Influence of site and smoking on malignant transformation in the oral cavity: Is the microbiome the missing link?
- 1Division of Oral and Maxillofacial Surgery, Oral Medicine and Oral Pathology, School of Dental Science, Trinity College Dublin, Dublin Dental University Hospital, Dublin, Ireland
- 2Division of Oral Biosciences, School of Dental Science, Trinity College Dublin, Dublin Dental University Hospital, Dublin, Ireland
The tongue and floor of the mouth are high-risk sites for oral squamous cell carcinoma (OSCC), while smoking is its most significant risk factor. Recently, questions have been raised as to the role of the oral microbiome in OSCC because of a wealth of evidence demonstrating that the microbiome of OSCC differs from that of healthy mucosa. However, oral site and smoking also have a significant impact on oral microbial communities, and to date, the role these factors play in influencing the dysbiotic microbial communities of OSCC and precursor lesions has not been considered. This review aims to examine the influence of site and smoking on the oral microbiome and, in turn, whether these microbiome changes could be involved in oral carcinogenesis.
1. Introduction: micro-organisms and cancer
Micro-organisms are estimated to cause up to 20% of fatal human malignancies, with approximately 2.2 million infection-attributed cancers diagnosed worldwide in 2018 (1). To date, 11 microbes have been designated as human carcinogens by the International Agency for Cancer Research (IARC): Helicobacter pylori, Hepatitis B and C viruses, Opisthorchis viverrini, Clonorchis sinensis, Human papillomavirus, Epstein–Barr virus, Human herpes virus 8, Human T-cell Lymphotropic Virus 1, Schistosoma haematobium, and Human Immunodeficiency Virus-1 (2). Recent advances in microbiome research, however, have demonstrated that many other microbial species are likely to play a role in carcinogenesis, including the oral bacterial pathogen Fusobacterium nucleatum, which is increasingly being linked with colorectal carcinoma (3).
There is general agreement that the microbiome of oral squamous cell carcinoma (OSCC) differs from that of healthy tissue, and there is emerging evidence that pre-cancerous lesions also harbour different microbial communities to healthy mucosa (4–10). There is also a consensus that the mucosal site and tobacco use have a significant impact on the composition of the oral microbiome (11–17). However, what has not been considered to date is the role these factors play in influencing the dysbiotic microbial communities of OSCC and precursor lesions and whether these microbiome changes are involved in malignant progression. This mini review will, therefore, examine the influence of site and smoking on the oral microbiome and, in turn, the evidence for any related dysbiosis in oral carcinogenesis.
2. Risk factors of OSCC
Established risk factors for OSCC are tobacco and alcohol consumption, betel nut use, age and presence of an oral potentially malignant disorder (OPMD), a group of mucosal conditions associated with a statistically increased risk of transformation to cancer. Tobacco is the most significant of these risk factors, with up to 25% of oral cancers directly attributable to smoking, the risk of OSCC increasing with the number of cigarettes smoked, and up to 80% of OSCCs occurring in smokers (18, 19). Tobacco and alcohol are known to act synergistically, increasing the risk of oral cancer up to 15-fold, particularly in the floor of the mouth (18, 20). However, up to 15% of OSCCs in older adults and up to 26% of OSCCs in younger adults have little to no exposure to tobacco or alcohol (21, 22), with Deneuve et al. also finding that their non-smoking tongue cancer cohort had a significantly higher prevalence of oral leukoplakia (OLK) than their smoking cohort (21). OLK is the most common OPMD with a global estimated incidence of 4.1% and malignant transformation rate of 9.8% (23). Risk factors for the development of OLK include the same risk factors as oral cancer, namely tobacco smoking, consumption of alcohol, and use of betel nut. However, OLKs in non-smokers are generally considered to be at a higher risk of malignant transformation than OLKs in smokers (24, 25).
Finally, epidemiological studies and meta-analyses have associated poor oral hygiene, tooth loss, and periodontal disease with OSCC (26–30). However, it is still unclear as to whether these associations are due to chronic periodontal disease sharing risk factors with OSCC, namely smoking and alcohol use, or to the microbial dysbiosis and/or inflammation associated with these conditions.
3. Microbiome of OSCC and OPMD
There is a wealth of literature confirming that the microbiome of OSCC is different from that of healthy mucosa. However, the literature differs as to what microbial changes are responsible for these differences, the conflicting evidence being due at least in part to the various methods by which the oral microbiome is sampled, including mucosal swab, tissue biopsy, and saliva collection. Mucosal surface swabs are non-invasive and enable direct and targeted sampling of specific oral mucosal surfaces. Their principal advantage, however, is that they allow for separate sampling of lesional and matched normal sites within the same individual and therefore control of confounding factors such as smoking, alcohol consumption, and oral hygiene. Direct tissue sampling enables the investigation of invasive species within oral lesions. This method of sampling is, however, invasive, requiring mucosal biopsy, and so carries risks for patients such as post-operative pain, bleeding, and infection. Therefore, in recent studies, ethical considerations have prevented the biopsy sampling of normal tissue as a control. Nonetheless, tissue microbiome studies of oral cancers have shown interesting results, particularly in relation to Fusobacterium nucleatum. Finally, the ease of collection of saliva makes it a popular choice for microbiome studies. However, saliva collection does not allow for sampling of specific sites/lesions and has also been shown to be strongly biased towards tongue and palate communities (12, 13, 31). The saliva sampled can also be contaminated with food and other debris, and although there are various protocols described for pre-sampling rinsing to minimise contamination, they also provide for different sampling methods among studies.
Studies have also used different methods, which have evolved significantly over the years, to characterise the oral microbiome, from initial culture-based methods to DNA-DNA hybridisation and more recently to next-generation sequencing (NGS)-based methods such as marker gene sequencing and metagenomics. Marker gene sequencing usually involves sequencing specific variable (V) regions of the highly conserved bacterial 16S rRNA gene to identify bacteria present in a sample, whereas metagenomic methods sequence the entire genome and therefore can provide not only taxonomy but also the metabolic pathways and functional profiles of the microbial community sampled (32, 33). Metagenomic methods are currently limited by cost and accessibility, but due to the kind of information they can provide, it is likely that they will supersede marker gene sequencing in time. It should be noted that the choice of the variable region (V1–V9) to sequence also needs consideration. Regions V1–V5 are typically used for the oral microbiota, with recent reports suggesting that the V1–V3 region is preferable to the more commonly used V3–V4 region as it is more divergent and therefore provides more phylogenetic resolution and a more accurate assessment of population diversity (34, 35). Several platforms are available for sequencing, of which Illumina remains the most commonly used due to its wide availability, high output, and high level of accuracy (33).
