Abstract
This study investigated how air bubbles in media affect tablet dissolution in a flow-through cell system (USP 4) using disintegrating (USP prednisone) and non-disintegrating (USP salicylic acid) tablets. Cell hydrodynamics were studied using particle image velocimetry (PIV) and computational fluid dynamics (CFD). The PIV analysis showed periodic changes in the local flow corresponding to the discharge and suction of the pump cycles. The absence of prior deaeration induced small air bubbles in the media and lower maximum flow during the cycle, explaining the slower dissolution of the USP salicylic acid tablets. Bubbles, occurring during the USP prednisone tablets study, induced the transition of floating disintegrated particles towards the cell outlet, whereas the particles precipitated to form a white layer on the glass beads used in the study with prior deaeration. CFD analysis showed local flow variation in multiple positions of small (ID 12 mm) and large (ID 22.6 mm) cells, explaining the different rates of dissolution of prednisone tablet particles depending on their distribution. These results emphasize the importance of prior deaeration in dissolution studies using a flow-through system. Bubbles in the flow-through cell system affected tablet dissolution by reducing the area in contact with the media (wettability), lowering the maximum instantaneous flow (pressure buffering), and altering the position of disintegrated particles in the cell.










Similar content being viewed by others
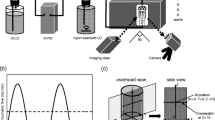
References
Fotaki N, Reppas C. The flow through cell methodology in the evaluation of intralumenal drug release characteristics. Dissolut Technol. 2005;12:17–21.
US Pharmacopeial Convention. USP 42-NF 37. Rockville, MD, USA: US Pharmacopeial Convention 2019. p. 6870–80.
Ministry of Health, Labour and Welfare. The Japanese Pharmacopoeia. 17th ed. 2016. p. 157–61.
European Directorate for the Quality of Medicines & HealthCare. European Pharmacopoeia 9.0. Strasbourg, France: Council of Europe; 2016. p. 302–9.
International Council for Harmonisation of Technical Requirements for Pharmaceuticals for Human Use (ICH). Quality guidelines. [cited 2021 6 July]; Available from: https://www.ich.org/page/quality-guidelines.
Kamba M, Seta Y, Takeda N, Hamaura T, Kusai A, Nakane H, Nishimura K. Measurement of agitation force in dissolution test and mechanical destructive force in disintegration test. Int J Pharm. 2003;250:99–109.
Morihara M, Aoyagi N, Kaniwa N, Katori N, Kojim S. Hydrodynamic flows around tablets in different pharmacopeial dissolution tests. Drug Dev Ind Pharm. 2002;28:655–62.
Moller H, Wirbitzki E. Regulatory aspects of modified release dosage forms: special cases of dissolution testing using the flow-through system. Boll Chim Farm. 1993;132:105–15.
Wennergren B, Lindberg J, Nicklasson M, Nilsson G, Nyberg G, Ahlgren R, Persson C, Palm B. A collaborative in vitro dissolution study: comparing the flow-through method with the USP paddle method using USP prednisone calibrator tablets. Int J Pharm. 1989;53:35–41.
Forrest WP, Reuter KG, Shah V, Kazakevich I, Heslinga M, Dudhat S, Patel S, Neri C, Mao Y. USP apparatus 4: a valuable in vitro tool to enable formulation development of long-acting parenteral (LAP) nanosuspension formulations of poorly water-soluble compounds. AAPS PharmSciTech. 2018;19:413–24. https://doi.org/10.1208/s12249-017-0842-x.
Tajiri T, Morita S, Sakamoto R, Mimura H, Ozaki Y, Reppas C, Kitamura S. Developing dissolution testing methodologies for extended-release oral dosage forms with supersaturating properties. Case example: solid dispersion matrix of indomethacin. Int J Pharm. 2015;490:368–74. https://doi.org/10.1016/j.ijpharm.2015.05.054.
Emara LH, Badr RM, Elbary AA. Improving the dissolution and bioavailability of nifedipine using solid dispersions and solubilizers. Drug Dev Ind Pharm. 2002;28:795–807. https://doi.org/10.1081/ddc-120005625.
Prieto-Escolar M, Torrado JJ, Álvarez C, Ruiz-Picazo A, Simón-Vázquez M, Govantes C, Frias J, García-Arieta A, Gonzalez-Alvarez I, Bermejo M. One and two-step in vitro-in vivo correlations based on USP IV dynamic dissolution applied to four sodium montelukast products. Pharmaceutics. 2021;13. https://doi.org/10.3390/pharmaceutics13050690.
