The central glial cell line-derived neurotrophic factor (GDNF) regulates pulmonary function in asthmatic rats
Highlight box
Key findings
• In an asthmatic rat model, GDNF expressions are increased in the airways and multiple brain regions during an asthma attack.
What is known and what is new?
• Asthma attacks are closely related to the central nervous system.
• The increased GDNF in the central nervous system would induce pulmonary function deterioration in the asthmatic rat.
What is the implication, and what should change now?
• The central GDNF may affect asthma attack and might serve as a therapeutic target.
Introduction
Asthma is a common airway inflammatory disease worldwide. Uncontrolled asthma imposes a huge medical burden and impairs patient quality of life (1). Allergens (including environmental pollutants, pollen, pathogenic microorganisms, etc.) and induced inflammatory factors could cause contraction of bronchial smooth muscle, resulting in clinical symptoms such as airway spasm and respiratory distress. The pathogenesis of asthma has not been fully clarified, and airway inflammation has been viewed as the main pathogenesis (2). It has been confirmed that asthma is not only caused by local immunogenic inflammatory mediators in the airway, but also in the brain (3,4). The microglia in the central nervous system (CNS), in addition to secreting various cytokines, can also secrete neurotrophic factors, such as glial cell line-derived neurotrophic factor (GDNF) (5). Classical neurotrophins are now known to be expressed and functional in non-neuronal systems including lung (6). Experimental airway allergy can activate microglia and increase brain-derived neurotrophic factor (BDNF) concentration of the airway (7), but it is unclear whether GDNF increases in the airway. GDNF is expressed in lung tissues (including airway smooth muscle) and contributes to the development of the lungs (8). GDNF receptors [ret proto-oncogene (RET) and glial cell line derived neurotrophic factor family receptor alpha 1 (GFRα1)] are expressed on the vagus afferent nerves innervating the airways (9). The level and duration of microglial activation determine whether GDNF overexpression is beneficial or detrimental, and the RET and GFRα1 in the microglia could be upregulated, implying that GDNF is closely associated with neuroinflammation (10). To date, a study regarding the role of GDNF have been mainly concerned with neurological and psychiatric diseases (11). However, the association with GDNF in the CNS and asthma has not been reported. Therefore, this study mainly aimed to confirm the role of central GDNF in asthma. We present the following article in accordance with the ARRIVE reporting checklist (available at https://atm.amegroups.com/article/view/10.21037/atm-22-6338/rc).
Methods
Animals
Male Sprague Dawley rats (250–350 grams, 7–8 weeks) were purchased from the Experimental Animal Center of Jiangsu Province and housed in a silent environment (18-25 ℃), provided with rhythmic lighting on a 10 h/14 h day/night cycle, and given free access to food and water. Prior to the experiments, the rats were fed for 1 week. Animal experiments were performed under a project license (No. 20180903) granted by the ethics board of the First People’s Hospital of Kunshan. All experimental procedures were conducted following the Guide for the Care and Use of Laboratory Animals, 8th edition. A protocol was prepared before the study without registration.
According to the previous study (12), the experimental group rats were injected intraperitoneally with ovalbumin (OVA; 100 mg), aluminum hydroxide (100 mg) and a mixed suspension of inactivated Bordetella pertussis (5×109 copies) on days 1 and 3 (1 mL each time). On days 15 to 17, 1% OVA was nebulized using an ultrasonic nebulizer and inhaled by rats for 150 min. Asthmatic rats exhibited irritability, shortness of breath, dyspnea, wheezing, marked abdominal muscle contraction, and cough.
Pulmonary function test
All the animals were anesthetized by intraperitoneal injection with 0.4% pentobarbital sodium (40 mg/kg). Rat’s limbs and heads were fixed in the supine position. An inverted “T” shaped incision was made in the trachea after the trachea was separated. An airflow exchanger-connected pipe was inserted. Incisions were made transversely in the esophagus, and a catheter with 4-sided holes was inserted. A venous pressure sensor was used to monitor esophageal pressure instead of intrathoracic pressure. Multi-channel physiological signal acquisition and processing system collects signals such as respiratory flow and esophageal pressure. The pulmonary function data was recorded within 30 min before and after the asthma attack. The relevant indexes of lung function were calculated automatically by the system.
