- 1Biotechnology Division, CSIR-Institute of Himalayan Bioresource Technology, Palampur, HP, India
- 2Academy of Scientific and Innovative Research (AcSIR), Ghaziabad, India
- 3Department of Botany, Goswami Ganesh Dutta Sanatan Dharma College, Chandigarh, India
- 4Department of Biotechnology, Guru Nanak Dev University, Amritsar, Punjab, India
Increased food production to cater the need of growing population is one of the major global challenges. Currently, agro-productivity is under threat due to shrinking arable land, increased anthropogenic activities and changes in the climate leading to frequent flash floods, prolonged droughts and sudden fluctuation of temperature. Further, warm climatic conditions increase disease and pest incidences, ultimately reducing crop yield. Hence, collaborated global efforts are required to adopt environmentally safe and sustainable agro practices to boost crop growth and productivity. Biostimulants appear as a promising means to improve growth of plants even under stressful conditions. Among various categories of biostimulants, microbial biostimulants are composed of microorganisms such as plant growth-promoting rhizobacteria (PGPR) and/or microbes which stimulate nutrient uptake, produce secondary metabolites, siderophores, hormones and organic acids, participate in nitrogen fixation, imparts stress tolerance, enhance crop quality and yield when applied to the plants. Though numerous studies convincingly elucidate the positive effects of PGPR-based biostimulants on plants, yet information is meagre regarding the mechanism of action and the key signaling pathways (plant hormone modulations, expression of pathogenesis-related proteins, antioxidants, osmolytes etc.) triggered by these biostimulants in plants. Hence, the present review focuses on the molecular pathways activated by PGPR based biostimulants in plants facing abiotic and biotic challenges. The review also analyses the common mechanisms modulated by these biostimulants in plants to combat abiotic and biotic stresses. Further, the review highlights the traits that have been modified through transgenic approach leading to physiological responses akin to the application of PGPR in the target plants.
Introduction
Agriculture is presently facing several challenges due to shortage of cultivable land, fluctuating weather conditions, increased incidence of pests and pathogens and rising weed infestations. To increase crop productivity, chemical fertilizers are used indiscriminately (Rouphael and Colla, 2020; Hendriksen, 2022). However, extreme usage of chemicals causes detrimental effects on the soil microorganisms, human and the environment leading to decreased water holding capacity, loss of soil fertility, imbalances in soil nutrients, and increased salinity levels (Wan et al., 2021; Jin et al., 2022). To meet the rising demand for food, boosting crop productivity is imperative. Hence there is a need for a green, efficient, sustainable and economically productive system to improve agronomic traits of crops (Drobek et al., 2019; Cataldo et al., 2022). Currently, biostimulants have emerged as one of the most potent and promising tools for enhancing the growth and productivity of crops naturally, simultaneously addressing the issues related to chemical fertilizers. Biostimulants/bioeffectors/bioprotectors or biobased products are different classes of organic or inorganic compounds which consists of bioactive substances or microorganisms and when applied on target plants promote its growth and productivity (Shahrajabian et al., 2021; Franzoni et al., 2022; Monteiro et al., 2022). Over the time, various researchers have classified biostimulants into nine broad categories including seaweeds and plant extracts, complex organic materials (obtained from sewage sludge extracts, composts, manure urban and agro-industrial waste products), humic substances, antitranspirants (kaolin and polyacrylamide), chitin and chitosan derivatives, elements (Al, Co, Se, Na, and Si), hydrolyzed proteins, nitrogen-containing compounds and microbial inoculants (Colla and Rouphael, 2020; Teklić et al., 2021; Franzoni et al., 2022; Monteiro et al., 2022). Out of this wide category, microbial-based biostimulants including plant growth-promoting bacteria (Bacillus, Serratia, Arthrobacter, Pseudomonas, Rhodococcus, Enterobacter, Ochrobactrum, Acinetobacter, Azospirillum, Rhizobium, Streptomyces and Stenotrophomonas) have surfaced as highly valuable and inexpensive agricultural input for improving plant yield (Orozco-Mosqueda et al., 2020; Baltazar et al., 2021; Miceli et al., 2021; Ayed et al., 2022; Fadiji et al., 2022). These microbiome based biostimulants trigger plant growth through solubilization of minerals (Zn, P and K), nitrogen fixation, production of phytohormones like indole-3-acetic acid (IAA), abscisic acid (ABA), ethylene (ET), cytokinin (CK), jasmonic acid (JA), secondary metabolites (siderophores, N-acyl homoserine lactone, lipopeptides, rhamnolipids, cyclic lipopeptides), enzymes (chitinases, cellulose, protease, glucanase etc.), volatile organic compounds (VOCs) (fatty acids and derivatives, hydrocarbons (alkanes, alkenes and alkynes), carbohydrates, (acids, alcohols, lactones, aldehydes, benzenoids, etc.) terpenoids, nitrogen (metalloid, amides, amines and imines), volatile inorganic compounds (HCN, H2S, NH3, CO2, CO, and NO) and lipopolysaccharides (Caulier et al., 2019; Lephatsi et al., 2021). These components modulate root morphology including biomass of roots, its surface area and newly formed lateral roots, shoot length, leaf area, soil structure (nutrient, water holding capacity, porosity and water filtration), improve nutrient and mineral acquisition (N, P, Fe, Zn, Mn etc.) and photosynthetic capacity of a plant. Further, they also enhance biotic and abiotic stress tolerance by activating genes responsible for antioxidant defense system, production of phenolics, enzymes, amino acids and organic acids (Backer et al., 2018; Rouphael and Colla, 2018; Hamid et al., 2021; Aremu et al., 2022; Lin and Jones, 2022; Vocciante et al., 2022), but the exact functionality at cellular and biomolecular mechanisms are yet to be deciphered (Lephatsi et al., 2021; Othibeng et al., 2022). To elucidate the mechanism of action of biostimulants on plants, combined potential of molecular tools, proteomics, transcriptomics and metabolomics have been harnessed by several researchers (Franzoni et al., 2022). Although progress has been made in understanding the physiological and biochemical aspect of plant-microbe interactions under stress, but the core mechanism and elucidation of molecular interactions are still in infancy. Thus, the present review comprehends the physiological and biochemical modulations along with the signaling components of PGPR based biostimulants in imparting abiotic (major detrimental stresses like temperature, drought and salinity) and biotic stress tolerance in plants. An in-depth understanding of the biochemical and molecular mechanisms triggered by the microbial biostimulants particularly PGPR based will aid in designing and developing novel bioformulations for sustainable agriculture.
Role of PGPR-based biostimulants in combating abiotic stress
Drought and heat stress
About 60% of the world’s region falls under arid and semi-arid areas and depends mainly on irrigated agriculture (Swain et al., 2017). With climate change, it is expected that there will be a decrease in rainfall, a rise in temperature, an increase in atmospheric CO2 and severe alternations in weather conditions leading to frequent floods and droughts (Torres and Henry, 2018). In upcoming years, agriculture will be increasingly challenged by water scarcity, and plants will experience drought and heat stress leading to compromised productivity. Drought stress occurs under low humidity levels in soil, air and high ambient temperature (Lipiec et al., 2013; Kaya et al., 2020; Kosar et al., 2021; Mansoor et al., 2021), while heat stress can be described as an increase in temperature beyond a threshold measure hampering the normal development of a plant. It is observed that the combined action of both stresses restrict the physiological (photosynthesis, respiration, etc.), biochemical and cellular metabolism of plants such as cell membrane fluidity, integrity, elasticity, water potential, stomatal conductance, the structure of amino acids, proteins, nucleic acids, enzymes etc. (Rana et al., 2021; Mitra et al., 2021; Murali et al., 2021a; Noor et al., 2022; Shaffique et al., 2022). In order to adapt under environmental stress plants regulate their diverse molecular signaling pathways such as phytohormones, stress responsive proteins, antioxidants machinery, and osmolytes (Kosar et al., 2021). While understanding the physiological response of plants, it is crucial that both drought and heat stresses must be considered together as the physiological responses are closely interlinked and dependent (Dreesen et al., 2012). Numerous studies have elucidated the positive impact of PGPR functioning as a biostimulant on plants challenged by heat and drought (Rashid et al., 2022; Yasmin et al., 2022). Basically, drought and thermotolerance is a complex mechanism, however, microbial metabolites including organic acids, sugars, trehalose, choline, amino acids, proline, glycine betaine, polyamines, exopolysaccahrides (EPS), production of heat shock proteins (HSPs), dehydrins, VOCs, ACC-deaminase, phytohormones etc. play a vital role in imparting drought and heat tolerance [Figures 1A, B; (Ahluwalia et al., 2021; Ansari et al., 2021; Yasmin et al., 2021a; Notununu et al., 2022; Kour and Yadav, 2022; Shaffique et al., 2022)].
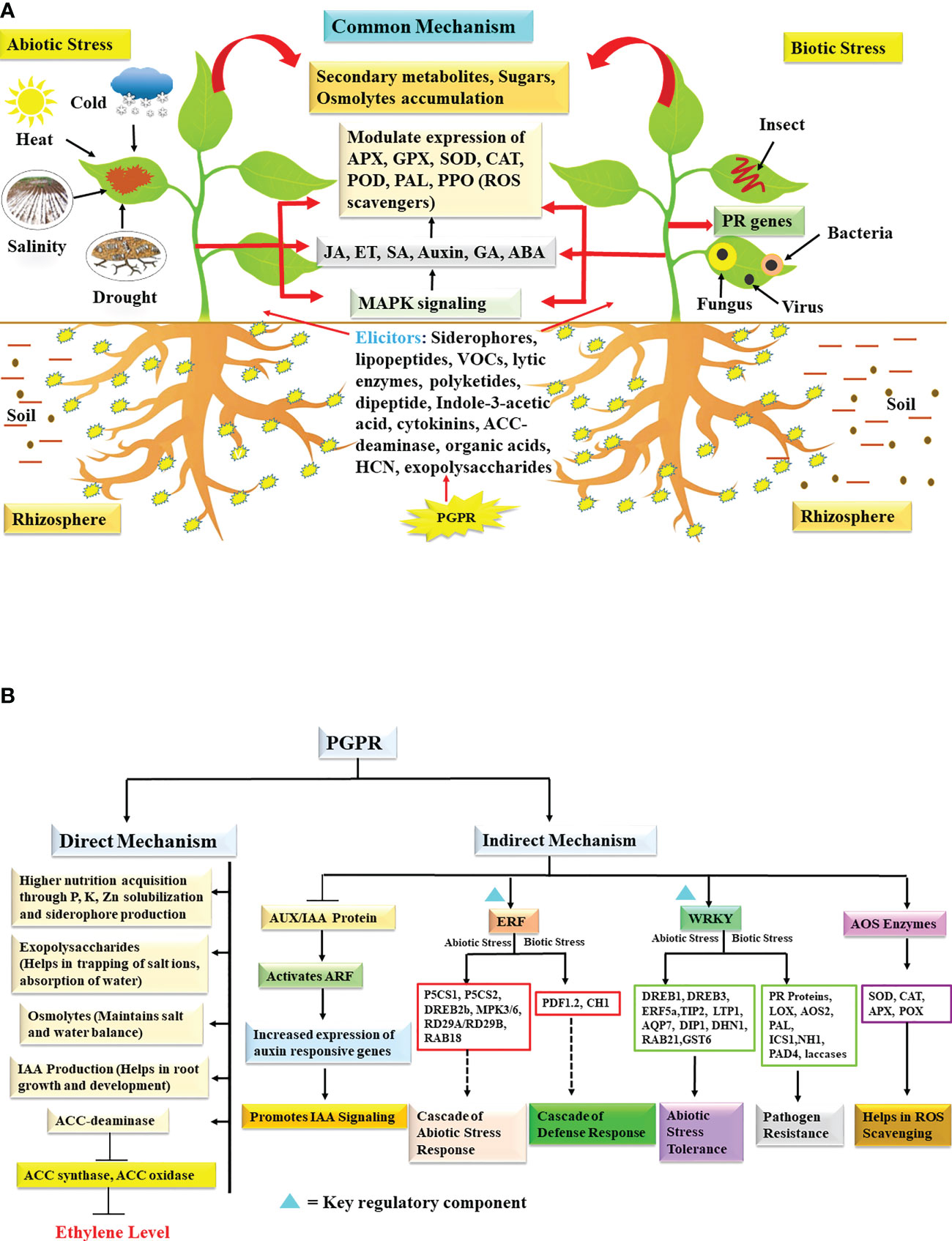
Figure 1 (A) Schematic representation of PGPR induced stress tolerance mechanism in plant challenged by abiotic and biotic stresses. Different elicitors released by PGPRs modulates endogenous phytohormones which in turn influences secondary metabolites, osmolytes production, activity of antioxidant enzymes and PR proteins. These combined metabolic pathways imparts stress tolerance and promotes plant growth under stressed environment. (B) PGPR-based direct and indirect mechanism involved in activating cascade of abiotic and biotic stress signaling in plants. The activation events are represented by arrows, inhibition process is represented by bar while dashed arrows represent signaling cascade. IAA, indole-3-acetic acid; ACC, 1-Amino Cyclopropane-1-Carboxylate; AUX/IAA, auxin/indole-3-acetic acid; ARF, auxin response factor; ERF, ethylene response factor; P5CS1, Δ1 - pyrroline-5-carboxylate synthase1; P5CS2, Δ1 - pyrroline-5-carboxylate synthase 2; DREB2b/DREB1/DREB3, drought-responsive element binding protein 2b, drought-responsive element binding protein 1, drought-responsive element binding protein 3; MPK3/MPK6, mitogen–activated protein kinase 3, mitogen-activated protein kinase 6; RD29A/RD29B, response-to-desiccation 29A, response-to-desiccation 29B; PDF1.2, protodermal factor 1.2; CH1, chitinase; WRKY, W-box domain binding transcription factor; TIP2, tonoplast intrinsic protein 2; LTP1, lipid transfer protein 1; AQP7, aquaporin 7; GST6, glutathione S-transferase; DIP1, dehydration stress-inducible protein 1; DHN1, dehydrin 1; RAB21, responsive to ABA protein 21; PR, pathogenesis related proteins; LOX, lipoxygenase; AOS2, allene oxide synthase 2; PAL, phenylalanine ammonia lyase; ICS1, isochorismate synthase 1; NH1, Arabidopsis NPR1 homolog 1; PAD4, p hytoalexin-deficient 4; AOS, antioxidant scavenging; SOD, superoxide dismutase; CAT, catalase; APX, ascorbate peroxidase; POX, peroxidases; ROS, reactive oxygen species.
