Silencing of AJAP1 expression by promoter methylation activates the Wnt/β-catenin signaling pathway to promote tumor proliferation and metastasis in salivary adenoid cystic carcinoma
Highlight box
Key findings
• The results of our study suggest that the downregulation of AJAP1 protein expression may be closely related to the occurrence, development, and prognosis of salivary adenoid cystic carcinoma (SACC), and may play a certain role in progression and metastasis.
What is known and what is new?
• SACC is highly invasive and prone to neurovascular invasion and distant metastasis; however, its specific molecular mechanism remains unclear.
• In this study, we clarified the mechanism through which AJAP1 regulates the progression of SACC both in vitro and in vivo. We also elucidated the causes of AJAP1 downregulation in SACC and revealed its mechanism.
What are the implications, and what should change now?
• We believe that the results of our study will provide new ideas and targets for treating SACC and provide a new direction for the development and research of new drugs against SACC.
Introduction
Salivary adenoid cystic carcinoma (SACC) is a unique malignant tumor of the salivary gland, accounting for approximately 10% of all salivary gland tumors and 30% of salivary gland malignancies (1). It is characterized by being highly invasive and prone to neurovascular aggression and distant metastasis (2). The 5-year survival rate of SACC is approximately 70–90% but only 20% of cases have distant invasion (3). Currently, there is a lack of effective chemotherapy and targeted drugs for patients with SACC, and surgical resection is the main treatment option (4). However, the treatment of SACC is very difficult, largely due to the particularity of the surgical location, namely, the trauma and propensity for complications as well as the high occurrence of local invasion and distant metastasis in patients. Therefore, it is important to explore the molecular mechanism underlying SACC invasion and metastasis to develop novel therapeutic strategies and targets in clinical research.
In recent years, a previous study has shown that the abnormal expression of some genes may play an important role in the malignant progression of SACC (5) and methylation of DNA promoter may be one of the main causes of abnormal gene expression. The aberrantly modified DNA methylation confers unique features on tumor cells, including sustained proliferative potential, resistance to growth-suppressive or cell death signals, augmented replicative immortality, invasion, and metastasis. As a result, DNA methylation abnormalities exhibit significant impacts on all stages of oncogenesis from its onset to progression to metastasis, which may eventually lead to tumor chemotherapy resistance. Thus, DNA methylation/demethylation pathway as a promising target for therapeutic intervention in cancer (6). Studies have shown that hypermethylation in promoters of Reversion-inducing cysteine-rich protein with kazal motifs (RECK), RAS association domain family protein1A gene (RASSF1), Suprabasin promotes the metastasis and progression of SACC, which is associated with poor prognosis of patients (7-9). This suggests that high levels of DNA methylation may play an important role in the occurrence and development of SACC. Adherens Junctions Associated Protein 1 (AJAP1) is a complete membrane protein with 411 amino acid residues; it includes a divisible N-terminal signal peptide (residues 1–43), extracellular domain (residues 44–282), transmembrane domain (residues 283–303), and intracellular cytoplasmic structure (residues 304–411) (10,11). In recent years, AJAP1 has been shown to play a certain role in tumor proliferation, apoptosis, invasion, and metastasis (12-14); however, its role in the promotion of tumor progression remains unclear. In glioblastoma multiforme (GBM), AJAP1 can increase intercellular adhesion, reduce invasion and diversion, and inhibit proliferation and tumorigenicity in vivo, and the downregulation of AJAP1 expression often suggests a poor prognosis (15). In liver cancer, a previous study demonstrated that Micro RNA-552 (miR-552) can promote tumor proliferation, invasion, and metastasis by downregulating AJAP1 expression, indicating a poor prognosis (16). Moreover, Yan et al. observed that low expression levels of both Tet methylcytosine dioxygenase 1 (TET1) and AJAP1 predict poor prognosis in urinary bladder cancer patients, and the frequently downregulated TET1 level reduces the hydroxymethylation of the AJAP1 promoter and subsequently activates β-catenin signaling to promote the development of urinary bladder cancer (17). Nevertheless, whether and how the mechanism of AJAP1 inhibits the progression of SACC is currently unclear.
In this study, we clarified the mechanism through which AJAP1 regulates the progression of SACC both in vitro and in vivo. We also elucidated the causes of AJAP1 loss in SACC and revealed its mechanism. We present this article in accordance with the ARRIVE and MDAR reporting checklists (available at https://gs.amegroups.com/article/view/10.21037/gs-23-127/rc).
Methods
Array data
The Cancer Genome Atlas (TCGA), Gene Expression Profiling Interactive Analysis (GEPIA), GeneCards, and other databases were used to analyze the expression of AJAP1 in various organs of the body and corresponding tumors. Genome-wide sequencing was performed on SACC tissues and paracancerous tissues to screen the differentially expressed genes and construct differentially gene expression tumor profiles. Gene Ontology (GO) and pathway enrichment were performed to analyze differentially expressed genes.
Cell culture and reagents
SACC-LM and SACC-83 were obtained from Peking University and cultured in Roswell Park Memorial Institute-1640 (RPMI-1640) medium supplemented with 10% fetal bovine serum (FBS) at 37 ℃ in a humid incubator (Thermo Fisher Scientific, USA) containing 5% carbon dioxide (CO2). The 5-Azacytidine (5-Aza) and Cell Counting Kit-8 (CCK-8 kit) were obtained from APExBIO (Houston, USA); puromycin and cell lysis buffer for western blot (WB) and IP (immunoprecipitation) were purchased from Beyotime (Shanghai, China); and the Protein Quantification Kit (BCA kit) was purchased from Thermo Fisher.
Immunohistochemistry
The SACC samples were collected by Zhejiang Provincial People’s Hospital, and patients with concomitant malignancies will be excluded. Immunohistochemistry was performed as follows: the samples were fixed with formaldehyde and embedded in paraffin, before being cut into 7-µm-thick sections, dewaxed, and rehydrated to extract the antigen. The sections were then incubated with anti-AJAP1 (1:5,000; Proteintech, USA) overnight. After washing with phosphate-buffered saline (PBS), the sections were incubated with Horseradish Peroxidase (HRP)-conjugated goat anti-rabbit immunoglobulin G (IgG), followed by hematoxylin and eosin (HE) staining. The slides were photographed and analyzed using a fluorescence microscope (Nikon Ni-U, Japan). The study was conducted in accordance with the Declaration of Helsinki (as revised in 2013). The study was approved by Medical Ethics Committee of Zhejiang Provincial People’s Hospital (No. QT2022326) and informed consent was taken from all the patients. A protocol was prepared before the study without registration.
