- 1Center of Excellence for Molecular Crop, Department of Biochemistry, Faculty of Science, Chulalongkorn University, Bangkok, Thailand
- 2Faculty of Agriculture Natural Resources and Environment, Naresuan University, Phitsanulok, Thailand
- 3Omics Sciences and Bioinformatics Center, Chulalongkorn University, Bangkok, Thailand
Agriculture has been considered as a fundamental industry for human survival since ancient times. Local and traditional agriculture are based on circular sustainability models, which produce practically no waste. However, owing to population growth and current market demands, modern agriculture is based on linear and large-scale production systems, generating tons of organic agricultural waste (OAW), such as rejected or inedible plant tissues (shells, peels, stalks, etc.). Generally, this waste accumulates in landfills and creates negative environmental impacts. The plant kingdom is rich in metabolic diversity, harboring over 200,000 structurally distinct metabolites that are naturally present in plants. Hence, OAW is considered to be a rich source of bioactive compounds, including phenolic compounds and secondary metabolites that exert a wide range of health benefits. Accordingly, OAW can be used as extraction material for the discovery and recovery of novel functional compounds that can be reinserted into the production system. This approach would alleviate the undesired environmental impacts of OAW accumulation in landfills, while providing added value to food, pharmaceutical, cosmetic, and nutraceutical products and introducing a circular economic model in the modern agricultural industry. In this regard, metabolomics-based approaches have gained increasing interest in the agri-food sector for a variety of applications, including the rediscovery of bioactive compounds, owing to advances in analytical instrumentation and data analytics platforms. This mini review summarizes the major aspects regarding the identification of novel bioactive compounds from agricultural waste, focusing on metabolomics as the main tool.
Introduction
For centuries, the agricultural industry has been vital for providing food and materials to humankind. Traditional and local agriculture utilize available plants and resources (water, soil, land, etc.) in a sustainable way, ensuring the subsistence of the local community. Practically no waste is produced in these approaches as waste and unexploited products are utilized further as fertilizers, which are easily absorbed by soils (Harris and Hillman, 2014). In the wine production industry, the generated grape marc would be further utilized in the production of other alcohols, and the final product would be used to fertilize the soil (Nerantzis and Tataridis, 2006). However, population growth and its consequent need to produce large amounts of food, as well as globalization and the pursuit of individual economic benefits have promoted the emergence of a linear-producing modern agricultural system. Unlike traditional and local agriculture, which are based on circular sustainability models, the modern system aims to meet global demand by increasing the profitability of production. To achieve this aim, modern agriculture overexploits natural resources by using the soil extensively along with large amounts of water and energy, applying pesticides to eliminate insects, and choosing monocultures over mixed production (Rockström et al., 2017; Ramankutty et al., 2018; Duque-Acevedo et al., 2020). The world population is predicted to reach ~10 billion by 2050 (data from Department of Economic and Social Affairs, United Nations (UN), n.d., Food and Agriculture Organization (FAO), n.d.). Accordingly, agricultural production also needs to grow, albeit in a sustainable way (Ramankutty et al., 2018). However, a major drawback of this modern system is the increased production of organic agricultural waste (OAW) from crops (Gustavsson et al., 2011), including rejected or inedible plant tissues such as pruning, fruit trimming, shelling or forestall residues, and food processing wastes such as rice husk and wheat straw. Notably, fruit pulp is also considered a major OAW because fruit juice production generates tons of squeezed pulp. In addition, huge quantities of fruit pulp may be rejected owing to post-harvest loss, as is commonly seen for climacteric fruits that possess a strikingly limited shelf life after harvesting. Generally, OAWs that are not further utilized accumulate uncontrollably in landfills. If poorly managed, the accumulated OAW would generate various biotic and abiotic by-products that would negatively impact the environment, health, and economy (El-Haggar, 2007; Nagendran, 2011; He et al., 2019). Moreover, such landfills generate considerable amounts of methane, nitrous oxide, sulfur dioxide, and smoke when the OAW is burned in open air, a practice which is common in many landfills and leads to atmospheric pollution with significant emissions of carbon dioxide (Wang et al., 2019).
Toward a Sustainable Bioeconomy Using Organic Agricultural Waste
The question arises as to why OAW is not reutilized. Different reutilization approaches for these residues have been described, including as animal feed and subjection to anaerobic digestion and composting. Although the effectiveness of these strategies has been described by several studies, such as those demonstrating the beneficial effects of using OAW as fertilizer (Sud et al., 2008; Meng et al., 2017), OAWs continue to accumulate. The reason for this is profitability. For instance, farmers are not willing to risk replacing synthetic fertilizers, which deliver a precise quantity of nutrients, with OAW, which provides an imprecise quantity (Innes, 2013). Moreover, these strategies do not typically generate significant economic value (Garcia-Garcia et al., 2019). Therefore, a shift toward a more sustainable approach is vital. Over the last decades, the development of novel value-added products based on the exploitation of bioactive compounds from OAW has gained considerable interest, which makes OAW a suitable feedstock for valorization. The plant kingdom is extensively rich in metabolic diversity, harboring over 200,000 structurally distinct metabolites (Wurtzel and Kutchan, 2016) that are naturally present in plants, especially under stress and/or damage conditions, which also magnifies their presence in OAWs. Hence, OAW is a rich source of bioactive compounds, including phenolic compounds (PCs) and secondary metabolites, which exert a wide range of health benefits such as antioxidant, anti-cancer, anti-inflammatory, cardioprotective, anti-microbial, and anti-allergenic activities (Coman et al., 2020; Jimenez-Lopez et al., 2020). PCs are a large group of secondary metabolites generated by plants in response to multiple environmental stimuli. Owing to the numerous health-beneficial properties associated with PCs and their abundance in OAW, PCs from OAW are increasingly attracting industrial interest. Moreover, since some of these compounds are difficult and/or expensive to synthesize, their availability from OAW makes chemical synthesis unnecessary (Burri et al., 2017; Jimenez-Lopez et al., 2020).
The importance of converting OAW into value-added products has been incorporated into various market sectors. According to a recent study, the market value of agricultural waste products peaked at USD 63.3 billion in the beverage industry, followed by USD 48 billion in the medical industry and approximately USD 46 billion in the food and consumer goods sector (Beltrán-Ramírez et al., 2019). Taken together, the valorization of OAW generates a significant economic value by increasing income per harvest and improving the livelihood of the local communities, while reducing the excessive costs for waste disposal and minimizing the carbon footprint (Lucarini et al., 2018; Singh et al., 2019).
