M., and Zachos, J. C. (2003). Significant Southern Ocean warming event in the late middle Eocene. Geology 31, 1017–1020. doi: 10.1130/G19800.1
Burke, K. (1975). Atlantic evaporates formed by evaporation of water spilled from Pacific, Tethyan, and Southern oceans. Geology 3, 613–616. doi: 10.1130/0091-7613(1975)3<613:AEFBEO>2.0.CO;2
Chopra, S., and Marfurt, K. J. (2007). Seismic Attributes for Prospect Identification and Reservoir Characterization. Tulsa: Society of Exploration Geophysicists.
Coccioni, R., Bancala, G., Catanzariti, R., Fornaciari, E., Frontalini, F., Giusberti, L., et al. (2012). An integrated stratigraphic record of the Paleocene-lower Eocene at Gubbio (Italy): new insights into the early Paleogene hyperthermals and carbon isotope excursions. Terranova 24, 380–386, doi: 10.1111/j.1365-3121.2012.01076.x
Condé, V. C., Lana, C. C., Pessoa Neto, O. C., Roesner, E. H., Morais Neto, J. M., and Dutra, D. C. (2007). Bacia do Ceará. Bol. Geoc. Petrobras 15, 347–355.
DeConto, R. M., Pollard, D., Wilson, P. A., Pälike, H., Lear, C. H., and Pagani, M. (2008). Thresholds for Cenozoic bipolar glaciation. Nature 455, 652–656. doi: 10.1038/nature07337
Dias, J. L. (1991). Análise Estratigráfica e Evolução da Fase Rift nas Bacias das Margens Leste e Sudeste do Brasil. Dissertação de Mestrado, Rio de Janeiro: Universidade Federal do Rio de Janeiro.
Dickens, G. R., Oneil, J. R., Rea, D. K., and Owen, R. M. (1995). Dissociation of oceanic methane hydrate as a cause of the carbon isotope excursion at the end of the Paleocene. Paleoceanography 10, 965–971. doi: 10.1029/95PA02087
Dowsett, H. J., Chandler, M. A., Cronin, T. M., and Dwyer, G. S. (2005). Middle Pliocene sea surface temperature variability. Paleoceanography 20:PA2014. doi: 10.1029/2005PA001133
Fainstein, R., and Milliman, J. D. (1979). Structure of three Continental-Margin Plateaus, Northeastern Brazil. AAPG Bull. 63, 218–238.
Figueiredo, J. J. P., Zalán, P. V., and Soares, E. (2007). Bacia da Foz do Amazonas. Bol. Geoc. Petrobras 15, 299–309.
Françolin, J. B. L., and Szatmari, P. (1987). Mecanismo de rifteamento da porção oriental da margem norte brasileira. Rev. Bras. Geoc. 17, 196–207.
Friedrich, O., and Erbacher, J. (2006). Benthic foraminiferal assemblages from Demerara Rise (ODP Leg 207, western tropical Atlantic): possible evidence for a progressive opening of the Equatorial Atlantic Gateway. Cretaceous Res. 27, 377–397. doi: 10.1016/j.cretres.2005.07.006
Genin, A., Dayton, P. K., Lonsdale, P. F., and Spiess, F. N. (1986). Corals on seamount peaks provide evidence of current acceleration over deep-sea topography. Nature 322, 59–61. doi: 10.1038/322059a0
Haq, B. U., Hardenbol, J., and Vail, P. R. (1987). Chronology of fluctuating sea levels since the Triassic (250 million years ago to present). Science 235, 1156–1167. doi: 10.1126/science.235.4793.1156
Heine, C., Zoethout, J., and Müller, R. D. (2013). Kinematics of the South Atlantic rift. Solid Earth 4, 215–253. doi: 10.5194/se-4-215-2013
Jovane, L., Coccioni, R., Marsili, A., and Acton, G. (2009). “The late Eocene greenhouse-icehouse transition: Observations from the Massignano global stratotype section point (GSSP)”, in The Late Eocene Earth—Hothouse, Icehouse, and Impacts, Vol. 452, eds C. Koeberl and A. Montanari (Boulder, CO: Geological Society of America Special Papers), 149–168. doi: 10.1130/2009.2452(10)
Jovane, L., Florindo, F., and Dinarès-Turell, J. (2004). Environmental magnetic record of paleoclimate change: revisiting the Eocene-Oligocene stratotype section, Massignano, Italy. Geophys. Res. Lett. 31:L15601. doi: 10.1029/2004gl020554
Jovane, L., Florindo, F., Dinarès-Turell, J., Marsili, A., Monechi, S., Roberts, A. P., et al. (2007). Evidences of the middle Eocene Climatic Optimum (MECO) event from the Contessa Highway section, Umbrian Apennines, Italy. Geol. Soc. Am. Bull. 119, 413–427. doi: 10.1130/B25917.1
Lear, C. H., Elderfield, H., and Wilson, P. A. (2000), Cenozoic deep-sea temperatures global ice volumes from Mg/Ca in benthic foraminiferal calcite. Science 287, 269–272. doi: 10.1126/science.287.5451.269
Leyden, R. (1976). Salt distribution and crustal models for the eastern Brazilian margin. An. Acad. Bras. Ciênc. 48, 159–168.
