Virtual Reality to Evaluate the Impact of Colorful Interventions and Nature Elements on Spontaneous Walking, Gaze, and Emotion
- UMR 9193–SCALab–Sciences Cognitives et Sciences Affectives, Univ. Lille, Lille, France
Green environments are said to have a positive impact on spontaneous physical activity and well-being. However, high quality psychological measures in natural settings are difficult to collect. In the present study, we offer a detailed report on how virtual reality may provide a controlled environment for immersive user testing. Virtual Reality (VR) was here used to test the impact of colorful floor markings on the spontaneous speed of walking, gaze behaviour, as well as perceived changes in and physiological mesures of affective states. The reactions of 36 adult participants were evaluated in Grey and Green VR environments of an urban university campus. Results in VR revealed similar results than that reported in natural settings: participants walked slower and had higher heart rates in Green than in Grey urban settings, indicating more pleasurable experiences. VR results provided nevertheless more detailed description of user experience with the possibility to quantify changes in gaze strategy as a function of the presence or absence of color designs. Spontaneous walking was slower with colorful designs than without. Gaze behaviour presented longer fixation times with colorful designs than without. Finally, physiological responses indicated that mean heart rates were similar across environments and predicted the physical effort of the task. However, greater means in heart rates were observed in the environments presenting colorful designs, suggesting that colors may be a powerful tool to trigger alertness and pleasure in Grey urban cities. Virtual reality is reported here as an innovative method to quantify psychological experiences during free exploration in gait. Applicable to a broad range of research topics in the psychological sciences, explicit guidelines are made available to share computer code and data sets for further exploitation.
1 Introduction
Living in the city can be unhealthy. The concrete infrastructure of a busy urban scenario plays an important role in the levels of psychological and emotional wellbeing of its citizens (Handy et al., 2002). Urban elements such as visibility (Knöll et al., 2018) and building facades (Elsadek et al., 2019) have been reported to trigger important levels of perceived stress and fatigue. Crowded streets elicit negative emotions and behaviors, which accentuate psychological stress and feelings of aggression (Engelniederhammer et al., 2019), whereas the exposure to road traffic and noise causes physiological stress, expressed as hypertension (Bluhm et al., 2007). The simple exposition to nature may support human wellbeing. Plantations and trees (Lin et al., 2014) as well as patches of grass (Huang et al., 2020) can play a major role in restoring positive moods and affective states in concrete urban environments. Individuals report feeling more relaxed when viewing green buildings ornated with climbing plants than when looking at concrete nuded buildings (Elsadek et al., 2019). These results have convinced the general public that green elements in the built environment offer beneficial effects (Gillis & Gatersleben, 2015; Bolten & Barbiero, 2020). Unfortunately, it is not always possible to introduce nature vegetation into a built environment. Many reasons have been pinpointed, e.g., design constraints, low budgets, climate changes and lack of political decisions. Hence, city designers have been inspired to use colors to include at minima green patches of color on building walls and streets. The question remains however whether color alone is sufficient to offer the restorative effects of vegetation.
The restorative power of nature is grounded in the psycho-evolutionary theory that humans evolved in nature from the beginning of time. Thus, human beings are physiologically better adapted to live in green vegetation than in concrete environments. Green nature would have the power to lower stress levels by providing positive affective states and mild physiological arousal compared to urban human-made habitats (Ulrich et al., 1991). The innate tendency to respond positively to nature would also trigger a spontanenous state of fascination that would lead to a perception of safety (S. Kaplan, 1992). Consequently, green environments should offer a setting in which mental fatigue, attentional effort and cognitive load are minimal (Berto, 2014; Berto et al., 2014). Following these theoretical considerations, the restorative beneficits of nature should have both a physiological affective component (as stated in the stress reduction theory) and a cognitive component (as stated in the attention restorative theory).
1.1 Spontaneous Walking and Affective States to Test the Stress Reduction Theory of Vegetation and Color-Designs
Walking speed in the urban space has been linked to stress. Environmental elements of the urban setting can cause a feeling of anxiety or alertness that trigger an increase in walking pace. Research has shown for example that elements such as light dimming and traffic noise make walkers speed up, suggesting an increase in stress under busy urban settings (Franěk et al., 2018; Pedersen & Johansson, 2018). On the other hand, vegetation lead walkers unconsciously to slow down (Franěk, 2013). In-laboratory studies have defined the spontaneous motor tempo (or SMT) as an automatic regular sequence of movements corresponding to the preferred and natural rhythm at which an individual decides to move (Fraisse, 1982). SMT is naturally observed in daily activities, such as clapping and walking (Rose et al., 2020) and has been associated to the pace for which movement production is the easiest, providing the most pleasure (Delevoye-Turrell et al., 2014). SMT is thought to be associated directly to the physiological affective nature of the walking experience. It is a value that can capture the daily rhythm of people’s lives and reveals how calm or hurried is one’s walking behavior (Bornstein & Bornstein, 1976).
Affect is a psycho-physiological response that describes a broad term of feelings that a person can have, consciously or unconsciously, towards an event or a stimuli (Batson et al., 1992). Affective states have two principal dimensions. Valence characterizes the perceived quality of a stimuli as pleasant or unpleasant (Russel, 1989). Arousal indicates the physiological levels of activation associated to the stimulus at a given moment in time. In cognitive psychology, the valence is mainly quantified through self-declared questionnaires, whereas arousal can be assessed objectively through the measurement of heart rate (Wang et al., 2018). The combination of declarative and objective measurements is recommended today in the affective field of sciences for a better description of changes in users’ affective states (Kondo et al., 2018).
Valence is associated to pleasantness. Urban environments such as traffic (Coensel et al., 2011) and build spaces (Berg et al., 2003; Özügner & Kendle, 2006; Wilkie & Stavridou, 2013) have been shown to impact negatively the pleasurable experience of walking. On the other hand, vegetation (Egorov et al., 2017), integrated urban vegetation (White & Gatersleben, 2011), walking in the forest (Hartig & Staats, 2006), and physical exercise in natural environments (Focht, 2009) lead to positive affective experiences. Similarly, changes in arousal have been linked to stress and many studies have reported the negative impact of concrete urban environments on emotional wellbeing. Elements such as crowd density (Fleming et al., 1987; Engel et al., 1997), urban noise (Münzel et al., 2018), and high building facades (Kalantari & Shepley, 2020) increase arousal levels. On the other hand, vegetation (Egorov et al., 2017) and green nature scenes (Ward Thompson et al., 2012) lead walkers to experience lower levels of arousal. The question is then can colors offer similar changes in affective states? A few studies in color psychology have reported that blue and green colors are linked to more positive affective states than other colors (Kaya, 2004; Savavibool et al., 2018). Ecological testing has also suggested that red triggers a significant increase whereas green decreases perceived and physiological arousal (Kutchma, 2014), which may indicate the power of colors to modulate stress levels. However, such studies are limited in number and suffer a lack of internal validity (cause-effect relationship in one condition is not forceably observed in others).
We will here test in a controlled virtual reality environment whether color-designs can offer positive affective states and decrease the speed of walking in urban settings at a similar degree than that provoked by nature green elements.
1.2 Gaze Behavior to Test the Attention Restorative Effects of Vegetation and Color-Designs
Following the attention theory by Kaplan, the restorative effects of nature should be associated to a strong cognitive component. The analysis of eye blinks and movements reveal what is being looked at across time: the sky, the ground or a specific Area Of Interest (AOI) (Chevalier et al., 2010). During spontaneous walking for example, individuals show distinct gaze strategies appropriate for the demands of different terrains, and this information can be used to characterize the cognitive load and mental fatigue associated to navigation (Matthis et al., 2018). Gaze patterns reveal both active exploration and passive detection, which are key processes when trying to understand human-environment interaction (Colombo, 2001). Hence, by quantifying eye blink frequency, gaze direction and fixation duration, it is possible to model the cognitive component of spontaneous motor behavior both in laboratory and real-world testing.
Blinking is the involuntary reflex of opening and closing the eyes (Volkmann et al., 1982). Blink rate varies systematically in cognitive tasks such as reading, talking and video watching (Fukuda, 1994; Himebaugh et al., 2009). This is typically attributed to variations in cognitive load (Orchard & Stern, 1991). Despite evidence for some systematic variation with dopamine level, blinking is universally regarded as epiphenomenal, rather than functional. Much more research has been conducted on gaze control. Gaze direction in the urban space has been linked to “journey fixation”. During free walk, participants with normal vision direct their gaze primarily straight ahead targeting a goal (Turano et al., 2001). Furthermore, the complexity of the walking surface (Matthis et al., 2018) as well as the density of circulation (Trefzger et al., 2018) modulate systematically gaze direction and lead it to focus more frequently towards the ground.
The duration of gaze fixations is predicted in the urban space by the levels of interest given to an object. Environmental elements placed in an urban scenery may increase alertness, which also tend to increase fixation duration because of an augmented focus of attention to interesting/meaningful/threatening elements (Leveque et al., 2020). Indeed, crossroads and traffic lights increase duration of fixations (Fotios et al., 2014; Biassoni et al., 2018). On the other hand, grass (Egorov et al., 2017) and nature scenes (Ward Thompson et al., 2012) lead walkers to decrease the number and duration of fixation points. Nevertheless, it is unclear whether these effects are due to the nature per se or to the color patches. Indeed, in the field of cognitive psychology, perceptual studies have reported that people scored more fixations in counts and in duration when presented with their preferred colors, which sometimes included the color green (Lee et al., n.d.).
In the present study, we questioned how colorful designs in the urban environment could influence gaze strategies. Using a virtual reality headset with an integrated eye-tracker, we report the number of blinks per minute to quantify changes in cognitive load. Gaze direction and fixation durations were also measured to test the attention restorative effects of vegetation and color-designs.
1.3 Paradigm Shift in Experimental Design to Model the Effects of Colorful Interventions
Paint is a flexible intervention tool applicable to urban architectures as it is ephemeral and non-invasive. Colorful floor markings have been used as design solutions in re-zoning urban spaces and guiding pedestrians. Colors are indeed an essential visual factor of the environment (Jalil et al., 2012). Empirical observations have reported the impact that colors may have on walking perception and behavior. For example, runners with red T-shirts were perceived as running faster than those wearing blue T-shirts (Mentzel et al., 2019). Pedestrians were reported to walk faster when presented with red stimuli compared to trials for which blue stimuli were used (Meier et al., 2012). These results echo the empirical data that have been reported for decades during color walks: color is a key solution to improve walking experience and pleasure in the city (Evans, 2019). Nevertheless, little quantification has been reported. Even with the advances of wearable technology, testing in natural habitats remains complicated with difficulties associated to data collection, limits in replicability (e.g., weather dependency) and the absence of baseline controlled conditions.
