Abstract
More stomatocytes, spherocytes, and erythrocytes with spicules and protrusions are found in blood from newborn infants than in blood from adults. The objective of this study was to measure volume, surface area, membrane rigidity, and viscosity of red blood cells (RBC) with irregular shapes in preterm and full-term neonates. RBC from 13 full-term and 12 preterm (25-36 wk of gestation) infants were studied by means of a micropipette system. In full-term neonates and in preterm infants, 19 and 26% of RBC, respectively, showed shapes different from those of discocytes. Keratocytes, spherocytes, akanthocytes (in full-term infants), and elliptocytes (in preterm infants) had smaller volumes than did discocytes (p < 0.05). Spherocytes showed decreased and elliptocytes had increased excess surface area and swelling capacity compared with discocytes, indicating both volume and membrane loss for spherocytes and only volume loss for elliptocytes. Immature RBC and knizocytes had similar large volumes and surface areas, suggesting that knizocytes in neonates are relatively young RBC. Increased membrane rigidity was found only in stomatocytes. Membrane viscosity was decreased in immature RBC and knizocytes and increased in stomatocytes, keratocytes, akanthocytes, and elliptocytes when compared with discocytes. There were inverse relationships between RBC volume and membrane rigidity (r = -0.42) and between RBC volume and membrane viscosity (r = -0.72). This suggests that volume loss of neonatal RBC is associated with impaired membrane deformability. Moreover, we conclude that the geometry and membrane deformability of some of the irregularly shaped neonatal RBC resemble properties of RBC in hemolytic anemias (e.g. spherocytosis).
Similar content being viewed by others
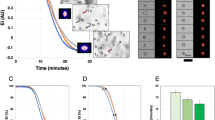
Main
RBC in the newborn infant show many variations in shape(1,2). Zipursky et al.(1) observed that only 39% of RBC in preterm infants and 43% of RBC in term neonates are discocytes (adults, 78%). In term neonates, 40% of RBC were stomatocytes or bowls (preterm, 29%; adults, 18%). The remainder of neonatal RBC were knizocytes, discocyte-bowls, keratocytes, echinocytes, acanthocytes, dacryocytes, schizocytes, spherocytes, and immature erythrocytes. Other groups observed smaller percentages of irregularly shaped RBC in neonates but confirmed the wide variation in cell shapes(2,3).
Irregular shapes may be associated with decreased RBC deformability(4) due to decreased (available) surface area or to altered membrane properties. We hypothesized that RBC with irregular shapes normally occurring in neonates may also show altered geometric (volume and surface area) and membrane mechanical (shear elastic modulus and V) properties. In the present investigation, geometric and mechanical properties of RBC with various shapes were studied by means of a micropipette system.
MATERIALS AND METHODS
Blood samples and RBC preparation. Placental blood samples from 12 healthy preterm infants (25-36 wk of gestation; mean, 31.0 ± 3.4 wk) and 13 healthy full-term neonates (38-42 wk of gestation) were studied with the approval of the Department of Pediatrics Human Subjects Committee and with informed consent of the mothers. Blood was collected from the placenta into EDTA immediately after cord clamping before delivery of the placenta. Infants with malformations, diabetic mothers, hemorrhage, or intrauterine asphyxia were excluded, as were infants with proven infection or high risk of infection. Blood type incompatibilities were excluded by determination of the blood types and the results of the Coombs' tests of the mothers and neonates. Hereditary erythrocyte disorders were unlikely in all neonates, because they had normal Hb concentration, erythroblast counts, and mean cell volume. None of the neonates developed bilirubin concentrations above 15 mg/dl, and none of the relatives had hereditary RBC disorders. Blood samples from 10 healthy, nonsmoking and nonobese male adults were taken by venipuncture into EDTA.
