Effects of tea residues-fermented feed on production performance, egg quality, antioxidant capacity, caecal microbiota, and ammonia emissions of laying hens
- 1Leshan Academy of Agriculture Science, Leshan, Sichuan, China
- 2College of Life Science and Engineering, Southwest University of Science and Technology, Mianyang, Sichuan, China
- 3Department of Animal Science and Technology, Chongqing Three Gorges Vocational College, Wanzhou, China
This study was to assess the effects of tea residues-fermented feed (TR-fermented feed) on production performance, egg quality, serum antioxidant capacity, caecal microbiota, and ammonia emissions of laying hens. A total of 1,296 Lohmann laying hens have randomly distributed four groups with six parallels and fed with diets TR-fermented feed at the rates of 0 (control), 1, 3, and 5%. The inclusion of 1% (TR)-fermented feed resulted in a significant increase in egg-laying rate and average egg weight of birds, and a reduction in the feed-to-egg ratio when compared to the control group (p < 0.05). The addition of 1 and 3% of (TR)-fermented feed significantly improved the Haugh unit of eggs (p < 0.05). The eggshell thickness was observed to increase by almost one-fold upon the inclusion of 3 and 5% (TR)-fermented feed in the basal diet (p < 0.05). The supplementation of 3% (TR)-fermented feed significantly increased the content of methionine, tyrosine, proline, essential amino acids (EAA), alpha linoleic acid (C18:3n6), docosanoic acid (C22:0), docosahexaenoic acid (C22:6n3), twenty-three carbonic acids (C23:0), ditetradecenoic acid (C24:1) and total omega-3 polyunsaturated fatty acids (∑ω-3 PUFA) in the eggs (p < 0.05). The addition of a certain amount of (TR)-fermented feed can enhance the activity of glutathione peroxidase (GSH-PX) and superoxide dismutase (SOD) in chicken serum, and reduce the level of malondialdehyde (MDA) (p < 0.05). The ammonia concentration in the hen house of laying hens in the treatment groups decreased significantly (p < 0.05). Bacteroidetes and Firmicutes, the main phyla in the cecal bacterial community, were differentially abundant in each group, comprising greater than 55 and 33%, respectively. Collectively, this research indicates that (TR)-fermented feed supplementation improves the performance of laying hens and reduces ammonia emissions and can be used in industry-scale layer production.
1. Introduction
Fermented feed is used in poultry production to improve performance, immunity, and intestinal health, and to reduce the use of antibiotics (1–3). It is because the microbial fermentation of feed can reduce or eliminate the contents of toxic and harmful substances in raw materials effectively, while increasing the feed ingredients nutritional value and improving feed quality, thereby promoting the health of poultry (4–6). Probiotics such as Lactobacillus, Saccharomyces, and Bacillus subtilis have been widely used in feed fermentation, but it has been shown that the amount of protease secreted by the strains through simple microbial fermentation alone is low and cannot meet the actual production demand (7). Bacterial and enzyme co-fermented feed refers to the addition of a certain amount of exogenous compound enzymes and probiotics for joint fermentation, thus degrading the anti-nutritional ingredients in the feed, regulating the bitterness of the feed, improving the palatability of the feed, compensating for the lack of enzyme production and poor enzymatic taste of a single microbial fermentation, promoting animal feeding, improving feed utilization and nutritional value (8–10).
Tea residues are the by-products formed after the deep processing of tea. Although tea residues are the by-products of tea after extraction, they still contain more tea polysaccharides, theanine, tea polyphenols and other bioactive substances, which have antibacterial, antioxidant and anti-cancer functions (11–15). The use of tea residues in animal products not only improves the production performance, antioxidant capacity, and immunity of animals, but also avoids the pollution of the environment when tea residues are discarded in large quantities (16, 17). Adding tea powder or tea polyphenols to the feed can enhance the immunity and intestinal health of laying hens, increase their egg production rate, reduce cholesterol content in eggs, and improve egg quality (18–20). Solid-state fermentation of tea residues resulted in a significant increase in crude protein (CP), reducing sugar, and cellulose activity by 19.32, 39.5, and 33.3%, respectively, compared to their levels before fermentation (21). It was found that fermented tea pomace could improve the growth capacity and gut morphology of fattening pigs via improving nutrient utilization and intestinal digestive enzymes activities, and improve pork qualities via improving antioxidant properties of fattening pigs (17), and it has also been found that fermented tea residues can improve product performance and resistance to A. hydrophila in Micropterus Salmoides (16). However, in poultry farming, most of the research has been conducted on the use of tea residues as feed ingredients or functional additives. Therefore, the major objective of the present research was to use tea residues as part of a fermented feed by using co-fermentation with bacteria and enzymes. Tea pomace fermented feed has been supplemented in the diet of laying hens to investigate the effect on production capacity, egg quality, anti-oxidant capacity of serum, gut microbial composition, and ammonia emission from the poultry house.
2. Materials and methods
2.1. Diets
Table 1 displays the composition and nutrient levels of the basal diet, which was prepared in accordance with the Chinese chicken feeding standard (NY/T 33-2004) (22). Tea residues which include tea leaves and tea stalks were provided by Sichuan Baiyue Tea Industry. The probiotic used for fermentation contains B. subtilis ≥ 1.5 × 109 CFU/g, L. plantarum ≥ 1.0 × 109 CFU/g were provided by the Leshan Academy of Agricultural Sciences, the enzymes used for fermentation contains β-mannanase ≥50 U/g, β-glucanase ≥3,000 U/g, cellulase ≥1,000 U/g, and xylanase ≥500 U/g were purchased from market, and product standard No. Q/12JX 4450-2019. Our fermentation procedure is to crush the feed ingredients for fermented feed, weigh all the ingredients accurately according to the ratio in Table 2 and place them in fermentation bags containing a one-way breathing valve, mix them thoroughly and then place them at 36°C for 96 h.
2.2. Animals
In this study, a total of 1,296 healthy Lohmann laying hens at 34 weeks of age were assigned randomly to 4 dietary groups (T0–T3), each with 6 parallels of 54 laying hens. Three chickens were raised in cages (47 cm × 45 cm × 44 cm). The chickens were hand-fed three times a day (06:30 am, 11:30 am, and 4:30 pm) for the duration of the experiment. The hens have access to free drinking water with 16 h of continuous light per day. T0 (control group) fed the basal diet, T1, T2 and T3 replaced 1, 3, and 5% of the basal diet with tea residues-fermented feed, respectively. The hens were acclimated to the experimental conditions for 7 days and the experiment lasted 6 weeks.
2.3. Sample collection
At the end of the trial, randomly collected 6 eggs per replicate, six chickens were selected randomly from each treatment (one per replicate) and fasted for 12 h before sampling. The blood was collected from the wing vein using a blood collection needle, and 5 mL of it was transferred to a vacuum tube. The tube was kept at room temperature to allow for serum separation, after which it was centrifuged at 3,000 × g for 30 min at 4°C. The serum was then stored at a temperature of −20°C. The cecum was collected in a sterilized centrifuge tube. Buffered peptone broth was used to homogenize each portion, which was then mixed with 10% sucrose and divided into aliquots. The aliquots were frozen at a temperature of −80°C (23).
2.4. Production performance
Throughout the trial, daily records were made of the number of feed intake, eggs produced, and total egg weight for each replicate. Subsequently, the egg-laying rate, average egg weight, and feed-to-egg ratio were determined for the periods of 1–22 days and 22–42 days.
Egg-laying rate (%) = (number of egg-laying/number of chickens) × 100
Average egg weight = total egg weight / number of eggs laid
Feed intake = feeding amount–surplus
Feed-to-egg ratio = feed intake/total eggs weight
2.5. Eggs quality
The collected eggs were tested using an automatic quality analyzer (TOUHOKU RHYTHM CO., LTD EMT-7300) for egg yolk color, albumen height and Haugh units. The eggshell thickness was measured using the YN-25L egg quality analyzer, which measured the eggshell at three different locations and took the average value as the eggshell thickness of the egg. To calculate the egg shape index, vernier calipers were used to measure the long and short diameters of the eggs.
Egg shape index = long diameter/short diameter
2.6. Determination of amino acids and fatty acids in eggs
Transfer the eggs to a clean small beaker, mix the egg yolk and egg white thoroughly, weigh 0.1 g of the sample and place it in a hydrolysis tube. Add 6 mol/L of concentrated hydrochloric acid to 10 mL, seal the tube, and place it in a constant temperature drying oven at 110°C for 24 h. After the digestion is complete, cool to room temperature, accurately pipette 1 mL of the filtrate, and place it in a rotary evaporator in a 50°C vacuum drying box. Then, add 2.0 mL of pH 2.2 sodium citrate buffer solution to the dried test tube, shake well, and filter the solution through a 0.22 um filter membrane. Place the supernatant in an amino acid analyzer (Hitachi, LA8080) for determination (24).