The majority of the microbiome studies on OSCC and OLK to date have sequenced various parts of the V1–V5 region of the 16S rRNA gene using NGS. While the choice of region can impact phylogenetic resolution and diversity as mentioned previously, the choice of the sampling method has an even greater impact on results. Studies using swab and biopsy sampling methods have found an increased abundance of Fusobacterium and reduced abundance of Streptococcus and Rothia at OSCC sites (4, 5, 36–38), while some of those sampling saliva have identified Firmicutes, Streptococci, and Rothia as more abundant in OSCC patients (7, 39, 40). However, no OSCC microbiome studies to date have controlled for significant influences such as smoking, oral site, or oral hygiene, all factors known to influence the oral microbiome and that need to be considered before any definite conclusions can be drawn on the influence of the microbiome on the development of OSCC.
To date, only two authors have looked at the microbiome of OPMDs sampled by swabbing. Schmidt et al. (4) demonstrated that abundances of Firmicutes, Actinobacteria, and Streptococcus spp. were significantly reduced at OPMD sites relative to contralateral normal sites, while Amer et al. (10) found a significant enrichment of OLKs with Fusobacterium, Leptotrichia, Rothia mucilaginosa, and Campylobacter spp. relative to contralateral normal sites. However, Amer et al. also demonstrated that site and smoking status had a more significant influence on the microbiome than the presence of an OLK. Changes in the abundance of Fusobacterium nucleatum subsp. vincentii were particularly site-dependent with increased abundance found on buccal OLKs and on lateral tongue contralateral normal sites. Lateral tongue normal sites also showed an enrichment of Rothia mucilaginosa. These data highlight the necessity for control samples to be matched by site, which is possible only by mucosal swab sampling.
4. Influence of site on the microbiome
The tongue is the most common intra-oral site for cancer, accounting for approximately 46.7% of oral cavity (excluding salivary gland) cancers in the United States in 2016 (41). Despite an overall decrease in tobacco and alcohol–associated OSCC in the US between 1985 and 2009, the proportion of tongue cancers in adults under 50 years of age increased over the same 25-year period (42, 43).
Unsurprisingly, the tongue is also the most common, or second most common, site of OLK and is considered a high-risk site for malignant transformation (23, 44, 45), with the most recent systematic review on the topic finding that 56.3% of OLKs that transformed to cancer were on the tongue (23). A slightly older systematic review identified the buccal mucosa (18.4%) and the tongue (16.4%) as the most common sites for OLK (45), with the authors commenting that the site involved was related to the lifestyle factors of populations studied; the buccal mucosa is more likely to be involved in regions where betel nut is used, whereas the tongue and floor of mouth are more commonly involved in Western populations where smoking and alcohol consumption are more prevalent. As stated previously, malignant transformation was most common in OLKs affecting the tongue (24.2%), followed by the tongue/floor of the mouth combined (14.9%), while the buccal mucosa had the lowest rate of malignant transformation (3.5%).
The oral microbiome also shows site specificity (11–13). Prior to the advent of next-generation sequencing (NGS), Mager et al. (12) used checkerboard DNA-DNA hybridisation to investigate the microbiomes of saliva, dorsum, ventral and lateral tongue, maxillary gingiva, floor of mouth, buccal mucosa, labial mucosa, and hard palate in 225 individuals. Saliva and lateral and dorsal tongue samples clustered together, with the other sites forming a second cluster. There were significantly higher proportions of Gram-negative, and mostly anaerobic, organisms such as Veillonella parvula, Prevotella melaninogenica, Eikenella corrodens, Neisseria mucosa, Actinomyces odontolyticus, Fusobacterium periodonticum, Fusobacterium nucleatum subsp. vincentii, and Porphyromonas gingivalis in the saliva/dorsal/lateral tongue cluster, while the second cluster was dominated by Gram-positive aerobes Streptococcus mitis and Streptococcus oralis and a single Gram-negative anaerobe, Selenomonas noxia. Aas et al.'s (11) findings, pre-NGS using 16S rRNA cloning, were broadly similar, although the dominant colonisers of the tongue differed, likely because of the different methodologies used for bacterial identification.
Finally, Segata et al. (13) sampled 200 subjects at seven oral sites: buccal mucosa, attached gingiva, hard palate, saliva, tongue, and two tooth surfaces along with tonsils, throat, and stool. Clustering similar to that in Mager et al.'s study was identified with buccal mucosa, gingiva, and hard palate clustered together (Group 1), while saliva and tongue grouped with throat and tonsils (Group 2). The Group 1 cluster consisted mostly of Firmicutes, followed by Proteobacteria, Bacteroidetes, Actinobacteria, and Fusobacteria, while the Group 2 cluster had a reduced relative abundance of Firmicutes and increased abundance of Bacteroidetes, Fusobacteria, Actinobacteria, and TM7. At the genera level, Group 1 was dominated by Streptococcus, while Group 2 had a more even distribution of Streptococcus, Veillonella, Prevotella, Neisseria, Fusobacterium, Actinomyces, and Leptotrichia.
It is thus clear that the tongue, a site known to have high rates of malignant transformation, also has high levels of colonisation with Gram-negative organisms of phyla Fusobacteria and Bacteroidetes, whereas the buccal mucosa and palate, which have much lower rates of malignant transformation, are predominantly colonised by Gram-positive Streptococci (Figure 1).
