Abstract
Ti6Al4V alloy samples with large pores suitable for bone implants are fabricated by pressing and sintering. Ti6Al4V powder is mixed with different volume fractions of salt particles. The sintering behavior up to 1260 °C is studied by dilatometry and pore features are observed by scanning electron microscopy and X-ray microtomography. Sintered materials with a relative density between 0.26 and 0.97 are obtained. 3D image analysis proves that large pores form a connected network when the amount of salt is 20% and above. The Young’s modulus and the yield stress of sintered materials deduced from compression tests span over wide ranges of values, which are consistent with real bone data. A simple analytical model is proposed to estimate the relative density as a function of the fraction of salt. This model combined with classical Gibson and Ashby’s power equations for mechanical properties can predict the fraction of salt required to obtain prescribed properties.








Similar content being viewed by others
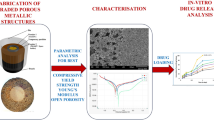
References
M.M. Dewidar, K.A. Khalil, and J.K. Lim: Processing and mechanical properties of porous 316L stainless steel for biomedical applications. Trans. Nonferrous Metals Soc. 17, 468 (2007).
S. Bender, V. Chalivendra, N. Rahbar, and S. El Wakil: Mechanical characterization and modeling of graded porous stainless steel specimens for possible bone implant applications. Int. J. Eng. Sci. 53, 67 (2012).
M. Eriksson, M. Andersson, E. Adolfsson, and E. Carlström: Titanium–hydroxyapatite composite biomaterial for dental implants. Powder Metall. 49, 70 (2006).
H.J. Rack and J. Qazi: Titanium alloys for biomedical applications. Mater. Sci. Eng., C 26, 1269 (2006).
Z. Oksiuta, J.R. Dabrowski, and A. Olszyna: Co–Cr–Mo-based composite reinforced with bioactive glass. J. Mater. Process. Technol. 209, 978 (2009).
M. Dourandish, D. Godlinski, A. Simchi, and V. Firouzdor: Sintering of biocompatible P/M Co–Cr–Mo alloy (F-75) for fabrication of porosity-graded composite structures. Mater. Sci. Eng., A 472, 338 (2008).
K. Crosby: Titanium–6Aluminum–4Vanadium for functionally graded orthopedic implant applications. Doct. Diss. 218, 1 (2013).
M. Long and H. Rack: Titanium alloys in total joint replacement—A materials science perspective. Biomaterials 19, 1621–1639 (1998).
M. Bahraminasab, B.B. Sahari, K.L. Edwards, F. Farahmand, M. Arumugam, and T.S. Hong: Aseptic loosening of femoral components—A review of current and future trends in materials used. Mater. Des. 42, 459 (2012).
M. Niinomi and M. Nakai: Titanium-based biomaterials for preventing stress shielding between implant devices and bone. Int. J. Biomater. 2011, 10 (2011).
B.J. Moyen, P.J. Lahey, E.H. Weinberg, and W.H. Harris: Effects on intact femora of dogs of the application and removal of metal plates. A metabolic and structural study comparing stiffer and more flexible plates. J. Bone Jt. Surg., Am. 60A, 940 (1978).
X. Wang, S. Xu, S. Zhou, W. Xu, M. Leary, P. Choong, and Y.M. Xie: Topological design and additive manufacturing of porous metals for bone scaffolds and orthopaedic implants: A review. Biomaterials 83, 127 (2016).
L.J. Gibson and M.F. Ashby: Cellular Solids: Structure and Properties, 2nd ed., Vol. 175 (Cambridge University Press, Cambridge, United Kingdom, 1999).
H. Shen and L.C. Brinson: A numerical investigation of porous titanium as orthopedic implant material. Mech. Mater. 43, 420 (2011).
V. Karageorgiou and D. Kaplan: Porosity of 3D biomaterial scaffolds and osteogenesis. Biomaterials 26, 5474 (2005).
M. Takemoto, S. Fujibayashi, M. Neo, J. Suzuki, T. Kokubo, and T. Nakamura: Mechanical properties and osteoconductivity of porous bioactive titanium. Biomaterials 26, 6014 (2005).
I.H. Oh, N. Nomura, N. Masahashi, and S. Hanada: Mechanical properties of porous titanium compacts prepared by powder sintering. Scr. Mater. 49, 1197 (2003).
Y. Chino and D.C. Dunand: Directionally freeze-cast titanium foam with aligned, elongated pores. Acta Mater. 56, 105 (2008).
F. Li, J. Li, T. Huang, H. Kou, and L. Zhou: Compression fatigue behavior and failure mechanism of porous titanium for biomedical applications. J. Mech. Behav. Biomed. Mater. 65, 814 (2017).
N.W. Hrabe, P. Heinl, B. Flinn, C. Körner, and R.K. Bordia: Compression-compression fatigue of selective electron beam melted cellular titanium (Ti–6Al–4V). J. Biomed. Mater. Res., Part B 99, 313 (2011).
