Abstract
Background
Stroke causes alterations in the sensorimotor rhythms (SMRs) of the brain. However, little is known about the influence of lesion location on the SMRs. Understanding this relationship is relevant for the use of SMRs in assistive and rehabilitative therapies, such as Brain-Computer Interfaces (BCIs)..
Methods
We reviewed current evidence on the association between stroke lesion location and SMRs through systematically searching PubMed and Embase and generated a narrative synthesis of findings.
Results
We included 12 articles reporting on 161 patients. In resting-state studies, cortical and pontine damage were related to an overall decrease in alpha (∼8–12 Hz) and increase in delta (∼1–4 Hz) power. In movement paradigm studies, attenuated alpha and beta (∼15–25 Hz) event-related desynchronization (ERD) was shown in stroke patients during (attempted) paretic hand movement, compared to controls. Stronger reductions in alpha and beta ERD in the ipsilesional, compared to contralesional hemisphere, were observed for cortical lesions. Subcortical stroke was found to affect bilateral ERD and ERS, but results were highly variable.
Conclusions
Findings suggest a link between stroke lesion location and SMR alterations, but heterogeneity across studies and limited lesion location descriptions precluded a meta-analysis.
Significance
Future research would benefit from more uniformly defined outcome measures, homogeneous methodologies, and improved lesion location reporting.
Similar content being viewed by others
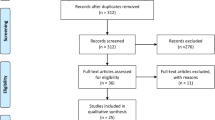
Avoid common mistakes on your manuscript.
Introduction
Stroke is a leading cause of mortality and disability worldwide with over 5.5 million deaths and 80 million survivors annually [1]. One of the most common clinical sequelae of stroke is motor impairment, which affects approximately 80% of patients and leads to difficulties with tasks of everyday life [2]. Different treatment strategies have been used to restore post-stroke motor deficits. Conventional therapeutic approaches typically focus on strength, endurance, and functional practice with the paretic limb, and have resulted in impairment decreases [3]. More intense interventions, such as constraint-induced movement therapy, have yielded better results, generalizing to increased functional independence [4].
In the past decades, Brain-Computer Interfaces (BCIs) emerged as assistive and rehabilitative technologies for individuals with profound motor disability, such as those with locked-in syndrome (LIS) or (severe) paralysis after stroke [5]. A BCI acquires brain signals in real time and extracts specific features from these signals that reflect the user’s intent. These features are then translated to digital control commands, which can operate computer applications, thereby replacing or restoring the brain’s lost natural output. To illustrate, there is increasing evidence that BCIs can be used for communication through brain-controlled typing software in individuals who have lost the ability to speak [6,7,8] or as a channel for environmental control (e.g., controlling an assistive robotic arm) for people with paralysis [9,10,11,12]. In addition, BCIs may provide users with sensory feedback about their movement attempts, through brain-signal-driven functional electrical stimulation of the muscles [13] or micro-stimulation of the somatosensory cortex surface [9].
Of particular relevance as BCI input are the sensorimotor rhythms (SMRs) present in the neuroelectrical responses recorded from the sensorimotor cortex [14]. The SMRs are generated by complex thalamocortical networks, and they are implicated in important motor functions. A prominent characteristic of SMRs is the attenuation of their amplitude during preparation for, and execution of, movement. This phenomenon, termed event-related desynchronization (ERD), occurs during imagined, passive, or active execution of a motor act and is typically detected in the power of the low-frequency band (LFB) alpha/mu (~ 8–12 Hz) component [15]. Similarly, beta rhythms (~ 13–30 Hz) exhibit a decrease prior to and during movement, which is often followed by a short-lasting amplitude enhancement upon its termination, known as event-related synchronization (ERS) or rebound [16]. Movement is also associated with increases in the high-frequency band (HFB; > 30 Hz) power in the sensorimotor cortex, which is most easily measured with implanted electrodes [17].
Previous studies have demonstrated that stroke survivors display abnormalities in the neural activity associated with rest or movement [18,19,20]. To illustrate, Kaiser et al. (2012) analyzed the ERD/ERS in stroke patients during motor imagery and execution with the paretic (i.e., affected by the stroke) hand [21]. They discovered a weaker ERD in the contralateral (i.e., ipsilesional), compared to the ipsilateral (i.e., contralesional) hemisphere, during motor imagery in patients with a higher degree of impairment. In addition, a more pronounced ERS was revealed on the lesioned side, compared to the non-lesioned side, during motor execution in individuals with greater motor deficits. Similarly, Rossiter et al. (2014) documented reduced beta desynchronization in the ipsilesional primary motor cortex (M1) during a visually guided grip task, which was also related to impairment level [22]. While this evidence suggests that the movement-related brain activity patterns undergo changes following stroke, data are often based on limited numbers of patients with various clinical presentations and heterogeneous brain damage. Hence, it remains unclear if there is a relationship between alterations in sensorimotor cortical activity and the locus of brain injury (lesion location). Understanding such a relationship is relevant for the use of SMRs within BCI settings, where these features are targeted for the generation of reliable control signals. Furthermore, increased knowledge about the role of stroke lesion location on SMR changes may guide the implementation of personalized neurorehabilitation protocols and BCI solutions [23].
Therefore, we systematically examined the literature on the association between lesion location and SMRs in stroke patients.
Methods
Protocol registration
This review was registered with the International Prospective Register of Systematic Reviews (PROSPERO) with registration number CRD42021255803 and can be accessed at https://www.crd.york.ac.uk/PROSPERO/display_record.php?ID=CRD42021255803. To enable PROSPERO to focus on COVID-19 registrations during the 2020 pandemic, the registration record of this review was automatically published exactly as submitted. The PROSPERO team has not checked eligibility.
Search strategy and selection criteria
Studies were identified by a systematic search in PubMed and Embase from inception and were extracted on May 6th, 2021, using Mesh terms and relevant keywords (the full electronic search strategy can be found in Supplementary Table 1) following the Preferred Reporting Items for Systematic Reviews and Meta-analyses (PRISMA) statement [24] and its extension PRISMA-S [25]. The completed PRISMA checklists are provided in Supplementary Tables 2 and 3, respectively.
