Abstract
The exploitation of plant extracts for the synthesis of nano selenium having antibacterial and antioxidant activities is an exciting approach to counteract the prevalence of infections caused by antibiotic-resistant bacteria, which holds relevance for medical and food industries. In the present work, a green and facile method for the preparation of nano selenium (nSe) using the fruit extract of Indian gooseberry (Phyllanthus Emblica) has been reported. The optical and structural properties of the as-synthesized nSe were studied through various characterization techniques. Eventually, the antioxidant potential of nSe was investigated via 2,2-diphenyl-1-picrylhydrazyl (DPPH) and hydroxyl free radical scavenging assays. Parallely, the antibacterial activity of nSe against Escherichia coli, Staphylococcus aureus and Pseudomonas aeruginosa was evaluated. The antioxidant assays indicated that even low dosage of nSe showed excellent activity with EC50 values of 0.21 μg ml−1 and 3.34 μg ml−1, respectively. Moreover, nSe exhibited significant inhibition in bacterial growth at low minimum inhibitory concentration (MIC) values against Escherichia coli (16 μg ml−1), Staphylococcus aureus (32 μg ml−1) and Pseudomonas aeruginosa (48 μg ml−1) compared to MIC values for standard drug ampicillin. Importantly, nSe did not induce any cytotoxic effects on normal human keratinocytes (HaCaT) at the tested concentrations; representing their biocompatible nature. The data obtained demonstrated the versatility of phytogreen nSe as a potent antioxidant and antibacterial agent to effectively prevent as well as treat multidrug-resistant bacterial infections.
Export citation and abstract BibTeX RIS

Original content from this work may be used under the terms of the Creative Commons Attribution 4.0 licence. Any further distribution of this work must maintain attribution to the author(s) and the title of the work, journal citation and DOI.
1. Introduction
Resistance to antibacterial drugs and food safety have turned into two of the most important health worries for the public, government, and regulatory agencies in the last two decades [1]. In this direction, infectious diseases caused by antibiotic resistant bacteria prerequisite higher doses of drugs, additional lethal treatments as well as extended hospital stays, eventually resulting in increased mortality rate. Moreover, Food and Agriculture Organization (FAO) of the United Nations reports a loss of ∼25% of the agricultural products worldwide owing to bacterial invasion which is measured as major threat to food industry [2]. Most common bacteria (in order of decreasing predominance) include Staphylococcus aureus (S. aureus), Pseudomonas aeruginosa (P. aeruginosa), Escherichia coli (E. coli), Klebsiella, Enterobacter, Serratia and Acinetobacter [3]. To address this issue, development of new antibacterial and antioxidant agents which could overcome multidrug-resistance (MDR) with limited detrimental effects have been encouraged for medical and food industries.
In this regard, different metallic nanoparticles (NPs) including silver (Ag) [4–6], gold (Au) [7], zinc (Zn) [8], selenium (Se) [9, 10], titanium [11], ceria [12] have been explored [13]. Se is an essential metalloid element that has been extensively used in medical applications owing to its antioxidative, antimicrobial and antitumor activities [14]. Unlike other metallic NPs, Se is required by humans in trace amounts as a micronutrient. It also forms an essential component of selenoproteins that are required for antioxidant activities such as protection of DNA from oxidative stress [15]. Several studies have demonstrated that nSe exhibit size dependent biological activities [14, 16]. For instance, the capability of nSe to scavenge free radicals enhances as the particle size decreases, thereby preventing oxidative stress efficiently compared to the larger particles [15, 17, 18]. In conjunct with its antioxidant activity, nSe also shows strong inhibitory action against broad spectrum infectious bacteria. The main mechanisms behind the antibacterial activity of nSe include oxidative stress reduction [5, 19], non-oxidative mechanisms [20] and, or metal ion release [21]. The antibacterial responses of nSe have been documented against major infection causing bacteria including E. coli [10, 22], S. aureus [9, 22, 23], P. aeruginosa, and Proteus mirabilis [23].
Nanoselenium can be synthesized by different methods such as: (a) physical which include laser ablation, ultraviolet (UV) irradiation, hydrothermal [22, 24, 25], (b) chemical which include catalytic reduction, acid decomposition [26] and, (c) biogreen synthesis which rely on the use of certain bacteria [9, 16, 27, 28], fungi [29] or plants species [30–32]. Amongst all, biogreen synthesis of nSe avoids the utilization of toxic reducing agents and post-modification steps which promotes their swift application in the food and medical industries. Various organic molecules present in the natural sources can act as stabilizers and prevent the aggregation of nSe [31].