X.Y. Cheng, S.J. Li, L.E. Murr, Z.B. Zhang, Y.L. Hao, R. Yang, F. Medina, and R.B. Wicker: Compression deformation behavior of Ti–6Al–4V alloy with cellular structures fabricated by electron beam melting. J. Mech. Behav. Biomed. Mater. 16, 153 (2012).
J. Parthasarathy, B. Starly, S. Raman, and A. Christensen: Mechanical evaluation of porous titanium (Ti6Al4V) structures with electron beam melting (EBM). J. Mech. Behav. Biomed. Mater. 3, 249 (2010).
Y.J. Liu, S.J. Li, H.L. Wang, W.T. Hou, Y.L. Hao, R. Yang, T.B. Sercombe, and L.C. Zhang: Microstructure, defects and mechanical behavior of beta-type titanium porous structures manufactured by electron beam melting and selective laser melting. Acta Mater. 113, 56 (2016).
E. Sallica-Leva, R. Caram, A.L. Jardini, and J.B. Fogagnolo: Ductility improvement due to martensite α′ decomposition in porous Ti–6Al–4V parts produced by selective laser melting for orthopedic implants. J. Mech. Behav. Biomed. Mater. 54, 149 (2016).
O. Scott-Emuakpor, C. Holycross, T. George, K. Knapp, and J. Beck: Fatigue and strength studies of titanium 6Al–4V fabricated by direct metal laser sintering. J. Eng. Gas Turbines Power 138, 022101 (2016).
B.V. Krishna, S. Bose, and A. Bandyopadhyay: Low stiffness porous Ti structures for load-bearing implants. Acta Biomater. 3, 997 (2007).
T. Furumoto, A. Koizumi, M.R. Alkahari, R. Anayama, A. Hosokawa, R. Tanaka, and T. Ueda: Permeability and strength of a porous metal structure fabricated by additive manufacturing. J. Mater. Process. Technol. 219, 10 (2015).
L. Reig, V. Amigó, D.J. Busquets, and J.A. Calero: Development of porous Ti6Al4V samples by microsphere sintering. J. Mater. Process. Technol. 212, 3 (2012).
Y. Torres, J.A. Rodríguez, S. Arias, M. Echeverry, S. Robledo, V. Amigo, and J.J. Pavón: Processing, characterization and biological testing of porous titanium obtained by space-holder technique. J. Mater. Sci. 47, 6565 (2012).
Y. Torres, J.J. Pavón, I. Nieto, and J.A. Rodríguez: Conventional powder metallurgy process and characterization of porous titanium for biomedical applications. Metall. Mater. Trans. B 42, 891 (2011).
D.J. Jorgensen and D.C. Dunand: Ti–6Al–4V with micro-and macropores produced by powder sintering and electrochemical dissolution of steel wires. Mater. Sci. Eng., A 527, 849 (2010).
S.M. Kalantari, H. Arabi, S. Mirdamadi, and S.A. Mirsalehi: Biocompatibility and compressive properties of Ti–6Al–4V scaffolds having Mg element. J. Mech. Behav. Biomed. Mater. 48, 183 (2015).
Y. Torres, S. Lascano, J. Bris, J. Pavón, and J.A. Rodriguez: Development of porous titanium for biomedical applications: A comparison between loose sintering and space-holder techniques. Mater. Sci. Eng., C 37, 148 (2014).
E.E. Aşık and Ş. Bor: Fatigue behavior of Ti–6Al–4V foams processed by magnesium space holder technique. Mater. Sci. Eng., A 621, 157 (2015).
H. Shang, A. Mohanram, and R.K. Bordia: Densification and microstructural evolution of hierarchically porous ceramics during sintering. J. Am. Ceram. Soc. 98, 3424 (2015).
L. Olmos, T. Takahashi, D. Bouvard, C.L. Martin, L. Salvo, D. Bellet, and M. Di Michiel: Analysing the sintering of heterogeneous powder structures by in situ microtomography. Philos. Mag. 89, 2949 (2009).
J. Serra: Image Analysis and Mathematical Morphology (Academic Press, London, 1982).
P. Babin, G. Della Valle, H. Chiron, P. Cloetens, J. Hoszowska, P. Pernot, A.L. Réguerre, L. Salvo, and R. Dendievel: Fast X-ray tomography analysis of bubble growth and foam setting during breadmaking. J. Cereal Sci. 43, 393 (2006).
L. Olmos, C.L. Martin, D. Bouvard, D. Bellet, and M. Di Michiel: Investigation of the sintering of heterogeneous powder systems by synchrotron microtomography and discrete element simulation. J. Am. Ceram. Soc. 92, 1492 (2009).
A. Vagnon, J.P. Rivière, J.M. Missiaen, D. Bellet, M. Di Michiel, C. Josserond, and D. Bouvard: 3D statistical analysis of a copper powder sintering observed in situ by synchrotron microtomography. Acta Mater. 56, 1084 (2008).