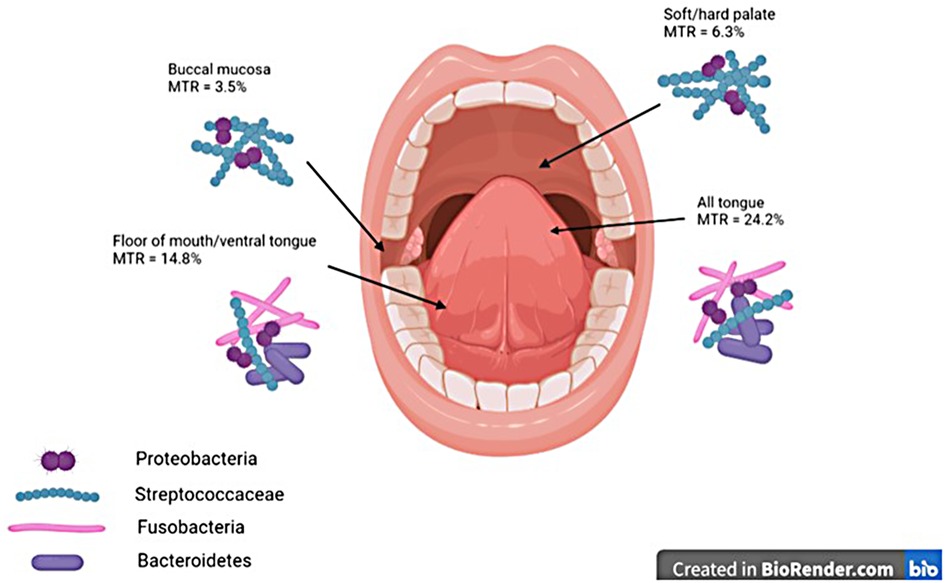
Figure 1. Graphic demonstrating MTRs at different oral mucosal sites and dominant taxonomic groups of bacteria at each site. MTRs, malignant transformation rates.
5. Influence of smoking, betel nut, and electronic cigarettes on the oral microbiome
Along with site, smoking is also known to have a profound effect on the oral microbiome, although as for OSCC microbiome studies, results differ because of variations in sampling methodology and failure to control for factors such as periodontal disease for which smoking is an established risk factor. Depletion of Neisseria spp. in smokers is widely reported and many of these studies also report an increase in Fusobacteria (Table 1) (10, 14–17, 46–48). A switch to a community rich in Fusobacteria may increase the inflammatory stimulation on mucosal surfaces; Fusobacterium nucleatum, in particular, has been shown to induce IL-6-mediated inflammation (49). However, smoking is also a risk factor for periodontal disease, which, in turn, can increase the burden of Gram-negative bacteria in the oral cavity and may explain why some studies identify increases in Fusobacterium nucleatum in smokers. What influence, if any, this has on OSCC risk or malignant transformation of OLK has yet to be elucidated.
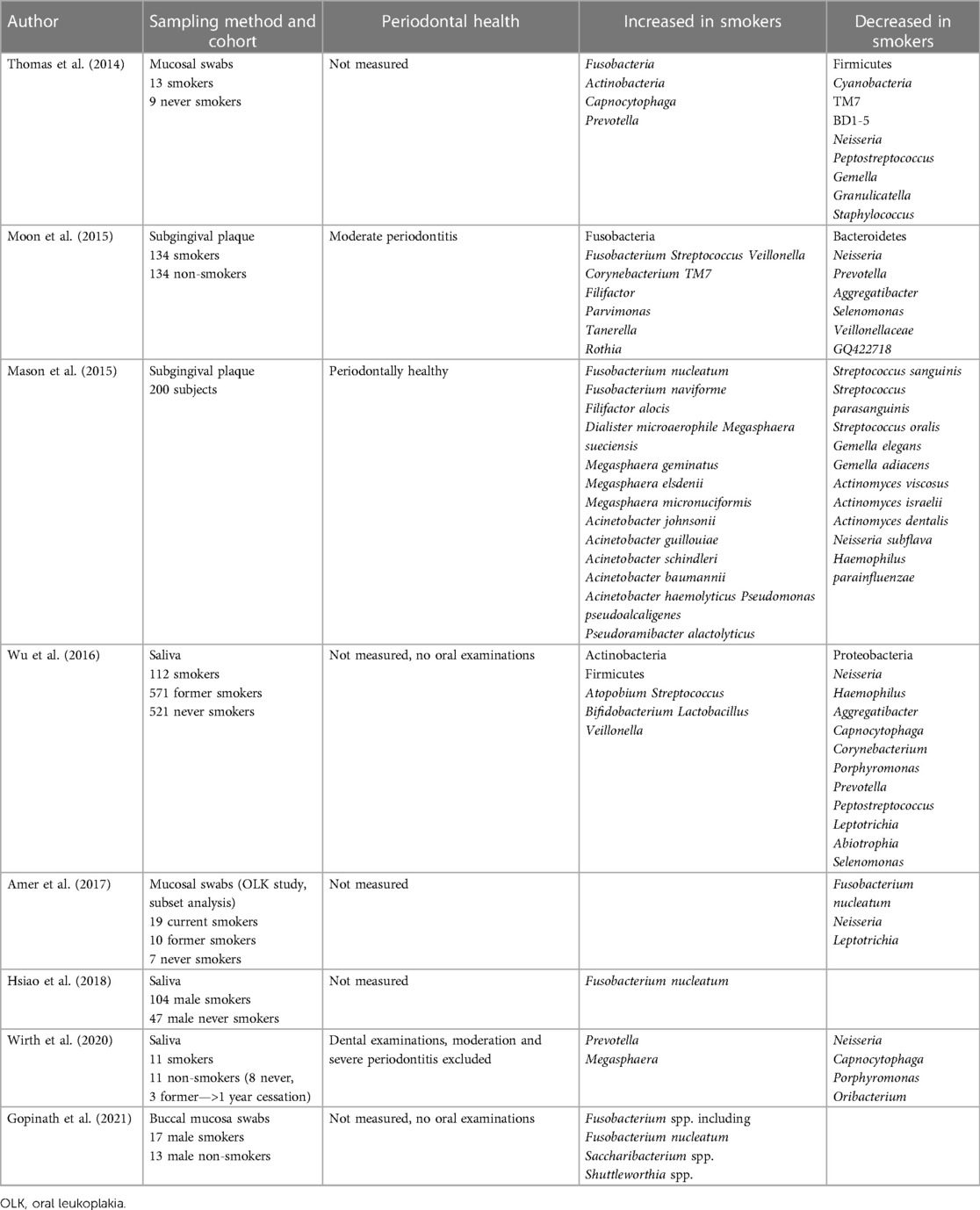
Table 1. Significant differences between the oral microbiomes of smokers and non-smokers in the literature.