Studies were included if they (1) were articles or articles in press; (2) reported on the association between SMR characteristics and lesion location in at least one individual with stroke; (3) used an electrophysiological technique to measure neural signals; (4) assessed LFB components relevant to motor function (i.e., alpha/mu and/or beta, or any other component alongside alpha/mu and/or beta); (5) included an eyes-open or eyes-closed resting-state or movement-related experimental condition (including active, passive, attempted, or imagined movement); (6) referred to upper limb motor function. Studies were excluded if they were (1) animal studies; (2) other systematic, narrative, or scoping reviews, or meta-analyses; (3) randomized clinical/controlled trials; (4) case reports on conditions other than stroke, (5) of irrelevant type, e.g., surveys, technical notes, conference proceedings, editorials, classifier performance studies; (6) focused on infants, children, or adolescents; (7) written in languages other than English. Importantly, we anticipated that most studies would be based on non-invasive, widely available electrophysiological monitoring systems, such as scalp EEG, which are less reliable in measuring HFB components [26, 27] and thus, decided to limit the scope of our search to LFB changes. Randomized clinical/controlled trials were regarded as ineligible because their study design is best tailored to the assessment of an intervention or treatment in the field of medicine, rather than on examining the influence of a prognostic factor of interest on certain outcome measures. To ensure that no relevant articles were omitted after extracting the search results, email alerts from both used databases were set-up to update the study inclusion and capture all publications until the present date. In addition, a hand-search in Google Scholar was carried out and reference lists of eligible papers were inspected.
One reviewer (I.K.) applied the eligibility criteria by screening titles and abstracts, and their decisions were cross-checked by a second investigator (M.V.). Both raters were involved in the full-text screening.
Data extraction
The following information was extracted from each study: (1) author(s) and year of publication; (2) study design; (3) study population with number of individuals affected by stroke and controls; (4) participants’ age; (5) participants’ demographics, as relevant to motor function (e.g., handedness and/or level of motor function of upper extremity); (6) stroke phase; (7) lesion location; (8) electrophysiological monitoring method used and number of electrode(s) or sensor(s); (9) location(s) of analyzed electrode(s) or sensor(s); (10) experimental paradigm; (11) outcome(s) of interest; (12) main study finding(s); (13) main study limitation(s). One reviewer (I.K.) extracted the data by means of a customized spreadsheet and a second rater (M.V.) verified them.
Methodological quality assessment
There is international consensus on how to appraise the quality of prognosis studies in systematic reviews [28]. To evaluate potential sources of bias, here we employed an adapted version of the Quality in Prognosis Studies (QUIPS) tool, because this is a commonly employed, evidence-based instrument with substantial inter-rater agreement. The tool includes questions linked to six bias domains, namely: (1) study participation; (2) study attrition; (3) prognostic factor measurement; (4) outcome measurement; (5) study confounding; (6) statistical analysis and reporting. It is designed so that it can be operationalized for specific study objectives, including specifying key characteristics, adding items if necessary, or omitting irrelevant items. Criteria within each domain are assessed, thereby generating an overall score for each domain as having a ‘low’, ‘moderate’, or ‘high’ risk of bias [29]. We decided to omit the original QUIPS item on study confounding because the studies we included typically focused on their respective outcomes of interest in relation to stroke lesion location as a prognostic factor, without providing a clear definition of confounders or adjusting for their potential influence. Thus, an adapted version of the QUIPS, without items addressing study confounding was used. In addition, we decided to assign ‘high’ risk of bias in the ‘study participation’ category only if stroke samples included fewer than 10 subjects, ‘moderate’ risk of bias for samples between 10 and 20, and ‘low’ risk of bias for samples larger than 20. This decision was based on our recognition of the inherent challenges in conducting research among people with stroke, particularly more severely affected individuals, including the difficulties with recruiting large numbers of subjects who are fit enough to participate, high subject attrition, and the laboriousness of performing electrophysiological assessments on patients.
Two reviewers assessed the quality of the included publications, with respect to the objectives of the current study, independently using an adapted QUIPS Excel spreadsheet (I.K. and M.V.). Each item of the five assessed categories was rated using ‘yes’, ‘partial’, or ‘no’ answers. Afterwards, sources of bias for each domain were evaluated as follows: high quality for studies with low risk of bias, moderate quality for studies with moderate risk, and low quality when there was high risk of bias, respectively. Any discrepancies between raters were resolved in discussion.
Data synthesis
We used narrative synthesis to summarize the evidence from included studies and present findings in a tabular format. Due to substantial variability in the used lesion location descriptions, frequency band definitions, techniques for measuring brain activity, experimental conditions, and outcome measures across studies, performing a meta-analysis was not feasible.
Selection of studies
A total of 11, 984 publications were screened, of which 64 were considered for full text review, and 12 met the inclusion selection criteria (Fig. 1).
PRISMA flow diagram of study selection. Note. Records were deduplicated using the EndNote reference management tool. Titles and abstracts were screened using the Rayyan software [30]. Full-text screening of eligible articles was performed using the Covidence software (Veritas Health Innovation, Melbourne, Australia, www.covidence.org)
General study characteristics
The search yielded 8 studies with retrospective case–control designs, 3 retrospective case–control design studies with longitudinal assessments, and 1 retrospective cohort study. Articles reported on 317 participants (161 patients and 156 controls) in total, of which 13 dropped-out or were lost to follow-up in the studies that included longitudinal assessments. The clinical status was acute for 62, subacute for 61, and chronic for 38 patients based on the authors’ descriptions. Stroke type was ischemic for 145, hemorrhagic for 2 (1 intracerebral and 1 pontine), and not specified for 14 subjects with stroke. Regarding the location of the lesions, 49 were cortical, 71 subcortical, 22 mixed (cortico-subcortical), 4 infratentorial (medullar or pontine), and 15 pontine. Four studies included a resting-state and 3 employed a movement attempt/execution paradigm, 4 studies involved both a rest (baseline) and a movement-related task, and 1 involved motor imagery. Concerning participant selection, 6 examinations compared one patient group with the same (cortical or subcortical) lesion location to a control group, 5 studies analyzed sub-groups of patients with different lesion locations against healthy volunteers, and 1 study reported on a single patient’s SMR features in the context of testing a BCI system for control. Table 1 summarizes the characteristics of all included studies.
Methodological quality of studies
Methodological quality assessment was performed at the included study level. Five publications fulfilled our criteria for high methodological quality, 7 were of moderate quality, and no studies were regarded as having low quality. Evaluation scores for each study can be consulted in Table 2.