In our previous works, we have demonstrated broad-spectrum antibacterial performance of metallic nanocomposites of silver and zinc oxide (Ag-ZnO) with nearly equal wt% of Ag and Zn against recombinant green fluorescent protein (GFP) expressing E. coli and S. aureus. The main objective was to synthesize a heterostructure combining the antibacterial activity of Ag and Zn, which hence needed two chemical precursors [33]. In this study, we aim to develop a single-precursor based metallic nanoparticles of Se following a green routeto exhibit killing of both Gram positive and Gram negative bacteria.
We demonstrate a phytogreen method for the synthesis of nSe using fruit extract of Phyllanthus emblica, popularly known as Indian gooseberry (IG) for mediating chemical reduction. This methodology is simple and produced nSe in one-step without any need for additional stabilizers. IG fruit is edible and rich in reducing agents mainly vitamin C (ascorbic acid) and supplementary phytochemicals such as tannins, phenols and flavonoids. Therefore, IG fruit extract served both as a reducing and capping agent for nSe synthesis, subsequently avoiding aggregation. The formation and physicochemical properties of synthesized nSe have been elucidated in detail. Besides, our study encompasses dual functional aspects of nSe such as potential antioxidant and antibacterial agents. To demonstrate the antibacterial property of nSe, most commonly infecting bacteria like E. coli, S. aureus and P. aeruginosa were selected. Furthermore, in vitro biocompatibility was assessed using HaCaT cells.
2. Experimental section
2.1. Chemicals, bacterial strains and growth media
All the chemicals used were of reagent grade and used without any purification. Fresh IG fruit was purchased from the local market. Sodium selenite (Na2SeO3) powder, ascorbic acid were procured from Sigma-Aldrich, India. DPPH (2,2-diphenyl-1-picrylhydrazyl), sodium phosphate monobasic monohydrate, trichloroacetic acid (TCA) and 2-Deoxy-2-Ribose were obtained from Sisco Research Laboratories (SRL), India. Sodium phosphate dibasic heptahydrate was bought from USB, India. Ferric chloride anhydrous (FeCl3), sucrose, Mueller Hinton broth, Brain-Heart Infusion (BHI) media and antibiotic Ampicillin was purchased from Himedia, India. Ethylenediaminetetraacetic acid (EDTA) was obtained from Loba Chemicals. Thiobarbituric acid (TBA) was bought from Spectrochem. Potassium permanganate (KMnO4) and sulphuric acid (H2SO4) were acquired from Chemigens, India. Hydrogen peroxide (H2O2) was purchased from Fischer Scientific. Standard bacterial strainsofE. coli (MTCC 739), P. aeruginosa (MTCC 1688) and a gram positive strain of S. aureus (MTCC 737) were obtained from Microbial Type Culture Collection Centre (MTCC), Institute of Microbial Technology, Chandigarh, India. All the preparations were made using deionised (DI) water.
2.2. Preparation of IG extract
50 g of fresh IG fruit was finely chopped and added to 200 ml DI water. The mixture was then boiled at 100 °C for 5 min. Upon cooling, the extract was strained using a fine mesh sieve and stored in dark under refrigerated conditions.
2.3. Synthesis of nSe
5 ml of 5 mM Na2SeO3 solution was prepared in DI water under magnetic stirring at room temperature to form a colorless solution. Then, 7 ml of IG extract was added dropwise to it to form a turbid solution. After 1-2 min, the colour of solution changed to orange-red, indicating formation of nSeThe solution was left for 24 h in dark for reaction stabilization. Thereafter, the formed nSe were purified by performing dialysis using 1 KDa filter device against water (2 L) overnight. Pure nSe were then stored at 4 °C for further experiments.