To date, few studies have investigated the influence of betel nut or electronic cigarette (e-cigarettes) use on the oral microbiome. Hernandez et al. (50) found significantly increased abundances of Streptococcus infantis and reduced abundances of Parascardovia and other Streptococci in current betel nut chewers, while Hsiao et al. (16) associated betel nut use with an increased abundance of Prevotella intermedia. E-cigarette use has been associated with increased abundances of Veillonella and Porphyromonas (51–54).
6. Microbial methods of carcinogenesis
To date, only one study has shown that bacteria may induce malignant transformation in the oral mucosa, where Porphyromonas gingivalis and Fusobacterium nucleatum were shown to promote carcinogenesis in a chemically induced murine model of tongue OSCC (55). The exact mechanisms of microbial carcinogenesis are still unclear, although research suggests that bacterial-induced epithelial change, promotion of cellular invasion and proliferation, acetaldehyde (ACH) production, and inflammation are likely involved.
The epithelial adhesion molecule E-cadherin, a marker of the epithelial state, is lost early in oral dysplasia (56) and is considered an early event in epithelial-mesenchymal transition (EMT), a biological process whereby an epithelial cell changes to a mesenchymal cell phenotype and is therefore able to migrate, invade, and resist apoptosis. EMT has, therefore, been associated with the initiation of carcinogenesis and facilitation of cancer spread. Bacteria can induce EMT, with Helicobacter pylori being a well-known example, inducing EMT via its cytotoxin-associated gene A (Cag-A) (57). Long-term infection with Porphyromonas gingivalis has also been associated with initial EMT changes in oral epithelial cells, including decreased E-cadherin expression and upregulation of Vimentin (58). Zhang et al. (49) recently showed that the Fusobacterium nucleatum adhesin, FadA, can facilitate EMT via downregulation and transfer of E-cadherin to the cytoplasm and upregulation of N-cadherin, Vimentin, and SNAI1 and miRNA MIR4435-2HG, thus providing a possible mechanism for the role of Fusobacterium nucleatum in carcinogenesis. Fusobacterium nucleatum is also known to promote cell proliferation via upregulation of multiple kinases (59), while both Fusobacterium nucleatum and Porphyromonas gingivalis promote cellular invasion (59, 60).
Microbial production of ACH is also likely to play a role in oral carcinogenesis. ACH, produced by the reduction of ethanol by host or microbial acetaldehyde dehydrogenase (61), is designated as a Class 1 carcinogen by the IARC (62, 63) and is a known risk factor for OSCC. ACH is a genotoxic carcinogen capable of inducing DNA damage and secondary hyperproliferation of epithelium (61, 64–67). Mutagenic levels (>100 µM) of ACH can be detected in saliva following the ingestion of 0.5 g alcohol per kg body weight. Smoking also significantly increases salivary ACH production, with active smoking resulting in an additional 200–400 µM peak ACH concentration in saliva for the duration of the smoking (64, 68–70). Interestingly, the amount of ACH produced is significantly reduced after 3 days of using a chlorhexidine mouthrinse (68), supporting the role of bacteria in ACH production.
All species of Neisseria, along with Rothia mucilaginosa, Streptococcus mitis, and Prevotella histicola, have been shown to produce ACH from ethanol (71–73). As Neisseria are known to be decreased in smokers (72), it is likely that other bacteria are responsible for ACH production in this cohort. Rothia mucilaginosa strains isolated from dysplastic OLKs have been shown to be capable of producing mutagenic levels of ACH in the presence of alcohol (74), while other studies have shown that certain Streptococci, particularly viridans group Streptococci, have significant ACH-producing ability. However, Streptococci are typically associated with a healthy microbiome and reduced in OPMDs and OSCC (16, 73, 75). Therefore, it is clear that more studies are needed to investigate the role of bacteria in ACH production and the various factors that influence its production; however, it is apparent that many bacteria within the normal oral microbiome are potent producers of ACH, raising questions about their role in oral carcinogenesis.
Finally, inflammation, which has been recognised as a fundamental component of malignant processes (76), is another potential link between the oral microbiome and OSCC. Chronic infection causes inflammation, and as demonstrated by the associations between hepatitis and hepatocellular carcinoma (HCC) and Helicobacter pylori–induced gastritis and gastric cancer, this infection-induced inflammation can precede cancer development (76). The role of infection-associated inflammation in OSCC is more complex as the oral cavity harbours hundreds of bacteria and fungi as commensals without being in a constant state of inflammation. However, polymicrobial dysbiosis in the oral cavity does lead to inflammation, as demonstrated by periodontal disease, and therefore may also play a role in carcinogenesis. Bacterial pathogens can stimulate host immune cells, such as macrophages and neutrophils, and oral epithelial cells, via their surface receptors to activate the STAT3 and NF-κB signalling pathways, which, through the promotion of proliferation, angiogenesis, invasion, and enhanced survival, can play a role in tumorigenesis (77, 78). Fusobacterium nucleatum, in particular, has been shown to bind and enter host epithelial and endothelial cells and induce NK-κB- and IL-6-mediated inflammation via its FadA adhesin molecule (79, 80).
Although the definitive pathways of bacterial carcinogenesis have yet to be elucidated, it is likely that, rather than a single bacteria or pathway, there is a polymicrobial dysbiosis and therefore multiple mechanisms are involved. This dysbiosis is likely to be influenced by smoking habit and it is also possible that due to baseline differences in mucosal site colonisation, some mucosal sites are more susceptible to colonisation by a potentially carcinogenic microbiome.
7. Discussion
OLK, the most common OPMD, is most commonly found on the buccal mucosa and tongue. However, OLKs at these sites display very different malignant transformation rates. In health, both sites have distinctly different microbial communities, which raises the question of the role of the microbiome in the malignant transformation of OLKs at these sites. While the microbiome may not initiate dysplastic change, sites with a significantly higher burden of Gram-negative bacteria (e.g., tongue) may be subject to greater pro-inflammatory stimuli, which could play a role in driving abnormal cellular phenotypes. OLKs on the palate and buccal mucosa where Streptococci predominate may not be exposed to the same level of inflammatory stimulation.