Virtual reality (VR) has made its entry in psychological testing by offering a solution to the traditional trade-off between control and generalization: (1) in lab protocols to provide systematic manipulations of the visual displays vs. (2) the use of naturalistic whole-body tasks to augment transferability in more natural organism-environmental conditions. VR is a paradigm shift in experimental design as it offers the means to present systematic representative designs while quantifying changes in human behavior and experience (Miller et al., 2019). In the present study, we report the use of VR as a method to test the impact of color and vegetation on pedestrian walking in an urban environment, while factoring in both the cognitive load and the emotional experience of the task. Physiological and behaviarol parameters (walking speed; gaze behaviors) were collected during free exploration of an urban university campus; questionnaires were included in a pre-post design procedure. The question was specifically to assess whether color patterns can offer similar positive restorative effects than vegetation in urban spaces. The following hypotheses were formulated:
H1: (a) Urban vegetation will be perceived as more pleasant than urban concrete. (b) Perceived pleasantness will be greater in urban concrete with color-designs than without.
H2: (a) Alertness and heart rate will be reduced in urban vegetation compared to urban concrete. (b) Color-designs will decrease aleterness and thus, lower heart rates will be observed in urban concrete with color-designs than without.
H3: (a) Speed of walking will be reduced in urban vegetation compared to urban concrete. (b) Speed of walking will be lower in urban concrete scenes with color-designs than without.
H4: Blink rates will be lower in urban concrete than in urban vegetation.
H5: (a) Gaze will be directed less frequently towards the ground in urban vegetation than in urban concrete. (b) Frequency of gaze towards the ground will be lower in urban concrete with color-designs than without.
H6: (a) Number of gaze fixations will be lower in urban vegetation than in urban concrete. (b) Number of gaze fixations will be lower in urban concrete with color-designs than without.
2 Methods
2.1 Participants
Young healthy adults were recruited from amongst the corpus of staff and students at the University of Lille. All participants had normal to corrected-to-normal vision, and did not present motor dysfunction, neurological/psychiatric disorders or colorblindness. Each participant provided written informed consent and demographic information (sex, age, and VR expertise) before coming to the laboratory to participate in a unique experimental session lasting 90 min. This protocol is registered by the CNIL for good management of human personal data (GED UnivLilleN1-201816).
A within-subjects design was applied wherein each condition was performed in a semi-randomized order. The sample size required for the present study was calculated using G*Power (3.1.9.2). The walking speed data of Franek et al. (2018) were used as group parameters and the power analysis indicated that a total of 32 participants was required for walking speed (f = 0.417; α = 0.05; 1-β = 0.80). The gaze data of Matthis et al. (2018) were used as group parameters and the power analysis indicated that a total of 18 participants was required for fixation duration (f = 2.565; α = 0.05; 1 − β = 0.80). Accordingly, a sample of 35 individuals (16 males, 19 females; mean age = 23.59 ± SD = 8.79: 18–51 years) was recruited to guard against technical failure.
2.2 Materials
2.2.1 Task and Visual Environments
The task was to wear a virtual reality (VR) helmet and to step-on-the-spot while discovering different versions of an urban university campus. We decided to use a minimalistic representation of the main library campus to favor the focus of attention of the participants on the effects of color and vegetation. All participants were familiar with the true University grounds and buildings, and the identical minimalistic representation was used across the six experimental trials. Each participant was invited to take their time to discover the library environment by following a pathway that appeared in front of them. Depending on the condition, the landscape displayed buildings and grey sidewalks or the same buildings set within a green environment that included plantations such as trees and large patches of grass. The path to follow was a painted line, which was white or colored (red-green-blue). Finally, colorful designs could be displayed upon the path. Hence, each participant walked around the university library six times in seperate trials, characterized by specific ambiances. During the VR task, participants were asked to stand on a textured mat to help maintain body stability during the stepping-on-the-spot task (Figure 1). Hence, at the start of the session, participants were invited to take their shoes off. Participants moved continuously, which is know to cause nausea (Birenboim et al., 2019). Air conditioning was used to maintain the temperature of the room at a constant 19.5°, to prevent overheating and sweating during stepping. Between the 15-min trial runs, 5-min breaks were taken to limit the emergence of nausea. During this break, participants were invited to sit and take the VR helmet off. For all participants, the Simulator Sickness Questionnaire was administered at the end of each trial in order to gain a self-declared description of the degree of simulator sickness experienced. The scale is from 1: no nausea; 4: severe nausea. In the present study, nausae levels was scored at 1.50 (1.2–1.8), confirming the absence of nausea across trials.
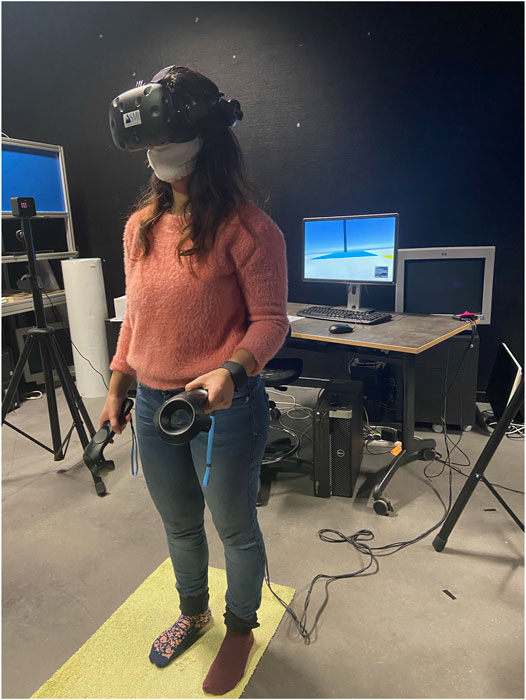
FIGURE 1. Picture of a typical participant performing the walking on the SPOT task. Breaks were taken between trials to limit nausea as the task required continuous movement.
Unity code was used to create the virtual replication of the University campus grounds. In the Concrete urban environment, there were no green elements, only buildings. In the Vegetation urban environment, grass and trees were included using different shades of green. The trees were placed far from the path to avoid the perception of the trees as obstacles. In both types of environments, a total of three design scenarios were tested as floor markings. The path was (1) a white line (control), (2) a line painted in three RGB colours (red, blue or green) and (3) color designs embedded within the RGB line. The position and size of the designs were randomized between participants. Illustrations of the VR environments are provided in Figure 2.
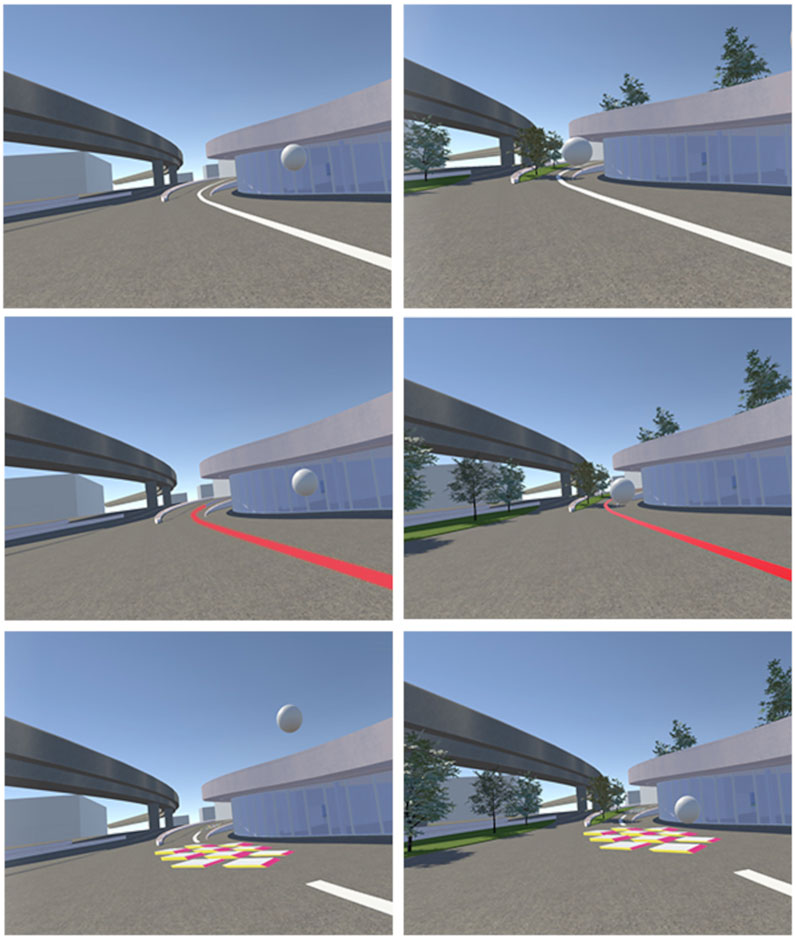
FIGURE 2. Illustration of the six virtual environments that were created using UNITY. The protocol provided the means to evaluate the impact of color visual environments on user experience. Top row = no color floor marking; Middle row = RGB color lines (red, blue, green); Bottom row = RGB colors embedded in playful designs.
2.2.2 Virtual Reality System and Eye Tracking
The virtual reality HTC Vive was used. The light-weight headset displayed visual environments with a resolution of 1,080 × 1,200 pixels per eye for a 110°field of view. The refreshing rate was 90 Hz. The HTC Vive headset was implemented with an eye-tracking system from Senso Motoric Instruments (SMI) that recorded gaze position and movement of both eyes at a rate of 250 Hz. The HTC Vive comes with a ensemble of sensors that capture behavioral changes during user testing (accelerometers, gyroscopes, proximity sensors).
In the present study, two vive controllers were used to offer the participant the possibility to interact and move freely in the VR environment. Held in both hands, the user pressed the trigger buttons and was required to maintain the light pressure on these triggers throughout the exploration. Then, she/he could swing the arms to move forward in the direction in which the controllers were pointed. The speed in the virtual environment depended on the speed of arm swing. Two vive base stations were placed 4 m away from the participant and tracked the vive controllers to create a 360°virtual space. This virtual “room scaled” environment offered a space in which the user could walk fast and slow, stop and interact (e.g., touch a leaf or the building walls). The speed scale was set to high. Thus, the true mean speed of spontaneous arm swing during stepping was in fact 15–20% slower than that presented in the VR environment. The vive base stations detected the 3D position of the helmet. This information was used to measure gaze direction. All tracking was performed with infrared pulses set at 60 Hz.