Shift Toward Sustainable Agriculture: Valorization of OAW as a Potential Source of Bioactive Compounds
Moving toward a systemic, circular model of “reuse, recycle, and regenerate” is vital for developing a sustainable agricultural industry. In this context, OAW biomass should be considered a sustainable resource rather than a waste product. OAW valorization is based on the concept that any residual material or by-product can be used as an extraction material, and the recovered bioactive compounds be reinserted into the production chain. The reutilization of these functional compounds not only represents various potential applications, including in the preparation of functional foods, food and feed additives, and nutraceutical and cosmeceutical products, but also alleviates certain negative effects of OAW accumulation in landfills, thus representing a favorable measure for the environment.
The extraction of these functional bioactive compounds is an important aspect of OAW valorization both in the context of economic benefit, owing to the recovery of valuable compounds, and in the context of waste detoxification, owing to the removal of some compounds which could be undesirable in subsequent biological post-treatments (Serrano et al., 2017; Negro et al., 2018). Over the last few decades, researchers have focused on optimizing the extraction processes. Different parameters have been investigated to optimize the extraction yields of bioactive compounds available in OAW (Kareem and Rahman, 2013; Dorta et al., 2014; Wong et al., 2014). Table 1 summarizes the different OAWs from various crops, the amounts generated in Asia (tons/year) as of 2020, the bioactive compounds extracted from them, and the main analytical platforms utilized according to the literature published in the last 5 years (2017 until now).
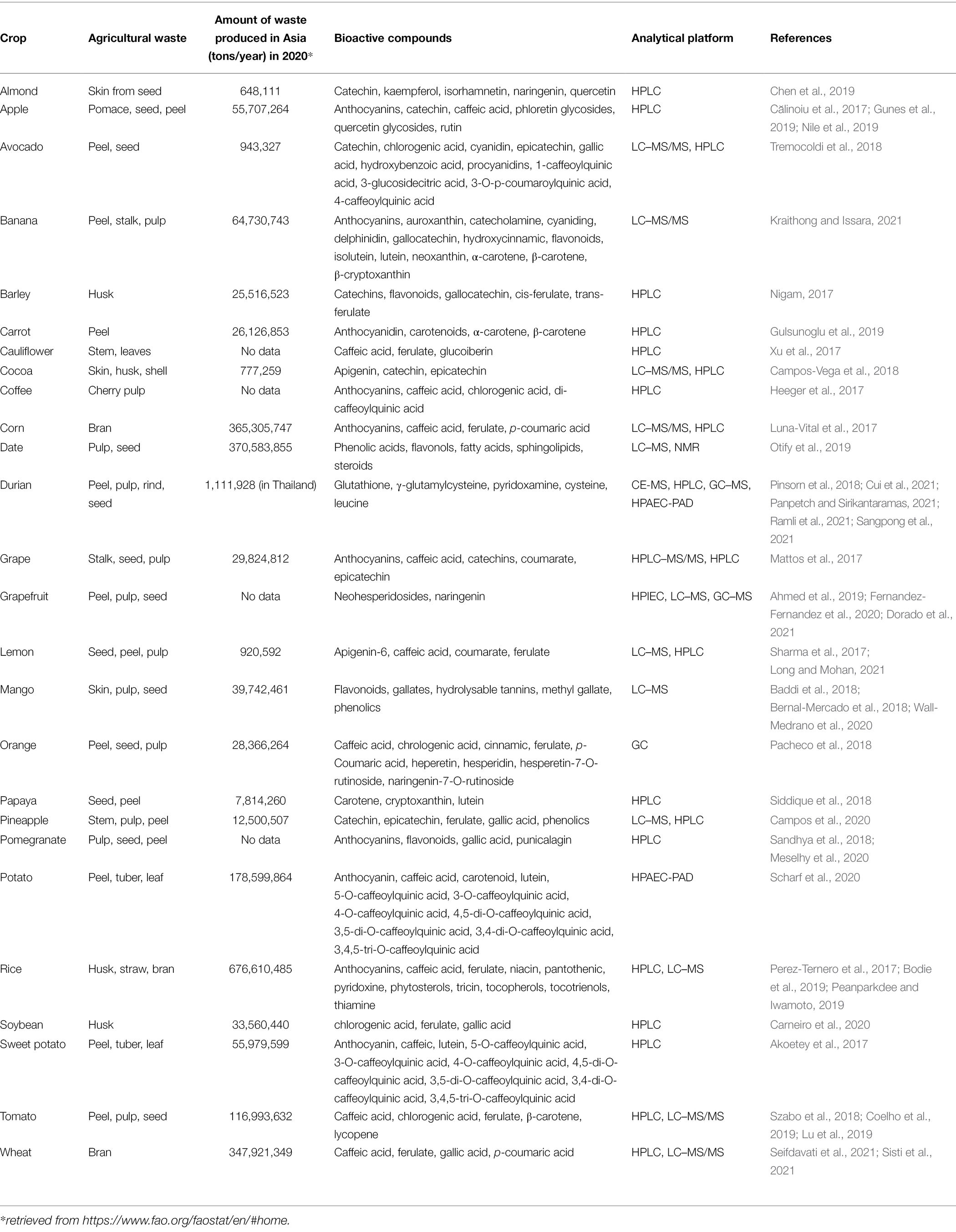
Table 1. List of organic agricultural wastes (OAWs) from various crops, the major bioactive compounds identified and/or extracted from them, main analytical platform utilized, and recent research studies (2017–present) in this area.