Methylation-specific polymerase chain reaction (MSP)
The DNA of SACC-LM and SACC-83 cells (SACC-LM is a subclone of SACC-83 cells, which are more capable of lung metastasis) was extracted according to the instructions of the DNA extraction kit (Servicebio, Wuhan, China), and its concentration and purity were determined by an Ultraviolet spectrophotometer (NanoDrop2000, Thermo Fisher Scientific, USA). The DNA that met the requirements was stored at −80 ℃ for use. Bisulfite modification was performed according to the instructions in the Methylation-Gold Kit (ZYMO, USA), and methylation-specific PCR (MSP) amplification was performed to analyze the Methylation state. The methylated primer design of AJAP1 was as follows: M-AJAP1-F: GTTAGGTAGTAGTTGCGTTGGGC, M-AJAP1-R: TTCCTAAAACGAAACTAAACCACG. The unmethylated primer design was as follows: U-AJAP1-F: TGGTTAGGTAGTAGTTGTGTTGGGT, U-AJAP1-R: ATTTCCTAAAACAAAACTAAACCACA.
The MSP products were then subjected to agarose gel electrophoresis. The homozygous methylation-positive results were defined as specific products and were mainly from the methylated primer rather than the unmethylated primer. If the unmethylated primer expanded the specific products but the methylated primer had no products, it was judged as being methylation-negative. If both primers had specific products, it was judged as being heterozygous methylation-positive. Both homozygous methylation-positive and heterozygous methylation-positive were considered methylation-positive.
Lentivirus-mediated transfection
SACC-LM and SACC-83 cells were plated in six-well plates, with 105 cells per well. After 24 h, lentiviruses (GENECHEM, Shanghai, China) with AJAP1 overexpression or knockdown were added to SACC-LM or SACC-83, respectively. After 48 h, the expression rate of GFP was examined to evaluate the infection efficiency, and puromycin was used for screening. The expression of AJAP1 was then verified using real-time quantitative polymerase chain reaction (RT-qPCR) and WB.
Real-time qPCR
Total RNA was harvested from the transfected SACC cells using TRIzol Reagent (Takara Biotechnology Co., Ltd., Kyoto, Japan) according to the manufacturer’s instructions, and complementary DNA (cDNA) was obtained using a reverse transcription kit (Takara Biotechnology Co., Ltd., Kyoto, Japan). The obtained cDNA was then amplified by real-time qPCR using the following primers (Table 1).
Table 1
Gene | Primer sequence |
---|---|
AJAP1 | Forward: 5'-CCTGCAGTGTTCTCACGAGT-3' |
Reverse: 5'-TCAGAAGAGGAGGGTCGGTT-3' | |
c-Myc | Forward: 5'-TGGAAAACCAGCCTCCCG-3' |
Reverse: 5'-TTCTCCTCCTCGTCGCAGTA-3' | |
CyclinD1 | Forward: 5'-ATCAAGTGTGACCCGGACTG-3' |
Reverse: 5'-CCTTGGGGTCCATGTTCTGC-3' | |
MMP1 | Forward: 5'-AGAGCAGATGTGGACCATGC-3' |
Reverse: 5'-TTGTCCCGATGATCTCCCCT-3' | |
GAPDH | Forward: 5'-AATGGGCAGCCGTTAGGAAA-3' |
Reverse: 5'-GCCCAATACGACCAAATCAGAG-3' |
AJAP1, adherens junctions associated protein 1; c-Myc, transcriptional regulator Myc-like; MMP1, matrix metallopeptidase 1; GAPDH, glyceraldehyde-3-phosphate dehydrogenase.
WB analysis
SACC cells were lysed with Cell lysis buffer for Western and IP (WBIP) containing phenylmethanesulfonyl fluoride (PMSF). The protein concentration was determined by BCA assay. Protein samples were isolated by Sodium Dodecyl Sulfate PolyAcrylamide Gel Electrophoresis (SDS-PAGE) and transferred to polyvinylidene fluoride (PVDF) membranes, which were then incubated with anti-AJAP1 (1:5,000; Proteintech), anti-E-cadherin (1:5,000; Proteintech), anti-β-catenin (1:5,000; Proteintech), anti-c-Myc (1:2,000; Proteintech), anti-CyclinD1 (1:5,000; Proteintech), anti-MMP1 (1:1,000; Proteintech), anti-actin (1:10,000; Proteintech), and anti-GAPDH (1:10,000; Proteintech) overnight. Following incubation, the membranes were washed three times with Tris-Buffered Saline and Tween 20 (TBST) and incubated with HRP-conjugated goat anti-rabbit/mouse IgG for 1 h. After washing with TBST three more times, the blots were analyzed using a gel imaging system (BIO-RAD, USA).
Cell viability and colony formation assays
For the cell viability assay, approximately 3,000 transfected SACC-LM and SACC-83 cells were plated in 96-well plates and cultured in a 37 ℃/5% CO2 incubator for 24, 48, 72, 96, and 120 h. Following incubation, the supernatant was discarded and 100 µL of CCK8 solution was added to each well before incubating at 37 ℃ for 2 h in a 5% CO2 incubator. Finally, the plates were read at 450 nm using a spectrometer (Bio Tek synergy LX, USA). For the colony formation assay, 1,000 cells were inoculated in each well of a six-well plate and cultured with RPMI 1640 containing 10% FBS in an incubator for 2 weeks. The colonies were stained with 0.1% crystal violet 20 min and photographed under a microscope (Nikon Ti, Japan).
Wound healing assay
SACC-LM and SACC-83 cells were plated in 12-well plates containing culture inserts (Ibidi, Germany). Next, 70 µL of cells were inoculated into each chamber of the culture insert. When the cell density reached 100%, the culture inserts were removed, and the cells were washed with PBS solution and cultured in RPMI 1640 medium without FBS. Images were taken at 0 and 24 h using a fluorescence microscope (Nikon).