2.4. Estimation of ascorbic acid content in IG extract
The content of ascorbic acid in the prepared extract was investigated by spectrophotometric method using potassium permanganate (KMnO4) [34]. Briefly, acidified KMnO4 solution (0.630 mM, 10 ml) was prepared in 0.5 M H2SO4. Simultaneously, ascorbic acid standard solutions of varying concentrations (1, 4, 8, 12, 16, and 20 μg ml−1) were also prepared. Then, 0.1 ml of KMnO4 was added to 1 ml of ascorbic acid solutions and incubated at room temperature for 5 min. A blank determination included 0.1 ml KMnO4 in 1 ml DI water. The absorption spectra of the solution were recorded at 532 nm against blank (only DI water) and a calibration curve was prepared. This curve was used to estimate the amount of ascorbic acid in the IG extract.
2.5. Characterization
The formation of the as-synthesized nSe was analysed using various spectroscopic and microscopic techniques. UV-visible spectra were recorded with DI water at room temperature using Cary 4000 UV–vis spectrophotometer (Varian) in the wavelength range of 200–800 nm. The nature of structural phase was identified using x-ray Diffraction (XRD) pattern obtained using a Model D8 Advance (M/s Bruker AXS, Germany) with monochromatic Cu-Kα radiation (λ = 1.5406 Å) in the 2θ range of 10°–90° and a scan speed of 0.5 min−1. For XRD analysis, a thin film was prepared by depositing 200 μl of the sample on a glass slide and drying thoroughly at 45 °C. Raman spectra was recorded over the range of 150–600 cm−1 on a InVia Raman spectrometer equipped with 514 nm excitation laser. The hydrodynamic diameter and charge distribution on nSe were evaluated by laser diffractometry techniques namely, Dynamic Light Scattering (DLS) and Zeta Potential, respectively using Malvern Zetasizer (ZS90) (Worcestershire, UK). For determining the hydrodynamic diameter and surface charge, 50 μl of the samples were dispersed in DI water by sonication for 10 min in a bath sonicator. The morphology and size of the synthesized samples were observed under High Resolution Transmission Electron Microscope (HRTEM) (FEI Tecnai G2-F20) at 200 kV coupled with an Energy-Dispersive x-ray spectrometer (EDX) accessory. Selected Area Electron Diffraction (SAED) pattern from the TEM image was obtained to confirm the structural phase of the sample. The TEM analysis was performed by drop-casting 10–20 μl of the sample on carbon coated copper grid and allowing it to dry thoroughly in air before analysis.
2.6. Antioxidant activity
2.6.1. Scavenging of DPPH radical
The scavenging of the free radical DPPH was used as the model reaction to assess the antioxidant potential of the synthesized nSe sample. The procedure was adopted from previous work with few modifications [35]. First, different concentrations (0.2, 0.3, 0.4, 0.5, 0.6, 0.7, 0.8 μg ml−1, 0.5 ml) of nSe solutions were prepared in DI water. The prepared solutions were added to the freshly prepared methanolic DPPH solution (100 μM, 0.5 ml). Methanolic DPPH solution without nSe solution was taken as a negative control. All the samples were incubated in the dark for 15 min. The change in the absorbance of the mixture was then recorded at 517 nm using UV–vis spectrophotometer.
2.6.2. Scavenging of hydroxyl radical
The hydroxyl radical (OH·) scavenging was estimated using deoxyribose method [36]. The reaction mixture contained the following: phosphate buffer (50 mM, 0.33 ml), EDTA (1 mM, 0.1 ml), FeCl3 (10 mM, 0.01 ml), ascorbic acid (1 mM, 0.1 ml), H2O2 (10 mM, 0.1 ml) and deoxyribose (10 mM, 0.36 ml). Further, 1 ml of varying concentration of nSe(1, 2, 3, 4, 5 and 10 μg ml−1) in phosphate buffer were prepared, added to the reaction mixture and incubated at 37 °C in water bath for 1 h. A blank was prepared without nSe solution. Then, 1 ml of each TBA (1% (w/v)) and TCA (2.8% (w/v)) was added to the 1 ml of reaction solution and incubated at 100 °C for 20 min in a water bath. The mixtures were allowed to cool down and the absorption spectra were recorded at 532 nm, against the blank.
The free radical scavenging activity (in percentage) (% SA) for DPPH and OH· was calculated as:
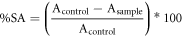
where Acontrol and Asample are the absorbance of the DPPH/ OH· solution
(without nSe) and sample solution (with nSe), respectively.
2.7. Antibacterial Studies
2.7.1. Cultivation of bacteria
The cultures of E. coli, P. aeruginosa and S. aureus were maintained on Mueller Hinton (MH) agar plates, and stored at 4 °C for further use.
