- 1Department of Botany, University of Malakand, Khyber Pakhtunkhwa, Pakistan
- 2Department of Botany and Microbiology, College of Science, King Saud University, Riyadh, Saudi Arabia
- 3Faculty of Science, Zarqa University, Zarqa, Jordan
- 4Department of Medical Labortory Sciences, Faculty of Allied Medical Sciences, Zarqa University, Zarqa, Jordan
- 5Integrated Molecular Plant Physiology Research, Department of Biology, Univeristy of Antwerp, Antwerp, Belgium
Recent anthropogenic sources and excess usage have immensely threatened the communities and habitat ecology of this region’s medicinally and economically significant crops. Therefore, our study aims to evaluate the community structure and related environmental characteristics sustaining Nasturtium officinale communities along the river basin (RB) in Northwest Pakistan, using the clustering procedure (Ward’s method) and Redundancy analysis (RDA). From 340 phytosociological plots (34 × 10 = 340), we identified four ecologically distinct assemblages of N. officinale governed by different environmental and anthropogenic factors for the first time. The floristic structure shows the dominance of herbaceous (100%), native (77%), and annual (58.09%) species indicating relatively stable communities; however, the existence of the invasive plants (14%) is perturbing and may cause instability in the future, resulting in the replacement of herbaceous plant species. Likewise, we noticed apparent variations in the environmental factors, i.e., clay percentage (p = 3.1 × 10−5), silt and sand percentage (p< 0.05), organic matter (p< 0.001), phosphorus and potassium (p< 0.05), and heavy metals, i.e., Pb, Zn, and Cd (p< 0.05), indicating their dynamic role in maintaining the structure and composition of these ecologically distinct communities. RDA has also demonstrated the fundamental role of these factors in species–environment correlations and explained the geospatial variability and plants’ ecological amplitudes in the Swat River wetland ecosystem. We concluded from this study that N. officinale communities are relatively stable due to their rapid colonization; however, most recent high anthropogenic interventions especially overharvesting and sand mining activities, apart from natural enemies, water deficit, mega-droughts, and recent flood intensification due to climate change scenario, are robust future threats to these communities. Our research highlights the dire need for the sustainable uses and conservation of these critical communities for aesthetics, as food for aquatic macrobiota and humans, enhancing water quality, breeding habitat, fodder crop, and its most promising medicinal properties in the region.
1 Introduction
Biodiversity regulates the ecosystem functioning and stability and is essential for human survival and economic wellbeing (Singh, 2002; Htun et al., 2011; Biplab et al., 2017; Baboo et al., 2017). The loss of biodiversity is a major challenge faced by humans in maintaining the stability and functional sustainability of ecosystems (Hua et al., 2022; Bisht et al., 2023). Wetland ecosystems are threatened by anthropogenic activities (Bisht et al., 2022). Among these, poverty, human population, agricultural expansion and intensification, and infrastructure development have been suggested as major threats to biodiversity in the tropics (Davidar et al., 2010; Bargali et al., 2019; Bargali et al., 2022; Bisht et al., 2023). Wetland ecosystem and its vegetation provide a wide range of ecosystem goods and services to the inhabitants (Gosain et al., 2015; Fartyal et al., 2022). Overexploitation of natural resources has created a big gap between the demand and supply of the natural goods (Manral et al., 2020; Pandey et al., 2023).
The biodiversity patterns along environmental gradients are one of the fundamental questions and are the synthetic reflection of all different types of ecological research (Khan S. et al., 2011; Raduła et al., 2020; Yu et al., 2021). The foundation of biodiversity research is based on the patterns of plant species diversity and the ecological variables affecting these patterns (Nogués-Bravo et al., 2008; Johnson et al., 2015; Li et al., 2018). Because of its importance in predicting future community composition and species performance, plant species diversity has received significant attention (Ullah et al., 2022; Khan et al., 2020; Wang et al., 2008; Subedi et al., 2020). The preservation of ecosystem biodiversity is significantly aided by habitat diversity, where topographical factors have multidimensional and multiscale effects on the pattern of species diversity (De Leo et al., 2014). These factors are interconnected and indicators of several abiotic parameters, including geographic, topographic and soil nutrient flow (Twilley and Rivera-Monroy, 2005).
The spatial scale will likely determine how an organism affects its surroundings, including ecosystem services (Ze-Hao and Xin-Shi, 2000; Kremen et al., 2007). In recent research using worldwide field survey data, organism–environment linkages may change with geography (Grace and Anderson, 2016). Abiotic factors at the regional scale determines the species distribution, as the effects of biotic factors (species types) on their environment are typically more localized (van de Koppel et al., 2012). However, direct and indirect interaction of these factors ultimately constitute organism–environment relationship (Grace and Anderson, 2016). The influence of biota may have been overstated in most studies due to the prevalence of statistical techniques (Alsterberg et al., 2013; Grace and Anderson, 2016).
The edaphic and geographical elements are also important for the variety of plant species and their distribution in aquatic emergent (char-land and water channels) plant communities (Schlaepfer et al., 2012). According to Chao et al. (2006), the soil’s physicochemical characteristics and parent materials may impact the plants growing there and the species diversity. Various direct ecological factors at large scales, including soil texture, nutrient level, and soil hydraulic and metal concentration, influence the distribution and structure of plant communities and species biodiversity in emergent plant communities (Stella et al., 2013). However, the altitudinal gradient is the primary factor forming different mountain habitats and is one of the key elements determining the spatial patterns of species diversity in many regions (Bhattarai and Vetaas, 2003; Wang et al., 2003; Zhao et al., 2005; Jiang et al., 2007; Bhattarai et al., 2014; Subedi et al., 2020).
According to Mitsch and Gosselink (1993), the main factor affecting the distribution of plant species at the land–water interface in wetlands is the water regime. In addition, wetland vegetation’s spatial heterogeneity is influenced by several variables, such as soil composition, microclimate, and topography since it controls the water regime (Naiman et al., 2005; Rybicki and Landwehr, 2007; Watt et al., 2007). The ranges of aquatic plants have been estimated using a variety of methods (Mcfarland and Shafer, 2011; Santos et al., 2011; Orellana et al., 2012; Singh et al., 2020). Field observations and large-scale analyses may now be combined with advances in spatial technology like aerial photography and other remote sensing techniques (Vis et al., 2003) and potent geo-statistical tools like plant cover/density estimators (Pepe et al., 2018). However, owing to the biophysical limitations in the environment, it is important to accurately assess aquatic plant species’ spatial distribution and plant community composition and comprehend their interactions.
The recent anthropogenic activities and rapid urbanization have deliberately changed the natural wetland communities in many areas of the world, particularly in developing countries like Pakistan. These activities threatened the communities’ lies at the junction of terrestrial and aquatic ecosystems or on wetlands. For example, recently, Ali et al. (2022) reported different mining operations along the riparian vegetation that adversely affect the community composition and structure. Therefore, this study evaluated Nasturtium officinale (R. Br. In Aiton) communities’ floristic composition and structure along the River Swat using quantitative ecological parameters. The study aims to assess plant communities dominated by N. officinale and associated species, and to provide scientific basis on the following questions: (i) How do these natural bog-plant communities and species diversity at char-land and channels along the Swat River Basin (SRB) respond to ecological factors? (ii) How do different degrees of anthropogenic factors act in the instability perspective of N. officinale at the community-level? By analyzing the ecological data of community composition and structure, we anticipated specifying a valid scientific basis for species assemblage’s distribution and characterization besides its protection and management in the region.
2 Materials and methods
2.1 Study area
The bogs of River Swat were selected for sampling because of rich Nasturtium officinale communities, from where the residents and local sellers often collect vegetables for edible purposes. The study area is located at 35.22271134 North and 72.42581572 East, in the northern region of Khyber Pakhtunkhwa, Pakistan, spreading over 14,737 square kilometres area covered by hilly glaciers having snowfall and rain (Figure 1) (Farooq et al., 2018). River Swat flows through the districts of Swat, Malakand, and Dir Lower (Ahmad et al., 2015), having subtropical and temperate climates (Barinova et al., 2013). The average higher temperature in the summer may reach 41.9°C, while the average low in the winter can reach 0.8°C. The Pakistan Meteorological Department reported an annual average rainfall of 1,003 mm; the highest rainfall was recorded in February and March (162 mm). According to Nafees et al. (2008), relative humidity may drop as low as 40% in April and rise as high as 85% in July.
Ali et al. (2018) report that the average monthly temperature in the study area is between 34.96°C and 1.36°C, and the average annual rainfall is between 384 to 639 mm (Figure 2). These factors maintain the local climate to comprehend the structure and composition of the vegetation that ultimately affects economic, social, and agricultural activities and hydrological characteristics (Deo and Şahin, 2015).
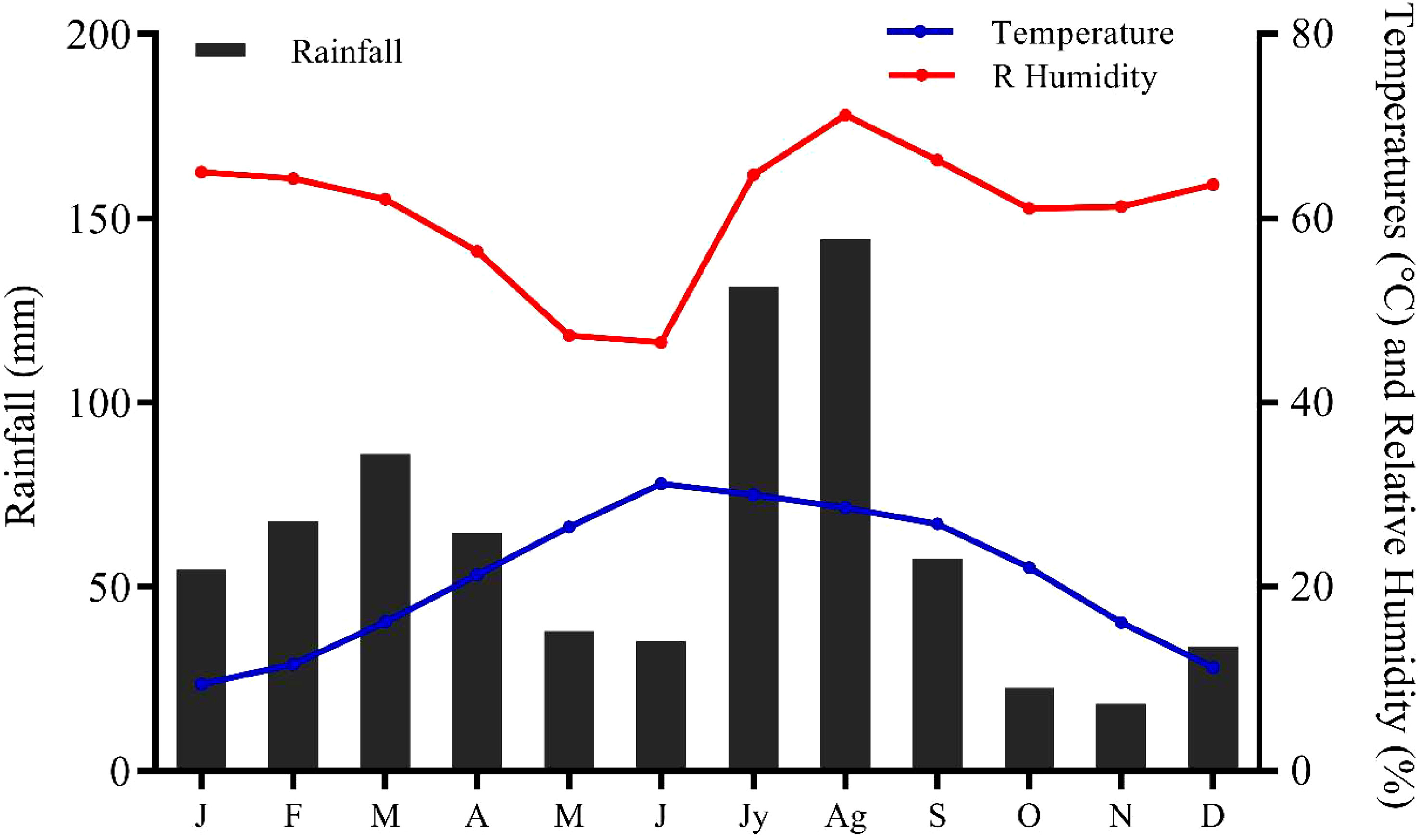
Figure 2 Important climate variables of the sampling sites. J, January; F, February; M, March; A, April; M, May; J, June; Jy, July; Ag, August; S, September; O, October; N, November; D, December.
