Baicalin Alleviates LPS-Induced Oxidative Stress via NF-κB and Nrf2–HO1 Signaling Pathways in IPEC-J2 Cells
- Department of Veterinary Medicine, College of Coastal Agricultural Sciences, Guangdong Ocean University, Zhanjiang, China
Baicalin is a natural plant extract with anti-inflammatory and anti-oxidant activities. However, the molecular mechanism of baicalin on oxidative stress in IPEC-J2 cells exposed to LPS remains to be unclear. In this study, LPS stimulation significantly increased Toll-like receptor 4, tumor necrosis factor-α, and interleukins (IL-6 and IL-1β) expression in IPEC-J2 cells, and it activated the nuclear factor (NF-κB) expression. While, baicalin exerted anti-inflammatory effects by inhibiting NF-κB signaling pathway. LPS stimulation significantly increased the levels of the oxidative stress marker MDA, inhibited the anti-oxidant enzymes catalase and superoxide dismutase, which were all reversed by baicalin pre-treatment. It was found that baicalin treatment activated the nuclear import of nuclear factor-erythroid 2 related factor 2 (Nrf2) protein, and significantly increased the mRNA and protein expression of its downstream anti-oxidant factors such as heme oxygenase-1 and quinone oxidoreductase-1, which suggested that baicalin exerted anti-oxidant effects by activating the Nrf2-HO1 signaling pathway. Thus, pretreatment with baicalin inhibited LPS - induced oxidative stress and protected the normal physiological function of IPEC-J2 cells via NF-κB and Nrf2–HO1 signaling pathways.
Introduction
Diarrhea is one of the most common causes of piglet weaning mortality, and it has a significant influence on the pig industry (1). The development of diarrhea in piglets is usually accompanied by intestinal inflammation and oxidative stress, which in turn induces damage in the intestinal epithelium (2, 3). Intestinal epithelial cells (IECs) are important sites of nutrient digestion and absorption in mammals, as well as important barriers for the entry of pathogenic microorganisms and toxins into the circulatory system (4). The maintenance of normal physiological function in animals requires intact and healthy intestinal epithelial tissue (5). IPEC-J2 cells, a jejunal epithelial cell line isolated from newborn piglets, represent an important model for studying porcine intestinal function in vitro (6). IPEC-J2 are cell line used as model in many studies. For example, IPEC-J2 cells can be used to study the interaction between E. coli and its host, the damage of mycotoxin and cadmium exposure to pig intestines, and the regulatory effect of Lactobacillus salivarius on pig intestines (7). The occurrence of intestinal inflammation and oxidative stress will lead to the deterioration of intestinal barrier function, thereby permitting harmful substances to enter the blood and leading to the occurrence of digestive system diseases (8–10). Even more, some pathogens just like Samonella can also exploit inflammation to penetrate enterocytes after the intestinal barrier was disrupted (11, 12).
The inflammatory response is a type of resistance to foreign pathogens. Inflammatory factors produced by the body during the inflammatory response disrupt the normal physiological structure of the intestinal epithelium and play a role in resisting the invasion of foreign pathogens. However, excessive inflammatory responses can damage bodily tissues, thereby suppressing intestinal barrier function (13). IECs recognize extracellular stimuli through Toll-like receptors (TLRs) on the cell surface (14), which can recognize lipopolysaccharide (LPS), a tissue component of the outer wall of gram-negative bacteria; this leads to the stimulation of nuclear factor (NF-κB) mediated pro-inflammatory cytokine production and immune cell recruitment (15). The recruited immune cells secrete pro-inflammatory factors that induce apoptosis of IECs, thereby disrupting intestinal epithelial integrity (16).
Oxidative stress is induced when free radicals in the body attack tissues and cells (17). Under normal conditions, free radicals and other oxides are in a dynamic equilibrium with anti-oxidants in the body, and oxidative stress does not occur. When the balance is disrupted by some cause (e.g., acute hypoxia, drug toxicity, acute inflammatory response), the levels of highly oxygenated reactive factors (e.g., free radicals, reactive oxygen species [ROS]) are increased, which leads to environmental damage in the organism, including DNA, mitochondrial, and organelle damage (18), Under normal circumstances, Keap1 binds with actin in the cytoplasm, and Nuclear factor-erythroid 2 related factor 2 (Nrf2) is a crucial transcription factor associated in the response to oxidative stress (19). When oxidative stress occurs, Keap1 dissociates from Nrf2, which enters the nucleus and binds to its corresponding target sites in DNA to induce the synthesis of corresponding detoxification and anti-oxidant enzymes (20). Therefore, the body's endogenous anti-oxidant signaling pathway (Keap1–Nrf2/ARE) has a significant anti-oxidant stress effect.
Baicalin (C21H18O11) is a flavonoid extracted from the natural plant Scutellaria baicalensis that has biological functions such as anti-inflammatory (21), anti-oxidant (22), and anti-viral effects (23). For example, baicalin can reverse LPS-induced inflammation in H9c2 cells (24). Baicalin exerted anti-oxidant effects against lung injury (25). Moreover, it has good therapeutic effects in various animal models of inflammation (26, 27), and oxidative stress in vitro (28). However, few reports were focused on the oxidative stress response of inflammation in the intestine of piglets (29). Thus, the present study observed the specific mechanism of LPS-induces oxidative stress in IPEC-J2 cells and the therapeutic effects of baicalin.
Materials and Methods
Construction of the Experimental Model
Porcine IECs (IPEC-J2) (The IPEC-J2 cells obtained from the Guoqiang Zhu of China Yangzhou University) were cultured in Dulbecco's modified Eagle's medium (DMEM/F-12 (1:1) basic, C11330500BT, Gibco, USA) supplemented with 10% fetal bovine serum (FBS, Z7186FBS, ZETA, France), 50 IU/mL penicillin, and 50 μg/mL streptomycin (Penicillin-Streptomycin, 15140-122, Gibco) at 37°C in a 5% CO2 atmosphere. The medium was replaced 48 h after the initial cell culture. When cells reached 70% confluence, they were stimulated with 40 μg/mL LPS from Escherichia coli (L2880, Sigma, USA) for 1 h or 10 mol/mL H2O2 for 3 h after pretreatment with baicalin for 24 h.
Baicalin (C21H18O11, purity 95.4%, Jiu- Ling- Cao biotechnology Company, Wuhan, China), which can be found in the pharmacopeia of China, were stored at the room temperature. Firstly, baicalin was dissolved in the DMSO as mother liquor (400 μg/mL). Secondly, an appropriate amount of mother liquor was added to the cell culture medium. In this way, baicalin solution with the required concentration was prepared. To assess viability, equal numbers of IPEC-J2 cells were seeded in 96-well plates (Wuxi NEST Life Technology Co., Ltd) and DMEM/F12 medium with 10% FBS was used to grow the cells. After achieving 95% viability, the cells were rinsed two times with PBS, serum starved for 2 h, and treated with various doses of baicalin (4–64 μg/mL) for 24 h, followed by the addition of 10 μl of CCK-8 reagent (C0038, Dojindo, Japan) to each well. Cells were incubated at 37°C in 5% CO2 for 1 h before absorbance was measured at 450 nm using a microplate reader.
To determine cell viability after baicalin treatment, equal numbers of IPEC-J2 cells were seeded in 96-well plates and cultured in DMEM/F12 medium containing 10% FBS. After getting 95% confluence, the cells were rinsed two times with PBS, serum starved for 2 h, and treated with different concentrations of baicalin for 24 h (4–16 μg/mL). The cells were then serum-starved for 2 h and treated with 40 μg/mL LPS for 1 h before adding 10 μl of CCK-8 reagent to each well. IPEC-J2 cells were incubated at 37°C with 5% CO2 for 1 h before absorbance was measured at 450 nm using a microplate reader.
ELISA
After IPEC-J2 cells were cultured to 95% confluence in six-well plates (Wuxi NEST Life Technology Co., Ltd), they were rinsed two times with PBS, serum starved for 2 h, and treated with different concentrations of baicalin for 24 h (4–16 μg/mL). Cells were then washed twice with PBS and serum-starved for 2 h, and supernatant was collected after treatment with 40 μg/mL LPS for 1 h. IL-6 (MM-0418O2, Meimian, China), IL-1β (MM-0422O2, Meimian), TNF-α (MM-0383O2, Meimian), MDA (MM-191601, Meimian), SOD (MM-0450O2, Meimian), and CAT levels (MM-32849O2, Meimian) were examined via ELISA according to the manufacturer's instructions. Normalization was performed according to the concentration of cellular proteins. The experimental groups and drug concentrations used are presented in Table 1.
RNA Extraction, Reverse Transcription, and Real-Time PCR
RNA was extracted from IPEC-J2 cells using TRIzol reagent (Invitrogen, Waltham, MA, USA) according to the manufacturer's instructions, and RNA concentrations were determined using an OD1000 instrument. The isolated RNA was used to reverse-transcribe cDNA using EasyScript One-Step gDNA Removal and cDNA Synthesis SuperMix (AE311, EasyScript, China) according to the manufacturer's instructions, cDNA was amplified using EasyScript green qPCR SuperMix EasyScript Green qPCR SuperMix (AQ101, EasyScript) and specific primers. Reverse transcription-generated cDNA encoding β-actin, IL-6, IL-1β, IL-8 TNF-α, TLR4, HO-1, and NQO-1 was amplified by real-time PCR using selective primers (Table 2).