Smoking, a major risk factor for oral cancer, also significantly influences the oral microbiome, depleting common oral taxa such as Neisseria spp., which may allow pathobionts to proliferate. Smoking may also contribute to the Gram-negative burden through its role in promoting periodontal disease. It is not yet known whether this effect of smoking on the microbiome contributes to oral carcinogenesis. However, it is clear that smoking is a potential confounding factor in establishing the carcinogenic potential of the oral microbiome. In future, site-specific studies, with anatomically matched control sites, in non-smokers and smokers may provide answers to these questions.
Author contributions
SG drafted and prepared the manuscript. GPM and CMH reviewed and edited the final version. All authors contributed to the article and approved the submitted version.
Funding
GPM and CMH were supported by an award from the Irish Health Research Board (grant no. ILP-POR-2019-030), which is funding several studies on the oral microbiome.
Conflict of interest
The authors declare that the research was conducted in the absence of any commercial or financial relationships that could be construed as a potential conflict of interest.
Publisher's note
All claims expressed in this article are solely those of the authors and do not necessarily represent those of their affiliated organizations, or those of the publisher, the editors and the reviewers. Any product that may be evaluated in this article, or claim that may be made by its manufacturer, is not guaranteed or endorsed by the publisher.
References
1. de Martel C, Georges D, Bray F, Ferlay J, Clifford GM. Global burden of cancer attributable to infections in 2018: a worldwide incidence analysis. Lancet Glob Health. (2019) 8:e180–90. doi: 10.1016/S2214-109X(19)30488-7
2. Carbone A, Blum HE, Cesarman E, Chen CJ, Fang Y, Fontham ETH. Biological agents. Volume 100 B. A review of human carcinogens. IARC Working Group on the Evaluation of Carcinogenic Risks to Humans. IARC Monogr Eval Carcinog Risks Hum. (2012) 100:1–441.
3. Castellarin M, Warren RL, Douglas Freeman J, Dreolini L, Krzywinski M, Strauss J, et al. Fusobacterium nucleatum infection is prevalent in human colorectal carcinoma. Genome Res. (2012) 22:299–306. doi: 10.1101/gr.126516.111
4. Schmidt BL, Kuczynski J, Bhattacharya A, Huey B, Corby PM, et al. Changes in abundance of oral microbiota associated with oral cancer. PLoS One. (2014) 9:e98741. doi: 10.1371/journal.pone.0098741
5. Zhao H, Chiu M, Huang Z, Yang X, Ran S, et al. Variations in oral microbiota associated with oral cancer. Sci Rep. (2017) 7:11773. doi: 10.1038/s41598-017-11779-9
6. Takahashi Y, Park J, Hosomi K, Yamada T, Kobayashi A, et al. Analysis of oral microbiota in Japanese oral cancer patients using 16S rRNA sequencing. J Oral Biosci. (2019) 127:512–9. doi: 10.1016/j.job.2019.03.003
7. Guerrero-Preston R, Godoy-Vitorino F, Jedlicka A, Rodriguez-Hilario A, Gonzalez H, et al. 16S rRNA amplicon sequencing identifies microbiota associated with oral cancer, human papilloma virus infection and surgical treatment. Oncotarget. (2016) 7:51320–34. doi: 10.18632/oncotarget.9710
8. Yang C-Y, Yeh Y-M, Yu H-Y, Chin C-Y, Hsu C-W, et al. Oral microbiota community dynamics associated with oral squamous cell carcinoma staging. Front Microbiol. (2018) 9:862. doi: 10.3389/fmicb.2018.00862
9. Pushalkar S, Ji X, Li Y, Estilo C, Yegnanarayana R. Comparison of oral microbiota in tumor and non-tumor tissues of patients with oral squamous cell carcinoma. BMC Microbiol. (2012) 12:144. doi: 10.1186/1471-2180-12-144
10. Amer A, Galvin S, Healy CM, Moran GP. The microbiome of potentially malignant oral leukoplakia exhibits enrichment for Fusobacterium, Leptotrichia, Campylobacter, and Rothia species. Front Microbiol. (2017) 8:2391. doi: 10.3389/fmicb.2017.02391
11. Aas JA, Paster BJ, Stokes LN, Olsen I, Dewhirst FE. Defining the normal bacterial flora of the oral cavity. J Clin Microbiol. (2005) 43:5721–32. doi: 10.1128/JCM.43.11.5721-5732.2005
12. Mager DL, Ximenez-Fyvie LA, Haffajee AD, Socransky SS. Distribution of selected bacterial species on intraoral surfaces. J Clin Periodontol. (2003) 30:644–54. doi: 10.1034/j.1600-051X.2003.00376.x
13. Segata N, Haake SK, Mannon P, Lermon KP, Waldron L, et al. Composition of the adult digestive tract bacterial microbiome based on seven mouth surfaces, tonsils, throat and stool samples. Genome Biol. (2012) 13:R42. doi: 10.1186/gb-2012-13-6-r42
14. Moon J-H, Lee J-H, Lee J-Y. Subgingival microbiome in smokers and non-smokers in Korean chronic periodontitis patients. Mol Oral Microbiol. (2015) 30:227–41. doi: 10.1111/omi.12086
15. Mason MR, Preshaw PM, Nagaraja HN, Dabdoub SM, Rahman A, et al. The subgingival microbiome of clinically healthy current and never smokers. ISME J. (2015) 9:268–72. doi: 10.1038/ismej.2014.114
16. Hsiao J-R, Chang C-C, Lee W-T, Huang C-C, Ou C-Y, et al. The interplay between oral microbiome, lifestyle factors and genetic polymorphisms in the risk of oral squamous cell carcinoma. Carcinogenesis. (2018) 39:778–87. doi: 10.1093/carcin/bgy053
17. Thomas AM, Gleber-Netto FO, Fernandes GR, Amorim M, Barbosa LF, et al. Alcohol and tobacco consumption affects bacterial richness in oral cavity mucosa biofilms. BMC Microbiol. (2014) 14:250. doi: 10.1186/s12866-014-0250-2
18. Hashibe M, Brennan P, Chuang S-C, Boccia S, Castellsague X, Chen C, et al. Interaction between tobacco and alcohol use and the risk of head and neck cancer: pooled analysis in the International Head and Neck Cancer Epidemiology Consortium. Cancer Epidemiol Prev Biomarkers. (2009) 18:541–50. doi: 10.1158/1055-9965.EPI-08-0347
19. Scully C, Bagan J. Oral squamous cell carcinoma: overview of current understanding of aetiopathogenesis and clinical implications. Oral Dis. (2009) 15:388–99. doi: 10.1111/j.1601-0825.2009.01563.x