2.7.2. Agar well diffusion assay
The preliminary antibacterial activity of nSe was shown by agar well diffusion assay. Briefly, 50 μl of logarithmic phase growing bacterial culture (∼105 CFU ml−1) was spread over MH agar plates. Wells were punched into agar plate through sterile 1 ml tip. Separately, different concentration of nSe were prepared in phosphate buffer saline (PBS) and added into designated wells. PBS and ampicillin solution (80 μg ml−1) were added as negative and positive controls, respectively. The plates were incubated at 37 °C for 24 h. The antibacterial activity of the nSe was assessed from measurement of diameter of clear zone of inhibition formed around the wells.
2.7.3. Minimum inhibitory concentration (MIC)
For MIC assay, few colonies of each culture were picked and seeded in 20 ml of MH broth and incubated at 37 °C for 24 h with gentle shaking (200 rpm). The bacterial growth was monitored through optical density at 600 nm. The determination of MIC of nSe was carried out in 96-well flat bottom microtiter plates through standard broth microdilution method in MH broth. For performing the assay, standard guidelines of the Clinical & Laboratory Standards Institute (CLSI) were followed. Briefly, bacterial cells (105 CFU ml−1) from logarithmic growing culture were grown into wells and then mixed with increasing concentration of nSe (in the range of 1-100 μg ml−1). The microtiter plates were incubated at 37 °C for 24 h without shaking. The bacterial growth was determined by recording and correlating the optical density (OD) of the MH broth at 600 nm using a microplate reader. The lowest concentration of nSe which could inhibit the growth of bacteria in the broth was considered as the MIC value against that particular bacterial species. A standard ampicillin was used as a positive control for the above study.
2.7.4. Time kill kinetic assay
The time kill assay of nSe and ampicillin against E. coli, P. aeruginosa and S. aureus was determined by incubating them with 105 CFU of bacteria and suspended in 20 ml MH broth for 24 h under constant shaking at 200 rpm. The MIC values of nSe and ampicillin were used for the study. 100 μl of each of the bacterial suspensions were drawn and spread on MH agar plates at different time intervals (0, 4, 8, 12, 16, 20, and 24 h). The petri plates were incubated for 24 h at 37 °C and the numbers of colonies were counted. The untreated bacterial cells were used as control.
2.7.5. SEM analysis
The morphology of the bacterial cells after treating with nSe was determined by SEM. Log phase cells of E. coli, P. aeruginosa and S. aureus were treated with nSe for 6 h at 37 °C, collected through centrifugation (5000 g × 10 min), washed 3–5 times with PBS (pH 7.4) and finally chemically fixed with 500 μl of 2% glutaraldehyde solution for 2 h. The fixed pellets were washed 3-5 times with PBS (pH 7.4) and gradually dehydrated by subsequent incubation for 15 min and washing with increasing concentrations of ethanol (10, 30, 50, 70, 90 and 100%) [37]. Finally, the samples were air dried on a clean silicon wafer and a thin gold layer was sputtered for obtaining better visualization under SEM.
2.8. Biocompatibility
The cytotoxicity analysis of nSe was preformed using 3-(4,5)-dimethylthiazol-2-yl)−2,5-diphenyl tetrazolium bromide (MTT) protocol [38]. In brief, normal human keratinocyte cells (HaCaT) (1 × 104 cells/well) were cultured in 96-well cell culture plates containing RPMI-1640 medium supplemented with 10% fetal bovine serum (FBS) at 37 °C for overnight. Next day, after adherence of the cells, different concentrations of nSe (1–64 μg ml−1) were added into the well and incubated at 37 °C under humid conditions with 5% CO2 for 24 h. Thereafter, the cells were treated with 20 μl of MTT solution (5 mg ml−1) in PBS and incubated at 37 °C for 4 h. Subsequently, the supernatant was removed and 100 μl of DMSO was added to dissolve insoluble formazan crystals. The percentage viability of cells was evaluated by the ratio of optical density (OD) (calculated at 570 nm) of treated cells to the untreated cells. For this assay, untreated cells and 10% DMSO were taken as negative and positive controls, respectively.
