Abstract
The objective of this study was to evaluate positron emission tomography(PET) of [18F]fluorodexoyglucose (18FDG) uptake as a measure of neonatal acute lung injury. Inasmuch as intrapulmonary sequestration of neutrophils is a hallmark of acute lung injury, quantification of neutrophil activity using 18FDG may offer a novel, in vivo technique to examine the progression and resolution of this disease. Ten newborn piglets were studied; six received bronchoalveolar lavage followed by 4 h of high pressure ventilation to create acute lung injury. Four healthy piglets served as controls. 18FDG (0.8 mCi/kg; 29.6 MBq) was given i.v. and PET (ECAT 953/31, Siemens) was performed for 90 min. During PET, all animals were sedated, paralyzed, and ventilated to maintain normal blood gases. The time course of radioactivity in lung regions and in plasma was used to calculate the rate constant for the metabolic trapping of 18FDG in tissue according to the method of C. S. Patlak. Median 18FDG influx constants were significantly higher in injured piglets (0.0187 min-1) than in control piglets (0.0052 min-1) (p < 0.01). Moreover, consistent with the 18FDG uptake data, injured piglets had moderate to severe injury on lung histology whereas control piglets had only slight and focal histologic changes. We conclude that PET of 18FDG uptake is an accurate, readily repeatable in vivo measure of neonatal acute lung injury.
Similar content being viewed by others
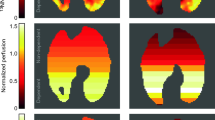
Main
Inflammation is a hallmark of acute lung injury(1). Intrapulmonary accumulation of neutrophils has been documented in animal models of acute lung injury(2, 3) as well as in patients with adult respiratory distress syndrome(4). In newborn infants, prolonged influx of neutrophils into the lungs during acute injury is associated with the development of pulmonary fibrosis or bronchopulmonary dysplasia(5–7).
It is well known that PET of 18FDG uptake can be used to differentiate benign from malignant pulmonary lesions, due to the increased glucose metabolism of malignant of malignant cells(8–11). More recently, PET of18 FDG has been validated as an in vivo technique to measure neutrophil activity in experimental Streptococcus pneumoniae-induced pneumonia and in bleomycin-induced lung inflammation(12). However, to date there have been no reports on the diagnostic accuracy of pulmonary 18FDG uptake in nonbacterial or nonchemical acute lung injury.
The present experiments were therefore designed to evaluate PET of18 FDG as a feasible and accurate measure of neonatal acute lung injury, induced by lung lavage and high pressure mechanical ventilation in newborn piglets.
METHODS
Animals. Ten Yorkshire piglets, less than 3 d old and of either sex, were obtained from a closed herd with excellent health status (Arkell Swine Research Center, University of Guelph, Ontario, Canada). All animal procedures were carried out in accordance with the guidelines of the Canadian Council on Animal Care and the regulations under the Province of Ontario Animals for Research Act. The experimental protocol was approved by the Animal Research Ethics Board at McMaster University.
Anesthesia and surgical preparation. Piglets were weighed and anesthetized with an intraperitoneal injection of sodium pentobarbital (30 mg/kg; MTC Pharmaceutics, Cambridge, Ontario, Canada). Endotracheal 3.5 Fr tubes (Concord/Portex, Keene, NH) were placed via tracheostomies and connected to an infant ventilator (model 102, Healthdyne Inc., Marietta, GA). Initial ventilator settings were chosen to ensure physiologic gas exchange: PIP of 1.5 kPa (15 cm H2O), PEEP of 0.3 kPa (3 cm H2O), IT of 0.5 s, RR of 30 breaths/min. A humidified gas mixture (warmed to 38 °C) of 40% oxygen in nitrogen was delivered at 8 L/min. A 3.5 Fr Argyle umbilical vessel catheter (Sherwood Medical, St. Louis, MO) was inserted into the left carotid artery. The catheter was secured at 5 cm, perfused with normal saline (1.5 mL/h; Baxter Corp., Toronto, Ontario, Canada), and used for blood sampling and continuous systemic blood pressure monitoring. The umbilical vein was cannulated with a second 3.5 Fr Argyle umbilical vessel catheter for the administration of maintenance fluids (5% dextrose at 80 mL kg-1·d-1; Baxter Corp., Toronto, Ontario, Canada), prophylactic ampicillin (100 mg·kg-1·d-1; Novopharm Ltd., Toronto, Ontario, Canada), and gentamicin (5 mg·kg-1·d-1; Schering Canada Inc., Pointe Claire, Quebec, Canada). To prevent tension pneumothorax, bilateral 10 Fr trochar catheters (Sherwood Medical, St. Louis, MO) were inserted into the chest, advanced 2 cm, and connected to Heimlich chest drain valves (Becton Dickinson Co., Lincoln Park, NJ). After these procedures, piglets were allowed to recover in prewarmed isolettes for 1 h.