1-Amino Cyclopropane-1-Carboxylate (ACC) deaminase produced by several PGPRs help in combating drought stress experienced by its host plant by interfering with the ethylene biosynthesis pathway leading to lowering of ethylene concentration thereby counteracting stress signals. In one of the studies, the application of B. licheniformis K11 capable of producing auxin and ACC deaminase reduced the negative impact of drought in pepper without the use of agrochemicals (Lim and Kim, 2013). Similarly, improved growth was noticed in pea and maize under drought conditions on treating with ACC deaminase producing strain Pseudomonas spp., Enterobacter cloacae, Achromobacter xylosoxidans and Leclercia adecarboxylata primarily due to reduced ethylene accumulation as compared to untreated plants (Arshad et al., 2008; Danish et al., 2020). Additionally, these bacteria are capable of supplying nitrogen by sequestering and degrading ACC to α-ketobutyrate using ACC deaminase (Gupta and Pandey, 2019) thereby promoting better vegetative growth of plants.
Production of reactive oxygen species (ROS) is a common phenomenon observed under drought conditions causing damage to cells (Cruz de Carvalho, 2008) and antioxidant enzymes such as catalase (CAT), peroxidase (POD) and polyphenol oxidase (PPO) scavenge ROS preventing stress related injury thereby imparting stress tolerance [Figures 1A, B; (Zandalinas et al., 2018)]. These antioxidants also promote faster recovery from water limitation and dehydration compared to the control plants (Laxa et al., 2019). Hence, the potential of PGPR in enhancing the production of antioxidants is a desirable attribute. Several studies illustrate the beneficial trend of antioxidant enzymes under severe drought, as was noticed in mentha (Chiappero et al., 2019; Asghari et al., 2020) and in tomato (Mekureyaw et al., 2022). Noticeably, seeds of Pearl millet treated with Bacillus amyloliquefaciens (MMR04) enhanced expression of ascorbate peroxidase (APX) and superoxide dismutase (SOD) genes leading to the enhanced concentration of SOD and APX and decreased level of malondialdehyde (MDA) compared to the untreated lot (Murali et al., 2021b).
Accumulation of proline is one of the common mechanisms involved in imparting drought tolerance. Proline helps to maintain protein structure and activity thereby supporting membrane integrity (Kishor et al., 2005). Also, proline has multiple roles as it can act as a chelator of metals, a signaling molecule, and a defense molecule triggering the production of a series of antioxidants (Hayat et al., 2012). It has been observed that Bacillus subtilis (HAS31), Pseudomonas strains apart from increasing antioxidants (SOD, CAT and POD) activity also significantly enhanced the total soluble sugars and proline in potato and sweet corn (Batool et al., 2020; Zarei et al., 2020).
PGPRs have an inherent capability to produce plant growth hormones like auxin, gibberellins (GA), JA, salicylic acid (SA) and ABA. These hormones may be directly responsible for triggering a series of responses in the host (plant) to combat stress conditions. The role of SA in imparting drought stress has been illustrated to alter nitrogen metabolism, triggering the production of antioxidants and accumulation of glycine betaine thus conferring protection against stress (Khan et al., 2022). An interesting research showed that under heat-stress conditions inoculation of Bacillus tequilensis SSB07 strain in soybean enhanced the endogenous level of JA, SA, and reduced the level of ABA content (Kang et al., 2019a). All the treated plants had better biomass and photosynthetic pigment compared to the control. Two PGPR strains Bacillus sp. WM13-24 and Pseudomonas sp. M30-35, promoted the growth of ryegrass subjected to drought stress by modulating auxin distribution and ABA content in the plant (He et al., 2021).
Several scientific investigations illustrated that microbial applications induce the expression of specific genes related to drought response. Treating Arabidopsis and soybean with Paenibacillus polymyxa CR1 upregulated dehydration-responsive genes (RD29A and RD29B), which equipped the plants to face drought conditions (Liu et al., 2020). Similarly, application of Bacillus subtilis strain GOT9 triggered up-regulation of numerous drought stress related genes particularly Response-to-desiccation 29B, 20 (RD29B, RD20), RAB18 (encodes dehydrin protein), 9-cis- epoxycarotenoid dioxygenase (NCED3)) in Arabidopsis and BrDREB1D, BrWRKY7 and BraCSD3 in Brassica thereby minimizing the physiological damage. Enhanced expression of ABA inducible genes clearly showed that GOT9 increased ABA accumulation in plant and hence provided drought tolerance (Woo et al., 2020). Inoculation of Pseudomonas putida GAP-P45 in Arabidopsis modulated several important polyamine biosynthetic genes (arginine decarboxylase (ADC), agmatine iminohydrolase (AIH), N- carbamoyl putrescine amidohydrolase (CPA), spermidine synthase (SPDS), spermine synthase (SPMS) and S-adenosylmethionine decarboxylase (SAMDC) thereby impacting cellular polyamine levels. The increased level of free cellular spermidine and putrescine positively correlated with water stress (Sen et al., 2018). In transgenic Arabidopsis overexpressing ABA stress ripening 6 (OsASR6) (auxin activated) gene increased the expression of auxin-responsive genes, small auxin up-regulated family (SAUR32), Ser/Thr protein kinase (PINOID), and auxin response factor 5 (ARF5) auxin transcription factor leading to greater root density and biomass. These effects of OsASR6 expression were found to mimic the beneficial effects of PGPRs in rice (Agarwal et al., 2019). PGPR strain Streptomyces mitigated drought stress in tomato and regulated the expression of transcription factors ethylene response factor 1 (ERF1) and WRKY70 [Figure 1B; (Abbasi et al., 2020)]. In another study, expression of ABA independent genes, i.e. drought-responsive element binding protein (DREB2 and DREB1-2) significantly enhanced due to PGPR (Bacillus sp.) inoculation in Brassica under water scarcity indicating Bacillus-mediated priming for drought tolerance (Bandeppa et al., 2019). Pepper treated with B. licheniformis K11 upregulated genes Cadhn, VA, sHSP and CaPR10 leading to higher production of dehydrin-like protein, vacuolar H+-ATPase, small heat shock protein and pathogenesis-related protein which helped in in survival of plants under severe drought conditions (Lim and Kim, 2013). An interesting study was carried out wherein it was elucidated that Pseudomonas putida modulates the expression of important stress-responsive miRNAs in response to drought and salt stresses (Jatan et al., 2019).
All these studies illustrate that PGPRs influence expression of heat/drought related genes there by triggering production of series of antioxidants, osmolytes, proline and several key biomolecules which may contribute in mitigating heat and drought stress. Also, several hormones produced by PGPRs as illustrated in Figure 1A trigger cascade of biochemical reaction in host which enable plants to tie over drought stress.
Cold stress
Low temperatures limit agriculture productivity in temperate ecosystems. In these areas, the plants constantly face chilling stress that leads to 51-82% annual yield loss (Hwarari et al., 2022). It generally affects the critical processes of plants such as ROS homeostasis, energy metabolism (electron transport chain), photosynthesis efficiency, cell wall structure, fluidity, root hydraulic conductance and structure of biomolecules (enzymes, proteins and nucleic acids) (Kazemi-Shahandashti and Maali-Amiri, 2018; Tiryaki et al., 2018; Zhou et al., 2021). In order to survive under prolonged low temperatures, the plants exhibit altered gene and transcription factor expression, leading to changes in membrane lipids, proteins, osmolytes levels, phytohormones, phenolic content and reactive oxygen scavenging enzymes (Brüggemann et al., 1999; Saltveit, 2000; Ait Barka et al., 2006; Amini et al., 2021; Guo et al., 2021; Ritonga et al., 2021; Saleem et al., 2021a; Eom et al., 2022; Hwarari et al., 2022; Wei et al., 2022).
The application of PGPR to improve crop productivity is a sustainable and safer means compared to chemical inputs. It has been found that treatment of Burkholderia phytofirmans strain PsJN (Bp PsJN) on Arabidopsis thaliana subjected to cold stress prevented disruption of plasmalemma, exhibited cell wall strengthening of mesophyll cells (Su et al., 2015). Inoculation of Vitis vinifera with PGPR strain PsJN exhibited better CO2 fixation, increased content of phenolics, proline and starch (Ait Barka et al., 2006). In another study, PsJN improved cold tolerance in Vitis vinifera wherein higher accumulation of proline, MDA and aldehydes (ALD) were observed along with higher expression of phenylalanine ammonia lyase (PAL) and stilbene synthase (STS) genes in primed plants compared to non-treated plants (Theocharis et al., 2012). Similarly, another finding revealed that under chilling stress treatment of tomato plants with psychrotolerant Pseudomonas vancouverensis (OB155) and P. frederiksbergensis (OS261) microbes minimized the stress effect by increasing the level of proline, antioxidant enzymes [(SOD, APX and glutathione (GSH)] (Subramanian et al., 2016). Further, Pseudomonas fragi, P. chloropaphis, P. fluorescens and Brevibacterium frigoritolerans inoculants also improved the growth of beans by regulating the activities of SOD, CAT, POX, APX and GR during low temperature stress [Figure 1A; (Tiryaki et al., 2018]. Subsequently, further research reflected the key role of Bacillus spp. in wheat under cold stress. Under cold stress, the bacterial treatment significantly reduced the level of ABA, ET, MDA by directly targeting the ABA- response element (ABARE), Ethylene response factor (ERF) and 4-Hydroxy-2-nonenal (4-HNE; encodes α, β- unsaturated aldehyde during lipid peroxidation) genes, but at the same time the expression of Δ1- pyrroline-5-carboxylate synthase (P5CS), expansin (expA1), cytokinin (CKX2) and auxin (ARF) increased (Zubair et al., 2019). Another study evaluated the role of Rhizobium inoculation (RI) in legumes model plant i.e. Medicago truncatula against cold stress. Compared to the control plant, the treated plant showed a significant increase in the SOD, CAT, APX, ascorbate, reduced glutathione, proline, soluble sugars and glycine betaine, whereas POD, lipoxygenase (LOX) activity and nitro-oxidative damage was notably reduced. Moreover, RI also stimulated the nitrogen (N) uptake in cold stress seedlings by enhancing the activity of nitrate reductase (NR) enzyme (Irshad et al., 2021). In addition, Streptomyces sp. 506 (TOR3209) played a distinct function in boosting tolerance to cold stress in tomato. Expression profile of TOR3209 treated stressed plants showed an increased level of HY5 (bZIP) mediated ABA signaling genes [(zeaxanthin epoxidase (ZEP1), 9-cis-epoxycarotenoid dioxygenase (NCED1), carotenoid dioxygenase and carotene beta-hydroxylase), dehydrin (TASI4) (that triggers accumulation of soluble sugars, proline). Besides this, TOR3209 also reduced the photosynthetic damage by modulating the activities of RUBISCO (Ribulose 1, 5- bisphosphate carboxylase/oxygenase), NAD-MDH and NADP-MDH (malate dehydrogenases) enzymes suggesting that main mechanism of imparting cold tolerance is by ABA pathway (Ma et al., 2022). Over and above, other finding highlights the role of cold active PGPR in rice growth and development. It was observed that strains of Pseudomonas, Enterobacter, Stenotrophomonas genera inoculation ameliorated the effect of cold in rice by increasing the accumulation of metabolites (proline and soluble sugars), protein content, nutrients (N, P and K), antioxidants (SOD, POD and CAT) (Expósito et al., 2022).
These studies illustrate that most of the cold tolerance by PGPRs are due to cross-interlinkage of antioxidants, soluble sugars, proteins, proline, phenolics, phytohormones etc. Further scientific investigations will provide better insight into PGPR mediated cold tolerance in plants but nevertheless, there are credential evidences which indicate that the right microbial strains can boost productivity in the temperate ecosystem.
Salinity stress
High salt concentration leads to osmotic stress that interferes with physiology, biochemical functioning (photosynthesis, stomatal conductance, enzyme activities, water and nutrient uptake), growth and yield of crops (Yasmin et al., 2021b; Choudhary et al., 2022). Microbial application triggers various mechanisms for improving plant growth under salinity stress (Sarkar et al., 2018). It generally includes the production of ACC-deaminase, EPS, phytohormones (auxin, CK and SA), antioxidant enzymes, VOCs, synthesis of osmoprotectant metabolites (proline, trehalose, alanine, glycine, glutamic acid, serine, threonine, aspartate, choline, betaine and organic acids), regulation of ion affinity transporters that in turn maintains ionic, osmotic, water homeostasis, thus resulting in improved plant growth under salt stress [Figure 1A; (Tewari and Arora, 2018; Abbas et al., 2019; Kumar et al., 2019; Bhat et al., 2020; Sunita et al., 2020; Choudhary et al., 2022; Gamalero and Glick, 2022; Kumawat et al., 2022)].