20. Radoi L, Paget-Bailly S, Cyr D, Papadopoulos A, Guida F. Tobacco smoking, alcohol drinking and risk of oral cavity cancer by subsite. Eur J Cancer Prev. (2013) 22:268–76. doi: 10.1097/CEJ.0b013e3283592cce
21. Deneuve S, Guerlain J, Dupres-Bories A, Majoufre C, Philouze P, Ceruse P, et al. Oral tongue squamous cell carcinomas in young patients according to their smoking status: a GETTEC study. Eur Arch Otorhinolaryngol. (2022) 279:415–24. doi: 10.1007/s00405-021-06793-7
22. Llewellyn CD, Linklater K, Bell J, Johnson NW, Warnakulasuriya KAAS. Squamous cell carcinoma of the oral cavity in patients aged 45 years and under: a descriptive analysis of 116 cases diagnosed in the South East of England from 1990 to 1997. Oral Oncol. (2003) 39:106–14. doi: 10.1016/S1368-8375(02)00026-X
23. Aguirre-Urizar JM, Lafuente-Ibáñez de Mendoza I, Warnakulasuriya S. Malignant transformation of oral leukoplakia: systematic review and meta-analysis of the last 5 years. Oral Dis. (2021) 27:1881–95. doi: 10.1111/odi.13810
24. Ho MW, Risk JM, Woolgar JA, Field EA, Field JK, Steele JC, et al. The clinical determinants of malignant transformation in oral epithelial dysplasia. Oral Oncol. (2012) 48:969–76. doi: 10.1016/j.oraloncology.2012.04.002
25. Rock LD, Rosin MP, Zhang L, Chan B, Shariati B, Laronde DM. Characterization of epithelial oral dysplasia in non-smokers: first steps towards precision medicine. Oral Oncol. (2018) 78:119–25. doi: 10.1016/j.oraloncology.2018.01.028
26. Yao Q-W, Zhou D-S, Peng H-J, Ji P, Liu D-S. Association of periodontal disease with oral cancer: a meta-analysis. Tumor Biol. (2014) 35:7073–7. doi: 10.1007/s13277-014-1951-8
27. Zheng T, Boyle P, Hu H, Duan J, Jiang P, Ma D, et al. Dentition, oral hygiene, and risk of oral cancer: a case-control study in Beijing, People’s Republic of China. Cancer Cause Control. (1990) 1:235–41. doi: 10.1007/BF00117475
28. Ahrens W, Pohlabeln H, Foraita R, Nelis M, Lagiou P, Lagiou A, et al. Oral health, dental care and mouthwash associated with upper aerodigestive tract cancer risk in Europe: the ARCAGE study. Oral Oncol. (2014) 50:616–25. doi: 10.1016/j.oraloncology.2014.03.001
29. Javed F, Warnakulasuriya S. Is there a relationship between periodontal disease and oral cancer? A systematic review of currently available evidence. Crit Rev Oncol Hemat. (2016) 97:197–205. doi: 10.1016/j.critrevonc.2015.08.018
30. Hashim D, Sartori S, Brennan P, Curado MP, Wunsch-Filho V, Divaris K, et al. The role of oral hygiene in head and neck cancer: results from International Head and Neck Cancer Epidemiology (INHANCE) consortium. Ann Oncol. (2016) 27:1619–25. doi: 10.1093/annonc/mdw224
31. Wade WG, Prosdocimi EM. Profiling of oral bacterial communities. J Dent Res. (2020) 99:621–9. doi: 10.1177/0022034520914594
32. Deo PN, Deshmukh R. Oral microbiome: unveiling the fundamentals. J Oral Maxillofac Pathology. (2019) 23:122–8. doi: 10.4103/jomfp.JOMFP_77_18
33. Quince C, Walker AW, Simpson JT, Loman NJ, Segata N. Shotgun metagenomics, from sampling to analysis. Nat Biotechnol. (2017) 35:833–44. doi: 10.1038/nbt.3935
34. Kim M, Morrison M, Yu Z. Evaluation of different partial 16S rRNA gene sequence regions for phylogenetic analysis of microbiomes. J Microbiol Meth. (2011) 84:81–7. doi: 10.1016/j.mimet.2010.10.020
35. Zheng W, Tsompana M, Ruscitto A, Sharma A, Genco R. An accurate and efficient experimental approach for characterization of the complex oral microbiota. Microbiome. (2015) 3(48). doi: 10.1186/s40168-015-0110-9
36. Al-hebshi NN, Nasher AT, Maryoud MY, Homeida HE, Chen T, et al. Inflammatory bacteriome featuring Fusobacterium nucleatum and Pseudomonas aeruginosa identified in association with oral squamous cell carcinoma. Sci Rep. (2017) 7:1834. doi: 10.1038/s41598-017-02079-3
37. Perera M, Al-hebshi NN, Perera I, Ipe D, Ulett GC, et al. Inflammatory bacteriome and oral squamous cell carcinoma. J Dent Res. (2018) 97:725–32. doi: 10.1177/0022034518767118
38. Zhang L, Liu Y, Zheng HJ, Zhang CP. The oral microbiota may have influence on oral cancer. Front Cell Infect Microbiol. (2019) 9:476. doi: 10.3389/fcimb.2019.00476
39. Mager DM, Ximenez-Fyvie LA, Haffajee AD, Socransky SS, et al. The salivary microbiota as a diagnostic indicator of oral cancer: a descriptive, non-randomized study of cancer-free and oral squamous cell carcinoma subjects. J Transl Med. (2005) 3:27. doi: 10.1186/1479-5876-3-27
40. Pushalkar S, Mane SP, Ji X, Li Y, Evans C, et al. Microbial diversity in saliva of oral squamous cell carcinoma. FEMS Immunol Med Microbiol. (2011) 61:269–77. doi: 10.1111/j.1574-695X.2010.00773.x
41. Ellington TD, Henley SJ, Senkomago V, O'Neil ME, Wilson RJ, et al. Trends in incidence of cancers of the oral cavity and pharynx—United States 2007–2016. MMWR Morb Mortal Wkly Rep. (2020) 69:433–8. doi: 10.15585/mmwr.mm6915a1
42. Montero PH, Patel PD, Palmer FL, Patel SG, Shah JP, et al. Changing trends in smoking and alcohol consumption in patients with oral cancer treated at memorial Sloan-Kettering Cancer Center from 1985 to 2009. Arch Otolaryngol Head Neck Surg. (2012) 138:817–22. doi: 10.1001/archoto.2012.1792
43. Tota JE, Anderson WF, Coffey C, Califano J, Cozen W, et al. Rising incidence of oral tongue cancer among white men and women in the United States, 1973–2012. Oral Oncol. (2017) 67:146–52. doi: 10.1016/j.oraloncology.2017.02.019
44. Pinto AC, Carames J, Francisco H, Chen A, Azul AM, Marques D. Malignant transformation rate of oral leukoplakia—systematic review. Oral Surg Oral Med Oral Pathol Oral Radiol. (2020) 129:600–11. doi: 10.1016/j.oooo.2020.02.017
45. Warnakulasuriya S, Ariyawardana A. Malignant transformation of oral leukoplakia: a systematic review of observational studies. J Oral Pathol Med. (2016) 45:155–66. doi: 10.1111/jop.12339
46. Wu J, Peters BA, Dominianni C, Zhang Y, Pei Z. Cigarette smoking and the oral microbiome in a large study of American adults. ISME J. (2016) 10:ismej201637.