2.2 Field sampling protocols
In our field research, we randomly selected N. officiale-dominated stands at 34 distinct locations throughout five districts as part of routine trips to gather vegetation data (Figure 1). The entire area was represented by taking 340 phytosociological plots (34 × 10 = 340 plots) and carefully selected according to the structural and floristic homogeneity. The variations in plot size were considered following Morsdorf et al. (2010), where the plot sizes of 3–5 m2 were taken as standard for herb and shrub communities. The standard procedure for computing plant species densities, frequencies, covers, relative densities, relative frequencies, relative covers, and important values in each plot and sites was adopted following Curtis and Mcintosh (1950). To reduce edge effects, a 10-m buffer zone was removed from the stand’s boundaries following Martínez-Falcón et al. (2018). Nomenclature for plant taxa was taken from Flora of Pakistan (Ali, 2008), and binomials were given accordingly.
2.3 Soil data acquisition and analysis
In each stand, a soil sample of 3 kg was collected randomly from each stand’s middle and two opposing corners to characterize soil parameters. Since topsoil is often the most nutrient-rich part of the soil, soil samples were collected at a depth of 0–30 cm bulked and fully mixed (Bargali et al., 2018) to reduce heterogeneity (Morsdorf et al., 2010; Kamrani et al., 2011). The electrical conductivity and pH of a soil–water suspension (1:5) were measured in the field using a digital pH meter and an EC meter, respectively. The soil samples were allowed to air dry at room temperature and then passed through a sieve set at 2 mm. The samples were homogenized and packed in transparent polythene bags for subsequent processing (Chawla et al., 2008).
The soil’s physiochemical composition and textural parameters were determined by air-drying the samples and passing them through a 2-mm sieve per USDA recommendations (Taubner et al., 2009). Organic matter was assessed using the Walkley-Black method, while total and organic carbon was computed utilizing moist burning with chromic acid digestion after dry combustion (Nelson and Sommers, 1996). Total nitrogen was determined using the micro-Kjeldahl method, and soluble phosphorus (P2+) and exchangeable potassium (K+) were determined using the methods described by Yadav et al. (2002). Lime (calcium carbonate; %) was calculated geometrically, and CO2 evolution was tracked geometrically, following Gómez-Díaz et al. (2006). Following the methodology established by Saxton et al. (1986), we used an online calculator (https://www.nrcs.usda.gov) to determine the soil’s field capacity (FC), available water (AW), bulk density (BD), and wilting and saturation point (WSP). The distribution of soil textural characteristics, i.e., sand, silt, and clay percentage, was assessed using the hydrometer approach (Gangwar and Baskar, 2019).
2.4 Anthropogenic variables assessment and quantification of diversity indices
Semi-structured interviews were conducted with 200 respondents to identify the anthropogenic factors threatening the diversity of N. officinale communities. The respondents were selected based on their connection with the collection and sale of plant species. Following Kefalew et al. (2015), the respondents were categorized into two groups; i.e., category “A” includes 100 respondents selected randomly, and category B has 100 respondents that were chosen purposefully having past and present knowledge of the site where the plant species grows naturally. These respondents identified factors, i.e., mining factor, agricultural field disturbance (cultivation factors), grazing intensity, and over-harvesting that disturbed these communities severely. Of the respondents, 12 respondents, i.e., 5 from Category A and 7 from Category B, were excluded from the analysis as they do not respond to the questionnaire.
A six-point scale (0–5) was used to evaluate whether these anthropogenic factors are affected quantitatively. A plot was considered undisturbed if it obtained a score of 0, while a score of 5 was considered severely disturbed (Mligo, 2011). Accordingly, 0 denotes no disturbance, 1 represents a disruption of 0%–20% of the plot, 2 denotes a disruption of 21%–40% of the plot, 3 denotes a disruption of 41%–60% of the plot, 4 denotes a disruption of 61%–80% of the plot, and 5 denotes a disruption of 81–100% of the plot. Each type of disturbance was assessed separately in this semi-quantitative evaluation. The degrees of disturbance were evaluated based on the percentage of the given parameter persisting in a disturbing plot of 10 × 10 m. The point scale values were assessed according to Barry (2006) and Lewis et al. (2017) to describe various forms of anthropogenic disturbance.
In addition, the species richness and diversity indices, i.e., Shannon index H’ and Evenness index E, were used to describe group diversity (Maan et al., 2021).
where Σ = summation, S = species richness, pi = proportion of the species (i) to total number of species, In = natural logarithm.
2.5 Community data analysis and ordination
The species’ phytosociological data and relevant environmental parameters for the 34 stands were collected and compiled for statistical analysis. An important value index (IVI) based on comparative phytosociological features was calculated following Khan et al. (2013). Ward’s agglomerative technique (McCune, 1997) was used to evaluate the classification of vegetative communities by choosing Euclidean distance. Each species in the stand was then assigned a phytosociological group based on the numbers assigned to them. We examined recent studies to see whether a particular species might be taken as illustrative of a stand or group (Biondi et al., 2015; Rahman et al., 2022). The converted relative phytosociological values were used for numerical classification to determine the diagnostic species of a particular group. The Redundancy analysis (RDA) was used to determine the relationship between species IVI and environmental variables (20 soil and 4 topographic) of the N. officinale-dominated vegetation. The statistical post-hoc interpretation of the RDA ordination axes was analyzed using the Monte Carlo permutation test. The ordination bi-plot displayed the essential variables as vectors that determined the community structure and composition. Microsoft Excel 2010 and PC-ORD version 6.0 were used to conduct the statistical analysis of the quantitative vegetation and environmental data (Barua et al., 2020).
3 Results
3.1 Floristic characteristics
The floristics of vegetation consists of 22 species dominated by N. officinale, where all the species were either emergent or floating aquatic habitats indicating a wetland community. The species belongs to 16 families; Poaceae and Asteraceae were represented by three species each, followed by Polygonaceae and Fabaceae (two species each). The remaining 12 families were monospecific (Figure 3), where herbaceous plants were evident (100%) in the floristic composition, with 13 species having annual life cycles, 8 perennials, and 1 having both (Table A1). Similarly, based on status, 17 species were classified as native to Pakistan, 3 as invasive, and 1 each for cosmopolitan and naturalized. In addition, based on aquatic habitat, 18 species were emergent, and 4 were emergent/floating (Figure 3).
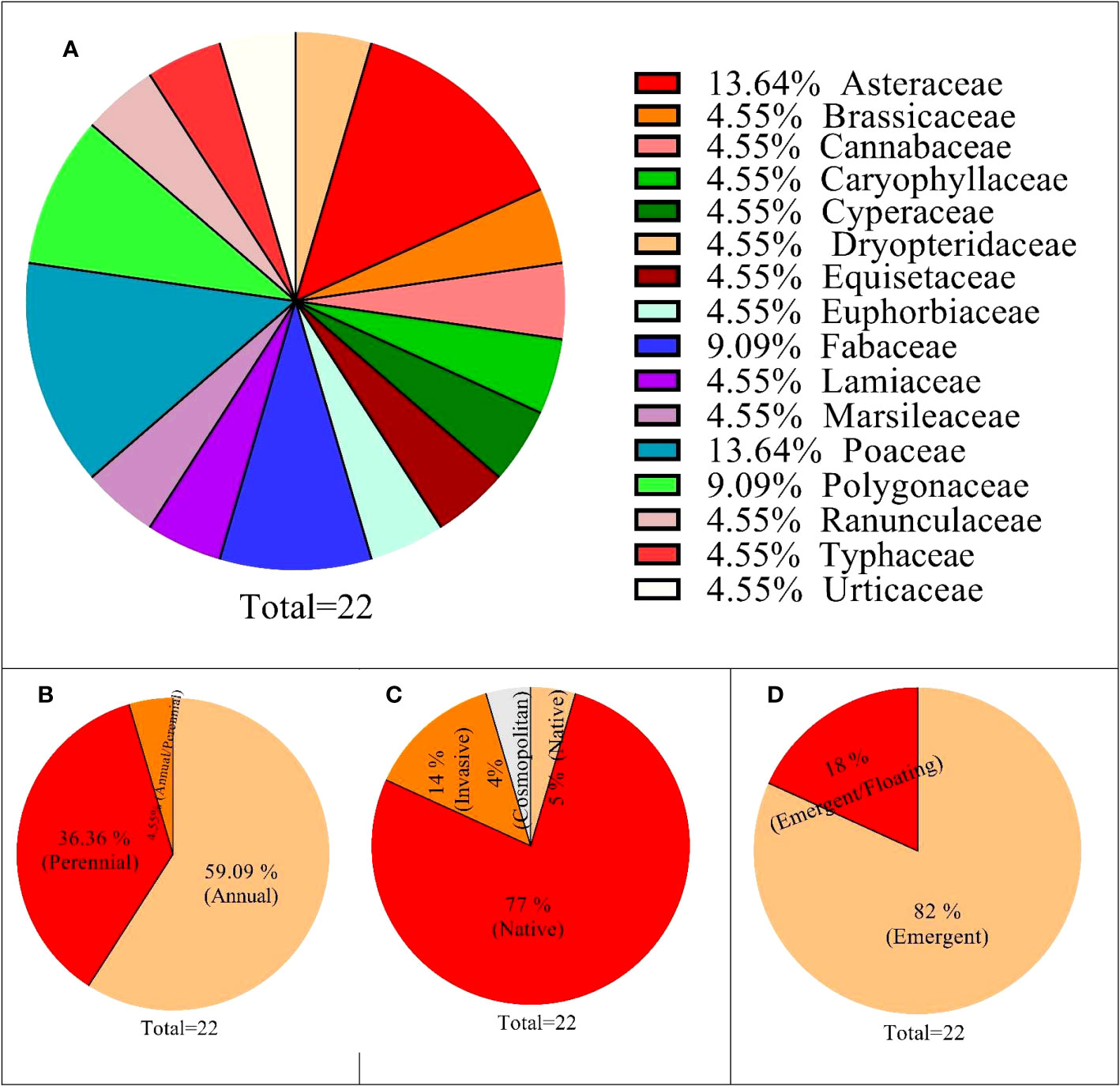
Figure 3 Floristic structure of Nasturtium officinale and associated species found in communities. (A) (family base distribution); (B) (life cycle), (C) (taxon), (D) (status in Pakistan), and (E) (aquatic habitat).