PGPR based compounds modulate the phytohormones, antioxidants and osmolytes levels in plants for their growth under stress conditions (Choudhary et al., 2022). A study demonstrated that under salt stress Rhodopseudomonas palustris G5 treated cucumber seedlings showed higher expression of SOD, POD, PPO and soluble sugars (Ge and Zhang, 2019) compared to untreated plants. Another ACC- deaminase producing endophytic strain Pseudomonas spp. OFT5 confers salt tolerance to tomato by reducing ethylene production (Win et al., 2018). Inoculating paddy with halotolerant Curtobacterium albidum strain SRV4 improved plant growth under various salinity levels by significantly increasing SOD, CAT, POX, APX expression, and by maintaining Na+/K+ homeostasis. This study showed that EPS production by strain reduced sodium ions availability to the plant and hence overcome effect of salinity stress (Vimal et al., 2019). Furthermore, Planomicrobium sp. MSSA-10 regulated antioxidants, phenolics and nutrients mobilization pathways in pea and promoted its growth in saline conditions. It was observed that bio-inoculant treatment increased total phenolics, POD, CAT and nutrients (N, P and K) uptake for reducing negative effects of salt [Figure 1B; (Shahid et al., 2018)]. Additionally, it was observed that salt tolerant Bacillus pumilus FAB10 significantly decreased antioxidant enzymes like SOD, CAT, glutathione reductase (GR) activities, proline and MDA content in wheat at different salt concentrations (Ansari et al., 2019). Furthermore, endophytic bacteria Curtobacterium sp. SAK1 treatment reduced the effect of salt stress in soybean by lowering endogenous ABA, JA, ROS, PPO and POD levels, whereas glutathione (cellular antioxidant) concentrations were found to be higher (Khan et al., 2019c). Another research team observed that inoculation of soybean with five halotolerant strains i.e. Arthrobacter woluwensis (AK1), Microbacterium oxydans (AK2), Arthrobacter aurescens (AK3), Bacillus megaterium (AK4) and Bacillus aryabhattai (AK5) conferred salt tolerance by elevating the expression of SOD, glutathione synthase (GSH) and enhancing K+ uptake. Besides the antioxidants, microbial inoculation significantly reduced Na+ ion concentration, ABA level, but increased the expression of IAA related gene i.e. auxin resistant 1 (GmLAX3) and salt tolerant gene (Soybean salt tolerance 1) (GmST1) (Khan et al., 2019b). In addition to this, it was found that halotolerant bacteria Leclercia adecarboxylata MO1 improved tomato growth under salt stress by significantly increasing sugars (sucrose, glucose and fructose), organic acids (citric acid and malic acid), amino acids (serine, glycine, methionine and proline) and simultaneously decreasing endogenous ABA level (Kang et al., 2019b). Similarly, PGPR Pseudomonas PS01 imparted salt tolerance in Arabidopsis by modulating the expression of stress related genes. Results illustrate that PS01 inoculation in salt stressed plants increased expression of lipoxygenase (LOX2) (related to JA synthesis), while decreased APX2, GLY17 (ROS scavenging and detoxification). No significant change was observed in the expression of RD29A and RD29B (ABA signaling genes) (Chu et al., 2019). One more study figured out that in rice inoculation with halotolerant Glutamicibacter sp. YD01 increased the expression of antioxidants such as OsPOX1, OsFeSOD, OsGR2, abiotic stress related genes (OsWRKY1, OsDREB2), OsHKT1 (related to ionic balance) and downregulated OsERF1 (related to ethylene production), thus enhancing their tolerance to salt stress (Ji et al., 2020). In a different fascinating research it was found that Pseudomonas pseudoalcaligenes (SR16) and Bacillus subtilis (SR3) also provide salt stress tolerance to hydroponically grown soybean seedlings. The strain SR16 effectively reduced 100mM NaCl stress by increasing the total protein, proline content, and activities of various antioxidants (SOD, CAT, APX, POD, PAL and PPO) (Yasmin et al., 2020). Recent findings suggest that soil application of Kosakonia sacchari improved mung bean performance by reducing the level of oxidative stress markers such as proline, MDA, H2O2 content, antioxidants like APX, CAT, SOD and GR. In contrast, the level of antioxidant metabolites i.e. ascorbic acid and glutathione increases in the foliage of treated plants which in turn reduced the toxicity of NaCl (Shahid et al., 2021). Moreover, inoculation of salt-tolerant PGPR Acinetobacter johnsonii provided tolerance to maize by downregulating SOD, CAT, proline and MDA content. It was observed that rhizobacterial inoculation improved dehydrogenase, alkaline phosphatase, acid phosphatase, urease and enzyme activity in the soil. Improved microbes mediated soil enzyme activities plays an important role in balancing nutrient profile, plant growth under salt stress (Shabaan et al., 2022). Additional evidence demonstrates role of halotolerant Bacillus strains (NMCN-1 and LLCG23) in mitigating 200mM salinity stress in wheat. Application of both inoculants significantly downregulated the expression of ABA- response element (ABARE), 4-Hydroxy-2-nonenal (4-HNE), whereas the P5CS gene was found to be upregulated. This clearly illustrates that these halophilic microbes regulate key stress signaling pathways (ABA synthesis, MDA and proline production) that subsequently lowered the effect of stress (Ayaz et al., 2022). From the cited literature (Table 1), it is evident that PGPR could alleviate salt stress by improving soil health, nutrient uptake, hormone production, antioxidant activity, and stress- responsive genes.
From the above studies it is clear that bacterial production of ACC deaminase (lowers ethylene concentration), enhanced production of a range of antioxidants (scavenges ROS) and higher production of proline (signaling molecule) in stress affected plants are some of the common mechanisms operate in PGPR treated plants to enable them combat temperature, drought and salinity stress. Hence, application of PGPR based biostimulants with proven PGP traits could be an ecofriendly sustainable means to boost crop productivity under single or combined abiotic stress.
Role of PGPR-based biostimulants in imparting tolerance against disease (biotic stress)
Plant resistance against pathogens is generally based upon two mechanisms i.e. induced systemic resistance (ISR) and systemic acquired resistance (SAR). ISR is mainly mediated by beneficial microorganisms through root colonization, root immunity modulation and production of certain elicitors like siderophores, polysaccharides, VOCs, plant hormones, enzymes etc. whereas SAR is defined as the plant’s acquired or adaptive resistance (Olowe et al., 2020; Hamid et al., 2021). Both ISR and SAR resistance mechanism is effective against wide group of pests and pathogens (Vlot et al., 2021; Meena et al., 2022; Yu et al., 2022). Though numerous studies reported that PGPR modulates various physiological, biochemical and molecular processes in plants and helps their survival under pathogens attack (Olowe et al., 2020; Castiglione et al., 2021; Yu et al., 2022), but the core mechanism of action is not fully understood. The results obtained through systematic studies indicate that induction of resistance against multiple pathogens including virus, fungi, bacteria rely on combined mechanisms that may work simultaneously (Yu et al., 2022). It includes induction of specific defense response genes/enzymes like ROS scavengers/antioxidants such as (CAT, APX, guaiacol peroxidase (GPX), GR, POD and SOD), accumulation of phytohormones (JA, ET, SA, GA and auxin), glucanases, chitinases, sugars, osmolytes, pathogenesis related proteins (PR) and secondary metabolites which in turn are directly involved in controlling growth and proliferation of pathogen [Figures 1A, B; (Baxter et al., 2014; Pieterse et al., 2014; Conrath et al., 2015; Camejo et al., 2016; Li et al., 2016; Guo et al., 2019; Ebrahimi et al., 2020; Olowe et al., 2020; da Silva et al., 2021; Luo et al., 2022)].
From the wide group of PGPR, Bacillus sp. has been considered as an excellent agent for controlling pathogens attack in various plants such as tomato, banana, tobacco, rice, wheat, cucumber, watermelon, cotton (Saechow et al., 2018; Gamez et al., 2019; Wu et al., 2019; Fu et al., 2020; Jiao et al., 2020; Dimopoulou et al., 2021; Kazerooni et al., 2021; Luo et al., 2022). Bacillus amyloliquefaciens (SN13) is a bio-protective agent against Rhizoctonia solani (causative agent of sheath blight disease), which enhances defense response in the rice plants. The colonized plants showed alteration in phytohormone content (increased level of SA, ABA and GA), and MAPKinases (increased level of phospholipase D and serine-threonine protein kinases) signaling pathways that helped in controlling disease proliferation. Apart from these, SN13 treatment also modulated the production of secondary metabolites (quinazoline) and ROS regulators (arabitol, proline and mannitol, sugars like β-D-glucopyranose, fructopyranose, and myoinositol, ferric reducatse glutathione S-transferase and peroxidase precursor) (Srivastava et al., 2016). Moreover, cotton (Gossypium hirsutum) plants treated with blend of Bacillus spp. enhanced secretion of gossypol (allelochemical) and JA (defense related phytohormone) which in turn reduced larval feeding of Spodoptera exigua (beet armyworm). In addition, treated cotton plants exhibited increased expression of genes involved in synthesis of allelochemicals i.e. (+)-δ-cadinene synthase (CAD1 gene family, including Cad1- C1, Cad1-A, Cad1-C14 and Cdn-C3) and jasmonates (allene oxide synthase) (GhAOS), 13-lipoxygenase (GhLOX1) and 12-oxo-phytodienoicacid reductase 3 (GhOPR3 [Figure 1A; (Zebelo et al., 2016)]. Another rhizobacterium strain Bacillus amyloliquefaciens SQRT3 strongly inhibited tomato bacterial wilt disease (caused by Ralstonia solanacearum). The application of SQRT3 increased the expression of POD, PPO, stress marker genes like proteinase inhibitor 2 (PIN2) (related to JA pathway) and pathogenesis related protein-1a (PR-1a) (related to SA pathway) and Omsotin-like (related to ET pathway) (Chunyu et al., 2017). Likewise, Bacillus velezensis enhanced resistance of pepper plants against Botrytis cinerea BC1301 (causative agent of gray mold disease) by triggering antioxidants SOD, CAT, POD and SA- mediated defense signaling genes namely non-expressor of pathogenesis-related genes 1 (NPR1), pathogenesis related protein-1 (PR1) and peroxidase. However, no effect was observed in the expression of proteinase inhibitor 2 (PIN2) and (TIN1) genes (Jiang et al., 2018). In another study, anti-pathogenic role of Bacillus amyloliquefaciens Ba13 against tomato yellow leaf curl virus (TYLCV) was observed. It was found that inoculation of this beneficial microbe improved tomato growth by elevating the expression of systemic resistance related genes including pathogenesis related protein-1, 2, 3 (PR1, PR2 and PR3), chitinase, PAL, POD, PPO, and β-1,3-glucanase (Guo et al., 2019). Furthermore, an interesting work underline the effectiveness of Bacillus amyloliquefaciens YN201732 against tobacco powdery mildew disease (caused by Erysiphe cichoracearum). Results highlight that bacterial treatment inhibited pathogenic fungi growth over tobacco cultivar by increasing the expression of disease-related genes like non-expressor of pathogenesis-related genes 1 (NPR1), plant defensin 1.2 (PDF1.2), chitinase (chit) and PPO, whereas, no significant change was observed in POD and PAL activity (Jiao et al., 2020). Also, Enterobacter asburiae BQ9 imparted tolerance against tomato yellow leaf curl virus by enhancing the expression of antioxidant enzymes such as POD, CAT, PAL and SOD; defense-related genes i.e. pathogenesis related protein-1a, 1b (PR1a and PR1b) (Li et al., 2016). These findings illustrate that treatment with PGPR may activate biochemical and molecular changes to restrict pathogenic invasions in plants. Additionally, in Nicotiana tabacum cv. plants Peanibacillus lentimorbus B-30488 inoculation reduced cucumber mosaic virus (CMV) RNA accumulation by ~12 fold (91%). This ISR was linked with an increase in expression of stress related genes Brassinosteroid signaling kinase 1 (BR-SK1), RNA dependent RNA polymerase 2 (RdRP2), zinc finger – homeodomain (ZF-HD), pathogenesis related protein 1(PR1), β-1,3-glucanase (Gluc), asparagine synthetase (AsSyn), tetrahydrocannabinolic acid synthase (TCAS) and antioxidant enzyme (APX, GPX, SOD and CAT) (Kumar et al., 2016). Similarly, Peanibacillus lentimorbus B-30488 also provides resistance against southern blight (caused by Scelerotium rolfsii) disease in tomato. The treated plants showed alteration of the ET pathway by significantly suppressing 1-aminocyclopropane-1-carboxylate synthase (ACC synthase) and oxidase (ACO) enzymes, and antioxidant enzyme activities (APX, GPX and SOD); whereas systemic tolerance was associated with expression of pathogenesis –related protein -1, 2A, 4, 7 (PR1, PR2A, PR4 and PR7), CAT, chitinase (CHI3 and CHI9), β-1, 3- glucanase (GLU), calmodulin and PPO (Dixit et al., 2016). Another report elucidates the role of Pseudomonas aeruginosa in controlling fungus (Botrytis cinerea) infection in Brassica napus by inducing the expression of transcription factor (BnWRKY33),mitogen-activated protein kinase 3, 4 (BnMPK3 and BnMPK4) and pathogenesis related protein-1 and 4 (BnPR1 and BnPR4) (Monnier et al., 2018).
Apart from this, some PGPRs also impart resistance against pathogens by secreting anti-microbial compounds (Hamid et al., 2021; Ji et al., 2021). For example, different Bacillus strains such as Bacillus velezensis QST713, Bacillus velezensis (C2), Bacillus velezensis OEE1, Bacillus subtilis release antifungal compounds such as lipopeptides (fengycin, bacillomycin and surfactin), polyketides (bacillaene, macrolactin and difficidin), dipeptide bacilysin, antifungal VOCs (phenylethyl alcohol, benzeneacetic acid, benzaldehyde, 1-decene, tetradecane) and lytic enzymes (chitinase, protease and β-glucanase), 3-indolylacetonitrile and suppresses green mold (caused by Trichoderma aggressivum f. europaeum), Verticillium wilt disease (caused by Verticillium dahliae), Septoria tritici blotch (caused by Zymoseptoria tritici), in button mushroom, tomato, olive, wheat (Mejri et al., 2018; Pandin et al., 2018; Dhouib et al., 2019; Azabou et al., 2020). Moreover, a research illustrated that application of Herbaspirillum seropedicae (BAC) suppressed Xanthomonas euvesicatoria (Xe), a causative agent of bacterial spot disease in ‘Micro-Tom’ Solanum lycopersicum L by downscaling the concentration of various organic acids such as oxalic acid, succinic acid, citric acid that enhances pathogens virulence (da Silva et al., 2021). The compiled studies (Table 2) clearly illustrate that PGPRs help in imparting tolerance against pathogen by activating pathway(s) that leads to production of array of defense related metabolites in plants.