Take a uniform sample weighing 2 g and transfer it to a flask. Add around 100 mg of pyrogallic acid and a small quantity of zeolite. Next, pour in 2 mL of 95% ethanol and 10 mL of hydrochloric acid solution, and mix the contents thoroughly. The flask should then be placed in a water bath maintained at a temperature of 70°C ~ 80°C for 40 min to facilitate hydrolysis. Rewrite the following passage:Weigh 2 g of a uniform sample and place it in a flask. Add approximately 100 mg of pyrogallic acid and a few grains of zeolite. Then add 2 mL of 95% ethanol and 10 mL of hydrochloric acid solution, mix well. Place the flask in a 70°C ~ 80°C water bath for 40 min to hydrolyze. In a 250 mL flask, collect the ether layer extract obtained from the previous step. Repeat the process thrice to extract the hydrolyzate. Lastly, rinse the separating funnel with a combination of ether and petroleum ether and collect the rinsing liquid in the flask until a constant weight is achieved. The flasks were dried on a water bath and then dried in an oven for 2 h at 100°C. Thereafter, add 2 mL of 2% sodium hydroxide methanol solution and place it in an 85°C-water bath for 30 min. Next, 3 mL of a 14% methanolic solution of boron trifluoride was added and placed in a water bath for 30 min at 85°C. When the water bath has finished, allow the temperature to drop to room temperature. Addition of 1 mL of n-hexane to the tube was centrifuged, shaken, and extracted for 2 min. Allow the contents to remain an hour to allow stratification. Extract 100 μL of the upper clear liquid, dilute it to 1 mL using n-hexane, and filter it through a 0.45 μm filter membrane. Finally, use a gas chromatography mass spectrometer (Trace1310 ISQ, Thermo) to determine the results.
2.7. Serum antioxidant capacity
The total superoxide dismutase (SOD) and glutathione peroxidase (GSH-PX) activities and serum malondialdehyde (MDA) levels were measured by spectrophotometer or enzyme marker using kits from Nanjing Jiancheng Institute of Biological Engineering according to the manufacturer’s instructions (25).
2.8. Ammonia emissions
The same sites in the hen houses were selected for the experimental and control groups at equal distances from top to bottom. An inverted 6-cm-diameter, the 12-cm-high container was placed above a cage at 15:00 every day. After 2 h, the ammonia gas was detected with a Wandi GASTiger 2000 (26).
2.9. Intestinal microflora
The collected cecum contents were weighed 250 mg and the microbial DNA was extracted from the samples using the EZNA soil DNA kit (Omega Bio-Tek, Norcross, GA, United States) as per the manufacturer’s instructions. Using an ABI Gene Amp 9700 PCR thermocycler (ABI, CA), the primer pairs 338F (5′-ACTCCTACGGGAGGCAGCA-3′) and 806R (5′-GGACTACHVGGGTWTCTAAT-3′) were employed to amplify the hypervariable region V3−V4 of the bacterial 16S rRNA gene. The PCR reactions commenced with an initial denaturation at 98°C for 2 min. This was followed by 30 cycles of denaturation at 98°C for 30 s, annealing at 55°C for 30 s, and extension at 72°C for 60 s. The final extension was carried out at 72°C for 5 min. The AxyPrep DNA Gel Extraction Kit (Axygen Biosciences, Union City, CA) was utilized to extract and purify the amplicons as per the manufacturer’s instructions. The kit was effective in removing excess primer dimers and dNTPs from the sample. Equal amounts of purified amplicons were combined and subjected to paired-end sequencing (2*250 bp) using the Illumina MiSeq platform at Tsingke Biotechnology Co., Ltd., (27).
2.10. Statistical analysis
The experimental data were recorded in Microsoft Excel 2010 and the results were analyzed using SPSS 23 was used to perform one-way ANOVA (One-way ANOVA) on the experimental results, and the Duncan’s method was used for multiple comparisons, with p < 0.05 as a significant difference. Data are presented in the table as mean and combined SEM. The figure was drawn using GraphPad Prism6 (GraphPad Software, United States).
3. Results
3.1. Production performance
Table 3 displays the production performance of laying hens fed (TR)-fermented feed; no significant changes in the ADFI between the experimental groups were found (p > 0.05). During the period of 1–21 days, the Egg-laying rate and Average egg weight of the T1 group of broiler chickens were significantly higher than those of the T0 and T3 groups (p < 0.05). During the period of 22–42 days, the Egg-laying rate of the T1 group was significantly higher than that of the T0, T2, and T3 groups. Additionally, the Feed-to-egg ratio of the T1 group was significantly lower than that of the T3 group (p < 0.05). Throughout the entire period of 1–42 days, the Egg-laying rate of the T1 group was significantly higher than that of the T0, T2, and T3 groups. Moreover, the Average egg weight of the T1 group was significantly higher than that of the T0 group, and the Feed-to-egg ratio of the T1 group was significantly lower than that of the T0 and T3 groups (p < 0.05).
3.2. Eggs quality
Table 4 displays the egg quality after 42 days of feeding the hens (TR)-fermented feed. Between each group’s albumin height and Egg shape index, there was no discernible variation (p > 0.05). When compared to the T0 group, T1 and T2 group significantly enhanced the Haugh unit of eggs (p < 0.05). It is notable that the T2 and T3 group considerably and nearly multiplicatively enhanced the eggshell thickness of the eggs (p < 0.05).
3.3. Amino acid and fatty acid contents
As shown in Table 5, we detected a total of 16 amino acids in the eggs, and the addition of (TR)-fermented feed has increased the content of most amino acids in the eggs. Compared with the T0 group, the T2 group enhanced the content of Methionine, Tyrosine, Proline, and EAA in the eggs (p < 0.05). The fatty acid content in eggs after supplementation of (TR)-fermented feed is shown in Table 6. The content of C17:0 in eggs with T1 and T3 was higher than that in the control group (p < 0.05). The T2 group significantly increased the content of C18:3n6, C22:0, C22:6n3, C23:0, C24:1, and ∑ω-3 PUFA in the eggs (p < 0.05). The content of ∑SFA, ∑MUFA, ∑PUFA, and ∑ω-6 PUFA in eggs supplemented with (TR)-fermented feed was higher than that in the control group (p > 0.05).
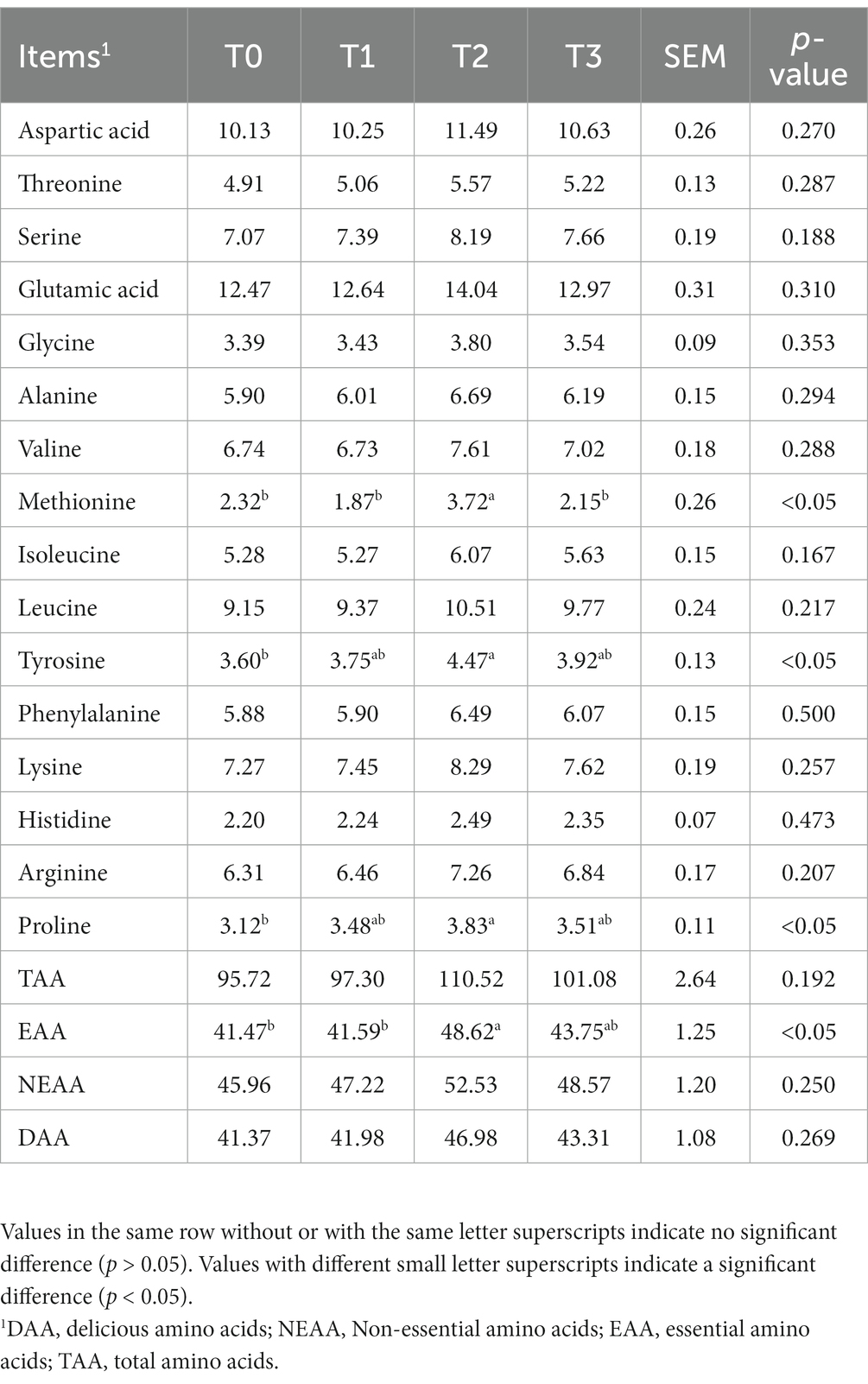
Table 5. The effects of supplemented (TR)-fermented feed on the amino acid contents of eggs from laying hens.