A common methodological shortcoming across studies concerned the descriptions of the prognostic factor of interest – lesion location, which were overall of limited detail. This is why we could not rule out some bias in the ‘prognostic factor measurement’ quality domain. Another domain, which we considered as more heavily biased, was ‘study participation’. Reasons were the lack of reporting on key characteristics of the source population across articles, the inherent selection bias in identifying patients, and the mostly limited demographic information about the control groups. Furthermore, some investigations did not use the same method and setting of outcome measurement (e.g., the same electrophysiological monitoring technique and respective electrode selection) for all subjects, thus causing the respective ‘outcome measurement’ category to be downgraded. The response rate (‘study attrition’), analytical strategy, and presentation of results (‘statistical analysis and reporting’) were adequate in the majority of studies.
Synthesis of evidence
We grouped studies according to the experimental paradigm they used, namely resting-state or movement-related, and decided to not inform our synthesis based on the assigned methodological quality scores. One reason was the small number of eligible articles and limited available data. Second, given the challenges in conducting research with stroke patients and the substantial heterogeneity found in these populations, we regarded the information extracted from studies with lower quality scores of significant theoretical value despite a higher risk of bias. A detailed narrative synthesis of each study’s principal findings is presented in Table 3.
Resting-state studies
Four investigations included resting-state paradigms (Table 1, section Resting-state studies) [31,32,33,34]. Four additional studies involved both a rest (baseline) and a movement-related task [35,36,37,38]. However, information concerning the rest task was discussed only in three of them [35, 37, 38].
Movement-related studies
Eight publications included movement-related conditions (Table 1, section Movement-related studies) [35,36,37,38,39,40,41,42]. Regarding the effort required for motor execution, as contingent upon subjects’ degree of motor impairment, and the performed motor tasks, studies employed the following: externally paced mouse button press in subjects with clear unilateral hemiparesis [39], open/close hand movement in patients with mostly moderate deficits [35], self-paced unilateral finger lifting in subjects with mild-to-moderate hand weakness [40], finger extension in patients with mild-to-moderate weakness, clumsiness, or spasticity in the affected hand [36], supination and grasping movements in individuals with unilateral motor problems that continued for a minimum of three months after their stroke [41], attempts to carry out repetitive hand movements in an individual with LIS [37], motor imagery of left or right hand used to play a computer-based game in a tetraparetic individual with some residual right-hand movement [42], and picture-word matching task with button press responses in minor stroke patients without significant hemiparesis or upper limb weakness [38]. Studies concentrated predominantly on the motor execution, rather than on the motor preparation time frame.
Results
We performed a systematic review on the association between lesion location and SMR characteristics in stroke patients and grouped eligible studies according to the use of resting-state or movement-related experimental paradigms.
SMR Characteristics in resting-state studies
In studies that assessed SMR characteristics using resting-state paradigms, a predominant pattern was found of an overall decrease in alpha (∼8–12 Hz) and beta (~ 13–30 Hz) and increase in delta (~ 1–4 Hz) spectral power in patients with cortical or pontine injury, compared to controls, whereas findings for subcortical stroke were mixed. To illustrate, Van Wijngaarden et al. (2016) documented lower alpha and higher delta band power in acute stroke patients with cortical lesions encompassing the middle cerebral artery (MCA) territory, relative to healthy volunteers [31]. Similarly, Finnigan et al. (2016) assessed several EEG indices indicative of pathophysiological slow-wave activity, such as the delta/alpha and (delta + theta)/(alpha + beta) power ratio and discovered that they were higher across the scalp in cortical stroke patients than in controls [32]. In addition, Tangwiriyasakul and colleagues (2014) discovered absent or reduced 8–13 Hz and 15–25 Hz components in the baseline EEG in subjects with cortical damage in the ipsilesional hemisphere, which was not observed in controls [35].
Two studies reported on patients with subcortical or mixed (subcortical with cortical involvement) stroke. Fanciullacci et al. (2017) demonstrated a shift towards low-frequency activity in subacute stroke patients with mixed or subcortical lesions, compared to controls. The mixed group exhibited higher delta/alpha ratio compared to the subcortical group and lower alpha power over each hemisphere and the whole scalp [33]. In contrast, a recent study on a group of stroke patients with small lesions far from sensorimotor areas (predominantly subcortical, some mixed, and 1 cortical), and bilateral, but minor, impairments in motor dexterity and speed showed reduced baseline beta power in patients, relative to healthy volunteers, that was independent of lesion hemisphere or location [38].
Furthermore, two investigations included chronic stroke patients with LIS caused by pontine injury. Babiloni et al. (2010) showed a decrease in alpha and a general increase in delta power over central, parietal, occipital, and temporal regions in patients with ventral pons lesions, relative to controls [34]. The other study by Freudenburg and colleagues (2019) examined a single subject whose lesion obliterated the descending cortico-thalamic projections to the rostral and caudal pontine nuclei. The patient displayed an alpha oscillatory peak between 6–10 Hz, as measured with subdural ECoG electrodes over the sensorimotor area, the amplitude of which was not compared to that of controls [37].
The authors referred to several mechanisms to explain their findings. Resting-state alpha rhythms of regular amplitude denote a healthy brain prepared to process information [43], whereas thalamic-generated delta rhythms characterize slow-wave sleep [44]. The balance between these rhythms is determined by glutamatergic, cholinergic, and/or GABAergic inputs from cortical, thalamic, and brainstem modules. Disruptions to thalamo-cortical circuits, due to for instance cortical [31] or pontine [37] lesions could interfere with the interactions of these systems and drive thalamic nuclei into a mode of pathological low-frequency bursting [45]. The stroke-induced pathological oscillations may propagate beyond the primary lesion and alter the physiological state of remote, but functionally connected brain regions, consistent with the notion of diaschisis [46].
SMR characteristics in movement-related studies
To interpret the findings from movement-related studies in stroke patients, it is important to outline the ERD patterns related to healthy voluntary movement in people without motor impairment or stroke. It has been shown that a circumscribed alpha and beta desynchronization occurs approximately 2 s prior to movement onset over the contralateral Rolandic region. The desynchronization typically becomes bilaterally symmetrical promptly before, as well as during the actual movement execution [47,48,49]. Movement-related alpha and beta ERD generated from precentral areas, as well as areas posterior to the central sulcus, and in both contralateral and ipsilateral sensorimotor cortices, have also been revealed using ECoG measured from able-bodied individuals with epilepsy [17].