For the micropipette studies, a solution of PBS (Na2HPO4, 3.53 g/L; KH2PO4, 0.702 g/L; NaCl, 7.0725 g/L, pH 7.4, 295 mosmol/kg) with 5% of autologous plasma was prepared. Two microliters of whole blood was diluted into 2 mL of the PBS-plasma solution. Studies in our laboratory have shown that the addition of plasma decreases the formation of irregularly shaped RBC and prevents cell adhesion on glass surfaces. Moreover, we observed an increase in the number of irregularly shaped RBC when neonatal RBC were anticoagulated with heparin instead of EDTA. All measurements were obtained at room temperature (22 ± 1°C) and were completed within 2 h of blood sampling.
RBC studies in a micropipette system. The RBC were studied in a transparent chamber that allowed microscopic observation and manipulation of individual RBC by means of micropipettes. The micropipette system and the microscope-video system have been described previously(5,6). Two consecutive experiments were conducted for each blood sample to obtain the geometric and mechanical parameters. In the first experiment, unselected RBC were studied. For this experiment, the microscope desk was systematically moved horizontally and vertically and the next well-focused cell was aspirated. Inclusion of RBC in different distances from the bottom of the chamber is necessary because sedimentation of RBC may be influenced by their shape(7). Forty unselected RBC were studied in each neonate and adult. These experiments were used for the measurements of the mean values shown in Table 1 and for the evaluation of the distribution of RBC with various shapes (Table 2). The second experiment was conducted to study geometric and membrane properties of cells with various shapes. The RBC were allowed to sediment for approximately 20 min. The RBC to be tested was selected by its shape. The classification and nomenclature of erythrocytes was performed as described by Bessis(8). Figure 1 shows neonatal RBC with different shapes. The second experiment was not performed for adult RBC, because adult blood contained only few cells with irregular shapes.
Erythrocytes with different shapes from full-term and preterm neonates. Discocyte (A), stomatocyte (B), echinocyte (C), keratocyte (D), dacryocyte (E), knizocyte (F), immature RBC (G), akanthocyte (H), spherocyte (I), sphero-echinocyte (J), elliptocyte (K), and spherocyte partly aspirated into a micropipette (L) are shown.
RBC volume and surface area. Pipettes with internal radii (Rp) of 0.95 to 1.05 µm were used to aspirate membrane-cytoplasm tongues of RBC (Fig. 1). An aspiration pressure of -15 mm H2O was applied. One portion (tongue) of the cell entered the pipette assuming a cylindrical shape, whereas the outer portion became spherical. The partly aspirated RBC was video recorded. The tested RBC were aspirated for less than 2 s to prevent loss of water during the measurements. The volumes and surface areas of individual RBC were calculated from the cross section of the sphere outside the pipette, the length of the aspirated cylindrical tongue, and the pipette diameter(9). Volume and surface area were used to calculate the excess surface area, the swelling capacity, and the minimum cylindrical diameter(9). The excess surface area defines the membrane area beyond that required to enclose the cell volume, and the swelling capacity defines the maximal volume expansion (e.g. in hypotonic solution). A sphere has no excess surface area and no swelling capacity.
RBC membrane elastic modulus and viscosity. Pipettes with internal diameters of 1.4 to 1.6 µm were used for studies of membrane E and RBC recovery time from extensional deformation(6,10). Both measurements were obtained for the same RBC(6). The pipette was used to extend (overall extension ratio of approximately 1.6) and quickly release point-attached RBC. From video recordings of the recovery process, the cell length:width ratio was measured every 1/50 s for 20 fields after releasing the cell.
The E was then studied after the extended cell had recovered its initial shape(6). A small membrane tongue was aspirated into the pipette from a flat portion of the cell surface. Tongue length (LT) was measured from video recordings at a series of increasing aspiration pressures (P). The extensional shear elastic modulus (E) was calculated from a linear relationship that exists between LT/RP and P · RP over a LT/RP range between 1.5 and 3(10): LT/RP = (1/E) · P · RP.