BALF
After injecting 3 mL of 0.3% phosphate-buffered saline (PBS) solution into the trachea and cycling it 5 times, BALF was collected in a centrifuge tube, incubated at 4 ℃ for 10 min, then centrifuged at 1,500 rpm for 10 min. The supernatant at was cryopreserved −20 ℃, and BDNF concentration was tested by enzyme-linked immunosorbent assay (ELISA). The pellets were collected for cell counting. The left lung and brain tissues were removed for hematoxylin and eosin (HE) staining and immunohistochemistry (IHC).
GDNF IHC staining
The left lung and brain tissues were placed in 4% paraformaldehyde (PFA), and then cryoprotected in 30% sucrose at 4 ℃ for 48 hours. Tissue was frozen by optimal cutting temperature (OCT) compound, and 20 µm coronal sections (40 µm lung tissue) were subjected to IHC using a Leica freezing microtome (Leica, Wetzlar, Germany). Then, 3% H2O2 was used to block endogenous peroxidase activity for 20 min, and the sections were washed with 0.3% PBS (3×5 min) and incubated with blocking solution (10% goat serum) for 1 hour at room temperature, followed by incubation with primary antibody (rabbit anti-GDNF; 1:500; Abcam, Cambridge, UK). Tissues were washed with 0.3% PBS (3×5 min) followed by biotinylated secondary antibody (goat anti-mouse; 1:300; Abcam). After washing with 0.3% PBS (3×5 min), sections were incubated with affinity/biotinylated horseradish peroxidase (HRP) for 30 min, washed with 0.3% PBS (3×5 min), and reacted with 3,3'-diaminobenzidine (DAB) as a chromogenic agent.
Microinjection of GDNF and anti-GDNF antibody into the lateral ventricle of rats
The rats in each group were anesthetized intraperitoneally before each stimulation at days 15–17. Then, a stereotaxic apparatus was fixed to ensure that the anterior and posterior fontanelle were equally aligned. The hair on the roof of the skull was shaved, routine skin disinfection was performed, and a median incision of the skin was made on the skull roof, to a length of 0.8 cm. The anterior fontanelle was exposed according to George Paxinos and Charles Watson’s atlas and was used as the reference point. The lateral ventricle [anterior-posterior (AP) −1.5 mm, right-left (RL) 1.5 mm, height (H) 3.5 mm] was positioned, and a dental drill was used to drill the skull at AP −1.5 mm, RL 1.5 mm. A micro sample injector was used to reach a depth of 3.5 mm below the skull and was kept inserted for 3 min. The control +normal saline (NS) and asthma + NS group rats were received NS microinjection (16 µL). The asthma + GDNF group rats received exogenous GDNF (1 µg/mL, 16 µL, Sigma Aldrich, St. Louis, MO, USA) microinjection, whereas the asthma + GDNF group rats received anti-GDNF antibody (1:50, 16 µL, Abcam) microinjection. A microinjector was utilized for a 7-min injection with at a constant rate (0.014 µL/min). After injection, the microinjector was kept inserted for 5 min before being rapidly pulled out. Then, the skin was sutured, and the incised skin was disinfected again. The rats were fed in a single cage after suture. On the day 19, pulmonary function was examined, and the levels of interferon-γ (IFN-γ) and interleukin-4 (IL-4) in the BALF of rats were detected.
Detection of IFN-γ and IL-4 levels in BALF
After the measurement of pulmonary function, BALF was collected as previously described and placed in a centrifuge tube in a 4 ℃ water bath. Then, BALF was centrifuged for 10 min in a 4 ℃ centrifugal center at 1,500 rpm. The supernatant at −20 ℃ was cryopreserved, and IFN-γ and IL-4 levels were tested by ELISA.
Statistical analysis
All the data were expressed as the mean ± standard deviation (SD), and analyzed using SPSS 18.0 software (IBM Corp., Chicago, IL, USA). One-way analysis of variance (ANOVA) was used in comparisons among multiple groups. A P value <0.05 was considered statistically significant.