Western Blotting
Proteins were extracted from IPEC-J2 cells using RIPA lysis buffer (P0013B, Beyotime, China) and a Nuclear and Cytoplasmic Protein Extraction Kit (KGP1000, KeyGEN, China). Proteins were quantified using a BCA protein assay kit (KGP1100, KeyGEN). Proteins (20 μg/sample) were separated by SDS-PAGE (Tricine-SDS-PAGE Gel Kit, CW2384S, Cwbio, China), transferred to nitrocellulose membranes (88585, Pierce, Rockford, USA), and then hybridized with specific antibodies. The following antibodies were used: NF-κB p65 (#8242, Cell Signaling Technology, Danvers, MA, USA), phospho–NF-κB p65 (#3033, Cell Signaling Technology), IκBα (#4814, Cell Signaling Technology), phospho-IκBα (#2859, Cell Signaling Technology), Nrf2 (#12721, Cell Signaling Technology), anti–HO-1 (EP1391Y, Abcam, UK), lamin B1 (ab16048, Abcam, UK), and β-actin (CW0096, Cwbio). Total protein and cytoplasmic protein levels were normalized using β-actin expression to correct for differences in protein loading. Nuclear protein blots were normalized using lamin A/C to correct for differences in protein loading. Blots were visualized using an ECL detection system, and proteins were quantified using a ChemiDoc XRS+ image analyzer (Bio-Rad, Hercules, CA, USA).
Immunofluorescence
After reaching approximately 50% confluence, IPEC-J2 cells were treated with drugs, fixed using 4% paraformaldehyde on ice for 15 min, washed three times with PBS, permeabilized on ice with 0.2% Triton-X 100 for 5 min, and washed three times with PBS. Cells were then blocked with 1% fetal bovine serum for 30 min, incubated on ice with primary antibody (1:100 dilution) overnight, and washed three times with PBS. Subsequently, Biotin-SP (long spacer) AffiniPure Donkey Anti-Mouse IgG (H+L) (715-065-151, Jackson Immunoresearch, UK) diluted 1:100 was added dropwise at 37°C. After incubation for 1 h, cells were washed three times with PBS, and a 1:100 dilution of a594-sav (016-580-084, Jackson Immunoresearch, UK) was added dropwise at 37°C. After incubation for 1 h, cells were washed three times with PBS. DAPI (1:500 dilution) was added dropwise for 3 min at room temperature. Images of IPEC-J2 cells were acquired using a confocal microscope (Nikon, Tokyo, Japan). Antibodies against phospho–NF-κB p65 and Nrf2 were used.
Data Analysis
When analyzing more than three means, the Student's t-test or one-way analysis of variance were used, and Tukey's multiple comparison test was used. The results were presented as a mean ± SEM, and p < 0.05 denoting statistical significance. SPSS12.0 software (IBM Company, Chicago, USA) was used for statistical analysis, and Origin 6.0 (National Institute of Health NY, USA) was used to make graphs.
Results
Cytotoxicity of Baicalin and Its Effects in Inflammatory and Oxidative Stress Models
To select the appropriate concentration of baicalin for treating IPEC-J2 cells, cell viability was determined using the CCK-8 method after cells were incubated with different concentrations of baicalin for 24 h. Baicalin at 4 μg/mL (p < 0.05) significantly increased IPEC-J2 cell viability (Figure 1A). Baicalin treatment at 8–16 μg/mL for 24 h did not significantly alter the viability of IPEC-J2 cells (Figure 1A). IPEC-J2 cell viability was significantly inhibited following treatment with Baicalin at ≥ 32 μg/mL (p < 0.01) for 24 h (Figure 1A). To investigate the regulatory effect of baicalin on IPEC-J2 cells, we therefore created low- (4 μg/mL), middle- (8 μg/mL), and high-concentration groups (16 μg/mL). In an inflammation model, LPS (p < 0.05) significantly reduced IPEC-J2 cell viability (Figure 1B), whereas low, middle, and high concentrations of baicalin reversed this effect (p < 0.05, Figure 1B).
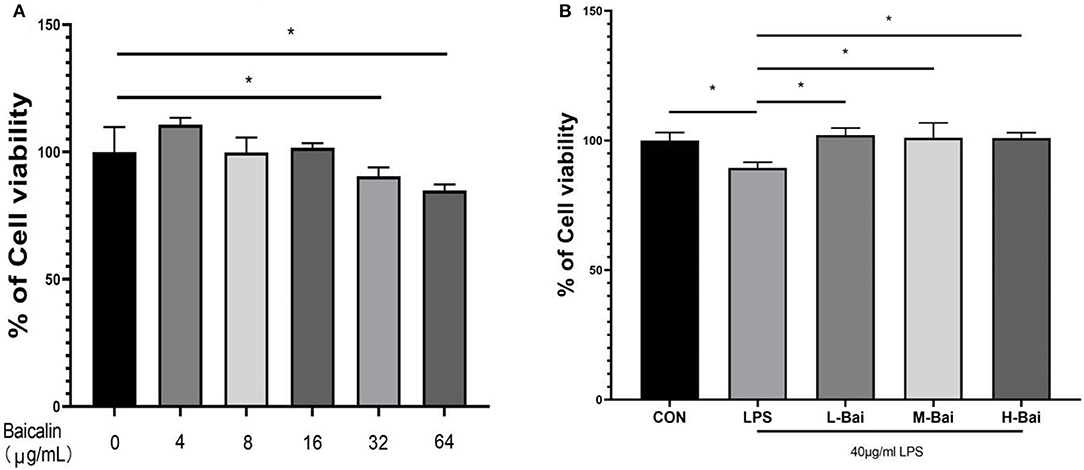
Figure 1. Cytotoxicity of baicalin in IPEC-J2 cells and the effect of baicalin on the viability of IPEC-J2 cells stimulated with LPS. Baicalin at 4 μg/mL increased the viability of IPEC-J2 cells, whereas 16 μg/mL baicalin was not cytotoxic to IPEC-J2 cells. Meanwhile, baicalin at concentrations of ≥ 64 μg/mL was significantly cytotoxic (A). LPS stimulation decreased IPEC-J2 cell viability, and this effect alleviated by baicalin pretreatment (B). Data are presented as the mean ± SEM (n = 6 per treatment, *p < 0.05 compared to the model group). CON, control; L-Bai, low-concentration baicalin; M-Bai, middle-concentration baicalin; H-Bai, high-concentration baicalin.
Effect of Baicalin on Inflammatory Factors in IPEC-J2 Cells
Interleukin IL-6, IL-1β,IL-8 and tumor necrosis factor TNF-α are main cellular inflammatory factors that play significant roles in the inflammatory response of IPEC-J2 cells. According to enzyme-linked immunosorbent assay (ELISA), LPS exposure significantly increased the mRNA expression of TLR4, IL-6, IL-1β, IL-8 and TNF-α (p < 0.05) in IPEC-J2 cells (Figures 2A–E). Low, middle, and high concentrations of baicalin (p < 0.05) significantly reversed the upregulation of IL-1β in IPEC-J2 cells induced by LPS (Figure 3C). Baicalin (p < 0.05) concentration-dependently reduced the mRNA expression of TLR4, IL-6, IL-8 and TNF-α in LPS-stimulated IPEC-J2 cells (Figures 2A,B,D,E). Meanwhile, LPS (p < 0.05) stimulation significantly increased IL-6 protein expression in IPEC-J2 cells (Figure 2H). Although low-concentration baicalin did not reverse the effect of LPS on IL-6 expression, the middle and high concentrations of this drug significantly suppressed IL-6 protein expression (p < 0.05, Figure 2H. LPS (p < 0.05) stimulation significantly upregulated IL-1β and IL-8 protein expression in IPEC-J2 cells (Figures 2G,I),which was reduced by middle and high concentrations of baicalin (p < 0.05, Figures 2G,I). LPS (p < 0.05)stimulation concentrations of baicalin reverse this effect (p < 0.05, Figure 2F). significantly increased TNF-α protein expression in IPEC-J2 cells (Figure 2F), and all concentrations of baicalin reverse.