The studies reviewed here presented converging evidence for alpha and beta ERD attenuation over the ipsilesional hemisphere and for interference of stroke with the balance between ipsilateral and contralateral ERD. Although this appears to be a recurrent pattern, cortical and subcortical damage seem associated with different levels of effect on the SMRs.
Cortical stroke
In cases of lesions with cortical involvement, alpha and beta ERD amplitudes over the ipsilesional hemisphere associated with paretic hand movement, were substantially reduced, compared to those in controls. To illustrate, Stępień et al. (2011) found decreased alpha ERD amplitude in an acute cortical stroke group in the ipsilesional hemisphere during contralateral hand movement, relative to healthy subjects [39]. Comparable results were reported by Tangwiriyasakul et al. (2014) in the mu and beta bands, who examined patients at 1, 2, and 4 months after stroke onset and found absent or reduced mu and beta ERD on the ipsilesional side shortly after stroke onset and a recovery of this feature in the course of time [35]. In no-recovery individuals with cortical injury [35], including in the chronic stage [41], ipsilesional ERD appears to remain dampened.
In the contralesional hemisphere, larger ERD was observed than in the ipsilesional hemisphere, both in the acute [35, 39] and chronic [41] phase of stroke. During recovery, a decreasing trend in contralesional ERD modulation was revealed, whereas the opposite tendency was documented in no-recovery cases [35].
Subcortical stroke
The influence of subcortical lesions on ipsilesional ERD appeared variable. In the (sub)-acute stage of stroke, this effect ranged from no substantial differences in ERD strength in comparison with controls during contralateral hand movement [35, 38, 39] to substantially diminished alpha and beta ERD amplitudes [40], Despite these inconsistencies, the two investigations that directly compared patients with cortical and subcortical injury were similar in reporting less pronounced effects of subcortical than of cortical lesions on ERD modulation [35, 39]. These observations agreed with those of Park and colleagues for chronic stroke [41]. In their study, individuals with stroke encompassing subcortical and cortical (including M1) regions demonstrated lower beta ERD/ERS on the ipsilesional, compared to contralesional side, during active and imagined movement. In comparison, in subcortical strokes that excluded M1, and infratentorial strokes, the same authors reported an opposite trend, with more widespread contralateral activation, which resembled more closely the tendency in controls. Others reported ipsilesional ERD increases in the course of recovery [35, 40], although persistently attenuated activation in the lesioned hemisphere into the chronic phase of stroke was also observed [36, 41].
On the side opposite to the lesion, ERD was not found to be significantly affected in the acute stage post-stroke [39, 40]. Interestingly, two studies examined moderately [40] or mildly, but bilaterally impaired stroke patients with mostly subcortical lesions [38] and documented a significant decrease in beta ERS in both the lesioned and the non-lesioned hemisphere [38, 40], indicating bilaterally altered Rolandic beta band activity. In later stages after injury, Tangwiriyasakul et al. discovered a pattern of decreasing contralesional ERD with reappearance of ipsilesional modulation in patients with better recovery [35], whereas Gerloff et al. noted larger ipsilateral activation in task-related power during movement of the affected limb in patients compared to controls [36].
Pontine stroke
Concerning pontine stroke, mixed evidence was revealed. To illustrate, Freudenburg et al. (2019) demonstrated minimal alpha modulation related to attempted hand movement in an individual with LIS due to brainstem stroke and excluded impaired task performance as a likely reason. Beta power changes linked to motor execution were practically absent [37]. On the other hand, Höhne and colleagues (2014) tested the applicability of an EEG-based BCI system for control that could be driven by a wide range of spectral features, such as mu, beta, and delta desynchronization, or beta synchronization. While no consistent ERD component was found in the alpha band, beta ERS was determined as a reliable EEG feature, which enabled a tetraparetic individual to gain control over the BCI. Of note, the beta synchronization displayed differing spatial distribution across sessions and regular calibration of the system was needed to maintain reliable decoding [42]. Interestingly, in contrast to the results from these two investigations, the infratentorial lesion group assessed by Park et al. (2016) whose lesions comprised the medial medulla or pons, exhibited similar beta band ERD and ERS power changes to controls with a stronger ipsilesional than contralesional beta desynchronization [41]. The apparent discrepancy could be related to the degree of motor impairment (moderate for the participants of Park et al. [41] versus severe for those of Freudenburg [37] and Höhne [42]). Nevertheless, data from these examinations are based on six patients and any firm conclusions remain to be confirmed in larger samples.
In summary, we established a common trend of global resting-state decreases in alpha power in individuals with cortical or pontine injury. Notably, the number of studies investigating resting-state phenomena was low, subcortical stroke in particular was rarely addressed, and the lesion location descriptions varied in detail. Therefore, further research is needed to gain full understanding of the resting-state SMRs. In movement-related studies, both cortical and subcortical strokes resulted in alpha and beta ERD attenuation over the contralateral hemisphere during paretic hand movement in patients relative to controls. Cortical damage was associated with significant ipsilesional decreases in alpha and beta ERD. Subcortical injury, on the other hand, appeared to interfere with ERD to a lesser extent than cortical stroke in direct comparisons between these populations, but results across studies on subcortical populations alone were highly variable. Regarding pontine strokes, results were inconclusive and based on a small number of cases, so more research is necessary to support our interpretations. Figure 2 provides a visual summary of the main tendencies demonstrated across studies.
Discussion
An important contribution of this study is that, to the best of the authors’ knowledge, it represents the first systematic examination of the association between lesion location and SMR characteristics in stroke patients. Other strong aspects involve the exhaustive methodological approach with a reproducible search strategy, as well as the pre-registration and adherence to a published protocol and the PRISMA guidelines.