Throughout the entire study period, the piglets were regularly monitored for maintenance of blood pressure, heart rate, and oxygen saturation (Ohmeda Biox 3700 Pulse Oximeter; Boc Health Care, Louisville, CO). A rectal ISC probe was inserted, and core body temperature was maintained at 39 °C. Additional sodium pentobarbital (16 mg/kg) was given i.v. as required to maintain adequate sedation. Intravenous pancuronium bromide (0.2 mg/kg; Abbott Laboratories Ltd., Sainte Laurent, Quebec, Canada) was administered at regular intervals to eliminate spontaneous breathing.
Experimental protocol. To induce lung injury, six piglets received bronchoalveolar lavage followed by 4 h of high pressure ventilation. Before bronchoalveolar lavage, Fio2 was increased to 1.0. Normal saline, 35 mL/kg, warmed to 37 °C, was instilled by gravity through the endotracheal tube and connecting tubing. The saline was allowed to dwell in the lungs for 5 s before it was recovered for 20 s by passive drainage. Approximately 80% of the instilled volume was retrieved. After each lavage, PIP was increased by 0.1 kPa (1 cm H2O) to compensate for decreases in compliance. Each piglet was lavaged six times at approximately 5-min intervals, to allow oxygen saturation (Sao2) to recover to values>90%. Three lavages were performed while the piglets were lying on the right side and three while lying on the left side.
High pressure ventilation was commenced immediately after the lavage procedure, using our previously published modifications(13) of the methods described initially by Tsuno et al.(14). PIP was increased to 3.9 kPa (40 cm H2O), and delivered at a flow rate of 15 L/min. PEEP was reduced to 0.2 kPa (2 cm H2O), RR was set at 20 breaths/min, whereas the IT was increased to 1.2 s. To maintain arterial CO2 tension (Paco2) between 3.3 and 4.0 kPa (25 and 30 torr), the dead space volume of the ventilator circuit was increased. Humidified pure oxygen was delivered at a rate of 15 L/min. During the 4 h period of mechanical ventilation at high peak pressures, the animals were nursed for 2 h each on either side.
At the end of the 4-h period of pulmonary overdistension, rescue ventilation was initiated. Ventilatory settings were modified as follows. PEEP was increased to 0.39 kPa (4 cm H2O), IT 0.5 s, and RR 30 breaths/min. The extra dead space was removed. PIP and Fio2 were adjusted to achieve normocarbia and normoxemia. All animals remained sedated and paralyzed.
Four piglets with no acute lung injury were used as controls. These animals were similarly instrumented, anesthetized, and mechanically ventilated, as described under “Anesthesia and Surgical Preparation” to maintain normal arterial blood gases.
Each piglet was transported to the Department of Nuclear Medicine for PET approximately 1 h after the initiation of rescue ventilation. During the 5-10 min transport, the animals were hand-bagged at the same pressures and frequency. The piglets were then transferred from the isolette into the PET scanner and were placed right-side down. To maintain a core body temperature of 39 °C, the animals were covered with a blanket, and the bed was equipped with a heating fan. After completion of the PET study, the piglets were sacrificed with an i.v. injection of a 3-mL bolus of sodium pentobarbital. At autopsy the animals were placed in a dorsal recumbent position, and a median sternotomy was performed. The heart and lungs were dissected free and removed en bloc.