2.2.3 Objective Measure and Declarative Evaluation of the Affective States
The Empatica E4 wristband (Empatica S.r.l, Milano, Italy) is a portable wristband that provides real-time physiological data streaming and visualization. It records photoplethysmographic data, temperature and electrodermal activity. In the absence of cables, it offers free movement and accurate measurements of cardiac responses (Richardson et al., 2020). Heart Rate (HR) means were calculated from the pulse peak intervals and are reported in beats per minutes (BPM). All signal processing steps were performed using the python’s package HeartPy (van Gent et al., 2018; van Gent et al., 2019). A third order band-pass Butterworth filter was applied with cutoff frequencies of 0.7 and 3.5 Hz. Before filtering, the matrix of pulse intervals was cleaned for outliers by applying the interquartile range method.
Within the current models of emotion, affect is a neuro-physiological state that is always present and can arise to consciousness by voluntary re-orientation of attention (Barrett & Bliss-Moreau, 2009). Hence, the use of a self-evaluating grid can help guide individuals to gain an insight on interior states of emotion (Brossard & Delevoye-Turrell, 2022). The self-declared affective experiences were collected with the Feeling and Affect Table to score Arousal and Appraisal (Figure 3). The FAtAAL is a 2-dimensional digital grid that is adapted from the Russell grid for Affective States (Russel, 1989). Implemented within the VR scene at the end of the trial, the 18-by-18 bingo grid guides a participant to evaluate her/his affective state, considering at the same time both the intensity (arousal) and the pleasantness (valence) of an experience. A simple number verbalized by the participant is sufficient to code the affective state at a given moment in time.
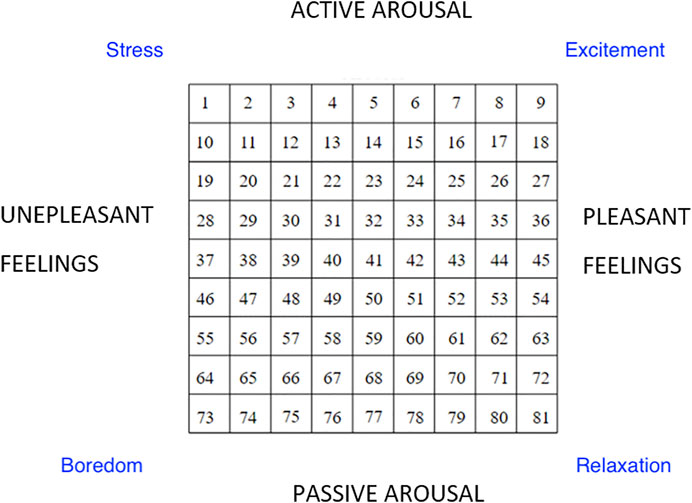
FIGURE 3. Illustration of the numerical grid used to self-guide the evaluation of an individual’s affective state at a given moment in time.
2.3 Design and Measures
Participants were encouraged to engage in a spontaneous and natural walking-on-the-spot movement during free exploration. A military sort of stepping movement was encouraged to have participants swing arms during body movement. Objective measures were collected throughout the unique session to characterize tempo of spontaneous gait, gaze strategy and affective states (Figure 1). Self-declared questionnaires were administered at the end of each trial to limit interfering with user experiences.
2.3.1 Spontaneous Walking Behavior
The time spent exploring each environment was measured in seconds as the duration of a trial. This time interval was calculated as the difference between the first moment participants stepped on the path and the instant they reached the end of the path. The number of stops was counted as the number of times participants released the triggerbutton of one of the two controllers. The mean duration of a trial in seconds and the mean number of stops are reported for each participant and condition.
Walking speed was extracted from the relative 3D coordinates of the headset, every 200 ms. The total distance for a trial was calculated by summing the vectorial distance of each frame. The average speed was then calculated in m/s and converted in km/h, for each participant and condition.
2.3.2 Gaze Behavior
The SMI eye-tracking system recorded gaze position and direction. The 9-point calibration procedure was performed before the start of each session. It provided the means to scale the visual environment as a function of the morphology of each participant.
The absence of eye tracking recordings at a given moment coded for the presence of an eye blink. After the detection of a possible eye blink, a time interval of 100 ms was applied before searching for the next event, as the minimum duration of a blink is 100 ms (Schiffman, 1990). Mean number of eye blinks was calculated for each participant and condition as an indicator of cognitive load.
Gaze direction was grouped in three distinct categories. Participants were considered to be looking straight ahead if their gaze remained within a range of 20°, centered around the horizontal gaze line (calculation based on the dot product–see supplementary data). The number and duration of fixation points higher, lower and around the horitonal gaze line were calculated for each participant and condition.
Gaze fixations were extracted using the MATLAB toolbox, EyeMMV (Krassanakis et al., 2014). Specifically, the XY coordinates were measured at each recording frame and averaged across both eyes. The EyeMMV algorithm relies on a two-step spatial dispersion thresold. Detection is then extracted to determine number of fixations and mean duration of fixation, for each participant and condition.
The VR environment was tagged with imaginary areas of interest (AOI). The AOI were locations in which the colorful designs could be present, i.e., they were present in the color-design conditions but absent in all other conditions. To gain an insight on the role played by color visual environments on gaze behavior, the mean number and duration of AOI fixations were calculated for each participant and condition.
2.4 Affective States
Self-declared affective states were collected using the FAtAAL grid. The levels of arousal and valance were calculated by converting each 9-point Likert-type scale with anchors coding for 1 = unpleasant, and 9 = pleasant feelings on the valence x-axis, and for 1 = active arousal, and 9 = passive arousal on the y-axis. A score above six on the valence scale indicated positive affective states. A score above six on the arousal scale indicated relatively high levels of perceived arousal.
Heart rate is an objective indicator of physiological arousal. Baseline levels were claculated during rest, for a total of 2 min when participants were seated. Then, during each trial, the mean HR was calculated during the two last minutes of each trial. Mean delta HR was calculated for each participant and condition.
2.5 Procedure
2.5.1 Welcome Phase
Upon arrival at the laboratory, the participant was asked to read and sign a consent form. The study was conducted in a large and quiet room, without windows. Care was taken to clear the area from any obstacles to provide a comforting and reassuring user experience. Participants were invited to put the VR helmet on, and to take a few minutes to get familiarized with the VR setup while performing the walking task, using the arms to navigate in the VR environment. For this familiarization phase, participants were immersed in a green neighborhood outdoor basketball court.
2.5.2 Baseline Phase
Before the start of the session, each participant was invited to sit for 4 min to relax. This was important in order to obtain two types of baseline (resting and stressor baselines) that would then be used to compute relative physiological reactions to the green or colorful visual environments.
After sitting calmly for 4 min, participants were invited to put the VR helmet on, and the resting baseline was taken during 3 min. Participants were then invited to engage in a series of mental calculation tasks that consisted in counting backwards from a random four-digit number, in steps of a random two-digit number. The participant was informed of the following: “You will be monitored carefully during the tasks by the research staff and a buzzer will sound every time you give an incorrect answer.” At the end of the mental calculation tasks that lasted 90 s, the stressor baseline was taken for 3 min (Dedovic et al., 2005; Al-Shargie et al., 2016).
2.5.3 Experimental Phase
After the baseline phase, participants were invited to stand and place their feet in the center of the mat. Participants were encouraged to engage in a gait pattern that was spontaneous and easy, to walk at their preferred pace, on-the-spot, while moving the arms naturally and freely. At the end of the exploration time, participants answered the FAtAAL grid, took the helmet off, and sat down to relax for 4 min by concentrating on their breathing. During pre-testing, these pre-set break times were found to be efficient in limiting the emergence of discomfort and nausea.
When ready to start the experimental trials, participants were invited to put the helmet on to record the 2-min HR baseline while remaining seated. Then, with the experimenter’s help, the participant stood and engaged in the trial. At the end of block 6, participants received a detailed debriefing of the general objectives of the study and was thanked for her/his time.
2.6 Data Processing and Statistical Analyses
Data processing and analyses were performed with personal code written in Python, which can be made available upon request (see Supplementary Data 2). The experiment was composed of 1 block of six trials. Classic null-hypothesis significance tests were applied to reveal the effect of Environment and Condition on the user experience. A two-way repeated measure ANOVA was conducted with Environment (Urban Concrete; Urban Nature) and Condition (White-line, Color-line, Color-design) as the two independent factors. The probability level was set to 0.05 for test interpretation. Partial etas squared (ηp2) were calculated to report effect sizes. Pairwise t-tests were computed when ANOVA was significant and were adjusted for multiple comparisons using Holm-Bonferroni corrections.
3 Results
3.1 Emotional Responses
The Empatica E4 wristband and the FAtAAl grid provided the means to collect the objective and perceived changes in affective states. Results test H1 and H2 and are presented in Figures 4–6.
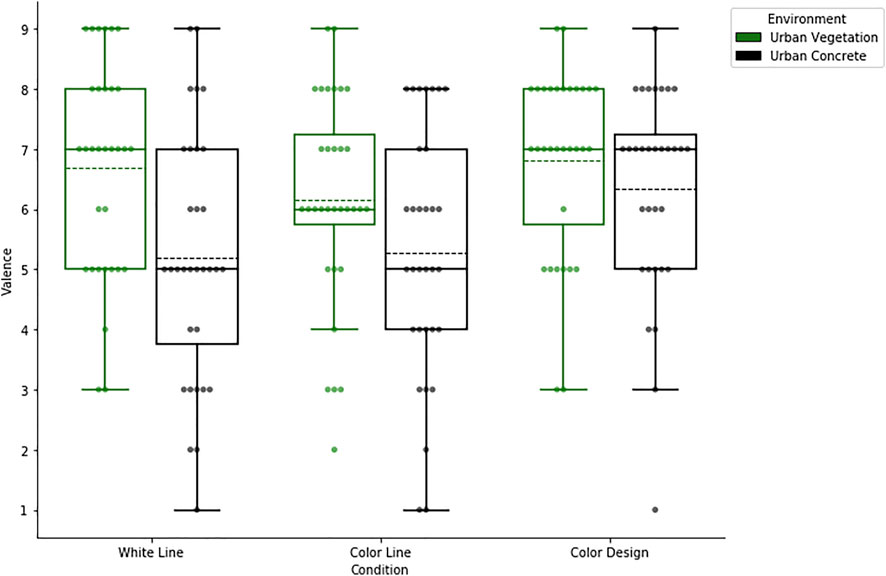
FIGURE 4. Bar charts illustrating the impact of Environment (Concrete vs. Vegetation) and Condition (White Line; Color Line; Color Design) on the valence dimension of the FAtAAL grid, which reports the perceived pleasure experienced during spontaneous walking in the six different urban virtual environments.