A limitation of this review is the substantial variability across publications. This prevented us from performing a formal meta-analysis, which would have enabled a more precise delineation of the relationship between lesion location and SMRs. Indeed, lesion location reporting was inconsistent, and descriptions were insufficiently detailed with most authors categorizing lesions only as either cortical or subcortical. In addition, some studies assessed a single group of patients with the same stroke damage, while others reported on multiple sub-groups of patients with different lesion locations. While common signal alterations were demonstrated in patients relative to controls, the analysis of subsets of patients according to lesion location revealed important differences in the resulting SMRs. Another source of variation in this review was the focus on either interhemispheric differences or comparisons between groups, as well as the sole examination of paretic hand movement in some investigations and contralateral patterns of both hands in others. Furthermore, there was large heterogeneity in patients’ motor deficit at stroke onset, which also relates to the type of movement and level of effort required to comply with the performed motor tasks in the movement paradigm studies. This leaves a possibility that some of the presented findings could be driven by subjects with poorer motor skill or recovery. Patients with very limited-to-no residual ability in their affected limb may rely on different mechanisms during overt motor performance, compared to healthy volunteers or less severely affected individuals, such as for instance engaging in motor imagery alongside the execution component [50], which conceptually could result in different cortical activation patterns. Thus, a consideration of lesion topography, as well as level of motor impairment, may provide a more exhaustive account of our results. In this regard, the findings of Kulasingham et al. [38] of decreased beta ERD and ERS in unilateral minor stroke patients with mild bilateral motor impairment, independent of lesion location, are worth mentioning. The authors argued that the observed bilateral deficits could reflect a more global stroke-induced disruption, not tied to lesion location, involving bilateral excitation/inhibition imbalance, bilateral modulation of motor planning and execution [51, 52], and disturbance of global network connectivity. Interestingly, bilateral cortical abnormalities, independent of lesion location, have previously been documented using functional magnetic resonance imaging (fMRI) following subcortical stroke [53,54,55]. In addition, the dynamic patterns of the cortical changes have been linked to functional connectivity between cortical motor areas remote from the infarct [54, 55] and parieto-frontal networks integrating visuomotor information [56], as well as impairment of specific subsets of the corticospinal tract (CST) and patients’ corresponding degree of recovery [53]. Such results suggest the possibility that lesion location is a determinant of SMR changes in the context of greater motor disability, including hemiparetic symptomatology, especially if the motor cortex is damaged, whereas a more globally altered connectivity could be the mechanism underlying minor strokes that lack severe motor disability and cortical features. These findings encourage the further exploration of the relationship between stroke lesion location and milder forms of motor impairment in future studies, with motor spasticity being a particularly interesting symptom, given its relevance to long-term clinical recovery [57], as well as contrasting reports regarding its relationship to ipsilesional ERD [58]. Another promising direction for future research is to complement electrophysiological measurements with lesion network mapping and functional connectivity analyses in order to identify the broader connectivity patterns of distinct lesion locations and their association with different clinical phenotypes [57]. This can provide greater insight into the impact of different lesion locations on both local and global properties of the intrinsic motor system [56, 57] and unravel the mechanisms by which even remotely localized lesions can directly impair the integrity of the input and output fibers connected with the sensorimotor cortex [53] and potentially impact subjects’ ability for volitional SMR modulation [56]. Lastly, the majority of studies suffered from inadvertent selection bias, did not offer exhaustive descriptions of their samples, particularly of the controls, and did not systematically identify potential confounders or formally adjust for them in their analyses. Including larger and more homogeneous patient populations, more detailed demographic information, as well as details on potential study confounders, and reporting anatomical lesion location more precisely in future examinations would help determine the robustness of the presented results. It would also be beneficial to standardize frequency band definitions and outcome measures in the field to enable more comprehensible cross-study comparison and synthesis of future research findings, and to facilitate research translation into clinical practice.
To summarize, this systematic examination partially supports the notion that SMR patterns in stroke patients differ according to lesion location in that cortical involvement in a lesion seems associated with more severe SMR alterations. In addition, recovery of ERD over time following stroke appears to relate to improvements in motor function. In line with this premise, literature has suggested a link between the strength and laterality of the ipsilesional desynchronization in the initial months after injury and better motor outcomes [21]. Therefore, ERD modulation at the acute and subacute post-stroke stages may be investigated as a potential motor recovery biomarker or as a tool to stratify patients who may benefit more from (certain) rehabilitative therapies. Furthermore, the revealed ipsilesional ERD reductions, particularly in patients with cortical damage, could inform the design of BCIs that can promote motor recovery by guiding patients to activate their lesioned hemisphere and prevent learned non-use [59]. Future investigations should clarify the extent to which BCI interventions can support recovery of ipsilesional ERD modulation and if so, what causal effects could be expected on functional outcomes. Our findings also set a foundation for developing more effective and personalized BCIs. For instance, BCIs may use neuroelectrical signals extracted from the contralesional hemisphere when decoding movement intention of patients with cortical damage to improve decoding performance. Another future step in developing patient-tailored treatment approaches and BCI solutions is a further exploration of how factors, such as differences in lesion size, severity, and distance to sensorimotor areas [38], brain structure [60] and functional network integrity [38, 56], or cognitive strategies [61], may influence subjects’ ability to modulate their brain activity.
Conclusion
We systematically reviewed the literature on the association between lesion location and SMRs in stroke patients. Despite the heterogeneity across studies and their methodological limitations, the data suggest that different lesion locations are associated with distinct levels of alterations in resting-state and movement-related sensorimotor signals. Based on our investigation, we argue that damage to cortical structures, particularly the motor cortex, relates to the severity of SMR changes following stroke, whereas subcortical damage may impact SMR modulation due to alterations in functional network integrity. We underscore the need of future examinations to address the shortcomings of current research to enable further elucidation of the role of lesion location on SMRs. We envision that this can contribute to patient stratification for neurorehabilitation protocols and improve our understanding of patients’ likelihood to benefit from assistive and rehabilitative BCIs.
Data availability
Not applicable.