A. Marmottant, L. Salvo, C.L. Martin, and A. Mortensen: Coordination measurements in compacted NaCl irregular powders using X-ray microtomography. J. Eur. Ceram. Soc. 28, 2441 (2008).
K.K. Phani and S.K. Niyogi: Young’s modulus of porous brittle solids. J. Mater. Sci. 22, 257 (1987).
J. Kováčik: Correlation between Young’s modulus and porosity in porous materials. J. Mater. Sci. Lett. 18, 1007 (1999).
L.F. Nielsen: Elasticity and damping of porous materials and impregnated materials. J. Am. Ceram. Soc. 67, 93 (1984).
A. Bandyopadhyay, F. Espana, V.K. Balla, S. Bose, Y. Ohgami, and N.M. Davies: Influence of porosity on mechanical properties and in vivo response of Ti6Al4V implants. Acta Biomater. 6, 1640 (2010).
J.P. Li, P. Habibovic, M. van den Doel, C.E. Wilson, J.R. de Wijn, C.A. van Blitterswijk, and K. de Groot: Bone ingrowth in porous titanium implants produced by 3D fiber deposition. Biomaterials 28, 2810 (2007).
Y. Takahashi and Y. Tabata: Effect of the fiber diameter and porosity of non-woven PET fabrics on the osteogenic differentiation of mesenchymal stem cells. J. Biomater. Sci., Polym. Ed. 15, 41 (2004).
A.I. Itälä, H.O. Ylänen, C. Ekholm, K.H. Karlsson, and H.T. Aro: Pore diameter of more than 100 μm is not requisite for bone ingrowth in rabbits. J. Biomed. Mater. Res., Part A 58, 679 (2001).
J.C. Piemme: Titanium PM for Orthopedic Implants. World PM2016 Proceedings—Biomedical Applications. Manuscript refereed by Dr. José Manuel Martin (2016).
M. Grimm and J. Williams: Measurements of permeability in human calcaneal trabecular bone. J. Biomech. 30, 743 (1997).
E. Nauman, K. Fong, and T. Keaveny: Dependence of intertrabecular permeability on flow direction and anatomic site. Ann. Biomed. Eng. 27, 517 (1999).
C.E. Wen, Y. Yamada, K. Shimojima, Y. Chino, T. Asahina, and M. Mabuchi: Processing and mechanical properties of autogenous titanium implant materials. J. Mater. Sci. Mater. Med. 13, 397 (2002).
G. Gagg, E. Ghassemieh, and F.E. Wiria: Effects of sintering temperature on morphology and mechanical characteristics of 3D printed porous titanium used as dental implant. Mater. Sci. Eng., C 33, 3858 (2013).
S. Barui, S. Chatterjee, S. Mandal, A. Kumar, and B. Basu: Microstructure and compression properties of 3D powder printed Ti–6Al–4V scaffolds with designed porosity: Experimental and computational analysis. Mater. Sci. Eng., C 70, 812 (2017).
R. Singh, P.D. Lee, T.C. Lindley, R.J. Dashwood, E. Ferrie, and T. Imwinkelried: Characterization of the structure and permeability of titanium foams for spinal fusion devices. Acta Biomater. 5, 477 (2009).
Z. Zhang, D. Jones, S. Yue, P.D. Lee, J.R. Jones, C.J. Sutcliffe, and E. Jones: Hierarchical tailoring of strut architecture to control permeability of additive manufactured titanium implants. Mater. Sci. Eng., C 33, 4055 (2013).
J.F. Despois and A. Mortensen: Permeability of open-pore microcellular materials. Acta Mater. 53, 1381 (2005).
M.R. Dias, P.R. Fernandes, J.M. Guedes, and S.J. Hollister: Permeability analysis of scaffolds for bone tissue engineering. J. Biomech. 45, 938 (2012).
H.U. Camron, R.M. Pilliar, and I. Macnab: The rate of bone ingrowth into porous metal. J. Biomed. Mater. Res., Part A 10, 295 (1976).
J.D. Bobyn, R.M. Pilliar, H.U. Cameron, and G.C. Weatherly: The optimum pore size for the fixation of porous-surfaced metal implants by the ingrowth of bone. Clin. Orthop. Relat. Res. 150, 263 (1980).
ACKNOWLEDGMENTS
The authors would like to thank CIC of the UMSNH and ECOS M15P01 for the financial support and the facilities to develop this study. The Laboratory “Lumir” Geosciences of the UNAM, Juriquilla for the 3D image acquisition and processing is also acknowledged with thanks.
Author information
Authors and Affiliations
Corresponding author
Rights and permissions
About this article
Cite this article
Cabezas-Villa, J.L., Olmos, L., Bouvard, D. et al. Processing and properties of highly porous Ti6Al4V mimicking human bones. Journal of Materials Research 33, 650–661 (2018). https://doi.org/10.1557/jmr.2018.35
Received:
Accepted:
Published:
Issue Date:
DOI: https://doi.org/10.1557/jmr.2018.35