Metabolomics in Agri-Food Sector: Current Practices for Valorization of OAW
Metabolomics is the comprehensive characterization of small molecules or metabolites present in a biological sample. Owing to the development of chemometrics and advanced analytical platforms, metabolomics has deepened our understanding of various metabolomic and pathway networks (Hollywood et al., 2006). Numerous high-throughput analytical platforms, including liquid chromatography and gas chromatography–mass spectrometry (LC–MS and GC–MS), and nuclear magnetic resonance (NMR) spectroscopy, have been extensively utilized for this purpose (Johanningsmeier et al., 2016). Metabolomics studies use untargeted or targeted approaches, and the selection of the analytical approach depends mainly on the research question and expected outcomes. Targeted analyses focus on a class of metabolites of interest based on our pre-existing knowledge. However, untargeted analyses utilize unbiased metabolite fingerprinting to profile the global metabolome of diverse chemical classes of metabolites associated with various known and/or unknown pathways (Scalbert et al., 2009; Patti et al., 2012). As shown in Table 1, high-performance liquid chromatography (HPLC) has been extensively utilized as the main analytical platform for the identification and/or discovery of various bioactive compounds from OAW using a targeted approach. However, over the past few years, a combination of both targeted and untargeted approaches (HPLC coupled with LC–MS and/or GC–MS) has been utilized to obtain a complete profile of the metabolites present in OAWs (as seen in Table 1).
Over the past few decades, metabolomics has been extensively applied to the valorization of different OAWs from various crops. Owing to recent advances in analytical instrumentation and data analytics platforms (Putri et al., 2013; Rubert et al., 2015), metabolomics-based approaches have gained significant interest in the agri-food sector for the identification and/or rediscovery of diverse high-value bioactive compounds, especially PCs, from OAWs. Over 10,000 different PC structures with diverse natures are currently known, the most well-known of which include phenolic acids, flavonoids, and tannins (Kennedy and Wightman, 2011). These exist naturally in various concentrations in different plant parts, from roots to shoots, as well as in fruits. Accordingly, they are also present in OAWs. Recently, numerous studies have focused on the research and development of natural compounds as substitutes for synthetic additives because synthetic substances are strongly associated with various health risks, such as the appearance of allergies or even carcinogenesis (Zheng and Wang, 2001). In this context, OAWs are considered as suitable source materials for the extraction of numerous natural bioactive compounds, such as PCs, and metabolomics can be considered as an ideal approach for the identification and/or rediscovery of these compounds from OAWs.
Numerous value-added products have been generated in the food, pharmaceutical, cosmetic, and nutraceutical industries by reinserting these high-value compounds into the production chain, as in a circular economic model. Typical examples include thickening, gelling, and food stabilizing agents from tomato and citrus waste (John et al., 2017; Morales-Contreras et al., 2017); food preservatives (meat and oil product preservatives), food stabilizers, and bactericidal agents from potato peel (Sampaio et al., 2020); essential oils with anti-cancer agents from orange waste (Yang et al., 2017); biobutanol from rice husk (Quispe et al., 2017); hydrogel from durian rind (Cui et al., 2021); and single-cell protein (SCP) from corn stover and orange peel (Diwan et al., 2018).
These value-added products, produced through OAW valorization, generate significant economic value. However, further research and development is vital to fully convert the still-evolving valorization process into a sustainable approach. By integrating metabolomics into this process, we can gain a deeper understanding of the metabolic profiles of OAWs, and this can further promote the valorization process and add greater value to such products. To the best of our knowledge, only a few studies have utilized metabolomics to fully profile the metabolome of OAW and enhance the value of such products. These include studies on the production of pineapple wine and vinegar from pineapple peel and pulp (Roda et al., 2017), essential oils from the aerial parts of plants belonging to the genus Lavandula, mainly L. angustifolia (LA) and L. × intermedia (LI; Truzzi et al., 2022), supplements with therapeutic applications from Passiflora mollissima seeds (Ballesteros-Vivas et al., 2020), and functional foods and nutraceuticals from bean (Vicia faba L.) by-products (Abu-Reidah et al., 2017).
Taken together, the recovery of functional bioactive compounds can be achieved by obtaining products that can be reinserted into the economy as new raw materials within a circular and sustainable bioeconomy. Figure 1 presents a schematic overview of the application of metabolomics in OAW valorization using a circular economy concept. OAW was considered as the input material for the valorization process in this overview. To gain a deeper understanding of the metabolic profile of OAW, we can take advantage of metabolomics to identify and/or rediscover bioactive compounds that can then be reinserted into the production chain to generate value-added products. Notably, for non-edible wastes such as peels and seeds, more studies, including toxicity tests and/or animal model studies, are needed to ensure their safety.
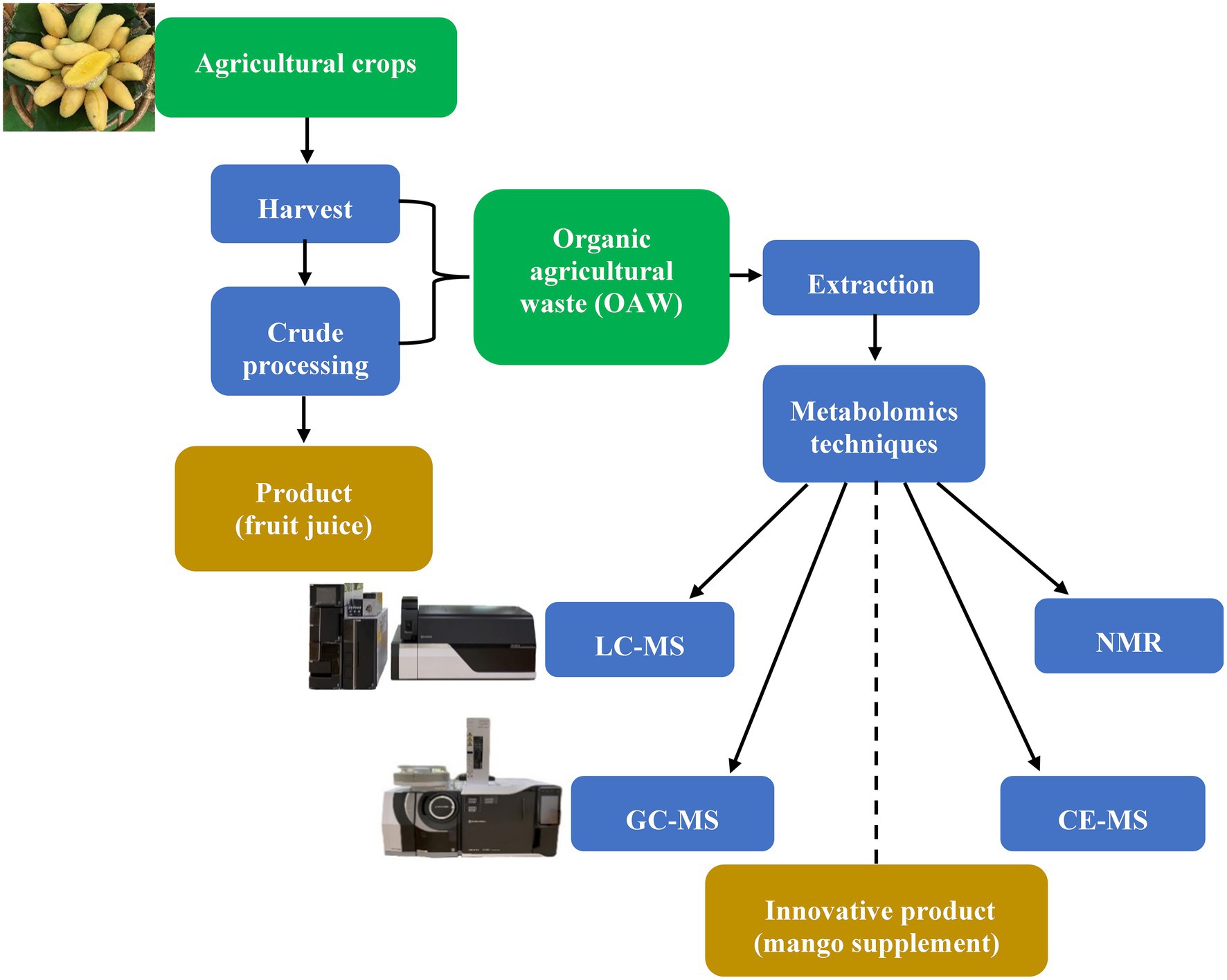
Figure 1. A schematic overview of the application of metabolomics in OAW valorization in a circular economy concept. The dotted line represents the multiple steps that might be needed to generate the final product.