Developing stress resilient crops
To full fill the goal of providing nutritious food for an increasing population, it is critical to develop resilient crops which can withstand the pressure of climate change. Scientists are strategizing, investigating, and discovering key genes which can aid in developing new transgenic crops tolerant to biotic and abiotic stresses without compromising on productivity. Wheat and rice are major staple crops grown across different regions of the world. Hence a lot of efforts are being made to develop better varieties through the classical breeding approach and newer biotechnological tools. The transgenic approach is one of the common techniques for inserting a gene of interest to achieve the desired trait. Among the transgenic approach, one of the studies illustrated that mutated transcription factor (HaHB4) from sunflower belonging to homeodomain-leucine zipper family (HD-Zip) improved water use efficiency and productivity of wheat (González et al., 2019). In another study introduction of beta gene-encoding choline dehydrogenase enhanced the glycine betaine content making the transformed wheat tolerant to drought (He et al., 2011). Similarly, in rice introduction of transcription factor PeSNAC-1 lead to increased production of proline, thereby making the plant tolerant to salinity and drought (Hou et al., 2020). Also, insertion of gene MYB49 lead to increased POD, SOD activity, chlorophyll content offering better resistance to drought, salinity and pathogen Phytophthora infestans in tomato (Cui et al., 2018). The transcription factor, SlDREB3 increased membrane stability and prevented ROS from imparting tolerance to chilling (Wang et al., 2019). The above studies clearly suggest that modifications at the genetic level either by inserting specific genes; altering transcription factor families like WRKY, DREB, MYB, NAC and ERF and modifying signal transduction genes significantly enhances the tolerance of plants to various stresses (Khan et al., 2019d). Similar physiological changes can be introduced in target plants by applying appropriate PGPRs (Tables 1, 2 and Figure 2). In wheat, over expression of TaWRKY2 (drought stress tolerance gene) improved drought tolerance by withholding water for 8-10 days before re-watering and enhancing proline content compared to wild type plant (Gao et al., 2018). Similarly, application of Azospirillum lipoferum significantly augmented the proline content in wheat seedling resulting in higher drought tolerance by withholding water for 10 days before watering (Kanwal et al., 2017). Engineering of E. coli cold shock protein (CspA and CspB) genes to convert it into plant-preferred codon namely SeCspA and SeCspB resulted in better stress tolerance potential by lowering MDA content, preventing water loss, reduced Na+ level and higher levels of chlorophyll, proline content under drought and salt stresses (200 mM NaCl) compared to the control wheat plants (Yu et al., 2017), similar response was evoked by endophytic strain Bacillus subtilis in wheat wherein lower MDA level was observed in plants grown under (340 mM NaCl) compared to control counter parts (Lastochkina et al., 2017). Cloning of Arabidopsis WRKY30 (AtWRKY30) transcription factor followed by its over-expression in wheat seedling subjected to drought stress by with-holding water for 12 days exhibited enhanced activities of antioxidant enzymes CAT, SOD, POX and APX (El-Esawi et al., 2019). Similar observation was made by (Akhtar et al., 2021) wherein antioxidant enzyme namely SOD, CAT and POX increased upon treating wheat with Bacillus sp., Azospirillum lipoferum and Azospirillum brasilense and subjected to drought stress by withholding water to 40% field capacity. Overexpression of TaFER-5B improved multiple stress including heat stress tolerance in wheat induced by keeping 10 days old seedling under 40°C, the protective mechanism was attributed to ROS scavenging activity (Zang et al., 2017). Similarly, modulation in expression of heat shock proteins (HSPs) was noticed in wheat seeds primed with Bacillus safensis. The treated plants tolerated heat shock (40°C) without generation of excessive ROS (Sarkar et al., 2021). Introduction of HVA1 (ABA-responsive barley gene) in wheat improved water use efficiency subjected to drought stress in wheat (Sivamani et al., 2000). Similarly, application of bacteria based bioformulation improved relative water content and range of antioxidants of wheat seedlings experiencing drought stress (40% the field capacity) (Akhtar et al., 2021). Several scientific studies conclusively indicate that applications of PGPRs could lead to development of resilient plants tolerant to sudden fluctuations in the weather, rise in temperature, salinity, drought, disease and insect attack. The association between plant and rhizospheric soil microbes is a functionally dynamic association and changes in the environment are perceived even at miniscule level triggering a cascade of appropriate stress-related responses making the plant tolerant to perceived challenges. In spite of beneficial attribute of transgenic crops, their acceptability for human consumption is still an issue of concern. Hence, knowledge-based application of the right PGPR could be a sustainable and ecofriendly approach to develop stress resilient crops.
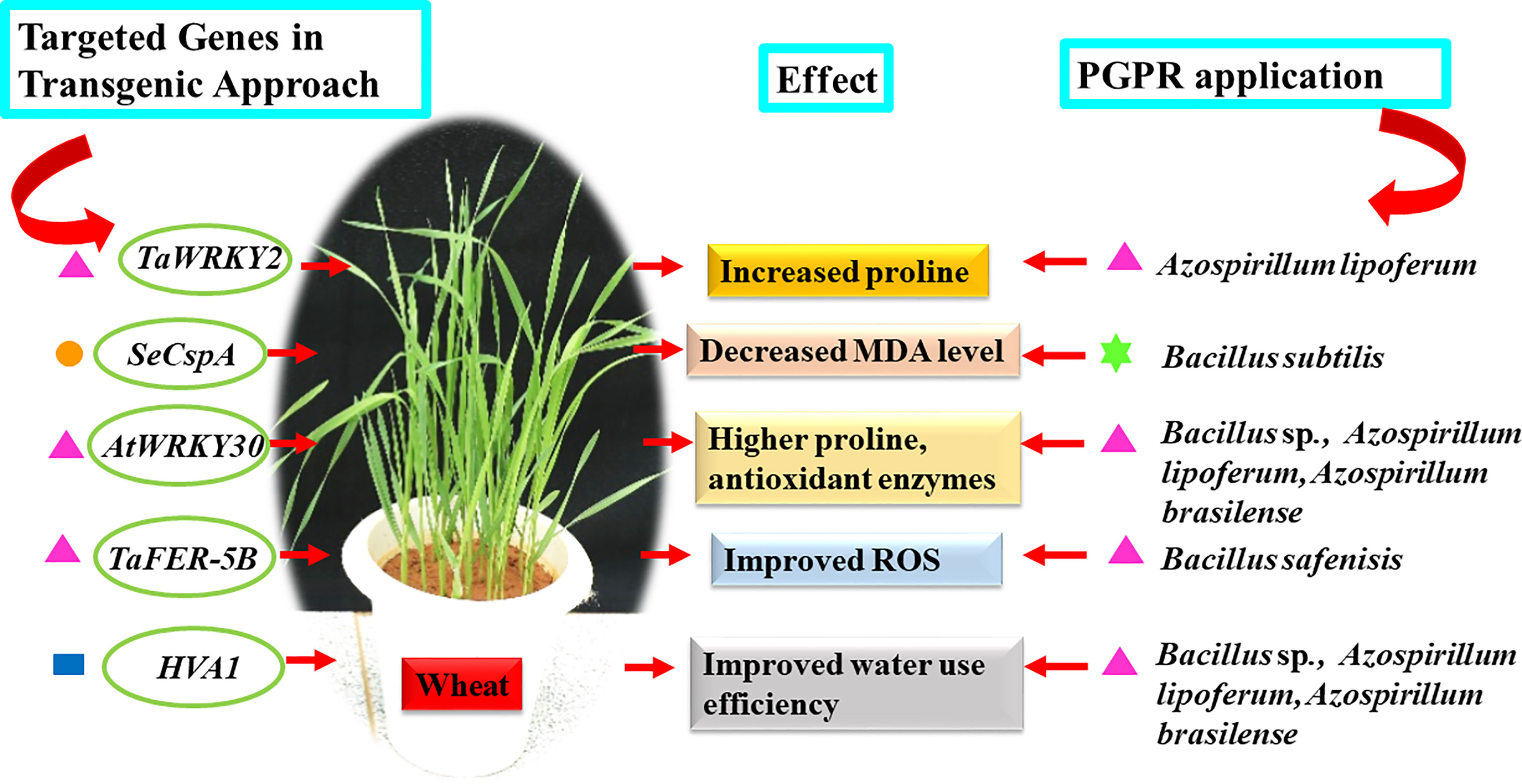
Figure 2 Model representing common physiological responses observed in wheat crop via transgenic approach and PGPRs application. Different colored symbols in the figure indicates the conditions of the experiment (Pots,
Filter Paper,
Hydroponics,
Greenhouse potting mix).
Conclusion
A sustainable agriculture system strives to protect the environment, without compromising crop yield to provide sufficient food for the growing population. In order to provide adequate food for all, approaches like conventional breeding and genetic engineering have been extensively used for crop improvement. But these processes are costly, tedious, labor intensive and raise safety issues. Further, to improve crop productivity, heavy doses of chemical fertilizers, pesticides, and fungicides have been rampantly used which lead to large scale deterioration of the environment and soil health ultimately impacting human health. Hence, ecofriendly sustainable means are being explored to increase plant productivity and in this respect application of microbial based biostimulants in agriculture is one of the promising means. PGPR modulates physiological responses in crops and equips them to survive under abiotic and biotic stresses. The majority of these responses are common to both biotic and abiotic challenges implying that the impact of PGPR in plant systems is broad-based, interaction is multifarious and beneficial in more than one way. Further studies are necessary to unravel the underlying mechanism of plant-microbe interactions and to understand the signal transduction network in an integrated perspective. Holistic information about plant-PGPR functionality will pave the way for developing novel microbial based biostimulants for boosting crop yield for future generations in a sustainable manner.
Author contributions
PK designed, drafted, revised, finalized the original manuscript and prepared the figures; NA drafted and revised the manuscript. Structuring, reviewing, and editing were done by SS. Conceptualization and input in hormonal interaction component was done by PP. Conceptualization, reviewing and editing was done by AP. All authors contributed to the article and approved the submitted version.
Funding
This work was funded by DBT grant number GAP-0307.
Acknowledgments
The authors thank the Director, CSIR- Institute of Himalayan Bioresource Technology, Palampur (HP) India, for encouraging and providing R&D facilities. This manuscript represent the CSIR-IHBT communication number 5151.
Conflict of interest
The authors declare that the research was conducted in the absence of any commercial or financial relationships that could be construed as a potential conflict of interest.
Publisher’s note
All claims expressed in this article are solely those of the authors and do not necessarily represent those of their affiliated organizations, or those of the publisher, the editors and the reviewers. Any product that may be evaluated in this article, or claim that may be made by its manufacturer, is not guaranteed or endorsed by the publisher.
References
Abbasi, S., Sadeghi, A., Safaie, N. (2020). Streptomyces alleviate drought stress in tomato plants and modulate the expression of transcription factors ERF1 and WRKY70 genes. Sci. Hortic. 265, 109206. doi: 10.1016/j.scienta.2020.109206
Abbas, R., Rasul, S., Aslam, K., Baber, M., Shahid, M., Mubeen, F., et al. (2019). Halotolerant PGPR: A hope for cultivation of saline soils. J. King Saud Univ. Sci. 31, 1195–1201. doi: 10.1016/j.jksus.2019.02.019
Abd El-Daim, I. A., Bejai, S., Meijer, J. (2014). Improved heat stress tolerance of wheat seedlings by bacterial seed treatment. Plant Soil 379, 337–350. doi: 10.1007/s11104-014-2063-3
Abd El-Daim, I. A., Bejai, S., Meijer, J. (2019). Bacillus velezensis 5113 induced metabolic and molecular reprogramming during abiotic stress tolerance in wheat. Sci. Rep. 9, 16282. doi: 10.1038/s41598-019-52567-x
Agarwal, P., Singh, P. C., Chaudhry, V., Shirke, P. A., Chakrabarty, D., Farooqui, A., et al. (2019). PGPR-induced OsASR6 improves plant growth and yield by altering root auxin sensitivity and the xylem structure in transgenic Arabidopsis thaliana. J. Plant Physiol. 240, 153010. doi: 10.1016/j.jplph.2019.153010
Ahluwalia, O., Singh, P. C., Bhatia, R. (2021). A review on drought stress in plants: Implications, mitigation and the role of plant growth promoting rhizobacteria. Resources Environ. Sustainability 5, 100032. doi: 10.1016/j.resenv.2021.100032
Ait Barka, E., Nowak, J., Clément, C. (2006). Enhancement of chilling resistance of inoculated grapevine plantlets with a plant growth-promoting rhizobacterium, Burkholderia phytofirmans strain PsJN. Appl. Environ. Microbiol. 72, 7246–7252. doi: 10.1128/AEM.01047-06
Akbar, A., Han, B., Khan, A. H., Feng, C., Ullah, A., Khan, A. S., et al. (2022). A transcriptomic study reveals salt stress alleviation in cotton plants upon salt tolerant PGPR inoculation. Environ. Exp. Bot. 200, 104928. doi: 10.1016/j.envexpbot.2022.104928
Akhtar, N., Ilyas, N., Mashwani, Z. R., Hayat, R., Yasmin, H., Noureldeen, A., et al. (2021). Synergistic effects of plant growth promoting rhizobacteria and silicon dioxide nano-particles for amelioration of drought stress in wheat. Plant Physiol. Biochem. 166, 160–176. doi: 10.1016/j.plaphy.2021.05.039
Ali, B., Hafeez, A., Ahmad, S., Javed, M. A., Afridi, M. S., Dawoud, T. M., et al. (2022). Bacillus thuringiensis PM25 ameliorates oxidative damage of salinity stress in maize via regulating growth, leaf pigments, antioxidant defense system, and stress responsive gene expression. Front. Plant Sci. 13. doi: 10.3389/fpls.2022.921668
Ali, S. Z., Sandhya, V., Grover, M., Kishore, N., Rao, L. V., Venkateswarlu, B. (2009). Pseudomonas sp. strain AKM-P6 enhances tolerance of sorghum seedlings to elevated temperatures. Biol. Fertil. Soils 46, 45–55. doi: 10.1007/s00374-009-0404-9
Ali, S. Z., Sandhya, V., Grover, M., Linga, V. R., Bandi, V. (2011). Effect of inoculation with a thermotolerant plant growth promoting Pseudomonas putida strain AKMP7 on growth of wheat (Triticum spp.) under heat stress. J. Plant Interact. 6, 239–246. doi: 10.1080/17429145.2010.545147
Amini, S., Maali-Amiri, R., Kazemi-Shahandashti, S. S., López-Gómez, M., Sadeghzadeh, B., Sobhani-Najafabadi, A., et al. (2021). Effect of cold stress on polyamine metabolism and antioxidant responses in chickpea. J. Plant Physiol. 258, 153387. doi: 10.1016/j.jplph.2021.153387