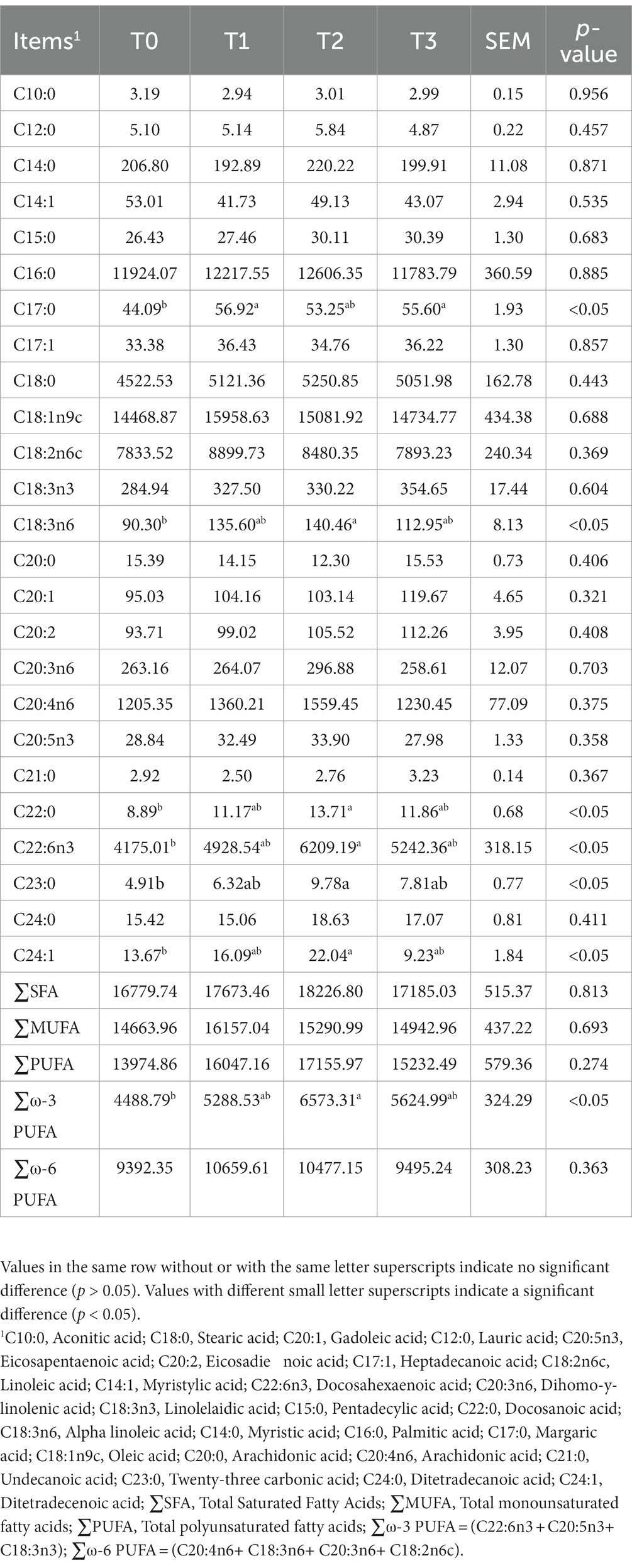
Table 6. The effects of supplemented (TR)-fermented feed on fatty acid content in eggs of laying hens.
3.4. Serum antioxidant capacity
Figure 1 displays the laying hens’ serum biochemical indicators. The addition of 3% (TR)-fermented feed substantially raised the GSH-PX and SOD activities of laying hens as compared to the control group (p < 0.05). There was a significant change with a 1% addition in the MDA content in the serum of laying hens compared to the control group (p < 0.05).
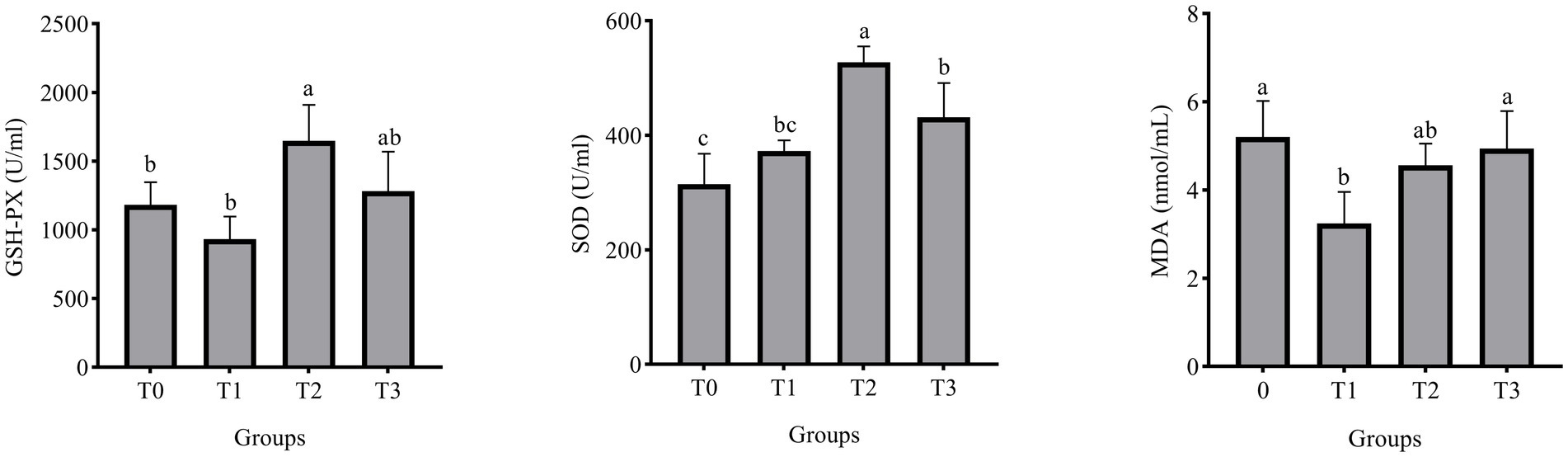
Figure 1. Effect of (TR)-fermented feed supplementation on the serum antioxidant capacity. MDA, malondialdehyde; SOD, superoxide dismutase; GSH- PX, glutathione peroxidase. Different letters indicate significant differences (p < 0.05).
3.5. Ammonia emissions
As shown in Figure 2, we counted the ammonia emissions of each trial group and found that feeding (TR)-fermented feed decreased the ammonia emissions of birds (p < 0.05), and the ammonia emissions were T0 > T2 > T3 > T1 between each trial group.
3.6. Intestinal microflora
The cecal samples collected from laying hens yielded a total of 646 OTUs. Among the four groups, 599 OTUs were shared, while the remaining OTUs were specific to each group. Specifically, the L, M, N, and P groups had 2, 1, 0, and 1 specific OTUs, respectively (Figure 3A). Taxonomic compositions were examined at the phylum and genus levels to determine the variations brought about by (TR)-fermented feed in the cecal microbiota (Figures 3B,C). There were high relative abundances of 10 bacterial phyla. The two main phyla in the cecal bacterial community, Bacteroidetes and Firmicutes, were differentially abundant among groups, making up more than 55 and 33%, respectively. The relative abundance of Tenericutes increased significantly under the L group (p < 0.05).