Transwell invasion assay
The invasiveness of SACC-LM and SACC-83 cells was validated using a 24-well Transwell chamber (Corning Inc., USA). A layer of matrix gel was applied to the bottom of the Transwell chamber in advance, and the two SACC cells were re-suspended in serum-free 1640 medium and inoculated into the chamber. The lower layer of the chamber was inoculated with a complete medium. After 24 h, the invading cells were stained with 0.1% crystal violet 20 min and photographed under a microscope (Nikon).
Confocal microscopy
SACC-LM and SACC-83 cells were inoculated into a four-chamber glass bottom dish (Cellvis, USA), with approximately 3×104 cells inoculated into each well. After 24 h, the cells were fixed with methanol for 20 min and washed with PBS three times. The cells were blocked with PBS containing BSA for 20 min and washed with PBS three times before incubating with anti-flag, anti-β-catenin, and anti-E-cadherin at 4 ℃ overnight. Following incubation, the cells were washed with PBS three times and incubated with fluorescently labeled goat anti-rabbit/mouse IgG at room temperature for 1 h, before washing with PBS three times and staining with 2-(4-Amidinophenyl)-6-indolecarbamidine dihydrochloride (DAPI). After 10–15 min, the cells were subjected to laser scanning microscopy (Leica, Germany) and imaged.
Co-immunoprecipitation (Co-IP)
SACC-LM and SACC-83 cells were plated in 10 cm dishes and lysed with 500 µL cell lysis buffer for WB and IP, each containing 1 mM of PMSF to obtain proteins. The prepared protein solution was incubated with the corresponding primary antibody at 4 ℃ overnight. Next, 20 µL of fully suspended ProteinA+G Agarose (Beyotime, Shanghai, China) was added to the mixture, shaken slowly at 4 ℃ for 2 h, centrifuged at 1,000 g for 5 min, and the supernatant was discarded. Next, the precipitate was cleaned with 500 mL of PBS and centrifuged at 1,000 g for 5 min; this step was repeated five times. The supernatant was discarded for the final time, and 20 µL of 1× SDS-PAGE electrophoresis loading buffer was added to the precipitate and dried at 95 ℃ for 10 min. WB was performed using the corresponding antibodies.
Dual-luciferase reporter gene analysis
Next, to conduct dual luciferase reporter gene analysis, 2.5×105 SACC cells were inoculated into 24-well plates and cultured for 24 h. When the concentration reached 70–80%, the cells were transfected with β-catenin reactive double luciferin reporter plasmid Top-Flash or a negative control FOPFLASH (Merck-Millipore, USA) using the fugene6 (Invitrogen, USA) kit. After 48 h of transfection, luciferase reporter gene detection was performed according to the dual luciferase reporter gene detection system (Promega, Madison, WI, USA). Each experiment was measured in triplicate.
In vivo subcutaneous xenograft tumor model
Four-week-old nude mice were purchased from Hangzhou Ziyuan Experimental Animal Science and Technology Co., Ltd (Hangzhou, China). Animal experiments were performed under a project license (No. A2022010101) granted by Experimental Animal Welfare Ethics Committee of Zhejiang Provincial People’s Hospital, in compliance with institutional guidelines for the care and use of animals.
The nude mouse transplanted tumor model was generated as follows: all nude mice were randomly divided into four groups with six mice in each group by simple randomization, and a suspension containing 106 SACC-LM (AJAP1-overexpressed and control) or SACC-83 (AJAP1 knockout and control) cells were injected into the axilla. The tumor size and volume were measured regularly and after 30 days, the animals were executed painlessly for tumor dissection, and the samples were prepared for immunohistochemical analysis.
Statistical analysis
SPSS 18.0 statistical software (SPSS, Chicago, IL, USA) was adopted for data analysis. Counting data comparisons between groups were subjected to the χ2 test or Fisher’s exact test. P<0.05 was considered statistically significant. Survival analysis was computed by means of the Kaplan-Meier method and significance was assessed by means of the log-rank test. A multivariate analysis of the Cox regression model was used to determine prognostic factors.
Results
AJAP1 expression is significantly down-regulated in SACC
In the previous experiment, we selected three pairs of SACC tissues and paracancerous tissues for whole-genome sequencing, and the results showed that 4,386 genes were upregulated and 3,119 genes were downregulated in SACC tumors. GO and pathway enrichment analyses were performed to predict the functions of the genes. The GO enrichment analysis results indicated that the enrichment degree of differentially expressed genes was the highest in Biological adhesion, followed by Regulation of cell mobility, Intrinsic component of plasma membrane, Regulation of phosphate metabolic process, Response to organic substance (P<0.01). The Pathway enrichment analysis results indicated that the enrichment degree of differentially expressed genes was the highest in Extracellular matrix organization, followed by Rheumatoid arthritis, O-linked glycosylation, Interferon alpha/beta signaling, Collagen formation (P<0.01) (Figure 1A-1D). As both of the different expressed genes in Biological adhesion and Extracellular matrix organization processes contain AJAP1, we selected AJAP1 as the candidate research target. Furthermore, to verify the expression of AJAP1 in SACC tissues, we detected the expression of AJAP1 protein in the cancer tissues of patients with SACC was 44.76% (47/105), while in paracancerous tissues, the positive rate of the AJAP1 protein was 86.67% (91/105), which was significantly higher than that in cancer tissues (P<0.01) (Figure 1E-1I).
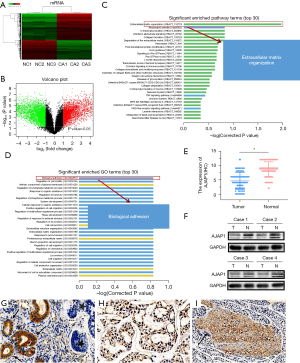
Low AJAP1 expression was significantly related to SACC lymph node metastasis and distant metastasis
We used the chi-squared test to further explore the correlation between AJAP1 expression and the various clinical factors of SACC. The results showed that the downregulation of AJAP1 expression was closely related to age, lymph node metastasis/distant metastasis, and tumor nodes metastasis (TNM) stage, but was not related to sex, primary tumor site, histotype, and nerve invasion. Moreover, AJAP1 expression was significantly downregulated in the elderly group, lymph node metastasis and distant metastasis group, and TNM stage III–IV group (P<0.05) (Table 2). These results suggest that AJAP1 plays an important role in the invasion and metastasis of SACC cells.