3. Results and discussion
Indian gooseberry, or Phyllanthus emblica or amla, is one of the significant medicinal plant native to India since ancient times [39, 40]. Being a natural source of reducing agents such as ascorbic acid, tannins, phenols and flavonoids, ithas been explored for the synthesis of Ag, Au nanoparticles, etc [41, 42]. In the present work, IG fruit extract was employed as a natural source of ascorbic acid to reduce Na2SeO3 into nSe (figure 1). Phytochemical analysis of the fruit extract was performed to determine the ascorbic acid content in the extract using KMnO4 titration and was estimated to be ∼6.8 mg ml−1 (figure S1 is available online at stacks.iop.org/NANOX/1/010031/mmedia).
Figure 1. Schematic representation of nSe synthesis using IG extract.
Download figure:
Standard image High-resolution imageWhen added to the Na2SeO3 solution, ascorbic acid present in the extract reduced the selenite ions (Se4+) into elemental Se (Se0). A visual colour change from colourless to dark orange indicated the formation of nSe (figure 2 inset) [23, 43]. Contrarily, no colour change was observed with Na2SeO3 solution without extract.
Figure 2. UV–vis absorption spectra of Na2SeO3 (1), extract (2) and nSe (3). Inset shows the gradual colour change depicting leading to formation of nSe.
Download figure:
Standard image High-resolution imageThe UV–vis spectra of the resulting nSe revealed a sharp characteristic peak at 272 nm, while no such peaks were observed in the absorption spectra of extract and Na2SeO3 solution (figure 2). The appearance of colour and subsequent absorption peak of nSe was attributed to the surface plasmon resonance (SPR) phenomenon [30, 44].
Chemical functionalization of nSe was ascertained by FTIR spectra (figure S2). Multiple intense peaks were detected at 3439, 2916, 2846, 1727, 1623, 1391, 1223, 1119, 880, 810 and 551 cm−1. A broad peak at 3439 cm−1 corresponded to the stretching vibrations of hydroxyl groups (O-H), suggesting the presence of phenols. Similarly, peak at 2916 and 2846 cm−1 represented the stretching vibrations of alkynes (C-H) due to the presence of various polysaccharides. A small peak at 1727 cm−1 corresponded to the carboxyl (C=O) stretching vibrations. Notably, the peaks at 1391, 1223, 1119 cm−1 indicated stretching vibrations of amine (C-N) groups, while the peak at 1623 cm−1 represented amide groups which ascertained the presence of peptides on the surface of nSe [45, 46]. The above findings suggested that nSe was functionalized with the various biomacromolecules from fruit extract.
TEM images revealed uniform distribution of spherical shaped particles with no signs of aggregation (figures 3(a)–(c)). Further, EDX spectrum of the as-synthesized particles depicted the distinctive peaks of elemental Se, confirming the formation of nSe (figure S3). The additional peaks of carbon (C) and copper (Cu) were attributed to carbon coated copper grid. As evident in the images, the capping layer surrounding the nSe could have been derived from the organic moeties present in the extract that stabilizes the particles by preventing their aggregation [46]. The presence of such organic molecules was also confirmed by FTIR analysis.
Figure 3. (a)–(c) TEM micrographs, (d) SAED pattern, (e) XRD pattern and, (f) Raman spectra of nSe synthesized using IG extract.
Download figure:
Standard image High-resolution imageThe size distribution histogram of nSe as obtained from TEM indicated the average particle size of nSe was ∼50.02 nm (figure S4). The nSe synthesized previously using other plant extracts showed larger particle sizes such as from Withania somnifera (45–90 nm )[45], Lycium barbarum (125 nm) [47]. On the other hand, Kokila et al demonstrated smaller size (4–16 nm) of nSe using Diospyrosmontana, but they were stable only upto 15 days [30]. In contrast, nSe synthesized by our methodology had particle size in the range of 30–70 nm and showed enhanced stability upto 60 days. The diffused rings depicted in SAED pattern of nSe demonstrated their amorphous nature (figure 3(d)). Similarly, XRD pattern also revealed the amorphous nature of nSe (figure 3(e)). The pattern depicted a broad 2θ diffraction peak at 23.30° in accordance with the previouly documented literature [45, 48, 49]. Raman spectrum of nSe in the range of 150 to 600 cm−1 exhibited a prominent resonance peak at 253 cm−1 owing to Se-Se stretching vibrations, confirming the amorphous nature of nSe (figure 3(f)) [46, 50–52]. Raman spectral analysis was in corroboration with the XRD and SAED results. Next, the zeta potential of aqueous solution of nSe was determined and was found to be highly negative (18.1 ± 0.65 mV), which did not change with time and therefore correlates with the stability of the particles for several weeks (figure S5(a)) [46, 53]. Moreover, DLS studies predicted an average hydrodynamic size of nSe around 164 ± 6.4 nm (figure S5(b)). As compared to TEM, the larger size of nSe was observed in DLS studies because of the presence of extract coating as also evident by TEM analysis. Also, water molecules used as the solvent bound to the nSe surface may increase its size [44, 49, 54].