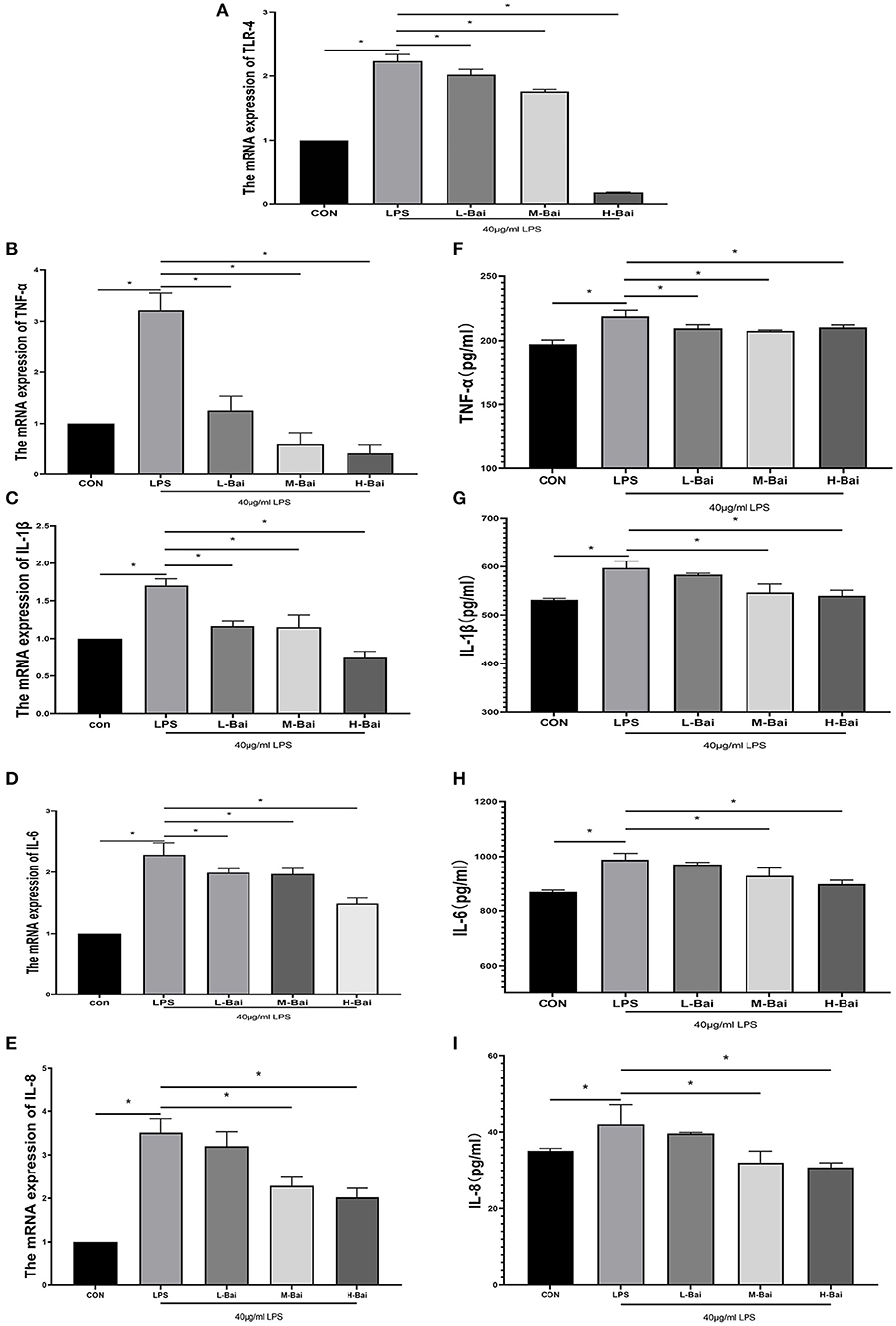
Figure 2. Effects of baicalin on inflammatory factor expression in LPS-stimulated IPEC-J2 cells. Quantitative effects of baicalin on TLR4 (A), TNF-α (B), IL-1β (C), IL-6 (D) and IL-8 (E) mRNA expression in IPEC-J2 cells. Effects of baicalin on TNF-α (F), IL-1β (G), IL-6 (H) and IL-8 (I) protein expression in IPEC-J2 cells. Data are presented as the mean ± SEM (n = 3 per treatment, *p < 0.05 compared with the model group). CON, control; L-Bai, low-concentration baicalin; M-Bai, middle-concentration baicalin; H-Bai, high-concentration baicalin.
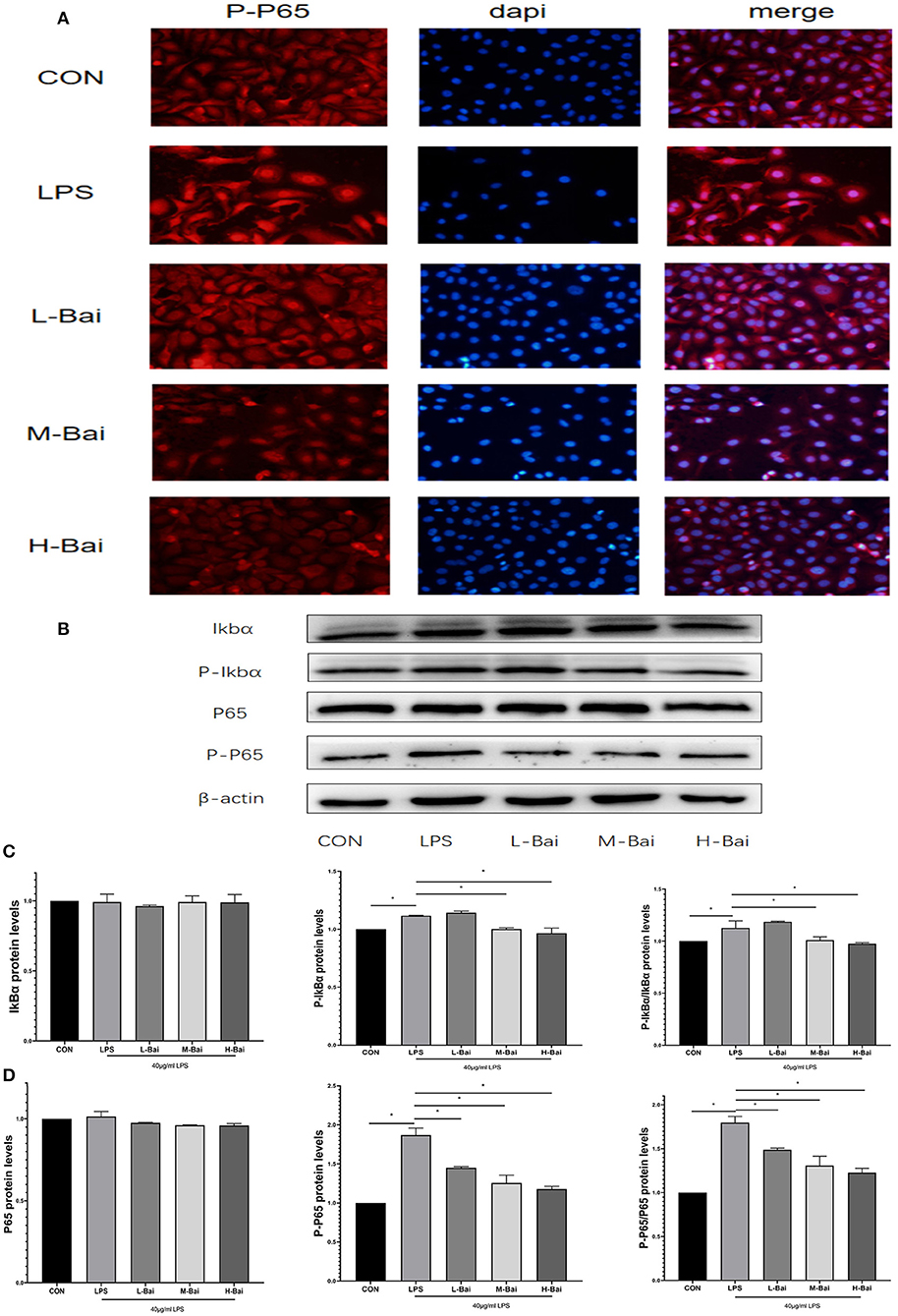
Figure 3. Effects of baicalin on key proteins in the NF-κB signaling pathway in LPS-stimulated IPEC-J2 cells. Immunofluorescence detection of P-p65 protein nuclear import in IPEC-J2 cells (A). Western blotting was used to determine the expression of p65, P-p65, IκBα, and P-IκBα (B). p65 and P-p65 protein expression and their ratio (C). IκBα and P-IκBα protein expression and their ratio (D). Data are presented as the mean ± SEM (n = 3 per treatment, *p < 0.05 compared with the model group). CON, control; L-Bai, low-concentration baicalin; M-Bai, middle-concentration baicalin; H-Bai, high-concentration baicalin.
Effect of Baicalin on NF-κB Expression and Signaling in IPEC-J2 Cells
The NF-κB signaling pathway is important for the inflammatory response. NF-κB signaling proteins in IPEC-J2 cells were detected by western blotting. Fluorescence microscopy of normal IPEC-J2 cells is presented in Figure 3A. LPS stimulation significantly increased the entry of P-p65 protein into the nuclei of IPEC-J2 cells. However, baicalin concentration-dependently reversed this effect (Figure 3A). LPS stimulation and baicalin treatment did not significantly affect p65 and inhibitor of κB alpha (IκBα) protein expression in IPEC-J2 cells (Figures 3C,D). LPS (p < 0.05) stimulation dramatically increased the protein expression of P-IκBα in IPEC-J2 cells, and this effect was canceled by the middle and high concentrations of baicalin (Figures 3B,D). The ratio of P-IκBα to IκBα displayed a similar trend as P-IκBα expression (Figure 3D). Stimulation with LPS (p < 0.05) significantly increased the protein expression of P-P65 in IPEC-J2 cells, whereas baicalin treatment reversed this effect in a concentration-dependent manner (p < 0.05, Figures 3C,D). The ratio of P-p65 to p65 exhibited a similar trend as P-p65 expression (Figure 3C).
Effect of Baicalin on Oxidase and Malondialdehyde (MDA) Levels in an IPEC-J2 Cell Oxidation Model
In an oxidative stress model, H2O2 (p < 0.05) significantly inhibited the viability of IPEC-J2 cells (Figure 4A), and this effect was only reversed by high-concentration baicalin (p < 0.05, Figure 4A).