For a given pipette radius, E defines the pressure required to produce a defined membrane tongue length. In other words, E determines RBC membrane deformation in response to a given force. A large E indicates high resistance to deformation and high membrane rigidity. The V was calculated as the product of the time constant for extensional shape recovery and membrane rigidity(6,10). Because we used different pipettes for the measurements of geometric and membrane mechanical parameters, we could not study geometric and mechanical parameters for the same RBC.
Statistical analyses. Statistical analyses were conducted to test for differences of the measured parameters among preterm infants, full-term neonates, and adults. Means and standard deviations were calculated for the entire cell populations studied in each group. The differently shaped RBC were compared with the discocytes of the same blood sample. If Kruskal-Wallis nonparametric analysis of variance led to rejection of the null hypothesis, Dunn's test was used to analyze which groups showed significant differences between the values of preterm infants, full-term neonates, and adults (Table 1) and between discocytes and RBC with other shapes (Table 2).
RESULTS
The measured and calculated geometric and membrane properties of unselected RBC in full-term and preterm neonates and adults are shown in Table 1. Preterm neonates showed larger RBC volumes and surface areas than did full-term neonates. The excess surface area and the swelling capacity were similar in the three groups. The minimum cylindrical diameter of neonatal RBC was also similar in preterm and full-term neonates but larger than in adults. The results indicate that the average neonatal RBC can enter cylindrical tubes with an internal diameter of 3.8 µm and more, whereas the average adult RBC can enter tubes with diameters of 3.4 µm and more.
The RBC membrane E was lower in the preterm infants than in the full-term neonates and lower in the full-term infants than in the adults. The time constant for extensional recovery of RBC was higher in neonates than in adults (Table 1). The RBC V (calculated as the product of E times the time constant) was similar in the three groups.
Distribution of RBC with various shapes was studied for unselected RBC. Preterm infants had less discocytes and more stomatocytes, keratocytes, knizocytes, dacryocytes, spherocytes, sphero-echinocytes, and elliptocytes than did full-term infants (Table 2). Adult blood contained only few RBC with irregular shapes; 97% were discocytes.
In full-term and preterm infants, stomatocytes, echinocytes, and dacryocytes showed volumes and surface areas similar to those of discocytes (Table 2). Spherocytes and keratocytes were smaller in full-term infants and elliptocytes in preterm infants when compared with discocytes. Knizocytes and immature RBC had similar volume and surface area in both groups but were larger than discocytes in full-term infants. Spherocytes and sphero-echinocytes showed decreased whereas dacryocytes and elliptocytes had increased excess surface area and swelling capacity than discocytes.
Increased membrane rigidity was found in stomatocytes from full-term and preterm infants when compared with discocytes (Table 2). V was decreased in immature RBC and knizocytes and increased in stomatocytes, keratocytes, akanthocytes, and spherocytes when compared with discocytes. Dacryocytes showed decreased membrane rigidity and viscosity in full-term infants.
Overall regression equations were calculated for the mean values of the RBC subpopulations. RBC volume was positively related to surface area (r = 0.94) and inversely related to membrane rigidity (r = -0.42), time constant (r = -0.63), and V (r = -0.72) (Fig. 2). Surface area was inversely related to membrane rigidity (r = -0.51), time constant (r = -0.59), and V (r = -0.73). No relationship was found between the RBC membrane elastic modulus and the time constant (r = 0.07), suggesting that these two membrane parameters were independent factors of V.
Overall relationship between mean values of RBC volume and V. Circles, full-term neonates; squares, preterm infants; Aca, acanthocytes; D, discocytes; Dac, dacryocytes; Ech, echinocytes; Ell, elliptocytes; I, immature RBC; Ker, keratocytes; Kni, knizocytes; SE, sphero-echinocytes; Sph, spherocytes; and Sto, stomatocytes.