Table 2
Parameters | Cases | AJAP1 | χ² | P | |
---|---|---|---|---|---|
Negative | Positive | ||||
Gender | |||||
Female | 63 | 33 | 30 | 0.520 | 0.417 |
Male | 42 | 25 | 17 | ||
Age (years) | |||||
<51 | 49 | 21 | 28 | 5.696 | 0.017* |
≥51 | 56 | 37 | 19 | ||
Primary tumor site | |||||
Minor salivary glands | 72 | 41 | 31 | 0.270 | 0.603 |
Major salivary glands | 33 | 17 | 16 | ||
Histotypes | |||||
Cribriform | 58 | 26 | 32 | 5.731 | 0.057 |
Tubular | 27 | 18 | 9 | ||
Solid | 20 | 14 | 6 | ||
Nerve invasion | |||||
− | 51 | 31 | 20 | 1.234 | 0.276 |
+ | 54 | 27 | 27 | ||
Lymph node/distant metastasis | |||||
− | 91 | 46 | 45 | 6.068 | 0.014* |
+ | 14 | 12 | 2 | ||
TNM stage | |||||
I–II | 45 | 14 | 31 | 18.54 | 0.000* |
III–IV | 60 | 44 | 16 |
*, P<0.05. TNM, tumor nodes metastasis.
Kaplan-Meier analysis showed that patients with lymph node/distant metastasis, TNM stage III–IV, and AJAP1 expression loss in the SACC group all had a poorer prognosis, including both progression-free survival (PFS) and overall survival (OS). In both the TNM stage I–II and III–IV subgroups, patients with SACC with AJAP1-deficient expression had a poorer prognosis, including PFS and OS (Figure 2). Cox regression analysis further revealed that low AJAP1 expression was an independent risk factor for SACC prognosis (P=0.035) (Table 3).
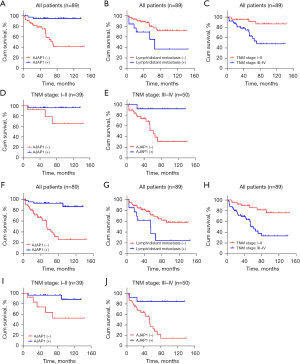
Table 3
Variables | Univariate analysis | Multivariate analysis | |||||||
---|---|---|---|---|---|---|---|---|---|
Exp(B) | 95% CI Exp(B) | Sig. | Exp(B) | 95% CI Exp(B) | Sig. | ||||
Lower | Upper | Lower | Upper | ||||||
Gender (female vs. male) | 0.845 | 0.353 | 2.025 | 0.706 | – | – | – | – | |
Age (<51 vs. ≥51 years) | 3.889 | 1.419 | 10.661 | 0.008 | 1.899 | 0.615 | 5.859 | 0.265 | |
Primary tumor site (minor vs. major) | 0.719 | 0.263 | 1.964 | 0.52 | – | – | – | – | |
Histotypes (cribriform vs. tubular/solid) | 3.22 | 1.295 | 8.007 | 0.012 | 1.687 | 0.574 | 4.962 | 0.342 | |
Lymph node/distant metastasis (negative vs. positive) | 2.87 | 1.107 | 7.439 | 0.03 | 1.835 | 0.697 | 4.831 | 0.219 | |
Nerve invasion (negative vs. positive) | 0.88 | 0.37 | 2.094 | 0.773 | – | – | – | – | |
TNM stage (I–II vs. III–IV) | 4.773 | 1.594 | 14.293 | 0.005 | 2.084 | 0.614 | 7.072 | 0.239 | |
AJAP1 (negative vs. positive) | 0.092 | 0.021 | 0.396 | 0.001 | 0.184 | 0.038 | 0.887 | 0.035 |
OS, overall survival; SACC, salivary adenoid cystic carcinoma; CI, confidence interval; TNM, tumor nodes metastasis.
High methylation of the promoter cytosine-phosphoric acid-guanine (CpG) island leads to silencing of AJAP1 expression in SACC tumors
Through pan-cancer analysis, we found that AJAP1 expression in various tumor tissues was lower than that in normal tissues (TCGA: http://ualcan.path.uab.edu/cgi-bin/Pan-cancer.pl?genenam=AJAP1) (GEPIA: http://gepia.cancer-pku.cn/detail.php?gene=AJAP1) and was highly expressed in normal salivary gland tissues (GeneCards: https://www.genecards.org/cgi-bin/carddisp.pl?gene=AJAP1) (Figure 3A-3C). To further explore the cause of downregulated AJAP1 expression in SACC tumors, we found that the AJAP1 promoter regions exist on numerous CpG islands through University of California, Santa Cruz (UCSC) database analysis (UCSC: http://genome.ucsc.edu/cgi-bin/hgTracks?db=hg38&lastVirtModeType=default&lastVirtModeExtraState=&virtModeType=default&virtMode=0&nonVirtPosition=&position=chr1%3A4654609-4792534&hgsid=1404994269_Afxnfju9XzXGW6eTYUVbavPiZ2aQ) (Figure 3D,3E). To further verify this result, DNA was extracted from the paraffin sections of 106 groups of SACC tumors and adjacent tissues. The methylation of the AJAP1 promoter was detected by MSP. The results showed that the methylation degree of the AJAP1 promoter in SACC tumor tissues was significantly higher than that in adjacent normal tissues. Correlation analysis showed that AJAP1 promoter methylation was closely related to low AJAP1 expression in tumor tissues (P<0.01) (Figure 3F). The MSP results indicated that the target gene band (M band) of methylated primers was significantly lighter than the target gene band (U band) of unmethylated primers in the control group. However, after being treated with 5-AZA, the target gene band (U band) of unmethylated primers became lighter, and the target gene band (M band) of methylated primers became dimmer (Figure 3G,3H). When the methylation inhibitor 5-Aza was added to SACC cells, the messenger RNA (mRNA) and protein levels of AJAP1 were significantly upregulated in a dose- and time-dependent manner, suggesting that the methylation of the AJAP1 promoter in SACC tumor cells may be the main reason for its downregulation (Figure 3I,3J).