Results
Features of pulmonary function and airway inflammation in asthmatic rats
Following a 5-min inhalation, asthma symptoms were observed in the rats. The symptoms peaked approximately 15 min into the episode and lasted 30 min. There was also cyanosis and limp limbs in severe cases. Among asthmatic rats, inflammation was observed in the airway, as well as a significant increase in eosinophils in BALF (15.54±3.68 in the asthma group vs. 6.11±2.03 in the control group, P<0.01) (Figure 1A,1B). There was no evidence of bronchial, alveolar, or pulmonary interstitial damage in the control rats’ lung tissue sections, and the epithelium was neatly organized in these sections.
After the asthma attack, the respiration frequency (RF) increased (121.14±10.53 vs. 101.86±9.23 n/min, P<0.01), and the tidal volume (VT) (0.57±0.18 vs. 0.91±0.21 mL, maximum voluntary ventilation (MVV) (78.44±8.32 vs. 108.56±11.69 mL/min) of rats decreased significantly (P<0.01, respectively). Over the course of about 30 min, the rats breathing was shallow and fast. Compared with the control rats, the expiratory time course/inspiratory time course ratio (TE/TI) (2.67±0.65 vs. 1.62±0.35), and airway resistance (Raw) (0.51±0.06 vs. 0.32±0.03 cmH2O·mL/s) increased (P<0.01, respectively), but dynamic pulmonary compliance (Cdyn) decreased (0.39±0.03 vs. 0.54±0.04 mL/cmH2O, P<0.01) (Figure 1C,1D).
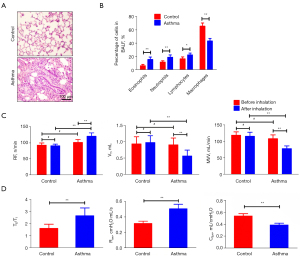
GDNF expressions in the airway
GDNF staining in the airway of asthmatic rats showed a high number of GDNF-positive substances (brown staining) around the bronchus (Figure 2A), but a low number was observed in the control group. The number of GDNF-positive cells in the asthma group was more than that in the control group (208.54±37.68 vs. 14.11±5.33, P<0.001) (Figure 2B). The level of GDNF in rat BALF was tested by ELISA. The GDNF content in the BALF of asthmatic rats was higher than that in the control group (82.45±12.68 vs. 67.45±6.19 pg/mL, P<0.01) (Figure 2C). The data showed during asthma attack, GDNF expressions were increased.
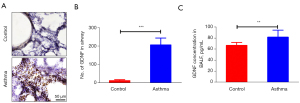
GDNF expressions in the brain
GDNF-positive substances (yellow staining) could be observed in multiple brain regions in the asthmatic rats, including medial amygdala (MeA), paraventricular nucleus (PVN), cortex, and nucleus of solitary tract (NTS), but few could be found the control rats (Figure 3A-3D). This indicated that asthma attack could induce the GDNF expressions in the brain.
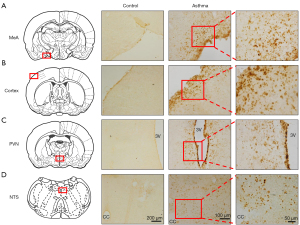
Effects of GDNF on pulmonary function in asthmatic rats
After microinjection into lateral ventricle (Figure 4A), compared with the asthma + NS group, the RF, TE/TI, and Raw increased in the asthma + GDNF group (P<0.01, respectively), whereas MVV and Cdyn decreased (P<0.01, respectively). Following administration with anti-GDNF, the indicators above improved (P<0.01, respectively) (Figure 4B,4C).
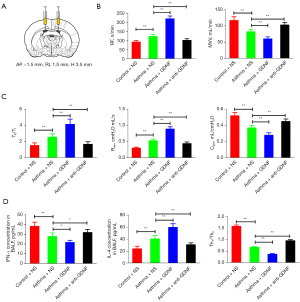
The results showed that injecting a specific dose of GDNF into the lateral ventricle could exacerbate the symptoms of asthma, whereas injection of anti-GDNF antibody could relieve the symptoms of asthma.