Abbreviations
- BCI:
-
Brain-Computer Interface
- ECoG:
-
Electrocorticography
- EEG:
-
Electroencephalography
- EMBASE:
-
Excerpta Medica Database
- ERD:
-
Event-related desynchronization
- ERS:
-
Event-related synchronization
- HFB:
-
High-frequency band
- LFB:
-
Low-frequency band
- M1:
-
Primary motor cortex
- MEG:
-
Magnetoencephalography
- PRISMA:
-
Preferred Reporting Items for Systematic Reviews and Meta-Analyses
- PROSPERO:
-
International Prospective Register of Systematic Reviews
- QUIPS:
-
Quality in Prognosis Studies
- SMRs:
-
Sensorimotor rhythms
References
Feigin VL, Stark BA, Johnson CO, Roth GA, Bisignano C, Abady GG, ... Hamidi S (2021) Global, regional, and national burden of stroke and its risk factors, 1990–2019: A systematic analysis for the Global Burden of Disease Study 2019. Lancet Neurol 20(10):795–820. https://doi.org/10.1016/S1474-4422(21)00252-0
Winstein CJ, Stein J, Arena R, Bates B, Cherney LR, Cramer SC, … Zorowitz RD (2016) Guidelines for adult stroke rehabilitation and recovery: a guideline for healthcare professionals from the American Heart Association/American Stroke Association. Stroke 47(6): e98-e169. https://doi.org/10.1161/STR.0000000000000098
Kollen B, Kwakkel G, Lindeman E (2006) Functional recovery after stroke: a review of current developments in stroke rehabilitation research. Rev Recent Clin Trials 1(1):75–80. https://doi.org/10.2174/157488706775246111
Langhorne P, Coupar F, Pollock A (2009) Motor recovery after stroke: a systematic review. Lancet Neurol 8(8):741–754. https://doi.org/10.1016/S1474-4422(09)70150-4
Wolpaw JR, Millán JDR, Ramsey NF (2020) Brain-computer interfaces: Definitions and principles. Handb Clin Neurol 168:15–23. https://doi.org/10.1016/B978-0-444-63934-9.00002-0
Jarosiewicz B, Sarma AA, Bacher D, Masse NY, Simeral JD, Sorice B, ... Hochberg LR (2015) Virtual typing by people with tetraplegia using a self-calibrating intracortical brain-computer interface. Sci Transl Med 7(313): 313ra179–313ra179. https://doi.org/10.1126/scitranslmed.aac7328
Oxley TJ, Yoo PE, Rind GS, Ronayne SM, Lee CS, Bird C, ... Opie NL (2021) Motor neuroprosthesis implanted with neurointerventional surgery improves capacity for activities of daily living tasks in severe paralysis: first in-human experience. J Neurointerventional Surg 13(2):102–108. https://doi.org/10.1136/neurintsurg-2020-016862
Vansteensel MJ, Pels EG, Bleichner MG, Branco MP, Denison T, Freudenburg ZV, ... Ramsey NF (2016) Fully implanted brain–computer interface in a locked-in patient with ALS. N Engl J Med 375(21):2060–2066. https://doi.org/10.1056/NEJMoa1608085
Flesher SN, Downey JE, Weiss JM, Hughes CL, Herrera AJ, Tyler-Kabara EC, ... Gaunt RA (2021) A brain-computer interface that evokes tactile sensations improves robotic arm control. Science, 372(6544):831–836. https://doi.org/10.1126/science.abd0380
Hochberg LR, Bacher D, Jarosiewicz B, Masse NY, Simeral JD, Vogel J, Haddadin S et al (2012) Reach and grasp by people with tetraplegia using a neurally controlled robotic arm. Nature 485(7398):372–375. https://doi.org/10.1038/nature11076
Onose G, Grozea C, Anghelescu A, Daia C, Sinescu CJ, Ciurea AV, Spircu T et al (2012) On the feasibility of using motor imagery EEG-based brain–computer interface in chronic tetraplegics for assistive robotic arm control: a clinical test and long-term post-trial follow-up. Spinal cord 50(8):599–608. https://doi.org/10.1038/sc.2012.14
Wang W, Collinger JL, Degenhart AD, Tyler-Kabara EC, Schwartz AB, Moran DW, Weber DJ et al (2013) An electrocorticographic brain interface in an individual with tetraplegia. PloS one 8(2):e55344. https://doi.org/10.1371/journal.pone.0055344
Pfurtscheller G, Müller-Putz GR, Pfurtscheller J, Rupp R (2005) EEG-based asynchronous BCI controls functional electrical stimulation in a tetraplegic patient. EURASIP J Adv Signal Process 2005(19):1–4. https://doi.org/10.1155/ASP.2005.3152
Pfurtscheller G, McFarland DJ (2012) 13| BCIS that use sensorimotor rhythms. Brain-computer interfaces: principles and practice. p 227
Pfurtscheller G, Brunner C, Schlögl A, Da Silva FL (2006) Mu rhythm (de) synchronization and EEG single-trial classification of different motor imagery tasks. Neuroimage 31(1):153–159. https://doi.org/10.1016/j.neuroimage.2005.12.003
Neuper C, Pfurtscheller G (2001) Event-related dynamics of cortical rhythms: frequency-specific features and functional correlates. Int J Psychophysiol 43(1):41–58. https://doi.org/10.1016/S0167-8760(01)00178-7
Crone NE, Miglioretti DL, Gordon B, Sieracki JM, Wilson MT, Uematsu S, Lesser RP (1998) Functional mapping of human sensorimotor cortex with electrocorticographic spectral analysis. I. Alpha and beta event-related desynchronization. Brain: J Neurol 121(12):2271–2299
Giaquinto S, Cobianchi A, Macera F, Nolfe G (1994) EEG recordings in the course of recovery from stroke. Stroke 25(11):2204–2209. https://doi.org/10.1161/01.STR.25.11.2204
Parkkonen E, Laaksonen K, Piitulainen H, Pekkola J, Parkkonen L, Tatlisumak T, Forss N (2017) Strength of~ 20-Hz rebound and motor recovery after stroke. Neurorehabil Neural Repair 31(5):475–486. https://doi.org/10.1177/154596831668879
Thibaut A, Simis M, Battistella LR, Fanciullacci C, Bertolucci F, Huerta-Gutierrez R, ... Fregni F (2017) Using brain oscillations and corticospinal excitability to understand and predict post-stroke motor function. Front Neurol 8:187. https://doi.org/10.3389/fneur.2017.00187
Kaiser V, Daly I, Pichiorri F, Mattia D, Müller-Putz GR, Neuper C (2012) Relationship between electrical brain responses to motor imagery and motor impairment in stroke. Stroke 43(10):2735–2740. https://doi.org/10.1161/STROKEAHA.112.665489
Rossiter HE, Boudrias MH, Ward NS (2014) Do movement-related beta oscillations change after stroke? J Neurophysiol 112(9):2053–2058. https://doi.org/10.1152/jn.00345.2014