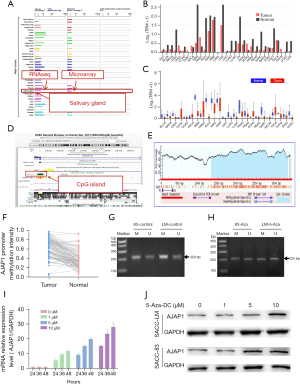
AJAP1 overexpression or knockdown inhibited or promoted the invasion, metastasis, and proliferation of SACC cells, respectively
To further explore the effects of changes in AJAP1 expression on the proliferation, invasion, and metastasis of SACC cells, we detected the expression of the AJAP1 protein in SACC-LM and SACC-83 cells under wild-type conditions. The results showed that the AJAP1 protein level was relatively low in SACC-LM cells but was relatively high in SACC-83 cells. Therefore, an AJAP1 overexpression test was performed on SACC-LM cells, and an AJAP1 knockdown test was conducted on SACC-83 cells in subsequent experiments (Figure 4A-4D). The CCK8 proliferation assay results showed that AJAP1 overexpression in SACC-LM cells significantly downregulated the proliferation of tumor cells, while AJAP1 knockdown in SACC-83 cells significantly upregulated the proliferation of tumor cells. Moreover, the colony formation assay results showed that AJAP1 overexpression in SACC-LM cells significantly downregulated the colony formation ability of tumor cells, whereas AJAP1 knockdown in SACC-83 cells significantly upregulated their colony formation ability (Figure 4E-4J). The wound healing assay results demonstrated that the migration ability of tumor cells was significantly downregulated after AJAP1 overexpression in SACC-LM cells, and was significantly upregulated after AJAP1 knockdown in SACC-83 cells. The Transwell assay showed that AJAP1 overexpression in SACC-LM cells significantly downregulated the invasion ability of tumor cells, while AJAP1 knockdown in SACC-83 cells significantly upregulated their invasion ability (Figure 4K-4R).
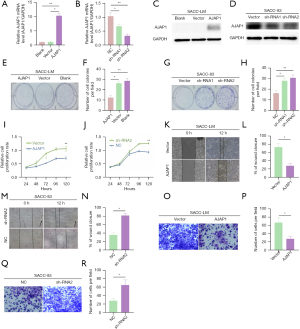
AJAP1 reduces the nuclear localization of β-catenin by forming the AJAP1/E-cadherin/β-catenin complex
To further explore the molecular mechanism through which AJAP1 regulates the proliferation, invasion, and metastasis of SACC cells, we pulled down the possible binding protein of AJAP1 by co-IP and found that the AJAP1 pull-down protein contained E-cadherin and β-catenin. Thus, we repeated the co-IP experiment with E-cadherin and β-catenin antibodies. Both AJAP1 and β-catenin were detected in the E-cadherin pull-down proteins, while both E-cadherin and AJAP1 were detected in the β-catenin pull-down proteins. These results suggested that AJAP1 may form a complex with E-cadherin and β-catenin in SACC cells (Figure 5A-5C).
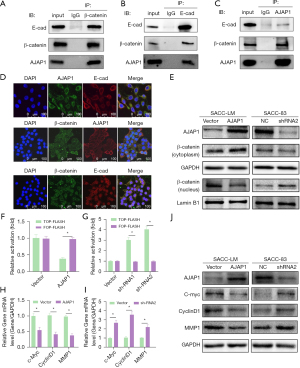
We then detected the subcellular localization of AJAP1, E-cadherin, and β-catenin by laser scanning confocal microscopy, and the results showed co-localization in the cytomembrane (Figure 5D). Moreover, when AJAP1 was knocked down or overexpressed in SACC-83/LM cells, we found that β-catenin showed increased or decreased nuclear localization, respectively. Additionally, β-catenin expression in the cytomembrane/cytoplasm and nucleus was detected by WB, which showed that when AJAP1 was overexpressed in SACC-LM, the expression of β-catenin in the cytoplasm was increased but was downregulated in the nucleus. The knockdown of AJAP1 expression in SACC-83 cells exhibited the opposite result (Figure 5E).
AJAP1 regulates the expression of β-catenin downstream genes by regulating its transcriptional activity
Previous results suggest that AJAP1 knockdown or overexpression affects the subcellular localization of β-catenin; however, whether this directly affects the transcriptional activity of β-catenin remains unclear. To this end, we further designed a β-catenin-responsive firefly luciferase reporter. Next, the plasmid T-cell factor/lymphoid enhancing factor (TCF/LEF) (TOP-FLASH) and non-responsive reporter plasmid (FOP-FLASH) were employed to evaluate the effect of AJAP1 on β-catenin transcriptional activity. The results showed that the upregulation of AJAP1 in SACC-LM cells reduced the transcriptional activity of β-catenin/TCF/LEF-dependent promoter (TOP-FLASH), but had little effect on FOP-FLASH. In contrast, AJAP1 knockout in SACC-83 cells significantly increased the transcriptional activity of β-catenin/TCF/LEF-dependent promoters (Figure 5F,5G). AJAP1 overexpression in SACC-LM cells decreased the expression of c-Myc, CyclinD1, and MMP1 at the mRNA and protein levels, while AJAP1 knockout in SACC-83 cells upregulated the mRNA and protein expression of c-Myc, CyclinD1, and MMP1 (Figure 5H-5J). These results suggest that AJAP1 deletion affects the nuclear localization of β-catenin, activates the transcriptional activity of β-catenin, and promotes the expression of its downstream genes.
Regulation of the Wnt/β-catenin signaling pathway could reverse the effect of AJAP1 on SACC cell function
Wnt/β-catenin agonist 2, an activator of the Wnt/β-catenin signaling pathway, was added into a culture medium of SACC-LM cells overexpressing AJAP1. The Transwell, wound healing, and CCK-8 assay results showed that Wnt/β-catenin agonist 2 could reverse the downregulation of invasion, migration, and proliferative ability of SACC-LM cells induced by AJAP1 overexpression, respectively (Figure 6). We then added LF3, an inhibitor of the Wnt/β-catenin signaling pathway, into a culture medium containing knocked-down AJAP1 SACC-83 cells. The Transwell, wound healing, and CCK-8 assay results showed that LF3 could reverse the upregulation of invasion, migration, and proliferative ability of SACC-83 cells induced by AJAP1 knockdown, respectively (Figure 6). These results suggest that AJAP1 may function by regulating the Wnt/β-catenin signaling pathway.