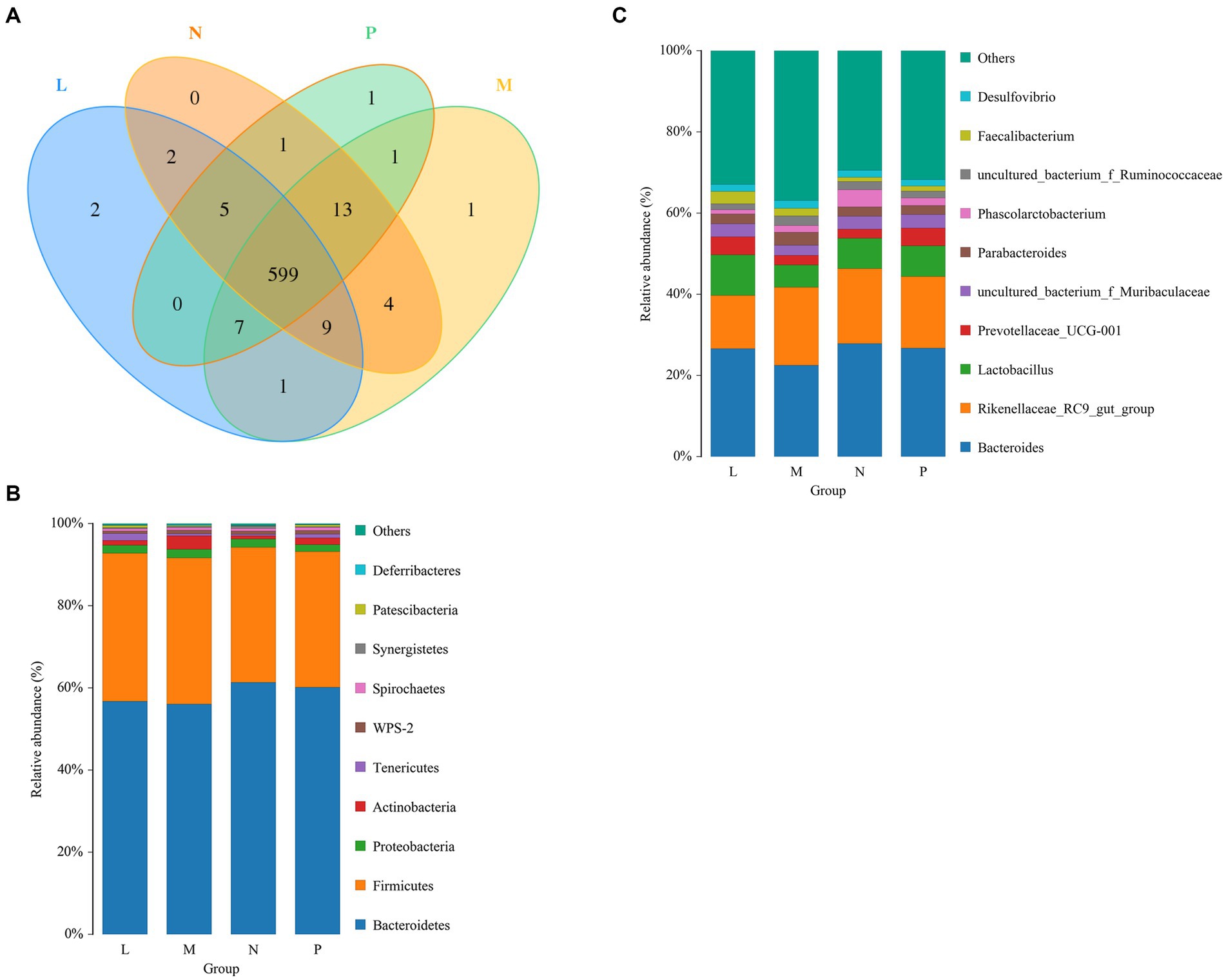
Figure 3. Laying hens’ bacterial community composition and Venn diagram on day 42. (A) The OTU Venn diagram. Phylum- or genus-level information on the composition of the bacteria in the cecum. “Other” includes all taxa with a relative abundance of less than 1%. P, control group; L, 1% (TR)-fermented feed; M, 3% (TR)-fermented feed; N, 5% (TR)-fermented feed. (B,C) The phylum and genus level compositions of the bacterial communities, respectively.
Bacteroides was the dominant genus, followed by the Lactobacillus and Rikenellaceae RC9 gut group. Lower abundances were 22.5% for Bacteroides, 14% for the Rikenellaceae RC9 gut group, and over 8% for Lactobacillus. In addition, cecal bacteria with abundances greater than 1% were Prevotellaceae UCG-001, uncultured bacterium f. Muribaculaceae, Parabacteroides, Phascolarctobacterium, uncultured bacterium f. Ruminococcaceae, Faecalibacterium, and Desulfovibrio.
The M and N group decreased the abundances of CHCKI001 and Faecalibacterium (p < 0.05; Table 7). The N group reduced the abundance of GCA-900066575 (p < 0.01) and significantly improved the abundance of Romboutsia (p < 0.05). The L group significantly improved the abundances of Flavonifractor, Pediococcus, Ruminiclostridium 9 (p < 0.01), and Ruminiclostridium 5 (p < 0.05). Groups M and N significantly enhanced the abundance of Fournierella (p < 0.05). The M group significantly improved Prevotellaceae Ga6A1 (p < 0.01) and significantly reduced the abundance of Sellimonas (p < 0.05). Groups L and M significantly improved the abundance of Ruminiclostridium (p < 0.01) and significantly decreased the abundance of Ruminococcaceae UCG-014 (p < 0.05).
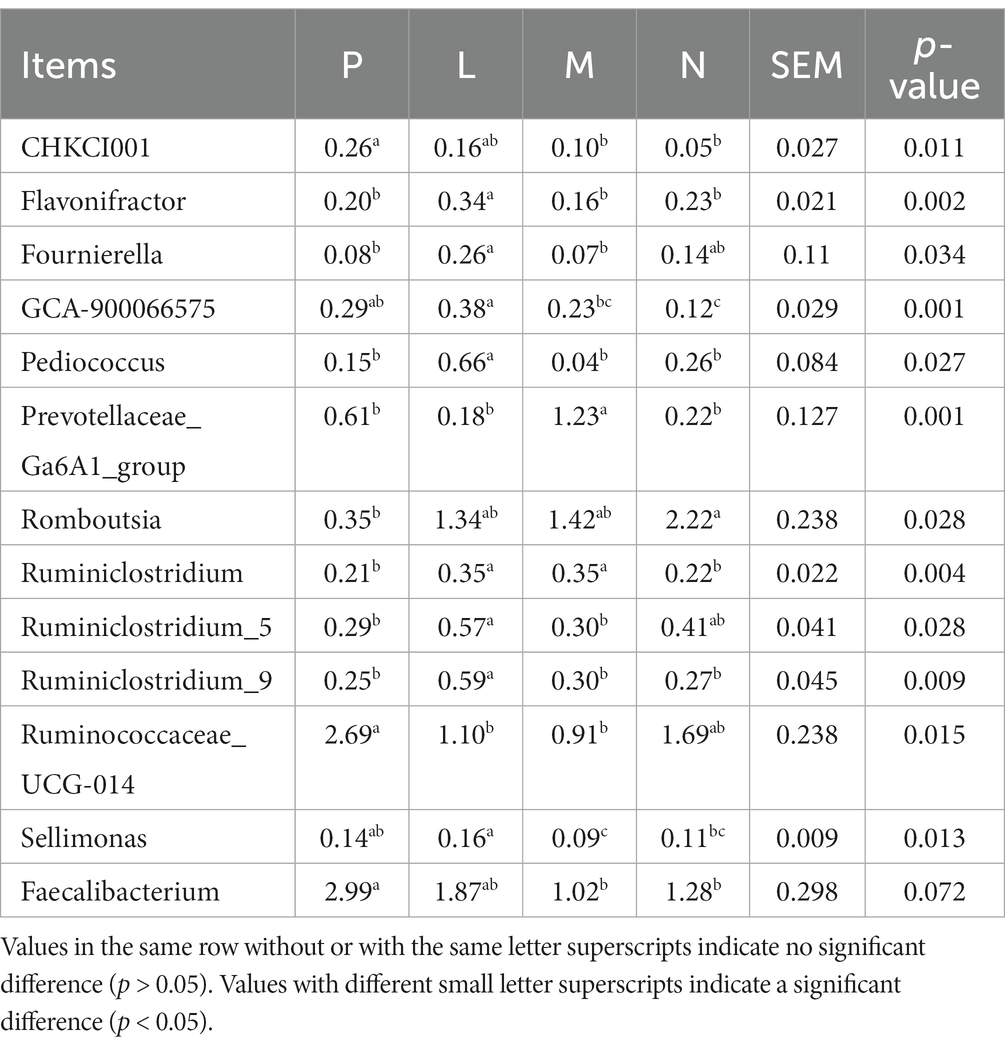
Table 7. Effect of different levels of fermented tea residue on the abundance of cecal bacterial genera (%).