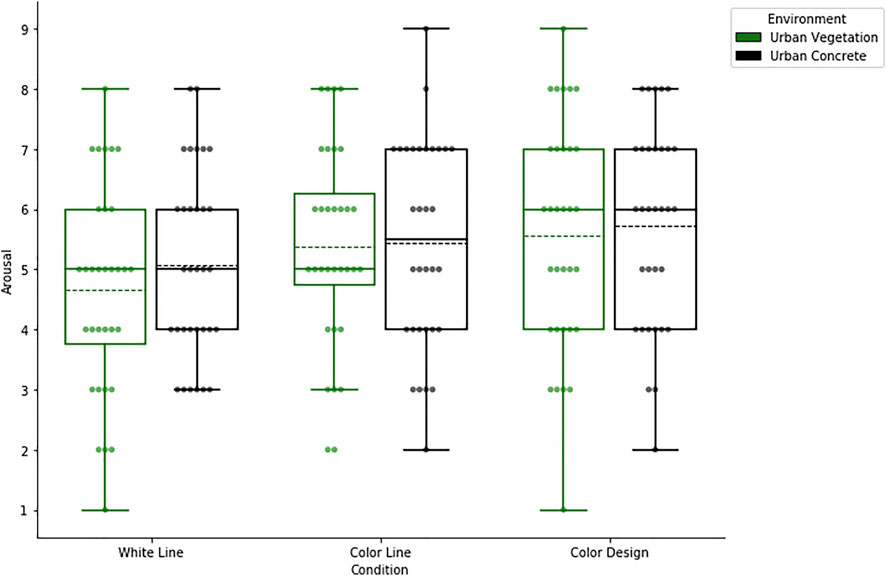
FIGURE 5. Bar charts illustrating the impact of Environment (Concrete vs. Vegetation) and Condition (White Line; Color Line; Color Design) on the arousal dimension of the FAtAAL grid, which reports the perceived alertness experienced during spontaneous walking in the six different urban virtual environments.
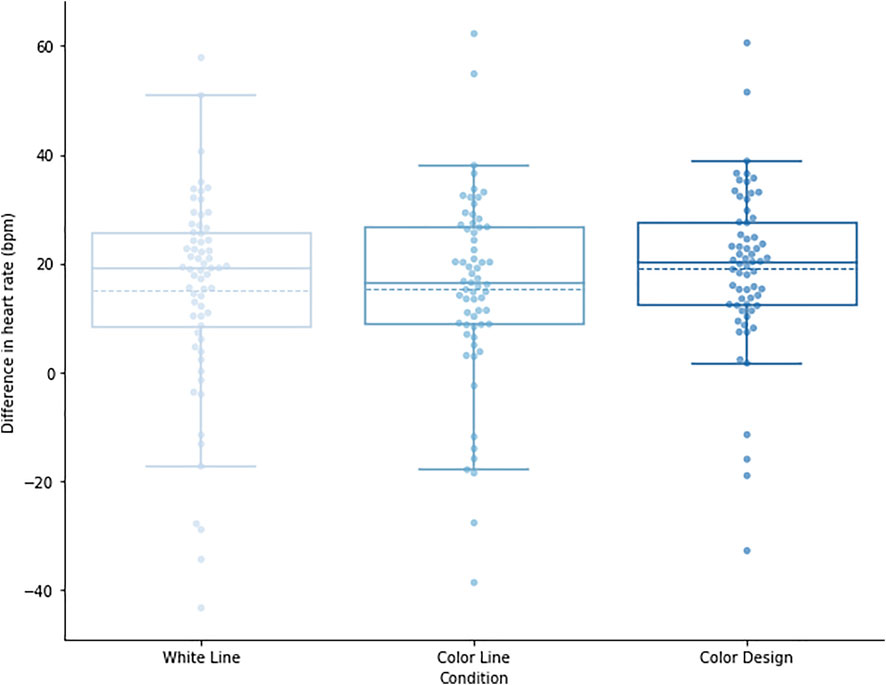
FIGURE 6. Bar charts illustrating the impact of Environment (Concrete vs. Vegetation) on the physiological changes in heart rate during spontaneous walking in the three urban virtual environments.
3.1.1 Perceived Valence and Arousal
The two-way ANOVA revealed a signficant main effect of Environment for valence, F (1,31) = 12.38, p = 0.001, ηp2 = 0.29, with higher scores in Urban Vegetation (M = 6.55, SD = 1.70) than in Urban Concrete (M = 5.60, SD = 2.01). There was also a main effect of Condition, F (2, 62) = 4.16, p = 0.02, ηp2 = 0.12, with higher scores in Color Design (M = 6.58, SD = 1.64) than in White Line (M = 5.94, SD = 2.05) and Color Line conditions (M = 5.72, SD = 1.96). Post hoc tests confirmed the differences between Color Design and Color Line (p = 0.001). The interaction effect was not significant, F (2,62) = 2.16, p = 0.12.
The two-way ANOVA revealed a signficant main effect of Condition for arousal, F (2,62) = 5.03, p = 0.001, ηp2 = 0.14, with lower scores in White Line (M = 4.86, SD = 1.65) than in Color Line (M = 5.41, SD = 1.70) and in Color Design conditions (M = 5.64, SD = 1.79). Post hoc tests confirmed the differences between Color Design and White Line (p = 0.001). Both the main effect of Environment, F (1,31) = 0.86, p = 0.36, and the interaction effect were none significant, F (2,62) = 0.39, p = 0.68.
3.1.2 Changes in Heart Rate
The two-way ANOVA revealed a signficant main effect of Condition on the changes in HR, F (2,62) = 4.22, p = 0.02, ηp2 = 0.12, with higher scores in Color Design (M = 19.09, SD = 14.94) than in White Line (M = 14.99, SD = 18.59) and in Color Line conditions (M = 15.43, SD = 17.37). Post hoc tests confirmed the differences between Color Design and the other conditions (p = 0.04). The main effect of Environment was none significant, F (1,31) = 0.60, p = 0.45, as well as the interaction effect, F (2,62) = 1.01, p = 0.37.
3.2 Walking Behavior
Results in this section test H3 and are presented in Figure 7.
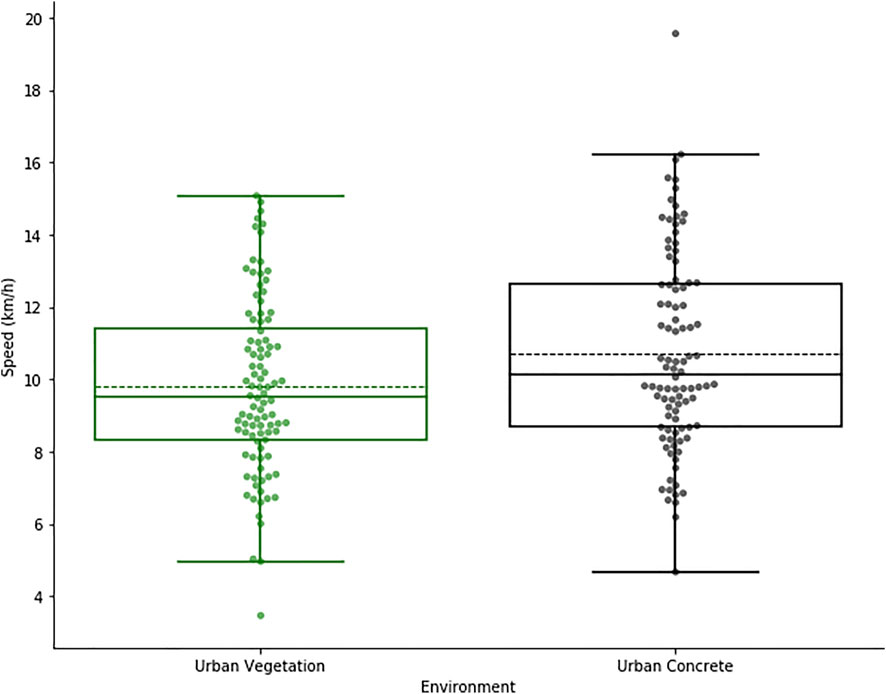
FIGURE 7. Bar charts illustrating the impact of Environment (Concrete vs. Vegetation) on the speed of spontaneous walking in the two urban virtual environments.
3.2.1 Number of Stops
The two-way ANOVA on the number of stops revealed an absence of main effects of Environment (F (1,31) = 0.17, p = 0.68) and Condition (F (2,62) = 1.65, p = 0.20). The interaction effect was not significant (F (2,62) = 0.03, p = 0.97). On average, participants stopped 1.791 times during the course of a trial (min = 0; max = 9).
3.2.2 Walking Speed
The two-way ANOVA revealed a signficant main effect of Environment on the speed of spontaneous walking, F (1,31) = 14.16, p < 0.001, ηp2 = 0.31, with higher scores in Urban Concrete (M = 10.73, SD = 2.76) than in Urban Vegetation (M = 9.78, SD = 2.41). There were no main effects of Condition, F (2,62) = 2.82, p = 0.07, and the interaction effect was not significant, F (2,62) = 0.80, p = 0.45.
3.3 Gaze Behavior
The eye-tracking system that was integrated within the HTC Vive helmet provided the means to characterize gaze strategies through the measurements of blink rates and gaze direction. These results test H4 and H5. Results are presented in Figures 8, 9. To test H6, we quantified the number and duration of gaze fixations overall but also specifically to the AOIs. These results are presented in Figures 10, 11.
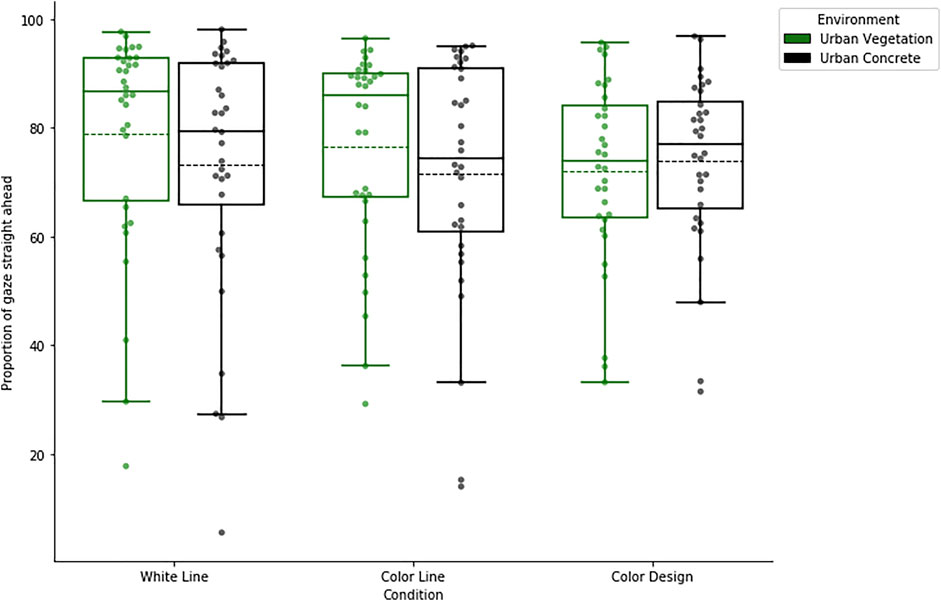
FIGURE 8. Bar charts illustrating the impact of Environment (Concrete vs. Vegetation) on the proportion of time spent looking straight ahead during spontaneous walking in the six urban virtual environments.