Selles RW, Andrinopoulou ER, Nijland RH, Van Der Vliet R, Slaman J, Van Wegen EE, ... Kwakkel G (2021) Computerised patient-specific prediction of the recovery profile of upper limb capacity within stroke services: the next step. J Neurol Neurosurg Psychiatry 92(6):574–581. https://doi.org/10.1136/jnnp-2020-324637
Page MJ, Moher D, Bossuyt PM, Boutron I, Hoffmann TC, Mulrow CD, ... McKenzie JE (2021) PRISMA 2020 explanation and elaboration: updated guidance and exemplars for reporting systematic reviews. BMJ, 372. https://doi.org/10.1136/bmj.n160
Rethlefsen ML, Kirtley S, Waffenschmidt S, Ayala AP, Moher D, Page MJ, Koffel JB (2021) PRISMA-S: an extension to the PRISMA statement for reporting literature searches in systematic reviews. Syst Rev 10(1):1–19. https://doi.org/10.1186/s13643-020-01542-z
Darvas F, Scherer R, Ojemann JG, Rao RP, Miller KJ, Sorensen LB (2010) High gamma mapping using EEG. Neuroimage 49(1):930–938. https://doi.org/10.1016/j.neuroimage.2009.08.041
Nottage JF, Horder J (2015) State-of-the-art analysis of high-frequency (gamma range) electroencephalography in humans. Neuropsychobiology 72(3–4):219–228. https://doi.org/10.1159/000382023
Hayden JA, Côté P, Bombardier C (2006) Evaluation of the quality of prognosis studies in systematic reviews. Ann Intern Med 144(6):427–437. https://doi.org/10.7326/0003-4819-144-6-200603210-00010
Hayden JA, van der Windt DA, Cartwright JL, Côté P, Bombardier C (2013) Assessing bias in studies of prognostic factors. Ann Intern Med 158(4):280–286. https://doi.org/10.7326/0003-4819-158-4-201302190-00009
Ouzzani M, Hammady H, Fedorowicz Z, Elmagarmid A (2016) Rayyan—a web and mobile app for systematic reviews. Syst Rev 5(1):1–10. https://doi.org/10.1186/s13643-016-0384-4
Van Wijngaarden JB, Zucca R, Finnigan S, Verschure PF (2016) The impact of cortical lesions on thalamo-cortical network dynamics after acute ischaemic stroke: a combined experimental and theoretical study. PLoS Computat Biol 12(8):048. https://doi.org/10.1371/journal.pcbi.1005048
Finnigan S, Wong A, Read S (2016) Defining abnormal slow EEG activity in acute ischaemic stroke: Delta/alpha ratio as an optimal QEEG index. Clin Neurophysiol 127(2):1452–1459. https://doi.org/10.1016/j.clinph.2015.07.014
Fanciullacci C, Bertolucci F, Lamola G, Panarese A, Artoni F, Micera S, ... Chisari C (2017) Delta power is higher and more symmetrical in ischemic stroke patients with cortical involvement. Front Human Neurosci 11:385. https://doi.org/10.3389/fnhum.2017.0038
Babiloni C, Pistoia F, Sarà M, Vecchio F, Buffo P, Conson M, ... Rossini PM (2010) Resting state eyes-closed cortical rhythms in patients with locked-in-syndrome: an EEG study. Clin Neurophysiol 121(11):1816–1824. https://doi.org/10.1016/j.clinph.2010.04.027
Tangwiriyasakul C, Verhagen R, Rutten WL, van Putten MJ (2014) Temporal evolution of event-related desynchronization in acute stroke: a pilot study. Clin Neurophysiol 125(6):1112–1120. https://doi.org/10.1016/j.clinph.2013.10.047
Gerloff C, Bushara K, Sailer A, Wassermann EM, Chen R, Matsuoka T, ... Hallett M (2006) Multimodal imaging of brain reorganization in motor areas of the contralesional hemisphere of well recovered patients after capsular stroke. Brain 129(3):791–808. https://doi.org/10.1093/brain/awh713
Freudenburg ZV, Branco MP, Leinders S, Vijgh BHVD, Pels EG, Denison T, ... Vansteensel MJ (2019) Sensorimotor ECoG signal features for BCI control: a comparison between people with locked-in syndrome and able-bodied controls. Front Neurosci 13:1058. https://doi.org/10.3389/fnins.2019.01058
Kulasingham JP, Brodbeck C, Khan S, Marsh EB, Simon JZ (2022) Bilaterally reduced rolandic beta band activity in minor stroke patients. Front Neurol 13. https://doi.org/10.3389/fneur.2022.819603
Stępień M, Conradi J, Waterstraat G, Hohlefeld FU, Curio G, Nikulin VV (2011) Event-related desynchronization of sensorimotor EEG rhythms in hemiparetic patients with acute stroke. Neurosci Lett 488(1):17–21. https://doi.org/10.1016/j.neulet.2010.10.072
Tang CW, Hsiao FJ, Lee PL, Tsai YA, Hsu YF, Chen WT, ... Lee IH (2020) Β-Oscillations reflect recovery of the paretic upper limb in subacute stroke. Neurorehabil Neural Repair 34(5):450–462. https://doi.org/10.1177/1545968320913502
Park W, Kwon GH, Kim YH, Lee JH, Kim L (2016) EEG response varies with lesion location in patients with chronic stroke. J Neuroeng Rehabil 13(1):1–10. https://doi.org/10.1186/s12984-016-0120-2
Höhne J, Holz E, Staiger-Sälzer P, Müller KR, Kübler A, Tangermann M (2014) Motor imagery for severely motor-impaired patients: evidence for brain-computer interfacing as superior control solution. PloS one 9(8):854. https://doi.org/10.1371/journal.pone.0104854
Brunia CHM (1999) Neural aspects of anticipatory behavior. Acta Physiol (Oxf) 101(2–3):213–242. https://doi.org/10.1016/S0001-6918(99)00006-2
Steriade M (2003) The corticothalamic system in sleep. Front Biosci-Landmark 8(4):878–899. https://doi.org/10.2741/1043
Llinás RR, Steriade M (2006) Bursting of thalamic neurons and states of vigilance. J Neurophysiol 95(6):3297–3308. https://doi.org/10.1152/jn.00166.2006
Feeney DM, Baron JC (1986) Diaschisis. Stroke 17(5):817–830
Pfurtscheller G (1997) EEG event-related desynchronization (ERD) and synchronization (ERS). Electroencephalogr Clin Neurophysiol 1(103):26
Pfurtscheller G, Aranibar A (1979) Evaluation of event-related desynchronization (ERD) preceding and following voluntary self-paced movement. Electroencephalogr Clin Neurophysiol 46(2):138–146. https://doi.org/10.1016/0013-4694(79)90063-4
Pfurtscheller G, Berghold A (1989) Patterns of cortical activation during planning of voluntary movement. Electroencephalogr Clin Neurophysiol 72(3):250–258. https://doi.org/10.1016/0013-4694(89)90250-2