3.1. Antioxidant activity
nSe plays an important role in prevention of oxidative stress generated by excess of free radicals such as OH·, DPPH and superoxide etc. Therefore, assessment of their scavenging property becomes essential and generally done by investigating their antioxidant activity. In this regard, the antioxidant potential of the nSe was evaluated through DPPH and OH· assay. Both the methods are based on the scavenging of coloured free radicals (DPPH and OH·) which result in the subsequent colour change or complete discoloration. The antioxidant activity of the extract was also evaluated along with nSe for comparison and no significant activity of extract in the similar concentration range was observed (data not shown). Figures 4(a)–(b) shows the percentage scavenging activity of the DPPH after 15 min incubation with varying concentrations of nSe. A colour change from violet to yellow was observed. The results showed a remarkable increase in the scavenging activity of nSe with increasing concentration. As it can be seen, even low concentrations of nSe i.e. 0.8 μg ml−1 was able to degrade ∼90% of the DPPH radicals. The antioxidant activity in terms of EC50 (concentration required to obtain a 50% antioxidant effect) was found to be 0.21 μg ml−1 which is much lower compared to nSe prepared using other methods as highlighted in previous literature reports. For example, Alagesan et al reported IC50 for nSe prepared using Withania somnifera leaf extract to be 14.81 μg ml−1 [45]. Similarly, Kokila et al synthesized nSe using Diospyros Montana leaf extract and observed EC50−22.5 μg ml−1 [30].
Figure 4. Dose dependent antioxidant activity of nSe.(a) DPPH free radical scavenging activity of nSe. (b) Photographic representation of bleaching of DPPH solution with a progressive increase in nSe concentration. (c) OH· radical scavenging activity of nSe. (d) Photographic representation of bleaching of OH· solution with a progressive increase in nSe concentration. Labelling on the photographic images denote concentration of nSe in μg ml−1. The error bars represent mean ± S.E.M. of three individual experiments.
Download figure:
Standard image High-resolution imageIn addition to DPPH, nSe induced scavenging of OH· radicals was also performed. The scavenging activity of nSe along with the corresponding decolorisation is depicted in figures 4(c)–(d). Similar to DPPH, the antioxidant activity of nSe against OH· was also found to be dose dependent with scavenging activity of ∼70.9% shown by 10 μg ml−1 nSe. The EC50 value obtained for OH· radicals was calculated to be 3.3 μg ml−1. These observations suggest that owing to their antioxidant nature, as-synthesized nSe might exhibit desired role in prevention of DNA damage, cardiovascular diseases, cancer development, and immunity disorders.
3.2. Antibacterial activity
The antibacterial activity of nSe was evaluated against both Gram positive (S. aureus) and Gram negative (E. coli and P. aeruginosa) bacteria which are generally associated with hospital-acquired infections and food spoilage [55, 56]. The MIC values of the nSe against E. coli, P. aeruginosa and S. aureus species were estimated using the agar well diffusion assay and standard broth microdilution method. Analysis of antibacterial activity data revealed that the tested bacteria were sensitive towards nSe. Till date, only a few studies have been performed for determination of MIC for bio or chemically synthesized nSe. In medical microbiology, determination of MIC has been encouraged for the treatment of serious microbial infections (such as endocarditis) or treatment of immunosuppressed patients. To the best of our knowledge, the current study was mostly focussed on evaluation of the MIC activity of the synthesized nSe.