Lourens, L. J., Sluijs, A., Kroon, D., Zachos, J. C., Thomas, E., Röhl, U., et al. (2005). Astronomical pacing of late Palaeocene to early Eocene global warming events. Nature 435, 1083–1087. doi: 10.1038/nature03814
Lyle, M., Gibbs, S., Moore, T. C., and Rea, D. K. (2007). Late Oligocene initiation of the Antartic Circumpolar current: evidence from the South Pacific. Geology 35, 691. doi: 10.1130/G23806A.1
Marchant, D. R., and Denton, G. H. (1996). Miocene and Pliocene paleoclimate of the dry valleys region, Southern Victoria land: a geomorphological approach. Mar. Micropaleontol. 27, 253–271. doi: 10.1016/0377-8398(95)00065-8
Marinho, M., and Mascle, J. (1987). Evolução estrutural do platô marginal da Guiné e sua relação com a formação do Oceano Atlântico Centro-Equatorial. Rev. Bras. Geoc. 17, 111–117.
Matos, R. M. D. (1999). “History of the northeast Brazilian rift system: Kinematic implications for the break-up between Brazil and West Africa,” in The Oil and Gas Habitats of the South Atlantic, Vol. 153. eds N. R. Cameron, R. H. Bate, and V. S. Clure (London: Geological Society London Special Publications), 153, 55–73. doi: 10.1144/GSL.SP.1999.153.01.04
Matos, R. M. D. (2000). “Tectonic evolution of the equatorial South Atlantic,” in Atlantic Rifts and Continental Margins, Vol. 115, eds W. U. Mohriak and M. Talwani (Washington, DC: American Geophysical Union. Geophysical Monograph), 331–354. doi: 10.1029/GM115p0331
McHone, J. G. (2006). Igneous Features and Geodynamic Models of Rifting and Magmatism Around the Central Atlantic Ocean. 2006. Available online at: http://www.mantleplumes.org/CAMP.html (Accessed Auguest 28, 2008).
Milani, E. J., and Thomaz Filho, A. (2000). “Sedimentary basins of South America,” in Tectonic Evolution of South America, Vol. 31, eds U. G. Cordani, E. J. Milani, A. Thomaz Filho, and D. A.Campos (Rio de Janeiro: International Geological Congress), 389–449.
Miller, K. G., Mountain, G. S., Browning, J. V., Kominz, M., Sugarman, P. J., Christie-Blick, N., et al. (1998). Cenozoic global sea level, sequences, and the New Jersey transect: results from coastal plain and continental slope drilling. Rev. Geophys. 36, 569–602. doi: 10.1029/98RG01624
Miller, K. G., Wright, J. D., and Fairbanks, R. G. (1991). Unlocking the ice house: oligocene–Miocene oxygen isotopes, eustasy, and margin erosion. J. Geophys. Res. 96, 6829–6848. doi: 10.1029/90JB02015
Mizusaki, A. M. P., Thomaz Filho, A., Milani, E. J., and Césero, P. (2002). Mesozoic and cenozoic igneous activity and its tectonic control in the northeastern region of Brazil. South America. J. South Am. Earth Sci. 15, 183–198. doi: 10.1016/S0895-9811(02)00014-7
Mohriak, W. U. (2003). “Bacias sedimentares da margem continental Brasileira,” in Geologia, Tectônica e Recursos Minerais do Brasil, eds L. A. Bizzi, C. Schobbenhous, R. M. Vidotti, and J. H. Gonçalves (Brasilia: CPRM), 87–168.
