Abstract
Facial paralysis is the inability to move facial muscles thereby impairing the ability to blink and make facial expressions. Depending on the localization of the nerve malfunction it is subcategorised into central or peripheral and is usually unilateral. This leads to health deficits stemming from corneal dryness and social ostracization. Objective: Electrical stimulation shows promise as a method through which to restore the blink function and as a result improve eye health. However, it is unknown whether a real-time, myoelectrically controlled, neurostimulating device can be used as assistance to this pathological condition. Approach: We developed NEURO-BLINK, a wearable robotic system, that can detect the volitional healthy contralateral blink through electromyography and electrically stimulate the impaired subcutaneous facial nerve and orbicularis oculi muscle to compensate for lost blink function. Alongside the system, we developed a method to evaluate optimal electrode placement through the relationship between blink amplitude and injected charge. Main results: Ten patients with unilateral facial palsy were enrolled in the NEURO-BLINK study, with eight completing testing under two conditions. (1) where the stimulation was cued with an auditory signal (i.e. paced controlled) and (2) synchronized with the natural blink (i.e. myoelectrically controlled). In both scenarios, overall eye closure (distance between eyelids) and cornea coverage measured with high FPS video were found to significantly improve when measured in real-time, while no significant clinical changes were found immediately after use. Significance: This work takes steps towards the development of a portable medical device for blink restoration and facial stimulation which has the potential to improve long-term ocular health.
Export citation and abstract BibTeX RIS

Original content from this work may be used under the terms of the Creative Commons Attribution 4.0 license. Any further distribution of this work must maintain attribution to the author(s) and the title of the work, journal citation and DOI.
1. Introduction
Facial paralysis (FP) results from impairment of cranial nerve (CN) VII leading to loss of facial movement and expression. FP can be peripheral (infranuclear; damage to ipsilateral CN) or central (supranuclear; contralateral lesion in the brain stem) [1], as well as being unilateral or bilateral. The most common type (20–30 per 100 000 people) is unilateral idiopathic palsy also known as Bell's palsy. However, FP can also occur as a result of stroke, tumor, infection, surgery or trauma [2].
The loss of facial muscle activity has both physiological complications and psychological consequences. The most important physiological consequence of FP for vision health is the impairment of the blink function [3]. Blinking is fundamental for eye health through maintaining the tear film and removing debris, facilitating vision and preventing chronic irritation and pain [4–6]. Additionally, optimal visual performance was shown to rapidly decrease after 4–5 s from a blink [7]. In general, the inability to move facial muscles (i.e. forehead, eyebrow, cheeks or the lips) also results in difficulties in eating, smiling, speaking and expressing emotions [8]. This can psychologically impact FP patients through social alienation, isolation, and emotional disruption with 42% of this population eventually developing depression [9–11].
FP is highly variable, describing mild weakness to complete paralysis [12], and has been shown to resolve completely on its own in 70%–85% percent of patients in a few weeks or months [2, 12]. However, FP patients who do not recover completely, or in the time before complete recovery, are still susceptible to health and social consequences.
The most common strategy to treat eye dryness caused by tear film degradation related to impaired blink function is the application of lubricating drops or ointment [13]. Other strategies such as the use of a moisture chamber, mechanical eyelid taping, and canalicular or punctal (tear drainage duct) occlusion are other popular non-invasive methods [12, 13]. Facial exercises and massages have a positive psychological impact on FP patients and are speculated to support facial muscle tone [2]. However, there is little evidence to support their effect on recovery. Various pharmaceutical approaches to treat facial muscle tone problems exist, but they are not widely used and have mixed success rates [2, 12, 14, 15]. Surgical procedures to reduce the palpebral fissure and corneal exposure include: the implantation of gold eyelid weights [16], lower eyelid ectropion surgery and tarsorrhaphy.
Neuroprosthetics have been used to deliver targeted electrical stimulation [17–20], to nerves and muscles restoring sensory-motor functions in individuals with different neurological disorders [17, 21, 22]. Electrical stimulation has also been used to improve facial function through non-invasive activation of muscles [23]. Although electrical stimulation has not demonstrated significant therapeutic effects in improving facial muscle function [24, 25], it shows promise as an assistive tool to restore both blink function [26–28] and facial muscle tone [15] without surgery in acute cases (2–3 months) [26, 28]. Moreover, restoration of the blink function was shown to improve visual acuity as well as ocular symptoms such as foreign body sensation and dryness after a few hours [26, 27].