Conclusion and Future Perspective
Owing to population growth and current market demands, modern agricultural systems are linear in nature and generate millions of tons of OAW. These wastes accumulate in landfills and create adverse environmental impacts. Since OAWs are rich in bioactive compounds, including secondary metabolites and PCs, which have various health benefits, their valorization will provide us with numerous exploitable economic, environmental, and social opportunities. To develop a circular and sustainable bioeconomy, OAW can be used as an extraction material, and the recovered bioactive compounds can be reinserted into the production chain. In this regard, metabolomics-based approaches have gained increasing interest in the agri-food sector for the identification and/or rediscovery of these bioactive compounds. Additionally, OAW valorization can be used as a powerful and effective approach for tackling current global issues, including food shortages, waste disposal, and landfill reserves. However, further investigation is still vital to optimize extraction techniques to obtain increased product yields in an eco-friendly and economical manner. Moreover, further developments are required to fully integrate the currently evolving valorization system into a sustainable and efficient industrial tool. In this context, metabolomics can be utilized as a powerful tool to obtain a complete metabolic profile of OAWs. An important risk factor in this process could be the presence of contamination from chemicals in the crop residues owing to excessive use of pesticides and synthetic fertilizers, which should be taken into consideration. There is a dilemma among gardeners and/or orchard owners whether to use pesticides and chemical fertilizers as much as needed to avoid any yield loss and gain profit from higher quality products, or to minimize or, if possible, avoid the use of pesticides and provide the valorization industry with non-contaminated OAWs and gain profit from the OAW valorization process. For the latter to occur, we need to increase public awareness regarding the importance and need to shift toward a circular and sustainable bioeconomy in which OAW is considered a natural resource for the valorization process. In addition, more companies should dedicate themselves to the valorization of OAW and production of value-added products. Taken together, it can be concluded that, although metabolomics can be used as an effective tool to improve the valorization potential of OAWs, the question as to which approach to follow remains open-ended.
Author Contributions
SS: conceptualization and supervision. GK and SS: writing—original draft preparation. GK, MS, NS, and SS: writing—review and editing. All authors contributed to the article and approved the submitted version.
Funding
Research in our laboratory was supported by Chulalongkorn University Fund (GRU 6407023008-1).
Conflict of Interest
The authors declare that the research was conducted in the absence of any commercial or financial relationships that could be construed as a potential conflict of interest.
Publisher’s Note
All claims expressed in this article are solely those of the authors and do not necessarily represent those of their affiliated organizations, or those of the publisher, the editors and the reviewers. Any product that may be evaluated in this article, or claim that may be made by its manufacturer, is not guaranteed or endorsed by the publisher.
Acknowledgments
GK is grateful to the Graduate School, Chulalongkorn University, for supporting his Postdoctoral Fellowship from the Ratchadapisek Somphot Fund. We also thank Center of Excellence for Molecular Crop for partly supporting this work.
References
Abu-Reidah, I. M., Arráez-Román, D., Warad, I., Fernández-Gutiérrez, A., and Segura-Carretero, A. (2017). UHPLC/MS2-based approach for the comprehensive metabolite profiling of bean (Vicia faba L.) by-products: A promising source of bioactive constituents. Food Res. Int. 93, 87–96. doi: 10.1016/j.gecco.2020.e00902
Ahmed, S., Rattanpal, H. S., Gul, K., Dar, R. A., and Sharma, A. (2019). Chemical composition, antioxidant activity and GC-MS analysis of juice and peel oil of grapefruit varieties cultivated in India. J. Integr. Agric. 18, 1634–1642. doi: 10.1016/S2095-3119(19)62602-X
Akoetey, W., Britain, M. M., and Morawicki, R. O. (2017). Potential use of byproducts from cultivation and processing of sweet potatoes. Cienc. Rural 47:e20160610. doi: 10.1590/0103-8478cr20160610
Baddi, J., Vijayalakshmi, D., and Kapale, M. (2018). Extraction of total polyphenols and dietary fiber from mango peel - as potential sources of natural phytonutrients. Int. J. Curr. Microbiol. App. Sci. 7, 1196–1205. doi: 10.20546/ijcmas.2018.705.146
Ballesteros-Vivas, D., Alvarez-Rivera, G., León, C., Morantes, S. J., Ibánez, E., Parada-Alfonso, F., et al. (2020). Foodomics evaluation of the anti-proliferative potential of Passiflora mollissima seeds. Food Res. Int. 130:108938. doi: 10.1016/j.foodres.2019.108938
Beltrán-Ramírez, F., Orona-Tamayo, D., Cornejo-Corona, I., González-Cervantes, J. L. N., de Jesús Esparza-Claudio, J., and Quintana-Rodríguez, E. (2019). “Agro-industrial waste revalorization: the growing biorefinery,” in Biomass for Bioenergy-Recent Trends and Future Challenges. ed. A. E.-F. Abomohra (London, UK: IntechOpen), 1–20.