Figure 4 displays findings about the alpha and beta diversity of the cecal microbiota in laying hens. There were no discernible differences between the four groups for any of the alpha diversity indices, including Shannon, Simpson, Chao1 and Ace (Figures 4A–D). As shown in Figure 4E, principal coordinates analysis (PCoA) showed differences between the control group and the groups fed diets supplemented with 1, 3, or 5% (TR)-fermented feed, indicating differences in the cecal microflora between the control and experimental groups, but the differences were not significant.
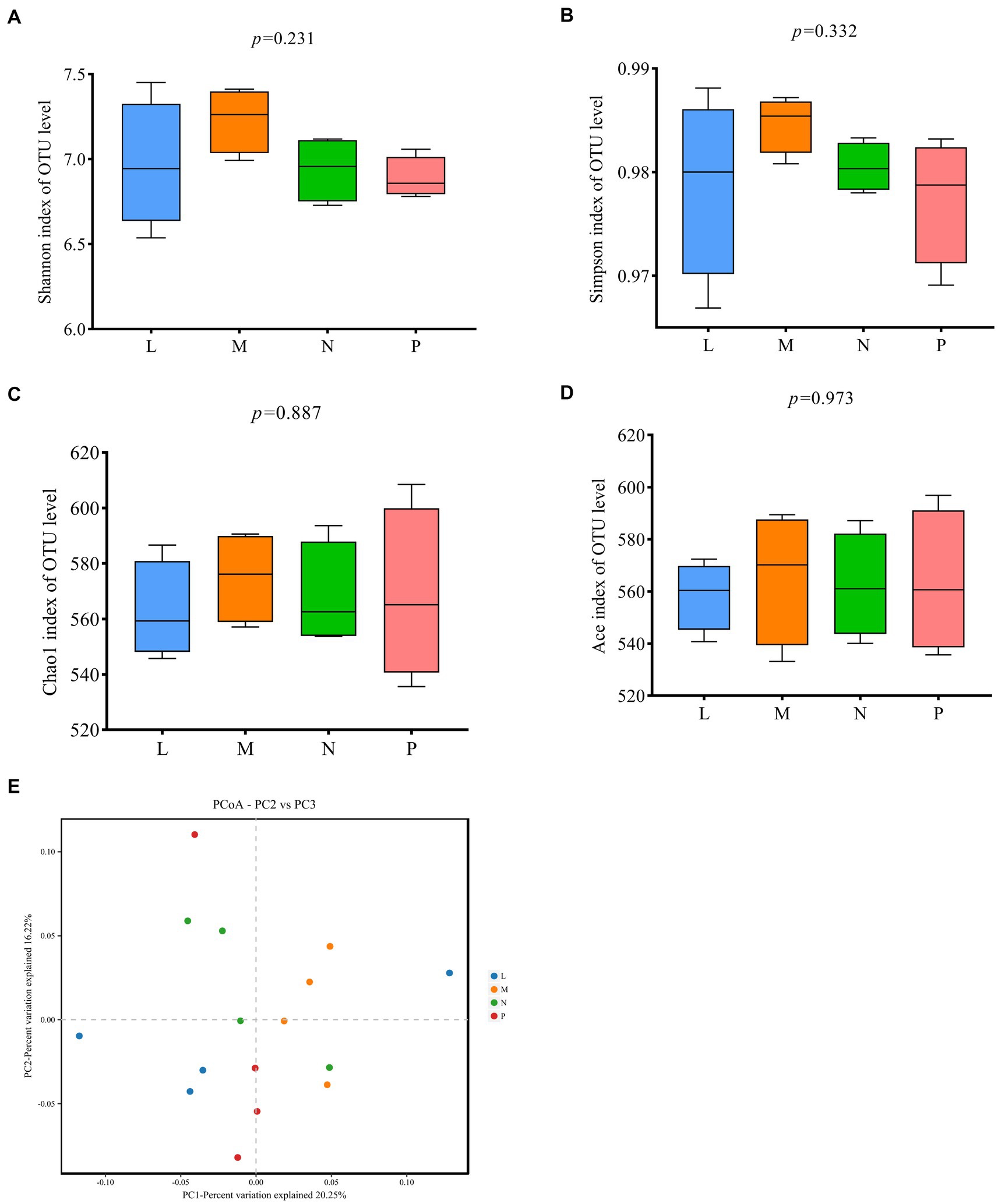
Figure 4. Analysis of the diversity of microbial communities between communities. (A–D) Alpha diversity analysis. (E) Principal coordinates analysis (PCoA) based on Bray-Curti’s distance. P, control group; L, 1% (TR)-fermented feed; M, 3% (TR)-fermented feed; N, 5% (TR)-fermented feed.
4. Discussion
Tea residues are rich in tea polyphenols, these flavonoids can improve the secretion of estrogen and luteinizing hormone, thus improving the production performance of laying hens (28). There are a large number of probiotics in fermented feed, which can convert feed materials into microbial bacteriological proteins and bioactive small peptide amino acids and reduce anti-nutritional factors, thus promoting the utilization of nutrients in feed, and consequently improving feed conversion rate and animal performance (29). Some researches indicated that tea or fermented feed could enhance the production performance of laying hens by improving the digestion and absorption of feed (30–33). We found that feeding a certain amount of (TR)-fermented feed can improve laying rate and average egg weight and reduce feed-to-egg ratio (1 and 3%), but excessive addition does not have a positive effect (5%). We speculate that the reason may be related to the high content of polyphenolic composition in green tea residues, which can have a positive effect when added in moderation, but excessive addition will make its anti-nutritional effect significant, and its combination with animal oral salivary proteins will produce a bitter taste and reduce animal feed intake, thus reducing the production performance of hens.
We evaluated the quality of eggs mainly by albumen height, shell thickness, egg shape index, haugh unit and yolk color. The egg shape index is a parameter describing the shape of the egg, which has no effect on the nutritional value of food, but is related to the hatching and breaking rates which affect the breeding value (34, 35). Our study found that (TR)-fermented feed had no significant effect on the egg shape index. A higher haugh unit means that the albumen is more viscous and the egg quality is better (36). The color of the yolk is also an important indicator of the freshness of the egg. The deeper the yolk color, the better of the egg quality (37). In this experiment, the addition of a certain amount of (TR)-fermented feed can increase the haugh unit and yolk color of eggs. Eggshell thickness can directly affect the breakage rate of eggs. As fragile products, most egg losses during transportation and storage are caused by poor shell thickness. In this study, we found that the eggshell thickness of eggs increased by nearly half at higher additions (3, 5%). The thickness of the eggshell is related to the digestion and absorption of vitamins, calcium and phosphorus in the feed (38, 39), because the fermented feed contains a large number of vitamins and organic acids, which have substances that can promote the absorption of metal ions by the organism and thus increase the thickness of the eggshell (3, 40).
Eggs contain abundant protein, amino acids, fatty acids, vitamins, and other nutrients, and are an important source of nutrition for the human body. The amino acid and fatty acid contents of eggs are essential indices for assessing the egg’s nutritional value, as well as important flavor substances that greatly affect the taste and flavor of eggs. In this experiment, a total of 16 amino acids were detected, and after supplementation of (TR)-fermented feed, the TAA, EAA, NEAA, and DAA in eggs all increased, indicating that (TR)-fermented feed supplementation increased the content and freshness of amino acids in eggs, resulting in better quality. This is consistent with previous studies, which have shown that supplementing with tea polyphenols can increase the content of some amino acids in eggs (21). Eggs are rich in various fatty acids, and different fatty acids have different physiological effects on human health. Unsaturated fatty acids are vital essential fatty acids for the human body. Monounsaturated fatty acids have effects such as lowering blood sugar, reducing cholesterol, and regulating blood lipids. Polyunsaturated fatty acids have effects such as promoting brain development and preventing cardiovascular diseases (41, 42). Docosahexaenoic acid and eicosapentaenoic acid, two members of the omega-3 class of polyunsaturated fatty acids, are helpful for gastrointestinal cancer patients by enhancing immune function and help to lower triglycerides. Which is beneficial for heart health and helps improve conditions such as rheumatoid arthritis and other diseases (43). It was found that the addition of 0.1% green tea extract significantly increased the content of linoleic acid, arachidonic acid, docosahexaenoic acid and total polyunsaturated fatty acids in egg yolk (44). Supplementation of green tea by-products in the diet can increase the content of linolenic acid and docosahexaenoic acid in egg yolk (45). Our study found that the content of SFA, MUFA, PUFA, ω-3 polyunsaturated fatty acids, and ω-6 polyunsaturated fatty acids in eggs increased after supplementation with (TR)-fermented feed, indicating that supplementation with (TR)-fermented feed improved the fatty acid content in eggs of laying hens and greatly improved the quality of eggs.