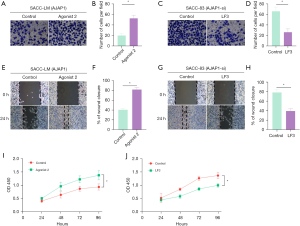
Subcutaneous xenograft assay confirmed that AJAP1 inhibited the growth and metastasis of SACC tumors
We next designed a subcutaneous xenograft assay in nude mice to verify the function of AJAP1 in vivo. The results showed that AJAP1 overexpression in SACC-LM cells significantly reduced the tumorigenesis ability of SACC cells compared to the control group, suggesting that the overexpression of AJAP1 significantly inhibited the tumorigenesis ability of SACC cells. However, when AJAP1 was knocked down in SACC-83 cells, the tumor-forming ability was significantly stronger than that in the control group, suggesting that AJAP1 knockdown can significantly promote the tumor-forming ability of SACC cells. Additionally, immunohistochemical detection was performed on the excised tumor tissues, and the results showed that the expression levels of the c-Myc, CyclinD1, and MMP1 downstream proteins of the β-catenin signaling pathway were significantly downregulated in the AJAP1 overexpression group and were significantly upregulated in the AJAP1 knockdown group (Figure 7).
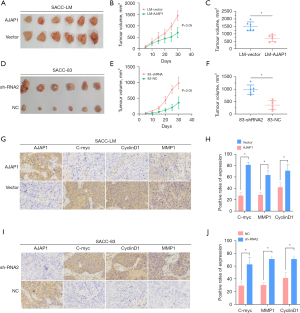
Discussion
AJAP1 was initially identified as a novel transmembrane protein for epithelial cell adhesion, located in the 1p36 region of chromosome 1 (18). Numerous studies have identified multiple tumor suppressor-related genes in the 1p36 region, including AJAP1, Calcium/calmodulin dependent protein kinase II gamma (CAMK2NA) (19), Chromodomain helicase DNA binding protein 5 (CHD5) (20), Kinesin family member 1B (KIF1B) (21), Castor zinc finger 1 (CASZ1) (22), and miR-34a (23), which may be related to the occurrence and development of neural, endocrine, and reproductive tumors. In this study, high-throughput chip screening and clinicopathological analysis revealed that AJAP1 expression deficiency may be associated with the progression and metastasis of SACC. Moreover, Cox regression analysis revealed that deficient AJAP1 expression is an independent risk factor for SACC prognosis, and therefore, may play an important role in the progression of SACC.
At present, the mechanism underlying the upstream regulation of AJAP1 is unclear. Qu et al. (16) found that AJAP1 mRNA has a direct binding site with miR-552 in hepatocellular carcinoma (HCC) cells, and its expression may be negatively regulated by miR-552. A previous study has shown that a high degree of methylation exists in the 1p36 region in some tumors, which may explain the loss of expression of related tumor suppressor genes (24). Through database retrieval and MSP, we found that the AJAP1 promoter region was highly methylated in SACC tumor cells and that demethylation drugs could significantly reduce the methylation level in this region and restore the expression of AJAP1. These results suggest that abnormal hypermethylation of the AJAP1 promoter in SACC tumors is an important cause of the loss of AJAP1 expression.
The role of AJAP1 in tumor progression remains controversial. In GBM, Di et al. (15) found that the AJAP1 protein could increase the intercellular adhesion, reduce invasion, metastasis, proliferation, and tumor-forming ability of tumor cells, and the loss of AJAP1 expression was frequently correlated with a poor prognosis. Chen et al. (25) found that AJAP1 works like a tumor suppressor in prostate cancer and hypermethylation of CpG islands of AJAP1 promoter affected AJAP1 gene expression, and low expression of AJAP1 could activate downstream JAK2/STAT3 signaling pathway, promoting prostate cancer cell migration. Moreover, Qu et al. (16) found that miR-552 could promote tumor proliferation, invasion, and metastasis by downregulating AJAP1 expression in liver cancer, and showed that patients with AJAP1 deletion had a significantly worse prognosis than those with normal expression. Furthermore, Xu et al. (26) demonstrated that AJAP1 had a highly negative correlation with β-catenin nuclear expression and was a novel tumor suppressor in breast cancer, while β-catenin nuclear localization positively fed back on EGF/EGFR-attenuated AJAP1 expression in breast cancer. In this study, by constructing AJAP1 overexpression and knockdown cell lines, we found that AJAP1 overexpression or knockdown could significantly inhibit or promote the proliferation, invasion, and metastasis of SACC cells, respectively, and reduce the tumorigenicity of SACC cells in vivo. The findings were consistent with those of Chunhui Di and Weiqing Qu, indicating that AJAP1 plays a pivotal role as a tumor suppressor gene in the progression and metastasis of SACC (15,16). Although recent studies have found that AJAP1 plays a certain role in cell proliferation (27), apoptosis (15), invasion (13), and metastasis, the specific mechanism remains unclear. Using confocal imaging, Han et al. showed that AJAP1 could stably transfect GBM cells and change the cell growth pattern, morphology, and distribution of β-tubulin and F-actin, thereby reorganizing the cytoskeletal structure, promoting intercellular adhesion, and inhibiting cell invasion and metastasis (28). AJAP1, which co-localizes and co-immunoprecipitates with endogenous E-cadherin, was initially described as a novel component of the adhesion of polarized epithelial cells and was suggested to bind directly to E-cadherin (29). Indeed, direct binding between E-cadherin and β-catenin has been found in a previous study (30). Therefore, in this study, the relationship between AJAP1/E-cadherin/β-catenin was detected by laser scanning confocal microscopy and immunoprecipitation assay, indicating co-localization between AJAP1/E-cadherin/β-catenin, which could directly bind to form complexes. Moreover, the loss of AJAP1 expression could lead to the nuclear translocation of β-catenin, which reduces the content of β-catenin in the cytoplasm and capsule, but increases its content in the nucleus, thereby activating the β-catenin signaling pathway and promoting the expression of the c-Myc, CyclinD1, and MMP-1 downstream genes, thus promoting the progression and metastasis of SACC tumors. Using the activator and inhibitor of the Wnt/β-catenin signaling pathway, we further confirmed that AJAP1 may exert its function in SACC cells by regulating the Wnt/β-catenin signaling pathway.