47. Wirth R, Maróti G, Mihók R, Simon-Fiala D, Antal M, Pap B, et al. A case study of salivary microbiome in smokers and non-smokers in Hungary: analysis by shotgun metagenome sequencing. J Oral Microbiol. (2020) 12:1773067. doi: 10.1080/20002297.2020.1773067
48. Gopinath D, Wie CC, Banerjee M, Thangavelu L, Pradeep K, Nallaswamy D, et al. Compositional profile of mucosal bacteriome of smokers and smokeless tobacco users. Clin Oral Invest. (2021) 26:1–10. doi: 10.1007/s00784-021-04137-7
49. Zhang S, Li C, Liu J, Geng F, Shi X, Li Q, et al. Fusobacterium nucleatum promotes epithelial-mesenchymal transiton through regulation of the lncRNA MIR4435-2HG/miR-296-5p/Akt2/SNAI1 signaling pathway. FEBS J. (2020) 287:4032–47. doi: 10.1111/febs.15233
50. Hernandez BY, Zhu X, Goodman MT, Gatewood R, Mendiola P. Betel nut chewing, oral premalignant lesions, and the oral microbiome. PLoS ONE. (2017) 12:e0172196. doi: 10.1371/journal.pone.0172196
51. Yang I, Rodriguez J, Wright CY, Hu Y. The oral microbiome of electronic cigarette users: a cross-sectional exploration. Oral Dis. (2022). doi: 10.1111/odi.14186
52. Chopyk J, Bojanowski CM, Shin J, Moshensky A, Fuentes AL, Bonde SS, et al. Compositional differences in the oral microbiome of e-cigarette users. Front Microbiol. (2021) 12:599664. doi: 10.3389/fmicb.2021.599664
53. Ganesan SM, Dabdoub SM, Nagaraja HN, Scott ML, Pamulapati S, Kumar PS, et al. Adverse effects of electronic cigarettes on the disease-naive oral microbiome. Sci Adv. (2020) 6:eaaz0108. doi: 10.1126/sciadv.aaz0108
54. Pushalkar S, Paul B, Li Q, Yang J, Vasconcelos R, Makwana S, et al. Electronic cigarette aerosol modulates the oral microbiome and increases risk of infection. Iscience. (2020) 23:100884. doi: 10.1016/j.isci.2020.100884
55. Gallimidi AB, Fischman S, Revach B, Bulvik R, Maliutina A, Rubinstein AM, et al. Periodontal pathogens Porphyromonas gingivalis and Fusobacterium nucleatum promote tumor progression in an oral-specific chemical carcinogenesis model. Oncotarget. (2015) 6:22613–23. doi: 10.18632/oncotarget.4209
56. Abdalla Z, Walsh T, Thakker N, Ward CM. Loss of epithelial markers is an early event in oral dysplasia and is observed within the safety margin of dysplastic and T1 OSCC biopsies. PLoS ONE. (2017) 12:e0187449. doi: 10.1371/journal.pone.0187449
57. Yu H, Zeng J, Liang X, Wang W, Zhou Y, Sun Y, et al. Helicobacter pylori promotes epithelial–mesenchymal transition in gastric cancer by downregulating programmed cell death protein 4 (PDCD4). PLoS ONE. (2014) 9:e105306. doi: 10.1371/journal.pone.0105306
58. Lee J, Roberts JS, Atanasova KR, Chowdhury N, Han K, Yilmaz Ö. Human primary epithelial cells acquire an epithelial-mesenchymal-transition phenotype during long-term infection by the oral opportunistic pathogen, Porphyromonas gingivalis. Front Cell Infect Microbiol. (2017) 7:493. doi: 10.3389/fcimb.2017.00493
59. Uitto V-J, Baillie D, Wu Q, Gendron R, Grenier D, Putnins EE, et al. Fusobacterium nucleatum increases collagenase 3 production and migration of epithelial cells. Infect Immun. (2005) 73:1171–9. doi: 10.1128/IAI.73.2.1171-1179.2005
60. Inaba H, Amano A, Lamont RJ, Murakami Y. Involvement of protease-activated receptor 4 in over-expression of matrix metalloproteinase 9 induced by Porphyromonas gingivalis. Med Microbiol Immun. (2015) 204:605–12. doi: 10.1007/s00430-015-0389-y
61. Nosova T, Jokelainen K, Kaihovaara P, Jousimies-Somer H, Siitonen A, Heine R, et al. Aldehyde dehydrogenase activity and acetate production by aerobic bacteria representing the normal flora of human large intestine. Alcohol Alcohol. (1996) 31:555–64. doi: 10.1093/oxfordjournals.alcalc.a008191
62. Baan R, Straif K, Grosse Y, Secretan B, Ghissassi FE, Bouvard V, et al. Carcinogenicity of alcoholic beverages. Lancet Oncol. (2007) 8:292–3. doi: 10.1016/S1470-2045(07)70099-2
63. Acetaldehyde . IARC monographs on the evaluations of carcinogenic risks to humans. IARC Monogr Eval Carcinog Risks Hum. (1999) 71:319–35.10476449
64. Homann N, Tillonen J, Rintamaki H, Salaspuro M, Lindqvist C, Meurman JH. Poor dental status increases acetaldehyde production from ethanol in saliva: a possible link to increased oral cancer risk among heavy drinkers. Oral Oncol. (2001) 37:153–8. doi: 10.1016/S1368-8375(00)00076-2