DISCUSSION
Circulating RBC need excess surface area to assume various shapes during their passage through narrow vessels. Our results indicate that the average neonatal and adult RBC had an excess surface area of approximately 24% (Table 1). The swelling capacity of RBC (e.g. in hypotonic solution) is usually assessed by the osmotic resistance(11). Similar swelling capacity of neonatal and adult RBC agrees with the finding of similar osmotic resistance of neonatal and adult RBC(2). However, neonatal RBC show a much wider range of osmotic resistances with distinct subpopulations of increased and decreased osmotic resistance(12). These subpopulations may represent RBC with decreased (spherocytes and spheroechinocytes) and increased (dacryocytes, elliptocytes) swelling capacity.
In agreement with previous studies, we observed smaller RBC membrane extensional elastic moduli (i.e. smaller membrane rigidity) in neonates than in adults(6) and smaller elastic moduli in RBC from preterm infants when compared with full-term neonates(13). The decreased membrane E indicates that the membrane of neonatal RBC deforms more in response to a given force when compared with adult RBC. The time constant for recovery from extensional deformation defines the ability of the RBC to recover its original shape after deformation(10). This time constant (tc) increases with rising V and decreasing membrane rigidity: tc = V/E. Studies on density-separated and hydrated RBC suggest that the V is mainly determined by the internal Hb concentration (MCHC)(6,10,11). Thus, similar V values in the average RBC from preterm infants, full-term neonates, and adults agree with similar MCHC(6,13).
We observed a high percentage of RBC with irregular shapes in full-term (19%) and preterm (26%) neonates. Zipursky et al.(1) observed that approximately 60% of neonatal RBC were nondiscocytic, whereas Matovcik and Mentzer(2) found that an average of 15% of neonatal RBC had nondiscocytic shapes. Zipursky et al.(1) studied glutaraldehyde fixed cells, whereas Matovcik and Mentzer(2) and we studied fresh unfixed RBC.
Approximately half of the neonatal RBC with irregular shapes were stomatocytes. Both stomatocytes and echinocytes are formed as a result of discongruity of the inner and outer RBC membrane leaflet. Expansion of the inner leaflet over the outer leaflet results in inward buckling of the membrane and stomatocytic shape, whereas expansion of the outer leaflet over the inner leaflet results in outward buckling of the membrane and formation of spicula(14). Because an expansion of one leaflet over the other of 1% is sufficient to produce buckling of the expanded leaflet, it is not surprising that we found normal surface area and volume in stomatocytes and echinocytes (Table 2). In hereditary stomatocytosis, the RBC volume may be 20 to 30% larger than in healthy children due to sodium and water influx(15).
Keratocytes and acanthocytes had smaller volume and higher V than did discocytes (Table 2). Keratocytes are observed in patients with hereditary keratocytosis(16), and acanthocytes are found in patients with abetalipoproteinemia or liver disorders(17,18). Keratocytes and acanthocytes are mainly found in the densest RBC fraction(16,17), suggesting that these cells are small and have a high MCHC. Increased MCHC may explain the increased V of keratocytes and acanthocytes observed in our study.
Spherocytes had less volume and surface area, smaller excess surface area and swelling capacity, and increased V when compared with discocytes (Table 2). Similar results have been shown for the densest neonatal RBC separated in a density gradient(6). In hereditary spherocytosis, RBC show decreased volume, surface area, excess surface area, and swelling capacity and membrane rigidity due to spectrin deficiency of the RBC membrane(19).
Elliptocytes in preterm infants had smaller volume but normal surface area and increased excess surface area and swelling capacity compared with discocytes (Table 2). In hereditary elliptocytosis, RBC volume is decreased and osmotic resistance is normal or decreased(20). We found normal membrane rigidity and viscosity in elliptocytes. In hereditary elliptocytosis, membrane rigidity and viscosity have been reported to be normal(19) and to be increased by more than 100%(21).
Dacryocytes had increased excess surface area and swelling capacity and (in full-term infants) decreased membrane rigidity and viscosity compared with discocytes (Table 2). Bessis(8) proposed that the teardrop of dacryocytes is formed in the spleen when a Heinz-body or inclusion is pulled away from the RBC. The membrane yields, and a permanent membrane extension is formed. The membrane of neonatal RBC is more fragile and less resistant to plastic deformation compared with adult RBC(22).