In the antioxidant system, SOD, GSH-PX, and MDA metabolize free radicals in vivo, which is important for limiting oxidative damage in animals (46). Oxygen free radicals are biologically damaging macromolecules that are involved in the development of many diseases and the aging process of the body (47). The end product of lipid peroxidation brought on by oxygen radicals is M DA, and the amount of MDA in the body can indirectly indicate the amount of oxygen radicals present as well as the degree of cell damage (48). SOD is the first line of defense for scavenging free radicals in animals, which can rapidly convert superoxide anion radical O2− into molecular oxygen and hydrogen peroxide (49). The main function of GSH-PX is to remove peroxide and H2O2 from the body (50). Our study found that feeding (TR)-fermented feed increased SOD and GSH-PX activity and decreased MDA content in laying hens. This indicates that feeding (TR)-fermented feed can reduce oxidative stress in laying hens. Some active substances in the (TR)-fermented feed and probiotics may increase the antioxidant capacity of the laying hens (51–53).
Ammonia is a harmful gas that is more harmful to laying hens. The concentration of ammonia is related to the protein levels in the diet and the digestibility of the poultry (54). Due to the short digestive tract of chickens, the digestion and absorption of feed are limited, and most of the nutrients are not digested and absorbed and excreted directly, especially proteins (55). Protein is decomposed by microbes under suitable conditions and releases a large amount of ammonia and other harmful gases, thus polluting the air of the chicken house (56). In this study, we found that feeding (TR)-fermented feed significantly reduced ammonia emissions in the laying hen house. According to similar studies, the addition of enzymes to the feed reduced nitrogen and ammonia emissions as well as the feeding of fermented corn fructus to broilers to reduce ammonia concentrations in broiler manure (57, 58). This is probably because the large molecules in the feed are broken down into small peptides and amino acids that are easily absorbed, reducing the amount of undigested protein in the intestine, and reducing the production of ammonia and toxic gases.
The intestinal flora of laying hens mainly colonizes the cecum, which can affect the health, immunity, digestive efficiency, and egg quality of laying hens (59). In this experiment, there was no significant difference in the relative abundance of Bacteroides and Firmicutes between the experimental and control groups, but adding 1% of (TR)-fermented feed had significantly more Tenericutes in the caecum of laying hens. It is possible that some probiotic bacteria in (TR)-fermented feed, such as B. subtilis, increased the abundance of Tenericutes (60). It was found that broilers fed fermented tea residue with probiotics or symbionts had a better feed conversion rate, but the relative abundances of CHKCI001 and Faecalibacterium were lower than those of the control group (61). This is consistent with our M and N results. Sellimonas were negatively correlated with the average daily feed intake, feed conversion rate, and isobutyric acid levels (62). Bacillus licheniformis fermentation products increased the Coccidiococcus resistance index of broilers, but the relative abundance of Sellimonas in the cecum of broilers decreased, which was consistent with the results of the M group. Pediococcus is considered a good candidate probiotic for chickens (63). In this study, the relative abundance of Pediococcus was increased. A series of changes may be related to the addition of probiotics in the fermented tea residue feed. Probiotics and their metabolites act on the intestinal tract of laying hens and change the abundance of microbial flora.
Propionic acid, which is the primary byproduct of starch fermentation by starch breakdown bacteria, and Ruminococcaceae UCG-014 have a negative correlation with one another (64). The abundance of Ruminococcaceae UCG-014 of the L and M group was reduced. Adding (TR)-fermented feed to the diet may help to promote the digestion and absorption of feed for laying hens. Studies have found that Romboutsia is positively correlated with egg production by laying hens, and can be used to predict egg production (65). In our experiment, adding 5% (TR)-fermented feed significantly improved the relative abundance of Romboutsia. It is possible to increase the egg production of laying hens by adding (TR)-fermented feed. Ruminiclostridium is closely related to maintaining intestinal health. It can degrade cellulose and hemicellulose in plants and convert them into short-chain fatty acids, which are absorbed and used by the host (66). Ruminiclostridium 9 is directly proportional to the lipid metabolism capacity in vivo (65). Ruminiclostridium 5 has a positive effect on chicken body growth (67). The relative abundance of Ruminiclostridium 5 increased in group L. This may be related to the increased use of fermented tea residue feed by regulating the microflora. Fournierella can metabolize and produce acetic and propionic acids (68). Groups L and N had increased relative abundances of Fournierella, which may be related to the function of tea residue to enhance lipid metabolism.
5. Conclusion
Our findings revealed that the inclusion of a certain amount of (TR) fermented feed in the diet of laying hens has a positive effect on their production performance during the laying period. Additionally, it improves egg quality and serum antioxidant capacity, regulates the diversity of gut microbiota, and reduces ammonia emissions from poultry houses.
Data availability statement
The original contributions presented in the study are publicly available. This data can be found at: https://www.ncbi.nlm.nih.gov/; PRJNA940200.
Ethics statement
The animal study was reviewed and approved by the Leshan Academy of Agriculture Science.
Author contributions
XC and XZ: conceptualization, methodology, formal analysis, investigation, and writing—original draft. SL: investigation, supervision, funding acquisition, and writing—review and editing. HZ and ZL: conceptualization, methodology, and formal analysis. All authors contributed to the study’s conception and design.
Funding
This work was supported by Sichuan Science and Technology Program 2021JDRC0142.
Conflict of interest
The authors declare that the research was conducted in the absence of any commercial or financial relationships that could be construed as a potential conflict of interest.
Publisher’s note
All claims expressed in this article are solely those of the authors and do not necessarily represent those of their affiliated organizations, or those of the publisher, the editors and the reviewers. Any product that may be evaluated in this article, or claim that may be made by its manufacturer, is not guaranteed or endorsed by the publisher.
References
1. Chang, Y, Saeed Omer, SH, Li, G, Lian, H, and Liu, Y. Research advance on application of microbial fermented fodder in broilers production: a short review. Open J Anim Sci. (2022) 12:200–9. doi: 10.4236/ojas.2022.122015
2. Lv, J, Guo, L, Chen, B, Hao, K, Ma, H, Liu, Y, et al. Effects of different probiotic fermented feeds on production performance and intestinal health of laying hens. Poult Sci. (2022) 101:101570. doi: 10.1016/j.psj.2021.101570
3. Sugiharto, S, and Ranjitkar, S. Recent advances in fermented feeds towards improved broiler chicken performance, gastrointestinal tract microecology and immune responses: a review. Anim Nutr. (2019) 5:1–10. doi: 10.1016/j.aninu.2018.11.001
4. Chebaibi, S, Leriche Grandchamp, M, Burgé, G, Clément, T, Allais, F, and Laziri, F. Improvement of protein content and decrease of anti-nutritional factors in olive cake by solid-state fermentation: a way to valorize this industrial by-product in animal feed. J Biosci Bioeng. (2019) 128:384–90. doi: 10.1016/j.jbiosc.2019.03.010