Bernal-Mercado, A. T., Acevedo-Hernandez, C., Silva-Espinoza, B. A., Cruz-Valenzuela, M. R., Gonzalez-Aguilar, G. A., Nazzaro, F., et al. (2018). Antioxidant and antimicrobial capacity of phenolic compounds of mango (Mangifera indica L.) seed depending upon the extraction process. J. Med. Plants Prod. 7, 209–219. doi: 10.22092/jmpb.2018.118149
Bodie, A. R., Micciche, A. C., Atungulu, G. G., Rothrock, M. J. Jr., and Ricke, S. C. (2019). Current trends of rice milling byproducts for agricultural applications and alternative food production systems. Front. Sustain. Food Syst. 3:47. doi: 10.3389/fsufs.2019.00047
Burri, S. C. M., Ekholm, A., Håkansson, Å., Tornberg, E., and Rumpunen, K. (2017). Antioxidant capacity and major phenol compounds of horticultural plant materials not usually used. J. Funct. Foods 38, 119–127. doi: 10.1016/j.jff.2017.09.003
Călinoiu, L. F., Mitrea, L., Precup, G., Bindea, M., Rusu, B., Dulf, F. V., et al. (2017). Characterization of grape and apple peel wastes’ bioactive compounds and their increased bioavailability after exposure to thermal process. Bull. Univ. Agric. Sci. Veter-Med. Cluj-Napoca. Food Sci. Technol. 74:80. doi: 10.15835/buasvmcn-fst:0028
Campos, D. A., Ribeiro, T. B., Teixeira, J. A., Pastrana, L., and Pintado, M. M. (2020). Integral valorization of pineapple (Ananas comosus L.) by-products through a green chemistry approach towards added value ingredients. Foods. 9:60. doi: 10.3390/foods9010060
Campos-Vega, R., Nieto-Figueroa, K. H., and Oomah, B. D. (2018). Cocoa (Theobroma Cacao L.) pod husk: renewable source bioactive compounds. Trends Food Sci. Technol. 81, 172–184. doi: 10.1016/j.tifs.2018.09.022
Carneiro, A. M., Moreira, E. A., Bragagnolo, F. S., Borges, M. S., Pilon, A. C., Rinaldo, D., et al. (2020). Soya agricultural waste as a rich source of isoflavones. Food Res. Int. 130:108949. doi: 10.1016/j.foodres.2019.108949
Chen, C. Y. O., Milbury, P. E., and Blumberg, J. B. (2019). Polyphenols in almond skins after blanching modulate plasma biomarkers of oxidative stress in healthy humans. Antioxidants. 8:95. doi: 10.3390/antiox8040095
Coelho, M., Pereira, R., Rodrigues, A. S., Teixeira, J. A., and Pintado, M. E. (2019). Extraction of tomato by-products’ bioactive compounds using ohmic technology. Food Bioprod. Process. 117, 329–339. doi: 10.1016/j.fbp.2019.08.005
Coman, V., Teleky, B. E., Mitrea, L., Martau, G. A., Szabo, K., Calinoiu, L. F., et al. (2020). “Bioactive potential of fruit and vegetable wastes,” in Advances in Food and Nutrition Research. ed. F. Toldra (Cambridge: Academic Press), 157–225.
Cui, X., Lee, J., Ng, K. R., and Chen, W. N. (2021). Food waste durian rind-derived cellulose organohydrogels: toward anti-freezing and antimicrobial wound dressing. ACS Sustain. Chem. Eng. 9, 1304–1312. doi: 10.1021/acssuschemeng.0c07705
Department of Economic and Social Affairs, United Nations (UN) (n.d.) Available at: https://www.un.org/en/desa/world-population-projected-reach-98-billion-2050-and-112-billion-2100 (Accessed 5 May 2022).
Diwan, B., Parkhey, P., and Gupta, P. (2018). From agro-industrial wastes to single cell oils: a step towards prospective biorefinery. Folia Microbiol. 63, 547–568. doi: 10.1007/s12223-018-0602-7
Dorado, C., Cameron, R. G., Manthey, J. A., Bai, J., and Ferguson, K. L. (2021). Analysis and potential value of compounds extracted from star ruby, rio red, and ruby red grapefruit, and grapefruit juice processing residues via steam explosion. Front. Nutr. 8:691663. doi: 10.3389/fnut.2021.691663
Dorta, E., González, M., Lobo, M. G., Sánchez-Moreno, C., and de Ancos, B. (2014). Screening of phenolic compounds in by-products extracts from mangoes (Mangifera indica L.) by HPLC-ESI-QTOF-MS and multivariate analysis for use as food ingredients. Food Res. Int. 57, 51–60. doi: 10.1016/j.foodres.2014.01.012
Duque-Acevedo, M., Belmonte-Ureña, L. J., Cortés-García, F. J., and Camacho-Ferre, F. (2020). Agricultural waste: Review of the evolution, approaches and perspectives on alternative uses. Glob. Ecol. Conserv., 22:e00902.
El-Haggar, S. M. (2007). “Sustainable Development and Environmental Reform,” in Sustainable Industrial Design and Waste Management. eds. S. M. B. T.-S. I. D. and W. M. El-Haggar (Oxford: Elsevier), 125–148.
Fernandez-Fernandez, A. M., Dellacassa, E., Medrano-Fernandez, A., and Del Castillo, M. D. (2020). “Citrus waste recovery for sustainable nutrition and health,” in Food Wastes and By-Products: Nutraceutical and Health Potential. eds. R. Campos-Vega, B. D. Oomah, and H. A. Vergara-Castaneda (Hoboken, New Jersey, U.S.: Blackwell Publishing), 193–222.
Food and Agriculture Organization (FAO) (n.d.). Available at: https://www.fao.org/faostat/en/#home (Accessed 7 February 2022).