Effects of GDNF on inflammatory mediators in BALF
ELISA determination of IFN-γ and IL-4 levels in BALF showed that compared with asthma + NS group rats, the IFN-γ content (21.95±2.21 vs. 28.01±3.68 pg/mL) and Th1/Th2 ratio (0.37±0.03 vs. 0.67±0.03) significantly reduced (P<0.05, P<0.01, respectively), whereas IL-4 content (59.95±6.19 vs. 40.79±5.61 pg/mL) increased significantly (P<0.01) in the asthma + GDNF groups. Following treatment with anti-GDNF, the levels of the IFN-γ (32.14±3.23 pg/mL) and IL-4 (31.03±3.15 pg/mL), and Th1/Th2 ratio (0.95±0.05) were improved (P<0.01, respectively) (Figure 4D).
The results indicated that Th1/Th2 imbalance may become more pronounced after GDNF injection into the lateral ventricle of asthmatic rats, and could be corrected to a certain extent after injection of GDNF antibody.
Discussion
During asthma attack, vagal afferent fibers are the main routes through which various stimuli, such as peripheral airway inflammation information and immune information, are transmitted to the brain (13). Two types of nerve fibers present in the vagal C fibers and Aδ fibers in the afferent nerves. One type is the peptidergic afferent nerve, which is sensitive to nerve growth factor (NGF) and expresses substance P (SP) and calcitonin gene-related peptide (CGRP), with the cell bodies mainly existing in the nodose ganglion. The other nerve fiber is a non-peptide afferent nerve that is sensitive to GDNF and characteristically expresses the ligand-gated ion channel P2X3, with the neuron bodies mainly present in the jugular ganglion. Peptidergic afferent nerves include vagal afferent nerves, which were previously involved in asthma research. The release of SP and CGRP could cause typical symptoms of asthma. NGF is a key cytokine in several lung diseases (14), and it amplifies airway inflammation by connecting the nervous and immune systems (15). In addition to inducing changes of neural plasticity of peptidergic afferent nerves, the persistent state of asthma also causes nerve function change.
GDNF is a new subfamily in the TGF-β family which can promote nerve cell function maintenance and injury repair, thus playing a role in neurological diseases. GDNF participates in the lung development,and is expressed in various lung tissues (including airway smooth muscle), and GDNF receptors are expressed on the vagal afferent nerves innervating the airway (16). It is shown that the persistent state of asthma can cause changes in non-peptidergic afferent neurons, which are sensitive to GDNF (17). This implies the relationship between GDNF and asthma, but the relationship between central sensitization and asthma is unclear.
This study revealed the increased GDNF expression in the lung tissues and high GDNF concentrations in BALF in asthmatic rats, meanwhile GDNF expressions were increased in multiple nuclei in the brain, suggesting abnormalities in these brain regions. By using resting-state functional magnetic resonance imaging (MRI), compared with the healthy participants, the voxel-wise degree centrality values were altered in asthma patients in several brain networks (18), and the hippocampus was shown to be significantly smaller in asthmatic patients compared to those without asthma (19). The function changes in multiple brains regions including the insula, anterior cingulate cortex would lead to asthma exacerbation (20). Previously, we reported that the activated neurons in PVN and MeA may participate the in central pathogenesis of asthma during asthma attack (21), and the amygdala might be related to the stress and anxiety of patients with asthma (22,23). The asthma attack is closely related to emotional disorder (24,25), which involves higher brain regions (26), and specific brain networks and brain regions are more active (27,28). The nucleus in the brainstem is a key site that regulates respiratory control (29,30), such as cough (31), and vagal nerves have been shown to be involved in modulation of the afferent signal (32,33). Therefore, we speculate that GDNF is a key mediator in both the CNS and peripheral nervous system during asthma. During an asthma attack, GDNF in the lungs acts on the corresponding receptors on non-peptidergic afferent nerves and information could be uploaded to the brain via non-peptidergic nerves. Brain responses are translated into peripheral immune inflammatory messages, including increased GDNF expression in the brain, which is involved in the inducement of a central sensitization state.