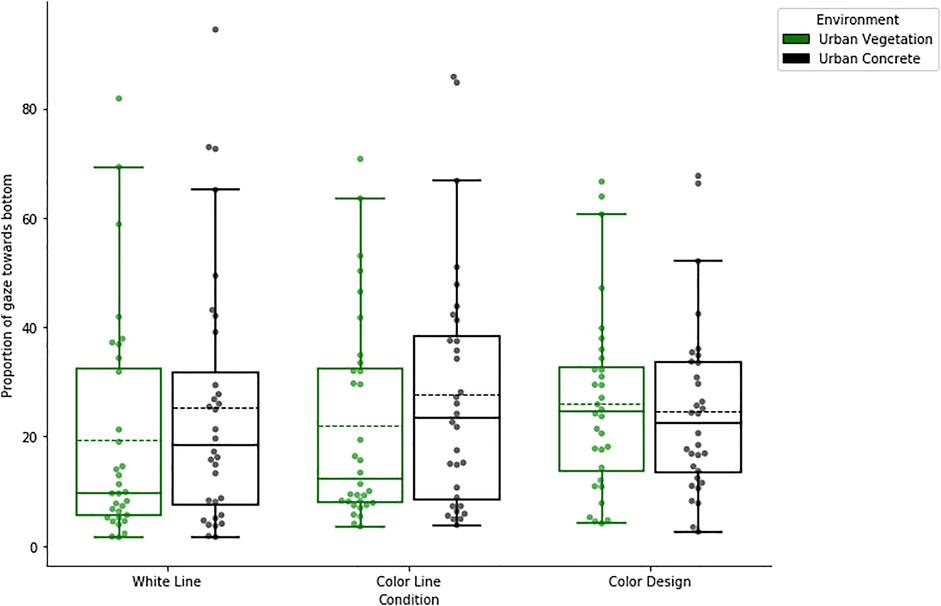
FIGURE 9. Bar charts illustrating the impact of Environment (Concrete vs. Vegetation) on the proportion of time spent looking towards the ground during spontaneous walking in the six urban virtual environments.
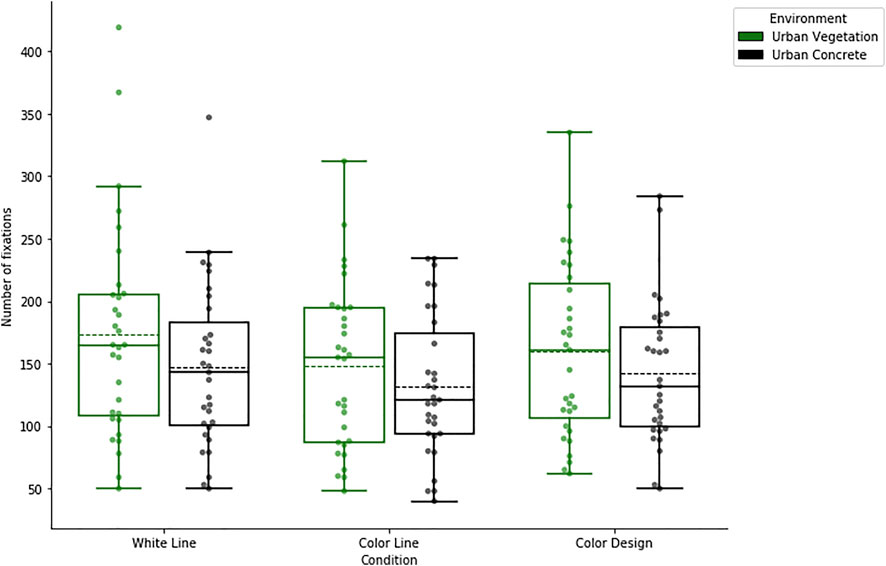
FIGURE 10. Bar charts illustrating the impact of Environment (Concrete vs. Vegetation) on the number of gaze fixations during spontaneous walking in the six urban virtual environments.
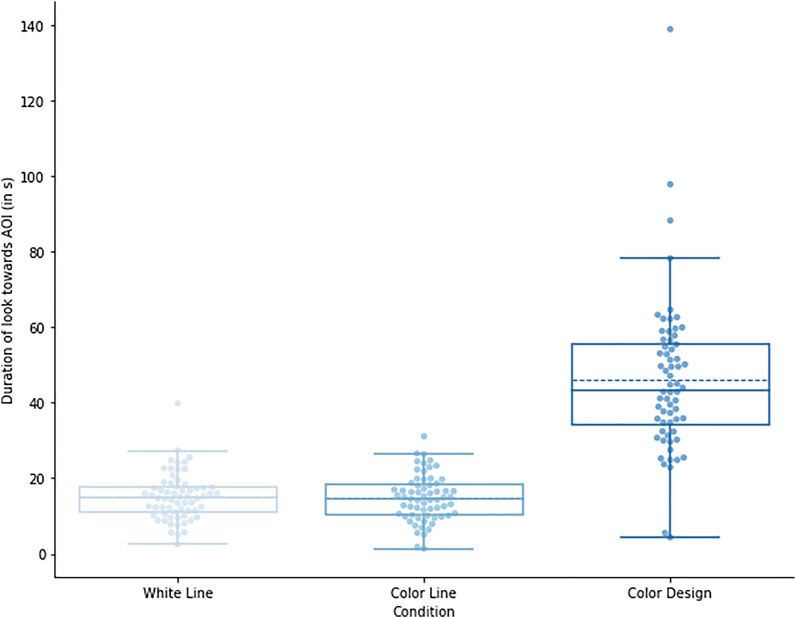
FIGURE 11. Bar charts illustrating the impact of Environment (Concrete vs. Vegetation) on the duration of gaze fixations to the areas of interest (AIO) during spontaneous walking in the six urban virtual environments.
3.3.1 Blink Rates
The two-way ANOVA revealed an absence of main effects of Environment (F (1,31) = 0.10, p = 0.75) and Condition (F (2,62) = 0.47, p = 0.63). The interaction effect was also not significant (F (2,62) = 0.37, p = 0.69) for the number of eye blinks per minute (M = 14.78, SD = 9.81, min = 2.59, max = 41.86).
3.3.2 Gaze Direction
The two-way ANOVA revealed a signficant main effect of Environment on the proportion of time spent with gaze direction toward the horizon, F (1,31) = 5.35, p = 0.03, ηp2 = 0.15. Results indicated higher scores in Urban Vegetation (M = 75.88, SD = 18.57) than in Urban Concrete (M = 72.85, SD = 20.38). The main effect of Condition was not significant, F (2,62) = 1.31, p = 0.28. However, the interaction was significant, F (2,62) = 3.96, p = 0.02, ηp2 = 0.11, indicating that the differences between Urban Concrete and Vegetation environments were only verified in the White Line and Color Line conditions.
The two-way ANOVA revealed a signficant main effect of Environment on the proportion of time spent with gaze direction towards the ground, F (1,31) = 6.11, p = 0.02, ηp2 = 0.16. Results indicated lower scores in Urban Vegetation (M = 22.54, SD = 18.76) than in Urban Concrete (M = 25.93, SD = 20.7). The main effect of Condition was not significant, F (2,62) = 1.38, p = 0.26. However, the interaction was significant, F (2,62) = 4.23, p = 0.02, ηp2 = 0.12, suggesting that the differences between Urban Concrete and Vegetation environments were only verified in the White Line and Color Line conditions.
3.4 Number and Duration of Gaze Fixations
The two-way ANOVA revealed a signficant main effect of Environment on the number of fixations, F (1,30) = 8.87, p = 0.006, ηp2 = 0.23, with higher scores in Urban Vegetation (M = 160.29, SD = 74.27) than in Urban Concrete (M = 140.33, SD = 59.65). The main effect of Condition was also significant, F (2,60) = 3.18, p = 0.049, ηp2 = 0.10, with higher scores in White Line (M = 160.05, SD = 76.89) than in Color Line (M = 139.71, SD = 61.81) and Color Design (M = 151.18, SD = 63.6). The interaction was not significant, F (2,60) = 0.28, p = 0.76.
The two-way ANOVA on duration of fixation indicated an absence of main effects of Environment (F (1,30) = 0.04, p = 0.84) and of Condition (F (2,60) = 2.85, p = 0.07). The interaction effect was not significant (F (2,60) = 0.14, p = 0.87). When considering specifically the gaze fixations to AOIs, the results on gaze fixation duration revealed a signficant main effect of Condition, F (2,62) = 112.72, p < 0.001, ηp2 = 0.78, with higher scores in Color Design (M = 46.12, SD = 20.41) than in White Line (M = 15.09, SD = 6.26) and Color Line conditions (M = 14.77, SD = 6.11). Post hoc tests confirmed the difference between Color Design and the other conditions (p < 0.001). Neither the main effect of Environment, F (1,31) = 0.15, p = 0.71, nor the interaction effect were significant, F (2,62) = 0.28, p = 0.75.
4 Discussion
Virtual reality (VR) is the use of computer technology to create a simulated environment. The benefits of VR testing for cognitive science have been discussed in thorough theoretical papers (Miller et al., 2019). By combining physiological and behavioral measures during spontaneous walking, we present novel data confirming that VR can help tackle the key question of whether color design can mimic the beneficial effects of nature on human spontaneous motor behavior and wellbeing.
4.1 Stepping-On-The-Spot to Mimic Spontaneous Walking
Our first aim was to report data and scripts that describe the psychological experiences of human users in an ecologically-valid behavioral task. We considered the difficulty in choosing the natural behavior of walking. VR studies have used different tasks to simulate walking in VR environments: joy-stick clicking, arm-swing, stepping-on-the spot. These approaches will never offer the exact same experience than true walking in 3D space (Nilsson et al., 2016). Nevertheless, the stepping-on-the-spot task has been reported to offer a good experience of immersion (Nilsson et al., 2013a) with better performance levels in orientation tasks than that observed when using joystick controllers (McCullough et al., 2015) or arm-swing (Wilson et al., 2013). The stepping task is also more realistic, with levels of perceived effort greater than that reported for arm-swing (Nilsson et al., 2013b; Pai & Kunze, 2017) and thus, more similar to that reported during real-world walking. Hence, we selected the stepping-on-the-spot task to assess whether green elements can promote similar changes in a virtual environment than that observed in natural settings.