3.2 Vegetation classification and structural attributes
The two-way cluster dendrogram indicates four vegetation groups showing stands on one side and species distributed in these groups on the other (Figure 4). Similarly, the red dots indicated the intensity of the species’ IVI, i.e., its increase or decrease in the studied stands. Based on the number of stands, Group II was the largest, including 13 stands in the middle of the dendrogram. Group III was the smallest, having five stands of N. officinale-dominated vegetation. Group I has seven stands with 14 species and is considered the third largest community of vegetation dominated by N. officinale (42.9 ± 1.0), followed by Polygonum glabrum (19.4 ± 0.5) and Cynodon dactylon (13.4 ± 0.5). In comparison, the remaining 11 species of this group have a mean IVI of less than 10 as presented in Table 1. Group II has 13 stands with seven species and was considered the largest group with low species richness. The dominant species of the group was N. officinale (53.4 ± 0.4), having co-dominance of Cynodon dactylon (31.8 ± 2.5).
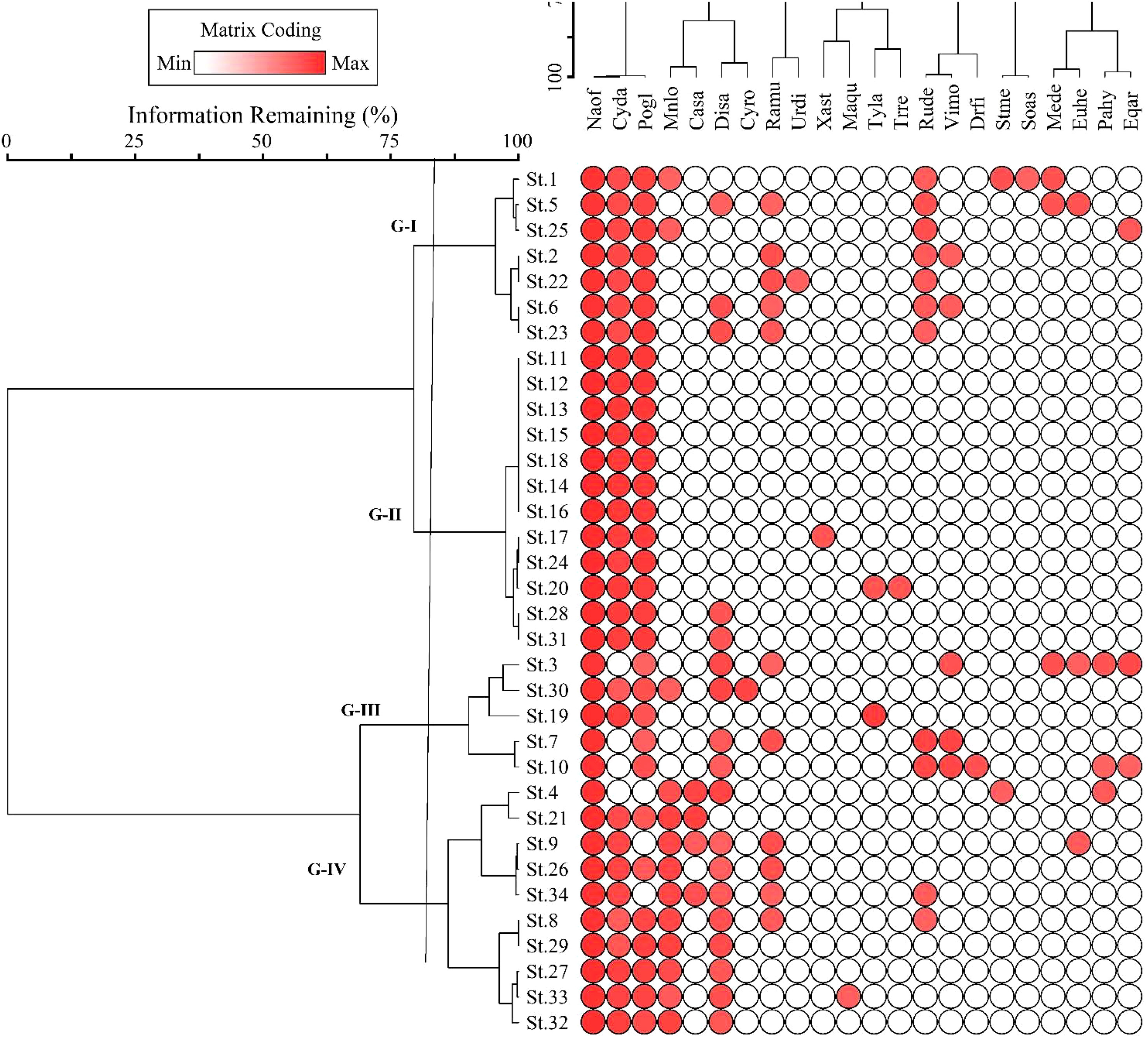
Figure 4 Two-way cluster dendrogram of Nasturtium officinale communities along the Swat River catchments. Plant species acronyms are the same as that in Table 1.
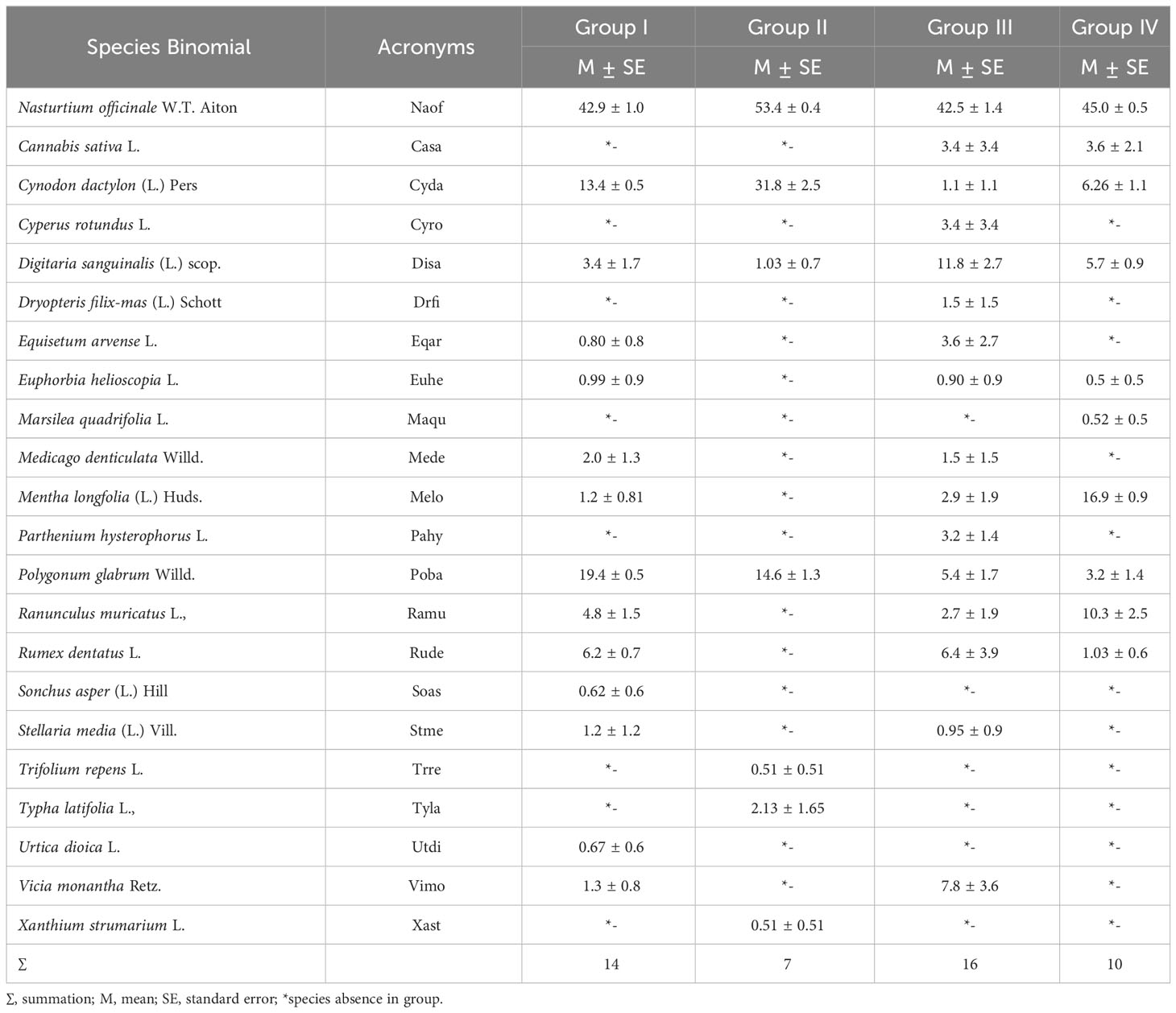
Table 1 Importance values index (IVI) of different plant species associated with Nasturtium officinale in different vegetation groups along the Swat River catchments.
Group III consists of five stands with 16 species dominated by N. officinale (42.5 ± 1.4) while the co-dominant species was Digitaria sanguinalis (11.8 ± 2.7). The important associated species of the group include Vicia monantha (7.8 ± 3.65), Rumex dentatus (6.4 ± 3.9), and Polygonum glabrum (5.4 ± 1.7). Similarly, Group IV consisted of 9 stands with 10 species and was considered the second largest community dominated by N officinale (45.0 ± 0.5), with Mentha longfolia (16.9 ± 0.9) being the co-dominant species. The other important species of the group are Ranunculus muricatus (10.3 ± 2.5) and Cynodon dactylon (6.26 ± 1.1).