Measurements. Lung function. Blood gases were measured in heparinized arterial samples (AVL 995 pH/Blood Gas Analyser, AVL Scientific Corporation, Roswell, GA). Before and after PET the ventilator efficiency index(15), and the ratio of arterial to alveolar oxygen tension (a/A ratio) were calculated from arterial blood gas data and concurrent ventilator settings.
Hematologic studies. Complete blood counts were performed at baseline in EDTA-anticoagulated blood samples using a Coulter counter (Coulter Electronics, Miami, FL).
Histologic examination. The left and right lungs were removed from the heart and trachea and were immersed in 10% buffered formalin for 72 h. Each lung was sliced, and samples were selected to provide a view of the lung from the hilum to the pleura. Sections cut at 5 μm were stained with hematoxylin/eosin or phosphotungstic acid/hematoxylin and were embedded in paraffin. An independent pathologist (D.d.S.), who was unaware of the experimental procedures, performed the histologic examination, using the scoring system described by Tsuno et al.(14). For the purposes of the present experiments, particular emphasis was placed on the following findings: alveolar macrophage proliferation, alveolar neutrophil infiltration, and hyaline membrane formation. These pathologic changes were graded as nil, slight, mild, moderate, or severe.
Pulmonary 18FDG uptake. PET was performed using an ECAT 953/31 (CTI/Siemens, Knoxville, TN) that allows the simultaneous examination of 31 overlapping slices. Images were reconstructed using a Hann filter with a cutoff frequency of 0.5. After a 30-min transmission scan (using a rotating rod source containing 68Ge) to correct for attenuation, piglets were given a bolus injection of 18FDG(0.8 mCi/kg; 29.7 MBq) via the umbilical venous catheter. Both lungs were scanned for 18 frames at 5 min/frame for a total of 90 min. Arterial blood samples (200 μL) were taken every minute for the first 5 min, at 7.5 and 10 min, and every 10 min thereafter. Radioactivity in these whole blood samples was counted in an automatic gamma counter, which was calibrated to the tomograph, and decay was corrected to the time of injection.
Regions of interest were defined interactively around both lungs. The time course of radioactivity was calculated for each region and, using the calibration factor between the automatic gamma counter and tomograph, expressed in the same units as the whole blood counts. Graphical evaluation of the time course of radioactivity in lung and in blood was performed using multiple-time tissue uptake data(16). This analysis, used to determine the rate of 18FDG uptake, has been rigorously derived, extended to models of any number of compartments, and found to be valid in situations where the input function is not constant(16, 17).
Statistical analysis. Data were summarized using medians or means ± SD, as appropriate. Differences in 18FDG influx constants between the two treatment groups were analyzed using Wilcoxon's rank sum test.
RESULTS
Experimental animals. A total of 10 piglets from five litters were studied. Six piglets were subjected to the lung injury protocol, and the remaining four piglets served as controls. The mean birth weights (SD) were comparable between injured and uninjured animals: 1433 (333) g and 1330 (174) g.
Lung function. Table 1 summarizes the mean(SD) values of a/A ratio and ventilator efficiency index by treatment group before and after PET.
Hematologic studies. Mean (SD) values for hemoglobin in injured and uninjured piglets were 78 (13) g/L and 65 (16) g/L, respectively. Mean(SD) values for total white blood cell counts in injured and uninjured piglets were as follows: 10.1 (2.9) × 109/L and 10.5 (0.8) × 109/L.
Histologic examination. The degree of histologic changes with respect to alveolar macrophage proliferation, alveolar neutrophil infiltration, and hyaline membrane formation is shown in Table 2. Figures 1 and 2 illustrate histologic changes that were found to be associated with the highest 18FDG influx constants, respectively, in the experimental(P2) and control group (P5).