Real-time, myoelectric control of neuroprosthetics make them more intuitive to the user and allow for precise control [29, 30]. Blink detection methods on the healthy side of the face have been explored in an effort to close the control loop and take steps towards usable devices.
Vision-based methods such as an infrared beam have been used to detect blinks [31] but is highly susceptible to false positives caused by looking down [32]. Camera-based methods, such as image classifying convolutional or recurrent neural networks are robust, but inherently computation heavy [33, 34] challenging real-time applicability, miniaturization and portability.
Electromyography (EMG) has been shown to robustly detect blinks from the healthy orbicularis oculi muscle (OOM) [5, 35, 36], but is susceptible to crosstalk with other facial muscles and movements [35]. EMG activity is particularly useful for a myoelectric control system development due to its anticipatory nature of muscle movement, typically preceding facial muscle actuation by 30–40 ms [37, 38] and OOM activity by 10–12 ms [39]. This is something vision-based methods fail to take advantage of. Using EMG, Rantanen et al developed and tested a myoelectric control pacing device that could detect a blink and deliver stimulation in real-time (<33 ms) in healthy individuals, but did not validate this myoelectric control system in patients [40]. McDonnell et al tested a myoelectric control system in 6 FP patients and demonstrated that both percutaneous and transcutaneous electrical stimulation could restore blink function detected through EMG [41]. Notably, both systems used large table-top stimulators completely lacking portability necessary for an everyday life adoption and do not take clinical outcomes such as tear film quality and visual acuity into consideration.
Here we present a real-time blink restoration system (NEURO-BLINK) for unilateral FP patients that detects the healthy blink with surface EMG [42] and supports the controlateral blink impaired by paralysis with surface electrical stimulation. We evaluated the success of real-time blink restoration using NEURO-BLINK as well as ocular symptoms (e.g. eye dryness) linked to the loss of blink function before and after use.
2. Method
The experiments were conducted in accordance with the Declaration of Helsinki and informed consent was obtained from all subjects. Subjects shown in video consented to have identifiable images and videos used for scientific publication. The experiments were approved by Swiss Ethics (BASEC ID: 202-D0065), Swiss Medics (Ref. Number 10000966), and were registered with ClinicalTrials.gov (NCT05504473).
2.1. Patient's recruitment
Ten patients with unilateral facial palsy were recruited through the Kantonspital Aarau Augenklinik (table 1). Subjects were included if they were over the age of 18 with a facial palsy diagnosis and have a noticeable lagophthalmos. They were excluded if they had a pacemaker or defibrillator, part of a vulnerable population, in an emergency situation, or had allergies to sticky electrodes or medical metallic sensors. No further tests were conducted to understand if palsy was more central or peripheral.
Table 1. Participants.
Age (years) | Sex | Time since diagnosis | Probable cause | |
---|---|---|---|---|
P1 | 40 | F | 4 years | Post-surgical |
P2 | 61 | M | 2 years | Idiopathic |
P3 | 76 | F | 4 months | Post-surgical |
P4 | 39 | F | 1 month | Idiopathic |
P5 | 57 | M | <1 month | Idiopathic |
P6 | 46 | M | <1 month | Idiopathic |
P7 | 58 | F | 3 months | Post-surgical |
P8 | 30 | M | <1 month | Infectious |
PX1 | 27 | M | 12 years | Post-surgical |
PX2 | 82 | M | 2 months | Post-surgical |
Two out of the ten recruited patients were excluded at the start of the trial, after the first characterization phase, since they demonstrated a high amount of sensory loss around the affected eye and no blink reaction to electrical stimulation (figure S1). In these cases (PX1 and PX2), neurostimulation to elicit a blink function would not be useful, so they were excluded from the remainder of the study. Quantitative sensory testing (QST) maps and electrically evoked sensation maps for all patients are displayed in figure S1.
2.2. Experimental setup
A GoPro 10 was mounted on a tripod and set to a framerate of 120 fps. Videos were acquired in landscape orientation at 1080p full HD resolution. The GoPro was placed at a distance that allowed the entire head and the tops of the shoulders to be in the frame (typically ∼30 cm). Patient position relative to the camera was kept consistent in all the recordings. Experiments were conducted in the same room with the same artificial lighting conditions for all the participants.