Density/hectare and cover/hectare of N. officinale, and associated species (Table A2), revealed that, in Group I, the density of N. officinale is 46,243 ± 4,119 individuals/hectare. At the same time, the co-dominant species was C. dactylon (18,730 ± 2,316). In Group II, N. officinale has a density/ha of 57,094 ± 2,469 followed by C. dactylon (13,162 ± 1,939). Similarly, Group III is the largest community by the number of species, including N. officinale, with a density/hectare of 47,555 ± 7,702. However, the co-dominant species was D. sanguinalis (10,222 ± 3,723) while Group IV included 10 species having C. dactylon (11,851 ± 2,051) as the co-dominant species. Similarly, the same patterns have been followed by cover/hectare of N. officinale and the remaining co-dominant species.
3.3 Analysis of environmental and anthropogenic variables
The results of associated soil variables showed a sandy or silty loamy soil having significant variation in sand and clay particles (p< 0.05). In contrast, Groups II and IV have high contents of silt and sand (Table 2). The soil pH was basic, showing non-significant variation, while the electrical conductivity of Group III was higher (0.3 ± 0.05) than others. Similarly, total dissolved solids were high in Groups III and II, i.e., 0.09 ± 0.005 and 0.09 ± 0.01, respectively, complementing the electrical conductivity results. The soil nutrients, Group IV, had higher contents of organic matter (0.97 ± 0.02), and varied significantly (p< 0.05) within the vegetation groups, while the nitrogen content of all the community groups was the same, i.e., 0.04 ± 0.002. In addition, the essential nutrients, i.e., phosphorus and potassium contents (mg kg−1) of Group II (35.0 ± 3.21) and Group III (126 ± 21), were higher, respectively, compared to the other groups. Moreover, the heavy metals, i.e., cadmium, lead, and copper, showed significant variation among the communities (p< 0.05), revealing their prominent role in maintaining communities’ structure and composition.
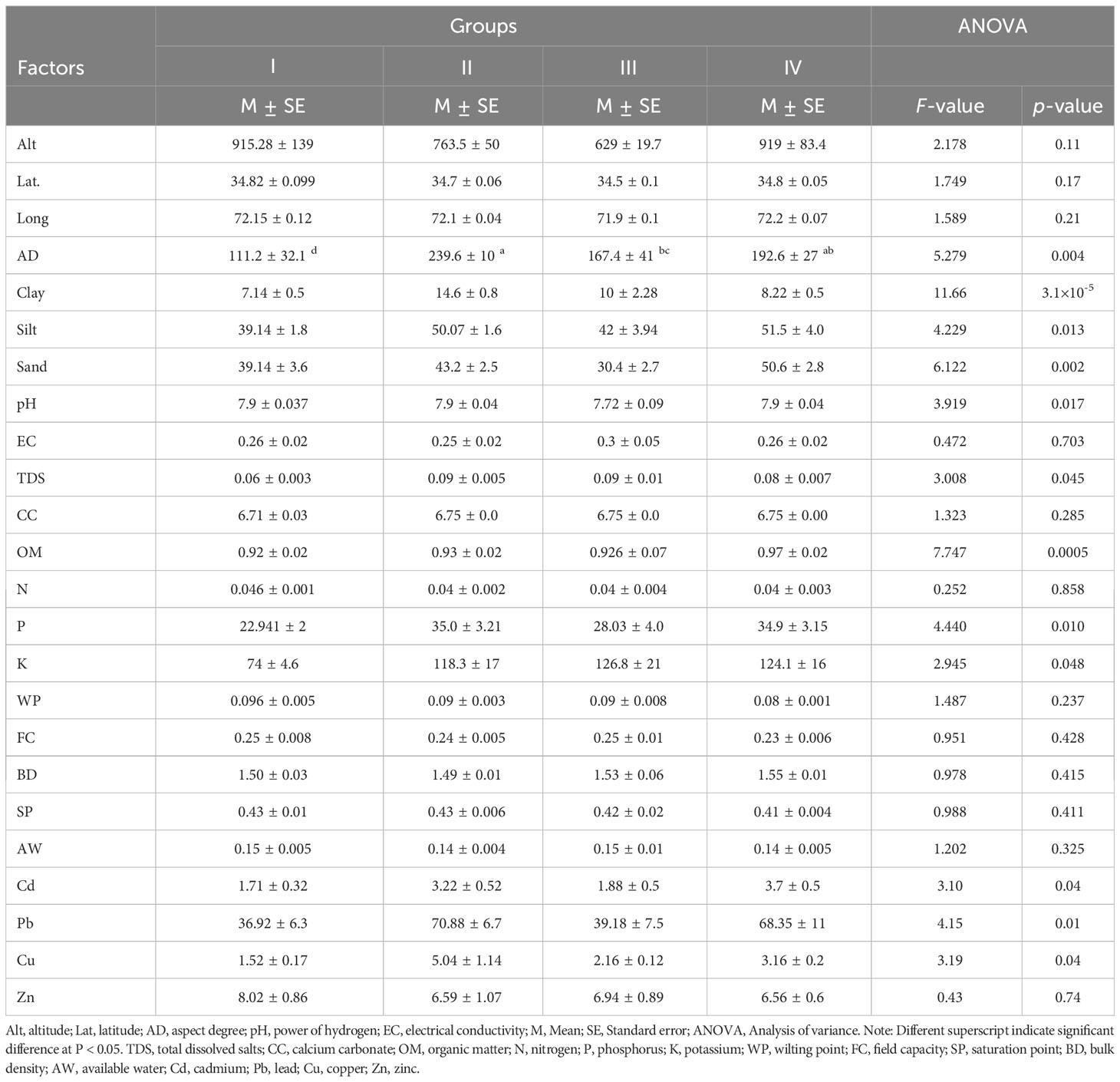
Table 2 Soil characteristics of different N. officinale dominated vegetation in the Swat River tributaries.
The anthropogenic factors identified by respondents during the semi-structure interview showed non-significant χ2 results between the Category “A” and Category “B” population, revealing that both the categories of factors affect the vegetation of N. officinale-dominated communities (Table 3). In addition, the lower cumulative variance percentage indicated that these anthropogenic factors equally contributed in disturbing vegetation groups. The quantitative anthropogenic factors (Table 4) vary across the communities coupled with IVI gradients; i.e., mining factor, cultivated fields percentage, and grazing intensity were higher in Group II and progressively decrease towards Group I via Groups III and IV (F = 3.56 and 7.61, respectively at p< 0.05). However, the reverse was true for over-harvesting and varied significantly, having F = 9.21 (p< 0.001). The diversity indices show increasing trends with increase in elevation. Similarly, the diversity indices were in reverse trends with N. officinale IVI; i.e., Group II has low species richness (3.33 ± 0.49) and higher IVI, while Group III with high species richness has low IVI. The Shannon–Wiener diversity index ranges from 0.97 ± 0.20 to 1.16 ± 0.24 and varies significantly (p< 0.05). Similarly, the species evenness index ranges from 0.62 ± 0.07 to 0.69 ± 0.05, showing significant variation (p > 0.05), indicating that the anthropogenic factors disturbed the group’s diversity.

Table 3 Anthropogenic disturbance semi-structure interview qualitative factor citation identified by respondents.
3.4 Response of communities’ composition to environmental and anthropogenic factors
In the RDA ordination (Table 5), the species–environment association was higher on the first two axes, contributing 44.8% of the overall variation (50.6%). Each of the 24 soil variables separately contributed to the overall ordination since none of the inflation factors had a score higher than 20.0. The Inter-set correlations, soil variables, like organic matter, clay, and pH, showed a significant positive correlation with the first axis (r = 0.51, 0.56, and 0.49, respectively). Similarly, silt, sand, and organic matter were significantly positively correlated on axis 2 (r = 0.32, 0.31, and 0.31 respectively). In contrast, sand percentage had a negative correlation (r = −0.25) on axis 3. Similarly, in anthropogenic variables, mining factor, cultivation factor, and grazing intensity showed positive correlation on axis 1 (r = 0.46, 0.57, and 0.67 respectively), while overexploitation showed negative correlation on axis 1 (r = −0.61). An unconstrained Monte Carlo permutation test (499 permutations) revealed that the F-ratio for the eigenvalue of axis 1 was significant (p = 0.05). The RDA ordination bi-plot demonstrated the correlation between the stands occupied on the right side and several soil characteristics, including organic matter, clay, sand, clay, phosphorus, pH, and aspect degree (Figure 5). On the other hand, electrical conductivity and calcium carbonate were associated with the remaining plant groups that filled the bi-plot’s left side. These factors also show higher biplot scores (Table A3).
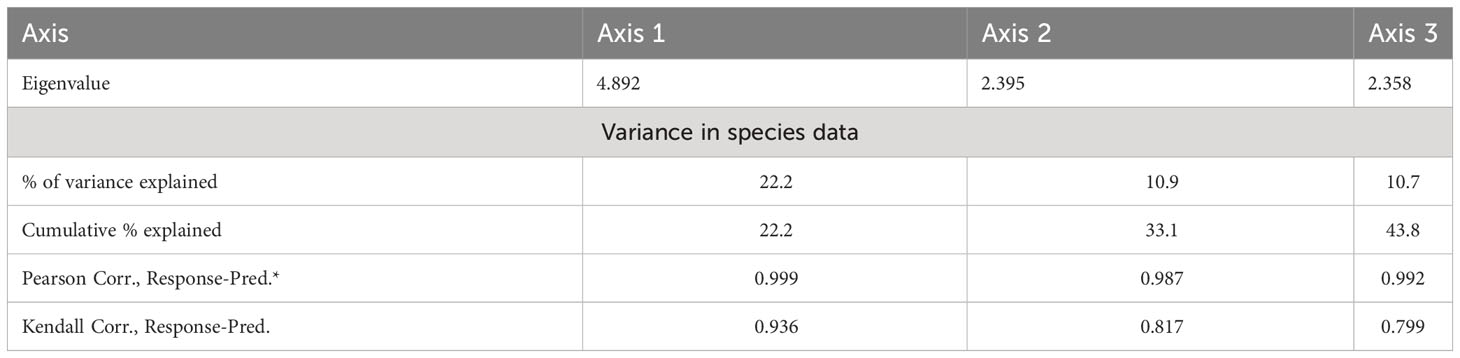
Table 5 Axis summary statistics (number of canonical axes: 3 of a possible 22; total variance in the species data: 43.8).