Immature RBC and discocytes in preterm infants had similar volumes and surface areas, whereas in full-term infants, immature RBC had a larger volume and surface area than did discocytes (Table 2). This suggests that circulating immature RBC in full-term infants lose surface area and volume during maturation, whereas in preterm infants, surface area and volume are maintained. The large volume and surface area of young RBC has previously been shown in full-term neonates and adults(2,6).
Knizocytes showed volume, surface area, and V similar to those of immature RBC in both full-term and preterm neonates. This suggests that knizocytes constitute a relatively young RBC population.
From these results, we conclude that in full-term neonates, keratocytes, acanthocytes, and spherocytes are smaller whereas immature RBC and knizocytes are larger than normal discocytes. In preterm infants, keratocytes, spherocytes, and elliptocytes were smaller than discocytes. We found a highly significant overall inverse relationship between the mean volume and V of the various RBC subpopulations (Fig. 2). Because the V is mainly determined by the MCHC(6,10,11), these data suggest that the small RBC had high MCHC (and density) due to water loss from the RBC. To evaluate this hypothesis, we reevaluated the video recordings of a previous study on density-separated RBC in full-term neonates(6). In the fraction containing the 3% most dense RBC, 26% were spherocytes, 21% stomatocytes, 19% discocytes, 9% keratocytes, and 7% acanthocytes. Immature RBC and knizocytes were not detected in this fraction. In the fraction containing the 3% least dense RBC, 58% were discocytes, 18% immature, 13% knizocytes, and 7% stomatocytes. Spherocytes, keratocytes, and acanthocytes were not observed in this fraction. This suggests that the increased V of spherocytes, keratocytes, and acanthocytes is indeed related to increased MCHC when compared with normal discocytes.
Studies on density-separated RBC suggest that the loss of membrane and volume and the resulting increase in MCHC and density are important steps in the aging process of RBC(6,11). Neonatal RBC appear to lose membrane and volume more rapidly than do adult RBC(2,6). This may be explained by various mechanisms as membrane loss by endocytosis(2), increased susceptibility of neonatal RBC proteins to oxidative damage(23), and decreased resistance to mechanical membrane fragmentation(6,22). Some of the irregular shapes of neonatal RBC may be a result of membrane and volume loss and may contribute to the shortened life span of neonatal RBC(24). Studies on the fragility and biochemical properties of neonatal RBC with various shapes may provide more insight into the causes and consequences of their formation.
Abbreviations
- E:
-
extensional elastic modulus
- MCHC:
-
mean cell Hb concentration
- RBC:
-
red blood cell
- V:
-
membrane viscosity
References
Zipursky A, Brown E, Palko J, Brown EJ 1983 The erythrocyte differential count in newborn infants. Am J Pediatr Hematol Oncol 5: 45–51.
Matovcik LM, Mentzer WC 1985 The membrane of the human neonatal red cell. Clin Haematol 14: 203–221.
Hovi LM, Siimes MA 1983 Red blood cell morphology in healthy full term newborns. Acta Paediatr Scand 72: 135–136.
Meiselman HJ 1981 Morphological determinants of red cell deformability. Scand J Clin Lab Invest 41( suppl 156): 27–34.
Ruef P, Bohler T, Linderkamp O 1991 Deformability and volume of neonatal and adult leukocytes. Pediatr Res 29: 128–132.
Linderkamp O, Friederichs E, Meiselman HJ 1993 Mechanical and geometrical properties of density-separated neonatal and adult erythrocytes. Pediatr Res 34: 688–693.
Reinhart WH, Singh A, Marchetti M, Straub PW 1992 The influence of erythrocyte shape on suspension viscosities. Eur J Clin Invest 22: 38–44.