Electrodes 1.5 cm in diameter were used to elicit a blink in subjects. The active electrode was placed on the paretic side at the innervation point of zygomatic branch to the OOM just lateral to the orbital rim [26].
Active EMG electrodes 1 cm in diameter were used to detect the healthy blink from the healthy contralateral side. One EMG electrode was placed directly under the eye and the other was placed towards the outside of the eye along the length of the OOM in the direction of its fibers. The EMG electrodes were connected directly to a MyoWare Muscle Sensor (Advancer Technologies, USA). The MyoWare signal was transmitted to a Raspberry Pi through an analog-to-digital converter (figure S2). Subsequently, the microcontroller unit was wire-connected to the portable stimulator, RehaMove 3 (HASOMED GmbH, Germany). To complete the setup, the stimulator was interfaced with the patient via the electrodes positioned on the paretic side of the face. The wiring of the electrodes was integrated into a glasses frame, and all electronic components were housed within a custom designed 3D-printed case.
The described NEURO-BLINK system is depicted in figure 1(A) and video S1.
Figure 1. Wearable bionic system to restore blink. (A) The NEURO-BLINK System. On the left side of the figure, a healthy blink is detected using EMG electrodes placed on the OOM. This information is processed in real-time on a microcontroller that determines if a blink has been detected and then stimulates the impaired side (right side of the figure) with electrodes placed over the innervation point of the OOM. (B) Experiment protocol overview.
Download figure:
Standard image High-resolution image2.3. Protocol description
The experimental protocol is graphically described in figure 1(B).
Prior to any electrical stimulation, an ophthalmologist assessed clinical outcomes including the visual acuity (LogMAR test), eye dryness (Oxford Grading Scheme) and lagophthalmos—measurement in mm of the eye opening when the patient closes his/her eyes as if they were sleeping.
Then, to optimize the NEURO-BLINK system for each patient, we performed electrical stimulation calibration and EMG calibration for each patient. Calibration procedures are described in the following two methods sections: 'Electrical Stimulation and Characterization' and 'EMG Blink Detection and Myoelectric Controlled System Development'.
During the experimental session, patients were seated on a chair, instructed to look straight ahead into the camera and to blink in two different conditions. In the first condition, patients were asked to blink every 3 s guided by a metronome, which was synchronized with the stimulation. This condition, called paced control, was used to evaluate the potential of electrical stimulation to restore blink function when coupled with residual blink activity. Patients performed 4 blocks each 1 min long with stimulation (WS) and 4 blocks without (NS). The order of the blocks was randomized.
In the second condition, patients were instructed to blink naturally, when they felt the need to. Here, the full NEURO-BLINK system was used to perform myoelectric control. This worked by capturing healthy blink activity through EMG and triggering electrical stimulation on the impaired side to support the residual blink (video S1). This is further described in the section titled 'EMG Blink Detection and Myoelectric Controlled System Development'. Similar to the paced control, patients performed 4 times 1 min WS blocks and 4 times 1 min NS blocks where they could blink whenever they needed. Block order was again randomized. This condition was performed to assess the potential of NEURO-BLINK with intuitive control (Myoelectric Control) to be useful in a real-life scenario compared to a controlled lab scenario (Paced Control).
Finally, at the end of the session, the patient performed the electrical stimulation calibration procedure again to assess fatigue or habituation. Similarly, clinical measurements (visual acuity, eye dryness and lagophthalmos) were performed by the same ophthalmologist to assess potential therapeutic effects.
2.4. Electrical stimulation and characterization
Neurostimulation parameters such as pulse amplitude, pulse width, and electrode placement are highly subject specific since they depend on specific anatomical differences [43]. This can be further complicated by the condition of the underlying impaired nerve. Because of this, careful calibration and characterization of the electric parameters must be performed for each patient to ensure a thorough understanding of their response to electrical stimulation. An ideal calibration of the injected charge would be able to achieve 100% closure with minimal discomfort.
During this calibration phase, charge magnitude (amplitude and pulse width) and electrode locations were varied to determine the optimal parameters (figure S3). Stimulation frequency and duration were kept constant at 100 Hz and 100 ms respectively, which according to previous studies mimic a natural blink [26, 28]. To determine the best location, the cathode of the stimulating electrode pair was placed in the corner of the eye to target the innervation point of the zygomatic branch of the facial nerve on the OOM [26–28]. Positions of the stimulating anode were: +90°, +60°, +30°, 0, −30°, −60˚, −90°, −120° as well as electrodes under eye with cathode below the orbital rim (position called 'under 1') and electrodes under eye shifted 1 cm towards the nose compared to 'under 1' (position called 'under 2'). Other possible locations were only considered in special cases.