Garcia-Garcia, G., Stone, J., and Rahimifard, S. (2019). Opportunities for waste valorisation in the food industry – a case study with four UK food manufacturers. J. Clean. Prod. 211, 1339–1356. doi: 10.1016/j.jclepro.2018.11.269
Gulsunoglu, Z., Karbancioglu-Guler, F., Raes, K., and Kilic-Akyilmaz, M. (2019). Soluble and insoluble-bound phenolics and antioxidant activity of various industrial plant wastes. Int. J. Food Prop. 22, 1501–1510. doi: 10.1080/10942912.2019.1656233
Gunes, R., Palabiyik, I., Toker, O. S., Konar, N., and Kurultay, S. (2019). Incorporation of defatted apple seeds in chewing gum system and phloridzin dissolution kinetics. J. Food Eng. 255, 9–14. doi: 10.1016/j.jfoodeng.2019.03.010
Gustavsson, J., Cederberg, C., Sonesson, U., Van Otterdijk, R., and Meybeck, A. (2011). “Global food losses and food waste.” in Proceedings of the Save Food Congress FAO, 2011. Rome, Italy.
Harris, D. R., and Hillman, G. C. (2014). Foraging and Farming: The Evolution of Plant Exploitation. London, UK: Routledge.
He, K., Zhang, J., and Zeng, Y. (2019). Knowledge domain and emerging trends of agricultural waste management in the field of social science: a scientometric review. Sci. Total Environ. 670, 236–244. doi: 10.1016/j.scitotenv.2019.03.184
Heeger, A., Kosińska-Cagnazzo, A., Cantergiani, E., and Andlauer, W. (2017). Bioactives of coffee cherry pulp and its utilisation for production of cascara beverage. Food Chem. 221, 969–975. doi: 10.1016/j.foodchem.2016.11.067
Hollywood, K., Brison, D. R., and Goodacre, R. (2006). Metabolomics: current technologies and future trends. Proteomics 6, 4716–4723. doi: 10.1002/pmic.200600106
Innes, R. (2013). “Economics of agricultural residuals and overfertilization: chemical fertilizer use, livestock waste, manure management, and environmental impacts,” in Encyclopedia of Energy, Natural Resource, and Environmental Economics. ed. J. F. Shogren (Hoboken, New Jersey, U.S.: Elsevier Inc.), 50–57.
Jimenez-Lopez, C., Fraga-Corral, M., Carpena, M., García-Oliveira, P., Echave, J., Pereira, A. G., et al. (2020). Agriculture waste valorisation as a source of antioxidant phenolic compounds within a circular and sustainable bioeconomy. Food Funct. 11, 4853–4877. doi: 10.1039/D0FO00937G
Johanningsmeier, S. D., Harris, G. K., and Klevorn, C. M. (2016). Metabolomic technologies for improving the quality of food: practice and promise. Annu. Rev. Food Sci. Technol. 7, 413–438. doi: 10.1146/annurev-food-022814-015721
John, I., Muthukumar, K., and Arunagiri, A. (2017). A review on the potential of citrus waste for D-limonene, pectin, and bioethanol production. Int. J. Green Energy, 14, 599–612. doi: 10.1080/15435075.2017.1307753
Kareem, S., and Rahman, R. (2013). Utilization of banana peels for citric acid production by Aspergillus Niger. Agric. Biol. J. North Am. 4, 384–387. doi: 10.5251/abjna.2013.4.4.384.387
Kennedy, D. O., and Wightman, E. L. (2011). Herbal extracts and phytochemicals: plant secondary metabolites and the enhancement of human brain function. Adv. Nutr. 2, 32–50. doi: 10.3945/an.110.000117
Kraithong, S., and Issara, U. (2021). A strategic review on plant by-product from banana harvesting: A potentially bio-based ingredient for approaching novel food and agro-industry sustainability. J. Saudi Soc. Agric. Sci. 20, 530–543. doi: 10.1016/j.jssas.2021.06.004
Long, J. M., and Mohan, A. (2021). Food flavoring prepared with lemon by-product. J. Food Process. Preserv. 45:e15462. doi: 10.1111/jfpp.15462
Lu, Z., Wang, J., Gao, R., Ye, F., and Zhao, G. (2019). Sustainable valorisation of tomato pomace: a comprehensive review. Trends Food Sci. Technol. 86, 172–187. doi: 10.1016/j.tifs.2019.02.020
Lucarini, M., Durazzo, A., Romani, A., Campo, M., Lombardi-Boccia, G., and Cecchini, F. (2018). Bio-based compounds from grape seeds: A biorefinery approach. Molecules 23:1888. doi: 10.3390/molecules23081888
Luna-Vital, D., Li, Q., West, L., West, M., and De Mejia, E. G. (2017). Anthocyanin condensed forms do not affect color or chemical stability of purple corn pericarp extracts stored under different pHs. Food Chem. 232, 639–647. doi: 10.1016/j.foodchem.2017.03.169
Mattos, G. N., Tonon, R. V., Furtado, A. A. L., and Cabral, L. M. C. (2017). Grape by-product extracts against microbial proliferation and lipid oxidation: a review. J. Sci. Food Agric. 97, 1055–1064. doi: 10.1002/jsfa.8062
Meng, F., Dungait, J. A. J., Xu, X., Bol, R., Zhang, X., and Wu, W. (2017). Coupled incorporation of maize (Zea mays L.) straw with nitrogen fertilizer increased soil organic carbon in Fluvic Cambisol. Geoderma 304, 19–27. doi: 10.1016/j.geoderma.2016.09.010
Meselhy, K. M., Shams, M. M., Sherif, N. H., and El-Sonbaty, S. M. (2020). Phytochemical study, potential cytotoxic and antioxidant activities of selected food byproducts (pomegranate peel, Rice bran, Rice straw & mulberry bark). Nat. Prod. Res. 34, 530–533. doi: 10.1080/14786419.2018.1488708
Morales-Contreras, B. E., Contreras-Esquivel, J. C., Wicker, L., Ochoa-Martínez, L. A., and Morales-Castro, J. (2017). Husk Tomato (Physalis ixocarpa Brot.). Waste as a Promising Source of Pectin: Extraction and Physicochemical Characterization. J. Food Sci. 82, 1594–1601. doi: 10.1111/1750-3841.13768
Nagendran, R. (2011). “Agricultural waste and pollution,” in Waste. eds. T. M. Letcher and D. A. Vallero (Amsterdam, The Netherlands: Elsevier, Inc.), 341–355.