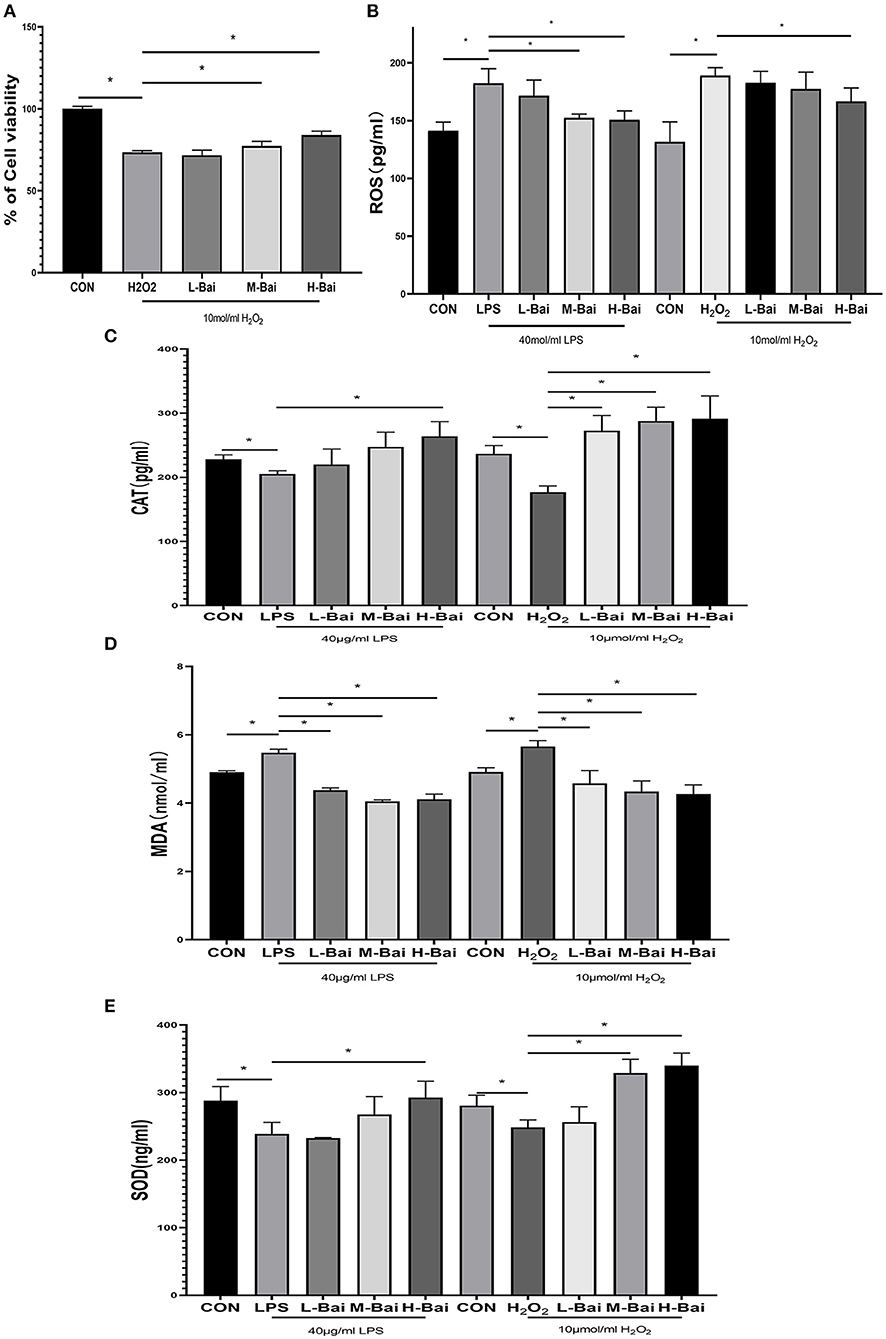
Figure 4. Effects of baicalin on anti-oxidant enzymes and oxidative indices in LPS-stimulated IPEC-J2 cells. H2O2 stimulation decreased the viability of IPEC-J2 cells, and this effect was alleviated by baicalin pretreatment (A). Effect of baicalin ROS (B), SOD (C), CAT (D), and MDA (E) levels in IPEC-J2 cells stimulated with LPS and H2O2. Data are presented as the mean ± SEM (n = 3 per treatment, *p < 0.05 compared with the model group). CON, control; L-Bai, low-concentration baicalin; M-Bai, middle-concentration baicalin; H-Bai, high-concentration baicalin.
Superoxide dismutase (SOD), catalase (CAT), MDA, and reactive oxygen species (ROS) have important roles in the induction of oxidative stress, and thus, we used ELISA to detect their levels in IPEC-J2 cells. LPS and H2O2 (p < 0.05) stimulation significantly reduced SOD and CAT expression in IPEC-J2 cells, and this effect was reversed by baicalin treatment (Figures 4C,E). Meanwhile, LPS (p < 0.05) stimulation enhanced MDA and ROS levels, and these changes were similar reversed by baicalin exposure (Figures 4B,D).
Effect of Baicalin on Nrf2 Signaling in IPEC-J2 Cells
The signaling pathways for Nrf2 and heme oxygenase-1 (HO-1) are essential in oxidative stress. In IPEC-J2 cells, LPS and H2O2 treatment significantly elevated HO-1 mRNA expression (p < 0.05), which was reversed by baicalin in a concentration-dependent manner (p < 0.05, Figure 5A) Meanwhile H2O2, but not LPS, significantly increased quinone oxidoreductase-1 (NQO-1) mRNA expression in IPEC-J2 cells, whereas baicalin (p < 0.05) significantly increased NQO-1 mRNA expression at all examined concentrations (Figure 5B).
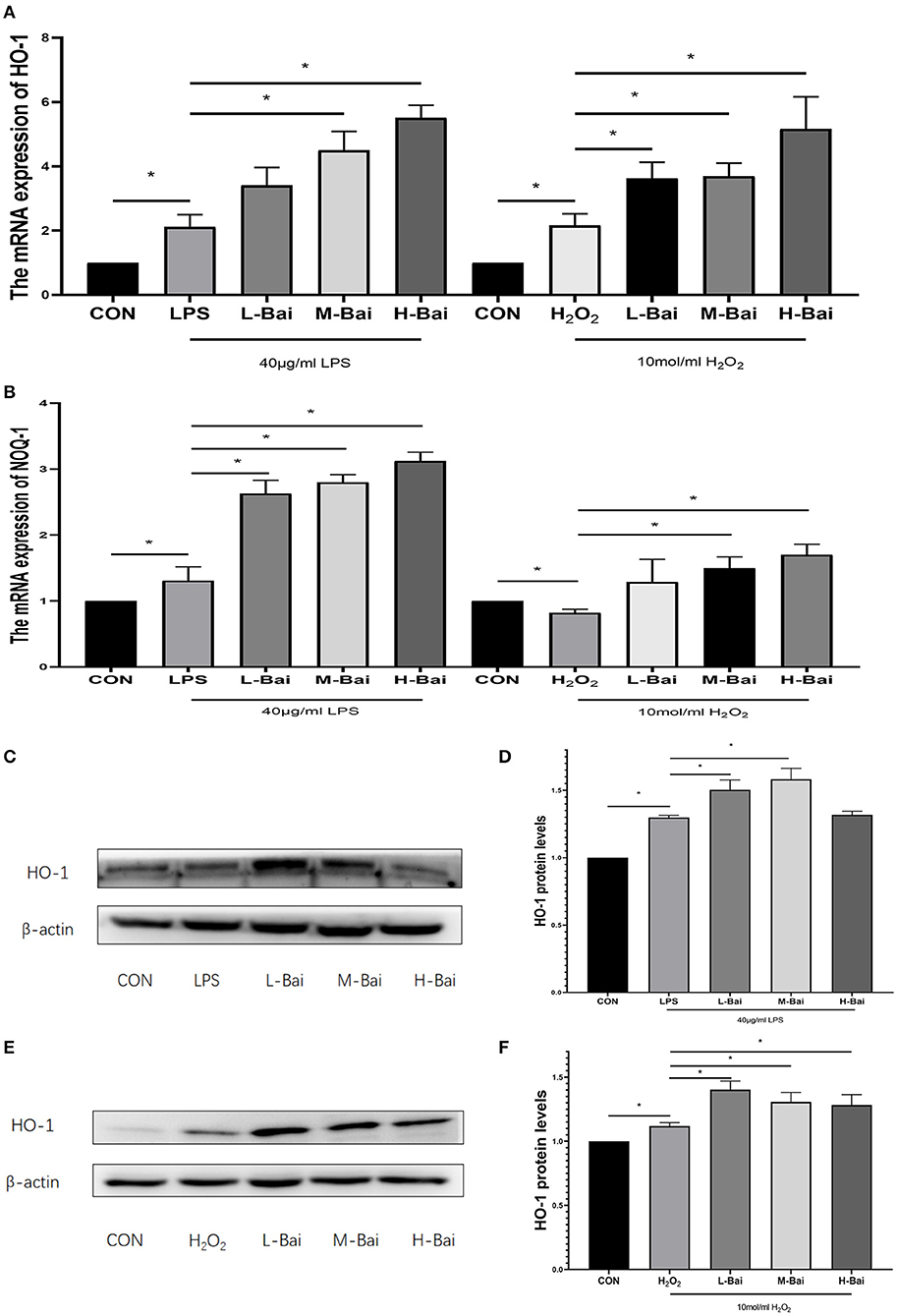
Figure 5. Effects of baicalin on key proteins and mRNAs in the Nrf2 signaling pathway in IPEC-J2 cells. Effect of baicalin on HO-1 mRNA expression in IPEC-J2 cells stimulated by LPS and H2O2 as determined by qPCR (A). Effect of baicalin on NQO-1 mRNA expression in IPEC-J2 cells stimulated by LPS and H2O2 as determined by qPCR (B). Immunofluorescence detection of nuclear Nrf2 protein expression in IPEC-J2 cells (C). Western blotting was used to determine the expression of HO-1, Nrf2, β-actin, and lamin B (D). HO-1 protein expression (E).Cytoplasmic and nuclear Nrf2 protein expression (F). Data are presented as the mean ± SEM (n = 3 per treatment, *p < 0.05 compared with the model group). CON, control; L-Bai, low-concentration baicalin; M-Bai, middle-concentration baicalin; H-Bai, high-concentration baicalin.