First, an 'amplitude ramp' composed of a fixed series of stimuli lasting for 100 ms each, presented every 3 s was delivered to each location. Each stimulus had a pulse width of 200 µs and an amplitude 1 mA higher than the previous stimulus in the series. The location of the perceived sensation, the type of the sensation [44] as well as the motor threshold charge and the saturation (charge to either achieve full closure or uncomfortable sensation) were recorded. The best location was chosen by considering the quality of the induced blink (full blink or maximum possible cornea coverage) and the quality of the sensation reported by the patient.
Subsequently, a procedure for charge response characterization of the best location was further evaluated with an additional charge ramp. This second ramp was composed of a fixed series of stimuli lasting for 100 ms each, presented every 3 s. Each sequential stimulus had an increased pulse width of 10 µs, starting from 100 µs, and fixed amplitude at the identified saturation value in the previous step.
This two-step calibration process served both technical and logistical purposes. The initial coarse evaluation served to expedite the identification of the correct electrode positioning, resulting in time savings. Additionally, the selected stimulator used for testing only allowed a resolution of a single mA for amplitude modulation. Therefore, a second, more precise refinement of the delivered charge was deemed necessary to achieve better control over the stimulation delivered.
2.5. EMG blink detection and myoelectric controlled system development
The raw output of the MyoWare signal was sent to the Raspberry Pi through an analog to digital converter with a sample frequency of 860 Hz to be processed in real time. In order to remove the motion artifact, 6th order Butterworth filter with a high pass cut off at 75 Hz is applied to 300 sample windows. The standard deviation of the last 100 values in the window is compared to the blink threshold to determine if the subject is blinking. This windowing and filtering process is shifted each time for 10 samples to acquire real time updates. Other algorithms such as the classical EMG envelope thresholding were also analyzed [45], however these methods' computational time was 5 times higher than our proposed algorithm and therefore discarded due to the importance of the simultaneous blink with the healthy side.
The EMG blink detection threshold was determined through a 15 s recording where the subject was asked to blink naturally without activating other facial muscles. The threshold was manually chosen to be approximately 2x the standard deviation of noise. If the noise was too high for determining a reliable threshold, electrode position was shifted slightly, but kept under the healthy eye.
2.6. Data acquisition and analysis
Frames at maximum (open) and minimum (closed) eye closure were manually extracted from the videos. IntelliJ was used to measure the distance in pixels between the upper and lower eyelids. Given camera placement and resolution, our system has a sensitivity of approximately 7% of overall eye closure. This was mitigated through repetitions allowing us to achieve statistical power. In addition, a second researcher analyzed some random data points that had been previously measured obtaining the same results, mitigating the impact of human error and strengthening the reliability of the measurements.
Percentage of eye closure was calculated using the following formula:
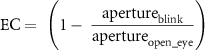
where EC represents the eye closure, apertureblink is the distance between eyelids during a blink, and apertureopen_eye is the distance between eyelids between blinks. This is illustrated in figure S4(A).
Percentage of cornea coverage (CC) was calculated in three cases (figure S4(B)). In case 1, the cornea was covered both above and below by the eyelid and CC was calculated using the following formula:
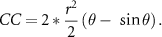
In case 2, the cornea was <50% covered by one eyelid and CC was calculated using the following formula:
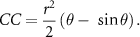
In case 3, the cornea was >50% covered by the eyelid and CC was calculated using the following formula:

In all previous formulas r is the radius of the cornea, and θ is the central angle created by the intersection of the eyelids and the cornea.
The overall testing time, including calibration was approximately 2 h for each subject.
All authors had complete access to anonymized data. The Wilcoxon signed-rank test was used to compare the change in percentage closure. An ANOVA for repeated measures was used to analyze the effect of NEURO-BLINK on all patient blinks together.
3. Results
Of the 10 enrolled patients, all participated in the initial characterization phase. Following this, 2 patients with no response were excluded. A total of 8 patients underwent paced control testing and 6 underwent myoelectric control testing.
3.1. Eye closure increases with increasing charge
Electrode placement for optimal eye closure and minimal discomfort for patients generally fell into two categories: bottom placement and lateral placement (figure 2(A)) with all locations on the OOM.