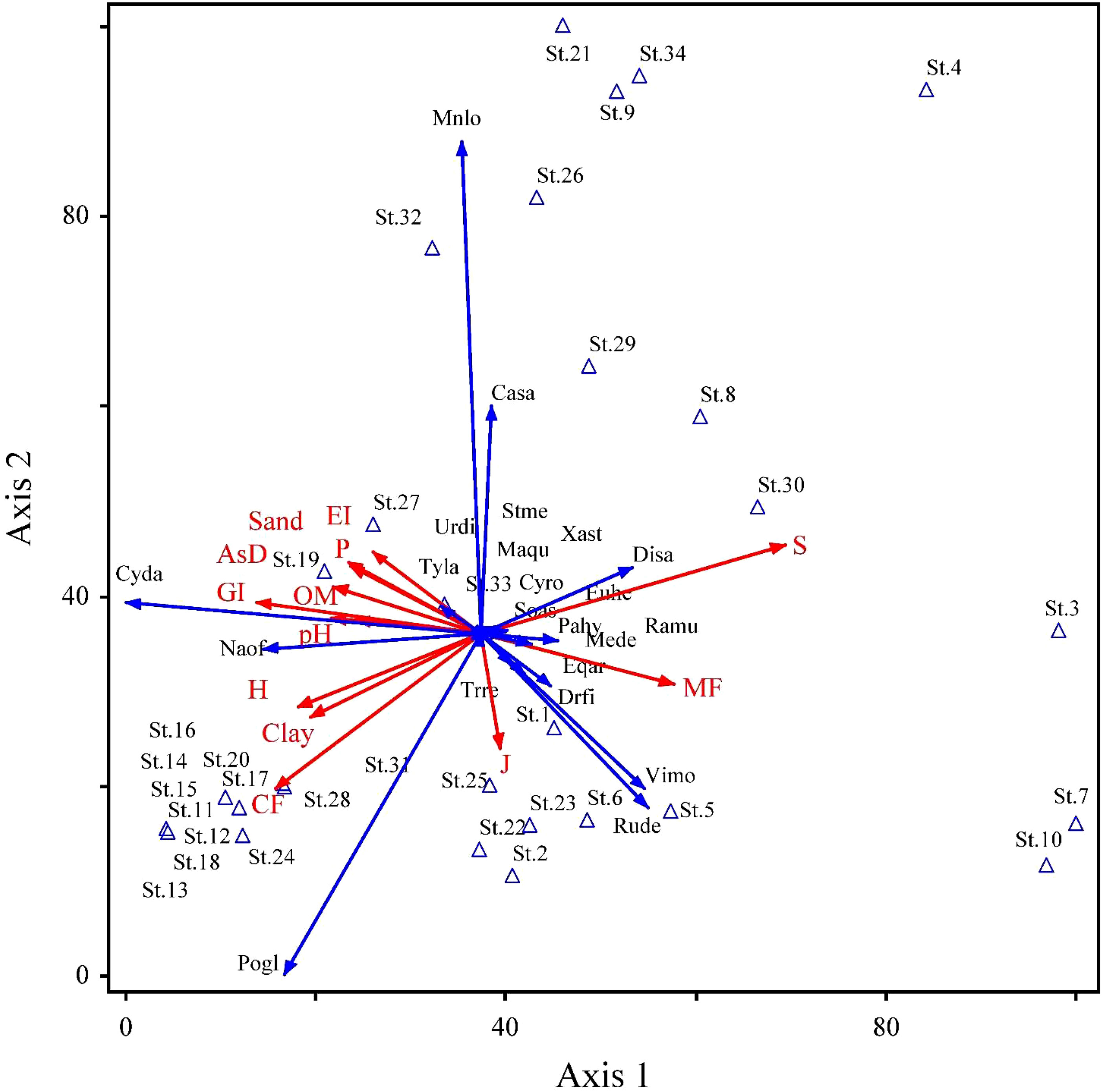
Figure 5 Projection of 22 species distributed in 34 stands with corresponding environmental variables on the 2D-RDA axis along the Swat River catchments and small tributaries. Importance values of species and 24 environmental variables were used in the analysis (for details, see Tables 2, 5, Table A1). Plant species, and environmental and anthropogenic factors’ acronyms are the same as that in Tables 1–4.
4 Discussion
The floristic composition shows a narrow spectrum of vegetation in the aquatic plant communities of N. officinale across the River Swat having 22 species, which are dominated by native species (77%) and non-native species (invasive, naturalized, and cultivated). Similarly, the range of life forms comprises annual herbaceous plants representative of 16 plant families. These spectrums show the physiognomy of the flora and vegetation, which is the result of all living activities combined with the environment, making it vital to comprehend the ecological foundation of vegetation of N. officinale (Amjad et al., 2016). The dominance of annual plant species in communities can bring community homogeneity that favors the invasion of foreign species (Milchunas et al., 1988; Tappeiner et al., 1991; Qian and Guo, 2010). The vegetative reproductive potential of N. officinale may also contribute to community homogenization, resulting in the formation of less diverse vegetation. Similarly, smaller herb species like Xanthium strumarium, Mentha longifolia, Cynodon dactylon, and Cannabis sativa may be advantageous over native species since they can more easily spread across the communities (Timsina et al., 2011). The future vegetation diversity will be at risk, due to this relocation, which ultimately brings communities’ disturbance. These results were contrary to what has been observed in other aquatic ecosystems; non-native species comprise a significant and substantially greater component of submerged aquatic plant communities (Santos et al., 2011; Du Toit et al., 2021), while in this case, N. officinale, a native invasive species, dominated the communities.
The cluster analysis segregated four unique vegetation groups, each dominated by N. officinale and have different co-dominant species. Polygonum glabrum was the co-dominant species in Group I, Cynodon dactylon in Group II, Digitaria sanguinalis in Group III, and Mentha longfolia in Group IV. Similarly, a study was conducted on the phytosociology of Sedum brevifolium and found six plant groups (Nabi et al., 2012), while Ali et al. (2022) reported two plant communities across the wetlands of Panjkora River in district Dir Lower. At the sample sites, N. officinale was often found with several associated species, including Polygonum glabrum, Cynodon dactylon, and Digitaria sanguinalis. The same environmental factors and nutritional requirements might cause their communities’ association, as reported by Khan (2012); Khan et al. (2014); Muhammad et al. (2016); Nasrullah et al. (2015), and Shah and Hussain (2009).
The soil characteristics revealed that the calcareous nature accounted for roughly 99% of the growth of N. officinale. The calcareous soils with high potassium, nitrogen, and low phosphorus concentrations range from sandy loam to loamy silty texture, resulting in gregarious communities, as that reported in the region by Ullah et al. (2021) and Ullah et al. (2022). The smaller communities having high density and cover in terms of species composition may be attributed to two factors, i.e., the communities are new to the region and the potential of N. officinale to regenerate (Ali et al., 2022). Our results were contrasted to that reported by Khan et al. (2020), who reported that seedlings and juvenile plants grow quicker than mature plants, resulting in a decreased density. However, these results complied with Hitimana et al. (2004), where the high density is linked to the plant species’ capacity for regeneration. The community structure and composition may change due to man-made activities, intra- and inter-specific competition, and the pattern of regeneration (Faridah-Hanum et al., 2012). These soils also allow water and air to move through them, letting roots penetrate more readily, providing nutrients and clay aggregation stability (Zimmermann, 1991). Certain invasive generalist species, such as C. sativa, X. strumarium, and C. dactylon, pioneer species of disturbed vegetation, were dominant in the communities (Iqbal et al., 2021). N. officinale probably establishes itself as the dominant species in wetlands in Khyber Pakhtunkhwa due to these environmental factors that favor their propagation and growth. In addition, the vegetation decomposition and microbial activities in the root zone may further add soil nutrients that add to the fertility of soil and resulting in the establishment and propagation of a particular community in an area (Bargali et al., 2018).
As expected, the anthropogenic variables substantially disrupted the communities’ structure, favoring non-native species invasion (Geiger and McPherson, 2005; Bhattarai et al., 2014). The study assessed mining factor, grazing pressure, cultivated fields, and over-harvesting to determine how these factors relate to the disturbance of N. officinale communities. However, these factors vary significantly along IVI gradients, showing that they are linked to invasive species and community disturbance. The sites of Group II had greater grazing intensity, agricultural activities or areas occupied by cultivated fields, and mining factors, making the populations more susceptible to invasion, as reported by Chhogyel et al. (2021) and Pretto et al. (2010). This could be due to the easy transportation of the alien species propagules in such areas. According to McDougall et al. (2005) and Sjödin et al. (2008), grazing pressure and mining factor disturbed communities by generating unoccupied niches for alien propagules, enabling the plant to invade the vacant habitats. Similarly, sand extraction from aquatic environments, like rivers and coasts, provides an ecosystem service (Grizzetti et al., 2019). Sand is primarily used in construction because concrete contains 75% sand (Jnr et al., 2018). All this equates to approximately 200 tons of sand for a home, 30,000 tons for every kilometer of roadway, and an astounding 12 million tons of sand for a nuclear power station (Ludacer, 2018; Rentier, and Cammeraat, 2022). This tremendous sand demand has made mining a global environmental concern that disturbed community structure (Asabonga et al., 2017). This mining operation caused habitat fragmentation that continuously disturbed these communities in the river bed and char-land areas.
RDA of vegetation–soil interactions revealed that organic matter, silt, sand, clay, phosphorus, potassium, pH, latitude, longitude, aspect degree, wilting point, field capacity, and available water were the key factors controlling the distribution of vegetation in the region. The role of organic carbon in soil fertility is well known and reported by many authors like Kononova (2013) and Rao et al. (2017). Similarly, increase in soil organic matter content results from the breakdown of plant residues, lower salt toxicity from dissolved potassium and calcium brought by rainwater (Taiz and Zeiger, 2002), and increasing vegetation diversity (Lyons et al., 2005). Moreover, many studies like Ali et al. (2022); Ullah et al. (2022), and Ullah et al. (2021) in the same region reported the significance of soil organic matter, nitrogen, and soil texture in sustaining plant communities. Similar research on the influence of surface sediment percentages of various size classes on the geographical distribution of soil moisture was reported by Dasti and Agnew (1994). In addition, many other factors, such as precipitation, rain, and flood, increase the percentages of silt and clay in the soil texture that play an important role in shaping plant communities (Duniway et al., 2010). The most significant factors closely related to the dispersion of vegetation communities are nutritional status, electrical conductivity, soil texture, slope, and aspect (Tavili and Jafari, 2009). Our results were also in agreement with those of Zare et al. (2011), who reported that the distribution of plants is influenced by slope, aspect elevation, soil texture, lime content, soil moisture, available nitrogen, potassium, and organic matter, and Bargali et al. (2018), revealing the importance of soil organic matter and nitrogen in maintaining plant communities.