65. Brooks PJ, Theruvathu JA. DNA adducts from acetaldehyde: implications for alcohol-related carcinogenesis. Alcohol. (2005) 35:187–93. doi: 10.1016/j.alcohol.2005.03.009
66. Seitz HK, Stickel F. Molecular mechanisms of alcohol-mediated carcinogenesis. Nat Rev Cancer. (2007) 7:599–612. doi: 10.1038/nrc2191
67. Meurman JH. Infectious and dietary risk factors of oral cancer. Oral Oncol. (2010) 46:411–3. doi: 10.1016/j.oraloncology.2010.03.003
68. Homann N, Jousimies-Somer H, Jokelainen K, Heine R, Salaspuro M. High acetaldehyde levels in saliva after ethanol consumption: methodological aspects and pathogenetic implications. Carcinogenesis. (1997) 18:1739–43. doi: 10.1093/carcin/18.9.1739
69. Homann N, Tillonen J, Meurman JH, Rintamaki H, Lindqvist C, Rautio M, et al. Increased salivary acetaldehyde levels in heavy drinkers and smokers: a microbiological approach to oral cavity cancer. Carcinogenesis. (2000) 21:663–8. doi: 10.1093/carcin/21.4.663
70. Marttila E, Uittamo J, Rusanen P, Lindqvist C, Salaspuro M, Rautemaa R. Acetaldehyde production and microbial colonization in oral squamous cell carcinoma and oral lichenoid disease. Oral Surg Oral Med Oral Pathol Oral Radiol. (2013) 116:61–8. doi: 10.1016/j.oooo.2013.02.009
71. Alnuaimi A, Ramadzan AN, Wiesenfeld D, O'Brien-Simpson NM, Kolev SD, Reynolds EC, et al. Candida virulence and ethanol-derived acetaldehyde production in oral cancer and non-cancer subjects. Oral Dis. (2016) 22:805–14. doi: 10.1111/odi.12565
72. Muto M, Hitomi Y, Ohtsu A, Shimada H, Kashiwase Y, Sasaki H, et al. Acetaldehyde production by non-pathogenic Neisseria in human oral microflora: implications for carcinogenesis in upper aerodigestive tract. Int J Cancer. (2000) 88:342–50. doi: 10.1002/1097-0215(20001101)88:3%3C342::AID-IJC4%3E3.0.CO;2-I
73. Moritani K, Takeshita T, Shibata Y, Ninomiya T, Kiyohara Y, Yamashita Y. Acetaldehyde production by major oral microbes. Oral Dis. (2015) 21:748–54. doi: 10.1111/odi.12341
74. Amer A, Whelan A, Al-Hebshi NN, Healy CM, Moran GP. Acetaldehyde production by Rothia mucilaginosa isolates from patients with oral leukoplakia. J Oral Microbiol. (2020) 12:1743066. doi: 10.1080/20002297.2020.1743066
75. Kurkivuori J, Salaspuro V, Kaihovaara P, Kari K, Rautemaa R, Grönroos L, et al. Acetaldehyde production from ethanol by oral streptococci. Oral Oncol. (2007) 43:181–6. doi: 10.1016/j.oraloncology.2006.02.005
76. Francescone R, Hou V, Grivennikov SI. Microbiome, inflammation, and cancer. Cancer J. (2014) 20:181–9. doi: 10.1097/PPO.0000000000000048
77. O’Grady I, Anderson A, O’Sullivan J. The interplay of the oral microbiome and alcohol consumption in oral squamous cell carcinomas. Oral Oncol. (2020) 110:105011. doi: 10.1016/j.oraloncology.2020.105011
78. Landskron G, De la Fuente M, Thuwajit P, Thuwajit C, Hermoso MA. Chronic inflammation and cytokines in the tumor microenvironment. J Immunol Res. (2014) 2014:149185. doi: 10.1155/2014/149185
79. Han Yw, Shi W, Huang GT-J, Haake SJ, Park N-H, Kuramitsu H, et al. Interactions between periodontal bacteria and human oral epithelial cells: Fusobacterium nucleatum adheres to and invades epithelial cells. Infect Immun. (2000) 68:3140–6. doi: 10.1128/IAI.68.6.3140-3146.2000
Keywords: oral cancer, oral microbiome, OSCC, smoking, oral leukoplakia, oral potentially malignant disorders
Citation: Galvin S, Moran GP and Healy CM (2023) Influence of site and smoking on malignant transformation in the oral cavity: Is the microbiome the missing link?. Front. Oral. Health 4:1166037. doi: 10.3389/froh.2023.1166037
Received: 14 February 2023; Accepted: 1 March 2023;
Published: 23 March 2023.
Edited by:
Arjun Singh, Tata Memorial Hospital, IndiaReviewed by:
Ashu Sharma, University at Buffalo, United States© 2023 Galvin, Moran and Healy. This is an open-access article distributed under the terms of the Creative Commons Attribution License (CC BY). The use, distribution or reproduction in other forums is permitted, provided the original author(s) and the copyright owner(s) are credited and that the original publication in this journal is cited, in accordance with accepted academic practice. No use, distribution or reproduction is permitted which does not comply with these terms.
*Correspondence: Sheila Galvin sheila.galvin@dental.tcd.ie
Specialty Section: This article was submitted to Oral Cancers, a section of the journal Frontiers in Oral Health