Dodakian L, Stewart JC, Cramer SC (2014) Motor imagery during movement activates the brain more than movement alone after stroke: a pilot study. J Rehabil Med 46(9):843. https://doi.org/10.2340/16501977-1844
Graziadio S, Tomasevic L, Assenza G, Tecchio F, Eyre JA (2012) The myth of the ‘unaffected’ side after unilateral stroke: Is reorganisation of the non-infarcted corticospinal system to re-establish balance the price for recovery? Exp Neurol 238(2):168–175. https://doi.org/10.1016/j.expneurol.2012.08.031
Schaefer SY, Haaland KY, Sainburg RL (2007) Ipsilesional motor deficits following stroke reflect hemispheric specializations for movement control. Brain 130(8):2146–2158. https://doi.org/10.1093/brain/awm145
Liu J, Wang C, Qin W, Ding H, Peng Y, Guo J, ... Yu C (2023) Cortical structural changes after subcortical stroke: Patterns and correlates. Hum Brain Mapp 44(2):727–743. https://doi.org/10.1002/hbm.26095
Sharma N, Baron JC, Rowe JB (2009) Motor imagery after stroke: relating outcome to motor network connectivity. Ann Neurol 66(5):604–616. https://doi.org/10.1002/ana.21810
Grefkes C, Nowak DA, Eickhoff SB, Dafotakis M, Küst J, Karbe H, Fink GR (2008) Cortical connectivity after subcortical stroke assessed with functional magnetic resonance imaging. Ann Neurol 63(2):236–246. https://doi.org/10.1002/ana.21228
Buch ER, ModirShanechi A, Fourkas AD, Weber C, Birbaumer N, Cohen LG (2012) Parietofrontal integrity determines neural modulation associated with grasping imagery after stroke. Brain 135(2):596–614. https://doi.org/10.1093/brain/awr331
Qin Y, Qiu S, Liu X, Xu S, Wang X, Guo X, ... Li H (2022) Lesions causing post-stroke spasticity localize to a common brain network. Front Aging Neurosci 14. https://doi.org/10.3389/fnagi.2022.1011812
Chen S, Shu X, Jia J, Wang H, Ding L, He Z, ... Zhu X (2022) Relation between sensorimotor rhythm during motor attempt/imagery and upper-limb motor impairment in stroke. Clinical EEG and Neuroscience, 53(3):238–247. https://doi.org/10.1177/15500594211019917
Daly JJ, Wolpaw JR (2008) Brain–computer interfaces in neurological rehabilitation. Lancet Neurol 7(11):1032–1043. https://doi.org/10.1016/S1474-4422(08)70223-0
Allison BZ, Neuper C (2010) Could anyone use a BCI? Brain-computer interfaces. Springer, London, pp 35–54. https://doi.org/10.1007/978-1-84996-272-8_3
Kober SE, Witte M, Ninaus M, Neuper C, Wood G (2013) Learning to modulate one’s own brain activity: the effect of spontaneous mental strategies. Front Hum Neurosci 7:695. https://doi.org/10.3389/fnhum.2013.00695
Acknowledgements
The authors would like to acknowledge the contribution of Utrecht University librarian Drs. Paulien H. Wiersma who helped improve the developed systematic literature search strategy, a key aspect of this work. This research was funded by the National Institute on Deafness and Other Communication Disorders (U01DC016686). The funding source was not involved in the design or writing of this manuscript.
Author information
Authors and Affiliations
Contributions
Ivana Kancheva: Conceptualization, Design, Methodology, including step-by-step study selection, (title and abstract screening, and full-text screening), and data extraction, Data interpretation, Data visualization using tables and figures, Writing – original draft preparation, Writing – review, editing, and final approval.
Sandra M.A. van der Salm: Conceptualization, Writing – review, editing, and final approval.
Nick F. Ramsey: Conceptualization, Writing – review, editing, and final approval.
Mariska J. Vansteensel: Conceptualization, Design, Methodology, including cross-validation of study selection and data extraction, Data interpretation, Writing – review, editing, and final approval.
Corresponding author
Ethics declarations
Ethics approval and consent
Not applicable since no participants were recruited during the execution of this study.
Conflict of interest
NF Ramsey receives consulting fees from the Wyss Center in Switzerland, and owns stock in BrainCarta, a startup clinical fMRI company. MJ Vansteensel is member of the advisory council of G3ICT. MJ Vansteensel is, and NF Ramsey was, a Board member of the International BCI Society. The other authors have no relevant financial or non-financial interests to disclose.
Additional information
Publisher's note
Springer Nature remains neutral with regard to jurisdictional claims in published maps and institutional affiliations.
Supplementary information
Below is the link to the electronic supplementary material.
Rights and permissions
Open Access This article is licensed under a Creative Commons Attribution 4.0 International License, which permits use, sharing, adaptation, distribution and reproduction in any medium or format, as long as you give appropriate credit to the original author(s) and the source, provide a link to the Creative Commons licence, and indicate if changes were made. The images or other third party material in this article are included in the article's Creative Commons licence, unless indicated otherwise in a credit line to the material. If material is not included in the article's Creative Commons licence and your intended use is not permitted by statutory regulation or exceeds the permitted use, you will need to obtain permission directly from the copyright holder. To view a copy of this licence, visit http://creativecommons.org/licenses/by/4.0/.
About this article
Cite this article
Kancheva, I., van der Salm, S.M.A., Ramsey, N.F. et al. Association between lesion location and sensorimotor rhythms in stroke – a systematic review with narrative synthesis. Neurol Sci 44, 4263–4289 (2023). https://doi.org/10.1007/s10072-023-06982-8
Received:
Accepted:
Published:
Issue Date:
DOI: https://doi.org/10.1007/s10072-023-06982-8