4.2 Walking in Green VR Environments
Natural elements such as green landscapes have a calming effect on physiological arousal (An et al., 2016). One of the long-term effects of access to nature is a positive attitude towards life and an increased satisfaction with one’s own home, one’s own work and generally one’s own life (R. Kaplan, 2001). Our VR data confirmed that urban vegetation was perceived as more pleasant than urban concrete environments (H1a confirmed). Speed of walking was reduced in urban vegetation compared to urban concrete (H3a confirmed) confirming previous ecological data (Franěk et al., 2018). Overall, these findings suggest that walking in green urban settings offers a certain level of physiological restoration (Valtchanov et al., 2010). However, we did not find that green elements impacted alertness and heart rates (H2a not confirmed). To decrease physiological arousal, time is needed. Hence, the possible calming effect of vegetation may have been masked by the random order design that was applied to short trial durations (<30 s).
Gaze in the urban space is rarely directed straight a head (von Stülpnagel, 2020), especially in the absence of plantations. In more clouted environments as it is the case in urban settings, gaze orientation is directed towards the ground in order to detect possible obstacles (Van Cauwenberg et al., 2016). Even when contrasting different types of urban environments, we revealed an effect of vegetation. Indeed, gaze was directed less frequently towards the ground in urban vegetation than in urban concrete trials (H5a confirmed). This change in strategy was possibly motivated by the intention to widen the angle of view, by opening the torso and raising the head. This change in posture would offer individuals the means to observe more clearly the implemented trees and green surroundings.
Contrary to our predictions fixation points were more numerous and longer in urban vegetation than in urban concrete settings (H6a not confirmed). In nature green, gaze is free and offers walking experiences that are characterized by fewer gaze fixations than in urban environments (Valtchanov & Ellard, 2015). Gaze fixation durations are also longer to offer time for the viewer to focus attention and experience fascination. In our VR experiment, care was taken to offer realistic green elements. The design of the trees was modelled to resemble as best possible the different shades of natural green observed in Northern France in the spring. Using a simple Lickert scale, participants reported being fascinated by the design green elements, which probably contributed in increasing the number of fixations. Hence, journey fixations similar to those observed in natural settings were revealed here in VR offering time to the viewers for fascination and curiosity. Overall, our vegetation VR environments offered both the physiological affective component and the fascination aspect of the restorative benefits of nature, which are key features for clinical applications (Browning et al., 2020; Calogiuri et al., 2021).
4.3 The Impact of Color-Designs in VR Environments
The third objective of our study was to assess the impact of colorful designs and to assess whether color-designs are sufficient to offer the restorative effects of vegetation. Hence, we measured walking speed, which in previous research was reported to be lower in natural environments implemented with green and blue design elements (Meier et al., 2012; Mentzel et al., 2019). In our VR study, participants were invited to walk around the campus grounds following a painted line that could be white, colored or augmented with patches of color-designs. Results did not reveal effects of color-designs on walking speed (H3b not confirmed). Interest and arousal were however triggered with colors: perceived pleasantness was greater with color-designs than without (H1b confirmed). Furthermore, mean heart rates were greater with color-designs in both types of urban environments indicating that color-designs increased physiological arousal (H2b not confirmed). Hence, color-designs impact significantly both the valence and the arousal component of the affective states. Nevertheless, we had predicted the opposite effect of color-designs on arousal. It is the case that our color patches were multi colored, i.e., contained a mix of red, green and blue. Hence, our results confirm those reported in an ecological situation for which red colors were reported to trigger significant increases in perceived and physiological arousal when compared to green colors (Kutchma, 2014). The use of colorful design techniques could be used to encourage spontaneous movement and motivate fun physical activity. To promote calm and wellness, future studies should test the impact of design elements using green shades of color only.
Nature walks free gaze (Martı́nez-Soto et al., 2019). Hence, we predicted that color-designs could do the same. However, individuals looked more frequently towards the ground with design-colors than without, especially in the vegetation urban environments (H5b not verified). They also revealed greater numbers of fixation points. In other words, with floor markings, participants looked down and more frequently at their feet. Color patches attracted attention and may be able to offer a restorative effect by focusing attention outwards, away from the everyday inner worries that bugger the mind. Future studies could confirm such hypothesis by including an estimation of the power of color-designs to help individuals lose track of time (Davydenko & Peetz, 2017). In the city, gaze is captured by the cluttered visual environment and engages attention; such noisy visual surroundings is a plausible explanation of why cities are perceived as mentally tiring. A freeing gaze is a source of mental relaxation. The data reported here confirm the restorative effect of virtual green environments with lower proportions of gaze fixation in the green environments (Nature and Nature Color Lines). On the other hand, the colorful designs limited the freezing of gaze.
Colors are identified faster than other features like shape and size (MacKay & Ahmetzanov, 2005). Therefore, urban planners could use our VR method and use scientifically based visual interventions to encourage citizens to pay attention to ground surfaces, e.g., when a road is under construction or in threat of flooding. The use of color-design principles could be also powerful to guide popup interventions that would control gaze travel through the city and the woods. Used to catch the attention, designs that stick out and automatically capture the curiosity of pedestrians could be created specifically to nudge more active life styles. In all these case studies, VR would be a well suited and innovating tool to guide design choices.
5 Limitations
Although our work reports significant results, several limiting factors remain. The number of blinks per minute was used as a measure of pedestrian cognitive process. Previous research has shown that blink rate is around, and a lower cognitive load produces a higher blink rate (Ledger, 2013). We report blink rates of similar frequency than that reported in natural settings e.g., (Barbato et al., 2007). However, there was an absence of effects of both environment and condition. Such measure may not be sensitive enough in VR and confirms similar levels of cognitive load across conditions.
Secondly, we adopted an experimental design that recorded short trials to avoid fatigue and nausea. Hence, the familiarisation phase was short, which may be the cause of certain order effects reported in the results section. More importantly, parameters such as cognitive performance and electrodermal activity could not be measured. Indirect measures were proposed with, for example, the measure of blink rates and heart rate variability (HRV) to estimate cognitive load and physiological stress, respectively. However, even if a tendency was observed for the later, we report an absence of significant effects; hence, we can not here report data to confirm the beneficial effects of green and colorful elements on well being. Further developments in VR technology will quickly offer the means to implement longer trials, which will be key to further study the influence of color interventions in the urban environment on cognitive stress.
Finally, this study used a limited number of designs and a unique urban setting. Hence, we can not report on the impact of, e.g., shape, size, brightness and achromatic patterns of design elements in the environment. Our aim here was to demonstrate the usefulness of VR technology in design choices. Future studies will be able now to further develop our code to consider more specifically what design principles can impact a pedestrian’s walking experience to nudge the desire to be active.
6 Conclusion
We have reported a pre-post method that demonstrated that trees and green patches of grass can offer beneficial levels of fascination and low stress levels by providing positive affective states. Color alone is not sufficient to offer the restorative effects of vegetation in urban cities, but color interventions in urban spaces can increase fascination, curiosity and physiological levels of arousal. We demonstrate that nature and color interventions do not have similar impacts on the users. Color-designs promote greater levels of arousal that can be used to engage individuals in soft mobility. Our results are important as they suggest the importance of prototyping and of the design-evaluation. Even if painting is a low-cost technique, the financial cost of street art and color design implementation is none negligible. Virtual reality applications may be used to predict behavioral changes and hence, may offer guidelines to orient decisions on what and how to implement changes in the real world.
Data Availability Statement
The datasets presented in this study can be found in online repositories. The names of the repository/repositories and accession number(s) can be found below: https://doi.org/10.5281/zenodo.5658185.
Ethics Statement
Ethical review and approval was not required for the study on human participants in accordance with the local legislation and institutional requirements. The patients/participants provided their written informed consent to participate in this study.
Author Contributions
AB and YD-T were responsible for the conceptualization and management of this study. AB and FV devised the methodology, conducted the investigation. FV contributed to the data and statistical analyses. AB wrote the original draft. FV contributed editing and visualization of data. YD-T contributed resources, review and editing.
Funding
The study received financial support by the I-SITE ULNE Foundation (MAUVE 2020) and from the ANR-21-ESRE-0030- Equipex+ Continuum National Grant from the Programme of Investments for the Future. AB was financed by the MEL and the region Hauts-de-France (France).
Conflict of Interest
The authors declare that the research was conducted in the absence of any commercial or financial relationships that could be construed as a potential conflict of interest.
Publisher’s Note
All claims expressed in this article are solely those of the authors and do not necessarily represent those of their affiliated organizations, or those of the publisher, the editors and the reviewers. Any product that may be evaluated in this article, or claim that may be made by its manufacturer, is not guaranteed or endorsed by the publisher.
Acknowledgments
The authors thank Julien Wylleman, Nicolas Bremard, and Samuel Degrande (CRIStAL/PIRVI) for their help in eye tracking implementation within the VR system. Special thanks to Yoann Sarels and his team (VERTICAL SARL) for the creation of the virtual reality environment of the university campus.
References
Al-Shargie, F., Kiguchi, M., Badruddin, N., Dass, S. C., Hani, A. F. M., and Tang, T. B. (2016). Mental Stress Assessment Using Simultaneous Measurement of Eeg and Fnirs. Biomed. Opt. Express 7 (10), 3882–3898. doi:10.1364/boe.7.003882
An, M., Colarelli, S. M., O'Brien, K., and Boyajian, M. E. (2016). Why We Need More Nature at Work: Effects of Natural Elements and Sunlight on Employee Mental Health and Work Attitudes. PloS one 11 (5), e0155614. doi:10.1371/journal.pone.0155614
Barbato, G., De Padova, V., Paolillo, A. R., Arpaia, L., Russo, E., and Ficca, G. (2007). Increased Spontaneous Eye Blink Rate Following Prolonged Wakefulness. Physiology Behav. 90 (1), 151–154. doi:10.1016/j.physbeh.2006.09.023
Barrett, L. F., and Bliss‐Moreau, E. (2009). Chapter 4 Affect as a Psychological Primitive. Adv. Exp. Soc. Psychol. 41, 167–218. doi:10.1016/s0065-2601(08)00404-8
Batson, C., Shaw, L. L., and Oleson, K. (1992). Differentiating Affect, Mood, and Emotion: Toward Functionally Based Conceptual Distinctions. undefined. Available at: https://www.semanticscholar.org/paper/Differentiating-affect%2C-mood%2C-and-emotion%3A-Toward-Batson-Shaw/9848f7eb1f8995c9f7d46321d1776c7b218741cb (Accessed July 13, 2021).
Berg, A. E. V. D., Belt, H., Koole, S., and Van der wulp, N. (2003). Environmental Preference and Restoration: (How) Are They Related? J. Environ. Psychol. 23 (2), 23. doi:10.1016/s0272-4944(02)00111-1
Berto, R., Massaccesi, S., and Pasini, M. (2014). Do eye Movements Measured across High and Low Fascination Photographs Differ? Addressing Kaplan’s Fascination Hypothesis. J. Environ. Psychol. 28 (2), 185–191.