We performed GDNF exogenous intervention in asthmatic rats to observe pulmonary function. Imbalance of the inflammatory cytokine network plays an important role, where Th1/Th2 cytokine imbalance is thought to underlie the pathogenesis, with hypo-Th1 responses and hyper-Th2 responses. IFN-γ and IL-4 are at the center of the Th1/Th2 cytokine network, with the former being the classical Th1 cytokine and the latter being the classical Th2 cytokine. The imbalance between these cytokines causes abnormal production of immunoglobulin E (IgE) and asthma attack, that is, the predominant response to Th2 cells leads to a series of IgE-mediated allergic inflammation (34). Neurotrophins participate in the pathophysiological process of asthma, especially the airway inflammation, and it is necessary to evaluate whether neurotrophins and their receptors might serve as therapeutic targets (35).
Central sensitization refers to the increase of the reactivity of neurons, release of excitatory neurotransmitters (such as glutamate and SP), and/or synaptic efficacy and changes in the morphology and functions of brain glial cells. In summary, central sensitization of asthma occurs as follows: immuno-inflammatory information from the airway, mucous membranes, and smooth muscles is transmitted from the vagal afferent fibers to the brain, which produces inflammatory and immune reactions. These changes can affect the functional activity of neurons and glial cells in the brain, forming a central sensitization state. Immune sensitization information in the brain enhances the activity of airway mucosal glands or smooth muscles through efferent nerves that dominate the airways, which may further exacerbate asthma attacks. A study on the central sensitization mechanism of asthma have focused mainly on the afferent pathway (the way in which peripheral immune and inflammatory information is transmitted to the brain), formation of intracerebral sensitization states (changes in the brain during asthma), and the efferent regulatory pathway (the regulation of peripheral asthma after inducement of intracerebral sensitization states). With regard to the afferent pathway of peripheral immune inflammatory information, research results have shown that peripheral inflammatory information could be transmitted via the vagus nerve to the brain during an asthma attack and is one of the important ways for immune system-to-brain communication (36). The occurrence, development, and prolongation of asthma are regulated by the neuroendocrine immune networks, and these networks are regulated by the CNS, with the neural mechanism playing an important role in asthma attack (37).
Conclusions
Our findings suggest that GDNF injection into the lateral ventricle can significantly aggravate the symptoms of asthma, indicating that central GDNF is an important transmitter involved in asthma attacks.
Acknowledgments
Funding: This work was supported by the National Natural Science Foundation of China (Nos. 81900100 and 81903024), Key Research and Development Plan of Jiangsu Province (No. BE2022761), Scientific Research Project of Gusu School of Nanjing Medical University (No. GSKY20210245).
Footnote
Reporting Checklist: The authors have completed the ARRIVE reporting checklist. Available at https://atm.amegroups.com/article/view/10.21037/atm-22-6338/rc
Data Sharing Statement: Available at https://atm.amegroups.com/article/view/10.21037/atm-22-6338/dss
Conflicts of Interest: All authors have completed the ICMJE uniform disclosure form (available at https://atm.amegroups.com/article/view/10.21037/atm-22-6338/coif). The authors have no conflicts of interest to declare.
Ethical Statement: The authors are accountable for all aspects of the work in ensuring that questions related to the accuracy or integrity of any part of the work are appropriately investigated and resolved. Animal experiments were performed under a project license (No. 20180903) granted by the ethics board of the First People’s Hospital of Kunshan. All experimental procedures were conducted following the Guide for the Care and Use of Laboratory Animals, 8th edition.
Open Access Statement: This is an Open Access article distributed in accordance with the Creative Commons Attribution-NonCommercial-NoDerivs 4.0 International License (CC BY-NC-ND 4.0), which permits the non-commercial replication and distribution of the article with the strict proviso that no changes or edits are made and the original work is properly cited (including links to both the formal publication through the relevant DOI and the license). See: https://creativecommons.org/licenses/by-nc-nd/4.0/.