Pulmonary 18FDG uptake.18 FDG influx constants are contrasted with lung histology scores in Table 2. Injured piglets had significantly higher18 FDG influx constants than uninjured piglets (p < 0.01).
Representative time courses of radioactivity in whole blood and in regions of interest around the lungs of an injured piglet (P10) after bolus injection of 18FDG are shown in Figure 3. Patlak plots for the lungs of the same piglet and its uninjured litter mate(P9) are shown in Figure 4.
DISCUSSION
Lung lavage and ventilation at high peak airway pressures are standard and pathophysiologically meaningful methods to induce experimental neonatal acute lung injury(14, 18). For the present study, we combined the two approaches to create an intense and lasting injury, which resulted in the histologic finding of moderate to severe hyaline membrane formation in all six piglets who underwent the lung injury protocol. Using this model, we have shown that pulmonary 18FDG uptake, measured by PET, was significantly higher in injured piglets than in control animals with healthy lungs who were mechanically ventilated only for sedation and muscle paralysis during PET.
18FDG differs from glucose only in the substitution of the hydroxyl group on the second carbon atom of glucose by a fluorine atom. This molecule is metabolized quantitatively like glucose until a point in the glycolytic pathway is reached where its structural dissimilarity prevents it from being metabolized further. 18FDG is transported by the same saturable carrier that transports glucose; in tissues it competes with glucose for hexokinase which phosphorylates both molecules to hexose 6-phosphates. However, unlike glucose 6-phosphate, fluorodeoxyglucose 6-phosphate cannot be isomerized to fructose 6-phosphate, and the tracer is effectively trapped in the tissues. This accumulation is in proportion to the flux of glucose through the entire pathway(19, 20).
The increased 18FDG signal, which was observed within 6-8 h of the initiation of the lung injury protocol, is due to rapid pulmonary sequestration of cells with high glucose metabolism such as neutrophils and macrophages(21). Using autoradiography of histologic sections after injection of [3H]deoxyglucose in experimental lung inflammation in rabbits, Jones et al.(12) have recently shown convincingly that deoxyglucose uptake was localized exclusively to neutrophils. Even in chronic inflammation, 2 wk after bleomycin challenge, when macrophages outnumbered neutrophils five to one, only neutrophils were labeled with [3H]deoxyglucose(12). The authors speculated that the increased18 FDG uptake during lung inflammation represents postmigratory neutrophil activity, and possibly the respiratory burst(12). In the present experiments, alveolar macrophage proliferation was consistently less evident on lung histology than alveolar neutrophil infiltration. This finding, together with the previous observations by Jones et al.(12) support our assumption that increased pulmonary 18FDG uptake after lung lavage and ventilation at high peak pressures was mostly, if not entirely, due to increased neutrophil activity.
Neutrophil sequestration is a characteristic feature of acute lung injury, irrespective of its etiology(1). In newborn infants, prolonged influx of neutrophils into the lungs during acute injury is associated with the development of bronchopulmonary dysplasia(5–7). Our present study suggests that PET of pulmonary 18FDG uptake may prove a valuable and readily repeatable research tool to study in vivo the progression and resolution of abnormal neutrophil activity during lung injury and to monitor the efficacy of antiinflammatory interventions. Pulmonary 18FDG uptake has so far only been used in humans to measure disease activity in two chronic inflammatory conditions, cryptogenic fibrosing alveolitis(22) and sarcoidosis(23).
Finally, pulmonary 18FDG uptake measured by PEt could also prove useful in testing the hypothesis that neutrophils may be responsible for intrapulmonary oxygen consumption during acute lung injury. The possibility that oxygen consumption occurs in inflamed lungs has previously been suggested by several investigators for conditions such as experimental pneumonia, tuberculosis, or lung cancer(24–26). Intrapulmonary oxygen consumption may contribute to the increased work of breathing during neonatal acute lung injury, and possibly also during bronchopulmonary dysplasia(27). Pulmonary 18FDG uptake allows the measurement of intrapulmonary neutrophil activity, which should be correlated in future studies with intrapulmonary oxygen consumption during neonatal lung injury.