Berto, R. (2014). The Role of Nature in Coping with Psycho-Physiological Stress: a Literature Review on Restorativeness. Behav. Sci. 4 (4), 394–409. doi:10.3390/bs4040394
Biassoni, F., Bina, M., Confalonieri, F., and Ciceri, R. (2018). Visual Exploration of Pedestrian Crossings by Adults and Children: Comparison of Strategies. Transp. Res. Part F Traffic Psychol. Behav. 56, 227–235. doi:10.1016/j.trf.2018.04.009
Birenboim, A., Dijst, M., Ettema, D., de Kruijf, J., de Leeuw, G., and Dogterom, N. (2019). The Utilization of Immersive Virtual Environments for the Investigation of Environmental Preferences. Landsc. Urban Plan. 189, 129–138. doi:10.1016/j.landurbplan.2019.04.011
Bolten, B., and Barbiero, G. (2020). Biophilic Design: How to Enhance Physical and Psychological Health and Wellbeing in Our Built Environments. Visions Sustain. 13, 11–16.
Bornstein, M. H., and Bornstein, H. G. (1976). The Pace of Life. Nature 259 (5544), 557–559. doi:10.1038/259557a0
Brossard, V., and Delevoye-Turrell, Y. N. (2022). Measuring the Embodied Nature of Emotional Experience: Aligning Methodology and Theory.
Browning, M. H. E. M., Mimnaugh, K. J., Van Riper, C. J., Laurent, H. K., and LaValle, S. M. (2020). Can Simulated Nature Support Mental Health? Comparing Short, Single-Doses of 360-degree Nature Videos in Virtual Reality with the Outdoors. Front. Psychol. 10, 2667. doi:10.3389/fpsyg.2019.02667
Calogiuri, G., Litleskare, S., and Fröhlich, F. (2021). Physical Activity and Virtual Nature: Perspectives on the Health and Behavioral Benefits of Virtual Green Exercise. Nat. Health 2021, 127–146. doi:10.4324/9781003154419-10
Chevalier, N., Blaye, A., Dufau, S., and Lucenet, J. (2010). What Visual Information Do Children and Adults Consider while Switching between Tasks? Eye-Tracking Investigation of Cognitive Flexibility Development. Dev. Psychol. 46, 955–972. doi:10.1037/a0019674
Coensel, B. D., Vanwetswinkel, S., and Botteldooren, D. (2011). Effects of Natural Sounds on the Perception of Road Traffic Noise. J. Acoust. Soc. Am. 129 (4), EL148–EL153. doi:10.1121/1.3567073
Colombo, J. (2001). The Development of Visual Attention in Infancy. Annu. Rev. Psychol. 52 (1), 337–367. doi:10.1146/annurev.psych.52.1.337
Davydenko, M., and Peetz, J. (2017). Time Grows on Trees: the Effect of Nature Settings on Time Perception. J. Environ. Psychol. 54, 20–26. doi:10.1016/j.jenvp.2017.09.003
Dedovic, K., Renwick, R., Mahani, N. K., Engert, V., Lupien, S. J., and Pruessner, J. C. (2005). The Montreal Imaging Stress Task: Using Functional Imaging to Investigate the Effects of Perceiving and Processing Psychosocial Stress in the Human Brain. J. Psychiatry Neurosci. 30 (5), 319–325.
Delevoye-Turrell, Y., Dione, M., and Agneray, G. (2014). Spontaneous Motor Tempo Is the Easiest Pace to Act upon for Both the Emergent and the Predictive Timing Modes. Procedia - Soc. Behav. Sci. 126, 121–122. doi:10.1016/j.sbspro.2014.02.338
Egorov, A. I., Griffin, S. M., Converse, R. R., Styles, J. N., Sams, E. A., Wilson, A., et al. (2017). Vegetated Land Cover Near Residence Is Associated with Reduced Allostatic Load and Improved Biomarkers of Neuroendocrine, Metabolic and Immune Functions. Environ. Res. 158, 508–521. doi:10.1016/j.envres.2017.07.009
Elsadek, M., Liu, B., and Lian, Z. (2019). Green Façades: Their Contribution to Stress Recovery and Well-Being in High-Density Cities. Urban For. Urban Green. 46, 126446. doi:10.1016/j.ufug.2019.126446
Engel, S., Zhang, X., and Wandell, B. (1997). Colour Tuning in Human Visual Cortex Measured with Functional Magnetic Resonance Imaging. Nature 388, 68–71. doi:10.1038/40398
Engelniederhammer, A., Papastefanou, G., and Xiang, L. (2019). Crowding Density in Urban Environment and its Effects on Emotional Responding of Pedestrians: Using Wearable Device Technology with Sensors Capturing Proximity and Psychophysiological Emotion Responses while Walking in the Street. J. Hum. Behav. Soc. Environ. 29 (5), 630–646. doi:10.1080/10911359.2019.1579149
Fleming, I., Baum, A., and Weiss, L. (1987). Social Density and Perceived Control as Mediators of Crowding Stress in High-Density Residential Neighborhoods. J. Personality Soc. Psychol. 52 (5), 899–906. doi:10.1037/0022-3514.52.5.899
Focht, B. C. (2009). Brief Walks in Outdoor and Laboratory Environments. Res. Q. Exerc. sport 80, 611–620. doi:10.1080/02701367.2009.10599600
Fotios, S., Uttley, J., Cheal, C., and Hara, N. (2014). Using Eye-Tracking to Identify Pedestrians' Critical Visual Tasks, Part 1. Dual Task Approach. Light. Res. Technol. 47, 133–148. doi:10.1177/1477153514522472
Fraisse, P. (1982). Rhythm and Tempo. Psychol. music 1, 149–180. doi:10.1016/b978-0-12-213562-0.50010-3
Franěk, M. (2013). Environmental Factors Influencing Pedestrian Walking Speed. Percept. Mot. Ski. 116 (3), 992–1019. doi:10.2466/06.50.PMS.116.3.992-1019
Franěk, M., Režný, L., Šefara, D., and Cabal, J. (2018). Effect of Traffic Noise and Relaxations Sounds on Pedestrian Walking Speed. Int. J. Environ. Res. Public Health 15 (4), 752. doi:10.3390/ijerph15040752
Fukuda, K. (1994). Analysis of Eyeblink Activity during Discriminative Tasks. Percept. Mot. Ski. 79 (3_Suppl. l), 1599–1608. doi:10.2466/pms.1994.79.3f.1599
Gillis, K., and Gatersleben, B. (2015). A Review of Psychological Literature on the Health and Wellbeing Benefits of Biophilic Design. Buildings 5 (3), 948–963. doi:10.3390/buildings5030948
Handy, S. L., Boarnet, M. G., Ewing, R., and Killingsworth, R. E. (2002). How the Built Environment Affects Physical Activity. Am. J. Prev. Med. 23 (2), 64–73. doi:10.1016/s0749-3797(02)00475-0
Hartig, T., and Staats, H. (2006). The Need for Psychological Restoration as a Determinant of Environmental Preferences. J. Environ. Psychol. 26 (3), 215–226. doi:10.1016/j.jenvp.2006.07.007
Himebaugh, N. L., Begley, C. G., Bradley, A., and Wilkinson, J. A. (2009). Blinking and Tear Break-Up during Four Visual Tasks. Optometry Vis. Sci. 86 (2), E106–E114. doi:10.1097/opx.0b013e318194e962
Huang, Q., Yang, M., Jane, H.-a., Li, S., and Bauer, N. (2020). Trees, Grass, or Concrete? the Effects of Different Types of Environments on Stress Reduction. Landsc. Urban Plan. 193, 103654. doi:10.1016/j.landurbplan.2019.103654
Jalil, N. A., Yunus, R. M., and Said, N. S. (2012). Environmental Colour Impact upon Human Behaviour: A Review. Procedia - Soc. Behav. Sci. 35, 54–62. doi:10.1016/j.sbspro.2012.02.062
Kalantari, S., and Shepley, M. (2020). Psychological and Social Impacts of High-Rise Buildings: a Review of the Post-occupancy Evaluation Literature. Hous. Stud. 36, 1–30. doi:10.1080/02673037.2020.1752630
Kaplan, R. (2001). The Nature of the View from Home. Environ. Behav. 33 (4), 507–542. doi:10.1177/00139160121973115
Kaplan, S. (1992). The Restorative Environment: Nature and Human Experience. Portland, Oregon: Timber Press.
Kaya, N. (2004). Relationship between Color and Emotion: a Study of College Students. Coll. student J. 38 (3), 396–405.
Knöll, M., Neuheuser, K., Cleff, T., and Rudolph-Cleff, A. (2018). A Tool to Predict Perceived Urban Stress in Open Public Spaces. Environ. Plan. B Urban Anal. City Sci. 45 (4), 797–813.
Kondo, M., Fluehr, J., McKeon, T., and Branas, C. (2018). Urban Green Space and its Impact on Human Health. Int. J. Environ. Res. Public Health 15 (3), 445. doi:10.3390/ijerph15030445
Krassanakis, V., Filippakopoulou, V., and Nakos, B. (2014). Eyemmv Toolbox: an Eye Movement Post-analysis Tool Based on a Two-step Spatial Dispersion Threshold for Fixation Identification. J. Eye Mov. Res. 7 (1), 1. doi:10.16910/jemr.7.1.1
Kutchma, T. (2014). The Effects of Room Color on Stress Perception: Red versus Green Environments. J. Undergrad. Res. A. T. Minn. State Univ. Mankato 3 (1). Available at: https://cornerstone.lib.mnsu.edu/jur/vol3/iss1/3.
Ledger, H. (2013). The Effect Cognitive Load Has on Eye Blinking. Plymouth, England: University of Plymouth, 19.
Lee, T.-R., Tang, D.-L., and Tsai, C.-M. (n.d.). Exploring Color Preference through Eye Tracking. Jeju, Korea: Congress of the International Colour Association, 4.