3.2.1. Agar well diffusion assay
To examine the antibacterial efficiency of the synthesized nSe, bacterial pathogens were cultured on an agar plate and incubated with different concentrations of nSe for 24 h and the observations are recorded in table 1 and figure 5. Different concentrations of nSe (well no. 2,3,4,5,7,8,9 and 10) showed significant zone of inhibition against all the tested microorganisms (figure S6). In contrast, PBS alone (control; well no 1 and 6) did not show any significant bacterial inhibition. Results from the agar well diffusion assay showed that the nSe indeed exhibit antibacterial activity which could be attributed to the released Se ions upon the disintegration of nSe combined with the oxidative stress generated by nanoparticles. The antibacterial response of nSe against E. coli, P. aeruginosa and S. aureus indicated broad spectrum of antibacterial activity towards both Gram positive and Gram negative bacteria. Some earlier studies established that nSe can inhibit the growth of bacteria (S. aureus) as compared with the control [57, 58]. In another study, different sizes of nSe (in the range of 80–220 nm) were used in antibacterial coatings to inhibit the growth and act as a antifouling agents against the pathogen S. aureus [54]. Our results are in agreement with these findings which show the antibacterial effect of nSe towards all the tested stains.
Table 1. Agar well diffusion assay. Zone of inhibition (cm) of nSe containing compounds against different bacterial species S. aureus, E. coli and P. aeruginosa). Ampicillin and saline were used as positive and negative controls, respectively.
Sample | Concentration (μg ml−1) | Zone of inhibition (cm) | ||
---|---|---|---|---|
nSe | S. aureus | E. coli | P. aeruginosa | |
18 | 1.4 | 1.3 | 0.25 | |
36 | 1.6 | 1.9 | 0.30 | |
54 | 2.1 | 2.1 | 0.31 | |
72 | 2.3 | 1.9 | 0.35 | |
90 | 2.1 | 2.1 | 0.35 | |
104 | 2.2 | 2.4 | 0.40 | |
Saline* | 0.0 | 0.0 | 0.0 | 0.0 |
(Negative control) | ||||
Antibiotics* | 80 | 2.2 | 1.9 | 0.40 |
(Positive control) |
• Saline*• Ampicillin**
Figure 5. Antimicrobial activity of nSe against S. aureus, E. coli and P. aeuroginosa microorganisms. The zone of inhibition (in cm) is represented in histogram.
Download figure:
Standard image High-resolution imageThe most interesting finding of the present study is that the minimum concentration of nSe to attain bacterial inhibition should be at least 18 μg ml−1, which is a lower value compared to already reported [58]. This variation reason may arise from the differences in the size, shape, purity of the nSe used and most importantly the fabrication route of nSe, which is from IG fruit extract in this study.
3.2.2. Micro broth dilution assay: minimum inhibitory concentration (MIC)
The MIC value of nSe was determined by the use of standard micro broth dilution assay. The results have been presented in table 2 and figure 6.
Table 2. Broth microdilution method. In vitro evaluation of synthesized nSe against various bacterial strains.
Compound | Bacterial Strains | ||
---|---|---|---|
E. coli,b | S. aureus,b | P.aeruginosa,b | |
nSe | 16.00 | 32.00 | 48 |
Ampicillina | 24.00 | 64.00 | 48 |
aStandard drug used: Ampicillin. bMIC: Minimum Inhibitory Concentration [μg ml−1].
Figure 6. Minimal inhibitory concentration (MIC) for synthesized nSe tested against different bacteria.
Download figure:
Standard image High-resolution imageInterestingly, nSe exhibited augmented activity against E. coli and S. aureus in comparison to P. aeruginosa. Notably, MIC value for nSe against E. coli showed greater antibacterial activity than the standard drug alone, indicated by lower MIC value for nSe i.e. 16 μg ml−1 and 24 μg ml−1 for standard drug ampicillin, respectively. Notably, in a recent study the MIC value of Emblica officinalis mediated phytofabricated nSe against E. coli was estimated to be 59.83 μg ml−1 , which is ∼3.75 fold higher compared to our reported value [32]. The MIC value for nSe and ampicillin, when tested against S. aureus, was calculated to be 32 and, 64 μg ml−1, respectively. On the other hand, nSe and ampicillin had equivalent MIC value of 48 μg ml−1 against P. aeruginosa. This could possibly be attributed to the clinical variability of the bacterial stains, which may prevent the action of the nSe through the bacterial cell wall.