Negro, V., Ruggeri, B., and Fino, D. (2018). Recovery of energy from orange peels through anaerobic digestion and pyrolysis processes after d-limonene extraction. Waste Biomass Valorization. 9, 1331–1337. doi: 10.1007/s12649-017-9915-z
Nerantzis, E. T., and Tataridis, P. (2006). Integrated enology-utilization of winery by-products into high added value products. E J. Sci. Technol 1, 79–89.
Nigam, P. S. (2017). An overview: recycling of solid barley waste generated as a by-product in distillery and brewery. Waste Manag. 62, 255–261. doi: 10.1016/j.wasman.2017.02.018
Nile, S. H., Nile, A., Liu, J., Kim, D. H., and Kai, G. (2019). Exploitation of apple pomace towards extraction of triterpenic acids, antioxidant potential, cytotoxic effects, and inhibition of clinically important enzymes. Food Chem. Toxicol. 131:110563. doi: 10.1016/j.fct.2019.110563
Otify, A. M., El-Sayed, A. M., Michel, C. G., and Farag, M. A. (2019). Metabolites profiling of date palm (Phoenix dactylifera L.) commercial by-products (pits and pollen) in relation to its antioxidant effect: a multiplex approach of MS and NMR metabolomics. Metabolomics 15:119. doi: 10.1007/s11306-019-1581-7
Pacheco, M. T., Moreno, F. J., and Villamiel, M. (2018). Chemical and physicochemical characterization of orange by-products derived from industry. J. Sci. Food Agric. 99, 868–876. doi: 10.1002/jsfa.9257
Patti, G. J., Yanes, O., and Siuzdak, G. (2012). Innovation: metabolomics: the apogee of the omics trilogy. Nat. Rev. Mol. Cell Biol. 13, 263–269. doi: 10.1038/nrm3314
Panpetch, P., and Sirikantaramas, S. (2021). Fruit ripening-associated leucylaminopeptidase with cysteinylglycine dipeptidase activity from durian suggests its involvement in glutathione recycling. BMC Plant Biology. 21, 1–14. doi: 10.1186/s12870-021-02845-6
Peanparkdee, M., and Iwamoto, S. (2019). Bioactive compounds from by-products of rice cultivation and rice processing: extraction and application in the food and pharmaceutical industries. Trends Food Sci. Technol. 86, 109–117. doi: 10.1016/j.tifs.2019.02.041
Perez-Ternero, C., De Sotomayor, M. A., and Herrera, M. D. (2017). Contribution of ferulic acid, γ-oryzanol and tocotrienols to the cardiometabolic protective effects of rice bran. J. Funct. Foods 32, 58–71. doi: 10.1016/j.jff.2017.02.014
Pinsorn, P., Oikawa, A., Watanabe, M., Sasaki, R., Ngamchuachit, P., Hoefgen, R., et al. (2018). Metabolic variation in the pulps of two durian cultivars: Unraveling the metabolites that contribute to the flavor. Food Chem. 268, 118–125. doi: 10.1016/j.foodchem.2018.06.066
Putri, S. P., Nakayama, Y., Matsuda, F., Uchikata, T., Kobayashi, S., Matsubara, A., et al. (2013). Current metabolomics: practical applications. J. Biosci. Bioeng. 115, 579–589. doi: 10.1016/j.jbiosc.2012.12.007
Quispe, I., Navia, R., and Kahhat, R. (2017). Energy potential from rice husk through direct combustion and fast pyrolysis: a review. Waste Manag. 59, 200–210. doi: 10.1016/j.wasman.2016.10.001
Ramankutty, N., Mehrabi, Z., Waha, K., Jarvis, L., Kremen, C., Herrero, M., et al. (2018). Trends in global agricultural land use: implications for environmental health and food security. Annu. Rev. Plant Biol. 69, 789–815. doi: 10.1146/annurev-arplant-042817-040256
Ramli, A. N. M., Sukri, B. M., Azelee, N. A. W., and Bhuyar, P. (2021). Exploration of antibacterial and antioxidative activity of seed/peel extracts of south-east Asian fruit durian (Durio zibethinus) for effective shelf-life enhancement of preserved meat. J. Food Process. Preserv. 45:e15662. doi: 10.1111/jfpp.15662
Rockström, J., Williams, J., Daily, G., Noble, A., Matthews, N., Gordon, L., et al. (2017). Sustainable intensification of agriculture for human prosperity and global sustainability. Ambio 46, 4–17. doi: 10.1007/s13280-016-0793-6
Roda, A., Lucini, L., Torchio, F., Dordoni, R., De Faveri, D. M., and Lambri, M. (2017). Metabolite profiling and volatiles of pineapple wine and vinegar obtained from pineapple waste. Food Chem. 229, 734–742. doi: 10.1016/j.foodchem.2017.02.111
Rubert, J., Zachariasova, M., and Hajslova, J. (2015). Advances in high-resolution mass spectrometry based on metabolomics studies for food-a review. Food Addit. Contam. Part A Chem. Anal. Control Expo. Risk Assess. 32, 1685–1708. doi: 10.1080/19440049.2015.1084539
Sandhya, S., Khamrui, K., Prasad, W., Kumar, M., and Kumar, C. M. (2018). Preparation of pomegranate peel extract powder and evaluation of its effect on functional properties and shelf life of curd. LWT. 92, 416–421. doi: 10.1016/j.lwt.2018.02.057
Scalbert, A., Brennan, L., Fiehn, O., Hankemeier, T., Kristal, B. S., and Van Ommen, B. (2009). Mass-spectrometry-based metabolomics: limitations and recommendations for future progress with particular focus on nutrition research. Metabolomics 5, 435–458. doi: 10.1007/s11306-009-0168-0
Sampaio, S. L., Petropoulos, S. A., Alexopoulos, A., Heleno, S. A., Santos-Buelga, C., Barros, L., et al. (2020). Potato peels as sources of functional compounds for the food industry: a review. Trends Food Sci. Technol. 103, 118–129. doi: 10.1016/j.tifs.2020.07.015
Sangpong, L., Khaksar, G., Pinsorn, P., Oikawa, A., Sasaki, R., Erban, A., et al. (2021). Assessing dynamic changes of taste-related primary metabolism during ripening of durian pulp using metabolomic and transcriptomic analyses. Front. Plant Sci. 12:687799. doi: 10.3389/fpls.2021.687799
Scharf, R., Wang, R., Maycock, J., Ho, P., Chen, S., and Orfila, C. (2020). Valorisation of potato (Solanum tuberosum) peel waste: extraction of fibre, monosaccharides, and uronic acids. Waste Biomass Valor. 11, 2123–2128. doi: 10.1007/s12649-018-0532-2
Seifdavati, J., Seifzadeh, S., Ramezani, M., Mashak, R. B., Seyedsharifi, R., Elghandour, M. M. M. Y., et al. (2021). Wastes valorization of wheat straw and wheat bran treated with urea, probiotic or organic acids to enhance ruminal gas production and digestibility of pumpkin by-product. Waste Biomass Valor. 12, 5979–5989. doi: 10.1007/s12649-021-01432-y
Serrano, A., Fermoso, F. G., Alonso-Fariñas, B., Rodríguez-Gutierrez, G., Fernandez-Bolaños, J., and Borja, R. (2017). Olive mill solid waste biorefinery: high-temperature thermal pretreatment for phenol recovery and biomethanization. J. Clean. Prod. 148, 314–323. doi: 10.1016/j.jclepro.2017.01.152
Sharma, K., Mahato, N., Cho, M. H., and Lee, Y. R. (2017). Converting citrus wastes into value-added products: economic and environmently friendly approaches. Nutrition 34, 29–46. doi: 10.1016/j.nut.2016.09.006
Siddique, S., Nawaz, S., Muhammad, F., Akhtar, B., and Aslam, B. (2018). Phytochemical screening and in-vitro evaluation of pharmacological activities of peels of Musa sapientum and Carica papaya fruit. Nat. Prod. Res. 32, 1333–1336. doi: 10.1080/14786419.2017.1342089
Singh, K., Kumar, T., Prince, V. K., Sharma, S., and Rani, J. (2019). A review on conversion of food wastes and by-products into value added products. IJCS 7, 2068–2073.