Moulin, M., Aslanian, D., and Unternher, P. (2010). A new starting point for the South and Equatorial Atlantic Ocean. Earth Sci. Rev. 97, 59–95. doi: 10.1016/j.earscirev.2009.08.001
Mudelsee, M., and Raymo, M. E. (2005). Slow dynamics of the Northern Hemisphere glaciation. Paleocenography 20:PA4022. doi: 10.1029/2005pa001153
Müller, R. D., and Roest, W. R. (1992). Fracture zones in the North Atlantic from combined Geosat and Seasat data. J. Geophys. Res. 97, 3337–3350. doi: 10.1029/91JB02605
Müller, R. D., Sdrolias, M., Gaina, C., and Roest, W. R. (2008). Age, spreading rates, and spreading asymmetry of the world's ocean crust. Geochem. Geophys. Geosyst. 9:Q04006. doi: 10.1029/2007gc001743
Pagani, M., Zachos, J. C., Freeman, K. H., Tipple, B., and Bohaty, S. (2005). Marked decline in atmospheric carbon dioxide concentrations during the Paleogene. Science 309, 600–603. doi: 10.1126/science.1110063
Pekar, S. F., and Christie-Blick, N. (2008). Resolving apparent conflicts between oceanographic and Antarctic climate records and evidence for a decrease in pCO2 during the Oligocene through early Miocene (34–16 Ma). Palaeogeogr. Palaeoclimatol. Palaeoecol. 260, 41–49. doi: 10.1016/j.palaeo.2007.08.019
Pekar, S. F., Harwood, D. M., and DeConto, R. (2006). Resolving a late Oligocene conundrum: Deep-sea warming versus Antarctic glaciation. Palaeogeogr. Palaeoclimatol. Palaeoecol. 231, 29–40. doi: 10.1016/j.palaeo.2005.07.024
Perlingeiro, G., Vasconcelos, P. M., Knesel, K. M., Thiede, D. S., and Cordani, U. G. (2013). 40Ar/39Ar geochronology of the Fernando de Noronha Archipelago and implications for the origin of alkaline volcanism in the NE Brazil. J. Volcanol. Geoth. Res. 249, 140–154. doi: 10.1016/j.jvolgeores.2012.08.017
Pessoa Neto, O. C., Soares, U. M., Silva, J. G. F., Roesner, E. H., Florencio, C. P., and Souza, C. A. V. (2007). Bacia Potiguar. Bol. Geoc. Petrobras 15, 357–369.
Pletsch, T., Erbacher, J., Holbourn, A. E. L., Kuhnt, W., Moullade, M., Oboh-Ikuenobede, F. E., et al. (2001). Cretaceous separation of Africa and South America: the view from the West African margin (ODP Leg 159). J. South Am. Earth Sci. 14, 147–174. doi: 10.1016/S0895-9811(01)00020-7
Poore, H. R., Samworth, R., White, N. J., Jones, S. M., and McCave, I. N. (2006). Neogene overflow of Northern component water at the Greenland-Scotland Ridge. Geochem. Geophys. Geosyst. 7:Q06010. doi: 10.1029/2005gc001085
Rabinowitz, P. D., and LaBrecque, J. (1979). The Mesozoic South Atlantic Ocean and evolution of its Continental Margins. J. Geophys. Res. 84, 5973–6002. doi: 10.1029/JB084iB11p05973
Ravelo, A. C., Andreasen, D. H., Lyle, M., Lyle, A. O., and Wara, M. W. (2004). Regional climate shifts caused by gradual global cooling in the Pliocene epoch. Nature 429, 263–267. doi: 10.1038/nature02567
Renne, P. R., Deino, A. L., Hilgen, F. J., Kuiper, K. F., Mark, D. F., Mitchell, W. S., et al. (2013). Time scales of critical events around the Cretaceous-Paleogene boundary. Science 339, 684–687. doi: 10.1126/science.1230492
Robinson, M. M., Dowsett, H. J., Dwyer, G. S., and Lawrence, K. T. (2008). Reevaluation of mid-Pliocene North Atlantic sea surface temperature. Paleoceanography 23:PA3213. doi: 10.1029/2008pa001608
Sá, N. C., Ussami, N., and Molina, E. C. (1993). Gravity map of Brazil. 1: representation of free-air and Bouguer Anomalies. J. Geophys. Res. 98, 2187–2197. doi: 10.1029/92JB00979