Leon Bluhm, G., Berglind, N., Nordling, E., and Rosenlund, M. (2007). Road Traffic Noise and Hypertension. Occup. Environ. Med. 64 (2), 122–126. doi:10.1136/oem.2005.025866
Leveque, L., Ranchet, M., Deniel, J., Bornard, J.-C., and Bellet, T. (2020). Where Do Pedestrians Look when Crossing? A State of the Art of the Eye-Tracking Studies. IEEE Access 8, 164833–164843. doi:10.1109/ACCESS.2020.3021208
Lin, Y.-H., Tsai, C.-C., Sullivan, W. C., Chang, P.-J., and Chang, C.-Y. (2014). Does Awareness Effect the Restorative Function and Perception of Street Trees? Front. Psychol. 5, 906. doi:10.3389/fpsyg.2014.00906
MacKay, D. G., and Ahmetzanov, M. V. (2005). Emotion, Memory, and Attention in the Taboo Stroop Paradigm. Psychol. Sci. 16 (1), 25–32. doi:10.1111/j.0956-7976.2005.00776.x
Martínez-Soto, J., de la Fuente Suárez, L. A., Gonzáles-Santos, L., and Barrios, F. A. (2019). Observation of Environments with Different Restorative Potential Results in Differences in Eye Patron Movements and Pupillary Size. IBRO Rep. 7, 52–58. doi:10.1016/j.ibror.2019.07.1722
Matthis, J. S., Yates, J. L., and Hayhoe, M. M. (2018). Gaze and the Control of Foot Placement when Walking in Natural Terrain. Curr. Biol. 28 (8), 1224–1233. e5. doi:10.1016/j.cub.2018.03.008
McCullough, M., Xu, H., Michelson, J., Jackoski, M., Pease, W., Cobb, W., et al. (2015). “Myo Arm: Swinging to Explore a Ve,” in Proceedings of the ACM SIGGRAPH symposium on applied perception, 107–113.
Meier, B. P., D’Agostino, P. R., Elliot, A. J., Maier, M. A., and Wilkowski, B. M. (2012). Color in Context: Psychological Context Moderates the Influence of Red on Approach- and Avoidance-Motivated Behavior. PLOS ONE 7 (7), e40333. doi:10.1371/journal.pone.0040333
Mentzel, S. V., Schücker, L., Hagemann, N., and Strauss, B. (2019). Perceiving Speed-The Color Can Matter. Color Res. Appl. 44 (6), 957–966. doi:10.1002/col.22429
Miller, L. C., Shaikh, S. J., Jeong, D. C., Wang, L., Gillig, T. K., Godoy, C. G., et al. (2019). Causal Inference in Generalizable Environments: Systematic Representative Design. Psychol. Inq. 30 (4), 173–202. doi:10.1080/1047840x.2019.1693866
Münzel, T., Schmidt, F. P., Steven, S., Herzog, J., Daiber, A., and Sørensen, M. (2018). Environmental Noise and the Cardiovascular System. J. Am. Coll. Cardiol. 71 (6), 688–697. doi:10.1016/j.jacc.2017.12.015
Nilsson, N. C., Serafin, S., Laursen, M. H., Pedersen, K. S., Sikström, E., and Nordahl, R. (2013a). “Tapping-in-place: Increasing the Naturalness of Immersive Walking-In-Place Locomotion through Novel Gestural Input,” in 2013 IEEE symposium on 3d user interfaces (3dui), 31–38. doi:10.1109/3dui.2013.6550193
Nilsson, N. C., Serafin, S., and Nordahl, R. (2013b). “The Perceived Naturalness of Virtual Locomotion Methods Devoid of Explicit Leg Movements,” in Proceedings of motion on games, 155–164. doi:10.1145/2522628.2522655
Nilsson, N. C., Serafin, S., and Nordahl, R. (2016). “Walking in Place through Virtual Worlds,” in International conference on human-computer interaction, 37–48. doi:10.1007/978-3-319-39516-6_4
Orchard, L. N., and Stern, J. A. (1991). Blinks as an Index of Cognitive Activity during Reading. Integr. Physiological Behav. Sci. 26 (2), 108–116. doi:10.1007/bf02691032
Özgüner, H., and Kendle, A. D. (2006). Public Attitudes towards Naturalistic versus Designed Landscapes in the City of Sheffield (UK). Landsc. Urban Plan. 74 (2), 139–157. doi:10.1016/j.landurbplan.2004.10.003
Pai, Y. S., and Kunze, K. (2017). “Armswing: Using Arm Swings for Accessible and Immersive Navigation in Ar/vr Spaces,” in Proceedings of the 16th international conference on mobile and ubiquitous multimedia, 189–198.
Pedersen, E., and Johansson, M. (2018). Dynamic Pedestrian Lighting: Effects on Walking Speed, Legibility and Environmental Perception. Light. Res. Technol. 50 (4), 522–536. doi:10.1177/1477153516684544
Richardson, D. C., Griffin, N. K., Zaki, L., Stephenson, A., Yan, J., Curry, T., et al. (2020). Engagement in Video and Audio Narratives: Contrasting Self-Report and Physiological Measures. Sci. Rep. 10 (1), 11298–8. doi:10.1038/s41598-020-68253-2
Rose, D., Cameron, D. J., Lovatt, P. J., Grahn, J. A., and Annett, L. E. (2020). Comparison of Spontaneous Motor Tempo during Finger Tapping, Toe Tapping and Stepping on the Spot in People with and without Parkinson's Disease. J. Mov. Disord. 13 (1), 47–56. doi:10.14802/jmd.19043
Russell, J. A., Weiss, A., and Mendelsohn, G. A. (1989). Affect Grid: A Single-Item Scale of Pleasure and Arousal. J. Personality Soc. Psychol. 57 (3), 493–502. doi:10.1037/0022-3514.57.3.493
Savavibool, N., Gatersleben, B., and Moorapun, C. (2018). The Effects of Colour in Work Environment: A Systematic Review. Asian J. Behav. Stud. 3 (13), 149. doi:10.21834/ajbes.v3i13.152
Schiffman, H. R. (1990). Sensation and Perception: An Integrated Approach. Hoboken, NJ, USA: John Wiley & Sons.
Trefzger, M., Blascheck, T., Raschke, M., Hausmann, S., and Schlegel, T. (2018). “A Visual Comparison of Gaze Behavior from Pedestrians and Cyclists,” in ETRA '18: Proceedings of the 2018 ACM Symposium on Eye Tracking Research & Applications, 5. doi:10.1145/3204493.3214307
Turano, K. A., Geruschat, D. R., Baker, F. H., Stahl, J. W., and Shapiro, M. D. (2001). Direction of Gaze while Walking a Simple Route: Persons with Normal Vision and Persons with Retinitis Pigmentosa. Optometry Vis. Sci. 78 (9), 667–675. doi:10.1097/00006324-200109000-00012
Ulrich, R. S., Simons, R. F., Losito, B. D., Fiorito, E., Miles, M. A., and Zelson, M. (1991). Stress Recovery during Exposure to Natural and Urban Environments. J. Environ. Psychol. 11 (3), 201–230. doi:10.1016/S0272-4944(05)80184-7
Valtchanov, D., Barton, K. R., and Ellard, C. (2010). Restorative Effects of Virtual Nature Settings. Cyberpsychology, Behav. Soc. Netw. 13 (5), 503–512. doi:10.1089/cyber.2009.0308
Valtchanov, D., and Ellard, C. G. (2015). Cognitive and Affective Responses to Natural Scenes: Effects of Low Level Visual Properties on Preference, Cognitive Load and Eye-Movements. J. Environ. Psychol. 43, 184–195. doi:10.1016/j.jenvp.2015.07.001
Van Cauwenberg, J., De Bourdeaudhuij, I., Clarys, P., Nasar, J., Salmon, J., Goubert, L., et al. (2016). Street Characteristics Preferred for Transportation Walking Among Older Adults: a Choice-Based Conjoint Analysis with Manipulated Photographs. Int. J. Behav. Nutr. Phys. Act. 13 (1), 6–17. doi:10.1186/s12966-016-0331-8
van Gent, P., Farah, H., Nes, N., and van Arem, B. (2018). “Heart Rate Analysis for Human Factors: Development and Validation of an Open Source Toolkit for Noisy Naturalistic Heart Rate Data,” in Proceedings of the 6th HUMANIST conference, 173–178.
van Gent, P., Farah, H., van Nes, N., and van Arem, B. (2019). Heartpy: a Novel Heart Rate Algorithm for the Analysis of Noisy Signals. Transp. Res. Part F Traffic Psychol. Behav. 66, 368–378. doi:10.1016/j.trf.2019.09.015
Volkmann, F. C., Riggs, L. A., Ellicott, A. G., and Moore, R. K. (1982). Measurements of Visual Suppression during Opening, Closing and Blinking of the Eyes. Vis. Res. 22 (8), 991–996. doi:10.1016/0042-6989(82)90035-9
von Stülpnagel, R. (2020). Gaze Behavior during Urban Cycling: Effects of Subjective Risk Perception and Vista Space Properties. Transp. Res. part F traffic Psychol. Behav. 75, 222–238.
Wang, C.-A., Baird, T., Huang, J., Coutinho, J. D., Brien, D. C., and Munoz, D. P. (2018). Arousal Effects on Pupil Size, Heart Rate, and Skin Conductance in an Emotional Face Task. Front. Neurol. 9, 01029. doi:10.3389/fneur.2018.01029
Ward Thompson, C., Roe, J., Aspinall, P., Mitchell, R., Clow, A., and Miller, D. (2012). More Green Space Is Linked to Less Stress in Deprived Communities: Evidence from Salivary Cortisol Patterns. Landsc. Urban Plan. 105 (3), 221–229. doi:10.1016/j.landurbplan.2011.12.015
White, E. V., and Gatersleben, B. (2011). Greenery on Residential Buildings: Does it Affect Preferences and Perceptions of Beauty? J. Environ. Psychol. 31 (1), 89–98. doi:10.1016/j.jenvp.2010.11.002
Wilkie, S., and Stavridou, A. (2013). Influence of Environmental Preference and Environment Type Congruence on Judgments of Restoration Potential. Urban For. Urban Green. 12 (2), 163–170. doi:10.1016/j.ufug.2013.01.004
Keywords: virtual environment, gait, affective responses, restorative theory, vegetation, eye tracking, systematic representative design
Citation: Batistatou A, Vandeville F and Delevoye-Turrell YN (2022) Virtual Reality to Evaluate the Impact of Colorful Interventions and Nature Elements on Spontaneous Walking, Gaze, and Emotion. Front. Virtual Real. 3:819597. doi: 10.3389/frvir.2022.819597
Received: 21 November 2021; Accepted: 02 May 2022;
Published: 17 June 2022.
Edited by:
Ali Oker, Université de Reims Champagne-Ardenne, FranceReviewed by:
Marius Rubo, Université de Fribourg, SwitzerlandLaurence Chaby, Université de Paris, France
Copyright © 2022 Batistatou, Vandeville and Delevoye-Turrell. This is an open-access article distributed under the terms of the Creative Commons Attribution License (CC BY). The use, distribution or reproduction in other forums is permitted, provided the original author(s) and the copyright owner(s) are credited and that the original publication in this journal is cited, in accordance with accepted academic practice. No use, distribution or reproduction is permitted which does not comply with these terms.
*Correspondence: Yvonne N. Delevoye-Turrell, yvonne.delevoye@univ-lille.fr, orcid.org/0000-0003-4034-3684