References
- Cao Y, Chen S, Chen X, et al. Global trends in the incidence and mortality of asthma from 1990 to 2019: An age-period-cohort analysis using the global burden of disease study 2019. Front Public Health 2022;10:1036674. [Crossref] [PubMed]
- Caminati M, Morais-Almeida M, Bleecker E, et al. Biologics and global burden of asthma: A worldwide portrait and a call for action. World Allergy Organ J 2021;14:100502. [Crossref] [PubMed]
- Ritz T, Kroll JL, Patel SV, et al. Central nervous system signatures of affect in asthma: associations with emotion-induced bronchoconstriction, airway inflammation, and asthma control. J Appl Physiol (1985) 2019;126:1725-36. [Crossref] [PubMed]
- Chatterjee J, Sanapala S, Cobb O, et al. Asthma reduces glioma formation by T cell decorin-mediated inhibition of microglia. Nat Commun 2021;12:7122. [Crossref] [PubMed]
- Pöyhönen S, Er S, Domanskyi A, et al. Effects of Neurotrophic Factors in Glial Cells in the Central Nervous System: Expression and Properties in Neurodegeneration and Injury. Front Physiol 2019;10:486. [Crossref] [PubMed]
- Roos BB, Teske JJ, Bhallamudi S, et al. Neurotrophin Regulation and Signaling in Airway Smooth Muscle. Adv Exp Med Biol 2021;1304:109-21. [Crossref] [PubMed]
- He D, Chen H, Zeng M, et al. Asthmatic Airway Vagal Hypertonia Involves Chloride Dyshomeostasis of Preganglionic Neurons in Rats. Front Neurosci 2020;14:31. [Crossref] [PubMed]
- Bhallamudi S, Roos BB, Teske JJ, et al. Glial-derived neurotrophic factor in human airway smooth muscle. J Cell Physiol 2021;236:8184-96. [Crossref] [PubMed]
- Undem BJ, Nassenstein C. Airway nerves and dyspnea associated with inflammatory airway disease. Respir Physiol Neurobiol 2009;167:36-44. [Crossref] [PubMed]
- Duarte Azevedo M, Sander S, Tenenbaum L. GDNF, A Neuron-Derived Factor Upregulated in Glial Cells during Disease. J Clin Med 2020;9:456. [Crossref] [PubMed]
- Baloh RH, Johnson JP, Avalos P, et al. Transplantation of human neural progenitor cells secreting GDNF into the spinal cord of patients with ALS: a phase 1/2a trial. Nat Med 2022;28:1813-22. [Crossref] [PubMed]
- Chen Z, Long L, Xiao J, et al. Activated Oxytocin Neurons in the PVN-DVC Pathway in Asthmatic Rats. Front Neuroanat 2020;14:47. [Crossref] [PubMed]
- Taylor-Clark TE, Undem BJ. Neural control of the lower airways: Role in cough and airway inflammatory disease. Handb Clin Neurol 2022;188:373-91. [Crossref] [PubMed]
- Liu P, Li S, Tang L. Nerve Growth Factor: A Potential Therapeutic Target for Lung Diseases. Int J Mol Sci 2021;22:9112. [Crossref] [PubMed]
- She W, Mei Z, Zhao H, et al. Nebulized Inhalation of Anti-Nerve Growth Factor Microspheres Inhibits Airway Remodeling in an Ovalbumin-Induced Rat Asthma Model. J Aerosol Med Pulm Drug Deliv 2019;32:70-7. [Crossref] [PubMed]
- Canning BJ, Spina D. Sensory nerves and airway irritability. Handb Exp Pharmacol 2009;194:139-83. [Crossref] [PubMed]
- McQuillan K, Lynch MA, Mills KH. Activation of mixed glia by Abeta-specific Th1 and Th17 cells and its regulation by Th2 cells. Brain Behav Immun 2010;24:598-607. [Crossref] [PubMed]
- Li QG, Zhou FQ, Huang X, et al. Alterations of resting-state functional network centrality in patients with asthma: evidence from a voxel-wise degree centrality analysis. Neuroreport 2018;29:1151-6. [Crossref] [PubMed]
- Carlson SM, Kim J, Khan DA, et al. Hippocampal volume in patients with asthma: Results from the Dallas Heart Study. J Asthma 2017;54:9-16. [Crossref] [PubMed]