Abbreviations
- PET:
-
positron emission tomography
- 18FDG:
-
[18F]fluorodeoxyglucose
- PIP:
-
peak inspiratory pressure
- PEEP:
-
positive end-expiratory pressure
- IT:
-
inspiratory time
- RR:
-
repiratory rate
- Fio2:
-
fractional concentration of inspired oxygen
REFERENCES
Bernard GR, Artigas A, Brigham KL, Carlet J, Falke K, Hudson L, Lamy M, Legall JR, Morris A, Spragg R and the Consensus Committee 1994 The American-European consensus conference on ARDS. Am J Respir Crit Care Med 149: 818–824.
Barry BE, Crapo JD 1985 Patterns of accumulation of platelets and neutrophils in rat lungs during exposure to 100% and 85% oxygen. Am Rev Respir Dis 132: 548–555.
Jackson JC, Chi EY, Wilson CB, Truog WE, Teh EC, Hodson WA 1987 Sequence of inflammatory cell migration into lung during recovery from hyaline membrane disease in premature newborn monkeys. Am Rev Respir Dis 135: 937–940.
Warshawski FJ, Sibbald WJ, Driedger AA, Cheung H 1986 Abnormal neutrophilpulmonary interaction in the adult respiratory distress syndrome. Am Rev Respir Dis 133: 797–804.
Merritt TA, Stuard ID, Puccia J, Wood B, Edwards DK, Finkelstein J, Shapiro DL 1981 Newborn tracheal aspirate cytology: classification during respiratory distress syndrome and bronchopulmonary dysplasia. J Pediatr 98: 949–956.
Ogden BE, Murphy SA, Saunders GC, Pathak D, Johnson JD 1984 Neonatal lung neutrophils and elastase/proteinase inhibitor imbalance. Am Rev Respir Dis 130: 817–821.
Watterberg KL, Carmichael DF, Gerdes JS, Werner S, Backstrom C, Murphy S 1994 Secretory leukocyte protease inhibitor and lung inflammation in developing bronchopulmonary dysplasia. J Pediatr 125: 264–269.
Nolop KB, Rhodes CG, Brudin LH, Beaney RP, Krausz T, Jones T, Hughes JMB 1987 Glucose utilization in vivo by human pulmonary neoplasms. Cancer 60: 2682–2689.
Rege SD, Hoh CK, Glaspy JA, Aberle DR, Dahlbom M, Razavi MK, Phelps ME, Hawkins RA 1993 Imaging of pulmonary mass lesions with whole-body positron emission tomography and fluorodeoxyglucose. Cancer 72: 82–90.
Dewan NA, Reeb SD, Gupta NC, Gobar LS, Scott WJ 1995 PET-FDG imaging and transthoracic needle lung aspiration biopsy in evaluation of pulmonary lesions. A comparative risk-benefit analysis. Chest 108: 441–446.
Sazon DAD, Santiago SM, Soo Hoo GW, Khonsary A, Brown C, Mandelkern M, Blahd W, Williams AJ 1996 Fluorodeoxyglucose-positron emission tomography in the detection and staging of lung cancer. Am J Respir Crit Care Med 153: 417–421.
Jones HA, Clark RJ, Rhodes CG, Schofield JB, Krausz T, Haslett C 1994 In vivo measurement of neutrophil activity in experimental lung inflammation. Am J Respir Crit Care Med 149: 1635–1639.
Schmidt B, Davis P, La Pointe H, Monkman S, Coates G, deSa D 1996 Thrombin inhibitors reduce intrapulmonary accumulation of fibrinogen and procoagulant activity of bonchoalveolar lavage fluid during acute lung injury induced by pulmonary overdistension in newborn piglets. Pediatr Res 39: 798–804.