5. Rui, X, Wang, MJ, Zhang, YQ, Chen, X, Li, L, Liu, Y, et al. Optimization of soy solid-state fermentation with selected lactic acid bacteria and the effect on the anti-nutritional components. J Food Proc Preserv. (2017) 41:e13290. doi: 10.1111/jfpp.13290
6. Soumeh, EA, Mohebodini, H, Toghyani, M, Shabani, A, Ashayerizadeh, A, and Jazi, V. Synergistic effects of fermented soybean meal and mannan-oligosaccharide on growth performance, digestive functions, and hepatic gene expression in broiler chickens. Poult Sci. (2019) 98:6797–807. doi: 10.3382/ps/pez409
7. Wang, G-H, Chen, C-Y, Lin, C-P, Huang, CL, Lin, CH, Cheng, CY, et al. Tyrosinase inhibitory and antioxidant activities of three Bifidobacterium bifidum-fermented herb extracts. Ind Crop Prod. (2016) 89:376–82. doi: 10.1016/j.indcrop.2016.05.037
8. Li, J, Tao, L, Zhang, R, and Yang, G. Effects of fermented feed on growth performance, nutrient metabolism and cecal microflora of broilers. Anim Biosci. (2022) 35:596–604. doi: 10.5713/ab.21.0333
9. Huang, CM, Chuang, WY, Lin, WC, Lin, LJ, Chang, SC, and Lee, TT. Production performances and antioxidant activities of laying hens fed Aspergillus oryzae and phytase co-fermented wheat bran. Anim Biosci. (2021) 34:371–84. doi: 10.5713/ajas.20.0116
10. Li, W, Cheng, P, Zhang, JB, Zhao, LM, Ma, YB, and Ding, K. Synergism of microorganisms and enzymes in solid-state fermentation of animal feed. a review. Roczniki Nauk Rolniczych Seria B Zootechniczna. (2021) 30:3–10. doi: 10.22358/jafs/133151/2021
11. Guo, S, Kumar Awasthi, M, Wang, Y, and Xu, P. Current understanding in conversion and application of tea waste biomass: a review. Bioresour Technol. (2021) 338:125530. doi: 10.1016/j.biortech.2021.125530
12. Sharangi, AB. Medicinal and therapeutic potentialities of tea (Camellia sinensis L.) – a review. Food Res Int. (2009) 42:529–35. doi: 10.1016/j.foodres.2009.01.007
13. Higdon, JV, and Frei, B. Tea catechins and polyphenols: health effects, metabolism, and antioxidant functions. Crit Rev Food Sci Nutr. (2003) 43:89–143. doi: 10.1080/10408690390826464
14. Mahlake, SK, Mnisi, CM, Kumanda, C, Mthiyane, DMN, and Montso, PK. Green tea (Camellia sinensis) products as alternatives to antibiotics in poultry nutrition: a review. Antibiotics. (2022) 11:565. doi: 10.3390/antibiotics11050565
15. Bag, S, Mondal, A, Majumder, A, and Banik, A. Tea and its phytochemicals: hidden health benefits & modulation of signaling cascade by phytochemicals. Food Chem. (2022) 371:131098. doi: 10.1016/j.foodchem.2021.131098
16. Jiang, L, Zhou, X, Yu, J, Bao, S, Li, J, Wu, Q, et al. Fermented tea residue improved growth performance, liver antioxidant capacity, intestinal morphology and resistance to Aeromonas hydrophila infection in juvenile largemouth bass (Micropterus salmoides). Front Mar Sci. (2022) 9:999947. doi: 10.3389/fmars.2022.999947
17. Ding, X, Li, H, Wen, Z, Hou, Y, Wang, G, Fan, J, et al. Effects of fermented tea residue on fattening performance, meat quality, digestive performance, serum antioxidant capacity, and intestinal morphology in fatteners. Animals. (2020) 10:185. doi: 10.3390/ani10020185
18. Wang, J, Yang, Z, Celi, P, Yan, L, Ding, X, Bai, S, et al. Alteration of the antioxidant capacity and gut microbiota under high levels of molybdenum and green tea polyphenols in laying hens. Antioxidants. (2019) 8:503. doi: 10.3390/antiox8100503
19. Xia, B, Liu, Y, Sun, D, Liu, J, Zhu, Y, and Lu, L. Effects of green tea powder supplementation on egg production and egg quality in laying hens. J Appl Anim Res. (2018) 46:927–31. doi: 10.1080/09712119.2018.1431240
20. Zhu, YF, Wang, JP, Ding, XM, Bai, SP, Qi, SRN, Zeng, QF, et al. Effect of different tea polyphenol products on egg production performance, egg quality and antioxidative status of laying hens. Anim Feed Sci Technol. (2020) 267:114544. doi: 10.1016/j.anifeedsci.2020.114544
21. Wang, J, Jia, R, Celi, P, Ding, X, Bai, S, Zeng, Q, et al. Green tea polyphenol epigallocatechin-3-gallate improves the antioxidant capacity of eggs. Food Funct. (2020) 11:534–43. doi: 10.1039/C9FO02157D
22. Wen, J, Cai, HY, Guo, YM, et al. Nutrient requirements of Chinese color-feathered chicken. Feeding Standard of Chicken (NY/T 33-2004). (2004):11–2.
23. Rabelo-Ruiz, M, Ariza-Romero, JJ, Zurita-González, MJ, Martín-Platero, AM, Baños, A, Maqueda, M, et al. Allium-based phytobiotic enhances egg production in laying hens through microbial composition changes in ileum and cecum. Animals. (2021) 11:448. doi: 10.3390/ani11020448
24. Zhou, X, Li, S, Jiang, Y, Deng, J, Yang, C, Kang, L, et al. Use of fermented Chinese medicine residues as a feed additive and effects on growth performance, meat quality, and intestinal health of broilers. Front Vet Sci. (2023):1157935:10. doi: 10.3389/fvets.2023.1157935
25. Zhou, X, Wang, Y, Yu, J, Li, J, Wu, Q, Bao, S, et al. Effects of dietary fermented Chinese herbal medicines on growth performance, digestive enzyme activity, liver antioxidant capacity, and intestinal inflammatory gene expression of juvenile largemouth bass (Micropterus salmoides). Aquac Rep. (2022) 25:101269. doi: 10.1016/j.aqrep.2022.101269
26. Reece, FN, Bates, BJ, and Lott, BD. Ammonia control in broiler houses. Poult Sci. (1979) 58:754–5. doi: 10.3382/ps.0580754
27. Lin, J, Comi, M, Vera, P, Alessandro, A, Qiu, K, Wang, J, et al. Effects of Saccharomyces cerevisiae hydrolysate on growth performance, immunity function, and intestinal health in broilers. Poult Sci. (2023) 102:102237. doi: 10.1016/j.psj.2022.102237
28. Abd El-Hack, ME, Salem, HM, Khafaga, AF, Soliman, SM, and El-Saadony, MT. Impacts of polyphenols on laying hens' productivity and egg quality: a review. J Anim Physiol Anim Nutr. (2022) 107:928–47. doi: 10.1111/jpn.13758
29. Dawood, MAO, and Koshio, S. Application of fermentation strategy in aquafeed for sustainable aquaculture. Rev Aquac. (2020) 12:987–1002. doi: 10.1111/raq.12368
30. Wang, X-c, Wang, X-h, Wang, J, Wang, H, Zhang, HJ, Wu, SG, et al. Dietary tea polyphenol supplementation improved egg production performance, albumen quality, and magnum morphology of Hy-Line Brown hens during the late laying period1. J Anim Sci. (2018) 96:225–35. doi: 10.1093/jas/skx0007
31. Xia, B, Liu, J, Sun, D, Liu, YL, and Liu, D. Changes in egg production, egg quality and egg nutritional values of laying hens in response to dietary supplementation with green tea water extracts. J Appl Anim Res. (2022) 50:254–8. doi: 10.1080/09712119.2022.2039155
32. Guo, W, Xu, L-n, Guo, X-j, Wang, W, Hao, QH, Wang, SY, et al. The impacts of fermented feed on laying performance, egg quality, immune function, intestinal morphology and microbiota of laying hens in the late laying cycle. Animal. (2022) 16:100676. doi: 10.1016/j.animal.2022.100676
33. Yang, R, Khalid, A, Khalid, F, Ye, M, Li, Y, Zhan, K, et al. Effect of fermented corn by-products on production performance, blood biochemistry, and egg quality indices of laying hens. J Anim Sci. (2022) 100:skac130. doi: 10.1093/jas/skac130
34. King`ori, AM. Review of the factors that influence egg fertility and hatchabilty in poultry. Int J Poult Sci. (2011) 10:483–92. doi: 10.3923/ijps.2011.483.492
35. Cucco, M, Grenna, M, and Malacarne, G. Female condition, egg shape and hatchability: a study on the grey partridge. J Zool. (2012) 287:186–94. doi: 10.1111/j.1469-7998.2012.00902.x
36. Nematinia, E, and Abdanan, MS. Assessment of egg freshness by prediction of Haugh unit and albumen pH using an artificial neural network. J Food Meas Charact. (2018) 12:1449–59. doi: 10.1007/s11694-018-9760-1
37. Joubrane, K, Mnayer, D, Hamieh, T, Barbour, G, Talhouk, R, and Awad, E. Evaluation of quality parameters of white and brown eggs in Lebanon. Am J Anal Chem. (2019) 10:488–503. doi: 10.4236/ajac.2019.1010035
38. Adhikari, R, White, D, House, JD, and Kim, WK. Effects of additional dosage of vitamin D(3), vitamin D(2), and 25-hydroxyvitamin D(3) on calcium and phosphorus utilization, egg quality and bone mineralization in laying hens. Poult Sci. (2020) 99:364–73. doi: 10.3382/ps/pez502