- Vafaee F, Shirzad S, Shamsi F, et al. Neuroscience and treatment of asthma, new therapeutic strategies and future aspects. Life Sci 2022;292:120175. [Crossref] [PubMed]
- Chen Z, Liu NN, Xiao J, et al. The amygdala via the paraventricular nucleus regulates asthma attack in rats. CNS Neurosci Ther 2020;26:730-40. [Crossref] [PubMed]
- Ritz T, Kroll JL, Aslan S, et al. Subcortical gray matter volumes in asthma: associations with asthma duration, control, and anxiety. Brain Imaging Behav 2020;14:2341-50. [Crossref] [PubMed]
- Rosenkranz MA, Esnault S, Gresham L, et al. Role of amygdala in stress-induced upregulation of airway IL-1 signaling in asthma. Biol Psychol 2022;167:108226. [Crossref] [PubMed]
- Sipowicz K, Podlecka M, Mokros Ł, et al. The feeling of loneliness and the sense of meaning in life in patients with various levels of bronchial asthma control. J Asthma 2022; Epub ahead of print. [Crossref]
- Lin P, Li X, Liang Z, et al. Association between depression and mortality in persons with asthma: a population-based cohort study. Allergy Asthma Clin Immunol 2022;18:29. [Crossref] [PubMed]
- Antunes GL, Silveira JS, Luft C, et al. Airway inflammation induces anxiety-like behavior through neuroinflammatory, neurochemical, and neurometabolic changes in an allergic asthma model. Metab Brain Dis 2022;37:911-26. [Crossref] [PubMed]
- Gholami-Mahtaj L, Salimi M, Nazari M, et al. Asthma induces psychiatric impairments in association with default mode and salience networks alteration: A resting-state EEG study. Respir Physiol Neurobiol 2022;300:103870. [Crossref] [PubMed]
- Huang H, Li SY, Shi L, et al. Altered spontaneous brain activity in patients with asthma: a resting-state functional MRI study using regional homogeneity analysis. Neuroreport 2021;32:1403-7. [Crossref] [PubMed]
- Trevizan-Baú P, Dhingra RR, Furuya WI, et al. Forebrain projection neurons target functionally diverse respiratory control areas in the midbrain, pons, and medulla oblongata. J Comp Neurol 2021;529:2243-64. [Crossref] [PubMed]
- Trevizan-Baú P, Furuya WI, Mazzone SB, et al. Reciprocal connectivity of the periaqueductal gray with the ponto-medullary respiratory network in rat. Brain Res 2021;1757:147255. [Crossref] [PubMed]
- Chen Z, Lin MT, Zhan C, et al. A descending pathway emanating from the periaqueductal gray mediates the development of cough-like hypersensitivity. iScience 2022;25:103641. [Crossref] [PubMed]
- Mazzone SB, Undem BJ. Vagal Afferent Innervation of the Airways in Health and Disease. Physiol Rev 2016;96:975-1024. [Crossref] [PubMed]
- Behrens R, McGovern AE, Farrell MJ, et al. Mini Review: Central Organization of Airway Afferent Nerve Circuits. Neurosci Lett 2021;744:135604. [Crossref] [PubMed]
- Ngoc PL, Gold DR, Tzianabos AO, et al. Cytokines, allergy, and asthma. Curr Opin Allergy Clin Immunol 2005;5:161-6. [Crossref] [PubMed]
- Barrios J, Ai X. Neurotrophins in Asthma. Curr Allergy Asthma Rep 2018;18:10. [Crossref] [PubMed]
- Wrona D. Neural-immune interactions: an integrative view of the bidirectional relationship between the brain and immune systems. J Neuroimmunol 2006;172:38-58. [Crossref] [PubMed]
- Audrit KJ, Delventhal L, Aydin Ö, et al. The nervous system of airways and its remodeling in inflammatory lung diseases. Cell Tissue Res 2017;367:571-90. [Crossref] [PubMed]
(English Language Editor: J. Jones)