Wnt/β-catenin signaling pathway has been found abnormal activation in many solid cancers, including SACC. Ji et al. found CLDN7 inhibits cell proliferation and metastasis by inactivating the Wnt/β-catenin signaling in SACC (31). Fatty acid synthase was conformed contributes to epithelial-mesenchymal transition and invasion of SACC through PRRX1/Wnt/β-catenin pathway (32). The overactivation of Wnt/β-catenin signaling pathway was found be associated with radioresistance of cervical cancer and esophageal squamous cell carcinoma, while its role in SACC radioresistance is still unclear and more research is needed (33,34).
Meanwhile, the in vivo subcutaneous xenograft assay in nude mice demonstrated that overexpression or knockdown of AJAP1 in SACC cells significantly inhibited or promoted tumorigenesis in nude mice, respectively. These results suggest that in SACC tumors, AJAP1 binds β-catenin to the membrane and cytoplasm through the formation of an AJAP1/e-cadherin/β-catenin complex and reduces the amount of β-catenin entering the nucleus, thus blocking the Wnt/β-catenin signaling pathway and mediating its anti-cancer effects.
Conclusions
In conclusion, the high methylation of the AJAP1 promoter leads to loss of expression, which then activates the Wnt/β-catenin signaling pathway and promotes tumor progression and metastasis. Until now, this mechanism has not been previously reported in SACC tumors. We believe that the results of our study will provide new ideas for the treatment of SACC, and direct toward the development of new targeted drugs.
Acknowledgments
We thank LetPub (www.letpub.com) for its linguistic assistance during the preparation of this manuscript.
Funding: This study was supported by the Natural Science Foundation of Zhejiang (No. LY23H160025), the Zhejiang Medicine and Health Project of Science and Technology (Nos. 2022KY525, 2021KY055), and the National Natural Science Foundation of China (No. 81702644).
Footnote
Reporting Checklist: The authors have completed the ARRIVE and MDAR reporting checklists. Available at https://gs.amegroups.com/article/view/10.21037/gs-23-127/rc
Data Sharing Statement: Available at https://gs.amegroups.com/article/view/10.21037/gs-23-127/dss
Peer Review File: Available at https://gs.amegroups.com/article/view/10.21037/gs-23-127/prf
Conflicts of Interest: All authors have completed the ICMJE uniform disclosure form (available at https://gs.amegroups.com/article/view/10.21037/gs-23-127/coif). The authors have no conflicts of interest to declare.
Ethical Statement: The authors are accountable for all aspects of the work in ensuring that questions related to the accuracy or integrity of any part of the work are appropriately investigated and resolved. The study was conducted in accordance with the Declaration of Helsinki (as revised in 2013). The study was approved by Medical Ethics Committee of Zhejiang Provincial People’s Hospital (No. QT2022326) and informed consent was taken from all the patients. Animal experiments were performed under a project license (No. A2022010101) granted by Experimental Animal Welfare Ethics Committee of Zhejiang Provincial People’s Hospital, in compliance with institutional guidelines for the care and use of animals.
Open Access Statement: This is an Open Access article distributed in accordance with the Creative Commons Attribution-NonCommercial-NoDerivs 4.0 International License (CC BY-NC-ND 4.0), which permits the non-commercial replication and distribution of the article with the strict proviso that no changes or edits are made and the original work is properly cited (including links to both the formal publication through the relevant DOI and the license). See: https://creativecommons.org/licenses/by-nc-nd/4.0/.
References
- Sahara S, Herzog AE, Nör JE. Systemic therapies for salivary gland adenoid cystic carcinoma. Am J Cancer Res 2021;11:4092-110. [PubMed]
- Gentili F, Guerrini S, Mazzei FG, et al. Dual energy CT in gland tumors: a comprehensive narrative review and differential diagnosis. Gland Surg 2020;9:2269-82. [Crossref] [PubMed]
- Wang HF, Wang SS, Zheng M, et al. Hypoxia promotes vasculogenic mimicry formation by vascular endothelial growth factor A mediating epithelial-mesenchymal transition in salivary adenoid cystic carcinoma. Cell Prolif 2019;52:e12600. [Crossref] [PubMed]
- Lassche G, van Boxtel W, Ligtenberg MJL, et al. Advances and challenges in precision medicine in salivary gland cancer. Cancer Treat Rev 2019;80:101906. [Crossref] [PubMed]
- Tang YF, Wu WJ, Zhang JY, et al. Reconstruction and analysis of the aberrant lncRNA-miRNA-mRNA network based on competitive endogenous RNA in adenoid cystic carcinoma of the salivary gland. Transl Cancer Res 2021;10:5133-49. [Crossref] [PubMed]
- Jung I, An J, Ko M. Epigenetic Regulators of DNA Cytosine Modification: Promising Targets for Cancer Therapy. Biomedicines 2023;11:654. [Crossref] [PubMed]
- Zhou XQ, Huang SY, Zhang DS, et al. Effects of 5-aza-2’deoxycytidine on RECK gene expression and tumor invasion in salivary adenoid cystic carcinoma. Braz J Med Biol Res 2015;48:254-60. [Crossref] [PubMed]