Remarkably, against E. coli and S. aureus, nSe exhibited higher inhibitory effect in comparison to the standard antibiotic ampicillin plausibly due to the small size of nSe that can permeate the membrane and induce cell lysis. It has been reported that metallic nanoparticles interfere with the respiratory sequence and transport protein synthesis, terminate cell division and induce microbial cell death [53, 57, 58]. Overall, the results clearly indicate that the nSe posesses broad spectrum antimicrobial activity towards both Gram positive and negative bacterial strains.
3.2.3. Time kill assay
In order to study the efficacy of nSe, time kill assay studies were executed. Evidently, the CFUs of the E. coli, P. aeruginosa and S. aureus reduced drastically after treatment with respective the MIC values of nSe. In time kill assay, a reduction in S. aureus cells after 24 h resulted in a decrease of 2.90 log units as compared to control (4.44 log units, 24 h). Reduction in E. coli and P. aeruginosa cells was also perceived after 24 h. A decrease of 2.46, 2.95 log units respectively as compared to control (E. coli, 4.43 and P. aeruginosa, 4.45 log units, 24 h) was observed (figure 7).
Figure 7. Time kill assay of nSe against (a) E. coli, (b) S. aureus and (c) P. aeruginosa cells.
Download figure:
Standard image High-resolution imageIt was evident that the maximum killing of E. coli, P. aeruginosa and S. aureus cells with nSe was observed after 12 h. Thus, it can be strongly interpreted that the nSe effectively inhibited the growth of Gram positive and Gram negative bacterial strains in a time dependent manner, thereby highlighting its bacteriostatic effect [59, 60].
3.2.4. SEM analysis
SEM analysis was performed to investigate the morphological changes in E. coli, P. aeruginosa and S. aureus after treatment with nSe. The untreated E. coli and P. aeruginosa cells retained their rod-shaped morphology and showed no evidence of membrane rupture and collapse (figure 8). In contrast, E. coli and P. aeruginosa cells treated with the nSe for 4 h appeared to undergo extensive membrane damage leading to leaking of the intracellular components and ultimately cell disruption. The untreated cells of S. aureus showed normal coccal cell morphology, while treated S. aureus demonstrated cell shrinkage, irregularities in shape and increased roughness. Collectively, significant architecture changes and cellular lysis were observed in all the treated bacterial strains which serve as clear evidence for the antibacterial effect of nSe.
Figure 8. SEM images of untreated and nSe treated bacterial cells. Scale bar: 2 μm.
Download figure:
Standard image High-resolution image3.2.5. Biocompatibility
Encouraged by the favorable outcomes of the antibacterial experiments, the biocompatibility assessment of nSe was done. In this regard, MTT assay was performed to estimate the cell vability of HaCaT cells treated with different concentrations of nSe. The results obtained clearly indicated that nSe did not show any significant toxicity against the cells (figure 9).
Figure 9. Cell viability of HaCaT cells treated with different concentrations of nSe as estimated by MTT assay. The error bar represent the mean ± SD of three independent experiments.
Download figure:
Standard image High-resolution imageNo appreciable decline in cell viability was observed at the tested concentrations (including MIC values), portraying their safety profile for antibacterial application [23, 44].
4. Conclusion
In summary, we have devised a direct, phytogreen approach for synthesizing nano selenium using Indian gooseberry fruit extract as a dual (reducing and stabilizing) agent, in one-step at room temperature. The reducing agents such as tannins, phenolics and flavonoids in the extract had synergistic effect to produce stable and uniform nSe. The nSe displayed inherent antioxidant activity with lower EC50 values in comparison to the previously reported studies on nSe synthesized via green routes. Further, nSe demonstrated remarkable antibacterial activity against broad spectrum of bacteria including S. aureus, E. coli and P. aeruginosa. Low cytotoxicity of nSe when tested on human keratinocytes in vitro prompt their possible use for antibacterial applications. Altogether, we have demonstrated a facile and direct method for the production of nSe for a myriad of practical applications which hold relevance for the prevention of bacterial associated infections.
Acknowledgments
We would like to sincerely thank Department of Science and Technology (DST/INSPIRE/04/2016/002181) and Science and Engineering Research Board (No. ECR/2018/000199/ES), Government of India for providing the financial support. DG is thankful to the Department of Biotechnology, Government of India, for the fellowship. Authors are thankful to CSIR-CSIO, Chandigarh for providing the access to various analytical facilities required for this work.
Conflicts of interest
There are no conflicts to declare.