Bessis, M 1973 Red cell shapes: An illustrated classification and its rationale. In: Bessis, M. Weed, R.I., Leblond, P.F.: Red cell shape. Physiology, Pathology, Ultrastructure. Springer, Heidelberg, New York, 1: 25
Linderkamp O, Wu PYK, Meiselman HJ 1983 Geometry of neonatal and adult red blood cells. Pediatr Res 17: 250–253.
Nash GB, Meiselman HJ 1991 Effect of dehydration on the viscoelastic behaviour of red cells. Blood Cells 17: 517–522.
Linderkamp O, Meiselman HJ 1982 Geometric, osmotic, and membrane mechanical properties of density-separated human red cells. Blood 59: 1121–1127.
Danon Y, Kleimann A, Danon D 1970 The osmotic fragility and density distribution of erythrocytes in the newborn. Acta Haematol 43: 242–247.
Linderkamp O, Kiau U, Ruef P 1997 Cellular and membrane deformability of red blood cells in preterm infants with and without growth retardation. Clin Hemorheol Microcirc 17: 279–283.
Schrier SL, Zachowski A, Devaux PF 1992 Mechanisms of amphipath-induced stomatocytosis in human erythrocytes. Blood 79: 782–786.
Eber SW, Lande WM, Iarocci TA, Mentzer WC, Höhn P, Wiley JS, Schröter W 1989 Hereditary stomatocytosis: consistent association with an integral membrane protein deficiency. Br J Haematol 72: 452–455.
Beutler E, West C, Tavassoli M, Grahn E 1980 The Woronets trait: a new familial erythrocyte anomaly. Blood Cells 6: 281–287.
Bessis M, Mohandas N, Feo C 1980 Automated ektacytometry: a new method of measuring red cell deformability and red cell indices. Blood Cells 6: 315–327.
Lange Y, Steck TL 1984 Mechanism of red blood cell acanthocytosis and echinocytosis in vivo. J Membr Biol 77: 153–159.
Waugh RE, Agre P 1988 Reductions of erythrocyte membrane viscoelastic coefficients reflect spectrin deficiencies in hereditary spherocytosis. J Clin Invest 81: 133–141.
Iarocci TA, Wagner GM, Mohandas N, Lane PA, Mentzer WC 1988 Hereditary poikilocytic anemia associated with the co-inheritance of two alpha spectrin abnormalities. Blood 71: 1390–1396.
Nash GB, Gratzer WB 1993 Structural determinants of the rigidity of the red cell membrane. Biorheology 30: 397–407.
Ruef P, Linderkamp O 1994 Plastic deformation of erythrocyte membrane in full-term neonates and adults. Clin Hemorheol 14: 659–662.
Bracci R, Martini G, Buonocore G, Talluri B, Berni S, Ottaviani MF, Picchi MP, Casini A 1988 Changes in erythrocyte properties during the first hours of life: electron spin resonance of reacting sulfhydryl groups. Pediatr Res 24: 391–395.
Bratteby LE, Garby L, Groth T, Schneider W, Wadman B 1968 Studies on erythrokinetics in infancy. XII. The mean life span and the life span frequency function of red blood cells formed during foetal life. Acta Paediatr Scand 57: 311–320.
Author information
Authors and Affiliations
Additional information
Supported by a grant from the Deutsche Forschungsgemeinschaft (Li 291).
Rights and permissions
About this article
Cite this article
Ruef, P., Linderkamp, O. Deformability and Geometry of Neonatal Erythrocytes with Irregular Shapes. Pediatr Res 45, 114–119 (1999). https://doi.org/10.1203/00006450-199901000-00019
Received:
Accepted:
Issue Date:
DOI: https://doi.org/10.1203/00006450-199901000-00019
This article is cited by
-
Single-cell evaluation of red blood cell bio-mechanical and nano-structural alterations upon chemically induced oxidative stress
Scientific Reports (2015)
-
Whole-blood viscosity in the neonate: effects of gestational age, hematocrit, mean corpuscular volume and umbilical cord milking
Journal of Perinatology (2014)