Cheng X, Gao J, Li J, Cheng G, Zou M, Piao H. In vitro-in vivo correlation for solid dispersion of a poorly water-soluble drug efonidipine hydrochloride. AAPS PharmSciTech. 2020;21:160. https://doi.org/10.1208/s12249-020-01685-1.
Stefanič M, Locatelli I, Vrečer F, Sever T, Mrhar A, Bogataj M. The influence of gastric emptying kinetics on the drug release from enteric coated pellets in fasted state: an in vitro/in vivo correlation. Eur J Pharm Biopharm. 2012;82:376–82. https://doi.org/10.1016/j.ejpb.2012.07.011.
Okumu A, DiMaso M, Löbenberg R. Dynamic dissolution testing to establish in vitro/in vivo correlations for montelukast sodium, a poorly soluble drug. Pharm Res. 2008;25:2778–85. https://doi.org/10.1007/s11095-008-9642-z.
Looney TJ. USP apparatus 4 [flow through method] primer. Dissolut Technol. 1996;3:10–2.
May S, Jensen B, Wolkenhauer M, Schneider M, Lehr CM. Dissolution techniques for in vitro testing of dry powders for inhalation. Pharm Res. 2012;29:2157–66. https://doi.org/10.1007/s11095-012-0744-2.
Wallenwein CM, Nova MV, Janas C, Jablonka L, Gao GF, Thurn M, Albrecht V, Wiehe A, Wacker MG. A dialysis-based in vitro drug release assay to study dynamics of the drug-protein transfer of temoporfin liposomes. Eur J Pharm Biopharm. 2019;143:44–50. https://doi.org/10.1016/j.ejpb.2019.08.010.
Sievens-Figueroa L, Pandya N, Bhakay A, Keyvan G, Michniak-Kohn B, Bilgili E, Davé RN. Using USP I and USP IV for discriminating dissolution rates of nano- and microparticle-loaded pharmaceutical strip-films. AAPS PharmSciTech. 2012;13:1473–82. https://doi.org/10.1208/s12249-012-9875-3.
Eaton JW, Tran D, Hauck WW, Stippler ES. Development of a performance verification test for USP apparatus 4. Pharm Res. 2012;29:345–51.
Cammarn SR, Sakr A. Predicting dissolution via hydrodynamics: salicylic acid tablets in flow through cell dissolution. Int J Pharm. 2000;201:199–209.
Yoshida H, Kuwana A, Shibata H, Izutsu K, Goda Y. Effects of pump pulsation on hydrodynamic properties and dissolution profiles in flow-through dissolution systems (usp 4). Pharm Res. 2016;33:1327–36. https://doi.org/10.1007/s11095-016-1874-8.
Qureshi SA. Calibration—the USP dissolution apparatus suitability test. Drug Inf J. 1996;30:1055–61.
Rohrs BR, Steiner DJ. Deaeration techniques for dissolution media. Dissolut Technol. 1995;2:7–8.
Cox D, Douglas C, Furman W, Kirchhoefer R, Myrick J, Wells C. Guidelines for dissolution testing. Pharm Technol. 1978;2:16–53.
Nithyanandan P, Deng G, Brown W, Manning R, Wahab S. Evaluation of the sensitivity of USP prednisone tablets to dissolved gas in the dissolution medium using USP Apparatus 2. Dissolut Technol. 2006;13:15–8.
Gao Z, Moore TW, Doub WH, Westenberger BJ, Buhse LF. Effects of deaeration methods on dissolution testing in aqueous media: a study using a total dissolved gas pressure meter. J Pharm Sci. 2006;95:1606–13.
Degenhardt OS, Waters B, Rebelo-Cameirao A, Meyer A, Brunner H, Toltl NP. Comparison of the effectiveness of various deaeration techniques. Dissolut Technol. 2004;11:6–11.
Diebold SM, Dressman JB. Dissolved oxygen as a measure for de- and reaeration of aqueous media for dissolution testing. Dissolut Technol. 1998;5:13–6.
Krämer J, Stippler E. Experiences with USP apparatus 4 calibration. Dissolut Technol. 2005;12:33–9.
Beckett AH, Quach TT, Kurs GS. Improved hydrodynamics for apparatus 2. Dissolut Technol. 1996;3:7–18.
Friedel HD, Brown CK, Barker AR, Buhse LF, Keitel S, Kraemer J, Morris JM, Reppas C, Sperry DC, Sakai-Kato K, Stickelmeyer MP, Shah VP. FIP guidelines for dissolution testing of solid oral products. J Pharm Sci. 2018;107:2995–3002.