Figure 2. Electrical Stimulation Characterization. (A) Optimal electrode placement per subject. (B) Calibration curves of optimally placed electrodes showing percentage of eye closure compared to charge injected. (C) Charge motor threshold of healthy and paretic patients defined as the moment of first visible muscle twitch. This threshold was found using steps of 10 μs pulse width. (D) Patients reported types of sensations elicited by optimally placed electrodes.
Download figure:
Standard image High-resolution imagePatients who had a motor response to stimulation all demonstrated a monotonically increasing relationship between eye closure percentage and charge injected, with many subjects falling inside a healthy range (figure 2(B)). Two subjects demonstrated no motor response and were excluded from further testing.
The motor threshold of paretic patients (0.91 ± 0.45 µC) was found to be significantly higher (p < 0.01) than that eight of healthy participants (0.45 ± 0.21 µC) when compared with a Wilcoxon rank sum test (figure 2(C)).
The types of sensation reported by patients were 38.4% electricity, 23.1% pulsation/pressure, 15.4% stinging, 7.7% tingling, 15.4% pinching (figure 2(D)). With words chosen from a list [44]. No patients reported pain.
3.2. Blink restoration with optimal charge
The stimulation amplitudes were chosen as the minimum amplitude that provided maximum eye closure as long as they were below the pain threshold. For patients 1–8 respectively were 24 mA, 7 mA, 9 mA, 7 mA, 7 mA, 6 mA, 8 mA, and 5 mA.
3.3. Electrical stimulation increases eye closure and cornea coverage with paced control
Overall eye closure and percentage of cornea coverage was evaluated with a metronome set to 60 BPM with a high tone every third beat to signal a blink every 3 s.
Eye closure over all subjects together was found to significantly (p < 0.01, n = 562, statistical power >99%) increase with NEURO-BLINK. The median change over 8 subjects is 14.51%. There was also a significant (p < 0.001) increase in eye closure with NEURO-BLINK in all eight patients individually (figures 3(A) and S5).
Figure 3. Eye closure and cornea coverage improved with paced electrical stimulation. (A) Percentage of eye closure measured through counting pixels with and without NEURO-BLINK. Box plot of the change in eye closure for each patient. Group statistics were performed using ANOVA for repeated measures. *** p < 0.001. Bar plots for individual patients with error bars representing confidence interval. *** p < 0.001 (B) Percentage of cornea coverage with and without NEURO-BLINK calculated in three different cases based on cornea position in eye aperture. Box plot of the change in cornea coverage for each patient. Group statistics were performed using ANOVA for repeated measures. *** p < 0.001. Bar plots for individual patients with error bars represent confidence interval. *** p < 0.001 (C) Lagophthalmos measured before NEURO-BLINK with a ruler and with NEURO-BLINK by counting pixels and converting this to millimetres. Error bars represent confidence interval.
Download figure:
Standard image High-resolution imageCornea coverage over all subjects together significantly (p < 0.001, n = 562, statistical power >99%) increased with NEURO-BLINK. The median change over 8 subjects is 7.42% There was also a significant (p < 0.001) increase in cornea coverage in P4, P5, P6, P7, and P8 (figures 3(B) and S5).
3.4. Lagophthalmos reduced with electrical stimulation
The size of lagophthalmos (the inability to close the palpebral fissure completely) was measured before NEURO-BLINK calibration and found to be 4, 1, 2, 2, 6, 1.5, 8, and 3 mm in P1–8 respectively. Larger lagophthalmos indicates higher corneal exposure to potential irritation and is therefore, considered worse. With NEURO-BLINK application, lagophthalmos was found to decrease to 1.17 ± 0.30, 0, 0.97 ± 0.16, 0, 0, 0, 7 ± 0.20, and 0 mm in P1–8 respectively. This decrease was found to be statistically significant in all eight subjects (figure 3(C)).
Lagophthalmos without stimulation was measured after the entire session (∼2 h). It was found to be 4, 1, 1, 1.5, 3, 1.5, 8, and 3 mm in P1–8 respectively indicating a reduction of lagophthalmos in P3, P4, and P5 (figure S1).