LPS and H2O2 (p < 0.05) stimulation significantly increased HO-1 protein expression, and its expression was further increased by pretreatment with low and middle concentrations of baicalin (p < 0.05, Figures 5C–F).
According to fluorescence microscopy, LPS stimulation significantly increased Nrf2 protein accumulation in the nucleus in IPEC-J2 cells (Figure 6A), and further increases were observed in the presence of low and middle concentrations of baicalin. Meanwhile, high-concentration baicalin (p < 0.05) significantly increased Nrf2 protein expression in the cytoplasm of IPEC-J2 cells (Figures 6B,C). LPS (p < 0.05) stimulation significantly increased nuclear Nrf2 expression in IPEC-J2 cells, whereas middle- and high-concentration baicalin exposure further increased the nuclear accumulation of Nrf2 (Figures 6B,D).
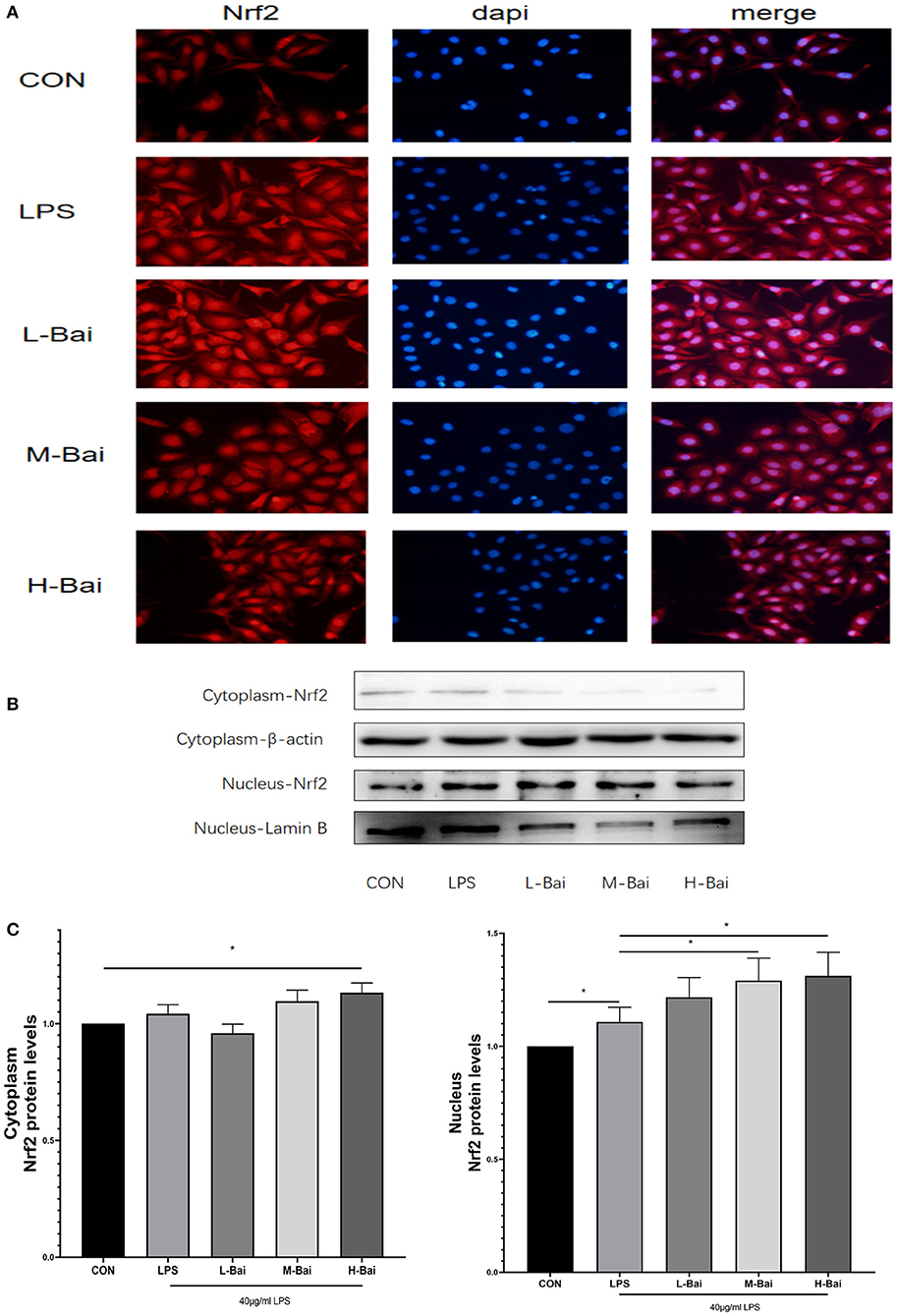
Figure 6. Effects of baicalin on key proteins in the Nrf2 signaling pathway in LPS-stimulated IPEC-J2 cells. Immunofluorescence detection of nuclear Nrf2 protein import in IPEC-J2 cells (A). Western blotting was used to determine the expression of HO-1, Nrf2, β-actin, and lamin B (B). Cytoplasmic and nuclear Nrf2 protein expression (C). Data are presented as the mean ± SEM (n = 3 per treatment, *p < 0.05 compared with the model group). CON, control; L-Bai, low-concentration baicalin; M-Bai, middle-concentration baicalin; H-Bai, high-concentration baicalin.
Similarly, H2O2 stimulation significantly increased Nrf2 protein accumulation in the nucleus in IPEC-J2 cells (Figure 7A), and this effect was potentiated by baicalin. H2O2 (p < 0.05) stimulation also significantly increased the cytoplasmic expression of Nrf2 (Figure 7C), but baicalin did not further increase Nrf2 protein expression in the cytoplasm.
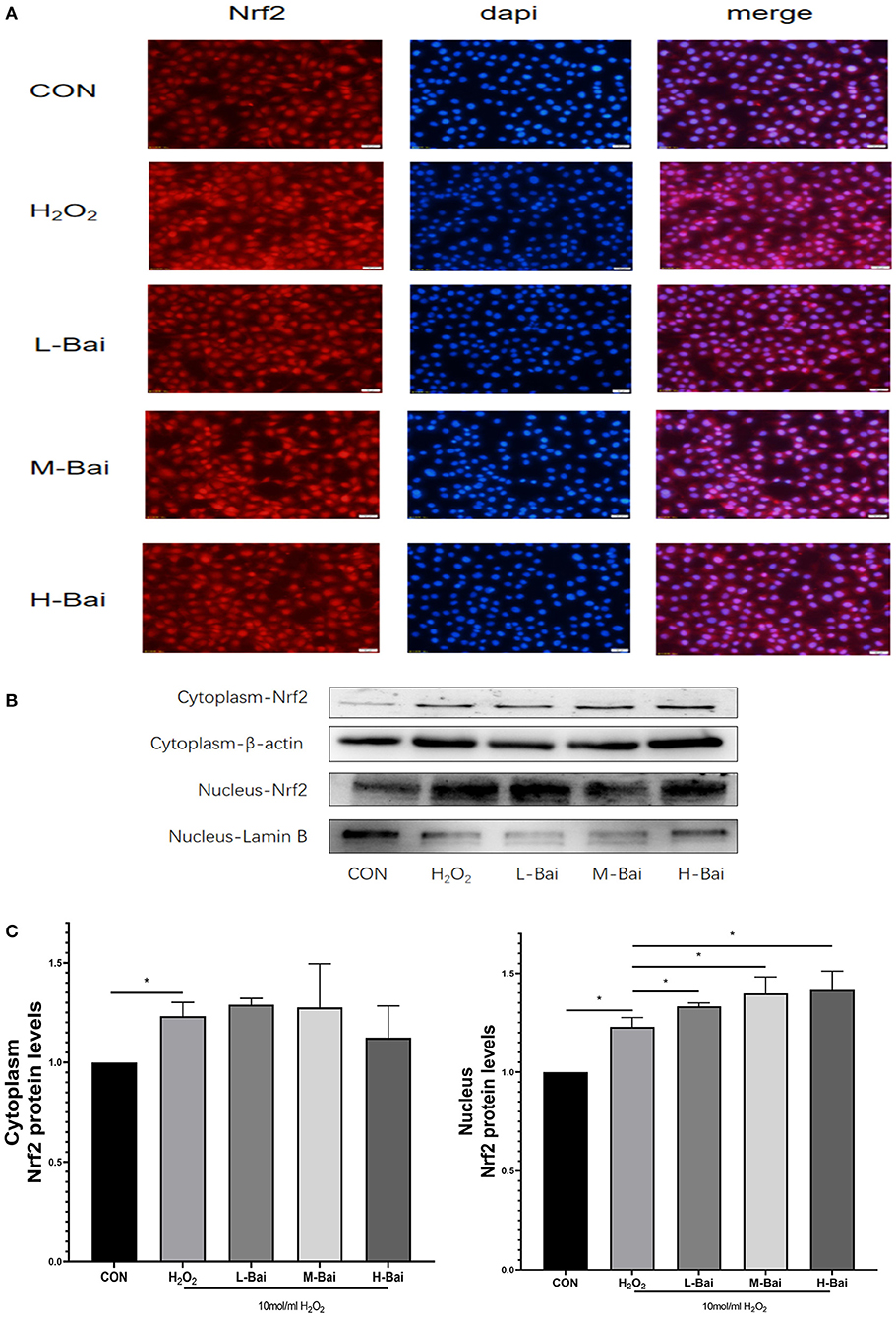
Figure 7. Effects of baicalin on key proteins in the Nrf2 signaling pathway in H2O2-stimulated IPEC-J2 cells. Immunofluorescence detection of nuclear Nrf2 protein import in IPEC-J2 cells (A). Western blotting was used to determine the expression of HO-1, Nrf2, β-actin, and lamin B (B). Cytoplasmic and nuclear Nrf2 protein expression (C). Data are presented as the mean ± SEM (n = 3 per treatment, *p < 0.05 compared with the model group). CON, control; L-Bai, low-concentration baicalin; M-Bai, middle-concentration baicalin; H-Bai, high-concentration baicalin.