Lindauer RL. USP Dissolution Calibrator Tablets—Is It Time to Reduce the Amount of Testing Required to Calibrate Apparatus 1 and 2? Pharmacopeial Forum. 1995;21:1397–402.
Todaro V, Persoons T, Grove G, Healy AM, D’Arcy DM. Characterization and simulation of hydrodynamics in the paddle, basket and flow-through dissolution testing apparatuses - a review. Dissolut Technol. 2017;24:24–36.
Martinez AF, Sinha K, Nere N, Slade R, Castleberry S. Characterization of the hydrodynamics in the USP basket apparatus using computational fluid dynamics. J Pharm Sci. 2020;109:1231–41. https://doi.org/10.1016/j.xphs.2019.11.008.
Ameur H, Bouzit M. 3D hydrodynamics and shear rates’ variability in the United States Pharmacopeia Paddle Dissolution Apparatus. Int J Pharm. 2013;452:42–51. https://doi.org/10.1016/j.ijpharm.2013.04.049.
Perivilli S, Kakhi M, Stippler E. Computational fluid dynamics simulation of hydrodynamics in USP apparatus 3-the influence of dip rate. Pharm Res. 2015;32:1304–15. https://doi.org/10.1007/s11095-014-1534-9.
Kakhi M. Classification of the flow regimes in the flow-through cell. Eur J Pharm Sci. 2009;37:531–44.
Sirasitthichoke C, Perivilli S, Liddell MR, Armenante PM. Experimental determination of the velocity distribution in USP Apparatus 1 (basket apparatus) using Particle Image Velocimetry (PIV). Int J Pharm X. 2021;3:100078. https://doi.org/10.1016/j.ijpx.2021.100078.
Perivilli S, Prevost R, Stippler E. Velocity field visualization in USP dissolution apparatus 3 using particle image velocimetry. Pharm Res. 2017;34:1330–7. https://doi.org/10.1007/s11095-017-2151-1.
Bai G, Wang Y, Armenante PM. Velocity profiles and shear strain rate variability in the USP Dissolution Testing Apparatus 2 at different impeller agitation speeds. Int J Pharm. 2011;403:1–14.
Yoshida H, Kuwana A, Shibata H, Izutsu K, Goda Y. Particle image velocimetry evaluation of fluid flow profiles in USP 4 flow-through dissolution cells. Pharm Res. 2015;32:2950–9.
Baxter JL, Kukura J, Muzzio FJ. Hydrodynamics-induced variability in the USP apparatus II dissolution test. Int J Pharm. 2005;292:17–28.
Curley T, Forsyth R, Sun S, Fliszar K, Mitch C, Martin GP. Measurement of dissolved oxygen as a determination of media equilibrium during dissolution testing. Dissolut Technol. 2004;11:6–11.
Kakhi M. Mathematical modeling of the fluid dynamics in the flow-through cell. Int J Pharm. 2009;376:22–40.
McDonnell D, D’Arcy DM, Crane LJ, Redmond B. A mathematical analysis of drug dissolution in the USP flow through apparatus. Heat Mass Transf. 2018;54:793–801.
D’Arcy DM, Liu B, Corrigan OI. Investigating the effect of solubility and density gradients on local hydrodynamics and drug dissolution in the USP 4 dissolution apparatus. Int J Pharm. 2011;419:175–85.
D’Arcy DM, Liu B, Bradley G, Healy AM, Corrigan OI. Hydrodynamic and species transfer simulations in the USP 4 dissolution apparatus: considerations for dissolution in a low velocity pulsing flow. Pharm Res. 2010;27:246–58.
Funding
This work was partly supported by the Research on Regulatory Harmonization and Evaluation of Pharmaceuticals, Medical Devices, Regenerative and Cellular Therapy Products, Gene Therapy Products, and Cosmetics of the Japan Agency for Medical Research and Development (AMED) under Grant Number JP19mk0101130.
Author information
Authors and Affiliations
Corresponding author
Additional information
Publisher’s Note
Springer Nature remains neutral with regard to jurisdictional claims in published maps and institutional affiliations.
Supplementary Information
ESM 1
(PNG 227 kb)
Rights and permissions
About this article
Cite this article
Yoshida, H., Teruya, K., Abe, Y. et al. Altered Media Flow and Tablet Position as Factors of How Air Bubbles Affect Dissolution of Disintegrating and Non-disintegrating Tablets Using a USP 4 Flow-Through Cell Apparatus. AAPS PharmSciTech 22, 227 (2021). https://doi.org/10.1208/s12249-021-02117-4
Received:
Accepted:
Published:
DOI: https://doi.org/10.1208/s12249-021-02117-4