39. Nie, W, Wang, B, Gao, J, Guo, Y, and Wang, Z. Effects of dietary phosphorous supplementation on laying performance, egg quality, bone health and immune responses of laying hens challenged with Escherichia coli lipopolysaccharide. J Anim Sci Biotechnol. (2018) 9:53. doi: 10.1186/s40104-018-0271-z
40. Mingmongkolchai, S, and Panbangred, W. Bacillus probiotics: an alternative to antibiotics for livestock production. J Appl Microbiol. (2018) 124:1334–46. doi: 10.1111/jam.13690
41. Djuricic, I, and Calder, PC. Beneficial outcomes of Omega-6 and Omega-3 polyunsaturated fatty acids on human health: an update for 2021. Nutrients. (2021) 13:2421. doi: 10.3390/nu13072421
42. Gillingham, LG, Harris-Janz, S, and Jones, PJH. Dietary monounsaturated fatty acids are protective against metabolic syndrome and cardiovascular disease risk factors. Lipids. (2011) 46:209–28. doi: 10.1007/s11745-010-3524-y
43. Bai, H, Li, Z, Meng, Y, Yu, Y, Zhang, H, Shen, D, et al. Effects of parenteral Omega-3 fatty acid supplementation in postoperative gastrointestinal cancer on immune function and length of hospital stay: a systematic review and meta-analysis. Asia Pac J Clin Nutr. (2018) 27:121–8. doi: 10.6133/apjcn.022017.19
44. Huang, J, Hao, Q, Wang, Q, Wang, Y, Wan, X, and Zhou, Y. Supplementation with green tea extract affects lipid metabolism and egg yolk lipid composition in laying hens. J Appl Poult Res. (2019) 28:881–91. doi: 10.3382/japr/pfz046
45. Uuganbayar, D, Bae, IH, Choi, KS, Shin, IS, Firman, JD, and Yang, CJ. Effects of green tea powder on laying performance and egg quality in laying hens. Asian Australas J Anim Sci. (2005) 18:1769–74. doi: 10.5713/ajas.2005.1769
46. Ozgocmen, S, Kaya, H, Fadillioglu, E, Aydogan, R, and Yilmaz, Z. Role of antioxidant systems, lipid peroxidation, and nitric oxide in postmenopausal osteoporosis. Mol Cell Biochem. (2007) 295:45–52. doi: 10.1007/s11010-006-9270-z
47. Speakman, JR, and Selman, C. The free-radical damage theory: accumulating evidence against a simple link of oxidative stress to ageing and lifespan. Bioessays. (2011) 33:255–9. doi: 10.1002/bies.201000132
48. Dai, X, Xing, C, Cao, H, Luo, J, Wang, T, Liu, P, et al. Alterations of mitochondrial antioxidant indexes and apoptosis in duck livers caused by molybdenum or/and cadmium. Chemosphere. (2018) 193:574–80. doi: 10.1016/j.chemosphere.2017.11.063
49. Reilly, PM, Schiller, HJ, and Bulkley, GB. Pharmacologic approach to tissue injury mediated by free radicals and other reactive oxygen metabolites. Am J Surg. (1991) 161:488–503. doi: 10.1016/0002-9610(91)91120-8
50. Delwing-Dal Magro, D, Roecker, R, Junges, GM, Rodrigues, AF, Delwing-de Lima, D, da Cruz, JGP, et al. Protective effect of green tea extract against proline-induced oxidative damage in the rat kidney. Biomed Pharmacother. (2016) 83:1422–7. doi: 10.1016/j.biopha.2016.08.057
51. Yuan, ZH, Zhang, KY, Ding, XM, Luo, YH, Bai, SP, Zeng, QF, et al. Effect of tea polyphenols on production performance, egg quality, and hepatic antioxidant status of laying hens in vanadium-containing diets. Poult Sci. (2016) 95:1709–17. doi: 10.3382/ps/pew097
52. Yin, B, Lian, R, Li, Z, Liu, Y, Yang, S, Huang, Z, et al. Tea polyphenols enhanced the antioxidant capacity and induced Hsps to relieve heat stress injury. Oxidative Med Cell Longev. (2021) 2021:1–13. doi: 10.1155/2021/9615429
53. Zhu, F, Zhang, B, Li, J, and Zhu, L. Effects of fermented feed on growth performance, immune response, and antioxidant capacity in laying hen chicks and the underlying molecular mechanism involving nuclear factor-κB. Poult Sci. (2020) 99:2573–80. doi: 10.1016/j.psj.2019.12.044
54. Naseem, S, and King, AJ. Ammonia production in poultry houses can affect health of humans, birds, and the environment—techniques for its reduction during poultry production. Environ Sci Pollut Res. (2018) 25:15269–93. doi: 10.1007/s11356-018-2018-y
55. Pan, D, and Yu, Z. Intestinal microbiome of poultry and its interaction with host and diet. Gut Microbes. (2014) 5:108–19. doi: 10.4161/gmic.26945
56. Carlile, FS. Ammonia in poultry houses: a literature review. Worlds Poult Sci J. (1984) 40:99–113. doi: 10.1079/WPS19840008
57. Ahmed, ST, Mun, HS, Islam, MM, and Yang, CJ. Effects of fermented corni fructus and fermented kelp on growth performance, meat quality, and emission of ammonia and hydrogen sulphide from broiler chicken droppings. Br Poult Sci. (2014) 55:745–51. doi: 10.1080/00071668.2014.960804
58. Nahm, KH. Feed formulations to reduce N excretion and ammonia emission from poultry manure. Bioresour Technol. (2007) 98:2282–300. doi: 10.1016/j.biortech.2006.07.039
59. Zheng, L, Oh, ST, Jeon, JY, Moon, BH, Kwon, HS, Lim, SU, et al. The dietary effects of fermented Chlorella vulgaris (CBT(®)) on production performance, liver lipids and intestinal microflora in laying hens. Asian Australas J Anim Sci. (2012) 25:261–6. doi: 10.5713/ajas.2011.11273
60. Yang, J, Qian, K, Zhang, W, Xu, Y, and Wu, Y. Effects of chromium-enriched bacillus subtilis KT260179 supplementation on chicken growth performance, plasma lipid parameters, tissue chromium levels, cecal bacterial composition and breast meat quality. Lipids Health Dis. (2016) 15:188. doi: 10.1186/s12944-016-0355-8
61. Emami, NK, White, MB, Calik, A, Kimminau, EA, and Dalloul, RA. Managing broilers gut health with antibiotic-free diets during subclinical necrotic enteritis. Poult Sci. (2021) 100:101055. doi: 10.1016/j.psj.2021.101055
62. Cheng, Y-H, Horng, Y-B, Chen, W-J, Hua, KF, Dybus, A, and Yu, YH. Effect of fermented products produced by Bacillus licheniformis on the growth performance and cecal microbial community of broilers under coccidial challenge. Animals. (2021) 11:1245. doi: 10.3390/ani11051245
63. Kathayat, D, Closs, G, Helmy, YA, Lokesh, D, Ranjit, S, and Rajashekara, G. Peptides affecting the outer membrane lipid asymmetry system (MlaA-OmpC/F) reduce avian pathogenic Escherichia coli (APEC) colonization in chickens. Appl Environ Microbiol. (2021) 87:e0056721. doi: 10.1128/AEM.00567-21
64. Ren, H, Su, X, Bai, H, Yang, Y, Wang, H, Dan, Z, et al. Specific enrichment of microbes and increased ruminal propionate production: the potential mechanism underlying the high energy efficiency of Holstein heifers fed steam-flaked corn. AMB Express. (2019) 9:209. doi: 10.1186/s13568-019-0937-8
65. Wen, C, Li, Q, Lan, F, Li, X, Li, G, Yan, Y, et al. Microbiota continuum along the chicken oviduct and its association with host genetics and egg formation. Poult Sci. (2021) 100:101104. doi: 10.1016/j.psj.2021.101104
66. Yan, J, Zhou, B, Xi, Y, Huan, H, Li, M, Yu, J, et al. Fermented feed regulates growth performance and the cecal microbiota community in geese. Poult Sci. (2019) 98:4673–84. doi: 10.3382/ps/pez169
67. Xiang, H, Gan, J, Zeng, D, Li, J, Yu, H, Zhao, H, et al. Specific microbial taxa and functional capacity contribute to chicken abdominal fat deposition. Front Microbiol. (2021) 12:643025. doi: 10.3389/fmicb.2021.643025
Keywords: laying hen, eggs quality, ammonia emissions, intestinal microflora, tea residues-fermented feed
Citation: Chen X, Zhou X, Li S, Zhang H and Liu Z (2023) Effects of tea residues-fermented feed on production performance, egg quality, antioxidant capacity, caecal microbiota, and ammonia emissions of laying hens. Front. Vet. Sci. 10:1195074. doi: 10.3389/fvets.2023.1195074
Edited by:
Fazul Nabi, Lasbela University of Agriculture, Water and Marine Sciences, PakistanReviewed by:
Sheeraz Mustafa, Ziauddin University, PakistanIn Ho Kim, Dankook University, Republic of Korea
Copyright © 2023 Chen, Zhou, Li, Zhang and Liu. This is an open-access article distributed under the terms of the Creative Commons Attribution License (CC BY). The use, distribution or reproduction in other forums is permitted, provided the original author(s) and the copyright owner(s) are credited and that the original publication in this journal is cited, in accordance with accepted academic practice. No use, distribution or reproduction is permitted which does not comply with these terms.
*Correspondence: Shiyi Li, shiyi.li2019@foxmail.com
†These authors have contributed equally to this work and share first authorship