Discussion
Our result proved that baicalin might be used as a potential medicine for the treatment of piglets diarrhea by the way of anti-inflammatory and antioxidant. The concentration range of baicalin was selected by the viabilities of IPEC-J2 cells. Baicalin within the concentration 0–16 μg/mL had no obvious cytotoxicity to IPEC-J2 cells, but when the concentration of baicalin was greater than or equal to 32 μg/mL, it would significantly decrease the cell viabilities. Baicalin pretreatment enhanced viability, reduced inflammatory responses, and enhanced anti-oxidant enzyme activity in IPEC-J2 cells. Low-concentration baicalin had enhanced the viability of IPEC-J2 cells, which may be related to the biological function of baicalin in promoting cell proliferation (32). The viability of IPEC-J2 cells was considerably reduced by LPS and H2O2 stimulation, which was reversed by pretreatment with baicalin. So, pretreatment of IPEC-J2 cells with baicalin at concentrations less than 16 μg/mL can safely and effectively enhance cell viability. As a result, 4, 8 and 16 μg/mL of baicalin were determined as the low, medium and high doses respectively. TLRs are important receptors on the cell surface (33). TLR4 can recognize extracellular stimulation and then activate the NF-κB signaling pathway, which stimulates P-p65 protein accumulation in the nucleus and the subsequent induction of inflammatory responses, promoting the secretion of inflammatory factor such as TNF-α, IL-6, IL-1β and IL-8 (34). Moreover, IL-8 has the biological function of organizing pathogen invasion and eliminating pathogen as a chemokine (35). In this study, LPS stimulation significantly increased TLR4 mRNA expression in IPEC-J2 cells, similar to previous findings (36). Moreover, LPS stimulation significantly increased the protein and mRNA expression of pro-inflammatory factors (TNF-α, IL-6, IL-1β and IL-8) in IPEC-J2 cells (37), whereas pretreatment with baicalin concentration-dependently reversed this trend, indicating that baicalin did have capacity to suppress LPS-induced inflammation in IPEC-J2 cells. Baicalin can reduce LPS induced IL-8 secretion at mRNA and protein levels, suggesting that baicalin may play a key role in preventing pathogen invasion. Further, the protein expression of p65 and IκBα which were important components of the NF-κB signaling pathway were investigated (38, 39). LPS stimulation significantly increased P-p65 and P-IκBα protein expression, and phosphorylation of p65 increased its nuclear accumulation in IPEC-J2 cells. After entering the nucleus, P-P65 protein can induce the expression of the downstream inflammatory factors TNF-α, IL-6, IL-1β and IL-8 to initiate an inflammatory response (40). The results of immunofluorescence indicated that LPS stimulation significantly increased the nuclear entry of P-p65 in IPEC-J2 cells, whereas baicalin pretreatment exerted a concentration-dependent inhibitory effect on the nuclear entry of P-p65. It illustrated that baicalin alleviated the inflammatory responses of IPEC-J2 cells by inhibiting TLR4–NF-κB signaling pathway.
Oxidative stress can cause cells to undergo death and irreparable oxidative damage ROS (41, 42), which can cause oxidative stress and then trigger cell damage (43). It is generally believed that excessively ROS levels and the decreased antioxidant enzyme and propylene glycol (MDA) levels indicate the occurrence of oxidative stress. By contrast with the LPS-induced oxidative stress model, an oxidative stress model was created by treating IPEC-J2 cells with 10 mol/mL H2O2, as previously reported (44, 45). In the H2O2 -induced oxidative stress model, baicalin significantly increased the mRNA expression of HO-1 and NQO-1 and significantly increased nuclear protein expression of Nrf2, which revealed that baicalin could alleviate oxidative stress responses in IPEC-J2 cells. Previous studies revealed that LPS can excessive ROS production, which can cause oxidative stress and then trigger cell damage (46). Therefore, we detected the levels of ROS, MDA, and the anti-oxidant enzymes SOD and CAT in IPEC-J2 cells. LPS stimulation elevated MDA levels in IPEC-J2 cells, suggesting that oxidative stress occurs in this model. CAT and SOD play important roles in maintaining normal cellular redox balance (47). LPS stimulation significantly decreased CAT and SOD levels in IPEC-J2 cells, but pretreatment with baicalin decreased MDA levels and increased CAT and SOD levels. This indicates that baicalin can inhibit oxidative stress induced by LPS in IPEC-J2 cells. It can also be seen that baicalin ameliorates oxidative stress in the spleen of chickens during Mycoplasma gallisepticum infection (48). In the bovine endometrial epithelial cells, andrographolide could attenuates LPS-indcued inflammatory response via activating Nrf2 signaling pathway (49), while Nrf2 may also be an important target of baicalin. Baicalin-pretreated IPEC-J2 cells exhibited significantly increased HO-1 and NQO-1 mRNA expression, increased HO-1 protein expression, and significantly increased nuclear Nrf2 protein expression relative to the findings in LPS-treated IPEC-J2 cells. Baicalin activates the Nrf2 signaling pathway, which alleviates LPS-induced oxidative stress in IPEC-J2 cells. In this study, baicalin significantly inhibited inflammation and oxidative stress in IPEC-J2 cells. Notably, HO-1 protein expression was higher in the high-concentration baicalin group than in the middle-concentration group, which may be related to the negative feedback regulation in cells (50). High-concentration baicalin significantly increased the expression of anti-oxidant enzymes, which may reduce HO-1 protein expression. These results illustrated that baicalin could exert anti-oxidant activity in the oxidative stress model of IPEC-J2 cells by activating the Nrf2 signaling pathway.
Although it is widely established that H2O2 can cause oxidative stress in cells by creating excessive ROS, but how LPS stimulation causes oxidative stress in the intestine of piglets is still unknown. It was believed that TNF-α can promote oxidative stress (25). In differentiated bone marrow-derived macrophages, TNF-α induced oxidative stress through the Keap1–Nrf2–TNF-α axis, which increased ROS accumulation (51). In Gpx4-deficient cells, inflammatory cytokines (TNF-α) could create a positive feedback loop involving ROS production, inflammation, and mutagenesis (52). In this study, LPS exposure enhance ROS levels and the expression of pro-inflammation cytokines such as TNF-α, IL-1β IL-6 and IL-8. Thus, it was supposed that inflammatory cytokines, especially TNF-α, might be the main factors of ROS production. LPS activated the NF-κB signaling pathway, increased the production of inflammatory factors and ROS, and decreased the expression of anti-oxidant enzymes, all of which promoted oxidative stress in IPEC-J2 cells. ROS content was similar in LPS- and H2O2-treated IPEC-J2 cells; however, ROS levels in baicalin-pretreated cells in the LPS-induced oxidative stress model were lower than that in the H2O2-induced oxidative stress model, which may be attributable to decreased cytokine expression and increased NQO-1 mRNA expression following baicalin exposure. In the process of LPS-induced oxidative stress, baicalin significantly suppressed LPS-induced cytokines by inhibiting the NF-κB signaling' pathway, activating the Nrf2 signaling' pathway, increasing the expression of anti-oxidant enzymes, and reducing the levels of ROS. Thus, baicalin can alleviate LPS-induced oxidative stress by inhibiting the NF-κB signaling pathway and stimulating the Nrf2–HO-1 signaling pathway in IPEC-J2 cells (Figure 8). In the process of LPS-induced oxidative stress, it seems that cytokines, especially TNF-α, seem to critical factors to crosstalk the inflammation response with oxidative stress, which need our deep research. Furthermore, we still want to explore how baicalin regulates the anti-inflammatory and antioxidant response in the intestine of piglets in vivo.
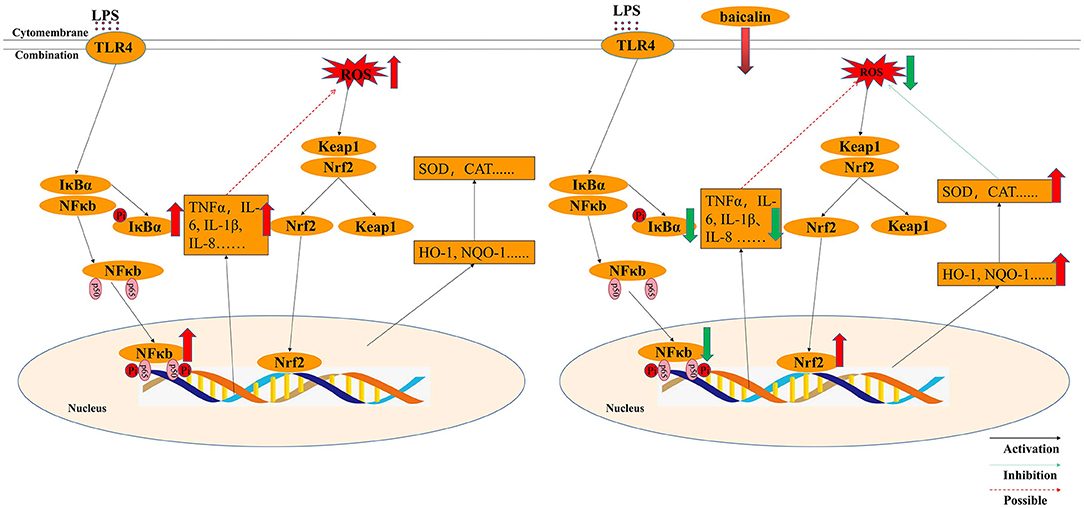
Figure 8. Molecular mechanism by which baicalin alleviated LPS-induced inflammatory responses and oxidative stress model in IPEC-J2 cells.