Ansari, F. A., Ahmad, I., Pichtel, J. (2019). Growth stimulation and alleviation of salinity stress to wheat by the biofilm forming Bacillus pumilus strain FAB10. Appl. Soil Ecol. 143, 45–54. doi: 10.1016/j.apsoil.2019.05.023
Ansari, F. A., Jabeen, M., Ahmad, I. (2021). Pseudomonas azotoformans FAP5, a novel biofilm-forming PGPR strain, alleviates drought stress in wheat plant. Int. J. Sci. Environ. Technol. 18, 3855–3870. doi: 10.1007/s13762-020-03045-9
Aremu, A. O., Makhaye, G., Tesfay, S. Z., Gerrano, A. S., Du Plooy, C. P., Amoo, S. O. (2022). Influence of commercial seaweed extract and microbial biostimulant on growth, yield, phytochemical content, and nutritional quality of five Abelmoschus esculentus genotypes. Agronomy 12, 428. doi: 10.3390/agronomy12020428
Arshad, M., Shaharoona, B., Mahmood, T. (2008). Inoculation with Pseudomonas spp. containing ACC-deaminase partially eliminates the effects of drought stress on growth, yield, and ripening of pea (Pisum sativum l.). Pedosphere 18, 611–620. doi: 10.1016/S1002-0160(08)60055-7
Asghari, B., Khademian, R., Sedaghati, B. (2020). Plant growth promoting rhizobacteria (PGPR) confer drought resistance and stimulate biosynthesis of secondary metabolites in pennyroyal (Mentha pulegium l.) under water shortage condition. Sci. Hortic. 263, 109132. doi: 10.1016/j.scienta.2019.109132
Ayaz, M., Ali, Q., Jiang, Q., Wang, R., Wang, Z., Mu, G., et al. (2022). Salt tolerant Bacillus strains improve plant growth traits and regulation of phytohormones in wheat under salinity stress. Plants 11, 2769. doi: 10.3390/plants11202769
Ayed, S., Bouhaouel, I., Jebari, H., Hamada, W. (2022). Use of biostimulants: Towards sustainable approach to enhance durum wheat performances. Plants 11, 133. doi: 10.3390/plants11010133
Azabou, M. C., Gharbi, Y., Medhioub, I., Ennouri, K., Barham, H., Tounsi, S., et al. (2020). The endophytic strain Bacillus velezensis OEE1: An efficient biocontrol agent against verticillium wilt of olive and a potential plant growth promoting bacteria. Biol. Control 142, 104168. doi: 10.1016/j.biocontrol.2019.104168
Backer, R., Rokem, J. S., Ilangumaran, G., Lamont, J., Praslickova, D., Ricci, E., et al. (2018). Plant growth-promoting rhizobacteria: Context, mechanisms of action, and roadmap to commercialization of biostimulants for sustainable agriculture. Front. Plant Sci. 9. doi: 10.3389/fpls.2018.01473
Baltazar, M., Correia, S., Guinan, K. J., Sujeeth, N., Bragança, R., Gonçalves, B. (2021). Recent advances in the molecular effects of biostimulants in plants: An overview. Biomolecules 11, 1096. doi: 10.3390/biom11081096
Bandeppa, S., Paul, S., Thakur, J. K., Chandrashekar, N., Umesh, D. K., Aggarwal, C., et al. (2019). Antioxidant, physiological and biochemical responses of drought susceptible and drought tolerant mustard (Brassica juncea l) genotypes to rhizobacterial inoculation under water deficit stress. Plant Physiol. Biochem. 143, 19–28. doi: 10.1016/j.plaphy.2019.08.018
Bano, A., Fatima, M. (2009). Salt tolerance in Zea mays (L). following inoculation with Rhizobium and Pseudomonas. Biol. Fertil. Soils 45, 405–413. doi: 10.1007/s00374-008-0344-9
Batool, T., Ali, S., Seleiman, M. F., Naveed, N. H., Ali, A., Ahmed, K., et al. (2020). Plant growth promoting rhizobacteria alleviates drought stress in potato in response to suppressive oxidative stress and antioxidant enzymes activities. Sci. Rep. 10, 1–19. doi: 10.1038/s41598-020-73489-z
Baxter, A., Mittler, R., Suzuki, N. (2014). ROS as key players in plant stress signalling. J. Exp. Bot. 65, 1229–1240. doi: 10.1093/jxb/ert375
Bharti, N., Pandey, S. S., Barnawal, D., Patel, V. K., Kalra, A. (2016). Plant growth promoting rhizobacteria Dietzia natronolimnaea modulates the expression of stress responsive genes providing protection of wheat from salinity stress. Sci. Rep. 6, 34768. doi: 10.1038/srep34768
Bhat, M. A., Kumar, V., Bhat, M. A., Wani, I. A., Dar, F. L., Farooq, I., et al. (2020). Mechanistic insights of the interaction of plant growth-promoting rhizobacteria (PGPR) with plant roots toward enhancing plant productivity by alleviating salinity stress. Front. Microbiol. 11 1952. doi: 10.3389/fmicb.2020.01952
Brüggemann, W., Beyel, V., Brodka, M., Poth, H., Weil, M., Stockhaus, J. (1999). Antioxidants and antioxidative enzymes in wild-type and transgenic Lycopersicon genotypes of different chilling tolerance. Plant Sci. 140, 145–154. doi: 10.1016/S0168-9452(98)00220-9
Camejo, D., Guzmán-Cedeño, Á., Moreno, A. (2016). Reactive oxygen species, essential molecules, during plant–pathogen interactions. Plant Physiol. Biochem. 103, 10–23. doi: 10.1016/j.plaphy.2016.02.035
Castiglione, A. M., Mannino, G., Contartese, V., Bertea, C. M., Ertani, A. (2021). Microbial biostimulants as response to modern agriculture needs: Composition, role and application of these innovative products. Plants 10, 1533 doi: 10.3390/plants10081533
Cataldo, E., Fucile, M., Mattii, G. B. (2022). Biostimulants in viticulture: A sustainable approach against biotic and abiotic stresses. Plants 11, 162. doi: 10.3390/plants11020162
Caulier, S., Nannan, C., Gillis, A., Licciardi, F., Bragard, C., Mahillon, J. (2019). Overview of the antimicrobial compounds produced by members of the Bacillus subtilis group. Front. Microbiol. 10. doi: 10.3389/fmicb.2019.00302
Chakraborty, U., Chakraborty, B., Dey, P., Chakraborty, A., Sarkar, J. (2019). Biochemical responses of wheat plants primed with Ochrobactrum pseudogrignonense and subjected to salinity stress. Agric. Res. 8, 427–440. doi: 10.1007/s40003-018-0394-7
Chandra, D., Srivastava, R., Glick, B. R., Sharma, A. K. (2018). Drought-tolerant Pseudomonas spp. improve the growth performance of finger millet (Eleusine coracana (L.) Gaertn.) under non-stressed and drought-stressed conditions. Pedosphere 28, 227–240. doi: 10.1016/S1002-0160(18)60013-X
Chiappero, J., del Rosario Cappellari, L., Alderete, L. G. S., Palermo, T. B., Banchio, E. (2019). Plant growth promoting rhizobacteria improve the antioxidant status in Mentha piperita grown under drought stress leading to an enhancement of plant growth and total phenolic content. Ind. Crop Prod. 139, 111553. doi: 10.1016/j.indcrop.2019.111553
Cho, S., Park, J., Han, S., Anderson, A., Yang, K.-Y., Gardener, B., et al. (2011). Identification and transcriptional analysis of priming genes in Arabidopsis thaliana induced by root colonization with Pseudomonas chlororaphis O6. Plant Pathol. J. 27, 272–279. doi: 10.5423/PPJ.2011.27.3.272
Choudhary, M., Chandra, P., Dixit, B., Nehra, V., Choudhary, U., Choudhary, S. (2022). Plant growth-promoting microbes: Role and prospective in amelioration of salt stress. Commun. Soil Sci. Plant Anal. 53, 1692–1711. doi: 10.1080/00103624.2022.2063316
Chunyu, L. I., Weicong, H. U., Bin, P. A. N., Yan, L. I. U., Saifei, Y. U. A. N., Yuanyuan, D. I. N. G., et al. (2017). Rhizobacterium Bacillus amyloliquefaciens strain SQRT3-mediated induced systemic resistance controls bacterial wilt of tomato. Pedosphere 27, 1135–1146. doi: 10.1016/S1002-0160(17)60406-5
Chu, T. N., Tran, B. T. H., Van Bui, L., Hoang, M. T. T. (2019). Plant growth-promoting rhizobacterium Pseudomonas PS01 induces salt tolerance in Arabidopsis thaliana. BMC Res. Notes 12, 1–7. doi: 10.1186/s13104-019-4046-1
Colla, G., Rouphael, Y. (2020). Microalgae: New source of plant biostimulants. Agronomy 10, 1240 doi: 10.3390/agronomy10091240
Conrath, U., Beckers, G. J., Langenbach, C. J., Jaskiewicz, M. R. (2015). Priming for enhanced defense. Annu. Rev. Phytopathol. 53, 97–119. doi: 10.1146/annurev-phyto-080614-120132
Cruz de Carvalho, M. H. (2008). Drought stress and reactive oxygen species: production, scavenging and signaling. Plant Signal. Behav. 3, 156–165. doi: 10.4161/psb.3.3.5536
Cui, J., Jiang, N., Zhou, X., Hou, X., Yang, G., Meng, J., et al. (2018). Tomato MYB49 enhances resistance to Phytophthora infestans and tolerance to water deficit and salt stress. Planta 248, 1487–1503. doi: 10.1007/s00425-018-2987-6
Curá, J. A., Franz, D. R., Filosofía, J. E., Balestrasse, K. B., Burgueño, L. E. (2017). Inoculation with Azospirillum sp. and Herbaspirillum sp. bacteria increases the tolerance of maize to drought stress. Microorganisms 5, 41. doi: 10.3390/microorganisms5030041
Danish, S., Zafar-Ul-Hye, M., Hussain, S., Riaz, M., Qayyum, M. F. (2020). Mitigation of drought stress in maize through inoculation with drought tolerant ACC deaminase containing PGPR under axenic conditions. Pak. J. Bot. 52, 49–60. doi: 10.30848/PJB2020-1(7)
da Silva, A. P. S., Olivares, F. L., Sudré, C. P., Peres, L. E. P., Canellas, N. A., da Silva, R. M., et al. (2021). Attenuations of bacterial spot disease Xanthomonas euvesicatoria on tomato plants treated with biostimulants. Chem. Biol. Technol. Agric. 8, 1–9. doi: 10.1186/s40538-021-00240-9
Desoky, E. S. M., Saad, A. M., El-Saadony, M. T., Merwad, A. R. M., Rady, M. M. (2020). Plant growth-promoting rhizobacteria: Potential improvement in antioxidant defense system and suppression of oxidative stress for alleviating salinity stress in Triticum aestivum (L.) plants. Biocatal. Agric. Biotechnol. 30, 101878. doi: 10.1016/j.bcab.2020.101878
Dhouib, H., Zouari, I., Abdallah, D. B., Belbahri, L., Taktak, W., Triki, M. A., et al. (2019). Potential of a novel endophytic Bacillus velezensis in tomato growth promotion and protection against verticillium wilt disease. Biol. Control 139, 104092. doi: 10.1016/j.biocontrol.2019.104092
Dimopoulou, A., Theologidis, I., Benaki, D., Koukounia, M., Zervakou, A., Tzima, A., et al. (2021). Direct antibiotic activity of bacillibactin broadens the biocontrol range of Bacillus amyloliquefaciens MBI600. Msphere 6, e00376–e00321. doi: 10.1128/mSphere.00376-21
Dixit, R., Agrawal, L., Gupta, S., Kumar, M., Yadav, S., Chauhan, P. S., et al. (2016). Southern blight disease of tomato control by 1-aminocyclopropane-1-carboxylate (ACC) deaminase producing Paenibacillus lentimorbus b-30488. Plant Signal. Behav. 11, e1113363. doi: 10.1080/15592324.2015.1113363
Dreesen, F. E., De Boeck, H. J., Janssens, I. A., Nijs, I. (2012). Summer heat and drought extremes trigger unexpected changes in productivity of a temperate annual/biannual plant community. Environ. Exp. Bot. 79, 21–30. doi: 10.1016/j.envexpbot.2012.01.005
Drobek, M., Frąc, M., Cybulska, J. (2019). Plant biostimulants: Importance of the quality and yield of horticultural crops and the improvement of plant tolerance to abiotic stress–a review. Agronomy 9, 335. doi: 10.3390/agronomy9060335
Ebrahimi, S., Eini, O., Koolivand, D. (2020). Arbuscular mycorrhizal symbiosis enhances virus accumulation and attenuates resistance-related gene expression in tomato plants infected with Beet curly top Iran virus. J. Plant Dis. Prot. 127, 341–348. doi: 10.1007/s41348-020-00299-w
Egamberdieva, D., Davranov, K., Wirth, S., Hashem, A., Abd Allah, E. F. (2017). Impact of soil salinity on the plant-growth - promoting and biological control abilities of root associated bacteria. Saudi J. Biol. Sci. 24, 1601–1608. doi: 10.1016/j.sjbs.2017.07.004
El-Esawi, M. A., Al-Ghamdi, A. A., Ali, H. M., Ahmad, M. (2019). Overexpression of AtWRKY30 transcription factor enhances heat and drought stress tolerance in wheat (Triticum aestivum l.). Genes 10, E163. doi: 10.3390/genes10020163
Eom, S. H., Ahn, M. A., Kim, E., Lee, H. J., Lee, J. H., Wi, S. H., et al. (2022). Plant response to cold stress: Cold stress changes antioxidant metabolism in heading type kimchi cabbage (Brassica rapa l. ssp. pekinensis). Antioxidants 11, 700. doi: 10.3390/antiox11040700