5 Conclusions
The study concluded that topography and spatial variability influenced the N. officinale vegetation’s distribution in the area. In addition, the anthropogenic factors qualitative and quantitative intensity have the most prominent role in disturbing the communities. In addition, it has been shown that the spread of N. officinale vegetation is more strongly correlated with physical environmental factors and substrate characteristics that affect water availability. The disturbance in communities can be minimized by land use to change the water conditions in wetlands directly or indirectly by draining them to make the surface drier and by introducing plant species like N. officinale that are tolerant to flooding and produce more crops and feed. In addition, non-wetland species swiftly builds up peaty soils in the wetlands, elevating the surface above the water building diverse communities. However, the presence of non-native species may subject the communities to unexpected changes in vegetation; therefore, proper management of non-native invasive species should be considered for future perspective.
Data availability statement
The original contributions presented in the study are included in the article/Supplementary Material. Further inquiries can be directed to the corresponding author.
Author contributions
NK and RU conceptualized this research, conducted the field and laboratory experiments, and compiled and analyzed the data; NK and RU wrote the original draft of the manuscript; MO and MA-M supervised the experiments; IS, H-AH, and TA contributed to data analysis; and HA helped in the review and edited the initial draft of the manuscript; all authors read and approved the manuscript.
Acknowledgments
We sincerely acknowledge the PCSIR Laboratories for providing the necessary equipment and chemicals for different soil physiochemical and nutrient analysis. The authors also extend their appreciation to the researchers supporting project number (RSP2023R374) King Saud University, Riyadh, Saudi Arabia.
Conflict of interest
The authors declare that the research was conducted in the absence of any commercial or financial relationships that could be construed as a potential conflict of interest.
Publisher’s note
All claims expressed in this article are solely those of the authors and do not necessarily represent those of their affiliated organizations, or those of the publisher, the editors and the reviewers. Any product that may be evaluated in this article, or claim that may be made by its manufacturer, is not guaranteed or endorsed by the publisher.
Supplementary material
The Supplementary Material for this article can be found online at: https://www.frontiersin.org/articles/10.3389/fpls.2023.1225030/full#supplementary-material.
References
Ahmad, L., Semotiuk, A., Zafar, M., Ahmad, M., Sultana, S., Liu, Q.-R., et al. (2015). Ethnopharmacological documentation of medicinal plants used for hypertension among the local communities of DIR Lower, Pakistan. J. Ethnopharmacol. 175, 138–146. doi: 10.1016/j.jep.2015.09.014
Ali, S. (2008). Significance of flora with special reference to Pakistan. Pak. J. Bot. 40 (3), 967–971.
Ali, A., Khan, T. A., Ahmad, S. (2018). Analysis of climate data of Khyber Pakhtunkhwa, Pakistan. Int. Res. J. Eng. Technol. 5 (5), 4266–4282.
Ali, K., Khan, N., Ullah, R., Shah, M., Khan, M. E. H., Jones, D. A., et al. (2022). Spatial Pattern and Key Environmental Determinants of Vegetation in Sand Mining and Non-Mining Sites along the Panjkora River Basin. Land 11 (10), 1801. doi: 10.3390/land11101801
Alsterberg, C., Eklöf, J. S., Gamfeldt, L., Havenhand, J. N., Sundbäck, K. (2013). Consumers mediate the effects of experimental ocean acidification and warming on primary producers. Proc. Natl. Acad. Sci. 110 (21), 8603–8608. doi: 10.1073/pnas.1303797110
Amjad, M. S., Arshad, M., Sadaf, H. M., Akrim, F., Arshad, A. (2016). Floristic composition, biological spectrum and conservation status of the vegetation in Nikyal valley, Azad Jammu and Kashmir. Asian Pac. J. Trop. Dis. 6 (1), 63–69. doi: 10.1016/S2222-1808(15)60986-0
Asabonga, M., Cecilia, B., Mpundu, M. C., Vincent, N. M. D. (2017). The physical and environmental impacts of sand mining. Trans. R. Soc. S. Afr. 72 (1), 1–5.
Baboo, B., Sagar, R., Bargali, S. S., Verma, H. (2017). Tree species composition, regeneration and diversity of an Indian dry tropical forest protected area. Trop. Ecol. 58 (2), 409–423.
Bargali, K., Manral, V., Padalia, K., Bargali, S. S., Upadhyay, V. P. (2018). Effect of vegetation type and season on microbial biomass carbon in Central Himalayan Forest soils, India. Catena 171, 125–135. doi: 10.1016/j.catena.2018.07.001
Bargali, S. S., Padalia, K., Bargali, K. (2019). Effects of tree fostering on soil health and microbial biomass under different land use systems in the Central Himalayas. Land. Degradation. Dev. 30 (16), 1984–1998. doi: 10.1002/ldr.3394
Bargali, S. S., Shahi, C., Bargali, K., Negi, B., Khatri, K. (2022). Energy and monetary efficiencies at the different altitudinal agroecosystems in central Himalaya, India. Heliyon 8 (11), 1–16. doi: 10.1016/j.heliyon.2022.e11500
Barinova, S., Ali, N., Barkatullah, S. F. (2013). Ecological adaptation to altitude of algal communities in the Swat Valley (Hindu Cush Mountains, Pakistan). Expert Opin. Environ. Biol. 2), 2. doi: 10.4172/2325-9655.1000104
Barry, S. (2006). Understanding Livestock Grazing Impacts: Strategies for the California Annual Grassland and Oak Woodland Vegetation Series: University of California, Division of Agriculture and Natural Resources. Berger Drive, San Jose, CA 95112
Barua, N., Aziz, M. A. I., Tareq, A. M., Sayeed, M. A., Alam, N., ul Alam, N., et al. (2020). In vivo and in vitro evaluation of pharmacological activities of Adenia trilobata (Roxb.). Biochem. Biophys. Rep. 23, 100772. doi: 10.1016/j.bbrep.2020.100772
Bhattarai, K. R., Måren, I. E., Subedi, S. C. (2014). Biodiversity and invasibility: Distribution patterns of invasive plant species in the Himalayas, Nepal. J. Mountain. Sci. 11, 688–696. doi: 10.1007/s11629-013-2821-3
Bhattarai, K. R., Vetaas, O. R. (2003). Variation in plant species richness of different life forms along a subtropical elevation gradient in the Himalayas, east Nepal. Global Ecol. Biogeogr. 12 (4), 327–340. doi: 10.1046/j.1466-822X.2003.00044.x
Biondi, E., Allegrezza, M., Casavecchia, S., Galdenzi, D., Gasparri, R., Pesaresi, S., et al. (2015). New insight on Mediterranean and sub-Mediterranean syntaxa included in the Vegetation Prodrome of Italy. Flora. Mediterr. 25 (Special Issue), 77–102. doi: 10.7320/FlMedit25SI.077
Biplab, B., Sagar, R., Bargali, S. S., Hariom, V. (2017). Tree species composition, regeneration and diversity of an Indian dry tropical forest protected area. Trop. Ecol. 58 (2), 409–423.
Bisht, S., Bargali, S. S., Bargali, K., Rawat, G. S., Rawat, Y. S., Fartyal, A. (2022). Influence of anthropogenic activities on forest carbon stocks—a case study from Gori Valley, Western Himalaya. Sustainability 14 (24), 16918. doi: 10.3390/su142416918
Bisht, S., Rawat, G. S., Bargali, S. S., Rawat, Y. S., Mehta, A. (2023). Forest vegetation response to anthropogenic pressures: a case study from Askot Wildlife Sanctuary, Western Himalaya. Environment. Dev. Sustainabil. 1–25. doi: 10.1007/s10668-023-03130-2
Chao, W., Xiaoan, W., Hua, G., Weiyi, F., Zhihong, Z. (2006). Species diversities of major communities in Malan forest region of the Loess Plateau. Acta Botanica Boreali-Occidentalia. Sin. 26 (4), 791–797.
Chawla, A., Rajkumar, S., Singh, K., Lal, B., Singh, R., Thukral, A. (2008). Plant species diversity along an altitudinal gradient of Bhabha Valley in western Himalaya. J. Mountain. Sci. 5, 157–177. doi: 10.1007/s11629-008-0079-y
Chhogyel, N., Kumar, L., Bajgai, Y. (2021). Invasion status and impacts of parthenium weed (Parthenium hysterophorus) in West-Central region of Bhutan. Biol. Invasions. 23 (9), 2763–2779. doi: 10.1007/s10530-021-02534-3
Curtis, J. T., Mcintosh, R. P. (1950). The interrelations of certain analytic and synthetic phytosociological characters. Ecology 31 (3), 434–455. doi: 10.2307/1931497
Dasti, A., Agnew, A. (1994). The vegetation of Cholistan and Thai deserts, Pakistan. J. Arid. Environments. 27 (3), 193–208. doi: 10.1006/jare.1994.1058
Davidar, P., Sahoo, S., Mammen, P. C., Acharya, P., Puyravaud, J. P., Arjunan, M., et al. (2010). Assessing the extent and causes of forest degradation in India: where do we stand? Biol. Conserv. 143 (12), 2937–2944. doi: 10.1016/j.biocon.2010.04.032
De Leo, F. C., Vetter, E. W., Smith, C. R., Rowden, A. A., McGranaghan, M. (2014). Spatial scale-dependent habitat heterogeneity influences submarine canyon macrofaunal abundance and diversity off the Main and Northwest Hawaiian Islands. Deep. Sea. Res. Part II.: Topical. Stud. Oceanogr. 104, 267–290. doi: 10.1016/j.dsr2.2013.06.015
Deo, R. C., Şahin, M. (2015). Application of the artificial neural network model for prediction of monthly standardized precipitation and evapotranspiration index using hydrometeorological parameters and climate indices in eastern Australia. Atmospheric. Res. 161, 65–81. doi: 10.1016/j.atmosres.2015.03.018
Duniway, M. C., Bestelmeyer, B. T., Tugel, A. (2010). Soil processes and properties that distinguish ecological sites and states. Rangelands 32 (6), 9–15. doi: 10.2111/Rangelands-D-10-00090.1
Du Toit, M. J., Du Preez, C., Cilliers, S. S. (2021). Plant diversity and conservation value of wetlands along a rural–urban gradient. Bothalia-African. Biodivers. Conserv. 51 (1), 1–18.
Faridah-Hanum, I., Kudus, K., Saari, N. S. (2012). Plant diversity and biomass of Marudu Bay mangroves in Malaysia. Pakistan J. Bot. 44 (Suppl 2), 151–156.
Farooq, M., Shafique, M., Khattak, M. S. (2018). Flood frequency analysis of river swat using Log Pearson type 3, Generalized Extreme Value, Normal, and Gumbel Max distribution methods. Arabian. J. Geosci. 11, 1–10. doi: 10.1007/s12517-018-3553-z
Fartyal, A., Khatri, K., Bargali, K., Bargali, S. S. (2022). Altitudinal variation in plant community, population structure and carbon stock of Quercus semecarpifolia Sm. forest in Kumaun Himalaya. J. Environ. Biol. 43 (1), 133–146. doi: jeb/43/1/MRN-2003
Gangwar, D., Baskar, M. (2019). Texture determination of soil by hydrometer method for forensic purpose (Chandigarh, India: Central Forensic Science Laboratory).