Conclusion
In this paper, it was proved that LPS induced the process of oxidative stress via NF-κB and Nrf2–HO1 signaling pathways, which was familiar with the process of H2O2-induced oxidative stress. Baicalin can reduce inflammatory response by inhibiting NF-κB signal pathway, and it can reduce oxidative stress response by stimulating Nrf2-HO-1 signal pathway. All in all, pretreatment with baicalin can inhibite LPS-induced oxidative stress and protect the normal physiological function of IPEC-J2 cells via NF-κB and Nrf2–HO1 signaling pathways.
Data Availability Statement
The original contributions presented in the study are included in the article/Supplementary Material, further inquiries can be directed to the corresponding author/s.
Author Contributions
MB, XL, and SM: conceptualization, writing-review, and editing. MB, ML, XS, and XM: analysis. SC, JW, and ZY: data curation. MB: writing-original draft preparation. XL, YY, and XJ: supervision. XL and XJ: project administration, methodology, and funding acquisition. All authors contributed to the article and approved the submitted version.
Funding
This work was supported by grants from the National Natural Science Foundation of China [No. 31902314], the Natural Science Foundation of Guangdong Province, China (No. 2019A1515011142), the Nanhai Scholars Program of Guangdong Ocean University (No. 002029002005) and the Program for Scientific Research Start-Up Funds of Guangdong Ocean University (101402/R17088), Shenzhen Projects for Basic Research [JCYJ20190813142005766] and Project of Enhancing School with Innovation of Guangdong Ocean University (GDOU230419057).
Conflict of Interest
The authors declare that the research was conducted in the absence of any commercial or financial relationships that could be construed as a potential conflict of interest.
Publisher's Note
All claims expressed in this article are solely those of the authors and do not necessarily represent those of their affiliated organizations, or those of the publisher, the editors and the reviewers. Any product that may be evaluated in this article, or claim that may be made by its manufacturer, is not guaranteed or endorsed by the publisher.
Supplementary Material
The Supplementary Material for this article can be found online at: https://www.frontiersin.org/articles/10.3389/fvets.2021.808233/full#supplementary-material
References
1. Chan G, Farzan A, Soltes G, Nicholson VM, Pei Y, Friendship R, et al. The epidemiology of Clostridium perfringens type A on ontario swine farms, with special reference to cpb2-positive isolates. BMC Vet Res. (2012) 8:156. doi: 10.1186/1746-6148-8-156
2. Zeng Y, Wang Z, Zou T, Chen J, Li G, Zheng L, et al. Bacteriophage as an alternative to antibiotics promotes growth performance by regulating intestinal inflammation, intestinal barrier function and gut microbiota in weaned piglets. Front Vet Sci. (2021) 8:2. doi: 10.3389/fvets.2021.623899
3. Sun Z, Li H, Li Y, Qiao J. Lactobacillus salivarius, a potential probiotic to improve the health of LPS-challenged piglet intestine by alleviating inflammation as well as oxidative stress in a dose-dependent manner during weaning transition. Front Vet Sci. (2020) 7:1089. doi: 10.3389/fvets.2020.547425
4. Campbell JM, Crenshaw JD, Polo J. The biological stress of early weaned piglets. J Anim Sci Biotechno. (2013) 4:19. doi: 10.1186/2049-1891-4-19
5. Neunlist M, Van Landeghem L, Mahé MM, Derkinderen P, des Varannes SB, Rolli-Derkinderen M. The digestive neuronal–glial–epithelial unit: a new actor in gut health and disease. Nat Rev Gastro Hepat. (2013) 10:90–100. doi: 10.1038/nrgastro.2012.221
6. Shi L, Fang B, Yong Y, Li X, Gong D, Li J, et al. Chitosan oligosaccharide-mediated attenuation of LPS-induced inflammation in IPEC-J2 cells is related to the TLR4/NF-κB signaling pathway. Carbohyd Polym. (2019) 219:269–79. doi: 10.1016/j.carbpol.2019.05.036
7. Horgan K, Jacques K, Madden G. 214 Yeast cell wall mannan rich fraction reduces the ability of enterotoxigenic E.coli (ETEC) to attach to porcine intestinal cells. J Anim Sci. (2018) 96:114. doi: 10.1093/jas/sky073.211
8. Xu X, Yan G, Chang J, Wang P, Yin Q, Liu C, et al. Astilbin ameliorates deoxynivalenol-induced oxidative stress and apoptosis in intestinal porcine epithelial cells (IPEC-J2). J Appl Toxicol. (2020) 40:1362–72. doi: 10.1002/jat.3989
9. Sánchez De Medina F, Romero-Calvo I, Mascaraque C, Martínez-Augustin O. Intestinal inflammation and mucosal barrier function. Inflamm Bowel Dis. (2014) 20:2394–404. doi: 10.1097/MIB.0000000000000204
10. Qiu Y, Yang X, Wang L, Gao K, Jiang Z. L-arginine inhibited inflammatory response and oxidative stress induced by lipopolysaccharide via arginase-1 signaling in IPEC-J2 Cells. Int J Mol Sci. (2019) 20:1800. doi: 10.3390/ijms20071800
11. Barbara C, Michele P, Rosanna D, Jessica R, Elisabetta R, Claudia P, et al. Salmonella Typhimurium exploits inflammation to its own advantage in piglets. Front Microbiol. (2015) 6:985. doi: 10.3389/fmicb.2015.00985
12. Liu X, Li H, Lu A, Zhong Y, Hou X, Wang N, et al. Reduction of intestinal mucosal immune function in heat-stressed rats and bacterial translocation. Int J Hyperther. (2012) 28:756–65. doi: 10.3109/02656736.2012.729173
13. Söderholm JD, Olaison G, Peterson KH, Franzén LE, Lindmark T, Wirén M, et al. Augmented increase in tight junction permeability by luminal stimuli in the non-inflamed ileum of Crohn's disease. Gut. (2002) 50:307. doi: 10.1136/gut.50.3.307
14. Zhao L, Li M, Sun K, Su S, Geng T, Sun H. Hippophae rhamnoides polysaccharides protect IPEC-J2 cells from LPS-induced inflammation, apoptosis and barrier dysfunction in vitro via inhibiting TLR4/NF-κB signaling pathway. Int J Biol Macromol. (2020) 155:1202–15. doi: 10.1016/j.ijbiomac.2019.11.088
15. Bein A, Zilbershtein A, Golosovsky M, Davidov D, Schwartz B. LPS induces hyper-permeability of intestinal epithelial cells. J Cell Physiol. (2017) 232:381–90. doi: 10.1002/jcp.25435
16. Brandtzaeg P. The gut as communicator between environment and host: Immunological consequences. Eur J Pharmacol. (2011) 668:S16–32. doi: 10.1016/j.ejphar.2011.07.006
17. Sack MN, Fyhrquist FY, Saijonmaa OJ, Fuster V, Kovacic JC. Basic biology of oxidative stress and the cardiovascular system: part 1 of a 3-part series. J Am Coll Cardiol. (2017) 70:196–211. doi: 10.1016/j.jacc.2017.05.034
18. Fandy TE, Jiemjit A, Thakar M, Rhoden P, Suarez L, Gore SD. Decitabine induces delayed reactive oxygen species (ROS) accumulation in leukemia cells and induces the expression of ros generating enzymes. Clin Cancer Res. (2014) 20:1249. doi: 10.1158/1078-0432.CCR-13-1453
19. Yang X, Yao W, Shi H, Liu H, Li Y, Gao Y, et al. Paeoniflorin protects Schwann cells against high glucose induced oxidative injury by activating Nrf2/ARE pathway and inhibiting apoptosis. J Ethnopharmacol. (2016) 185:361–9. doi: 10.1016/j.jep.2016.03.031
20. Li L, Dong H, Song E, Xu X, Liu L, Song Y. Nrf2/ARE pathway activation, HO-1 and NQO1 induction by polychlorinated biphenyl quinone is associated with reactive oxygen species and PI3K/AKT signaling. Chem-Biol Interact. (2014) 209:56–67. doi: 10.1016/j.cbi.2013.12.005
21. Huang Y, Sun M, Yang X, Ma A, Ma Y, Zhao A. Baicalin relieves inflammation stimulated by lipopolysaccharide via upregulating TUG1 in liver cells. J Physiol Biochem. (2019) 75:463–73. doi: 10.1007/s13105-019-00698-0
22. Pan Y, Chen D, Lu Q, Liu L, Li X, Li Z. Baicalin prevents the apoptosis of endplate chondrocytes by inhibiting the oxidative stress induced by H2O2. Mol med rep. (2017) 16:2985–91. doi: 10.3892/mmr.2017.6904
23. Zhang Y, Li X, Ciric B, Ma C, Gran B, Rostami A, et al. Therapeutic effect of baicalin on experimental autoimmune encephalomyelitis is mediated by SOCS3 regulatory pathway. Sci Rep. (2015) 5:17407. doi: 10.1038/srep17407
24. Liu X, Wang S, Zhao G. Retracted: baicalin relieves lipopolysaccharide-evoked inflammatory injury through regulation of miR-21 in H9c2 cells. Phytother Res. (2020) 34:1134–41. doi: 10.1002/ptr.6583