Erice, G., Ruíz-Lozano, J. M., Zamarreño, Á.M., García-Mina, J. M., Aroca, R. (2017). Transcriptomic analysis reveals the importance of JA-ile turnover in the response of Arabidopsis plants to plant growth promoting rhizobacteria and salinity. Environ. Exp. Bot. 143, 10–19. doi: 10.1016/j.envexpbot.2017.08.006
Expósito, C. D. V., López, J.Á., Liu, J., Bao, N., Liang, J., Zhang, J. (2022). Development of a cold-active microbial compound biofertilizer on the improvement for rice (oryza sativa l.) tolerance at low-temperature. Rhizosphere 24, 100586. doi: 10.1016/j.rhisph.2022.100586
Fadiji, A. E., Babalola, O. O., Santoyo, G., Perazzolli, M. (2022). The potential role of microbial biostimulants in the amelioration of climate change-associated abiotic stresses on crops. Front. Microbiol. 12. doi: 10.3389/fmicb.2021.829099
Franzoni, G., Cocetta, G., Prinsi, B., Ferrante, A., Espen, L. (2022). Biostimulants on crops: Their impact under abiotic stress conditions. Horticulturae 8, 189. doi: 10.3390/horticulturae8030189
Fu, H. Z., Marian, M., Enomoto, T., Hieno, A., Ina, H., Suga, H., et al. (2020). Biocontrol of tomato bacterial wilt by foliar spray application of a novel strain of endophytic bacillus sp. Microbes Environ. 35, ME20078. doi: 10.1264/jsme2.ME20078
Gamalero, E., Glick, B. R. (2022). Recent advances in bacterial amelioration of plant drought and salt stress. Biology 11, 437. doi: 10.3390/biology11030437
Gamez, R., Cardinale, M., Montes, M., Ramirez, S., Schnell, S., Rodriguez, F. (2019). Screening, plant growth promotion and root colonization pattern of two rhizobacteria (Pseudomonas fluorescens Ps006 and Bacillus amyloliquefaciens Bs006) on banana cv. williams (Musa acuminata colla). Microbiol. Res. 220, 12–20. doi: 10.1016/j.micres.2018.11.006
Gao, H., Wang, Y., Xu, P., Zhang, Z. (2018). Overexpression of a WRKY transcription factor TaWRKY2 enhances drought stress tolerance in transgenic wheat. Front. Plant Sci. 9. doi: 10.3389/fpls.2018.00997
Ge, H., Zhang, F. (2019). Growth-promoting ability of Rhodopseudomonas palustris G5 and its effect on induced resistance in cucumber against salt stress. J. Plant Growth Regul. 38, 180–188. doi: 10.1007/s00344-018-9825-8
González, F. G., Capella, M., Ribichich, K. F., Curín, F., Giacomelli, J. I., Ayala, F., et al. (2019). Field-grown transgenic wheat expressing the sunflower gene HaHB4 significantly outyields the wild type. J. Exp. Bot. 70, 1669–1681. doi: 10.1093/jxb/erz037
Guo, Q., Li, Y., Lou, Y., Shi, M., Jiang, Y., Zhou, J., et al. (2019). Bacillus amyloliquefaciens Ba13 induces plant systemic resistance and improves rhizosphere microecology against tomato yellow leaf curl virus disease. Appl. Soil Ecol. 137, 154–166. doi: 10.1016/j.apsoil.2019.01.015
Guo, Q., Li, X., Niu, L., Jameson, P. E., Zhou, W. (2021). Transcription-associated metabolomic adjustments in maize occur during combined drought and cold stress. Plant Physiol. 186, 677–695. doi: 10.1093/plphys/kiab050
Gupta, S., Pandey, S. (2019). ACC deaminase producing bacteria with multifarious plant growth promoting traits alleviates salinity stress in French bean (Phaseolus vulgaris) plants. Front. Microbiol. 10. doi: 10.3389/fmicb.2019.01506
Gururani, M. A., Upadhyaya, C. P., Baskar, V., Venkatesh, J., Nookaraju, A., Park, S. W. (2013). Plant growth-promoting rhizobacteria enhance abiotic stress tolerance in Solanum tuberosum through inducing changes in the expression of ROS-scavenging enzymes and improved photosynthetic performance. J. Plant Growth Regul. 32, 245–258. doi: 10.1007/s00344-012-9292-6
Habib, S. H., Kausar, H., Saud, H. M. (2016). Plant growth-promoting rhizobacteria enhance salinity stress tolerance in okra through ROS-scavenging enzymes. BioMed. Res. Int. 2016, e6284547. doi: 10.1155/2016/6284547
Hamid, B., Zaman, M., Farooq, S., Fatima, S., Sayyed, R. Z., Baba, Z. A., et al. (2021). Bacterial plant biostimulants: A sustainable way towards improving growth, productivity, and health of crops. Sustain 13, 2856. doi: 10.3390/su13052856
Hayat, S., Hayat, Q., Alyemeni, M. N., Wani, A. S., Pichtel, J., Ahmad, A. (2012). Role of proline under changing environments: A review. Plant Signal. Behav. 7, 1456–1466. doi: 10.4161/psb.21949
Hendriksen, N. B. (2022). Microbial biostimulants–the need for clarification in EU regulation. Trends Microbiol. 30, 311–313. doi: 10.1016/j.tim.2022.01.008
He, A., Niu, S., Yang, D., Ren, W., Zhao, L., Sun, Y., et al. (2021). Two PGPR strains from the rhizosphere of Haloxylon ammodendron promoted growth and enhanced drought tolerance of ryegrass. Plant Physiol. Biochem. 161, 74–85. doi: 10.1016/j.plaphy.2021.02.003
He, C., Zhang, W., Gao, Q., Yang, A., Hu, X., Zhang, J. (2011). Enhancement of drought resistance and biomass by increasing the amount of glycine betaine in wheat seedlings. Euphytica 177, 151–167. doi: 10.1007/s10681-010-0263-3
Hou, D., Zhao, Z., Hu, Q., Li, L., Vasupalli, N., Zhuo, J., et al. (2020). PeSNAC-1 a NAC transcription factor from moso bamboo (Phyllostachys edulis) confers tolerance to salinity and drought stress in transgenic rice. Tree Physiol. 40, 1792–1806. doi: 10.1093/treephys/tpaa099
Hwarari, D., Guan, Y., Ahmad, B., Movahedi, A., Min, T., Hao, Z., et al. (2022). ICE-CBF-COR signaling cascade and its regulation in plants responding to cold stress. Int. J. Mol. Sci. 23, 1549. doi: 10.3390/ijms23031549
Irshad, A., Rehman, R. N. U., Kareem, H. A., Yang, P., Hu, T. (2021). Addressing the challenge of cold stress resilience with the synergistic effect of Rhizobium inoculation and exogenous melatonin application in Medicago truncatula. Ecotoxicol. Environ. Saf. 226, 112816. doi: 10.1016/j.ecoenv.2021.112816
Issa, A., Esmaeel, Q., Sanchez, L., Courteaux, B., Guise, J.-F., Gibon, Y., et al. (2018). Impacts of Paraburkholderia phytofirmans strain PsJN on tomato (Lycopersicon esculentum l.) under high temperature. Front. Plant Sci. 9. doi: 10.3389/fpls.2018.01397
Jatan, R., Chauhan, P. S., Lata, C. (2019). Pseudomonas putida modulates the expression of miRNAs and their target genes in response to drought and salt stresses in chickpea (Cicer arietinum l.). Genomics 111c, 509–519. doi: 10.1016/j.ygeno.2018.01.007
Jiang, C. H., Liao, M. J., Wang, H. K., Zheng, M. Z., Xu, J. J., Guo, J. H. (2018). Bacillus velezensis, a potential and efficient biocontrol agent in control of pepper gray mold caused by Botrytis cinerea. Biol. Control 126, 147–157. doi: 10.1016/j.biocontrol.2018.07.017
Jiao, R., Munir, S., He, P., Yang, H., Wu, Y., Wang, J., et al. (2020). Biocontrol potential of the endophytic Bacillus amyloliquefaciens YN201732 against tobacco powdery mildew and its growth promotion. Biol. Control 143, 104160. doi: 10.1016/j.biocontrol.2019.104160
Jin, N., Jin, L., Wang, S., Li, J., Liu, F., Liu, Z., et al. (2022). Reduced chemical fertilizer combined with bio-organic fertilizer affects the soil microbial community and yield and quality of lettuce. Front. Microbiol. 13. doi: 10.3389/fmicb.2022.863325
Ji, J., Yuan, D., Jin, C., Wang, G., Li, X., Guan, C. (2020). Enhancement of growth and salt tolerance of rice seedlings (Oryza sativa l.) by regulating ethylene production with a novel halotolerant PGPR strain Glutamicibacter sp. YD01 containing ACC deaminase activity. Acta Physiol. Plant 42, 1–17. doi: 10.1007/s11738-020-3034-3
Ji, C., Zhang, M., Kong, Z., Chen, X., Wang, X., Ding, W., et al. (2021). Genomic analysis reveals potential mechanisms underlying promotion of tomato plant growth and antagonism of soilborne pathogens by Bacillus amyloliquefaciens Ba13. Microbiol. Spectr. 9, e01615–e01621. doi: 10.1128/Spectrum.01615-21
Kang, S. M., Khan, A. L., Waqas, M., Asaf, S., Lee, K. E., Park, Y. G. (2019a). Integrated phytohormone production by the plant growth-promoting rhizobacterium Bacillus tequilensis SSB07 induced thermotolerance in soybean. J. Plant Interact. 14, 416–423. doi: 10.1080/17429145.2019.1640294
Kang, S. M., Shahzad, R., Bilal, S., Khan, A. L., Park, Y. G., Lee, K. E., et al. (2019b). Indole-3-acetic-acid and ACC deaminase producing Leclercia adecarboxylata MO1 improves Solanum lycopersicum l. growth and salinity stress tolerance by endogenous secondary metabolites regulation. BMC Microbiol. 19, 1–14. doi: 10.1186/s12866-019-1450-6
Kanwal, S., Ilyas, N., Batool, N., Arshad, M. (2017). Amelioration of drought stress in wheat by combined application of PGPR, compost, and mineral fertilizer. J. Plant Nutr. 40, 1250–1260. doi: 10.1080/01904167.2016.1263322
Kaya, C., Şenbayram, M., Akram, N. A., Ashraf, M., Alyemeni, M. N., Ahmad, P. (2020). Sulfur-enriched leonardite and humic acid soil amendments enhance tolerance to drought and phosphorus deficiency stress in maize (Zea mays l.). Sci. Rep. 10, 1–13. doi: 10.1038/s41598-020-62669-6
Kazemi-Shahandashti, S. S., Maali-Amiri, R. (2018). Global insights of protein responses to cold stress in plants: Signaling, defence, and degradation. J. Plant Physiol. 226, 123–135. doi: 10.1016/j.jplph.2018.03.022
Kazerooni, E. A., Maharachchikumbura, S. S., Adhikari, A., Al-Sadi, A. M., Kang, S. M., Kim, L. R., et al. (2021). Rhizospheric Bacillus amyloliquefaciens protects Capsicum annuum cv. geumsugangsan from multiple abiotic stresses via multifarious plant growth-promoting attributes. Front. Plant Sci. 12. doi: 10.3389/fpls.2021.669693
Khan, S., Anwar, S., Yu, S., Sun, M., Yang, Z., Gao, Z. Q. (2019d). Development of drought-tolerant transgenic wheat: Achievements and limitations. Int. J. Mol. Sci. 20, 3350. doi: 10.3390/ijms20133350
Khan, M. A., Asaf, S., Khan, A. L., Adhikari, A., Jan, R., Ali, S., et al. (2019b). Halotolerant rhizobacterial strains mitigate the adverse effects of NaCl stress in soybean seedlings. BioMed. Res. Int. 2019, 9530963. doi: 10.1155/2019/9530963
Khan, M. A., Asaf, S., Khan, A. L., Jan, R., Kang, S. M., Kim, K. M., et al. (2020). Thermotolerance effect of plant growth-promoting Bacillus cereus SA1 on soybean during heat stress. BMC Microbiol. 20, 175. doi: 10.1186/s12866-020-01822-7
Khan, M. A., Asaf, S., Khan, A. L., Ullah, I., Ali, S., Kang, S. M., et al. (2019c). Alleviation of salt stress response in soybean plants with the endophytic bacterial isolate curtobacterium sp. SAK1. Ann. Microbiol. 69, 797–808. doi: 10.1007/s13213-019-01470-x
Khan, M. I., Poor, P., Janda, T. (2022). Salicylic acid: A versatile signaling molecule in plants. J. Plant Growth Regul. 41, 1887–1890. doi: 10.1007/s00344-022-10692-4
Khan, A., Uddin, S., Zhao, X. Q., Javed, M. T., Khan, K. S., Bano, A., et al. (2016). Bacillus pumilus enhances tolerance in rice (Oryza sativa l.) to combined stresses of NaCl and high boron due to limited uptake of na+. Environ. Exp. Bot. 124, 120–129. doi: 10.1016/j.envexpbot.2015.12.011
Khan, M. A., Ullah, I., Waqas, M., Hamayun, M., Khan, A. L., Asaf, S., et al. (2019a). Halo-tolerant rhizospheric Arthrobacter woluwensis AK1 mitigates salt stress and induces physio-hormonal changes and expression of GmST1 and GmLAX3 in soybean. Symbiosis 77, 9–21. doi: 10.1007/s13199-018-0562-3
Kim, K., Jang, Y. J., Lee, S.-M., Oh, B. T., Chae, J. C., Lee, K. J. (2014). Alleviation of salt stress by Enterobacter sp. EJ01 in tomato and Arabidopsis is accompanied by up-regulation of conserved salinity responsive factors in plants. Mol. Cells 37, 109–117. doi: 10.14348/molcells.2014.2239
Kim, S. T., Yoo, S. J., Weon, H. Y., Song, J., Sang, M. K. (2022). Bacillus butanolivorans KJ40 contributes alleviation of drought stress in pepper plants by modulating antioxidant and polyphenolic compounds. Sci. Hortic. 301, 111111. doi: 10.1016/j.scienta.2022.111111
Kishor, P. K., Sangam, S., Amrutha, R. N., Laxmi, P. S., Naidu, K. R., Rao, K. S., et al. (2005). Regulation of proline biosynthesis, degradation, uptake and transport in higher plants: Its implications in plant growth and abiotic stress tolerance. Curr. Sci. 88, 424–438.