Tsuno K, Miura K, Takeya M, Kolobow T, Morioka T 1991 Histopathologic pulmonary changes from mechanical ventilation at high peak airway pressures. Am Rev Respir Dis 143: 1115–1120.
Notter RH, Egan EA, Kwong MS, Holm BA, Shapiro DL 1985 Lung surfactant replacement in premature lambs with extracted lipids from bovine lung lavage: effect of dose, dispersion technique and gestational age. Pediatr Res 19: 567–577.
Patlak CS, Blasberg RG, Fenstermacher JD 1983 Graphical evaluation of blood-to-brain transfer constants from multiple-time uptake data. J Cereb Blood Flow Metab 3: 1–7.
Patlak CS, Blasberg RG 1985 Graphical evaluation of blood-to-brain transfer constants from multiple-time uptake data. Generalizations. J Cereb Blood Flow Metab 5: 584–590.
Lachman B, Robertson B, Vogel J 1980 In vivo lung lavage as an experimental model of the respiratory distress syndrome. Acta Anaesthiol Scand 24: 231–236.
Sokoloff L, Reivich M, Kennedy C, Des Rosiers MH, Patlak CS, Pettigrew KD, Sakurada O, Shinohara M 1977 The [C-14]deoxyglucose method for the measurement of local cerebral glucose utilization: theory, procedure and normal values in the conscious and anesthetized albino rat. J Neurochem 28: 897–916.
Phelps ME, Huang SC, Hoffman EJ, Selin C, Sokoloff L, Kuhl DE 1979 Tomographic measurement of local cerebral glucose metabolic rate in humans with [18F]-2-fluoro-2-deoxy-D-glucose: validation of method. Ann Neurol 6: 371–388.
Rhodes CG, Hughes JMB 1995 Pulmonary studies using positron emission tomography. Eur Respir J 8: 1001–1017.
Pantin CF, Valind SO, Sweatman M, Lawrence R, Rhodes CG, Brudin L, Britten A, Hughes JMB, Turner-Warwick M 1988 Measures of the inflammatory response in cryptogenic fibrosing alveolitis. Am Rev Respir Dis 138: 1234–1241.
Brudin LH, Valind SO, Rhodes CG, Pantin CF, Sweatman M, Jones T, Hughes JMB 1994 Fluorine-18 deoxyglucose uptake in sarcoidosis measured with positron emission tomography. Eur J Nucl Med 21: 297–305.
Fritts HW, Richards DW, Cournad A 1961 Oxygen consumption of tissues in the human lung. Science 133: 1070–1072.
Fritts HW, Strauss B, Wichern W, Cournand A 1963 Utilization of oxygen in the lungs of patients with diffuse, non-obstructive pulmonary disease. Trans Assoc Am Physicians 76: 302–311.
Light RB 1988 Intrapulmonary oxygen consumption in experimental pneumococcal pneumonia. J Appl Physiol 64: 2490–2495.
Weinstein MR, Oh W 1981 Oxygen consumption in infants with bronchopulmonary dysplasia. J Pediatr 99: 958–961.
Acknowledgements
The authors gratefully acknowledge the excellent technical assistance of Mary Ann dela Cruz, B.Sc., and Shelley Monkman, R.R.T.
Author information
Authors and Affiliations
Additional information
Supported by a grant from the Medical Research Council of Canada (B.S. and D.d.S.), B.S. is a Career Investigator of the Heart and Stroke Foundation, Ontario, Canada.
Presented at the Annual Meeting of the Society for Pediatric Research, Washington, DC, 1996.
Rights and permissions
About this article
Cite this article
Kirpalani, H., Abubakar, K., Nahmias, C. et al. [18F]Fluorodeoxyglucose Uptake in Neonatal Acute Lung Injury Measured by Positron Emission Tomography. Pediatr Res 41, 892–896 (1997). https://doi.org/10.1203/00006450-199706000-00015
Received:
Accepted:
Issue Date:
DOI: https://doi.org/10.1203/00006450-199706000-00015