3.5. Myoelectrically controlled stimulation increases eye closure and cornea coverage
Eye closure and cornea coverage were also evaluated in the six of the patients who opted to continue testing the myoelectric control system that delivered electrical stimulation to aid the paretic blink when a blink was detected by EMG on the healthy side (figure 4(A)). The EMG detection algorithm was tested offline with 95% accuracy (4% false positives, 1% false negatives) when compared to blinks observed in video footage (as in experimental set up) where a blink was defined as having at least one frame with full eye closure. Electrical stimulation was delivered 46 ± 13 ms after the blink being detected. This delay was measured by timing the difference between blinks in two healthy people, where one is controlling the electrical stimulation being applied to the other.
Figure 4. Myoelectrically controlled blink detection improves eye closure and cornea coverage. (A) Real time blink detection and accuracy. (B) EMG threshold determination for blink detection. (C) Functional results: Eye Closure and Cornea Coverage with box plots representing change in each patient and bar plots for individual patients with error bars representing confidence interval. Group statistics were performed using ANOVA for repeated measures *** p < 0.001, ** p < 0.01, * p < 0.05.
Download figure:
Standard image High-resolution imageEye closure over all subjects significantly (p < 0.05, n = 528, statistical power >99%) increased with the myoelectric control NEURO-BLINK system. The median change over 6 subjects is 24.64%. There was also a statistically significant (p < 0.05 in P8 and p < 0.001 in all others) increase in eye closure with NEURO-BLINK individually in all six patients who tried the myoelectric control system (figure 4(C)).
Cornea coverage over all subjects together significantly (p < 0.05, n = 528, statistical power >99%) increased with the myoelectric control NEURO-BLINK system. The median change over 6 subjects is 24.14%. There was a statistically significant (p < 0.01) increase in cornea coverage with NEURO-BLINK individually in all patients except P8 (figures 4(C) and S5).
3.6. Clinical outcomes
Visual acuity and Oxford Grade were not found to change before and after NEURO-BLINK application (figure S1(A)).
4. Discussion
This study presents NEURO-BLINK, a fully portable myoelectric control blink restoration system, that can successfully detect healthy blinks using EMG and trigger electrically assisted blinks in the impaired eye. NEURO-BLINK increased eye closure and cornea coverage in eight patients who had a functional response to electrical stimulation eliciting unnatural, but not uncomfortable sensations. The most common electrode placement to best induce a blink was lateral to the eye, consistent to what was found by previous studies [26, 28]. However, we also present an alternative optimal bottom location that proved especially assistive in patients who had residual muscle activity in response to an intentional blink movement mainly in the upper lid. Unlike any previous attempt with assistive devices, seven out of eight patients (87.5%) had an elicitable blink movement that covered their entire pupil corresponding to a full blink [46]. This contrasts with the 53% of patients in whom Mäkelä et al were able to elicit a blink [26], 55% by Frigerio et al [28] and 66% by McDonnall et al [41]. This could be attributed to the combination of a methodological calibration approach and synchronized nature of the myoelectric control system. NEURO-BLINK's comprehensive calibration methodology allowed us to consider positions under the eye as opposed to only lateral positions [26, 28]. Notably, our methodological calibration additionally allowed NEURO-BLINK to achieve significant motor improvement while completely avoiding pain, something previous work [26, 28, 41] has failed to do. Our larger active area likely helped us avoid the unnecessary pain that accompanied the use of small electrodes [47] by McDonnall et al on the eyelid [41], but limited us in our testable locations.
Additionally, the myoelectric control NEURO-BLINK system takes advantage of the patient's natural bilateral activation of a blink, therefore delivering stimulation near to the time of natural muscle activation. This synchronizes electrical stimulation with any residual muscle activity on the impaired side allowing the stimulation to intuitively support the activity without requiring the subject to think about correctly timing a blink as in the paced control condition. Synchronized blinking also improves overall facial symmetry [11, 48] and takes advantage of a relaxed levator palpebrae muscle that would be active if the subject was trying to keep their eye open reflecting important psychological benefits of using a myoelectric control device. These results and implications underline the importance of moving forward with a myoelectric control system that can synchronously pair stimulation with the intention to blink. It also highlights the usefulness of NEURO-BLINK as an assistive device in addition to the known therapeutic benefits of OOM stimulation [26, 27].