Savian, J. F., Jovane, L., Frontalini, F., Trindade, R. I. F., Coccioni, R., Bohaty, S. M., et al. (2014). Enhanced primary productivity and magnetotactic bacterial production in response to middle Eocene warming in the Neo-Tethys Ocean. Palaeogeogr. Palaeoclimatol. Palaeoecol. 414, 32–45. doi: 10.1016/j.palaeo.2014.08.009
Savian, J. F., Jovane, L., Giorgioni, M., Iacoviello, F., Rodelli, D., Roberts, A. P., et al. (2016). Environmental magnetic implications of magnetofossil occurrence during the Middle Eocene Climatic Optimum (MECO) in pelagic sediments from the equatorial Indian Ocean. Palaeogeogr. Palaeoclimatol. Palaeoecol. 441, 212–222. doi: 10.1016/j.palaeo.2015.06.029
Sexton, P. F., and Wilson, P. A. (2009). Preservation of benthic foraminifera and reliability of deep-sea temperature records: importance of sedimentation rates, lithology, and the need to examine test wall structure. Paleoceanography 24:PA2208. doi: 10.1029/2008PA001650
Silva, L. S., Miranda, L. B., and Castro Filho, B. M. (2005). Numerical study of circulation and thermohaline structure in the São Sebastião Channel. Rev. Brasileira de Geofísica 23, 407–425. doi: 10.1590/S0102-261X2005000400005
Soares, E., Zalán, P. V., Figueiredo, J. J. P., and Trosdtorf, I. Jr. (2007). Bacia do Pará-Maranhão. Bol. Geoc. Petrobras 15, 321–329.
Soares, J., and Castro Filho, B. M. (1996). Numerical modeling of the response of Ceará continental shelf waters to wind stress forcing. Revista Brasileira Oceanografia 44, 135–153. doi: 10.1590/s1679-87591996000200004
Soares, A. V. Jr., Costa, J. B. S., and Hasui, Y. (2008). Evolução da Margem Atlântica Equatorial do Brasil: Três Fases Distensivas. Geociências 27, 427–437.
Souza-Lima, W., and Hamsi, G. P. Jr. (2003). Bacias Sedimentares Brasileiras- Bacias da Margem Continental, Vol. 50, Fundação Paleontológica Phoenix, 1–4. Available online at: http://www.phoenix.org.br/Phoenix50_Fev03.html;http://www.mantleplumes.org/CAMP.html (Accessed January 28, 2016).
Storey, M., Duncan, R. A., and Swisher, C. C. (2007). Paleocene–Eocene thermal maximum and the opening of the northeast Atlantic. Science 316, 587–589. doi: 10.1126/science.1135274
Sugden, D. E., Marchant, D. R., and Denton, G. H. (1993). The case for a stable East Antarctic ice sheet: the background. Geogr. Ann. Ser. A Phys. Geogr. 75, 151–154. doi: 10.2307/521199
Svensen, H., Planke, S., Malthe-Sørenssen, A., Jamtveit, B., Myklebust, R., Rasmussen Eidem, T., et al. (2004). Release of methane from a volcanic basin as a mechanism for initial Eocene global warming. Nature 429, 524–527. doi: 10.1038/nature02566
Szatmari, P., Françolin, J. B. L., Zanotto, O., and Wolff, S. (1987). Evolução tectônica da margem equatorial Brasileira. Rev. Bras. Geoc. 17, 180–188.
Thomaz Filho, A., Mizusaki, A. M. P., and Antonioli, L. (2008). Magmatismo nas bacias sedimentares brasileiras e sua influência na geologia do petróleo. Rev. Bras. Geoc. 38, 128–137.
Torsvik, T. H., Rousse, S., Labails, C., and Smethurst, M. A. (2009). South Atlantic reconstructions and the Aptian Salt Enigma. Geophys. J. Int. 177, 1315–1333. doi: 10.1111/j.1365-246X.2009.04137.x
Trosdtorf, I. Jr., Zalán, P. V., Figueiredo, J. J. P., and Soares, E. (2007). Bacia de Barreirinhas. Bol. Geoc. Petrobras 15, 331–339.