25. Meng X, Hu L, Li W. Baicalin ameliorates lipopolysaccharide-induced acute lung injury in mice by suppressing oxidative stress and inflammation via the activation of the Nrf2-mediated HO-1 signaling pathway. Naunyn Schmiedebergs Arch Pharmacol. (2019) 392:1421–33. doi: 10.1007/s00210-019-01680-9
26. Cheng P, Wang T, Li W, Muhammad I, Wang H, Sun X, et al. Baicalin alleviates lipopolysaccharide-induced liver inflammation in chicken by suppressing TLR4-mediated NF-κB pathway. Front Pharmacol. (2017) 8:547. doi: 10.3389/fphar.2017.00547
27. Shi H, Zhang Y, Xing J, Liu L, Qiao F, Li J, et al. Baicalin attenuates hepatic injury in non-alcoholic steatohepatitis cell model by suppressing inflammasome-dependent GSDMD-mediated cell pyroptosis. Int Immunopharmacol. (2020) 81:106195. doi: 10.1016/j.intimp.2020.106195
28. Gong L, Zhu J. Baicalin alleviates oxidative stress damage in trabecular meshwork cells in vitro. Naunyn Schmiedebergs Arch Pharmacol. (2018) 391:51–8. doi: 10.1007/s00210-017-1433-9
29. Guo Q, Xuan MF, Luo ZB, Wang JX, Kang JD. Baicalin improves the in-vitro developmental capacity of pig embryos by inhibiting apoptosis, regulating mitochondrial activity and activating sonic hedgehog signaling. Mol Hum Reprod. (2019) 25:538–49. doi: 10.1093/molehr/gaz036
30. Chen S, Zhang Y, Niu X, Mohyuddin SG, Wen J, Bao M, et al. Coral-derived endophytic fungal product, butyrolactone-I, alleviates Lps induced intestinal epithelial cell inflammatory response through TLR4/NF-κB and MAPK signaling pathways: an in vitro and in vivo studies. Front Nutr. (2021) 8:748118. doi: 10.3389/fnut.2021.748118
31. Liu X, Liu F, Ma Y, Li H, Ju X, Xu J, et al. Effect of puerarin, baicalin and berberine hydrochloride on the regulation of IPEC-J2 Cells infected with enterotoxigenic Escherichia coli. Evid Based Compl Alt. (2019) 2019:7438593. doi: 10.1155/2019/7438593
32. Perruchot M-H, Gondret F, Robert F, Dupuis E, Quesnel H. Effect of the flavonoid baicalin on the proliferative capacity of bovine mammary cells and their ability to regulate oxidative stress. PEERJ. (2019) 7:e6565. doi: 10.7717/peerj.6565
33. Zeng X, Liu X, Bao H. Sulforaphane suppresses lipopolysaccharide- and Pam3CysSerLys4-mediated inflammation in chronic obstructive pulmonary disease via toll-like receptors. FEBS Open Bio. (2021) 11:1313–21. doi: 10.1002/2211-5463.13118
34. Tang J, Xu L, Zeng Y, Gong F. Effect of gut microbiota on LPS-induced acute lung injury by regulating the TLR4/NF-kB signaling pathway. Int Immunopharmacol. (2021) 91:107272. doi: 10.1016/j.intimp.2020.107272
35. Russo RC, Garcia CC, Teixeira MM, Amaral FA. The CXCL8/IL-8 chemokine family and its receptors in inflammatory diseases. Expert Rev Clin Immu. (2014) 10:593–619. doi: 10.1586/1744666X.2014.894886
36. Burkey TE, Skjolaas KA, Dritz SS, Minton JE. Expression of Toll-like receptors, interleukin 8, macrophage migration inhibitory factor, and osteopontin in tissues from pigs challenged with Salmonella enterica serovar Typhimurium or serovar Choleraesuis. Vet Immunol Immunop. (2007) 115:309–19. doi: 10.1016/j.vetimm.2006.11.012
37. Zhang J, Wan J, Chen D, Yu B, He J. Low-molecular-weight chitosan attenuates lipopolysaccharide-induced inflammation in IPEC-J2 cells by inhibiting the nuclear factor-κB signalling pathway. Molecules. (2021) 26:569. doi: 10.3390/molecules26030569
38. Wullaert A. Role of NF-κB activation in intestinal immune homeostasis. Int J Med Microbiol. (2010) 300:49–56. doi: 10.1016/j.ijmm.2009.08.007
39. Liu M, Song S, Li H, Jiang X, Yin P, Wan C, et al. The protective effect of caffeic acid against inflammation injury of primary bovine mammary epithelial cells induced by lipopolysaccharide. J Dairy Sci. (2014) 97:2856–65. doi: 10.3168/jds.2013-7600
40. Xu X, Yin P, Wan C, Chong X, Liu M, Cheng P, et al. Punicalagin inhibits inflammation in LPS-induced RAW264.7 macrophages via the suppression of TLR4-mediated MAPKs and NF-κB activation. Inflammation. (2014) 37:956–65. doi: 10.1007/s10753-014-9816-2
41. Yin J, Wu MM, Xiao H, Ren WK, Duan JL, Yang G, et al. Development of an antioxidant system after early weaning in piglets2. J Anim Sci. (2014) 92:612–9. doi: 10.2527/jas.2013-6986
42. Yin J, Duan J, Cui Z, Ren W, Li T, Yin Y. Hydrogen peroxide-induced oxidative stress activates NF-κB and Nrf2/Keap1 signals and triggers autophagy in piglets. RSC Adv. (2015) 5:15479–86. doi: 10.1039/C4RA13557A
43. Gou Z, Jiang S, Zheng C, Tian Z, Lin X. Equol inhibits LPS-induced oxidative stress and enhances the immune response in chicken HD11 macrophages. Cell Physiol Biochem. (2015) 36:611–21. doi: 10.1159/000430124
44. Yang J, Song X, Feng Y, Liu N, Fu Z, Wu J, et al. Natural ingredients-derived antioxidants attenuate H2O2-induced oxidative stress and have chondroprotective effects on human osteoarthritic chondrocytes via Keap1/Nrf2 pathway. Free Radical Bio Med. (2020) 152:854–64. doi: 10.1016/j.freeradbiomed.2020.01.185
45. Zhao Y, Wang Y, Zhang M, Gao Y, Yan Z. Protective effects of ginsenosides (20R)-Rg3 on H2O2-induced myocardial cell injury by activating keap-1/Nrf2/HO-1 signaling pathway. Chem Biodivers. (2021) 18:e2001007. doi: 10.1002/cbdv.202001007
46. Dudzińska E, Gryzinska M, Ognik K, Gil-Kulik P, Kocki J, Jakovljevic V. Oxidative stress and effect of treatment on the oxidation product decomposition processes in IBD. Oxid Med Cell Longev. (2018) 2018:7918261. doi: 10.1155/2018/7918261
47. Qiao Y, Bai X, Du Y. Chitosan oligosaccharides protect mice from LPS challenge by attenuation of inflammation and oxidative stress. Int Immunopharmacol. (2011) 11:121–7. doi: 10.1016/j.intimp.2010.10.016
48. Ishfaq M, Chen C, Bao J, Zhang W, Wu Z, Wang J, et al. Baicalin ameliorates oxidative stress and apoptosis by restoring mitochondrial dynamics in the spleen of chickens via the opposite modulation of NF-κB and Nrf2/HO-1 signaling pathway during mycoplasma gallisepticum infection. Poultry Sci. (2019) 98:6296–310. doi: 10.3382/ps/pez406
49. Fu K, Chen H, Wang Z, Cao R. Andrographolide attenuates inflammatory response induced by LPS via activating Nrf2 signaling pathway in bovine endometrial epithelial cells. Res Vet Sci. (2021) 134:36–41. doi: 10.1016/j.rvsc.2020.11.022
50. Gu A, Yang L, Wang J, Li J, Shan A. Protective effect of glutamine and alanyl-glutamine against zearalenone-induced intestinal epithelial barrier dysfunction in IPEC-J2 cells. Res Vet Sci. (2021) 137:48–55. doi: 10.1016/j.rvsc.2021.04.027
51. Reziwan K, Sun D, Zhang B, Zhao Z. MicroRNA-1225 activates Keap1-Nrf2-HO-1 signalling to inhibit TNFα-induced osteoclastogenesis by mediating ROS generation. Cell Biochem Funct. (2019) 37:256–65. doi: 10.1002/cbf.3394
Keywords: baicalin, lipopolysaccharide, inflammatory effect, oxidative stress, NF-κB, Nrf2-HO-1
Citation: Bao M, Liang M, Sun X, Mohyuddin SG, Chen S, Wen J, Yong Y, Ma X, Yu Z, Ju X and Liu X (2022) Baicalin Alleviates LPS-Induced Oxidative Stress via NF-κB and Nrf2–HO1 Signaling Pathways in IPEC-J2 Cells. Front. Vet. Sci. 8:808233. doi: 10.3389/fvets.2021.808233
Received: 03 November 2021; Accepted: 28 December 2021;
Published: 25 January 2022.
Edited by:
Cristian Piras, University of Catanzaro, ItalyReviewed by:
Elisabetta Razzuoli, Liguria and Valle d'Aosta (IZSTO), ItalyKun Li, Nanjing Agricultural University, China
Copyright © 2022 Bao, Liang, Sun, Mohyuddin, Chen, Wen, Yong, Ma, Yu, Ju and Liu. This is an open-access article distributed under the terms of the Creative Commons Attribution License (CC BY). The use, distribution or reproduction in other forums is permitted, provided the original author(s) and the copyright owner(s) are credited and that the original publication in this journal is cited, in accordance with accepted academic practice. No use, distribution or reproduction is permitted which does not comply with these terms.
*Correspondence: Xiaoxi Liu, liuxiaoxi_06@163.com