Sisti, L., Gioia, C., Totaro, G., Verstichel, S., Cartabia, M., Camere, S., et al. (2021). Valorization of wheat bran agro-industrial byproduct as an upgrading filler for mycelium-based composite materials. Ind. Crop. Prod. 170:113742. doi: 10.1016/j.indcrop.2021.113742
Sud, D., Mahajan, G., and Kaur, M. P. (2008). Agricultural waste material as potential adsorbent for sequestering heavy metal ions from aqueous solutions - a review. Bioresour. Technol. 99, 6017–6027. doi: 10.1016/j.biortech.2007.11.064
Szabo, K., Cătoi, A. F., and Vodnar, D. C. (2018). Bioactive compounds extracted from tomato processing by-products as a source of valuable nutrients. Plant Foods Hum. Nutr. 73, 268–277. doi: 10.1007/s11130-018-0691-0
Tremocoldi, M. A., Rosalen, P. L., Franchin, M., Massarioli, A. P., Denny, C., Daiuto, E. R., et al. (2018). Exploration of avocado by-products as natural sources of bioactive compounds. PLoS One 13:e0192577. doi: 10.1371/journal.pone.0192577
Truzzi, E., Chaouch, M. A., Rossi, G., Tagliazucchi, L., Bertelli, D., Benvenuti, S., et al. (2022). Characterization and Valorization of the Agricultural Waste Obtained from Lavandula Steam Distillation for Its Reuse in the Food and Pharmaceutical. Fields. Mol. 27:1613. doi: 10.3390/molecules27051613
Wall-Medrano, A., Olivas-Aguirre, F. J., Ayala-Zavala, J. F., Domínguez-Avila, J. A., Gonzalez Aguilar, G. A., Herrera-Cazares, L. A., et al. (2020). “Health benefits of mango by-products,” in Food Wastes and By-Products: Nutraceutical and Health Potential. eds. R. Campos-Vega, B. D. Oomah, and H. A. Vergara-Castaneda (Hoboken, New Jersey, U.S.: Blackwell Publishing), 159–191.
Wang, W., Akhtar, K., Ren, G., Yang, G., Feng, Y., and Yuan, L. (2019). Impact of straw management on seasonal soil carbon dioxide emissions, soil water content, and temperature in a semi-arid region of China. Sci. Total Environ. 652, 471–482. doi: 10.1016/j.scitotenv.2018.10.207
Wong, Y. S., Sia, C. M., Khoo, H. E., Ang, Y. K., Chang, S. K., and Yim, H. S. (2014). Influence of extraction conditions on antioxidant properties of passion fruit (Passiflora Edulis) peel. Acta. Sci. Pol. Technol. Aliment. 13, 257–265. doi: 10.17306/J.AFS.2014.3.4
Wurtzel, E. T., and Kutchan, T. M. (2016). Plant metabolism, the diverse chemistry set of the future. Science 353, 1232–1236. doi: 10.1126/science.aad2062
Xu, Y., Li, Y., Bao, T., Zheng, X., Chen, W., and Wang, J. (2017). A recyclable protein resource derived from cauliflower by-products: potential biological activities of protein hydrolysates. Food Chem. 221, 114–122. doi: 10.1016/j.foodchem.2016.10.053
Yang, C., Chen, H., Chen, H., Zhong, B., Luo, X., and Chun, J. (2017). Antioxidant and anticancer activities of essential oil from Gannan navel orange peel. Molecules 22:1391. doi: 10.3390/molecules22081391
Keywords: bioactive compound, metabolomics, organic agricultural waste, valorization, value-added product
Citation: Khaksar G, Sirijan M, Suntichaikamolkul N and Sirikantaramas S (2022) Metabolomics for Agricultural Waste Valorization: Shifting Toward a Sustainable Bioeconomy. Front. Plant Sci. 13:938480. doi: 10.3389/fpls.2022.938480
Edited by:
Laigeng Li, Center for Excellence in Molecular Plant Sciences (CAS), ChinaReviewed by:
Rocio Rodriguez Arcos, Institute for Fats (CSIC), SpainCopyright © 2022 Khaksar, Sirijan, Suntichaikamolkul and Sirikantaramas. This is an open-access article distributed under the terms of the Creative Commons Attribution License (CC BY). The use, distribution or reproduction in other forums is permitted, provided the original author(s) and the copyright owner(s) are credited and that the original publication in this journal is cited, in accordance with accepted academic practice. No use, distribution or reproduction is permitted which does not comply with these terms.
*Correspondence: Supaart Sirikantaramas, supaart.s@chula.ac.th