Kosar, F., Akram, N. A., Ashraf, M., Ahmad, A., Alyemeni, M. N., Ahmad, P. (2021). Impact of exogenously applied trehalose on leaf biochemistry, achene yield and oil composition of sunflower under drought stress. Physiol. Plant 172, 317–333. doi: 10.1111/ppl.13155
Kour, D., Yadav, A. N. (2022). Bacterial mitigation of drought stress in plants: Current perspectives and future challenges. Curr. Microbiol. 79, 1–19. doi: 10.1007/s00284-022-02939-w
Kumar, S., Chauhan, P. S., Agrawal, L., Raj, R., Srivastava, A., Gupta, S., et al. (2016). Paenibacillus lentimorbus inoculation enhances tobacco growth and extenuates the virulence of Cucumber mosaic virus. PLos One 11, e0149980. doi: 10.1371/journal.pone.0149980
Kumar, A., Patel, J. S., Meena, V. S., Srivastava, R. (2019). Recent advances of PGPR based approaches for stress tolerance in plants for sustainable agriculture. Biocatal. Agric. Biotechnol. 20, 101271. doi: 10.1016/j.bcab.2019.101271
Kumawat, K. C., Nagpal, S., Sharma, P. (2022). Potential of plant growth-promoting rhizobacteria-plant interactions in mitigating salt stress for sustainable agriculture: A review. Pedosphere 32, 223–245. doi: 10.1016/S1002-0160(21)60070-X
Lastochkina, O., Pusenkova, L., Yuldashev, R., Babaev, M., Garipova, S., Blagova, D., et al. (2017). Effects of Bacillus subtilis on some physiological and biochemical parameters of Triticum aestivum l. (wheat) under salinity. Plant Physiol. Biochem. 121, 80–88. doi: 10.1016/j.plaphy.2017.10.020
Laxa, M., Liebthal, M., Telman, W., Chibani, K., Dietz, K. J. (2019). The role of the plant antioxidant system in drought tolerance. Antioxidants 8, 94. doi: 10.3390/antiox8040094
Lephatsi, M. M., Meyer, V., Piater, L. A., Dubery, I. A., Tugizimana, F. (2021). Plant responses to abiotic stresses and rhizobacterial biostimulants: Metabolomics and epigenetics perspectives. Metabolites 11, 457. doi: 10.3390/metabo11070457
Li, H., Ding, X., Wang, C., Ke, H., Wu, Z., WANG, Y., et al. (2016). Control of tomato yellow leaf curl virus disease by Enterobacter asburiaeBQ9 as a result of priming plant resistance in tomatoes. Turkish J. Biol. 40, 150–159. doi: 10.3906/biy-1502-12
Lim, J. H., Kim, S. D. (2013). Induction of drought stress resistance by multi-functional PGPR Bacillus licheniformis K11 in pepper. Plant Pathol. J. 29, 201–208. doi: 10.5423/PPJ.SI.02.2013.0021
Lin, Y., Jones, M. L. (2022). Evaluating the growth-promoting effects of microbial biostimulants on greenhouse floriculture crops. HortScience 57, 97–109. doi: 10.21273/HORTSCI16149-21
Lipiec, J., Doussan, C., Nosalewicz, A., Kondracka, K. (2013). Effect of drought and heat stresses on plant growth and yield: A review. Int. Agrophys. 27, 463–477. doi: 10.2478/intag-2013-0017
Li, X., Sun, P., Zhang, Y., Jin, C., Guan, C. (2020). A novel PGPR strain Kocuria rhizophila Y1 enhances salt stress tolerance in maize by regulating phytohormone levels, nutrient acquisition, redox potential, ion homeostasis, photosynthetic capacity and stress-responsive genes expression. Environ. Exp. Bot. 174, 104023. doi: 10.1016/j.envexpbot.2020.104023
Liu, S., Hao, H., Lu, X., Zhao, X., Wang, Y., Zhang, Y., et al. (2017). Transcriptome profiling of genes involved in induced systemic salt tolerance conferred by Bacillus amyloliquefaciens FZB42 in Arabidopsis thaliana. Sci. Rep. 7, 1–13. doi: 10.1038/s41598-017-11308-8
Liu, W., Sikora, E., Park, S. W. (2020). Plant growth-promoting rhizobacterium, Paenibacillus polymyxa CR1, upregulates dehydration-responsive genes, RD29A and RD29B, during priming drought tolerance in arabidopsis. Plant Physiol. Biochem. 156, 146–154. doi: 10.1016/j.plaphy.2020.08.049
Luo, L., Zhao, C., Wang, E., Raza, A., Yin, C. (2022). Bacillus amyloliquefaciens as an excellent agent for biofertilizer and biocontrol in agriculture: An overview for its mechanisms. Microbiol. Res. 259, 127016. doi: 10.1016/j.micres.2022.127016
Mansoor, S., Kour, N., Manhas, S., Zahid, S., Wani, O. A., Sharma, V., et al. (2021). Biochar as a tool for effective management of drought and heavy metal toxicity. Chemosphere 271, 129458. doi: 10.1016/j.chemosphere.2020.129458
Ma, J., Peng, J., Tian, S., He, Y., Zhang, C., Jia, N., et al. (2022). Streptomyces sp. TOR3209 alleviates cold stress in tomato plants. N. Z. J. Crop Hortic. Sci., 1–21. doi: 10.1080/01140671.2022.2066141
Meena, M., Yadav, G., Sonigra, P., Nagda, A., Mehta, T., Swapnil, P., et al. (2022). Role of elicitors to initiate the induction of systemic resistance in plants to biotic stress. Plant Stress 5, 100103. doi: 10.1016/j.stress.2022.100103
Mejri, S., Siah, A., Coutte, F., Magnin-Robert, M., Randoux, B., Tisserant, B., et al. (2018). Biocontrol of the wheat pathogen Zymoseptoria tritici using cyclic lipopeptides from Bacillus subtilis. Environ. Sci. pollut. Res. 25, 29822–29833. doi: 10.1007/s11356-017-9241-9
Mekureyaw, M. F., Pandey, C., Hennessy, R. C., Nicolaisen, M. H., Liu, F., Nybroe, O., et al. (2022). The cytokinin-producing plant beneficial bacterium Pseudomonas fluorescens G20-18 primes tomato (Solanum lycopersicum) for enhanced drought stress responses. J. Plant Physiol. 270, 153629. doi: 10.1016/j.jplph.2022.153629
Miceli, A., Moncada, A., Vetrano, F. (2021). Use of microbial biostimulants to increase the salinity tolerance of vegetable transplants. Agronomy 11, 1143. doi: 10.3390/agronomy11061143
Mishra, P. K., Bisht, S. C., Ruwari, P., Selvakumar, G., Joshi, G. K., Bisht, J. K., et al. (2011). Alleviation of cold stress in inoculated wheat (Triticum aestivum l.) seedlings with psychrotolerant Pseudomonads from NW Himalayas. Arch. Microbiol. 193, 497–513. doi: 10.1007/s00203-011-0693-x
Mitra, D., Rodriguez, A. M. D., Cota, F. I. P., Khoshru, B., Panneerselvam, P., Moradi, S., et al. (2021). Amelioration of thermal stress in crops by plant growth-promoting rhizobacteria. Physiol. Mol. Plant Pathol. 115, 101679. doi: 10.1016/j.pmpp.2021.101679
Mokabel, S., Olama, Z., Ali, S., El-Dakak, R. (2022). The role of plant growth promoting rhizosphere microbiome as alternative biofertilizer in boosting Solanum melongena l. adaptation to salinity stress. Plants 11, 659. doi: 10.3390/plants11050659
Monnier, N., Furlan, A., Botcazon, C., Dahi, A., Mongélard, G., Cordelier, S., et al. (2018). Rhamnolipids from Pseudomonas aeruginosa are elicitors triggering Brassica napus protection against Botrytis cinerea without physiological disorders. Front. Plant Sci. 9. doi: 10.3389/fpls.2018.01170
Monteiro, E., Gonçalves, B., Cortez, I., Castro, I. (2022). The role of biostimulants as alleviators of biotic and abiotic stresses in grapevine: A review. Plants 11, 396. doi: 10.3390/plants11030396
Moreno-Galván, A. E., Cortés-Patiño, S., Romero-Perdomo, F., Uribe-Vélez, D., Bashan, Y., Bonilla, R. R. (2020). Proline accumulation and glutathione reductase activity induced by drought-tolerant rhizobacteria as potential mechanisms to alleviate drought stress in Guinea grass. Appl. Soil Ecol. 147, 103367. doi: 10.1016/j.apsoil.2019.103367
Mukhtar, T., Rehman, S. U., Smith, D., Sultan, T., Seleiman, M., Alsadon, A., et al. (2020). Mitigation of heat stress in Solanum lycopersicum l. by ACC-deaminase and exopolysaccharide producing Bacillus cereus: Effects on biochemical profiling. Sustain 12, 2159. doi: 10.3390/su12062159
Murali, M., Gowtham, H. G., Singh, S. B., Shilpa, N., Aiyaz, M., Niranjana, S. R., et al. (2021a). Bio-prospecting of ACC deaminase producing rhizobacteria towards sustainable agriculture: A special emphasis on abiotic stress in plants. Appl. Soil Ecol. 168, 104142. doi: 10.1016/j.apsoil.2021.104142
Murali, M., Singh, S. B., Gowtham, H. G., Shilpa, N., Prasad, M., Aiyaz, M., et al. (2021b). Induction of drought tolerance in Pennisetum glaucum by ACC deaminase producing PGPR-Bacillus amyloliquefaciens through antioxidant defense system. Microbiol. Res. 253, 126891. doi: 10.1016/j.micres.2021.126891
Naseem, H., Bano, A. (2014). Role of plant growth-promoting rhizobacteria and their exopolysaccharide in drought tolerance of maize. J. Plant Interact. 9, 689–701. doi: 10.1080/17429145.2014.902125
Noor, R., Yasmin, H., Ilyas, N., Nosheen, A., Hassan, M. N., Mumtaz, S., et al. (2022). Comparative analysis of iron oxide nanoparticles synthesized from ginger (Zingiber officinale) and cumin seeds (Cuminum cyminum) to induce resistance in wheat against drought stress. Chemosphere 292, 133201. doi: 10.1016/j.chemosphere.2021.133201
Notununu, I., Moleleki, L., Roopnarain, A., Adeleke, R. (2022). Effects of plant growth-promoting rhizobacteria on the molecular responses of maize under drought and heat stresses: A review. Pedosphere 32, 90–106. doi: 10.1016/S1002-0160(21)60051-6
Olowe, O. M., Akanmu, A. O., Asemoloye, M. D. (2020). Exploration of microbial stimulants for induction of systemic resistance in plant disease management. Ann. Appl. Biol. 177, 282–293. doi: 10.1111/aab.12631
Orozco-Mosqueda, M., del, C., Glick, B. R., Santoyo, G. (2020). ACC deaminase in plant growth-promoting bacteria (PGPB): An efficient mechanism to counter salt stress in crops. Microbiol. Res. 235, 126439. doi: 10.1016/j.micres.2020.126439
Othibeng, K., Nephali, L., Myoli, A., Buthelezi, N., Jonker, W., Huyser, J., et al. (2022). Metabolic circuits in sap extracts reflect the effects of a microbial biostimulant on maize metabolism under drought conditions. Plants 11, 510. doi: 10.3390/plants11040510
Pandin, C., Le Coq, D., Deschamps, J., Védie, R., Rousseau, T., Aymerich, S., et al. (2018). Complete genome sequence of Bacillus velezensis QST713: A biocontrol agent that protects Agaricus bisporus crops against the green mould disease. J. Biotechnol. 278, 10–19. doi: 10.1016/j.jbiotec.2018.04.014
Park, Y. G., Mun, B. G., Kang, S. M., Hussain, A., Shahzad, R., Seo, C.-W., et al. (2017). Bacillus aryabhattai SRB02 tolerates oxidative and nitrosative stress and promotes the growth of soybean by modulating the production of phytohormones. PLos One 12, e0173203. doi: 10.1371/journal.pone.0173203
Pereira, S. I. A., Abreu, D., Moreira, H., Vega, A., Castro, P. M. L. (2020). Plant growth-promoting rhizobacteria (PGPR) improve the growth and nutrient use efficiency in maize (Zea mays l.) under water deficit conditions. Heliyon 6, e05106. doi: 10.1016/j.heliyon.2020.e05106
Pieterse, C. M., Zamioudis, C., Berendsen, R. L., Weller, D. M., Van Wees, S. C., Bakker, P. A. (2014). Induced systemic resistance by beneficial microbes. Annu. Rev. Phytopathol. 52, 347–375. doi: 10.1146/annurev-phyto-082712-102340
Pinedo, I., Ledger, T., Greve, M., Poupin, M. J. (2015). Burkholderia phytofirmans PsJN induces long-term metabolic and transcriptional changes involved in Arabidopsis thaliana salt tolerance. Front. Plant Sci. 6. doi: 10.3389/fpls.2015.00466
Rana, S., Chauhan, R., Walia, A., Sharma, G. D., Datt, N. (2021). Beneficial microbes in agriculture under abiotic stress conditions: An overview. Pharma Innov. 10, 360–368. doi: 10.22271/tpi.2021.v10.i1e.5542
Rashid, U., Yasmin, H., Hassan, M. N., Naz, R., Nosheen, A., Sajjad, M., et al. (2022). Drought-tolerant Bacillus megaterium isolated from semi-arid conditions induces systemic tolerance of wheat under drought conditions. Plant Cell Rep. 41, 549–569. doi: 10.1007/s00299-020-02640-x
Ritonga, F. N., Ngatia, J. N., Wang, Y., Khoso, M. A., Farooq, U., Chen, S. (2021). AP2/ERF, an important cold stress-related transcription factor family in plants: A review. Physiol. Mol. Biol. Plants 27, 1953–1968. doi: 10.1007/s12298-021-01061-8
Rouphael, Y., Colla, G. (2018). Synergistic biostimulatory action: Designing the next generation of plant biostimulants for sustainable agriculture. Front. Plant Sci. 9. doi: 10.3389/fpls.2018.01655
Rouphael, Y., Colla, G. (2020). Toward a sustainable agriculture through plant biostimulants: From experimental data to practical applications. Agronomy 10, 1461. doi: 10.3390/agronomy10101461
Saechow, S., Thammasittirong, A., Kittakoop, P., Prachya, S., Thammasittirong, S. N. R. (2018). Antagonistic activity against dirty panicle rice fungal pathogens and plant growth-promoting activity of Bacillus amyloliquefaciens BAS23. J. Microbiol. Biotechnol. 28, 1527–1535. doi: 10.4014/jmb.1804.04025
Saikia, J., Sarma, R. K., Dhandia, R., Yadav, A., Bharali, R., Gupta, V. K., et al. (2018). Alleviation of drought stress in pulse crops with ACC deaminase producing rhizobacteria isolated from acidic soil of northeast India. Sci. Rep. 8, 3560. doi: 10.1038/s41598-018-21921-w
Saleem, M., Fariduddin, Q., Janda, T. (2021a). Multifaceted role of salicylic acid in combating cold stress in plants: A review. J. Plant Growth Regul. 40, 464–485. doi: 10.1007/s00344-020-10152-x
Saleem, S., Iqbal, A., Ahmed, F., Ahmad, M. (2021b). Phytobeneficial and salt stress mitigating efficacy of IAA producing salt tolerant strains in. Gossypium hirsutum. Saudi J. Biol. Sci. 28, 5317–5324. doi: 10.1016/j.sjbs.2021.05.056