Geiger, E. L., McPherson, G. R. (2005). Response of semi-desert grasslands invaded by non-native grasses to altered disturbance regimes. J. Biogeogr. 32 (5), 895–902. doi: 10.1111/j.1365-2699.2004.01235.x
Gómez-Díaz, D., Navaza, J. M., Sanjurjo, B. (2006). Analysis of mass transfer in the precipitation process of calcium carbonate using a gas/liquid reaction. Chem. Eng. J. 116 (3), 203–209. doi: 10.1016/j.cej.2005.12.004
Gosain, B. G., Negi, G. C. S., Dhyani, P. P., Bargali, S. S., Saxena, R. (2015). Ecosystem services of forests: Carbon Stock in vegetation and soil components in a watershed of Kumaun Himalaya, India. Int. J. Ecol. Environ. Sci. 41 (3-4), 177–188.
Grace, J., Anderson, T. (2016). Seabloom EWet al. Integrative modelling reveals mechanisms linking productivity and plant species richness. Nature 529, 390–393.310. doi: 10.1038/nature16524
Grizzetti, B., Liquete, C., Pistocchi, A., Vigiak, O., Zulian, G., Bouraoui, F., et al. (2019). Relationship between ecological condition and ecosystem services in European rivers, lakes and coastal waters. Sci. Total. Environ. 671, 452–465. doi: 10.1016/j.scitotenv.2019.03.155
Hitimana, J., Kiyiapi, J. L., Njunge, J. T. (2004). Forest structure characteristics in disturbed and undisturbed sites of Mt. Elgon Moist Lower Montane Forest, western Kenya. For. Ecol. Manage. 194 (1-3), 269–291. doi: 10.1016/j.foreco.2004.02.025
Htun, N. Z., Mizoue, N., Yoshida, S. (2011). Tree species composition and diversity at different levels of disturbance in Popa Mountain Park, Myanmar. Biotropica 43 (5), 597–603. doi: 10.1111/j.1744-7429.2011.00753.x
Hua, F., Bruijnzeel, L. A., Meli, P., Martin, P. A., Zhang, J., Nakagawa, S., et al. (2022). The biodiversity and ecosystem service contributions and trade-offs of forest restoration approaches. Science 376 (6595), 839–844. doi: 10.1126/science.abl4649
Iqbal, M. F., Feng, Y.-L., Feng, W.-W., Liu, M.-C., Lu, X.-R. (2021). Ecological impacts of the invasive plant Xanthium strumarium and the impacts of three aboveground herbivores on the invader. Ecol. Indic. 131, 108140. doi: 10.1016/j.ecolind.2021.108140
Jiang, Y., Kang, M., Zhu, Y., Xu, G. (2007). Plant biodiversity patterns on Helan mountain, China. Acta Oecol. 32 (2), 125–133. doi: 10.1016/j.actao.2006.12.003
Jnr, A. K. L., Yunana, D., Kamsouloum, P., Webster, M., Wilson, D. C., Cheeseman, C. (2018). Recycling waste plastics in developing countries: Use of low-density polyethylene water sachets to form plastic bonded sand blocks. Waste. Manage. 80, 112–118. doi: 10.1016/j.wasman.2018.09.003
Johnson, P. T., Ostfeld, R. S., Keesing, F. (2015). Frontiers in research on biodiversity and disease. Ecol. Lett. 18 (10), 1119–1133. doi: 10.1111/ele.12479
Kamrani, A., Jalili, A., Naqinezhad, A., Attar, F., Maassoumi, A. A., Shaw, S. C. (2011). Relationships between environmental variables and vegetation across mountain wetland sites, N. Iran. Biologia 66, 76–87. doi: 10.2478/s11756-010-0127-2
Kefalew, A., Asfaw, Z., Kelbessa, E. (2015). Ethnobotany of medicinal plants in Ada’a District, East Shewa Zone of Oromia regional state, Ethiopia. J. Ethnobiol. Ethnomed. 11 (1), 1–28.
Khan, N. (2012). A community analysis of Quercus baloot Griff, forest District Dir, Upper Pakistan. Afr. J. Plant Sci. 6 (1), 21–31. doi: 10.5897/AJPS11.231
Khan, N., Ali, K., Shaukat, S. (2014). Phytosociology, structure and dynamics of Pinus roxburghii associations from Northern Pakistan. J. Forestry. Res. 25, 511–521. doi: 10.1007/s11676-014-0490-x
Khan, S. M., Harper, D. M., Page, S., Ahmad, H. (2011). Species and community diversity of vascular flora along environmental gradient in Naran Valley: A multivariate approach through indicator species analysis. Pak. J. Bot. 43 (5), 2337–2346.
Khan, S. A., Khan, S. M., Ullah, Z., Ahmad, Z., Alam, N., Shah, S. N., et al. (2020). Phytogeographic classification using multivariate approach; a case study from the Jambil Valley Swat, Pakistan. Pak. J. Bot. 52, 279–290. doi: 10.30848/PJB2020-1(11)
Khan, N., Shaukat, S. S., Ahmed, M., Siddiqui, M. F. (2013). Vegetation-environment relationships in the forests of Chitral district Hindukush range of Pakistan. J. Forestry. Res. 24 (2), 205–216. doi: 10.1007/s11676-013-0346-9
Kononova, M. (2013). Soil organic matter: its nature, its role in soil formation and in soil fertility (Oxford London: Pergamon Press).
Kremen, C., Williams, N. M., Aizen, M. A., Gemmill-Herren, B., LeBuhn, G., Minckley, R., et al. (2007). Pollination and other ecosystem services produced by mobile organisms: a conceptual framework for the effects of land-use change. Ecol. Lett. 10 (4), 299–314. doi: 10.1111/j.1461-0248.2007.01018.x
Lewis, J. S., Farnsworth, M. L., Burdett, C. L., Theobald, D. M., Gray, M., Miller, R. S. (2017). Biotic and abiotic factors predicting the global distribution and population density of an invasive large mammal. Sci. Rep. 7 (1), 1–12. doi: 10.1038/srep44152
Li, S., Su, P., Zhang, H., Zhou, Z., Xie, T., Shi, R., et al. (2018). Distribution patterns of desert plant diversity and relationship to soil properties in the Heihe River Basin, China. Ecosphere 9 (7), e02355. doi: 10.1002/ecs2.2355
Rentier, E. S., Cammeraat, L. H. (2022). The environmental impacts of river sand mining. Sci. Total Environ. 838, 155877. doi: 10.1016/j.scitotenv.2022.155877
Lyons, K. G., Brigham, C., Traut, B., Schwartz, M. W. (2005). Rare species and ecosystem functioning. Conserv. Biol. 19 (4), 1019–1024. doi: 10.1111/j.1523-1739.2005.00106.x
Maan, I., Kaur, A., Singh, H. P., Batish, D. R., Kohli, R. K. (2021). Exotic avenue plantations turning foe: Invasive potential, distribution and impact of Broussonetia papyrifera in Chandigarh, India. Urban. Forestry. Urban. Greening. 59, 127010. doi: 10.1016/j.ufug.2021.127010
Manral, V., Bargali, K., Bargali, S. S., Shahi, C. (2020). Changes in soil biochemical properties following replacement of Banj oak forest with Chir pine in Central Himalaya, India. Ecol. Processes. 9, 1–9. doi: 10.1186/s13717-020-00235-8
Martínez-Falcón, A. P., Zurita, G. A., Ortega-Martínez, I. J., Moreno, C. E. (2018). Populations and assemblages living on the edge: dung beetles responses to forests-pasture ecotones. PeerJ 6, e6148. doi: 10.7717/peerj.6148
McCune, B. (1997). Influence of noisy environmental data on canonical correspondence analysis. Ecology 78 (8), 2617–2623. doi: 10.1890/0012-9658(1997)078[2617:IONEDO]2.0.CO;2
McDougall, K. L., Morgan, J. W., Walsh, N. G., Williams, R. J. (2005). Plant invasions in treeless vegetation of the Australian Alps. Perspect. Plant Ecol. Evol. Syst. 7 (3), 159–171. doi: 10.1016/j.ppees.2005.09.001
Mcfarland, D. G., Shafer, D. (2011). Protocol considerations for aquatic plant seed bank assessment. J. Aquat. Plant Manage. 49 (9), 9–19.
Milchunas, D. G., Sala, O. E., Lauenroth, W. K. (1988). A generalized model of the effects of grazing by large herbivores on grassland community structure. Am. Nat. 132 (1), 87–106. doi: 10.1086/284839
Mitsch, W., Gosselink, J. (1993). Wetlands Van Nostrand Reinhold 5th Edn. (John Wiley & Sons, Inc. New York), 722.
Mligo, C. (2011). Anthropogenic disturbance on the vegetation in Makurunge woodland, Bagamoyo district, Tanzania. Tanzania. J. Sci. 37, 94–108.
Morsdorf, F., Mårell, A., Koetz, B., Cassagne, N., Pimont, F., Rigolot, E., et al. (2010). Discrimination of vegetation strata in a multi-layered Mediterranean forest ecosystem using height and intensity information derived from airborne laser scanning. Remote Sens. Environ. 114 (7), 1403–1415. doi: 10.1016/j.rse.2010.01.023
Muhammad, Z., Khan, N., Ali, S., Ullah, A., Khan, S. M. (2016). Density and taxonomic diversity of understory vegetation in relation to site conditions in natural stands of Acacia modesta in Malakand Division, Khyber Pakhtunkhwa, Pakistan. Science 35 (1), 26–34. doi: 10.3923/std.2016.26.34
Nabi, G., Ali, I., Tahir, T., Ahmed, M. (2012). Growth and yield of Kinnow (Citrus reticulata Blanco) and soil physical properties as affected by orchard floor management practices in Punjab, Pakistan. Soil Environ. 31 (2), 163–170.
Nafees, M., Jan, M. R., Khan, H., Ali, A. (2008). Status of soil texture and required associated soil conservation measure of river swat catchments area, NWFP, Pakistan. Sarhad. J. Agric. 24 (2), 251–259.
Naiman, R. J., Bechtold, J. S., Drake, D. C., Latterell, J. J., O’Keefe, T. C., Balian, E. V. (2005). Origins, patterns, and importance of heterogeneity in riparian systems. Ecosyst. Funct. Heterogeneous. Landscapes. 279–309. doi: 10.1007/0-387-24091-8_14
Nasrullah, K., Fayaz, A., Kishwar, A., Shahid, S. (2015). Composition, structure and regeneration dynamics of Olea ferruginea Royle forests from Hindukush range of Pakistan. J. Mountain. Sci. 12, 647–658. doi: 10.1007/s11629-013-2831-1
Nelson, D. W., Sommers, L. E. (1996). Total carbon, organic carbon, and organic matter. Methods Soil Analysis.: Part 3. Chem. Methods 5, 961–1010. doi: 10.2134/agronmonogr9.2.2ed.c29
Nogués-Bravo, D., Araújo, M. B., Romdal, T., Rahbek, C. (2008). Scale effects and human impact on the elevational species richness gradients. Nature 453 (7192), 216–219.