Two patients with no response to electrical stimulation were excluded from the remainder of the study. Among these, one had impaired sensation in the entire check and side of the chin and the other had loss near the eyebrow and in the cheek (figure S1(A)). Both demonstrated little to no movement in the OOM even when electrodes were placed in locations they could feel. Although the sensory component of the facial nerve (CN VII) innervates the external ear and the tongue [49, 50] not the skin on the face, we considered sensory feedback to be an intuitive measure of facial nerve health. Indeed recent research suggests that sensory axons in the face play an important role in maintaining facial muscle tone [51]. In patients with intact cutaneous sensory feedback, eye closure was consistently shown to increase with injected charge at various electrode placements around the eye. Impaired cutaneous feedback in the face (innervated by CN V) and lack of response to OOM (innervated by CN VII) stimulation in the two excluded patients may indicate that the pathology of their palsy affected both CN V and VII or that these patients were both more impaired in CN VII causing a high sensory-motor mismatch which can lead to perceived numbness in the cheek [52]. Although impaired sensory feedback coincided with poor response to stimulation in these two patients, sensory feedback testing cannot be a robust indicator of stimulation response in the OOM. While NEURO-BLINK primarily targets the innervation point of the OOM, non-invasive stimulation has the potential to affect both the nerve and the underlying muscle tissue. This could theoretically lead to the direct activation of a deinnervated muscle. However, in individuals with chronic conditions, factors such as muscle atrophy may contribute to their limited response. As a result, more research is required to pinpoint the specific criteria that indicate a patient's potential response to NEURO-BLINK therapy. Regarding the clinical value of this intervention, eye closure and cornea coverage reflect the ability to remove debris from the eye and reduce lagophthalmos, and keep the cornea moist to improve vision respectively [32, 53]. Patients demonstrated a comfortable charge range similar to that of healthy subjects with all responding to charge between 0 and 3 µC except for P1. This suggests that the distal peripheral nerve is functional or that the OOM was not atrophied allowing it to respond to stimulation, and that damage is typically more proximal than the innervation point of the OOM where stimulation is given. In this charge range, lagophthalmos reduction was consistently demonstrated in all patients. NEURO-BLINK consistently elicited a complete blink suggesting its potential to longitudinally improve ocular health. In the most acute patients with idiopathic palsy (P4, P5, P6), cornea coverage was improved both in paced and myoelectric control cases from below 75% (incomplete blink) [46, 54] to a complete blink. It is worth noting that NEURO-BLINK yielded positive results in patients diagnosed up to 4 years ago. This contrasts with previous studies that observed effects in individuals diagnosed only 2–3 months earlier [26, 28]. Additionally, the consistent complete blink restoration, supported by NEURO-BLINK, has the potential to reduce corneal dryness and its symptoms such as visual acuity [55, 56]. This is an improvement with respect to previous devices that cannot consistently elicit complete blinks [28, 41] or have only been tested on healthy participants [5, 40]. In our study, we did not observe any of these clinical changes likely due to the short time of application of our assistive device. However, reducing these dry eye and corneal symptoms in the long term could lead to a difference in visual acuity of 0.05 on the LogMar scale [57], which is equivalent to about 5 years of age-related visual acuity decay [58, 59]. On the other hand, NEURO-BLINK is less effective in patients with a Bell's reflex whose cornea rolls up to be covered by the eyelid even in an incomplete blink. This was especially true for P1 whose blink was assisted from the bottom, reducing lagophthalmos from the bottom but not improving coverage (videos S1 and S2). This may be further exacerbated by the electrical stimulation itself, which has been linked to a missing Bell's reflex [60]. While NEURO-BLINK has shown proof-of-concept promising results, in this short-term study, it opens for a next long-term study, which would help us to better understand its long-term clinical effects.
The myoelectric control (with synchronized blink intention and stimulation) system was tested in the six available patients. This allowed us to assess both the effectiveness and the enhanced portability of the system (5 × 7 × 3 cm wearable stimulator and 15 × 10 × 5 cm processor with a 10 mAh power bank) compared with existing systems (25 × 20 × 10 cm or 8 × 11 × 19 cm tabletop EMG recorder, stimulator and PC computer) [40, 41]. Furthermore, NEURO-BLINK, weighing approximately 500 g, stands in contrast to the several-kilogram counterparts of other systems, making them unsuitable for portability. The EMG detection proved robust to small facial movements present during testing and demonstrated high accuracy in blink detection. The myoelectric control system was able to improve both overall eye closure and cornea coverage in all patients except the one with the most residual muscle activity (least disability). Out of these five with significant changes, two had improvement in cornea coverage with respect to the paced control condition. Although results seem slightly better in the paced control scenario, focusing on the timing of the blink required significant effort in some subjects causing highly unnatural blinks that involved other facial muscles. Consequently, eye closure in the paced control condition was sometimes higher than in the more myoelectric control condition. Despite this, the median change in percentage of eye closure and cornea coverage are both higher with the myoelectric control system than the paced control system (figure S5), suggesting a greater effect on more natural blinks. Due to these differences in blinking effort introduced by using a timer, intra-scenario comparison is more meaningful for understanding the effect of NEURO-BLINK.