- Zhang CY, Zhao YX, Xia RH, et al. RASSF1A promoter hypermethylation is a strong biomarker of poor survival in patients with salivary adenoid cystic carcinoma in a Chinese population. PLoS One 2014;9:e110159. [Crossref] [PubMed]
- Shao C, Tan M, Bishop JA, et al. Suprabasin is hypomethylated and associated with metastasis in salivary adenoid cystic carcinoma. PLoS One 2012;7:e48582. [Crossref] [PubMed]
- Bharti S, Handrow-Metzmacher H, Zickenheiner S, et al. Novel membrane protein shrew-1 targets to cadherin-mediated junctions in polarized epithelial cells. Mol Biol Cell 2004;15:397-406. [Crossref] [PubMed]
- Xu C, Wang F, Hao L, et al. Expression Patterns of Ezrin and AJAP1 and Clinical Significance in Breast Cancer. Front Oncol 2022;12:831507. [Crossref] [PubMed]
- Li R, Chen Y, Wu J, et al. LncRNA FGF14-AS2 represses growth of prostate carcinoma cells via modulating miR-96-5p/AJAP1 axis. J Clin Lab Anal 2021;35:e24012. [Crossref] [PubMed]
- Klemmt PA, Resch E, Smyrek I, et al. Alternative exon usage creates novel transcript variants of tumor suppressor SHREW-1 gene with differential tissue expression profile. Biol Open 2016;5:1607-19. [Crossref] [PubMed]
- Han J, Xie C, Pei T, et al. Deregulated AJAP1/β-catenin/ZEB1 signaling promotes hepatocellular carcinoma carcinogenesis and metastasis. Cell Death Dis 2017;8:e2736. [Crossref] [PubMed]
- Di C, Mladkova N, Lin J, et al. AJAP1 expression modulates glioma cell motility and correlates with tumor growth and survival. Int J Oncol 2018;52:47-54. [PubMed]
- Qu W, Wen X, Su K, et al. MiR-552 promotes the proliferation, migration and EMT of hepatocellular carcinoma cells by inhibiting AJAP1 expression. J Cell Mol Med 2019;23:1541-52. [Crossref] [PubMed]
- Yan YL, Huang ZN, Zhu Z, et al. Downregulation of TET1 Promotes Bladder Cancer Cell Proliferation and Invasion by Reducing DNA Hydroxymethylation of AJAP1. Front Oncol 2020;10:667. [Crossref] [PubMed]
- Lin N, Di C, Bortoff K, et al. Deletion or epigenetic silencing of AJAP1 on 1p36 in glioblastoma. Mol Cancer Res 2012;10:208-17. [Crossref] [PubMed]
- Peng JM, Tseng RH, Shih TC, et al. CAMK2N1 suppresses hepatoma growth through inhibiting E2F1-mediated cell-cycle signaling. Cancer Lett 2021;497:66-76. [Crossref] [PubMed]
- Xu L, Shao F, Luo T, et al. Pan-Cancer Analysis Identifies CHD5 as a Potential Biomarker for Glioma. Int J Mol Sci 2022;23:8489. [Crossref] [PubMed]
- Guan Y, Yang W, Zhang F, et al. CircPOSTN competes with KIF1B for miR-185-5p binding sites to promote the tumorigenesis of glioma. Brain Res Bull 2022;180:86-96. [Crossref] [PubMed]
- Liu Z, Zhang X, Xu M, et al. Loss of CASZ1 tumor suppressor linked to oncogenic subversion of neuroblastoma core regulatory circuitry. Cell Death Dis 2022;13:871. [Crossref] [PubMed]
- Li S, Wei X, He J, et al. The comprehensive landscape of miR-34a in cancer research. Cancer Metastasis Rev 2021;40:925-48. [Crossref] [PubMed]
- Titus AJ, Way GP, Johnson KC, et al. Deconvolution of DNA methylation identifies differentially methylated gene regions on 1p36 across breast cancer subtypes. Sci Rep 2017;7:11594. [Crossref] [PubMed]
- Chen L, Wang Y, Zhang B. Hypermethylation in the promoter region inhibits AJAP1 expression and activates the JAK/STAT pathway to promote prostate cancer cell migration and stem cell sphere formation. Pathol Res Pract 2023;241:154224. [Crossref] [PubMed]
- Xu C, Liu F, Xiang G, et al. β-Catenin nuclear localization positively feeds back on EGF/EGFR-attenuated AJAP1 expression in breast cancer. J Exp Clin Cancer Res 2019;38:238. [Crossref] [PubMed]
- Yang C, Li YS, Wang QX, et al. EGFR/EGFRvIII remodels the cytoskeleton via epigenetic silencing of AJAP1 in glioma cells. Cancer Lett 2017;403:119-27. [Crossref] [PubMed]
- Han L, Zhang KL, Zhang JX, et al. AJAP1 is dysregulated at an early stage of gliomagenesis and suppresses invasion through cytoskeleton reorganization. CNS Neurosci Ther 2014;20:429-37. [Crossref] [PubMed]
- Hötte K, Smyrek I, Starzinski-Powitz A, et al. Endogenous AJAP1 associates with the cytoskeleton and attenuates angiogenesis in endothelial cells. Biol Open 2017;6:723-31. [Crossref] [PubMed]
- Katuri V, Tang Y, Li C, et al. Correction: critical interactions between TGF-β signaling/ELF, and E-cadherin/β-catenin mediated tumor suppression. Oncogene 2021;40:3348-9. [Crossref] [PubMed]
- Ji H, Ding X, Zhang W, et al. Claudin-7 Inhibits Proliferation and Metastasis in Salivary Adenoid Cystic Carcinoma Through Wnt/β-Catenin Signaling. Cell Transplant 2020;29:963689720943583. [Crossref] [PubMed]
- Zhang WL, Wang SS, Jiang YP, et al. Fatty acid synthase contributes to epithelial-mesenchymal transition and invasion of salivary adenoid cystic carcinoma through PRRX1/Wnt/β-catenin pathway. J Cell Mol Med 2020;24:11465-76. [Crossref] [PubMed]
- Fang F, Guo C, Zheng W, et al. Exosome-Mediated Transfer of miR-1323 from Cancer-Associated Fibroblasts Confers Radioresistance of C33A Cells by Targeting PABPN1 and Activating Wnt/β-Catenin Signaling Pathway in Cervical Cancer. Reprod Sci 2022;29:1809-21. [Crossref] [PubMed]
- Zhou H, Wang G, Xiao Z, et al. NRAGE Confers Radiation Resistance in 2D and 3D Cell Culture and Poor Outcome in Patients With Esophageal Squamous Cell Carcinoma. Front Oncol 2022;12:831506. [Crossref] [PubMed]
(English Language Editor: A. Kassem)