Orellana, F., Verma, P., Loheide, S. P., Daly, E. (2012). Monitoring and modeling water-vegetation interactions in groundwater-dependent ecosystems. Rev. Geophys. 50 (3), 1–24. doi: 10.1029/2011RG000383
Pandey, R., Bargali, S. S., Bargali, K., Pandey, V. C. (2023). Temporal variability in fine root dynamics in relation to tree girth size in sub-tropical sal (Shorea robusta) forests. Land. Degradation. Dev. 34 (5), 1522–1537. doi: 10.1002/ldr.4550
Pepe, M., Fregonese, L., Scaioni, M. (2018). Planning airborne photogrammetry and remote-sensing missions with modern platforms and sensors. Eur. J. Remote Sens. 51 (1), 412–436. doi: 10.1080/22797254.2018.1444945
Pretto, F., Celesti-Grapow, L., Carli, E., Blasi, C. (2010). Influence of past land use and current human disturbance on non-native plant species on small Italian islands. Plant Ecol. 210, 225–239. doi: 10.1007/s11258-010-9751-8
Qian, H., Guo, Q. (2010). Linking biotic homogenization to habitat type, invasiveness and growth form of naturalized alien plants in North America. Diversity Distributions. 16 (1), 119–125. doi: 10.1111/j.1472-4642.2009.00627.x
Raduła, M. W., Szymura, T. H., Szymura, M., Swacha, G., Kącki, Z. (2020). Effect of environmental gradients, habitat continuity and spatial structure on vascular plant species richness in semi-natural grasslands. Agricult. Ecosyst. Environ. 300, 106974. doi: 10.1016/j.agee.2020.106974
Rahman, A., Khan, N., Bräuning, A., Ullah, R., Rahman, I. U. (2022). Effects of environmental and spatial gradients on Quercus-dominated Mountain forest communities in the Hindu-Kush ranges of Pakistan. Saudi. J. Biol. Sci. 29 (4), 2867–2877. doi: 10.1016/j.sjbs.2022.01.013
Rao, C. S., Indoria, A., Sharma, K. (2017). Effective management practices for improving soil organic matter for increasing crop productivity in rainfed agroecology of India. Curr. Sci. 112, 1497–1504. doi: 10.18520/cs/v112/i07/1497-1504
Rybicki, N. B., Landwehr, J. M. (2007). Long-term changes in abundance and diversity of macrophyte and waterfowl populations in an estuary with exotic macrophytes and improving water quality. Limnol. Oceanogr. 52 (3), 1195–1207. doi: 10.4319/lo.2007.52.3.1195
Santos, M. J., Anderson, L. W., Ustin, S. L. (2011). Effects of invasive species on plant communities: an example using submersed aquatic plants at the regional scale. Biol. Invasions. 13, 443–457. doi: 10.1007/s10530-010-9840-6
Saxton, K., Rawls, W. J., Romberger, J. S., Papendick, R. (1986). Estimating generalized soil-water characteristics from texture. Soil Sci. Soc. America J. 50 (4), 1031–1036. doi: 10.2136/sssaj1986.03615995005000040039x
Schlaepfer, D. R., Lauenroth, W. K., Bradford, J. B. (2012). Ecohydrological niche of sagebrush ecosystems. Ecohydrology 5 (4), 453–466. doi: 10.1002/eco.238
Shah, M., Hussain, F. (2009). Phytosociological Study of the Vegetation of Hayat Abadpeshawar, Pakistan. Pakistan J. Plant Sci. 15 (2), 1–12.
Singh, G., Reynolds, C., Byrne, M., Rosman, B. (2020). A remote sensing method to monitor water, aquatic vegetation, and invasive water hyacinth at national extents. Remote Sens. 12 (24), 4021. doi: 10.3390/rs12244021
Sjödin, N. E., Bengtsson, J., Ekbom, B. (2008). The influence of grazing intensity and landscape composition on the diversity and abundance of flower-visiting insects. J. Appl. Ecol. 45, 763–772.
Stella, J. C., Rodríguez-González, P. M., Dufour, S., Bendix, J. (2013). Riparian vegetation research in Mediterranean-climate regions: common patterns, ecological processes, and considerations for management. Hydrobiologia 719, 291–315. doi: 10.1007/s10750-012-1304-9
Subedi, S. C., Bhattarai, K. R., Perez, T. M., Sah, J. P. (2020). Gymnosperm species richness patterns along the elevational gradient and its comparison with other plant taxonomic groups in the Himalayas. Front. Biogeogr. 12 (1). doi: 10.21425/F5FBG44232
Taiz, L., Zeiger, E. (2002). Photosynthesis: physiological and ecological considerations. Plant Physiol. 9, 172–174.
Tappeiner, J., Zasada, J., Ryan, P., Newton, M. (1991). Salmonberry clonal and population structure: the basis for a persistent cover. Ecology 72 (2), 609–618. doi: 10.2307/2937201
Taubner, H., Roth, B., Tippkötter, R. (2009). Determination of soil texture: Comparison of the sedimentation method and the laser-diffraction analysis. J. Plant Nutr. Soil Sci. 172 (2), 161–171. doi: 10.1002/jpln.200800085
Tavili, A., Jafari, M. (2009). Interrelations between plants and environmental variables. Int. J. Environ. Res. 3 (2), 239–246.
Timsina, B., Shrestha, B. B., Rokaya, M. B., Münzbergová, Z. (2011). Impact of Parthenium hysterophorus L. invasion on plant species composition and soil properties of grassland communities in Nepal. Flora-Morphol. Distribution. Funct. Ecol. Plants 206 (3), 233–240. doi: 10.1016/j.flora.2010.09.004
Twilley, R. R., Rivera-Monroy, V. H. (2005). Developing performance measures of mangrove wetlands using simulation models of hydrology, nutrient biogeochemistry, and community dynamics. J. Coast. Res. 79–93.
Ullah, R., Khan, N., Hewitt, N., Ali, K., Jones, D. A., Khan, M. E. H. (2022). Invasive Species as Rivals: Invasive Potential and Distribution Pattern of Xanthium strumarium L. Sustainability 14 (12), 7141. doi: 10.3390/su14127141
Ullah, R., Khan, N., Rahman, A. (2021). Comparison of Invaded And Non-Invaded Sites: A Case Study Of Rough Cocklebur (Xanthium strumarium L.) An Alien Invasive Species. Appl. Ecol. Environ. Res. 19 (2), 1533–1548. doi: 10.15666/aeer/1902_15331548
van de Koppel, J., Bouma, T. J., Herman, P. M. (2012). The influence of local-and landscape-scale processes on spatial self-organization in estuarine ecosystems. J. Exp. Biol. 215 (6), 962–967. doi: 10.1242/jeb.060467
Vis, C., Hudon, C., Carignan, R. (2003). An evaluation of approaches used to determine the distribution and biomass of emergent and submerged aquatic macrophytes over large spatial scales. Aquat. Bot. 77 (3), 187–201. doi: 10.1016/S0304-3770(03)00105-0
Wang, X., Hao, Z., Ye, J., Zhang, J., Li, B., Yao, X. (2008). Spatial pattern of diversity in an old-growth temperate forest in Northeastern China. Acta Oecol. 33 (3), 345–354. doi: 10.1016/j.actao.2008.01.005
Wang, G., Zhou, G., Yang, L., Li, Z. (2003). Distribution, species diversity and life-form spectra of plant communities along an altitudinal gradient in the northern slopes of Qilianshan Mountains, Gansu, China. Plant Ecol. 165, 169–181. doi: 10.1023/A:1022236115186
Watt, S., García-Berthou, E., Vilar, L. (2007). The influence of water level and salinity on plant assemblages of a seasonally flooded Mediterranean wetland. Plant Ecol. 189, 71–85. doi: 10.1007/s11258-006-9167-7
Yadav, R., Tomar, S., Sharma, U. (2002). Output: Input ratios and apparent balances of N, P and K inputs in a rice-wheat system in North-West India. Exp. Agric. 38 (4), 457–468. doi: 10.1017/S0014479702000479
Yu, Y., Li, J., Zhou, Z., Ma, X., Zhang, X. (2021). Response of multiple mountain ecosystem services on environmental gradients: How to respond, and where should be priority conservation? J. Cleaner. Production. 278, 123264. doi: 10.1016/j.jclepro.2020.123264
Zare, S., Jafari, M., Tavili, A., Abbasi, H., Rostampour, M. (2011). Relationship between environmental factors and plant distribution in arid and semiarid area (Case Study: Shahriyar Rangelands, Iran). American-Eurasian. J. Agric. Environ. Sci. 10 (1), 97–105.
Ze-Hao, S., Xin-Shi, Z. (2000). Spatial pattern analysis and topographical interpretation of species diversity in the forests of Dalaoling in the region of the Three Gorges. J. Integr. Plant Biol. 42 (6), 620.
Zhao, C. M., Chen, W. L., Tian, Z. Q., Xie, Z. Q. (2005). Altitudinal pattern of plant species diversity in Shennongjia Mountains, Central China. J. Integr. Plant Biol. 47 (12), 1431–1449. doi: 10.1111/j.1744-7909.2005.00164.x
Keywords: physical environment, vegetation structure, anthropogenic factors, charland, Nasturtium officinale
Citation: Khan N, Ullah R, Okla MK, Abdel-Maksoud MA, Saleh IA, Abu-Harirah HA, AlRamadneh TN and AbdElgawad H (2023) Environmental and anthropogenic drivers of watercress (Nasturtium officinale) communities in char-lands and water channels across the Swat River Basin: implication for conservation planning. Front. Plant Sci. 14:1225030. doi: 10.3389/fpls.2023.1225030
Received: 18 May 2023; Accepted: 05 September 2023;
Published: 27 September 2023.
Edited by:
Qing Zhang, Inner Mongolia University, ChinaReviewed by:
Suresh Chandra Subedi, Arkansas Tech University, United StatesSurendra Singh Bargali, Kumaun University, India
Mahinda Martinez, Autonomous University of Queretaro, Mexico
Copyright © 2023 Khan, Ullah, Okla, Abdel-Maksoud, Saleh, Abu-Harirah, AlRamadneh and AbdElgawad. This is an open-access article distributed under the terms of the Creative Commons Attribution License (CC BY). The use, distribution or reproduction in other forums is permitted, provided the original author(s) and the copyright owner(s) are credited and that the original publication in this journal is cited, in accordance with accepted academic practice. No use, distribution or reproduction is permitted which does not comply with these terms.
*Correspondence: Nasrullah Khan, nasrullah@uom.edu.pk