Saltveit, M. E. (2000). Wound induced changes in phenolic metabolism and tissue browning are altered by heat shock. Postharvest Biol. Technol. 21, 61–69. doi: 10.1016/S0925-5214(00)00165-4
Saravanakumar, D., Kavino, M., Raguchander, T., Subbian, P., Samiyappan, R. (2011). Plant growth promoting bacteria enhance water stress resistance in green gram plants. Acta Physiol. Plant 33, 203–209. doi: 10.1007/s11738-010-0539-1
Sarkar, J., Chakraborty, U., Chakraborty, B. (2021). High-temperature resilience in Bacillus safensis primed wheat plants: A study of dynamic response associated with modulation of antioxidant machinery, differential expression of HSPs and osmolyte biosynthesis. Environ. Exp. Bot. 182, 104315. doi: 10.1016/j.envexpbot.2020.104315
Sarkar, A., Ghosh, P. K., Pramanik, K., Mitra, S., Soren, T., Pandey, S., et al. (2018). A halotolerant enterobacter sp. displaying ACC deaminase activity promotes rice seedling growth under salt stress. Res. Microbiol. 169, 20–32. doi: 10.1016/j.resmic.2017.08.005
Sarma, R. K., Saikia, R. (2014). Alleviation of drought stress in mung bean by strain Pseudomonas aeruginosa GGRJ21. Plant Soil 377, 111–126. doi: 10.1007/s11104-013-1981-9
Sen, S., Ghosh, D., Mohapatra, S. (2018). Modulation of polyamine biosynthesis in Arabidopsis thaliana by a drought mitigating Pseudomonas putida strain. Plant Physiol. Biochem. 129, 180–188. doi: 10.1016/j.plaphy.2018.05.034
Shabaan, M., Asghar, H. N., Zahir, Z. A., Zhang, X., Sardar, M. F., Li, H. (2022). Salt-tolerant PGPR confer salt tolerance to maize through enhanced soil biological health, enzymatic activities, nutrient uptake and antioxidant defense. Front. Microbiol. 13. doi: 10.3389/fmicb.2022.901865
Shaffique, S., Khan, M. A., Wani, S. H., Pande, A., Imran, M., Kang, S. M., et al. (2022). A review on the role of endophytes and plant growth promoting rhizobacteria in mitigating heat stress in plants. Microorganisms 10, 1286. doi: 10.3390/microorganisms10071286
Shahid, M., Akram, M. S., Khan, M. A., Zubair, M., Shah, S. M., Ismail, M., et al. (2018). A phytobeneficial strain planomicrobium sp. MSSA-10 triggered oxidative stress responsive mechanisms and regulated the growth of pea plants under induced saline environment. J. Appl. Microbiol. 124, 1566–1579. doi: 10.1111/jam.13732
Shahid, M., Ameen, F., Maheshwari, H. S., Ahmed, B., AlNadhari, S., Khan, M. S. (2021). Colonization of Vigna radiata by a halotolerant bacterium Kosakonia sacchari improves the ionic balance, stressor metabolites, antioxidant status and yield under NaCl stress. Appl. Soil Ecol. 158, 103809. doi: 10.1016/j.apsoil.2020.103809
Shahrajabian, M. H., Chaski, C., Polyzos, N., Petropoulos, S. A. (2021). Biostimulants application: A low input cropping management tool for sustainable farming of vegetables. Biomolecules 11, 698. doi: 10.3390/biom11050698
Sivamani, E., Bahieldin, A., Wraith, J. M., Al-Niemi, T., Dyer, W. E., Ho, T. H. D., et al. (2000). Improved biomass productivity and water use efficiency under water deficit conditions in transgenic wheat constitutively expressing the barley HVA1 gene. Plant Sci. 155, 1–9. doi: 10.1016/s0168-9452(99)00247-2
Srivastava, S., Bist, V., Srivastava, S., Singh, P. C., Trivedi, P. K., Asif, M. H., et al. (2016). Unraveling aspects of Bacillus amyloliquefaciens mediated enhanced production of rice under biotic stress of Rhizoctonia solani. Front. Plant Sci. 7. doi: 10.3389/fpls.2016.00587
Subramanian, P., Kim, K., Krishnamoorthy, R., Mageswari, A., Selvakumar, G., Sa, T. (2016). Cold stress tolerance in psychrotolerant soil bacteria and their conferred chilling resistance in tomato (Solanum lycopersicum mill.) under low temperatures. PLos One 11, e0161592. doi: 10.1371/journal.pone.0161592
Su, F., Jacquard, C., Villaume, S., Michel, J., Rabenoelina, F., Clément, C., et al. (2015). Burkholderia phytofirmans PsJN reduces impact of freezing temperatures on photosynthesis in Arabidopsis thaliana. Front. Plant Sci. 6. doi: 10.3389/fpls.2015.00810
Sunita, K., Mishra, I., Mishra, J., Prakash, J., Arora, N. K. (2020). Secondary metabolites from halotolerant plant growth promoting rhizobacteria for ameliorating salinity stress in plants. Front. Microbiol. 11. doi: 10.3389/fmicb.2020.567768
Swain, P., Raman, A., Singh, S. P., Kumar, A. (2017). Breeding drought tolerant rice for shallow rainfed ecosystem of eastern India. F. Crop Res. 209, 168–178. doi: 10.1016/j.fcr.2017.05.007
Teklić, T., Parađiković, N., Špoljarević, M., Zeljković, S., Lončarić, Z., Lisjak, M. (2021). Linking abiotic stress, plant metabolites, biostimulants and functional food. Ann. Appl. Biol. 178, 169–191. doi: 10.1111/aab.12651
Tewari, S., Arora, N. K. (2018). Role of salicylic acid from Pseudomonas aeruginosa PF23EPS+ in growth promotion of sunflower in saline soils infested with phytopathogen Macrophomina phaseolina. Environ. Sustain. 1, 49–59. doi: 10.1007/s42398-018-0002-6
Theocharis, A., Bordiec, S., Fernandez, O., Paquis, S., Dhondt-Cordelier, S., Baillieul, F., et al. (2012). Burkholderia phytofirmans PsJN primes Vitis vinifera l. and confers a better tolerance to low nonfreezing temperatures. Mol. Plant Microbe Interact. 25, 241–249. doi: 10.1094/MPMI-05-11-0124
Tirry, N., Kouchou, A., Laghmari, G., Lemjereb, M., Hnadi, H., Amrani, K., et al. (2021). Improved salinity tolerance of Medicago sativa and soil enzyme activities by PGPR. Biocatal. Agric. Biotechnol. 31, 101914. doi: 10.1016/j.bcab.2021.101914
Tiryaki, D., Aydın, İ., ATICI, Ö. (2018). Psychrotolerant bacteria isolated from the leaf apoplast of cold-adapted wild plants improve the cold resistance of bean (Phaseolus vulgaris l.) under low temperature. Cryobiology 86, 111–119. doi: 10.1016/j.cryobiol.2018.11.001
Torres, R. O., Henry, A. (2018). Yield stability of selected rice breeding lines and donors across conditions of mild to moderately severe drought stress. F. Crop Res. 220, 37–45. doi: 10.1016/j.fcr.2016.09.011
Turan, M., Güllüce, M., Çakmak, R., Şahin, F. (2013). Effect of plant growth-promoting rhizobacteria strain on freezing injury and antioxidant enzyme activity of wheat and barley. J. Plant Nutr. 36, 731–748. doi: 10.1080/01904167.2012.754038
Vimal, S. R., Patel, V. K., Singh, J. S. (2019). Plant growth promoting Curtobacterium albidum strain SRV4: An agriculturally important microbe to alleviate salinity stress in paddy plants. Ecol. Indic. 105, 553–562. doi: 10.1016/j.ecolind.2018.05.014
Vlot, A. C., Sales, J. H., Lenk, M., Bauer, K., Brambilla, A., Sommer, A., et al. (2021). Systemic propagation of immunity in plants. New Phytol. 229, 1234–1250. doi: 10.1111/nph.16953
Vocciante, M., Grifoni, M., Fusini, D., Petruzzelli, G., Franchi, E. (2022). The role of plant growth-promoting rhizobacteria (PGPR) in mitigating plant’s environmental stresses. Appl. Sci. 12, 1231. doi: 10.3390/app12031231
Wang, G., Xu, X., Wang, H., Liu, Q., Yang, X., Liao, L., et al. (2019). A tomato transcription factor, SlDREB3 enhances the tolerance to chilling in transgenic tomato. Plant physiol. Biochem 142, 254–262. doi: 10.1016/j.plaphy.2019.07.017
Wan, L. J., Tian, Y., He, M., Zheng, Y. Q., Lyu, Q., Xie, R. J., et al. (2021). Effects of chemical fertilizer combined with organic fertilizer application on soil properties, citrus growth physiology, and yield. Agriculture 11, 1207. doi: 10.3390/agriculture11121207
Wei, Y., Chen, H., Wang, L., Zhao, Q., Wang, D., Zhang, T. (2022). Cold acclimation alleviates cold stress-induced PSII inhibition and oxidative damage in tobacco leaves. Plant Signal. Behav. 17, 2013638. doi: 10.1080/15592324.2021.2013638
Win, K. T., Tanaka, F., Okazaki, K., Ohwaki, Y. (2018). The ACC deaminase expressing endophyte Pseudomonas spp. enhances NaCl stress tolerance by reducing stress-related ethylene production, resulting in improved growth, photosynthetic performance, and ionic balance in tomato plants. Plant Physiol. Biochem. 127, 599–607. doi: 10.1016/j.plaphy.2018.04.038
Woo, O. G., Kim, H., Kim, J. S., Keum, H. L., Lee, K. C., Sul, W. J., et al. (2020). Bacillus subtilis strain GOT9 confers enhanced tolerance to drought and salt stresses in Arabidopsis thaliana and brassica campestris. Plant Physiol. Biochem. 148, 359–367. doi: 10.1016/j.plaphy.2020.01.032
Wu, Y., Zhou, J., Li, C., Ma, Y. (2019). Antifungal and plant growth promotion activity of volatile organic compounds produced by Bacillus amyloliquefaciens. MicrobiologyOpen 8, e00813. doi: 10.1002/mbo3.813
Yasmin, H., Bano, A., Wilson, N. L., Nosheen, A., Naz, R., Hassan, M. N., et al. (2022). Drought-tolerant pseudomonas sp. showed differential expression of stress-responsive genes and induced drought tolerance in Arabidopsis thaliana. Physiol. Plant 174, e13497. doi: 10.1111/ppl.13497
Yasmin, H., Mazher, J., Azmat, A., Nosheen, A., Naz, R., Hassan, M. N., et al. (2021b). Combined application of zinc oxide nanoparticles and biofertilizer to induce salt resistance in safflower by regulating ion homeostasis and antioxidant defence responses. Ecotoxicol Environ. Saf. 218, 112262. doi: 10.1016/j.ecoenv.2021.112262
Yasmin, H., Naeem, S., Bakhtawar, M., Jabeen, Z., Nosheen, A., Naz, R., et al. (2020). Halotolerant rhizobacteria Pseudomonas pseudoalcaligenes and Bacillus subtilis mediate systemic tolerance in hydroponically grown soybean (Glycine max l.) against salinity stress. PLos One 15, e0231348. doi: 10.1371/journal.pone.0231348
Yasmin, H., Rashid, U., Hassan, M. N., Nosheen, A., Naz, R., Ilyas, N., et al. (2021a). Volatile organic compounds produced by Pseudomonas pseudoalcaligenes alleviated drought stress by modulating defense system in maize (Zea mays l.). Physiol. Plant 172, 896–911. doi: 10.1111/ppl.13304
Yu, Y., Gui, Y., Li, Z., Jiang, C., Guo, J., Niu, D. (2022). Induced systemic resistance for improving plant immunity by beneficial microbes. Plants 11, 386. doi: 10.3390/plants11030386
Yu, T. F., Xu, Z. S., Guo, J. K., Wang, Y. X., Abernathy, B., Fu, J. D., et al. (2017). Improved drought tolerance in wheat plants overexpressing a synthetic bacterial cold shock protein gene SeCspA. Sci. Rep. 7, 44050. doi: 10.1038/srep44050
Zandalinas, S. I., Mittler, R., Balfagón, D., Arbona, V., Gómez-Cadenas, A. (2018). Plant adaptations to the combination of drought and high temperatures. Physiol. Plant 162, 2–12. doi: 10.1111/ppl.12540
Zang, X., Xiaoli, G., Wang, F., Liu, Z., Zhang, L., Zhao, Y., et al. (2017). Overexpression of wheat ferritin gene TaFER-5B enhances tolerance to heat stress and other abiotic stresses associated with the ROS scavenging. BMC Plant Biol. 17, 1–13. doi: 10.1186/s12870-016-0958-2
Zarei, T., Moradi, A., Kazemeini, S. A., Akhgar, A., Rahi, A. A. (2020). The role of ACC deaminase producing bacteria in improving sweet corn (Zea mays l. var saccharata) productivity under limited availability of irrigation water. Sci. Rep. 10, 1–12. doi: 10.1038/s41598-020-77305-6
Zebelo, S., Song, Y., Kloepper, J. W., Fadamiro, H. (2016). Rhizobacteria activates (+)-δ-cadinene synthase genes and induces systemic resistance in cotton against beet armyworm (Spodoptera exigua). Plant Cell Environ. 39, 935–943. doi: 10.1111/pce.12704
Zhou, Y., Sommer, M. L., Hochholdinger, F. (2021). Cold response and tolerance in cereal roots. J. Exp. Bot. 72, 7474–7481. doi: 10.1093/jxb/erab334
Zubair, M., Hanif, A., Farzand, A., Sheikh, T. M. M., Khan, A. R., Suleman, M., et al. (2019). Genetic screening and expression analysis of psychrophilic bacillus spp. reveal their potential to alleviate cold stress and modulate phytohormones in wheat. Microorganisms 7, 337. doi: 10.3390/microorganisms7090337
Keywords: PGPR, biostimulant, antioxidants, phytohormones, signaling, stress tolerance, crop productivity, systemic resistance
Citation: Kaushal P, Ali N, Saini S, Pati PK and Pati AM (2023) Physiological and molecular insight of microbial biostimulants for sustainable agriculture. Front. Plant Sci. 14:1041413. doi: 10.3389/fpls.2023.1041413
Received: 10 September 2022; Accepted: 13 January 2023;
Published: 30 January 2023.
Edited by:
Shubhpriya Gupta, Palacký University, Olomouc, CzechiaReviewed by:
Manoj Gajanan Kulkarni, University of KwaZulu-Natal, South AfricaHumaira Yasmin, COMSATS University, Islamabad Campus, Pakistan
Copyright © 2023 Kaushal, Ali, Saini, Pati and Pati. This is an open-access article distributed under the terms of the Creative Commons Attribution License (CC BY). The use, distribution or reproduction in other forums is permitted, provided the original author(s) and the copyright owner(s) are credited and that the original publication in this journal is cited, in accordance with accepted academic practice. No use, distribution or reproduction is permitted which does not comply with these terms.
*Correspondence: Aparna Maitra Pati, aparna@ihbt.res.in