Ussami, N., Sá, N. C., and Molina, E. C. (1993). Gravity map of Brazil.2: regional and residual isostatic anomalies and their correlation with major tectonic provinces. J.Geophys. Res. 98, 2199–2208. doi: 10.1029/92JB01398
Wagner, T., and Pletsch, T. (1999). “Tectono-sedimentary controls on Cretaceous black shale deposition along the opening of the Equatorial Atlantic Gateway (ODP Leg 159),” in The Oil and Gas Habitats of the South Atlantic, Vol. 153, eds N. R. Cameron, R. H. Bate, and V. S. Clure (London: Geological Society London Special Publications), 241–265.
Warny, S., Askin, R. A., Hannah, M. J., Mohr, B. A. R., Raine, J. I., Harwood, D. M., et al. (2009). Palynomorphs from a sediment core reveal a sudden remarkably warm Antarctica during the Middle Miocene. Geology 37, 955–958. doi: 10.1130/G30139A.1
Westerhold, T., Bickert, T., and Röhl, U. (2005). Middle to late Miocene oxygen isotope stratigraphy of ODP site 1085 (SE Atlantic): new constrains on Miocene climate variability and sea-level fluctuations. Palaeogeogr. Palaeoclimatol. Palaeoecol. 217, 205–222. doi: 10.1016/j.palaeo.2004.12.001
Wright, J. D., and Miller, K. G. (1996). Control of North Atlantic deep water circulation by the Greenland-Scotland Ridge. Paleoceanography 11, 157–170. doi: 10.1029/95PA03696
Wright, J. D., Miller, K. G., and Fairbanks, R. G. (1992). Early and middle Miocene stable isotopes; implications for deepwater circulation and climate. Paleoceanography 7, 357–389. doi: 10.1029/92PA00760
Zachos, J. C., Dickens, G. R., and Zeebe, R. E. (2008). An early Cenozoic perspective on greenhouse warming and carbon-cycle dynamics. Nature 451, 279–283. doi: 10.1038/nature06588
Zachos, J. C., McCarren, H., Murphy, B., Röhl, U., and Westerhold, T. (2010). Tempo and scale of late Paleocene and early Eocene carbon isotope cycles: implications for the origin of hyperthermals. Earth Planet. Sci. Lett. 299, 242–249. doi: 10.1016/j.epsl.2010.09.004
Zachos, J. C., Röhl, U., Schellenberg, S. A., Sluijs, A., Hodell, D. A., Kelly, D. C., et al. (2005). Rapid acidification of the ocean during the Paleocene–Eocene thermal maximum. Science 308, 1611–1615. doi: 10.1126/science.1109004
Zachos, J., Pagani, M., Sloan, L., Thomas, E., and Billups, K. (2001). Trends, rhythms, and aberrations in global climate 65 Ma to present. Science 292, 686–693. doi: 10.1126/science.1059412
Keywords: ceará plateau, potiguar basin, brazilian equatorial margin, cenozoic, seismostratigraphy
Citation: Jovane L, Figueiredo JJP, Alves DPV, Iacopini D, Giorgioni M, Vannucchi P, Moura DS, Bezerra FHR, Vital H, Rios ILA and Molina EC (2016) Seismostratigraphy of the Ceará Plateau: Clues to Decipher the Cenozoic Evolution of Brazilian Equatorial Margin. Front. Earth Sci. 4:90. doi: 10.3389/feart.2016.00090
Received: 29 April 2016; Accepted: 03 October 2016;
Published: 25 October 2016.
Edited by:
Andrea Billi, National Research Council, ItalyReviewed by:
Andres Folguera, University of Buenos Aires, ArgentinaMarvin Andrew Speece, Montana Tech, USA
Copyright © 2016 Jovane, Figueiredo, Alves, Iacopini, Giorgioni, Vannucchi, Moura, Bezerra, Vital, Rios and Molina. This is an open-access article distributed under the terms of the Creative Commons Attribution License (CC BY). The use, distribution or reproduction in other forums is permitted, provided the original author(s) or licensor are credited and that the original publication in this journal is cited, in accordance with accepted academic practice. No use, distribution or reproduction is permitted which does not comply with these terms.
*Correspondence: Luigi Jovane, jovane@usp.br