Invasive stimulation has been shown to be more precise that non-invasive stimulation likely due to the proximity of active sites to the nerve [18]. While this may be a solution that could further reduce delay time and also more selectively activate OOM muscle fibers only, the requirement of a surgery make it less appropriate than non-invasive stimulation in these patients whom often heal spontaneously [2, 12] and only require assistance before that.
5. Conclusion
The NEURO-BLINK system has the potential to match the wearability of other glasses mounted blink detection systems [31, 33] and is significantly less complex than visual blink detection systems [33, 34] through its use of EMG. NEURO-BLINK is also significantly more portable, taking advantage of a wearable stimulator that can be clipped on the belt, as opposed to table top stimulators used in other studies [26, 28, 40]. It was demonstrated to be useful at improving eye closure and cornea coverage in facial palsy patients diagnosed up to 4 years ago who have residual motor and sensory facial function.
Further development of the EMG detection system can improve its robustness to noise and other movements such as facial expressions in a way that infrared systems cannot be improved [32]. Further work can also aim at reducing delay time so that blinks are perceived more naturally [28] and increasing robustness to crosstalk from other facial movements with more complex EMG decoding algorithms [35]. This could be achieved through integration of a more sensitive EMG hardware, faster processor, improved software, and more selective stimulation strategies to precisely activate OOM fibers and therefore actuate them faster. Electrical stimulation policies like the one used in NEURO-BLINK can target both muscles and nerves restoring both sensory and motor control [22]. This suggests a promising future for devices exploiting electrical stimulation to improve both facial muscle tone and restore blink function. Therefore, further miniaturization and integration into a single wearable (i.e. a pair of glasses) would allow for more long-term investigations that could further examine the effect of electrical stimulation and blink restoration on lagophthalmos overtime, overall recovery time, and improvements in ocular health.
This work takes exciting steps towards the development of an accessible myoelectric control device that could be used for real-time assistance in FP patients at home and in their daily life.
Acknowledgments
The authors are immensely grateful to the volunteers who freely donated their time to the advancement of knowledge and the betterment of the lives of those with unilateral facial paralysis.
Data availability statement
The data presented in this study are available on request from the corresponding author. The data are not publicly available due to ethical reason.
Funding
This project has received funding from the Research Council of the Cantonal Hospital Aarau (Funding Number. 1410.000.164). This project has received funding from the European Research Council (ERC) under the European Union's Horizon 2020 research and innovation program (FeelAgain Grant Agreement No. 759998), Swiss National Science Foundation (SNSF) (MOVEIT No. 197271). The project received the support of a fellowship from 'la Caixa' Foundation (ID 100010434). The fellowship code is LCF/BQ/EU20/11810072.
Author contributions
M C developed the NEURO-BLINK system, performed the experiments and the analysis, made the figures, and wrote the manuscript; L C designed and performed the experiments and the analyses, made the figures, and wrote the manuscript; A P designed the experiments, prepared the clinical documentation, performed recruitment and experiments, and reviewed the manuscript; A C designed and performed the experiments, supervised the analyses, made the figures, discussed the results and reviewed the manuscript; G V prepared the clinical documentation, discussed the results, and reviewed the manuscript; M T performed the experiments and reviewed the manuscript; M M designed and supervised the experiments, discussed the results and reviewed the manuscript; S R designed and supervised the experiments, supervised the analyses, discussed the results and reviewed the manuscript.
All authors had complete access to anonymized data. All the authors authorized submission of the manuscript, while the final submission decision was taken by the corresponding author.
Conflict of interest
Nothing to disclose.
Supplementary data (1.6 MB PDF)
Supplementary movie 1 (69.1 MB MP4) NEURO-BLINK improves real-time eye closure
Supplementary movie 2 (22.3 MB MP4) NEURO-BLINK reduces lagophthalmos